- Department of Forest Health, Norwegian Institute of Bioeconomy Research, Ås, Norway
Dieback of European ash, caused by the ascomycete Hymenoscyphus fraxineus originating from Asia, has rapidly spread across Europe, and is threatening this keystone tree at a continental scale. High propagule pressure is characteristic to invasive species. Consistently, the enormous production of windborne ascospores by H. fraxineus in an ash forest with epidemic level of disease obviously facilitates its invasiveness and long distance spread. To understand the rate of build-up of propagule pressure by this pathogen following its local introduction, during 2011–2017 we monitored its sporulation at a newly infested ash stand in south-western Norway characterized with mild winters and cool summers. We also monitored the propagule pressure by Hymenoscyphus albidus, a non-pathogenic native species that competes for the same sporulation niche with H. fraxineus. During the monitoring period, crown condition of ash trees had impaired, and 20% of the dominant trees were severely damaged in 2017. H. fraxineus showed an exponential increase in spore production between 2012 and 2015, followed by drastic decline in 2016 and 2017. During 2011–2013, the two Hymenoscyphus species showed similar sporulation level, but thereafter spores of H. albidus were no longer detected. The data suggest that following local introduction, the population of H. fraxineus reaches rapidly an exponential growth stage if the local weather conditions are favorable for ascomata maturation across years. In the North Atlantic climate, summer temperatures critically influence the pathogen infection pressure, warm summers allowing the population to grow according to its biotic potential, whereas cold summers can cause a drastic decline in propagule pressure.
Introduction
The invasive fungus Hymenoscyphus fraxineus (syn. H. pseudoalbidus, anamorph Chalara fraxinea) (Kowalski, 2006; Queloz et al., 2011; Baral et al., 2014) is threatening the future existence of European ash (Fraxinus excelsior) in Europe (Pautasso et al., 2013; Mitchell et al., 2014). This ascomycete has probably been introduced to Europe from Asia, where it is regarded as an endophyte with some parasitic capacity in leaves of Manchurian ash (F. mandshurica)(Drenkhan et al., 2017), a close relative of European ash. The dieback of European ash represents a showcase scenario of forest damage that can follow from exposure of evolutionary naïve trees to invasive alien pests that have a high level of adaptation to congeneric tree species in their native range. Since the first European record of ash dieback in Poland in the early 1990s (Przybył, 2002), the disease has spread across Central, Northern, Eastern and Western Europe (Timmermann et al., 2011; McKinney et al., 2014) and currently only populations at the southern and eastern range margins of European ash remain disease free (Solheim and Hietala, 2017).
In Europe, ash dieback has shown an annual spread rate of 50–75 km (Gross et al., 2014; Hamelin et al., 2016; Solheim and Hietala, 2017). The natural spread of H. fraxineus takes place by windborne ascospores released from ascomata formed on previous-year ash-leaf-litter. Typical to foliar pathogens, the ascospores of H. fraxineus are released during early morning during summer months (Timmermann et al., 2011; Hietala et al., 2013). While Steinböck (2013) and Chandelier et al. (2014) showed that the ascospore amount of H. fraxineus in air reaches a low plateau already a few hundred meters away from an infested stand, the effective dispersal distance of the pathogen propagules remains to be established. Besides ascospores, H. fraxineus produces also conidia that have been proposed to function as spermatia (review by Gross et al., 2014) or have a role in secondary local spread of the fungus (Fones et al., 2016). Particularly during the 1990s and early 2000s, when the causative agent of ash dieback still was unknown, trade and movement of infected ash seedlings (Sansford, 2013) and timber (Husson et al., 2012) may have facilitated the spread of ash dieback.
A study from south-eastern Norway located at the edge of continental climate, with average temperature around 15–16°C during the summer months (June–August), showed that Hymenoscyphus fraxineus exerts a high ascospore pressure in the period between mid-July and mid-August (Hietala et al., 2013). In the warmer climate of central Europe, a high pathogen propagule pressure can occur already in early June (Chandelier et al., 2014; Dvorak et al., 2016). The high propagule pressure obviously enables the fungus to overcome leaf defence responses and competition imposed by indigenous endophytes that are at this stage still in a quiescent growth mode characterized by low population density (Cross et al., 2017). There is increasing evidence that propagule pressure is a crucial ecological trait that influences the success of introduction but also the transition of invasive species to the subsequent stages; local establishment followed by spread outside the area of introduction and eventual widespread dominance (Lockwood et al., 2005; Colautti et al., 2006). The aim of the present study was to document the build-up rate of propagule pressure of H. fraxineus at an initially healthy ash stand. For the purpose, we installed a volumetric spore sampler in an ash stand at the west coast of Norway in 2011. In this region, the first signs of ash dieback were observed in some forests in 2010. At the experimental stand, we monitored the level of airborne H. fraxineus propagules over seven growing seasons. We also profiled the propagule level of Hymenoscyphus albidus, a fungus that competes for the same sporulation niche as H. fraxineus, but is indigenous to Europe and harmless to European ash.
Materials and Methods
Spore Sampling and Assessment of Tree Health Condition
To monitor the release of ascospores by H. fraxineus and H. albidus, in 2011 we installed a solar power-driven Burkard 7-day volumetric spore sampler (Burkard Scientific, Uxbridge, United Kingdom) at ground level in the center of a mixed deciduous stand dominated by European ash and located in Fana, 15 km south of Bergen (Bergen municipality, 60° 15′ 59″ N, 5° 20′ 12″ E, 30 m a.s.l.). The sampler was equipped with a standard wind vane that directs the trapping orifice into the wind. This stand was chosen because it was still devoid of ash dieback symptoms in 2011 and H. albidus ascomata were present at that time. At an air throughput of 10 l/min, airborne particles were collected on the adhesive-coated (50 ml vaseline, 6 g paraffin wax, toluene), transparent Melinex tape, fastened to a revolving drum which moved past the air intake of the spore sampler at a rate of 2 mm per hour. With the collection tape changed weekly, this provided a continuous temporal record of airborne propagules. In 2011, sampling started July 26th and was continued until September 5th. In the years 2012–2017 spore sampling was repeated, starting already during the second half of June and continuing until the beginning/mid of October, with the exception of 2016 when sampling was terminated September 19th. The spore sampler was kept precisely at the same position in the subjected stand across the seven monitoring seasons.
As reference, weather data were obtained from a meteorological station located at Bergen airport Flesland 7 km northwest of the studied ash stand1. Missing data in some of measured meteorological parameters occurred due to technical problems at the station. Degree days were calculated by using a basal temperature of 5, 10, or 15°C.
In summer 2017, a damage assessment using defoliation as the main parameter (Eichhorn et al., 2016) was conducted on 20 dominant and 17 subdominant ash trees, subjectively selected and representative for the stand. Trees were considered to be healthy when they had up to 10% defoliation, slightly damaged when having 11–25% defoliation, moderately damaged when having 26–50% defoliation and severely damaged when the crown was more than 50% defoliated.
DNA Isolation and Real-Time PCR
For two randomly selected days per each sampling week in each year, DNA was isolated from tape segments corresponding to the period from midnight to noon (24:00 – 12:00) with protocol #8 (Isolation of DNA from Mouse Tails) of Easy-DNATM Kit (Invitrogen, Carlsbad, CA, United States) according to the manufacturer’s instructions. The resulting 50-μl-volume DNA samples were stored at −20°C until processed by real-time PCR. As one spore sampler was employed, each sampling day represents a single biological replicate.
The real-time PCR detection of H. fraxineus and H. albidus DNA was performed using FAST BLUE qPCR MasterMix Plus w/o UNG for probe Assay Low ROX (Eurogentec, Seraing, Belgium) without any modifications in reagent concentrations. For detection of H. fraxineus, we used the forward primer Cfrax-F 5′-ATTATATTGTTGCTTTAGCAGGTC-3, reverse primer Cfrax-R 5′-TCCTCTAGCAGGCACAGTC-3′ and probe C-frax-P5′-FAM- CTCTGGGCGTCGGCCTCG-BHQ1-3′ designed and tested for species specificity by Ioos et al. (2009). For detection of H. albidus, we used the primer probe set designed and tested for species specificity by Husson et al. (2011), with the modification of using JOE as the reporter dye instead of YY: forward primer Halb-F 5′TATATTGTTGCTTTAGCAGGTCGC-3′, reverse primer Halb-R 5′-ATCCTCTAGCAGGCACGGTC-3′, and probe Halb-P5′-JOE-CCGGGGCGTTGGCCTCG-BHQ1-3′.
For quantification of H. fraxineus DNA, the primer and probe concentrations were 300 nM and 100 nM, respectively, as described by Ioos et al. (2009). For quantification of H. albidus DNA, 900 nM concentrations were used for the primers and the probe (Hietala et al., 2013).
To obtain fungal DNA for construction of standard curves, three Norwegian H. fraxineus (2009-106/1/2, 2009-107/1/2, 2009-112/1/3) and three H. albidus strains (2009-111/1/4, 2009-124/3/2, 2009-124/4/1), deposited at the culture collection of the Norwegian Institute of Bioeconomy Research, were grown for 3 weeks at 21°C on 2% malt extract agar coated by a cellophane membrane, and subjected to DNA isolation with a DNeasy plant mini kit (Qiagen) according to the manufacturer’s instructions. The obtained DNA was quantified by using micro-volume spectrophotometer NanoDrop 2000 (Thermo Scientific, Wilmington, DE, United States), and pooled together in equal concentrations to prepare fungal DNA standard curve samples that contained 10, 1, 0.1, 0.01, and 0.001 ng of H. fraxineus or H. albidus DNA in a volume of 3 μl. To ensure that the cycle threshold values from the experimental samples fell within the standard curves and to investigate the presence of compounds inhibitory to PCR, 3-log dilution series were prepared for all the experimental samples. Each experimental sample had undiluted DNA as the most concentrated, and all log dilutions of a sample were used as templates in real-time PCR. For both the experimental and standard curve samples, 3 μl of the DNA solution was used as the template for each 25-μl PCR reaction. Each reaction was repeated twice. PCR cycling parameters were 95°C for 10 min, followed by 40 cycles of 95°C for 15 s and 65°C for 55 s. Fluorescence emissions were detected with an ABI Prism 7700 (Applied Biosystems). The data acquisition and analysis were performed with the Sequence Detection System software package (1.7a; Applied Biosystems). Standard curves were constructed based on the relationship of Ct values and known DNA concentrations: the Ct values were plotted against log-transformed DNA amounts, and linear regression equations were calculated for the quantification of DNA pools by interpolation in unknown samples.
To obtain an estimate for ascospore amount of H. fraxineus and H. albidus, we divided their real-time PCR based DNA amount estimates by the weight of 0.0634 or 0.0549 pg that correspond to the estimated haploid genome sizes of H. fraxineus (62 Mb, McMullan et al., 2018) and H. albidus (53 MB, Sønstebø et al., 2017).
Statistical Analyses
For each sampling year, Spearman’s rank correlation coefficients (ρ) were calculated for spore levels of H. fraxineus and H. albidus and weather parameters (mean daily temperature, precipitation, relative air humidity and wind speed), days with missing values either for the fungi or for the weather parameters were excluded from calculations. We also calculated the degree days for each year, by using 5, 10, or 15°C as basal temperatures. The relationship between initiation, peaking and ceasing of sporulation by H. fraxineus and degree days across the 7-year-long monitoring period was considered by calculating Pearson product moment correlations (r). ANOVA and Tukey HSD were used to consider species- and year-specific differences in maximum sporulation level; log transformation was made to reduce skewness in spore amount data. All the calculations were made by using SPSS 22.0 (IBM Inc., Armonk, NY, United States); correlations between variables and differences between treatments were considered significant at the 0.05 level.
Results
Development of Ash Health Condition
During installation of the spore sampler in 2011, no visible symptoms of ash dieback were detected in the stand and the health condition of the ash trees was generally considered as good, however, a structured assessment was not conducted. The results of the assessment in 2017 showed that 20% of the dominant trees were severely damaged, 35% moderately damaged, 35% slightly damaged and only 10% were still healthy (Supplementary Figure 1). Most of the severely damaged trees showed a large amount of epicormic shoots. Dead trees have so far not been observed in the stand.
Changes in Infection Pressure by H. albidus and H. fraxineus During the Experimental Period
Spores of H. albidus were detected only during the first three seasons of monitoring, while spores of H. fraxineus were present in all seven sampling years. In 2011, 2012 and 2013, the mean spore levels of H. albidus during the 5 days with most spores detected (TOP5) were estimated as 217, 19 and 144, respectively. The respective TOP5 spore levels for H. fraxineus were 302 (2011), 120 (2012) and 1384 (2013), the levels in 2012 and 2013 being significantly higher than for H. albidus. After 2013, only spores of H. fraxineus were detected: the mean TOP5 spore levels were estimated as 35305 (2014), 437360 (2015), 20786 (2016) and 30 (2017). The increments in TOP5 spore level of H. fraxineus between 2013 and 2014, and between 2014 and 2015 were significant, as were the declines in top spore level between 2015 and 2016, and between 2016 and 2017. The exponential increase in maximum spore level between 2012 and 2015 can be described by the model f(x) = 6.7504e2.7842x (R2 = 0.997), where x is the time in years (i.e., 1, 2012; 2, 2013 etc.).
Within Season Variation in Sporulation Period and Peak by H. albidus and H. fraxineus
There was considerable variation between different years in the initiation, peaking and ceasing of sporulation by H. albidus and H. fraxineus. In 2011, when spore sampling was started first in late July and continued only to the beginning of September, the first detection, maximum level (a single day with most spores detected, TOP1) and last detection of H. fraxineus spores occurred on calendar days 209, 209 and 246, respectively, and those of H. albidus on calendar days 209, 209 and 239, respectively. Between 2012 and 2017, the spore sampling was started in the second half of June. In 2012 the first detection, TOP1 and last detection of H. fraxineus spores occurred on calendar days 204, 225 and 233, and those of H. albidus on calendar days 187, 197 and 211, respectively. In 2013 the first detection, TOP1 and last detection of H. fraxineus spores occurred on calendar days 200, 242 and 267, and those of H. albidus on calendar days 172, 176 and 249, respectively. From 2014 to 2017 spores of H. albidus were no longer detected, and the first detection, TOP1 and last detection of H. fraxineus spores occurred on calendar days 168, 201 and 276 (2014), 184, 240 and 272 (2015), 177, 225 and 260 (2016), 233, 236 and 236 (2017), respectively.
Correlation of Weather and Sporulation of H. albidus and H. fraxineus
No significant relationship was generally observed between spore level of the two Hymenoscyphus species and temperature (Figure 1), precipitation (Figure 2), relative air humidity (Figure 3) or average wind speed (Figure 4) during the sporulation period. The exceptions were 2011 (ρ between H. fraxineus spore levels and average wind speed: 0.58), 2012 (ρ between H. albidus spore levels and average wind speed: −0.63) and 2016 (ρ between H. fraxineus spore levels and temperature: 0.45).
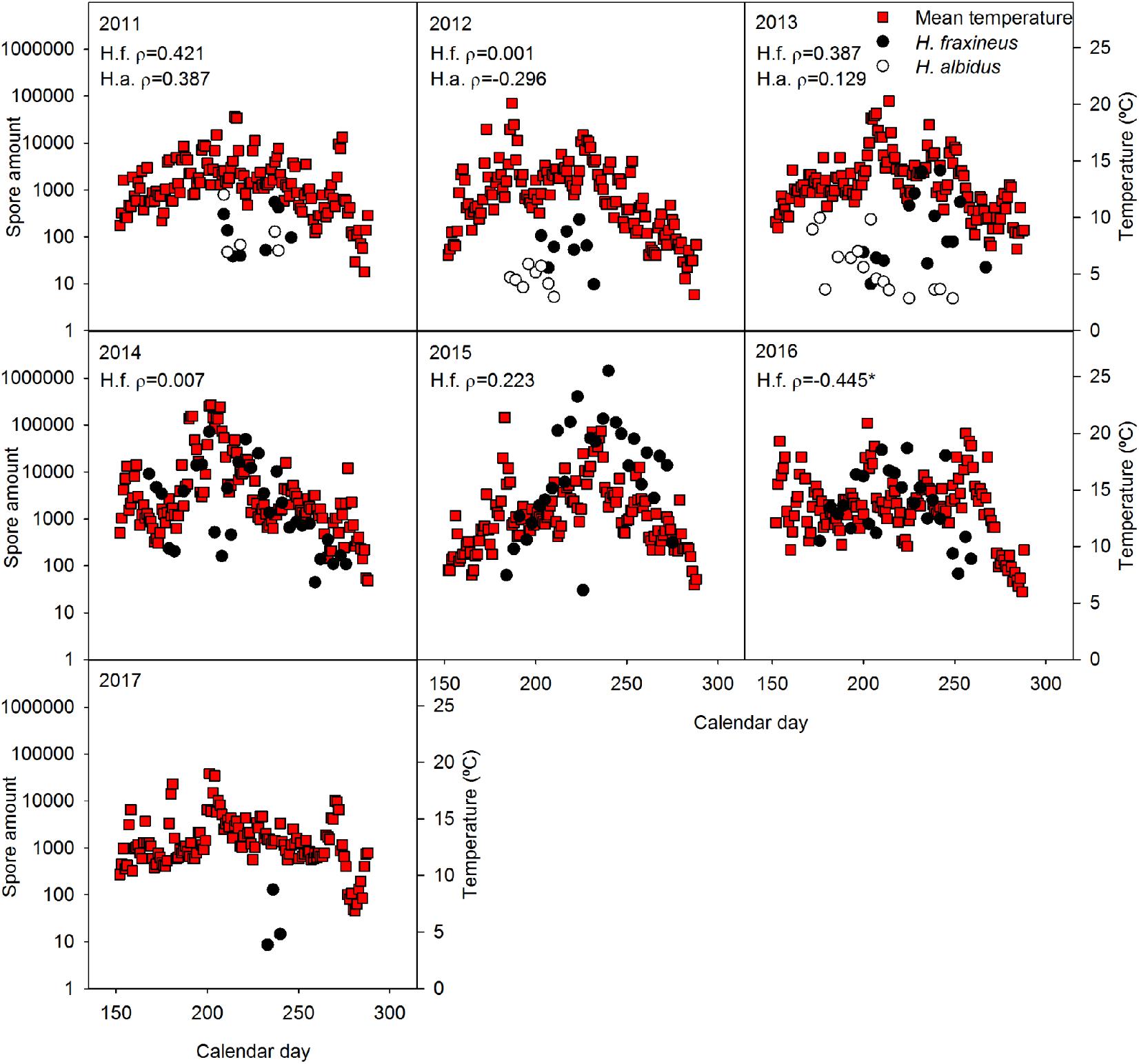
FIGURE 1. The amount of H. fraxineus (H.f.) and H. albidus (H.a.) spores 2011–2017 in relation to mean temperature (°C). The spore amount is calculated from amount DNA (pg/12 h) in the day period 12 midnight to 12 noon, each data-point constituting a single biological replicate. The spore data represent two randomly chosen days per each sampling week, whereas temperature data are provided for each day. Spearman correlation coefficient (ρ) between the spore amount and temperature data sets is enclosed, significant relationships being indicated by ∗.
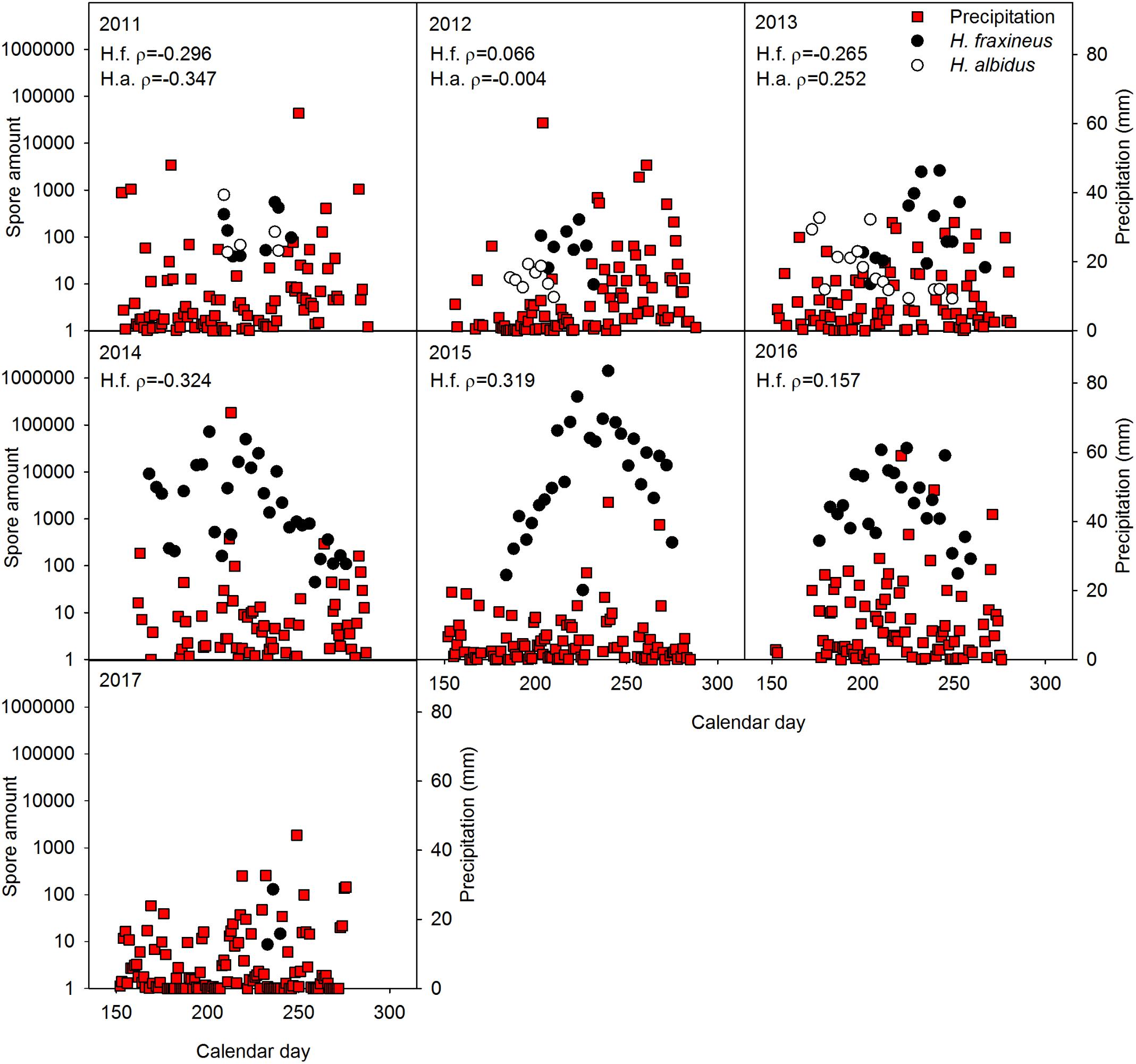
FIGURE 2. The amount of H. fraxineus (H.f.) and H. albidus (H.a.) spores during 2011–2017 in relation to precipitation (in mm). The spore amount is calculated from amount DNA (pg/12 h) in the day period 12 midnight to 12 noon, each data-point constituting a single biological replicate. The spore data represent two randomly chosen days per each sampling week, whereas precipitation data are provided for each day. Spearman correlation coefficient (ρ) between the spore amount and precipitation data sets is enclosed, significant relationships being indicated by ∗.
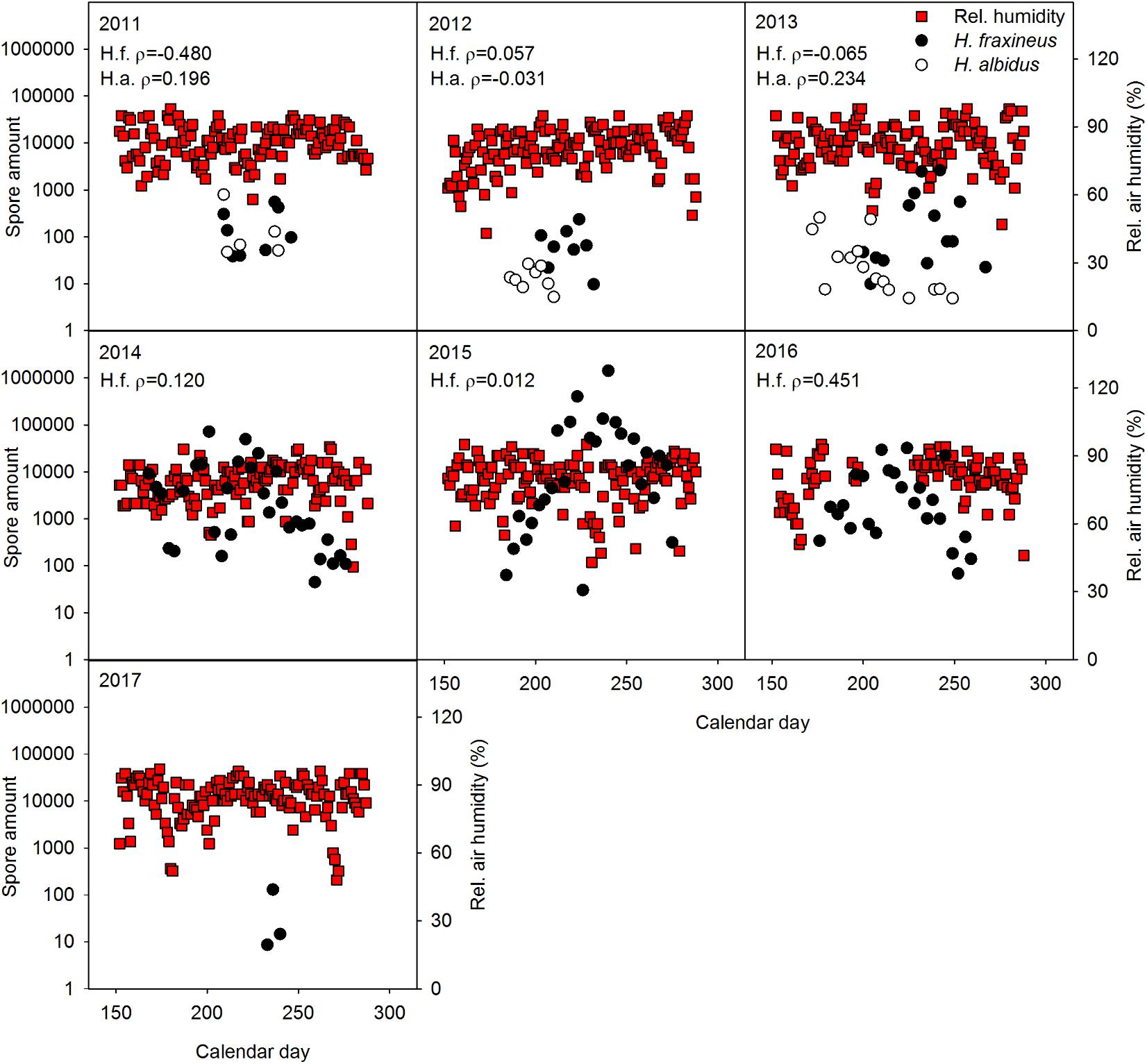
FIGURE 3. The amount of H. fraxineus (H.f.) and H. albidus (H.a.) spores during 2011–2017 in relation to relative air humidity (%). The spore amount is calculated from amount DNA (pg/12 h) in the day period 12 midnight to 12 noon, each data-point constituting a single biological replicate. The spore data represent two randomly chosen days per each sampling week, whereas air humidity data are provided for each day. Spearman correlation coefficient (ρ) between the spore amount and relative air humidity data sets is enclosed, significant relationships being indicated by ∗.
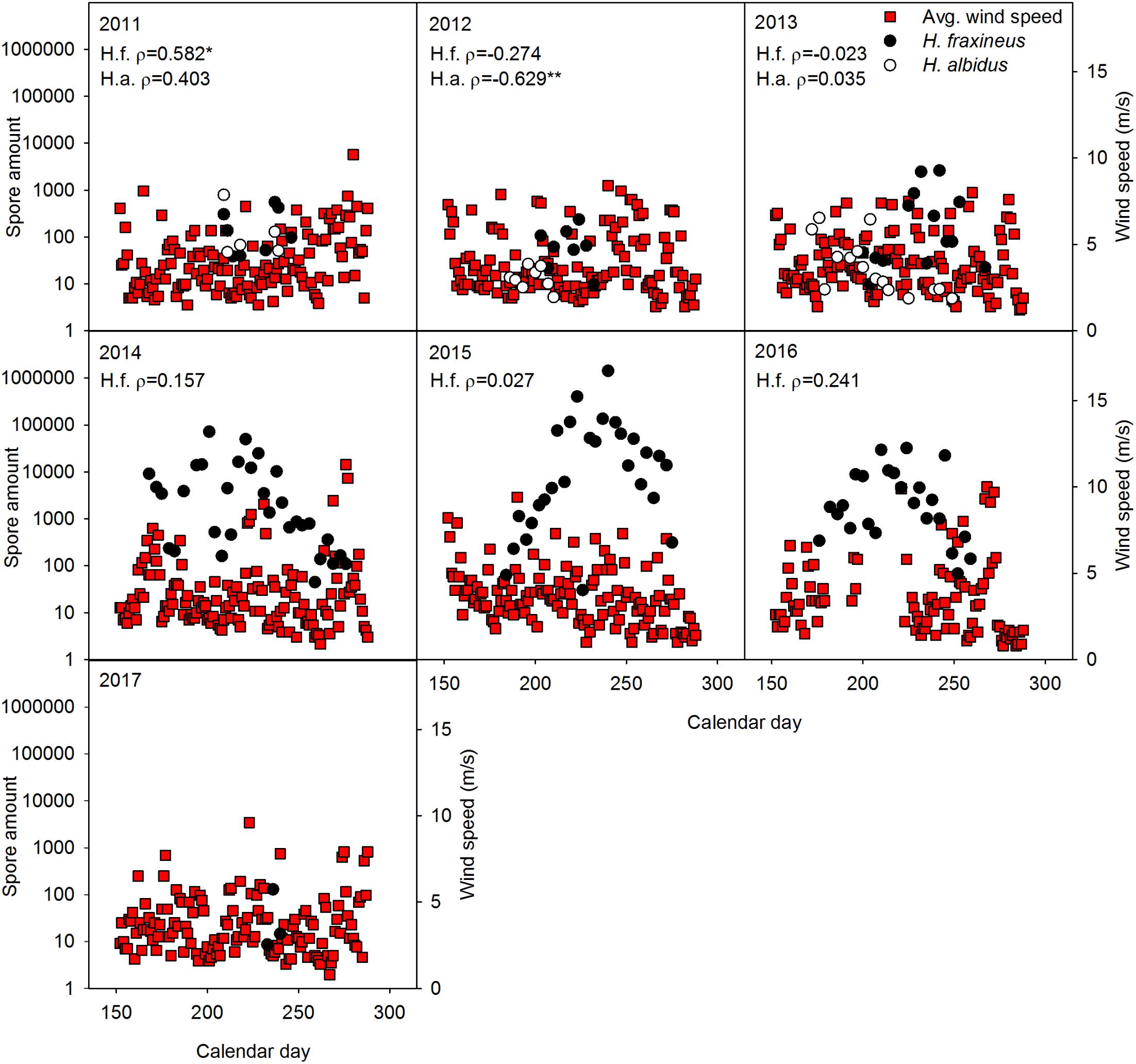
FIGURE 4. The amount of H. fraxineus (H.f.) and H. albidus (H.a.) spores during 2011–2017 in relation to average wind speed (m/s). The spore amount is calculated from amount DNA (pg/12 h) in the day period 12 midnight to 12 noon, each data-point constituting a single biological replicate. The spore data represent two randomly chosen days per each sampling week, whereas wind data is provided for each day. Spearman correlation coefficient (ρ) between the spore amount and average wind speed data sets is enclosed, significant relationships being indicated by ∗.
There were significant relationships between TOP5 spore level of H. fraxineus and the calendar day of first or last spore detection; the corresponding r were −0.85 and 0.86. We also calculated the relationship between fungal sporulation and degree days across the seven-year-long monitoring period, using 5°C (T5), 10°C (T10), or 15°C (T15) as basal temperatures (Figure 5). Since H. albidus was detected only between 2011 and 2013, this calculation was done only for H. fraxineus. The r between TOP1 spore level of H. fraxineus and sum of degree days by that calendar day were −0.29 (T5), −0.12 (T10) and 0.76 (T15), the latter being statistically significant. The r between the sum of degree days by August 31st and TOP5 spore level of H. fraxineus in the sampling year were 0.86 (T5), 0.87 (T10) and 0.82 (T15), all statistically significant. Since ascospore production in a given year is obviously influenced by the success of leaf infection during the previous year, we also calculated the relationship between the degree day sum by August 31st during the previous year and TOP5 spore level of H. fraxineus during the following year (i.e., degree day sum in 2010 vs. spore level in 2011); the corresponding r were 0.88 (T5), 0.90 (T10) and 0.92 (T15), all statistically significant.
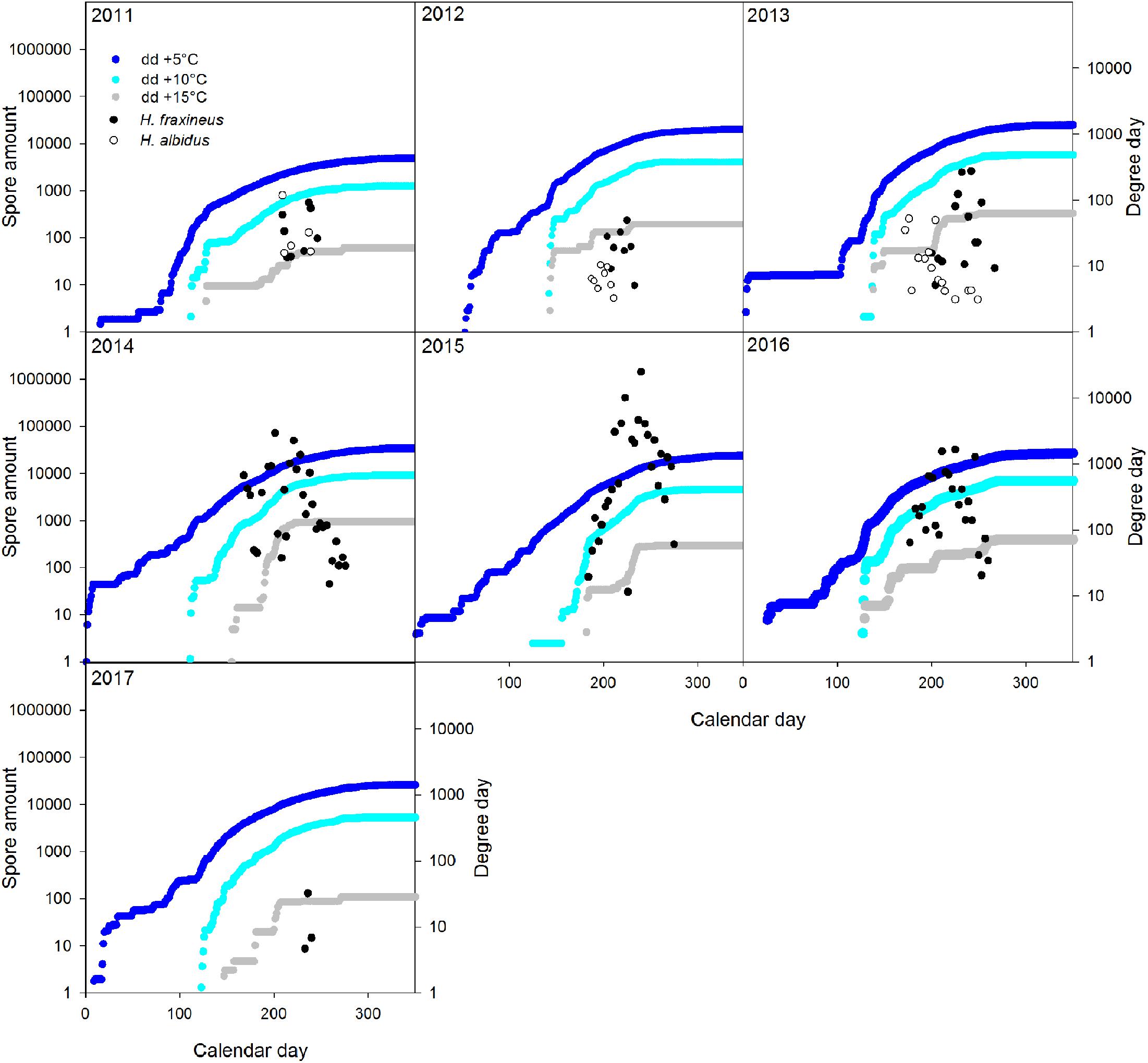
FIGURE 5. Accumulated degree days and amount of H. fraxineus and H. albidus spores detected 2011–2017. 5°C, 10°C or 15°C were used as basal temperature in degree day calculation. For the spores, each data-point constitutes a single biological replicate.
Discussion
The infection pressure by ascospores of H. albidus and H. fraxineus was comparable and low during the first year of monitoring. The noted level of H. albidus inoculum is consistent with the few records of its ascomata in Europe, the fungus being regarded as a relatively rare species (Baral and Bemmann, 2014). The lack of detection of H. albidus spores after 2013 suggests that the fungus was outcompeted by H. fraxineus, which showed an exponential increase in maximum spore level between 2012 and 2015. Studies from Denmark (Mckinney et al., 2012) and UK (King and Webber, 2016) also reported that H. albidus has become rare and probably even locally extinct due, whereas spore trapping studies from the Czech Republic, though based only on one year of sampling, imply that H. albidus could persist even after several years of competition by H. fraxineus (Dvorak et al., 2016; Koukol et al., 2016). For H. albidus detection, these Czech studies, like us, employed the primer/probe set designed by Husson et al. (2012). Whether the contrasting result could be influenced by study-specific differences in real-time PCR parameters (annealing temperature 65°C in the study of Husson et al. (2012) and the present work, and 60°C in the studies by Dvorak et al., 2016 and Koukol et al., 2016) is not clear to us. In Central Europe, first fruiting bodies of H. fraxineus appear already in May–June, distinctly earlier than H. albidus, and early initiation of sporulation has been proposed to provide an advantage to the invader (Baral and Bemmann, 2014). In the current stand sporulation of H. fraxineus initiated in 2012 and 2013 clearly later than that of H. albidus. This inconsistency between the present study and observations from central Europe relates presumably to different climatic conditions. Our ash stand represents oceanic climate with average daily temperatures normally between 13 and 14°C in summer, this being well below the optimum growth temperature that is close to 20–22°C for H. fraxineus (Kowalski and Bartnik, 2010; Pham et al., 2013) but also for H. albidus (Isabella Børja, pers. comm.). Taken together, the observations of fruiting body formation by H. albidus and H. fraxineus in central Europe and the sporulation data recorded now suggest that the order of sporulation initiation by H. albidus and H. fraxineus is not a decisive factor in their competition.
The exponential increase in maximum spore level of H. fraxineus between 2012 and 2015 is typical to invasive species, which may increase in population size according to their biotic potential in a new environment where access to resources is not a growth-limiting factor (e.g., Arim et al., 2006). Based on experiments under defined laboratory conditions, carpogenic germination of fungal pseudosclerotia to form fruiting bodies requires sufficient moisture, cumulative chill-hours and degree-days (e.g., Scherm et al., 2001). All these factors presumably influence also carpogenic germination of H. fraxineus pseudosclerotia but controlled experiments to determine the relations, to the best of our knowledge, have not yet been carried out. The region of the current stand is characterized by mild winters with average temperature around 1°C, high level of precipitation throughout the year and cool summers with average temperatures around 13–14°C in June, July and August. The significant correlation between annual maximum spore levels and degree-day sums suggest that in oceanic climate, cool summer temperatures are presumably the most limiting factor when it comes to carpogenic germination of H. fraxineus pseudosclerotia. An opposite scenario has been proposed for southern France, where high summer temperatures presumably restrict dissemination of H. fraxineus (Grosdidier et al., 2018). However, it is challenging to use field data to model the relationship between weather parameters and annual changes in species population size, especially during early stand infestation. At this phase invasive species may increase in population size according to their biotic potential (e.g., Arim et al., 2006), which makes distinguishing between inherent increase in population size and influence of weather on infection success and rate of carpogenic germination of pseudosclerotia difficult. For example, the maximum spore level of H. fraxineus was recorded in 2015, which was temperature-wise a typical summer in the region, with average temperature of 13.3°C in July which is usually the warmest month in the area. The previous summer was exceptionally warm, with average temperature of 17.8°C in July, and it seems plausible that besides the biotic potential of H. fraxineus to increase in population size, also the warm 2014 summer facilitated success of leaf infection and thereby contributed to the high level of spore production in 2015.
The drastic decline in sporulation level of H. fraxineus after 2015 resembles a population crash that takes place when a population overshoots the carrying capacity of the environment. Considering the increased defoliation of ash trees during the experimental period, the carrying capacity for H. fraxineus might have been reduced in the stand along with the degree of defoliation. Results from two of our ash dieback monitoring plots at the Norwegian west coast with a time series from 2012 have shown rapidly increasing damage and mortality for young and intermediate ash trees, while disease development in the large, dominant trees has been progressing slowly (Timmermann et al., 2017). The monitoring plots are located 110 km south and 40 km north, respectively, and show currently a health condition comparable to that observed in the present stand (Supplementary Figure 1). Regarding precipitation, rainfall can facilitate the deposition of spores on the ground and thereby reduce the amount of spores captured by volumetric air sampling, this wet deposition of spores becoming more important as the distance from spore source increases (Aylor and Sutton, 1992). The record level of precipitation in summer 2016 could have somewhat reduced aerial dispersal distances of H. fraxineus ascospores at the stand. However, the drastic reduction in spore levels of H. fraxineus between 2016 and 2017 can hardly be explained by changes in the carrying capacity alone or by rainfall scavenging of spores – it is likely that the low degree-day sum in June and July in 2016 and especially in 2017 were less favorable to carpogenic germination of H. fraxineus pseudosclerotia. In this context it is highly significant that H. fraxineus can survive several months in air-dried petioles covered with a pseudosclerotial layer (Gross and Holdenrieder, 2013). Further, ascomata of H. fraxineus can be formed not only in the year after leaf fall but also on older petioles, at least up to five growing seasons after the leaves have been shed (Kirisits, 2015). This testifies for a strong competence in defending the saprobic niche against abiotic and biotic stress, an ability that may allow survival of H. fraxineus through unfavorable seasons for ascomata production. Should the summer 2018 turn out to be warm in southwestern Norway, we predict that the spore levels of H. fraxineus increase again in the subjected stand, via carpogenic germination of pseudosclerotia formed during previous years.
According to the stochastic niche theory of resource competition, invasion and community assembly (Tilman, 2004), the probability that an invader can survive, reach maturity and reproduce depends on its traits relative to the traits of the established species. This theory predicts that successful invaders should decrease the abundance mainly of species that are competitively similar to themselves. The genome sequence data indicate that H. albidus and H. fraxineus are very close to each other on the trade-off surface (Stenlid et al., 2017) but the species show some obvious differences in life history traits (e.g., review by Hietala et al., 2018). The largest ascomata recorded for H. fraxineus and H. albidus were 8.5 and 4 mm in diameter, respectively (Baral and Bemmann, 2014). Due to the larger hymenium, H. fraxineus has probably a higher ascospore production than H. albidus. Moreover, in laboratory experiments H. fraxineus shows a considerably larger ascocarp production capacity than H. albidus (Wey et al., 2016), and in forest H. fraxineus can form as many as 10000 ascocarps per m2 (Hietala et al., 2013) or 13 million per hectare (Kowalski et al., 2013). As discussed by Wey et al. (2016), H. albidus may simply have inherently low fecundity, and thereby be at a disadvantage to H. fraxineus. When it comes to adaptation to changes in environmental conditions, a characteristic of invasive species, also in offspring quality H. fraxineus has a clear advantage over H. albidus; while H. fraxineus is an outcrossing heterothallic species with recombinant offspring, H. albidus is homothallic species reproducing via haploid selfing (Gross et al., 2012; Wey et al., 2016; Brasier et al., 2017).
Besides offspring quantity and quality, H. fraxineus differs from H. albidus owing to its extensive pseudosclerotial layers that may cover the entire vein system of the compound leaf, while the pseudosclerotial layer of H. albidus appears usually sharply isolated on the otherwise straw-colored rachis (Baral and Bemmann, 2014). The high investment in survival structures, high fecundity and production of recombinant offspring by H. fraxineus may represent an adaptation to harsh environmental conditions, the native hosts of the fungus spanning across multiple climate zones in Asia, these including regions with cold dry winters and either hot, warm or cold summers (Wei et al., 2004; Peel et al., 2007). To our knowledge, there are no other long-term data available about annual changes in H. fraxineus population size, either from Asia or from Europe. It is noteworthy that in European arboreta the Asian host trees of H. fraxineus support equally well pathogen ascomata formation as common ash (Nielsen et al., 2017). We hypothesize that under Asian climatic conditions H. fraxineus is not able to grow according to its biotic potential over several years and rarely reaches a critical population size when crowding effects become a selective force, whereas the more stable continental climate of Europe enables H. fraxineus to frequently increase in population size over several years, to the effect that the fungus overshoots the environment carrying capacity and causes ash decline. We predict that the boom and bust cycle now observed in H. fraxineus sporulation level is not specific to the subjected stand but a more general phenomenon, and that the length of the exponential increase in H. fraxineus population size depends on how stable the climatic conditions that favor ascocarp sporulation and leaf infection are locally across consequent years. Our results are coherent with the predictions of Goberville et al. (2016), who proposed that climate change will expand the range of H. fraxineus.
Author Contributions
AH, IB, HS, NN, and VT contributed to the design of the project, analysis and interpretation of data, and to drafting and revising of the manuscript. Processing of qPCR data was done by AH. AH and NN conducted the statistical analysis.
Funding
The project was financed by the Norwegian Institute of Bioeconomy Research and the Norwegian forest monitoring program.
Conflict of Interest Statement
The authors declare that the research was conducted in the absence of any commercial or financial relationships that could be construed as a potential conflict of interest.
Acknowledgments
We thank Wibecke Nordstrøm and Hans Nyeggen in Bergen for operating the spore sampler and conducting damage assessments, and Helge Meissner for the processing of real-time PCR assays.
Supplementary Material
The Supplementary Material for this article can be found online at: https://www.frontiersin.org/articles/10.3389/fpls.2018.01087/full#supplementary-material
Footnotes
References
Arim, M., Abdes, S. R., Neill, P. E., Lima, M., and Marquet, P. A. (2006). Spread dynamics of invasive species. Proc. Natl. Acad. Sci. U.S.A. 103, 374–378. doi: 10.1073/pnas.0504272102
Aylor, D. E., and Sutton, T. B. (1992). Release of Venturia inaequalis ascospores during unsteady rain: relationship to spore transport and deposition. Phytopathology 82, 532–540. doi: 10.1094/Phyto-82-532
Baral, H. O., and Bemmann, M. (2014). Hymenoscyphus fraxineus vs. Hymenoscyphus albidus – a comparative light microscopic study on the causal agent of European ash dieback and related foliicolous, stroma-forming species. Mycology 5, 228–290. doi: 10.1080/21501203.2014.963720
Baral, H. O., Queloz, V., and Hosoya, T. (2014). Hymenoscyphus fraxineus, the correct scientific name for the fungus causing ash dieback in Europe. IMA Fungus 5, 79–80. doi: 10.5598/imafungus.2014.05.01.09
Brasier, C. M., King, K. M., Kirisits, T., and Webber, J. (2017). High frequency of vegetative incompatibility combined with haploid selfing in the native european ash foliage coloniser Hymenoscyphus albidus. Fungal Ecol. 28, 11–24. doi: 10.1016/j.funeco.2017.04.002
Chandelier, A., Helson, M., Dvorak, M., and Gischer, F. (2014). Detection and quantification of airborne inoculum of Hymenoscyphus pseudoalbidus using real-time PCR assays. Plant Pathol. 63, 1296–1305. doi: 10.1111/ppa.12218
Colautti, R. I., Grigorovich, I. A., and MacIsaac, H. J. (2006). Propagule pressure: a null model for biological invasions. Biol. Invasions 12, 157–172. doi: 10.1371/journal.pbio.2005987
Cross, H., Sønstebø, J. H., Nagy, N. E., Timmermann, V., Solheim, H., Børja, I., et al. (2017). Fungal diversity and seasonal succession in ash leaves infected by the invasive ascomycete Hymenoscyphus fraxineus. New Phytol. 213, 1405–1417. doi: 10.1111/nph.14204
Drenkhan, R., Solheim, H., Bogacheva, A., Riit, T., Adamson, K., Drenkhan, T., et al. (2017). Hymenoscyphus fraxineus is a leaf pathogen of local Fraxinus species in the Russian far East. Plant Pathol. 66, 490–500. doi: 10.1111/ppa.12588
Dvorak, M., Rotkova, G., and Botella, L. (2016). Detection of airborne inoculum of Hymenoscyphus fraxineus and H. albidus during seasonal fluctuations associated with absence of apothecia. Forests 7:7010001.
Eichhorn, J., Roskams, P., Potočić, N., Timmermann, V., Ferretti, M., Mues, V., et al. (2016). “Part IV: visual assessment of crown condition and damaging agents,” in Manual on Methods and Criteria for Harmonized Sampling, Assessment, Monitoring and Analysis of the Effects of Air Pollution on Forests, ed. UNECE ICP Forests Programme Coordinating Centre (Eberswalde: Thünen Institute of Forest Ecosystems), 54.
Fones, H. N., Mardon, C., and Gurr, S. J. (2016). A role for the asexual spores in infection of Fraxinus excelsior by the ash-dieback fungus Hymenoscyphus fraxineus. Sci. Rep. 6:34638. doi: 10.1038/srep34638
Goberville, E., Hautekeete, N.-C., Kirby, R. R., Piquot, Y., Luczak, C., and Beugrand, G. (2016). Climate change and the ash dieback crisis. Sci. Rep. 6:35303. doi: 10.1038/srep35303
Grosdidier, M., Ioos, R., and Marçais, B. (2018). Do higher summer temperatures restrict the dissemination of Hymenoscyphus fraxineus in France? For. Path. doi: 10.1111/efp.12426
Gross, A., and Holdenrieder, O. (2013). On the longevity of Hymenoscyphus pseudoalbidus in petioles of Fraxinus excelsior. For. Pathol. 43, 168–170. doi: 10.1111/efp.12022
Gross, A., Holdenrieder, O., Pautasso, M., Queloz, V., and Sieber, T. N. (2014). Hymenoscyphus pseudoalbidus, the causal agent of European ash dieback. Mol. Plant Pathol. 15, 5–21. doi: 10.1111/mpp.12073
Gross, A., Zaffarano, P. L., Duo, A., and Grünig, C. R. (2012). Reproductive mode and life cycle of the ash dieback pathogen Hymenoscyphus pseudoalbidus. Fungal Genet. Biol. 49, 977–986. doi: 10.1016/j.fgb.2012.08.008
Hamelin, F. M., Castella, F., Doli, V., Marçais, B., Ravigné, V., and Lewis, M. A. (2016). Mate finding, sexual spore production, and the spread of fungal plant parasites. Bull. Math. Biol. 78, 695–712. doi: 10.1007/s11538-016-0157-1
Hietala, A. M., Børja, I., Cross, H., Nagy, N. E., Solheim, H., and Timmermann, V. (2018). “Dieback of European ash: what can We learn from the microbial community and species-specific traits of endophytic fungi associated with ash?,” in eds A. M. Pirttilä and C. Frank (Basel: Springer International Publishing).
Hietala, A. M., Timmermann, V., Børja, I., and Solheim, H. (2013). The invasive ash dieback pathogen Hymenoscyphus pseudoalbidus exerts maximal infection pressure prior to the onset of host leaf senescence. Fungal Ecol. 6, 302–308. doi: 10.1016/j.funeco.2013.03.008
Husson, C., Caël, O., Grandjean, J. P., Nageleisen, L. M., and Marçais, B. (2012). Occurrence of Hymenoscyphus pseudoalbidus on infected ash logs. Plant Pathol. 61, 889–895. doi: 10.1111/j.1365-3059.2011.02578.x
Husson, C., Scala, B., Caël, O., Frey, P., Feau, N., Ioos, R., et al. (2011). Chalara fraxinea is an invasive pathogen in France. Eur. J. Plant Pathol. 130, 311–324. doi: 10.1007/s10658-011-9755-9
Ioos, R., Kowalski, T., Husson, C., and Holdenrieder, O. (2009). Rapid in planta detection of Chalara fraxinea by a real-time PCR assay using a dual-labelled probe. Eur. J. Plant Pathol. 125, 329–335. doi: 10.1007/s10658-009-9471-x
King, K. M., and Webber, J. F. (2016). Development of a multiplex PCR assay to discriminate native Hymenoscyphus albidus and introduced Hymenoscyphus fraxineus in Britain and assess their distribution. Fungal Ecol. 23, 79–85. doi: 10.1016/j.funeco.2016.06.002
Kirisits, T. (2015). Ascocarp formation of Hymenoscyphus fraxineus on several-year-old pseudo-sclerotial leaf rachises of Fraxinus excelsior. For. Pathol. 45, 254–257. doi: 10.1111/efp.12183
Koukol, O., Haňáčková, Z., Dvořák, M., and Havrdova, L. (2016). Unseen, but still present in Czechia: Hymenoscyphus albidus detected by real-time PCR, but not by intensive sampling. Mycol. Prog. 15:6. doi: 10.1007/s11557-015-1149-5
Kowalski, T. (2006). Chalara fraxinea sp. nov. associated with dieback of ash (Fraxinus excelsior) in Poland. For. Pathol. 36, 264–270. doi: 10.1111/j.1439-0329.2006.00453.x
Kowalski, T., and Bartnik, C. (2010). Morphological variation in colonies of Chalara fraxinea isolated from ash (Fraxinus excelsior L.) stems with symptoms of dieback and effects of temperature on colony growth and structure. Acta Agrobot. 63, 99–106. doi: 10.5586/aa.2010.012
Kowalski, T., Białobrzeski, M., and Ostafiñska, A. (2013). The occurrence of Hymenoscyphus pseudoalbidus apothecia in the leaf litter of Fraxinus excelsior stands with ash dieback symptoms in southern Poland. Acta Mycol. 48, 135–146. doi: 10.5586/am.2013.031
Lockwood, J. L., Cassey, P., and Blackburn, T. (2005). The role of propagule pressure in explaining biological invasions. Trends Ecol. Evol. 20, 223–228. doi: 10.1016/j.tree.2005.02.004
Mckinney, L., Thomsen, I. M., Kjaer, E., Bengtsson, S. B. K., and Nielsen, L. (2012). Rapid invasion by an aggressive pathogenic fungus (Hymenoscyphus pseudoalbidus) replaces a native decomposer (Hymenoscyphus albidus): a case of local cryptic extinction? Fungal Ecol. 5, 663–669. doi: 10.1016/j.funeco.2012.05.004
McKinney, L. V., Nielsen, L. R., Collinge, D. B., Thomsen, I. M., Hansen, J. K., Kjær, E. D., et al. (2014). The ash dieback crisis: genetic variation in resistance can prove a long-term solution. Plant Pathol. 63, 485–499. doi: 10.1111/ppa.12196
McMullan, M., Rafiqi, M., Kaithakottil, G., Clavijo, B. J., Bilham, L., Orton, E., et al. (2018). The ash dieback invasion of Europe was founded by two genetically divergent individuals. Nature Ecol. Evol. 2, 1000–1008. doi: 10.1038/s41559-018-0548-9
Mitchell, R. J., Beaton, J. K., Bellamy, P. E., Broome, A., Chetcuti, J., Eaton, S., et al. (2014). Ash dieback in the UK: a review of the ecological and conservation implications and potential management options. Biol. Conserv. 175, 95–109. doi: 10.1016/j.biocon.2014.04.019
Nielsen, L. R., McKinney, L. V., Hietala, A. M., and Kjær, E. D. (2017). The susceptibility of Asian, European and North American Fraxinus species to the ash dieback pathogen Hymenoscyphus fraxineus reflects their phylogenetic history. Eur. J. For. Res. 136, 59–73. doi: 10.1007/s10342-016-1009-0
Pautasso, M., Aas, G., Queloz, V., and Holdenrieder, O. (2013). European ash (Fraxinus excelsior) dieback – a conservation biology challenge. Biol. Conserv. 158, 37–49. doi: 10.1016/j.biocon.2012.08.026
Peel, M. C., Finlayson, B. L., and McMahon, T. A. (2007). Updated world map of the Köppen-Geiger climate classification. Hydrol. Earth Syst. Sci. 11, 1633–1644. doi: 10.5194/hess-11-1633-2007
Pham, T., Zaspel, I., Schuemann, M., Stephanowitz, H., and Krause, E. (2013). Rapid in-vivo and in-vitro detection of Chalara fraxinea by means of mass spectrometric techniques. Am. J. Plant Sci. 4, 444–453. doi: 10.4236/ajps.2013.42A057
Przybył, K. (2002). Fungi associated with necrotic apical parts of Fraxinus excelsior shoots. For. Pathol. 32, 387–394. doi: 10.1046/j.1439-0329.2002.00301.x
Queloz, V., Grunig, C. R., Berndt, R., Kowalski, T., Sieber, T. N., and Holdenrieder, O. (2011). Cryptic speciation in Hymenoscyphus albidus. For. Pathol. 41, 133–142. doi: 10.1111/j.1439-0329.2010.00645.x
Sansford, C. E. (2013). Pest Risk Analysis for Hymenoscyphus pseudoalbidus (Anamorph Chalara fraxinea) for the UK and the Republic of Ireland. Accra: Forestry Commission, 128.
Scherm, H., Savelle, A. T., and Pusey, P. L. (2001). Interactions between chill-hours and degree-days affect carpogenic germination in Monilia vaccinia-corymbosi. Phytopathology 91, 77–83. doi: 10.1094/PHYTO.2001.91.1.77
Solheim, H., and Hietala, A. M. (2017). Spread of ash dieback in Norway. Baltic For. 23, 144–149. doi: 10.1038/s41559-018-0548-9
Sønstebø, J. H., Vivian-Smith, A., Adamson, K., Drenkhan, R., Solheim, H., and Hietala, A. M. (2017). Genome-wide population diversity in Hymenoscyphus fraxineus points to an eastern Russian origin of European Ash dieback. bioRxiv [Preprint]. doi: 10.1101/154492
Steinböck, S. (2013). Ash Dieback Caused by Hymenoscyphus pseudoalbidus in Norway: Phenology and Etiology of Leaf Symptoms and Ascospore Dispersal Distances. Master thesis: University of Natural Resources and Life Sciences, Vienna.
Stenlid, J., Elfstrand, M., Cleary, M., Ihrmark, K., Karlsson, M., Davydenko, K., et al. (2017). Genomes of Hymenoscyphus fraxineus and Hymenoscyphus albidus encode surprisingly large cell wall degrading potential, balancing saprotrophic and necrotrophic signatures. Baltic For. 23, 41–51.
Tilman, D. (2004). A stochastic theory of resource competition, community assembly and invasions. Proc. Natl. Acad. Sci. U.S.A. 101, 10854–10861. doi: 10.1073/pnas.0403458101
Timmermann, V., Børja, I., Hietala, A. M., Kirisits, T., and Solheim, H. (2011). Ash dieback: pathogen spread and diurnal patterns of ascospore dispersal, with special emphasis on Norway. EPPO Bull. 41, 14–20. doi: 10.1111/j.1365-2338.2010.02429.x
Timmermann, V., Nagy, N. E., Hietala, A. M., Børja, I., and Solheim, H. (2017). Progression of ash dieback in Norway related to tree age, disease history and regional aspects. Baltic For. 23, 150–158.
Wei, X., Reardon, D., Wu, Y., and Sun, J. H. (2004). Emerald ash borer, Agrilus planipennis Fairmaire (Coleoptera: Buprestidae), in China: a review and distribution survey. Acta Entomol. Sin. 47, 679–685. doi: 10.1146/annurev-ento-011613-162051
Keywords: ash dieback, climate, invasive species, population size, r/K selection
Citation: Hietala AM, Børja I, Solheim H, Nagy NE and Timmermann V (2018) Propagule Pressure Build-Up by the Invasive Hymenoscyphus fraxineus Following Its Introduction to an Ash Forest Inhabited by the Native Hymenoscyphus albidus. Front. Plant Sci. 9:1087. doi: 10.3389/fpls.2018.01087
Received: 30 April 2018; Accepted: 05 July 2018;
Published: 30 July 2018.
Edited by:
Aikaterini Dounavi, Forstliche Versuchs- und Forschungsanstalt Baden-Württemberg, GermanyReviewed by:
Miloň Dvořák, Mendel University in Brno, CzechiaDimitrios N. Avtzis, Hellenic Agricultural Organization – ELGO, Greece
Copyright © 2018 Hietala, Børja, Solheim, Nagy and Timmermann. This is an open-access article distributed under the terms of the Creative Commons Attribution License (CC BY). The use, distribution or reproduction in other forums is permitted, provided the original author(s) and the copyright owner(s) are credited and that the original publication in this journal is cited, in accordance with accepted academic practice. No use, distribution or reproduction is permitted which does not comply with these terms.
*Correspondence: Ari M. Hietala, YXJpLmhpZXRhbGFAbmliaW8ubm8=