- 1Institute of Bio- and Geosciences, IBG-2: Plant Sciences, Forschungszentrum Jülich GmbH, Jülich, Germany
- 2Institute of Ecology, Leuphana University Lüneburg, Lüneburg, Germany
Improving fertility of marginal soils for the sustainable production of biomass is a strategy for reducing land use conflicts between food and energy crops. Digestates can be used as fertilizer and for soil amelioration. In order to promote plant growth and reduce potential adverse effects on roots because of broadcast digestate fertilization, we propose to apply local digestate depots placed into the rhizosphere. We grew Sida hermaphrodita in large mesocosms outdoors for three growing seasons and in rhizotrons in the greenhouse for 3 months both filled with marginal substrate, including multiple sampling dates. We compared digestate broadcast application with digestate depot fertilization and a mineral fertilizer control. We show that depot fertilization promotes a deep reaching root system of S. hermaphrodita seedlings followed by the formation of a dense root cluster around the depot-fertilized zone, resulting in a fivefold increased biomass yield. Temporal adverse effects on root growth were linked to high initial concentrations of ammonium and nitrite in the rhizosphere in either fertilizer application, followed by a high biomass increase after its microbial conversion to nitrate. We conclude that digestate depot fertilization can contribute to an improved cultivation of perennial energy-crops on marginal soils.
Introduction
Biomass is the primary source of energy in Europe’s renewable energy mix, contributing 67% to the total (Eurostat, 2017). However, if biomass (e.g., energy maize) for bioenergy purposes is produced on agricultural soils, it is competing with food crops for the same land resources, resulting in land use conflicts (FAO, 2009; Fritsche et al., 2010). The cultivation of energy crops on marginal soils is currently discussed as a more sustainable alternative, minimizing potential conflicts with food production (Schröder et al., 2008; Graham-Rowe, 2011). A sustainable use of marginal soils to produce biomass for bioenergy purposes requires adapted cropping strategies that give consideration to the specific soil conditions of marginal substrates and the environment with special attention to the biodiversity of plants and animals (Fang et al., 2007; Blanco-Canqui, 2010; Brüll, 2015).
Following the idea of a closed nutrient loop, organic residues such as biogas digestates are applied to the fields as organic fertilizers after the biomass conversion to bioenergy (Arthurson, 2009; Vaneeckhaute et al., 2013) thereby reducing the need for synthetically produced fertilizers (Haraldsen et al., 2011; Walsh et al., 2012). Further, the carbon content of such organic fertilizers can play an important role as soil amendment increasing the soil fertility of marginal substrates in the long-term (Beare et al., 1994; Tiessen et al., 1994; Reeves, 1997; Nabel et al., 2017). Combinations of perennial energy crops with organic fertilization have been discussed as a possible cropping scenario on marginal sites (Blanco-Canqui, 2010; Nabel et al., 2016). Perennial energy crops are of special interest for the cultivation of marginal soils, as their extensive and perennial root system increases their ability to access the limited water and nutrient resources, often characteristic for marginal soils (Voigt et al., 2012).
However, there are still obstacles that hold farmers off from bringing cropping systems based on organic fertilization, perennial plants and the use of marginal soils into agricultural practice. As first obstacle, physical soil properties like the low water-holding capacity of sandy marginal substrates may increase by surface application of organic fertilization such as digestates. On sandy soils, surface application of digestates strongly decreases the wettability of the substrate by increasing the water repellency of the sand via CH-groups, coating the sand grains, resulting in increased surface water runoff (Voelkner et al., 2015a,b). A second obstacle is the fact that digestates contain a high concentration of NH4+ (Möller and Müller, 2012), which can have a negative impact on the establishment of an extensive root system (Forde and Lorenzo, 2001; Nabel et al., 2014). As a third obstacle, sandy substrates with low water holding capacity (WHC) can be very prone to nitrogen leaching as nutrients are rapidly washed out of the rhizosphere (Di and Cameron, 2002; Nabel et al., 2016). Further risks for nitrogen losses are linked to the fact that digestates cannot be plowed into an established stand of perennial energy crops, because that would cause severe damage of the perennial root systems of the crops. Furthermore, the risk of nitrogen losses via gaseous NH3 and N2O emissions is high, irrespective of soil surface or subsurface application (Möller and Stinner, 2009; Rochette et al., 2009). In addition, perennial energy crops need special attention in the year of crop establishment, before they establish their extensive root system and become competitive against weeds (Borkowska et al., 2009). For the above-mentioned reasons broadcast digestate fertilization could counteract a fast and successful establishment of a vigorous and competitive perennial energy crop canopy.
In order to overcome these problems and allow for an efficient and sustainable use of digestates on sandy marginal substrates for plant biomass production, we aim to adapt the digestate fertilization to the Controlled Uptake Long Term Ammonium Nutrition (CULTAN) method proposed by Sommer (2003). According to this method developed for mineral NH4+-rich fertilizers, fertilizers are not applied broadcast but injected locally directly into the rhizosphere (Sommer, 2005). As the plant roots adjust to the heterogeneous distribution of nutrients in the rhizosphere, they can increase the rooting density and share of fine roots in the fertilized zone (Nkebiwe et al., 2016).
In recent years, the method has been adapted to organic fertilizers that are rich in NH4+ like sludge, manure slurries and digestates (Dell et al., 2000; Pote et al., 2011; Bittman et al., 2012). In various studies on agricultural soils planted with conventional agricultural crops or grasslands, a positive impact of a localized application of organic fertilizers on drought (Garwood and Williams, 1967; Ma et al., 2009), nitrate leaching (Baker, 2001), root growth and nutrient use efficiency (Hodge, 2004; Weligama et al., 2008) as well as NH3 volatilization (Nyord et al., 2008) was shown. All these positive effects of localized organic fertilizer placement sum up in increased biomass yields (Cooke, 1954; Jing et al., 2012). For more information about the underlying processes of the methodology we refer to the review by Nkebiwe et al. (2016), who also focused on localized placement of organic fertilizers into the rhizosphere.
The fertilizer placement technology, already well established on conventional agricultural sites, is however, not yet used for the application of digestates to perennial energy crop cultures grown on marginal sandy field sites. Still, the technology of controlled fertilizer placement tackles major problems of digestate fertilization on sandy marginal sites and is therefore of interest for investigation. In this study, we tested digestate fertilization, applied as localized nutrient depots in the rhizosphere of the perennial energy crop Sida hermaphrodita, grown on a sandy marginal substrate. We compared localized to a broadcast digestate fertilization and mineral fertilization using a conventional NPK-fertilizer and an unfertilized control. S. hermaphrodita is a prairie forb species from North America that grows well on sandy or rocky soils with low organic matter content, producing relatively high biomass yields even with low nutrient levels in the soil (Spooner et al., 1985; Borkowska et al., 2009). In order to follow the structural adaptation of the root system of S. hermaphrodita to the different fertilizer applications a rhizotron experiment was performed under controlled greenhouse conditions. To verify our results under conditions that resemble more the field conditions, we also conducted a 3-year outdoor mesocosm experiment where root proliferation of depot-fertilized plants were followed by using mini-rhizotrons.
The study was designed to answer the following research questions and hypotheses:
Question 1: What causes the reduced root growth on S. hermaphrodita seedlings following digestate application as fertilizer on marginal soil and how does it develop over time?
Hypothesis 1: The root growth is affected by the nitrogen turnover of the fertilizer. High concentrations of ammonium and nitrite following the fertilizer application instantly are toxic to plant roots. The mineralization of ammonium to nitrate will allow enhanced root growth at a later stage.
Question 2: What could be an effective measure to apply digestate fertilization and promote root growth at the same time?
Hypothesis 2: The application of localized patches of digestate (digestate depot) into the rhizosphere will promote root growth, as the major part of the rhizosphere remains unaffected by the fertilization and therefore by initial adverse effects on root growth.
Materials and Methods
Study Site and Plant Cultivation
A glasshouse rhizotron study and an outdoor mesocosm experiment were performed at Forschungszentrum Jülich GmbH, IBG-2: Plant Sciences, Germany (50°54′34″N 6°24′47″E). For the glasshouse experiment, 80 Sida hermaphrodita seedlings of BBCH stage 12-13 (Jablonowski et al., 2017) were transplanted in February 2016 to a rhizotron (inner dimensions: height: 75 cm; width: 36 cm; depth: 2.6 cm) filled with a sandy substrate (RBS GmbH, Inden, Germany; particle size: ≤1 mm; pH 6.6, no detectable amounts of N, P, K, and C), accounting for 80 rhizotrons in total. Rhizotrons were irrigated manually three times a week to 60–70% of substrate WHC. The detailed climate data for temperature and exposure to light over the 3 months experimental time are presented in Table 1.
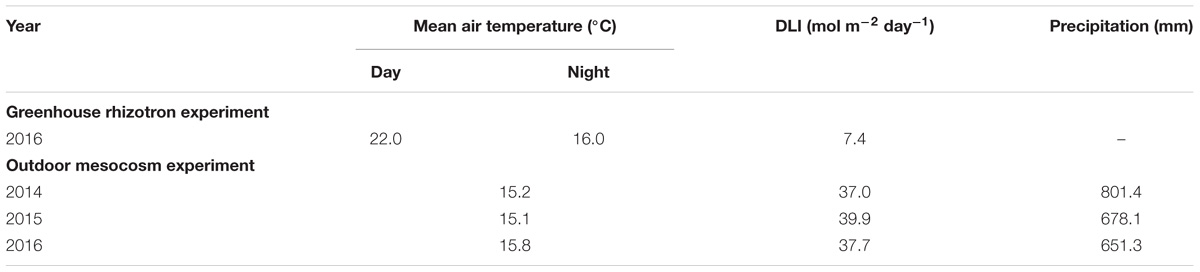
TABLE 1. Climate data for the greenhouse rhizotron experiment and the outdoor mesocosm experiment: mean temperature, precipitation and daily light integral (DLI) values during the experimental time from 2014 to 2016 at the Forschungszentrum Jülich GmbH (50°53′47″ north and 6°25′32″ east; 80 m a.s.l.).
An outdoor mesocosm experiment was conducted using 21 containers, each filled with 250 L of the same sandy substrate as used in the rhizotrons. Big outdoor containers were chosen to keep growing conditions of S. hermaphrodita as close to field conditions as possible (Poorter et al., 2012a, 2016). Seedlings of S. hermaphrodita of BBCH stage 13–14 were transplanted into the mesocosms in May 2014 (Jablonowski et al., 2017). The detailed establishment of S. hermaphrodita plants into the mesocosms was described earlier (Nabel et al., 2016). The climate data over the 3-year experimental time can be found in Table 1.
Treatments and Fertilization
Rhizotrons and mesocosms received digestate fertilization, applied either homogeneously incorporated (here after referred to as “broadcast fertilization”) or as a localized depot (here after referred to as “depot fertilization”) in the rhizosphere, broadcast mineral fertilization or no fertilizer supplement. The digestate was obtained from an operating biogas plant using maize silage as feedstock (digestate dry matter content: 7.2%; Ntotal: 0.53%; NH4+: 0.32%; P: 0.14%; K: 0.68%; Mg 0.037%; Ca: 0.16%; S: 0.03%; organic matter: 5.3%, C:N ratio: 6; pH 8.2; all values referring to fresh weight; ADRW Naturpower GmbH and Co. Kg, Titz-Ameln, Germany). An NPK-fertilizer with an N:P:K-ratio similar to the digestate and a high share of ammonium was chosen to allow a comparison between the mineral and the organic digestate fertilization (NPK-fertilizer composition: N: 15% [1% nitrate; 9.5% ammonium; 4.5% isobutylidenediurea]; P: 5%; K: 8%; Mg: 3%; Compo Rasendünger, Compo GmbH, Münster, Germany). Both fertilizers were calculated to simulate a total N application of 40 t digestate ha−1, which was identified earlier as the optimum dose of digestate fertilization for S. hermaphrodita grown on sandy substrate (Nabel et al., 2014). In mesocosms fertilization treatments were applied in May 2014–2016 to the soil surface and immediately incorporated to minimize possible losses of nitrogen via NH3 (Hayashi et al., 2009). In rhizotrons, broadcast fertilization treatments were applied by homogeneously mixing fertilizers into the top 20 cm of the rhizotrons. For the localized digestate depot fertilization, the same amount of digestate was applied locally as a patch of 4.6 cm diameter. In mesocosms, the digestate depot was injected at a 20 cm distance from the shoot of S. hermaphrodita, at 30 cm depth. In rhizotrons, digestate depots were placed in 15 cm depth and 6 cm distance to the rhizotron wall (Figure 1). In rhizotrons each fertilization treatment was applied with 24 replicates (8 replicates × 3 harvests), the unfertilized control treatment was applied in eight replicates (only one harvest). In mesocosms all treatments were applied in six replicates (one harvest after each growing season).
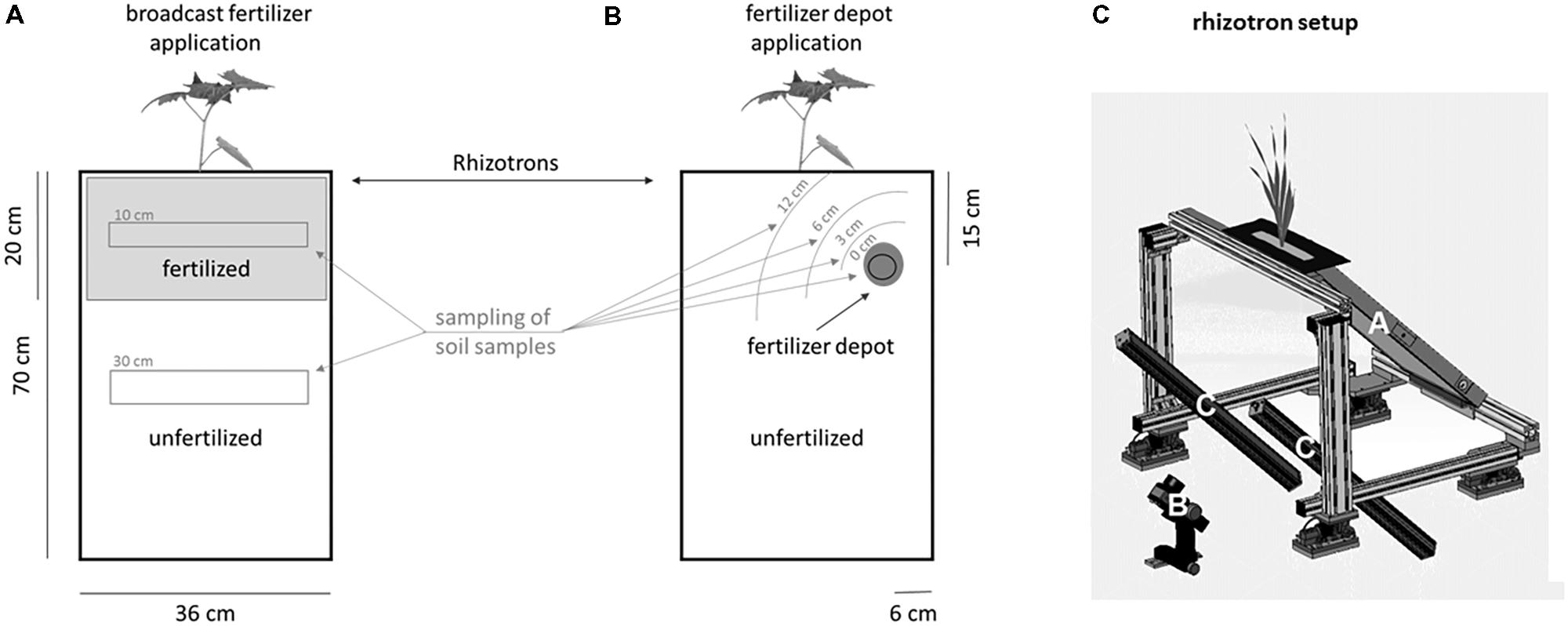
FIGURE 1. Illustration of the fertilizer application as broadcast (A: homogeneous digestate/NPK application) or depot (B: localized digestate application) fertilization in rhizotrons and soil sampling schema. Control rhizotrons did not receive any fertilization and are therefore not included in the illustration. (C) Schematic drawing of the imaging station of the automated phenotyping platform, GrowScreen-Rhizo2. The inclination angle of the rhizotron (A) is fixed to 45° with the transparent surface of the rhizotron facing downward. The image of the root system is taken by a high-resolution camera (B), positioned at a distance of 1 m to the rhizotron surface. The positions and angles of the two light panels (C) are adjusted to allow homogeneous illumination and prevent reflections in the images.
Measurements
Rhizotrons with S. hermaphrodita plants were placed into a novel phenotyping platform enabling simultaneous and non-destructive measurements of root and shoot growth of rhizotrons-grown plants (GrowScreen-Rhizo2, developed at Forschungszentrum Jülich GmbH, Germany). The design of the new phenotyping system GrowScreen-Rhizo2 is based on the experiences with the GrowScreen-Rhizo1 platform published in 2012 (Nagel et al., 2012). However, the GrowScreen-Rhizo1 concept of having fixed positions for rhizotrons and a moving cabinet for imaging the rhizotrons has been changed to enable higher flexibility and modularity. In the new platform, rhizotrons are moved in trays (five rhizotrons per tray) on a conveyor system and transported to an imaging station which has a fixed position. The inclination angle of the rhizotrons is adjusted always to 45° with the transparent plate of the rhizotrons facing downward to force the roots to grow toward the transparent plate. Inside the imaging station individual rhizotrons are taken out of the tray and positioned automatically in front of a high resolution camera (AV GT6600, Allied Vision Technologies, Germany; resolution 142 μm per pixel) which is taking one image of the whole transparent rhizotron surface (Figure 1C). During image acquisition, the door of the imaging station is closed automatically with rolling cutter gates to prevent undesired light reflections on the Plexiglas plate of the rhizotrons and the roots are illuminated using two LED panels (30 W 40 mm × 40 mm × 940 mm, color temperature 4000 K, item Industrietechnik GmbH, Germany). After imaging, the gate is opened and the rhizotron is placed back to into the tray completing the routine. Pictures were taken automatically once a week. In addition, plants were harvested at 30, 60, and 90 days after transplanting seedlings to determine leaf, stem, and root biomass. For harvest, rhizotrons were opened and the sandy substrate was carefully washed out with tap water and roots were cleaned thoroughly. Leaves, stems, and roots were cut and separately dried at 70°C to constant weight to determine the biomass dry weight. The pictures of the roots, acquired by GrowScreen-Rhizo2 during growth were evaluated for total visible root length and root distribution by using the software GROWSCREEN-Root in combination with a graphic tablet with pens (Wacom Cintiq 21UX, 31 CANCOM Deutschland GmbH, Düsseldorf, Germany) (Nagel et al., 2009). In order to visualize the spatial distribution of roots for any given treatment, we wrote an additional software to combine the data from all root images of each treatment in one image, representing root structure and distribution for any given treatment. To create these images, the area of a given root picture, as acquired by the GrowScreen-Rhizo2 system, was subdivided into a grid of cells, sized 25 × 25 pixels each. Each cell was initialized with zero. Every time, any part of the root structure was found in a given cell, the value of this particular cell increased by one. Further limiting the maximum increase per pixel per plant to one, allowed us to visualize root distribution in relation (%) to the number of plants per treatment. The generated images were converted from gray scale single channel images to three channel false-color images (Ware, 1988). This conversion was done using Opencv’s build-in function applyColorMap using the JET colormap (Bradski, 2000).
In mesocosms, the above-ground biomass was harvested at the end of the growth season in 2014, 2015, and 2016, and dried at 70°C to constant weight to determine the biomass dry mass. In 2016, the root biomass of four replicates for each treatment was determined by washing out the substrate, cleaning roots thoroughly and drying them at 70°C to constant weight. Root growth of plants grown in mesocosms was monitored non-invasively by mini-rhizotrons, which are Plexiglas tubes horizontally installed in 30 and 60 cm depth of the mesocosms. Pictures of root systems were taken after 6, 18, and 30 months after planting, using the CI In-Situ Root Imager (CID Bio-Science Inc., Camas, WA, United States). The pictures were than further processed as described above by using the software GROWSCREEN-Root to determine root length and distribution.
Daily light integral values (DLI) were directly measured at place on the rhizotron and mesocosm facility, employing a LI-COR: Li-190 device (LI-COR Environmental – GmbH, Bad Homburg, Germany).
Soil samples were taken at the date of destructive biomass harvests in each rhizotron. In unfertilized-, mineral-fertilized and broadcast digestate-fertilized rhizotrons, one mixed sample was taken in a depth from 0 to 20 cm and a second in the depth of 25–50 cm. In rhizotrons with digestate depot fertilization, one sample was taken in a radius of 3, 6, and 12 cm around the digestate patch, in each rhizotron (Figure 1). Soil samples were stored at 4°C until determination of NH4+, NO2−, NO3−, electric conductivity and pH were performed. NH4+, NO2−, and NO3− were measured via ion chromatography (Dionex DX-500, AS23; eluent: 0.8 mM sodium bicarbonate and 4.5 mM sodium carbonate) in 0.1 M KCl extraction. Soil pH was determined using standard electrodes (Hanna Instruments pH 209 pH-meter, Vöhringen, Germany), using 0.01 M CaCl2 solution at 20°C. Nitrogen content of S. hermaphrodita leaves was determined by elemental analysis (VarioELcube, Elementar Analysensysteme GmbH, Langenselbold, Germany) after milling dried leaves for 60 sec. in a ball mill at 30 Hz (Retsch Mixer Mill MM 400, Retsch GmbH, Haan, Germany).
Statistical Analysis
The rhizotron experiment consisted of four treatments (digestate broadcast fertilization, localized digestate depot fertilization, mineral broadcast fertilization, and unfertilized control) with three sampling dates (30, 60, and 90 days), with eight repetitions per treatment. The mesocosm experiment had the same treatments in six repetitions of which the above ground biomass was harvested at the end of the vegetation period in 2014, 2015, and 2016. The below-ground biomass of the mesocosms was harvested (n = 4) for each treatment in October 2016. Statistical analysis was performed with analysis of variance (ANOVA) with an a posteriori test in R 3.0.3 (The R Foundation for Statistical Computing, 2014) using the work package “Agricolae” (de Mendiburu, 2014).
Results
Soil Analysis in Rhizotrons
Thirty days after transplanting the seedlings, all fertilization treatments showed a rapid conversion from ammonium to nitrate (Table 2). However, for the two digestate fertilized variants (broadcast and depot) also the intermediate product of mineralization, nitrite, was found in high concentrations, especially in a 3–6 cm radius around the digestate depot. In digestate broadcast fertilized rhizotrons, 85% of the measured nitrogen was found in the form of nitrate. In digestate depot fertilized rhizotrons, 20% of the measured nitrogen remained as ammonium and 33% was found as nitrite. Sixty days after planting, nitrite was not detected, while nitrate concentrations reached their maximum levels in the digestate-fertilized horizon of broadcast fertilized digestate rhizotrons as well as around the depot fertilized zones. Nitrogen in the form of ammonium was only detected in a 6–12 cm radius around the digestate depots. In digestate broadcast and NPK fertilized rhizotrons, only minor amounts of ammonium were detected in the fertilized horizon. Ninety days after planting, almost all nitrogen in all treatments was present in the form of nitrate, mainly located in the fertilized horizons of digestate broadcast -, NPK fertilization and a 3–6 cm radius around the digestate depots.
After 30 days, nitrogen fertilization, regardless of application technique, lowered the pH to 6.4. Contrastingly, the pH of the sandy substrate outside the 12 cm zone that was affected by depot fertilization had values of pH 7.0. Only within the radius closest to the digestate depots (<3 cm) pH 8.5 was reached. In the following 2 months, pH values generally increased and leveled off between pH 7 and 7.5. Lower pH values (6.6) were only found within a 6 cm radius around the digestate depots and the NPK fertilized horizon of mineral fertilized rhizotrons.
Root Growth and Root System Architecture
Rhizotrons
Thirty and sixty days after planting of S. hermaphrodita into rhizotrons, the form and application technique of the different fertilizers had a strong influence on the distribution of roots (Figure 2). However, root mass did not differ significantly between treatments until day 60 but differed strongly in their foraging behavior in the rhizotron experiment (Figure 3). Unfertilized control and digestate depot fertilized plants accessed the maximum depth of the rhizotrons within 60 days. Digestate broadcast and NPK fertilized plants only reached a rooting depth of 40 cm with 99% of the measured root length located in the fertilized horizon for digestate broadcast and 60 % for NPK fertilization, respectively. Digestate depot fertilization resulted in a strongly heterogeneous distribution of roots over the rhizotrons. Even though after 30 and 60 days when roots accessed deeper areas of the rhizotrons, no roots were present within a 6 cm radius around the digestate depots. After 60 days, only 1% of the total root length was located within this radius. After 90 days, the root growth pattern changed dramatically and 45% of the root biomass of digestate depot fertilized plants were found within this 6 cm radius around the digestate depots resulting in the formation of a dense root cluster around the digestate depot. Even after 90 days, roots in NPK and digestate broadcast fertilized rhizotrons did not reach the maximum depth of the rhizotrons and major part of their roots remained located in the fertilized horizon (60% for NPK and 80% for digestate broadcast fertilization).
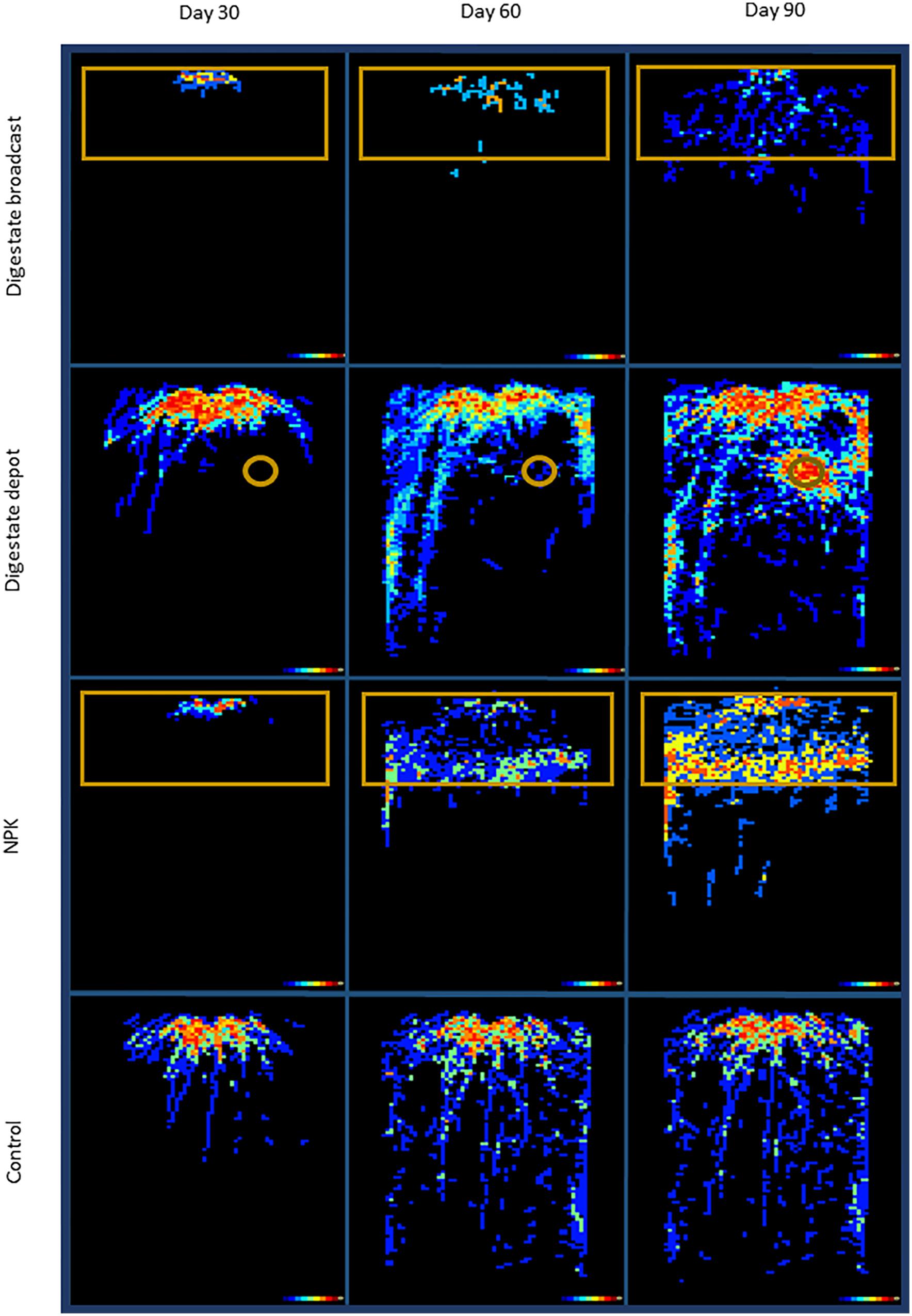
FIGURE 2. Hit-Map of rhizotrons. In digestate depot fertilized rhizotrons roots of S. hermaphrodita avoid the digestate depot zone for 60 days but form a dense root cluster in the depot zone after 90 days. Colors indicate the number of replicates that grew roots at the specific pixel (black: none; blue: 1–2; green 3–4; orange 5–6; red 7–8 replicates). Orange frames illustrate the fertilized zones of the rhizotrons. All fertilization treatments were adjusted to a digestate application of 40 t ha−1: Control: no fertilization; Digestate broadcast: homogeneously incorporated in the top 20 cm; Digestate depot: localized digestate application; NPK homogeneously incorporated in the top 20 cm; n = 8 replicates for each treatment and time of harvest.
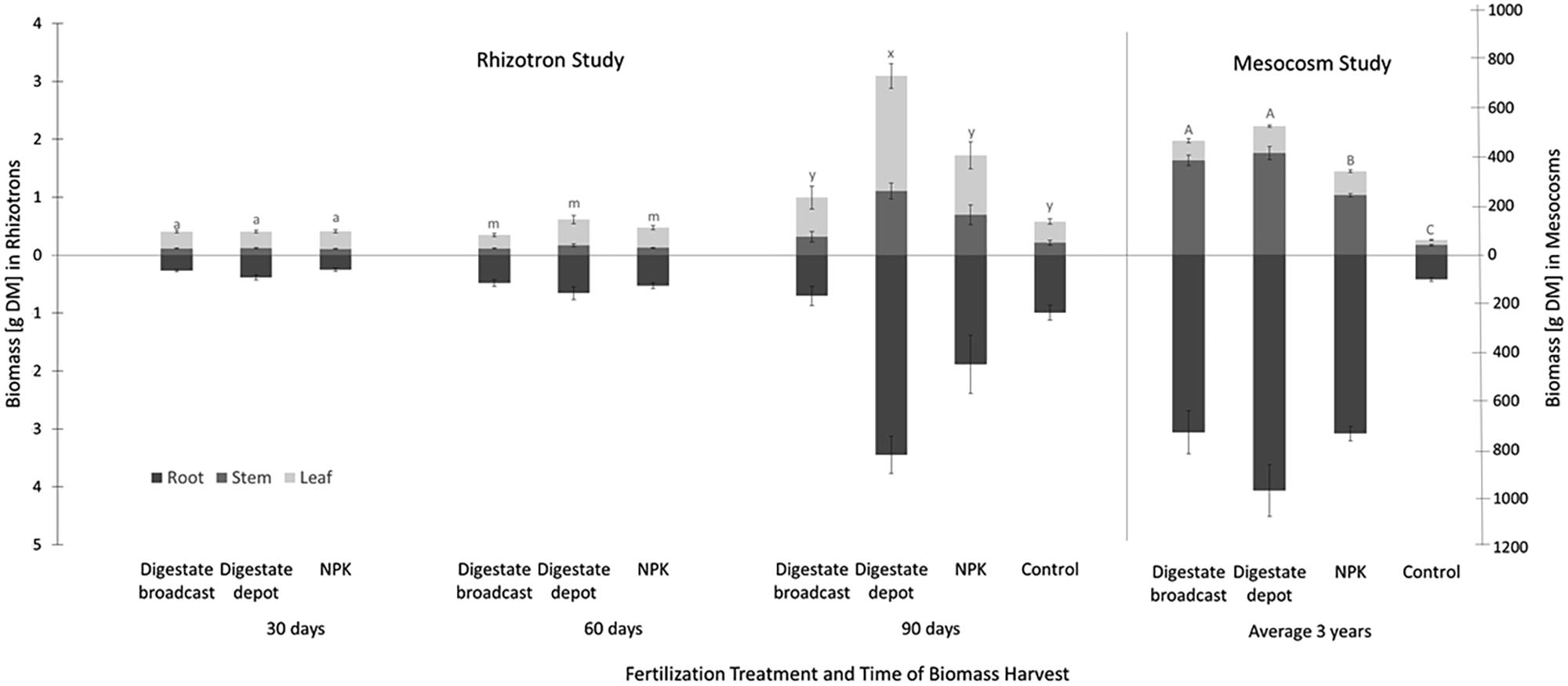
FIGURE 3. Plant biomass yield of S. hermaphrodita in rhizotrons and mesocosms. All fertilization treatments were adjusted to a digestate application of 40 t ha−1. Digestate broadcast: homogeneously incorporated in the top 20 cm; Digestate depot: localized digestate application; NPK: homogeneously incorporated in the top 20 cm; Control: no fertilization. Rhizotrons: n = 8 replicates for each treatment and time of harvest; Mesocosms: n = 7 replicates for each treatment harvested in 2014, 2015, and 2016. Root biomass was only measured in 2016. Values labeled with the same letter are not significantly different at p < 0.05; error bars indicate the standard error.
Mesocosms
After 1 year of growth, S. hermaphrodita in mesocosms located 60% of the measured root length in a depth of 30 cm when fertilized with broadcast digestate or NPK-fertilizer. When fertilized with localized digestate depot, a root cluster was visible at 30 cm depth, accumulating 90% of the measured root length in 30 cm depth, while unfertilized control plants had 70% of the measured root length in the same depth. After 3 years root distribution generally shifted more toward the lower soil levels with 60% of the measured root length at 60 cm depth for digestate broadcast fertilized plants and 55% for NPK fertilized plants. Digestate depot fertilized plants as well as control plants still had the higher share of the measured root length in 30 cm depth with 55 and 60%, respectively.
Biomass and Mass Fraction
Rhizotrons
In rhizotrons biomass increased on average by 40% from 0.7 g after 1 month to 1 g after 2 months, with no significant difference between treatments (Figure 3). However, after 90 days the biomass of digestate depot fertilized plants had increased by a factor 5–6.5 g. Broadcast fertilization with digestate or NPK did not result in a significant increase in biomass compared to the unfertilized control. At that time, unfertilized control plants as well as NPK and digestate depot fertilized plants accumulated approx. 50% of their total biomass in roots. Further, for digestate depot fertilized plants, half of the root biomass was located within a 6 cm radius around the digestate depot (Figure 4). Plants that received digestate broadcast fertilization had a 40% smaller root mass fraction than unfertilized control plants while localized digestate depot fertilization and mineral NPK fertilization did not show a significant difference to unfertilized control plants.
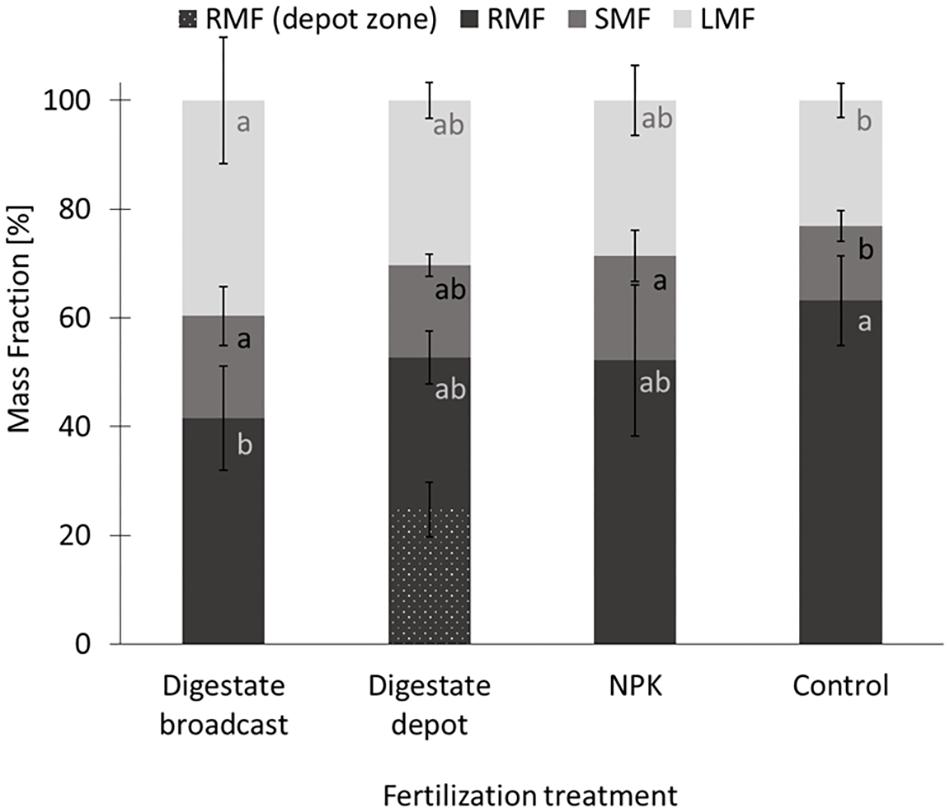
FIGURE 4. Mass fractions of S. hermaphrodita grown in rhizotrons for 90 days. RMF, root mass fraction; depot zone, zone in a 6 cm radius around the digestate depot; SMF, stem mass fraction; LMF, leaf mass fraction. All fertilization treatments were adjusted to a digestate application of 40 t ha−1. Digestate broadcast: homogeneously incorporated in the top 20 cm; Digestate depot: localized digestate application; NPK: homogeneously incorporated in the top 20 cm; Control: no fertilization; n = 8 replicates for each treatment. Values labeled with the same letter are not significantly different at p < 0.05. Error bars indicate the standard error.
Mesocosms
After three growing seasons in mesocosms, no significant difference between total biomass of plants fertilized with biogas digestate broadcast or depot fertilization was found (Figure 3). Depot fertilized plants developed a 25% larger root system than broadcast fertilized plants. NPK fertilized plants produced 20% less biomass than digestate-fertilized plants but six times more than the unfertilized control plants. Digestate depot fertilization resulted in nine times more biomass compared to the unfertilized control and broadcast digestate fertilization seven times, respectively.
Leaf Nitrogen Content in Rhizotrons
In rhizotrons, the nitrogen concentration of the leaves for digestate broadcast and NPK fertilized plants was between 4 and 5% throughout the experimental time of 3 months (Table 2). After 30 days, digestate depot fertilized rhizotron plants only contained 2.9% of nitrogen in their leaves. After 60 days, nitrogen concentration increased to 4.1% and after 90 days it was in the same range as measured for digestate broadcast and NPK fertilized plants. Unfertilized control plants only contained 1.7% of nitrogen.
Discussion
Nitrogen Turnover in Rhizotrons
In accordance with the processes described of the CULTAN Method – proposed by Sommer (2003) for mineral ammonium fertilizers, the main nitrogen form of the digestate was mineralized from ammonium to nitrate. For digestate broadcast and NPK fertilization 80–90% of the measured nitrogen was available in the form of nitrate as early as 30 days after fertilization. Nitrate, compared to ammonium, is very mobile in the soil. Accordingly, nitrate was also the form of nitrogen that was found in the non-fertilized lower horizon of the rhizotrons. Since to the lower horizon, no nutrients were added, they must have arrived there by leaching (Baker, 2001). In rhizotrons receiving digestate depot fertilization, conversion from ammonium to nitrite was faster than the conversion from nitrite to nitrate. Accordingly, nitrite accumulated in a radius of 6 cm around the digestate depot. In digestate depot fertilized rhizotrons, digestate was applied locally in very high amounts and thus contained highest NH4+ concentrations. The combination of a regular watering of the rhizotrons with high concentration of organic material with a C/N-ratio of approx. 6 in the depot fertilized zone may have allowed for high microbial activity and created an environment with partially anoxic conditions that favors the formation of nitrite (Nelson and Bremner, 1969; Van Cleemput and Samater, 1995; Möller and Müller, 2012). In addition, the alkaline pH near the digestate fertilized zone favors the formation of nitrite in sandy soils (Greco et al., 2012). Once nitrite starts to accumulate in the soil, it can inhibit microbial activity. In a feedback loop, this may have further favored the formation of even more nitrite as transformation from ammonium to nitrite is not as much effected as the succeeding transformation from nitrite to nitrate (Grant et al., 1979). Nitrate, the mobile form of nitrogen was found from 12 cm distance to the digestate depot (Baker, 2001). Ammonium was only found up to a distance of 6 cm from the digestate depot, as it is not very mobile in soils (Clarke and Barley, 1968; Pang et al., 1973). In our study, 60 days after fertilization, ammonium was almost completely mineralized to nitrate, leaving only traces of ammonium and nitrite in digestate broadcast and NPK fertilized rhizotrons. In digestate depot fertilized rhizotrons, also after 60 days, high concentrations of ammonium were present, indicating a delayed conversion from ammonium to nitrate compared to broadcast fertilization (Grant et al., 1979; Sommer, 2005). Even though the high pH of >8 in the near depot zone was found to be very favorable for the mineralization of organic N-compounds from corn residues, like digestate (Deng and Tabatabai, 2000). The pH in the depot zone dropped to a range of pH 6.8–7.5 until day 60 after fertilization partially due to diffusion and partially due to the release of protons in the mineralization process (Giusquiani et al., 1995; Barak et al., 1997). Yet, also in digestate depot fertilized rhizotrons almost all ammonium was mineralized to nitrate within 90 days leaving only traces of nitrite and ammonium near the digestate depot.
Nutrient Status and Root-Growth
Rhizotrons
The root growth of S. hermaphrodita plants responded to the different fertilizer distributions and consequently different available forms of nitrogen over time. Even though there were no significant differences between fertilizer treatments in terms of biomass, clear differences in root distribution were observed (Figures 2, 3). The broadcast application of digestate and NPK fertilizer, combined with a regular irrigation resulted in optimal supply of nutrients for seedlings of S. hermaphrodita (also indicated by the leaf nitrogen content >4%), reducing the necessity of seedlings to invest into root-growth (Forde and Lorenzo, 2001). Contrastingly, no nutrients were available in unfertilized control plants, while nutrients for digestate depot fertilized plants were not available in the rhizosphere (observable via the low leaf nitrogen content <3%). Consequently, plants invested into a deep and far-reaching root system to gain access to nutrients. These findings match the theory behind the CULTAN-method proposed by Sommer (2003) and also correspond well with results reviewed by Nkebiwe et al. (2016), focusing on controlled placement of fertilizers rich in ammonium. Additionally, the roots of plants growing in digestate depot fertilized rhizotrons, avoided to colonize a zone of approximately 6 cm radius from the digestate depot. This growing pattern was even more pronounced after 60 days. Here, roots in zones of the rhizotrons, not effected by fertilization, reached already the bottom of rhizotron while still very little root growth was observed in the 6 cm radius zone around the digestate depot. Given the high concentrations of ammonium and nitrite found within the 6 cm radius zone around the digestate depot in combination with root damages observed at root tips that contacted the area (Figure 5), we conclude that nitrite and ammonium toxicity are the main causes for the avoidance of this zone by the roots within the first 60 days after transplanting (Pan et al., 2016). Again, this temporarily zone of avoidance in a localized depot has been described earlier by Sommer (2003). Oke (1966) found that on sandy soil already nitrite concentrations >40 ppm can be toxic, especially to plant seedlings. In this experiment, we measured nitrite concentrations of <90 ppm in the depot zone. Further, ammonium toxicity was already studied intensively and observed when organic fertilizers, based on biogenic residues after anaerobic digestion were applied (Shaviv, 1988; Salminen and Rintala, 2002; Nkebiwe et al., 2016; Pan et al., 2016). Overall, results show consistently that high ammonium doses from digestate negatively affect seedling growth, mainly due to hampered root development.
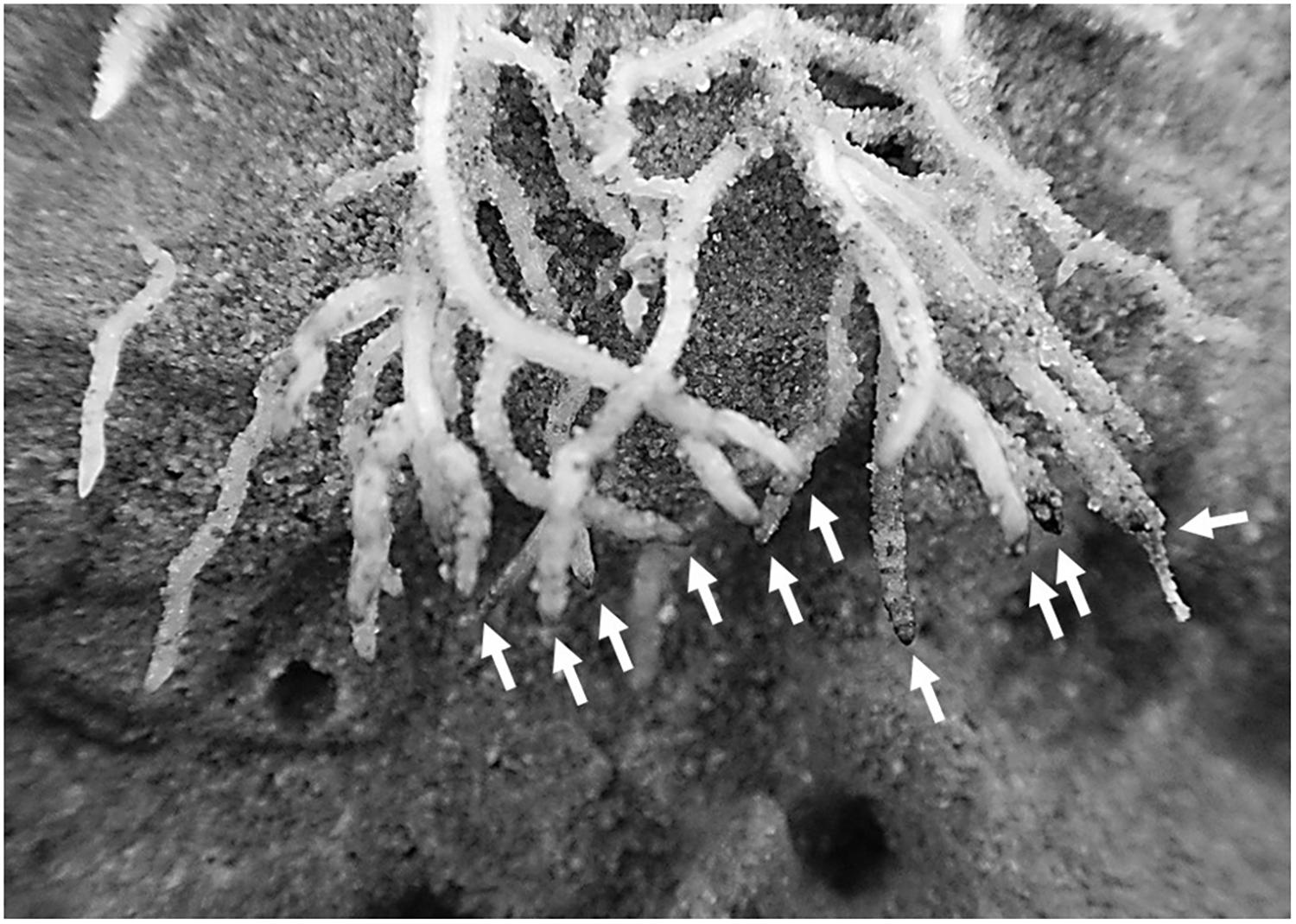
FIGURE 5. Brown and rotten root tips of S. hermaphrodita grown in digestate depot fertilized rhizotrons occurred in a 6 cm radius around the digestate depot 60 days after planting. Digestate depot fertilization was calculated to simulate a digestate application of 40 t ha−1. Arrows indicate the damaged root tips.
After 60 days, the root growth pattern in depot-fertilized rhizotrons changed dramatically and 90 days after planting a dense root cluster of S. hermaphrodita around the nutrient rich digestate depot was observed. Root proliferation in nutrient rich patches was not observed yet for S. hermaphrodita, but for several herbaceous plant species (Rajaniemi and Reynolds, 2004). Plants can increase their rooting density in nutrient rich patches to forage for these nutrients, increasing the nutrient uptake efficiency and thus confer a competitive advantage toward weeds without access to nutrient patches (Robinson et al., 1999; Hodge, 2004; Rajaniemi, 2007). The increased access to nutrients is also shown by a parallel increase in leaf nitrogen content from only 2.9% at 60 days after transplanting to 4.4% measured in leaves of digestate depot fertilized S. hermaphrodita plants 90 days after transplanting. The massive increase of root biomass in the depot zone between day 60 and day 90 after planting, contributing to 50% of the total root mass of digestate depot fertilized S. hermaphrodita plants, resulted in the highest root mass across the four treatments (Figure 1). Also in our mesocosm study, the formation of dense root clusters in the digestate depot fertilized zone was observable throughout the 3-year experimental time under outdoor conditions (Figure 6).
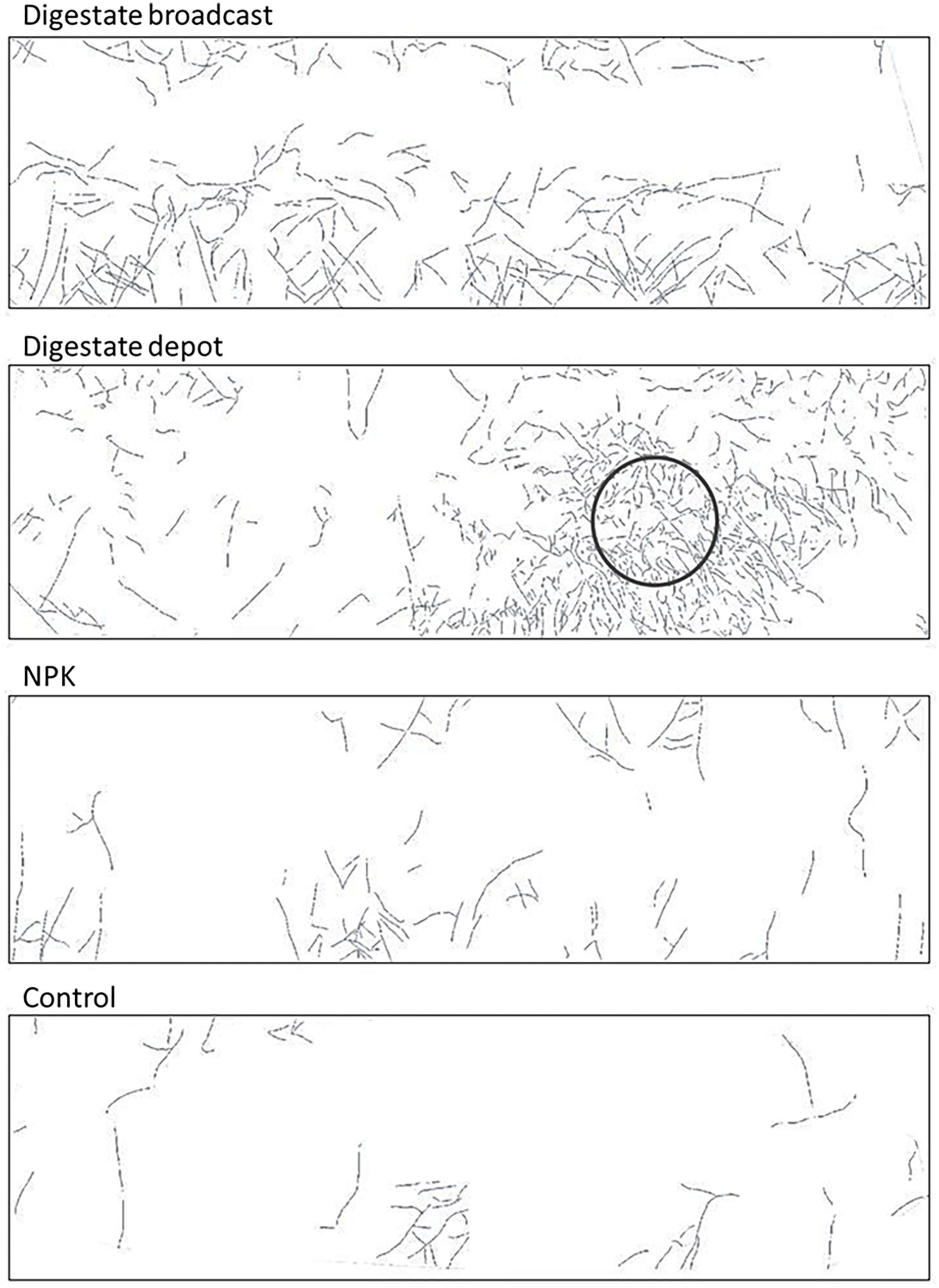
FIGURE 6. Root distribution of S. hermaphrodita cultivated in mesocosms measured by mini-rhizotrons after 2 years in 30 cm depth: roots form a dense root cluster in the depot-fertilized zone. Control: no fertilization; Digestate broadcast: homogeneous surface application; Digestate depot: localized digestate application; NPK homogeneous surface application. Pictures show a representative example of n = 4 replicates for each treatment and time of harvest.
In rhizotrons, after 90 days, NPK and digestate broadcast fertilization did not result in maximum possible rooting depth (i.e., depth of the rhizotron), and the major share of the root systems were located in the fertilized horizon. As plants were watered throughout the experiment, and roots had already sufficient access to the nutrients (indicated by the leaf nitrogen content >4%) plants did not invest into the formation of a deep reaching root system (Poorter et al., 2012b). Overall, the root systems that developed following digestate broadcast treatment remained smaller than the root systems of NPK fertilized plants. Phytotoxic effects of digestates, particularly affecting root growth of plants at early developmental stages, have been reported earlier and have besides ammonium toxicity also been explained by high concentrations of organic acids in fresh digestates (Salminen and Rintala, 2002; Abdullahi et al., 2008; Drennan and DiStefano, 2014). Control plants that had no access to nitrogen in the sandy substrate, resulting in a low leaf nitrogen content <2% and high investment into a deep reaching root-system, resulted in the highest root mass fraction of all variants (Figure 4) and thereby corresponded well to findings of previous studies (Poorter et al., 2012b).
Mesocosms
In mesocosms, the root mass fraction of S. hermaphrodita was generally higher after three growing seasons than found in rhizotrons. We explain this by the fact, that S. hermaphrodita is a perennial species, of which the shoots die off at the end of each vegetation period, while the major part of the root system stays intact and also serves as reservoir for a successful regrowth in the following growing period (Borkowska et al., 2009). As we only measured the root mass after three growing periods of mesocosms grown plants, this has a clear effect in the root mass fraction when compared to root mass measured after 90 days in rhizotrons.
Biomass Yield
Rhizotrons
In rhizotrons, the above ground biomass increased gradually until day 60 of the experiment as plants invested mainly in root-growth. Accordingly, no significant differences in biomass of S. hermaphrodita were observed at day 60 throughout all treatments. This changed drastically when roots in digestate depot fertilized rhizotrons started to access the nutrient rich depot zone. The intense foraging for nutrients resulted in a faster increase in stem and leaf biomass as observed in all other variants. In his meta-analysis over 39 studies, Nkebiwe et al. (2016) also found a positive effect of localized fertilizer placement on biomass yield, especially for early plant development stages. Specifically for organic fertilizers like poultry litter (Pote et al., 2011), separated dairy sludge (Bittman et al., 2012) and manure slurries (Dell et al., 2000), the localized application had a positive effect on the total biomass.
Broadcast application of digestate yielded less biomass than NPK fertilization, even though leaf and soil analysis showed an adequate supply with nutrients and water for both variants. However, the root system of digestate broadcast fertilized plants was smaller and less outstretched as the root system of NPK fertilized plants as discussed earlier.
Mesocosms
After three vegetation periods of S. hermaphrodita in mesocosms under outdoor conditions, the different fertilization treatments showed different responses with respect to biomass than the same treatments in the rhizotrons. No significant biomass yield difference between digestate depot and digestate broadcast fertilization was observed. As discussed earlier, the localized placement of fertilizers has beneficial effects, especially in the early development stages (Nkebiwe et al., 2016). Further, the phytotoxic effects of the digestate, especially observed on the root growth of digestate broadcast fertilized plants in the rhizotrons, also relates to the early development stages of plants (Möller and Müller, 2012). Accordingly, it cannot be expected that effects last over this 3-year experimental time, especially as S. hermaphrodita is a perennial plant that keeps its extensive root system over the years (Borkowska et al., 2009).
The fact that digestate fertilization, independently from the type of application, produced higher biomass yields than mineral NPK fertilization, can be related to an increase of soil fertility over the years and has been discussed in a separate publication (Nabel et al., 2017).
General Discussion
Localized application of biogas digestate as digestate depot in the rhizosphere of S. hermaphrodita seedlings fostered the successful establishment of S. hermaphrodita on marginal soils. Digestate depot fertilization strongly increased the rooting depth of seedlings, allowing improved access to water which could make them less susceptible to drought stress (Ma et al., 2009; Su et al., 2015). Drought can be the main growth-limiting factor, especially on marginal sandy soils with low WHC, making this deep rooting side effect of depot fertilization a positive one for adapting to drought regimes (Borkowska et al., 2009). The root-cluster formation around the digestate depot zone allowed the S. hermaphrodita seedling good access to the nutrients, resulting in rapid increase of biomass. Earlier studies showed that this can be an efficient way to make plants more competitive against weeds and thus lower the need for additional weed control (Blackshaw et al., 2002; Melander et al., 2005). Even though in the presented mesocosms study digestate depot fertilization did not show an increased biomass yield compared to digestate broadcast fertilization after three growing seasons, we still see a high potential in the application of digestate in the form of localized depots in the rhizosphere in marginal soils. As S. hermaphrodita is a perennial crop with an extensive root system, any form of soil cultivation in order to incorporate the digestate after a broad surface application via, e.g., plowing could do severe harm to the plants. In addition, a lack of digestate incorporation could cause high volatile losses of ammonia, with high environmental costs (Rochette et al., 2009; Ma et al., 2010). A controlled placement of digestate depots in the rhizosphere, e.g., via spike-weal injection, would minimize the impact on soil and also minimize volatile losses of ammonia (Kozlovský et al., 2009). As this application technique might also contribute to an increased competitiveness of S. hermaphrodita over weeds and thus reduce the need to weed control, it would further strengthen the concept of extensive biomass production on marginal soils.
Conclusion
The application of digestate as a fertilizer in local depots into the rhizosphere of S. hermaphrodita grown in a marginal sandy substrate in rhizotrons and mesocosms resulted in a dense root formation around the depot-fertilized zone. After 3 months, half of the root biomass of depot-fertilized plants grown in rhizotrons was associated with the depot zone as a dense root-cluster; in contrast, root growth in the digestate broadcast fertilization treatment was strongly reduced. Overall, digestate depot fertilization resulted in a fivefold increase of total biomass compared to digestate broadcast fertilization in rhizotrons. Under outdoor conditions in a 3-year mesocosm experiment the increase in plant biomass was not significant, however. We conclude that digestate depot fertilization can contribute to an improved cultivation of S. hermaphrodita as a perennial energy-crop on marginal soils, especially for a successful establishment of seedlings, but its potential growth stimulation effect now needs more research under field conditions.
Author Contributions
MN, SDS, HP, RK, and NDJ conceived the study. MN performed the main experiments and conducted the research under the supervision of SDS, HP, RK, and NDJ. MN wrote the manuscript. KN planned and designed GrowScreen-Rhizo2. CD and CB processed images and analyzed data. VT helped with study design and data evaluation. All authors discussed the results, assisted in the manuscript preparation, and contributed to revisions.
Funding
This study conducted at Forschungszentrum Jülich GmbH, IBG 2: Plant Sciences was institutional funded by the Helmholtz Association (POFIII, Key Technologies for the Bioeconomy). Part of this work was supported by the German Plant-Phenotyping Network (DPPN), which is funded by the German Federal Ministry of Education and Research (BMBF, FKZ: 031A053).
Conflict of Interest Statement
The authors declare that the research was conducted in the absence of any commercial or financial relationships that could be construed as a potential conflict of interest.
Acknowledgments
The digestate and the sand were kindly provided by ADRW Naturpower GmbH and Co. Kg, Ameln, and Rheinische Baustoffwerke, Inden, respectively. The kind provision of the bins by EGN mbH, Viersen, used as mesocosms for plant cultivation is highly appreciated. Many thanks to Lucy Harrison, Marlene Müller, Edelgard Schölgens, Sabine Willbold, and colleagues from ZEA-3 for the maintenance, sampling, and chemical analysis of the plant and soil samples. We thank Andre Schallenberg for the help of setting up and harvesting of the mesocosm experiment. We thank Benedikt Janssen and Tim Schiffer for the technical support with the phenotyping infrastructure and Alexander Putz for providing the drawing of the image station of GrowScreen-Rhizo2. We highly acknowledge the financial support of numerous students’ apprentices by the DAAD and IAESTE program, providing great support for this experiment. We thank the reviewers for their valuable comments and suggestions to improve this manuscript.
References
Abdullahi, Y. A., Akunna, J. C., White, N. A., Hallett, P. D., and Wheatley. R. (2008). Investigating the effects of anaerobic and aerobic post-treatment on quality and stability of organic fraction of municipal solid waste as soil amendment. Bioresour. Technol. 99, 8631–36. doi: 10.1016/j.biortech.2008.04.027
Arthurson, V. (2009). Closing the global energy and nutrient cycles through application of biogas residue to agricultural land – Potential benefits and drawbacks. Energies 2, 226–242. doi: 10.3390/en20200226
Baker, J L. (2001). Limitations of improved nitrogen management to reduce nitrate leaching and increase use efficiency. ScientificWorldJournal 1(Suppl. 2), 10–16. doi: 10.1100/tsw.2001.457
Barak, P., Jobe, B. O., Krueger, A. R., Peterson, L. A., and Laird, D. A. (1997). Effects of long-term soil acidification due to nitrogen fertilizer inputs in wisconsin. Plant Soil 197, 61–69. doi: 10.1023/A:1004297607070
Beare, M. H., Hendrix, P. F., and Coleman, D. C. (1994). Water-stable aggregates and organic matter fractions in conventional- and no-tillage soils. Soil Sci. Soc. Am. J. 58: 777. doi: 10.2136/sssaj1994.03615995005800030020x
Bittman, S, Liu, A., Hunt, D. E., Forge, T. A., Kowalenko, C. G., Chantigny, M. H., and Buckley, K. (2012). Precision placement of separated dairy sludge improves early phosphorus nutrition and growth in corn (Zea mays L.). J. Environ. Qual. 41, 582–91. doi: 10.2134/jeq2011.0284
Blackshaw, R. E., Semach, G., and Janzen, H. H. (2002). Fertilizer application method affects nitrogen uptake in weeds and wheat. Weed Sci. 50, 634–41.
Blanco-Canqui, H. (2010). Energy crops and their implications on soil and environment. Agron. J. 102, 403–419. doi: 10.2134/agronj2009.0333
Borkowska, H.,. Molas, R., and Kupczyk, A. (2009). Virginia fanpetals (Sida hermaphrodita rusby) cultivated on light soil; height of yield and biomass productivity. Pol. J. Environ. Stud. 18, 563–568.
Bradski, G. (2000). The openCV library. Dr Dobbs J. Softw. Tools 25, 120–25. doi: 10.1111/0023-8333.50.s1.10
Brüll, A. (2015). Biomass – A Renewable Energy Source? Sustainable Complementary Biomass Production through Landscape Quality Management, Dissertation, Leuphana University of Lüneburg.
Clarke, A. L., and Barley, K. P. (1968). The uptake of nitrogen from soils in relation to solute diffusion. Austr. J. Soil Res. 6, 75–92. doi: 10.1071/SR9680075
Cooke, W. (1954). Recent advances in fertilizer placement. II.-fertilizer placement in England. J. Sci. Food Agric. 5, 429–440. doi: 10.1002/jsfa.2740050907
de Mendiburu, F., (2014). Agricolae: Statistical Procedures for Agricultural Research. R package version 1.2–0.
Dell, C. J., Meisinger, J. J., and Beegle, D. B. (2000). Subsurface application of manures slurries for conservation tillage and pasture soils and their impact on the nitrogen balance. J. Environ. Qual. 40, 352–61. doi: 10.2134/jeq2010.0069
Deng, S. P., and Tabatabai, M. A. (2000). Effect of cropping systems on nitrogen mineralization in soils. Biol. Fertil. Soils 31, 211–218. doi: 10.1007/s003740050647
Di, H. J., and Cameron, K. C. (2002). Nitrate leaching in temperate agroecosystems: sources, factors and mitigating strategies. Nutr. Cycl. Agroecosyst. 64, 237–256. doi: 10.1023/A:1021471531188
Drennan, M. F., and DiStefano, T. D. (2014). High solids co-digestion of food and landscape waste and the potential for ammonia toxicity. Waste Manage. 34, 1289–1298. doi: 10.1016/j.wasman.2014.03.019
Fang, S., Xue, J., and Tang, L. (2007). Biomass production and carbon sequestration potential in poplar plantations with different management patterns. J. Environ. Manage. 85, 672–679. doi: 10.1016/j.jenvman.2006.09.014
FAO (2009). The State of Food Insecurity in the World Economic Crises – Impacts and Lessons Learned 2009 Key Messages. Rome: FAO.
Forde, B. G., and Lorenzo, H. (2001). The nutritional control of root development. Plant Soil 232, 51–68. doi: 10.1023/A:1010329902165
Fritsche, U. R., Sims, R. E. H. H., and Monti, A. (2010). Direct and indirect land-use competition issues for energy crops and their sustainable production – An overview. Biofuels Bioprod. Biorefin. 4, 692–704. doi: 10.1002/bbb.258
Garwood, E A., and Williams, T E. (1967). Growth, water use and nutrient uptake from the subsoil by grass swards. J. Agric. Sci. Cambridge 69, 125–130. doi: 10.1017/S002185960001652X
Giusquiani, P. L., Pagliai, M., Gigliotti, G., Businelli, D., and Benetti, A. (1995). Urban waste compost: effects on physical, chemical, and biochemical soil properties. J. Environ. Qual. 24, 175–82. doi: 10.2134/jeq1995.00472425002400010024x
Graham-Rowe, D. (2011). Agriculture: beyond food versus fuel. Nature 474, S6–S8. doi: 10.1038/474S06a
Grant, I. F., Bancroft, K., and Alexander, M. (1979). SO2 and NO2 Effects on microbial activity in an acid forest soil. Microb. Ecol. 5, 85–89. doi: 10.1007/BF02010499
Greco, M., Chiappetta, A., Bruno, L., and Bitonti, M. B. (2012). In posidonia oceanica cadmium induces changes in DNA methylation and chromatin patterning. J. Exp. Bot. 63, 695–709. doi: 10.1093/jxb/err313
Haraldsen, T. K., Andersen, U., Krogstad, T., and Sørheim, R. (2011). Liquid digestate from anaerobic treatment of source-separated household waste as fertilizer to barley. Waste Manage. Res. J. Int. Solid Wastes Public Cleans. Assoc. ISWA 29, 1271–1276. doi: 10.1177/0734242X11411975
Hayashi, K., Koga, N., and Yanai, Y. (2009). Effects of field-applied composted cattle manure and chemical fertilizer on ammonia and particulate ammonium exchanges at an upland field. Atmos. Environ. 43, 5702–5707. doi: 10.1016/j.atmosenv.2009.07.043
Hodge, A. (2004). The plastic plant: root responses to heterogeneous supplies of nutrients. New Phytol. 162, 9–24. doi: 10.1111/j.1469-8137.2004.01015.x
Jablonowski, N., Tobias, D., Nabel, K. M., Damm, T., Klose, H., Müller, M., et al. (2017). Valorization of sida (Sida hermaphrodita) biomass for multiple energy purposes. GCB Bioenergy 9, 202–214. doi: 10.1111/gcbb.12346
Jing, J., Zhang, F., Rengel, Z., and Shen. J. (2012). Localized fertilization with P plus N elicits an ammonium-dependent enhancement of maize root growth and nutrient uptake. Field Crops Res. 133, 176–85. doi: 10.1016/j.fcr.2012.04.009
Kozlovský, O., Balík, J., Černý, J., Kulhánek, M., Kos, M., and Prášilová, M. (2009). Influence of nitrogen fertilizer injection (CULTAN) on yield, yield components formation and quality of winter wheat grain. Plant Soil Environ. 55, 536–543. doi: 10.17221/165/2009-PSE
Ma, B. L., Wu, T. Y., Tremblay, N., Deen, W., McLaughlin, N. B., Morrison, M. J., et al. (2010). On-farm assessment of the amount and timing of nitrogen fertilizer on ammonia volatilization. Agron. J. 102, 134–144. doi: 10.2134/agronj2009.0021
Ma, Q., Rengel, Z., and Rose, T. (2009). The effectiveness of deep placement of fertilisers is determined by crop species and edaphic conditions in mediterranean-type environments: a review. Austr. J. Soil Res. 47, 19–32. doi: 10.1071/SR08105
Melander, B., Rasmussen, I., and Bàrberi, P. (2005). Integrating physical and cultural methods of weed control— examples from european research. Weed Sci. 53, 369–381. doi: 10.1614/WS-04-136R
Möller, K., and Müller, T. (2012). Effects of anaerobic digestion on digestate nutrient availability and crop growth: a review. Eng. Life Sci. 12, 242–257. doi: 10.1002/elsc.201100085
Möller, K., and Stinner, W. (2009). Effects of different manuring systems with and without biogas digestion on soil mineral nitrogen content and on gaseous nitrogen losses (ammonia, nitrous oxides). Eur. J. Agron. 30, 1–16. doi: 10.1016/j.eja.2008.06.003
Nabel, M., Barbosa, D. B. P., Horsch, D., and Jablonowski, N. D. (2014). Energy crop (Sida hermaphrodita) fertilization using digestate under marginal soil conditions: a dose-response experiment. Energy Proc. 16:14000. doi: 10.1016/j.egypro.2014.10.358
Nabel, M., Schrey, S. D., Poorter, H., Koller, R., and Jablonowski, N. D. (2017). Effects of digestate fertilization on Sida hermaphrodita: boosting biomass yields on marginal soils by increasing soil fertility. Biomass Bioenergy 107, 207–213. doi: 10.1016/j.biombioe.2017.10.009
Nabel, M., Temperton, V. M., Poorter, H., Lücke, A., and Jablonowski, N. D. (2016). Energizing marginal soils – The establishment of the energy crop Sida hermaphrodita as dependent on digestate fertilization, NPK, and legume intercropping. Biomass Bioenergy 87, 9–16. doi: 10.1016/j.biombioe.2016.02.010
Nagel, K. A., Kastenholz, B., Jahnke, S., Van Dusschoten, D., Aach, T., Mühlich, M., et al. (2009). Temperature responses of roots: impact on growth, root system architecture and implications for phenotyping. Funct. Plant Biol. 36, 947–959. doi: 10.1071/FP09184
Nagel, K. A., Putz, A., Gilmer, F., Heinz, K., Fischbach, A., Pfeifer, J., et al. (2012). GROWSCREEN-rhizo is a novel phenotyping robot enabling simultaneous measurements of root and shoot growth for plants grown in soil- filled rhizotrons. Funct. Plant Biol. 39, 891–904. doi: 10.1071/FP12023
Nelson, D. W., and Bremner, J. M. (1969). Factors affecting chemical transformations of nitrite in soils. Soil Biol. Biochem. 1, 229–239. doi: 10.1016/0038-071790023-6
Nkebiwe, P. M., Weinmann, M., Bar-Tal, A., and Müller, T. (2016). Fertilizer placement to improve crop nutrient acquisition and yield: a review and meta-analysis. Field Crops Res. 196, 389–401. doi: 10.1016/j.fcr.2016.07.018
Nyord, T., Søgaard, H. T., Hansen, M. N., and Jensen, L. S. (2008). Injection methods to reduce ammonia emission from volatile liquid fertilisers applied to growing crops. Biosyst. Eng. 100, 235–44. doi: 10.1016/j.biosystemseng.2008.01.013
Pan, W. L., Madsen, I. J., Bolton, R. P., Graves, L., and Sistrunk, T. (2016). Ammonia/ammonium toxicity root symptoms induced by inorganic and organic fertilizers and placement. Agron. J. 108, 2485–92. doi: 10.2134/agronj2016.02.0122
Pang, P. C, Hedlin, R. A., and Cho, C. M. (1973). Transformation and movement of band-applied urea, ammonium sulfate, and ammonium hydroxide during incubation in several manitoba soils. Can. J. Soil Sci. 341, 331–341. doi: 10.4141/cjss73-048
Poorter, H., Bühler, J., Van Dusschoten, D., Climent, J., and Postma, J. A. (2012a). Pot size matters: a meta-analysis of the effects of rooting volume on plant growth. Funct. Plant Biol. 39, 839–50. doi: 10.1071/FP12049
Poorter, H., Niklas, K. J., Reich, P. B., Oleksyn, J., Poot, P., and Mommer, L. (2012b). Biomass allocation to leaves, stems and roots: meta-analyses of interspecific variation and environmental control. New Phytol. 193, 30–50. doi: 10.1111/j.1469-8137.2011.03952.x
Poorter, H., Fiorani, F., Pieruschka, R., Wojciechowski, T., van der Putten, W. H., Kleyer, M., et al. (2016). Pampered inside, pestered outside? Differences and similarities between plants growing in controlled conditions and in the field. New Phytol. 212, 838–55. doi: 10.1111/nph.14243
Pote, D. H., Way, T. R., Kleinman, P. J. A., Moore, P. A., Meisinger, J. J., Sistani, K. R., et al. (2011). Subsurface application of poultry litter in pasture and no-till soils. J. Environ. Qual. 40, 402–411. doi: 10.2134/jeq2010.0352
Rajaniemi, T. K. (2007). Root foraging traits and competitive ability in heterogeneous soils. Oecologia 153, 145–152. doi: 10.1007/s00442-007-0706-2
Rajaniemi, T. K., and Reynolds, H. L. (2004). Root foraging for patchy resources in eight herbaceous plant species. Oecologia 141, 519–25. doi: 10.1007/s00442-004-1666-4
Reeves, D. W. (1997). The role of soil organic matter in maintaining soil quality in continuous cropping systems. Soil Tillage Res. 43, 131–167. doi: 10.1016/S0167-1987(97)00038-X
Robinson, D., Hodge, A., Griffiths, B. S., and Fitter, A. H. (1999). Plant root proliferation in nitrogen-rich patches confers competitive advantage. Proc. R. Soc. B Biol. Sci. 266, 431–35. doi: 10.1098/rspb.1999.0656
Rochette, P., Angers, D. A., Chantigny, M. H., MacDonald, J. D., Gasser, M., and Bertrand, N. (2009). Reducing ammonia volatilization in a no-till soil by incorporating urea and pig slurry in shallow bands. Nutr. Cycl. Agroecosyst. 84, 71–80. doi: 10.1007/s10705-008-9227-6
Salminen, E., and Rintala, J. (2002). Anaerobic digestion of organic solid poultry slaughterhouse waste-a review. Bioresour. Technol. 83, 13–26. doi: 10.1016/S0960-852400199-7
Schröder, P., Herzig, R., Bojinov, B., Ruttens, A., Nehnevajova, E., Stamatiadis, S., et al. (2008). Bioenergy to save the world. producing novel energy plants for growth on abandoned land. Environ. Sci. Pollut. Res. Int. 15, 196–204. doi: 10.1065/espr2008.03.481
Shaviv, A. (1988). Control of nitrification rate by increasing ammonium concentration. Fert. Res. 17, 177–188. doi: 10.1007/BF01050278
Sommer, K. (2003). Foundation of CULTAN technique. Crop experience with N-injection (CULTAN). Results, perspective, practice. FAL Braunschweig Textbook 245, 1–22.
Sommer, K. (2005). CULTAN-Düngung: Physiologisch, Ökologisch, Ökonomisch Optimiertes Düngungsverfahren für Ackerkulturen, Grünland, Gemüse, Zierpflanzen und Obstgehölze. Essen: Verlag Th. Mann.
Spooner, D. M, Cusick, A. W., Hall, G. F., and Baskin, J. M. (1985). Observations on the distribution and ecology of Sida hermaphrodita (L.) Rusby (Malvaceae). Contribut. Bot. 11, 215–225.
Su, W., Liu, B., Liu, X., Li, X., Ren, T., Cong, R., et al. (2015). Effect of depth of fertilizer banded-placement on growth, nutrient uptake and yield of oilseed rape (Brassica napus L.). Eur. J. Agron. 62, 38–45. doi: 10.1016/j.eja.2014.09.002
Tiessen H., Cuevas, E., and Chacon, P. (1994). The role of soil organic matter in sustaining soil fertility. Nature 371, 783–85. doi: 10.1038/371783a0
Van Cleemput, O., and Samater, A. H. (1995). Nitrite in soils: accumulation and role in the formation of gaseous N compounds. Fert. Res. 45, 81–89. doi: 10.1007/BF00749884
Vaneeckhaute, C., Meers, E., Michels, E., Ghekiere, G., Accoe, F., and Tack, F. M. G. (2013). Closing the nutrient cycle by using bio-digestion waste derivatives as synthetic fertilizer substitutes: a field experiment. Biomass Bioenergy 55, 175–189. doi: 10.1016/j.biombioe.2013.01.032
Voelkner, A., Holthusen, D., Ellerbrock, R. H., and Horn, R. (2015a). Quantity of hydrophobic functional CH-groups – Decisive for soil water repellency caused by digestate amendment. Int. Agrophys. 29, 247–55. doi: 10.1515/intag-2015-0024
Voelkner, A., Ohl, S., Holthusen, D., Hartung, E., Dörner, J., and Horn, R. (2015b). Impact of mechanically pre-treated anaerobic digestates on soil properties. J. Soil Sci. Plant Nutr. 15, 882–895. doi: 10.4067/S0718-95162015005000061
Voigt, T. B., Lee, O. K., and Kling, G. J. (2012). Perennial herbaceous crops with potential for biofuel production in the temperate regions of the USA. CAB Rev. 7, 45–57. doi: 10.1079/PAVSN
Walsh, J. J., Jones, D. L., Edwards-Jones, G., and Williams, A. P. (2012). Replacing inorganic fertilizer with anaerobic digestate may maintain agricultural productivity at less environmental cost. J. Plant Nutr. Soil Sci. 175, 840–845. doi: 10.1002/jpln.201200214
Ware, C. (1988). Color/Maps color sequences for univariate maps: theory, experiments, and principles. IEEE Comput. Graph. Appl. 8, 41–49. doi: 10.1109/38.7760
Keywords: digestate fertilization, localized fertilizer placement, marginal substrate, perennial plants, rhizotron, root plasticity
Citation: Nabel M, Schrey SD, Poorter H, Koller R, Nagel KA, Temperton VM, Dietrich CC, Briese C and Jablonowski ND (2018) Coming Late for Dinner: Localized Digestate Depot Fertilization for Extensive Cultivation of Marginal Soil With Sida hermaphrodita. Front. Plant Sci. 9:1095. doi: 10.3389/fpls.2018.01095
Received: 19 February 2018; Accepted: 06 July 2018;
Published: 30 July 2018.
Edited by:
Rocio Millán, Centro de Investigaciones Energéticas, Medioambientales y Tecnológicas, SpainReviewed by:
Manoj Shrivastava, Indian Agricultural Research Institute (ICAR), IndiaJunye Wang, Athabasca University, Canada
Copyright © 2018 Nabel, Schrey, Poorter, Koller, Nagel, Temperton, Dietrich, Briese and Jablonowski. This is an open-access article distributed under the terms of the Creative Commons Attribution License (CC BY). The use, distribution or reproduction in other forums is permitted, provided the original author(s) and the copyright owner(s) are credited and that the original publication in this journal is cited, in accordance with accepted academic practice. No use, distribution or reproduction is permitted which does not comply with these terms.
*Correspondence: Nicolai D. Jablonowski, bi5kLmphYmxvbm93c2tpQGZ6LWp1ZWxpY2guZGU=
†Present address: Moritz Nabel, Federal Nature Conservation Agency (BfN), Bonn, Germany