- 1Linking Landscape, Environment, Agriculture and Food (LEAF), School of Agriculture, University of Lisbon, Lisbon, Portugal
- 2Plant Functional Biology Centre, Biosystems and Integrative Sciences Institute, University of Minho, Braga, Portugal
- 3Instituto Nacional de Investigação Agrária e Veterinária, Oeiras, Portugal
The most discriminating characteristic between the cultivated Vitis vinifera subsp. vinifera and the wild-form Vitis vinifera subsp. sylvestris is their sexual system. Flowers of cultivars are mainly hermaphroditic, whereas wild plants have female and male individuals whose flowers follow a hermaphroditic pattern during early stages of development and later develop non-functional reproductive organs. In angiosperms, the basic developmental system for floral organ identity is explained by the ABCDE model. This model postulates that regulatory gene functions work in a combinatorial way to confer organ identity in each whorl. In wild Vitis nothing is known about the function and expression profile of these genes. Here we show an overall view of the temporal and spatial expression pattern of the ABCDE genes as well as the pattern of VviSUPERMAN that establishes a boundary between the stamen and the carpel whorls, in the male, female and complete flower types. The results show a similar pattern in Vitis species suggesting that the pathway leading to unisexuality acts independently and/or downstream of B- and C- function genes.
Introduction
Flower organ development is under the control of highly specialized genetic networks that have been well studied during the past decades (Wellmer and Riechmann, 2010; O’Maoileidigh et al., 2014). The genus Vitis presents a notorious variability of flower types. The flowers of the cultivated V. v. vinifera are mainly hermaphrodite, while the wild type, V. v. sylvestris is dioecious with male plants producing flowers with erect stamens but without pistils and female plants displaying flowers with a fully formed pistil but reflexed stamens with infertile pollen (Valleau, 1916; Carmona et al., 2008; Ramos et al., 2014). The shift in sexual system from dioecy to hermaphroditism in Vitis species is not yet completely understood.
Several attempts were made to understand and provide insight into the molecular mechanism regarding the origins of this sexual dimorphism present in V. v. sylvestris individuals. Several genetic mapping studies based on the 8x version of the Vitis genome1 annotation (Dalbó et al., 2000; Riaz et al., 2006; Marguerit et al., 2009) located a locus responsible for sex determination at the vicinity of the genetic markers VviMD34 and VviIB23 (Dalbó et al., 2000; Riaz et al., 2006) on chromosome 2 in the 8x version2. Using these markers putatively linked to the sex locus, a new genetic map was developed and refined to restrict the sex locus to 143 kb in the chromosome 2, between 4,907,434 and 5,050,616 bp (Fechter et al., 2012). A more recent study, focusing on the 143 kb region of chromosome 2 extended the sex locus region to 158 kb downstream of the genetic marker VviIB23 and encompassing the previous 143 kb region (Picq et al., 2014). This new locus showed haplotype diversity, linkage disequilibrium, and several genes segregating to typically associated X-Y sex determining region.
The canonical hermaphrodite flower structure can be divided into four whorls. First and second whorls comprise the sterile perianth of the flower, containing sepals and petals, respectively. Reproductive organs are formed in the innermost whorls, the stamens in the third whorl and carpel in the flower center, the fourth whorl (Dellaporta and Calderon-Urrea, 1993; Bowman et al., 2012). The first genes involved in flower organ identity were described in the model plants Antirrhinum majus and Arabidopsis thaliana (Coen and Meyerowitz, 1991). Functional analysis of these genes allowed the postulation of the ABC model that assumes that three classes of proteins act in a combinatorial way to confer organ identity in each whorl. In A. thaliana, the A- class homeotic genes APETALA1 (AP1) and APETALA2 (AP2) specify sepal identity and in combination with the B- class genes, APETALA3 (AP3) and PISTILLATA (PI), specify petal identity. When B- is combined with C- class, conferred by the gene AGAMOUS (AG), stamen identity is specified, whereas C- class alone specifies carpel identity and floral determinacy (Coen and Meyerowitz, 1991). Later, the ABC model was expanded and classes D and E were included. The D- class genes, SEEDSTICK (STK) and SHATTERPROOF (SHP) 1 and 2, are required for ovule identity within the carpel (Favaro et al., 2003; Pinyopich et al., 2003). The E- class genes SEPALLATA (SEP) act redundantly in the specification of sepals, petals, stamens, carpels and ovules by participating in complexes with the A, B, C, and D proteins (Pelaz et al., 2000; Ditta et al., 2004; Castillejo et al., 2005).
Previous studies of flower development genes in grapevine were based on the identification and functional analysis of Vitis vinifera subsp. vinifera homologous of the corresponding ABCDE Arabidopsis genes (Boss et al., 2001, 2002; Calonje et al., 2004; Sreekantan et al., 2006; Poupin et al., 2007). The grapevine AP1 homologous gene, VviAP1, is expressed during flower development, becoming excluded from the sepal-forming region, being preferentially expressed in the growing petals, stamens, and carpels (Calonje et al., 2004). The A- class homeotic gene AP2 is the only non-MADS box gene that acts as a negative regulator of AG, as observed in ap2 Arabidopsis mutant flowers in which sepals are replaced by carpels (Yant et al., 2010). In the grapevine genome there are several genes encoding AP2/ERF proteins expressed in both vegetative and reproductive tissues at different developmental stages (Licausi et al., 2010).
Several studies have shown that the expression of VviPI and VviAP3 in the cultivated Vitis is consistent with PI and AP3 expression in A. thaliana, being restricted to the petal and stamen whorls (Poupin et al., 2007; Díaz-Riquelme et al., 2009). In the hermaphrodite grapevine VviTM6, the homolog of TOMATO MADS BOX GENE 6 (TM6), a gene closely related to AP3 (Kramer and Irish, 2000), is expressed during flower organ identity in the three inner whorls as well as during fruit growth and ripening (Díaz-Riquelme et al., 2009).
In cultivated grapevine the expression pattern of the E- class VviSEP1 and VviSEP3 genes, is similar to the Arabidopsis counterparts. VviSEP1 is expressed in all floral whorls whereas VviSEP3 expression is only excluded from sepal whorl (Boss et al., 2002).
The ABCDE model genes have been long considered as candidate genes for sex determination in monoecious and dioecious species. In the dioecious species Spinacia oleracea, with unisexual flowers (Sather et al., 2010), the expression of B- class floral identity genes is absent in female flowers whereas in male flowers the genes are strongly expressed (Pfent et al., 2005). In male plants, SpPI-silencing originates normal female flowers, indicating that sexual dimorphism occurs through the regulation of B- class gene expression that, by suppressing the formation of the gynoecium act as masculinizing genes (Sather et al., 2010). Contrary to Spinacia, in the dioecious Silene latifolia, unisexuality arises by organ abortion (Hardenack et al., 1994). The expression patterns of SlM2 and SlM3, the PI and AP3 homologous genes, differ during male and female flower organogenesis. At early flower development stages SlM2 and SlM3 are exclusively expressed in petal and stamen primordia, both in male and in female flower meristems (Hardenack et al., 1994). However, during the late stages of female flower development, SlM2 is not expressed in the primordial of the aborted stamen (Kazama et al., 2005). In the dioecious Rumex acetosa, C- class transcripts were detected in the third and fourth whorls of young male and female flowers. However, in male flowers the expression in the carpel whorl is transient, disappearing from the arrested fourth-whorl, while in female flowers the expression is retained in the carpel but is absent from the stamen primordia (Ainsworth et al., 1995, 2005).
Another important gene acting during flower development is SUPERMAN (SUP), necessary to the establishment of the boundaries between third and fourth whorl. SUP is thought to coordinate the proliferation of stamen and carpel specific meristematic cells, keeping the proper structure of the whorls and maintaining the boundary between whorl 3 and whorl 4 at the right position (Sakai et al., 2000). The SUP homolog of S. latifolia, SlSUPERMAN (SlSUP), shows gender-specific expression. SlSUP is a female flower specific gene, expressed in the second and third whorls and in the ovules, suggesting that SlSUP has a positive role in female flower development (Kazama et al., 2009). Also in Cucumis sativus, a SUP ortholog (CsSUP) is predominantly expressed in female organs, and absent in male flowers (Zhao et al., 2014), suggesting a conserved role in flower organ determination.
In female and male flowers of wild Vitis vinifera, the involvement of homeotic genes in the establishment of dioecy or flower type specification has not yet been deeply analyzed. RNA-seq and qRT-PCR analysis during the inflorescence development of male and female plants of the dioecious species (V. v. sylvestris) and the hermaphrodite plants of V. v. vinifera, showed that there was no significant differences in the overall levels of ABCDE gene expression that could account for the specification of the different flower types (Ramos et al., 2014). However, it is possible that rather than differences in the overall level of expression of these genes, a distinct spatial pattern of expression in the three flower types during reproductive organ development could be responsible for conferring different flower organ identity. Therefore, in the current work we performed a detailed analysis of the spatial pattern of expression of the ABCDE genes during the development of male, female and hermaphrodite flowers of Vitis. Also, we analyzed the expression profile of VviSUP, to infer whether there was a different establishment of the borders between reproductive whorls in these flowers. We observed the expression profile of the genes analyzed is similar in the three flower types. Therefore, this work provides further evidence to suggest that the ABCDE genes might not be directly involved in sex specification and may act upstream of the pathways leading to organ abortion in Vitis unisexual flowers.
Materials and Methods
Plant Material
Inflorescences from female and male plants of Vitis v. sylvestris and hermaphrodite flowers of Vitis v. vinifera (Touriga Nacional) (Figure 1) were collected from the Portuguese Ampelographic Collection (PRT051), property of Instituto Nacional de Investigação Agrária e Veterinária, in Dois Portos (Lisbon district, Portugal). Inflorescence/floral buds at late stages B to G [according to phenological classification of developmental stage by Baggiolini (1952)] were collected from several male, female and hermaphrodite plants during April and May (Figure 1).
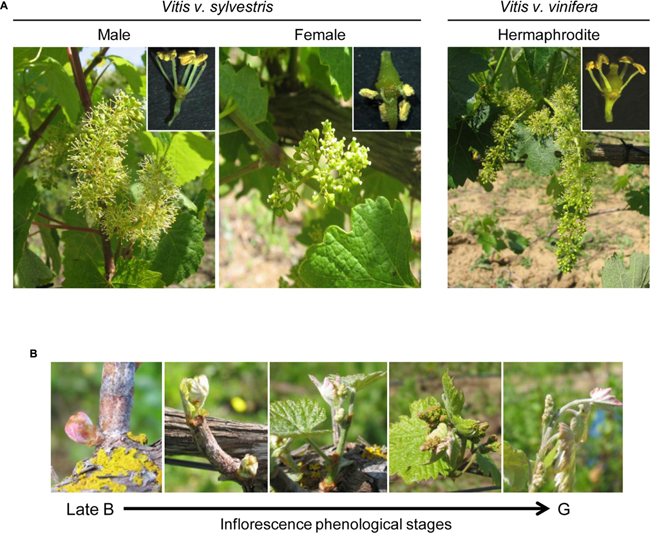
FIGURE 1. Inflorescence morphology in the three Vitis flower types. (A) Morphological disparities between the three flower types found between V. v. sylvestris and V. v. vinifera. Male plants produce a large inflorescence that display flowers with erect stamens but reduced pistil. Female plants produce clusters with few flowers bearing a complete and functional pistil but reflexed stamens with infertile pollen. Hermaphrodite plants produce a large inflorescence with both functional male and female organs. (B) Phenological stages of inflorescence development collected to performed in situ hybridization.
RNA Extraction, cDNA Synthesis and Cloning
Total RNA was extracted from inflorescences using a plant RNA extraction kit, SpectrumTM Plant Total RNA Kit (Sigma-Aldrich, Inc) following the manufacturer’s instructions. cDNA synthesis was performed by reverse transcription with heat denaturation according to the Two Step RT-PCR Procedure of RETROscript Reverse Transcription Kit (Ambion, Life Technologies, Spain). For each sample 100 ng of total RNA were used. cDNA amplification was performed through PCR in 25 μl total volume composed by 1 μg of cDNA, PCR buffer (20 mM Tris–HCl [pH 8.4], 50 mM KCl), 1.5 mM of MgCl2, 0.2 mM of dNTP mix, 0.4 μM of each forward and reverse primers, 5 U of Taq DNA polymerase and autoclaved MiliQ water. The initial 4 min denaturation occurred at 94 C followed by 30 cycles of 45 s at 94°C (denaturation), 45 s at 55°C (annealing), 90 s at 72°C (extension), and a final extension step of 4 min at 72 C. PCR fragments were cloned in the linearized vector pGEM®-T Easy Vector System (Promega, Leiden, Netherlands) according to the manufacturer’s instructions. The extraction of plasmid DNA of Escherichia coli cells was carried out with the PureLink® Quick Plasmid Miniprep Kit (InvitrogenTM, Carlsbad, California) following the manufacturer’s instructions.
Gene Sequence Identification
The protein sequence of AP1, AP2, AP3, PI, AG, SUP, SEP1, and SEP3 from Arabidopsis were retrieved from TAIR3 and TM6 from tomato was retrieved from NCBI4 and blasted (Camacho et al., 2009) against the Vitis database5 using the more recent annotation 12x v2.1. The same approach was used to identify the same genes in the other species.
Phylogenetic analysis of protein sequences was performed with the Maximum Likelihood method through MEGA (Molecular Evolutionary Genetics Analysis) version 6 (Tamura et al., 2013). The bootstrap consensus tree was inferred from 1,000 replicates.
RNA in Situ hybridization
Plant tissue fixation, clearing and in situ hybridization experiments were performed as previously described (Coen et al., 1990; Coito et al., 2017). Primers for riboprobes synthesis used in the in situ hybridization were design using the software Primer Premier 5.0 (Premier Biosoft International) using a primer length of 20 ± 2 bp (Supplementary Table 1). cDNA probes were cloned into the pGEM® T-easy vector system and amplified by PCR with the M13 forward/reverse primers and specific forward/reverse primers (Supplementary Table 1). The PCR product was purified using the MinElute PCR Purification Kit (QIAGEN, Valencia, CA, United States), according to the manufacturer’s instructions, and used as template for the riboprobe synthesis, which was carried with SP6 and T7 RNA polymerase to obtain the sense and antisense strands. The paraffin embedded material was sectioned at 7 μm and the tissue slices mounted with distilled water. Images were captured with a fluorescence microscope (Wild Leitz, Laborlux S) with an AxioCam HRM (Zeiss). Hybridizations were performed at 50°C with the exception of VviAP3 and VviTM6 that were performed with higher stringency at 55°C due to relatively high homology between both probes. All in situ hybridization procedures were made with sense (data not show) and anti-sense probes.
Results and Discussion
The ABCDE model genes are good candidates to be involved in the establishment of male and female flowers in dioecious species, particularly the B- and C- class genes due to their role in reproductive organ identity. Therefore, it is essential to analyze the expression of these genes in a systematic way, regarding the temporal and spatial dynamics throughout flower development in male, female and hermaphrodite Vitis plants.
Phylogenetic Analysis of ABCDE Model Genes in Vitis
Several grapevine genes involved in flower organ identity have been previously reported (Boss et al., 2001, 2002; Calonje et al., 2004; Poupin et al., 2007; Díaz-Riquelme et al., 2009). However, in the Vitis genome database6 there are more than one gene annotated as VviAP1, VviAP2, VviAG, VviSEP1, and VviSEP3 (Supplementary Table 2). Therefore, to make sure we were analyzing the tissue expression pattern during flower development of the right ABCDE homolog, phylogenetic trees were generated (Supplementary Figure 1) with the deduced amino acid sequences for the MADS-box proteins SHP1, STK, AG, PI, AP1, AP3, FRUITFULL (FUL), TM6 and SEP, and as well as the non MADS-box protein AP2 from the following species: Vitis vinifera (Vvi), Arabidopsis thaliana (At) and other dicots plants such as Citrus sinensis (Ci), Cucumis sativus (Cs), Antirrhinum majus (Am), Malus domestica (Md), Petunia hybrida (Ph), Populus trichocarpa (Pt), Prunus persica (Pp), Castanea mollissima (Cm), Solanum lycopersicum (Sl), Pinus radiate (Pr), and the monocot plant Orysa sativa (Os) (Supplementary Table 3). The resulting phylogenetic trees (Supplementary Figure 1A) show that VviAP1 proteins (VIT_201s0011g00100, VIT_214s0083g01030 and VIT_217s0000g04990) are grouped in three clades. In the current work, we have chosen the gene VIT_201s0011g00100 to work with (VviAP1), previously identified as the closest AtAP1 homolog (Calonje et al., 2004). Three VviAP2 (VIT_207s0031g00220, VIT_208s0040g03180 and VIT_213s0019g03550) genes were found in the Vitis genome. Nevertheless, VIT_207s0031g00220 displays higher protein homology with AP2 genes with proven functions (Supplementary Figure 1A) and also, RNA-seq data (flowers from V. v. sylvestris and V. v. vinifera) showed that VIT_207s0031g00220 is expressed in distinct flower developmental stages (Ramos et al., 2014) suggesting that VviAP2 could be a worthy candidate to fulfill the AP2 canonical function.
As far as B- class genes are concerned, the two VviAP3 genes annotated (VIT_218s0001g13460 and VIT_204s0023g02820) were grouped in the AP3 and TM6 clade, respectively (Supplementary Figure 1B). In a previous study VIT_204s0023g02820 was assigned as VviTM6 (Poupin et al., 2007). In order to clarify these annotations, we took a closer look into the VviAP3 genes (VIT_218s0001g13460 and VIT_204s0023g02820). The B- class gene AP3/TM6, form a divergent linage with C-terminal specific motifs. The motif DLTTFALLE define the euAP3 linage present in higher eudicot plants such as Arabidopsis thaliana, Petunia hybrid, and Antirrhinum majus while the motif DLRLA is present in the paleoAP3 linage in lower eudicots, dicots and monocots (Vandenbussche et al., 2003). Comparison of VIT_218s0001g13460 and VIT_204s0023g02820 protein sequences allowed the identification of the DLRLA motif in VIT_204s0023g02820, while VIT_218s0001g13460 shows the euAP3 motif: DLTFTLLE (Supplementary Figure 2). Therefore, in this study we considered VIT_218s0001g13460 gene as VviAP3 and VIT_204s0023g02820 gene as VviTM6 (Poupin et al., 2007). Regarding VviPI (VIT_218s0001g01760), also a B- class gene, falls in the PI clade (Supplementary Figure 1B).
The C- class gene, VviAG (VIT_210s0003g02070), and the D- class genes, VviSHP1 (VIT_212s0142g00360) and VviSTK (VIT_218s0041g01880), belong to the same sub-family, as suggested by a previous work (Becker and Theissen, 2003; Pinyopich et al., 2003). VviSHP1 (VIT_212s0142g00360) present in chromosome 12 was subsequently considered to be the AG homolog (Joly et al., 2004) (Supplementary Figure 1C). In the current work we decided to carry out a detailed analysis of these three protein sequences. The data show that the VviSHP1 protein sequence shares higher similarity with AtSHP2 (82%) than with AtSHP1 (70%) (Supplementary Figures 3A,B). VviAG, is present on chromosome 10 (VIT_210s0003g02070) was chosen, that is the one with highest homology to AtAG.
The genes associated with the E- class, VviSEP1 (VIT_214s0083g01050) and VviSEP3 (VIT_201s0010g03900) are highly related with SEPALLATA homologs of other species, (Supplementary Figure 1D) and were previously described as VviSEP1 and VviSEP3, respectively (Boss et al., 2002; Joly et al., 2004).
Expression of Floral Identity Genes During Vitis Flower Development
A-Class Genes: VviAPETALA1 and VviAPETALA2
In Arabidopsis thaliana, AP1 together with AP2, contributes to sepal and petal identity in the first and second whorls (Theissen, 2001; Krizek and Fletcher, 2005). Defective mutants in AP1 exhibit various defective phenotypes due to the role of this gene in organ and floral meristem identify along with LEAFY (Wagner et al., 1999). ap1 flowers have sepals converted into bracts and additional flowers are formed in the axis of the bracts (Irish and Sussex, 1990) suggesting that AP1 not only specifies the identities of sepals and petals but also determines the identity of floral meristem (Theissen and Saedler, 2001). Studies of eudicot species point to a conservation of the role of the AP1 gene in the floral meristem specification. However, their involvement in the development of perianth organs is unclear (Litt, 2007; Rijpkema et al., 2010). Even in some Arabidopsis ap1 mutants sepals are still formed proving the dubious role of AP1 perianth identity (Bowman et al., 1993; Yu et al., 2004; Castillejo et al., 2005).
In Vitis, expression of VviAP1 occurs early in floral meristem development in the three flower types (Figures 2A–C), in accordance with its potential floral meristem identity role (Mandel et al., 1992). However, when stamens start to develop, VviAP1 expression persists in the center of the flower primordium, instead of being restricted to the first and second whorls (where sepals and petals form) (Figures 2D–F). The VviAP1 expression pattern is similar in the three flower types from early to later flower developmental stages and is detected in petal, stamen and carpel primordia (Figure 2). However, it is almost absent in petals, when they encapsulate the stamens and carpel (Figures 2G–I). The absence of VviAP1 expression in the sepal regions and its expression in the flower center was not expected, considering that in Arabidopsis the expression of AtAP1 is restricted to the sepal and petal whorls and absent from the developing stamens and carpels (Sundstrom et al., 2006). However, the Vitis expression pattern in not completely unforeseen. The expression of an AP1 homolog in the carpel region was also reported for the SQUAMOSA (SQUA) gene in A. majus (Huijser et al., 1992) as well as for the AP1-like gene in Gerbera hybrida (Yu et al., 1999). Also, in grapevine it has been reported the presence of VviAP1 transcripts in the third and fourth whorls (Calonje et al., 2004). AP1 is canonically credited with two functions in flower development: (1) flower meristem initiation and (2) perianth identity (Huijser et al., 1992). However, variability in perianth organs identity exists and seems to be a consequence of a multiple events of evolution within angiosperms, as has been achieved through phylogenetic reconstructions using A- function genes (Zanis et al., 2003; Hileman and Irish, 2009). In the case of Vitis the absence of VviAP1 expression in the flower first whorl reinforce the unclear role of this gene in sepal specification in species other then Arabidopsis.
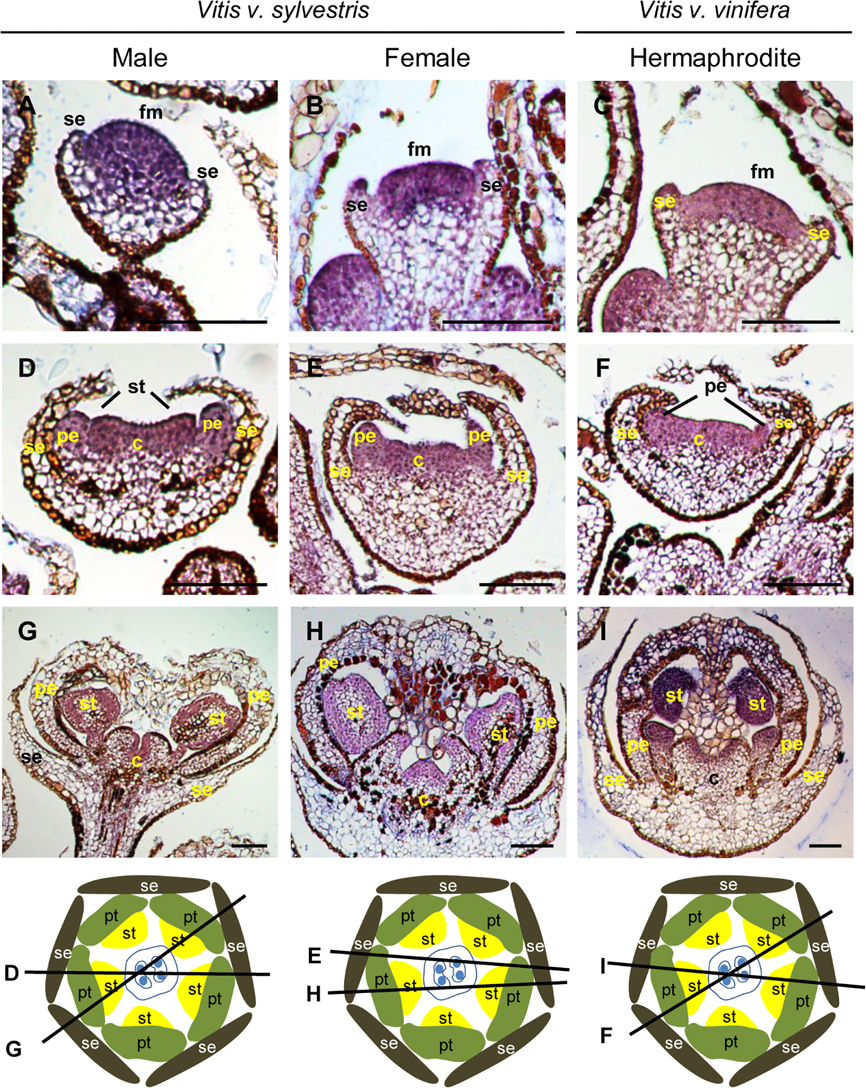
FIGURE 2. In situ hybridization of A- class gene VviAPETALA1 (VviAP1) in developing flowers. VviAP1 (VIT_201s0011g00100) expression analysis on longitudinal sections of wild and hermaphrodite Vitis inflorescences. Male: A,D,G; Female: B,E,H; Hermaphrodite (Her): C,F,I. The expression pattern is similar in the three flower types throughout development and is first detected in the central dome of the flower (A–C) when sepal formation start developing. When petals emerge, VviAP1 remaining in the second, third, and fourth whorl (D–F). At later developmental stages, VviAP1 expression weakens at the petal base but remains in the third and fourth whorl (G–I). The diagrams represent cross-sections with the corresponding figure indicated on each line. Abbreviations are as follows: fm, flower meristem; se, sepals; pe, petals; st, stamens; c, carpel. Scale bar, 100 μm.
In Arabidopsis, AP2 is the other A- class gene that plays a role in specifying sepals and petals (Huala and Sussex, 1992; Jofuku et al., 1994; Husbands et al., 2009). AtAP2 is also expressed in the third and fourth whorls (Jofuku et al., 1994), where it is post-transcriptionally targeted by miRNA172 (Chen, 2004). ap2 flowers have leaf-like structures or carpels instead of sepals and stamens or stamenoid petals instead of petals (Bowman et al., 1989, 1991; Kunst et al., 1989). In Vitis, VviAP2 expression is detected in early flower meristem and becomes excluded from early sepal primordia (Figures 3A–C). The expression of VviAP2 in early flower primordia suggests a conserved role in flower meristem identity (Huala and Sussex, 1992). When petal primordia initiate, VviAP2 is excluded from this whorl (Figures 3D–F) and remains in the third and fourth whorl throughout later stages of flower development. In Arabidopsis, AP2 is expressed in all whorls throughout flower development (Jofuku et al., 1994; Wurschum et al., 2006; Zhao et al., 2007) but more recently, the AP2 expression pattern was reanalyzed reporting a distinct behavior from the one previously described (Wollmann et al., 2010). These authors report that AP2 is expressed in sepals but is absent from the center of flower primordia. Subsequently, AtAP2 mRNA is excluded from the first whorl and appears in stamen and petal primordia. Later, AtAP2 signal remains in petals, stamens and carpels including the ovules (Wollmann et al., 2010). The presence of AtAP2 in the stamens and carpels shows that not only AtAP2 has a role in ovule development (Wollmann et al., 2010) but also that AtAG does not antagonize AtAP2 transcripts in the stamens (Wollmann et al., 2010). To perform this work, Wollmann et al. (2010) used a probe against the 3′ region of the transcript to avoid cross hybridization with other AP2 homologs. In Vitis the full-length VviAP2 transcript was used and it is possible that the detection of mRNA in the dome of the flower meristem is a result of cross hybridization. However, Wollmann et al. (2010) also tested the full-length cDNA of AtAP2 and the accumulation pattern of mRNA was similar with the expression pattern using only a 3′ region RNA probe.
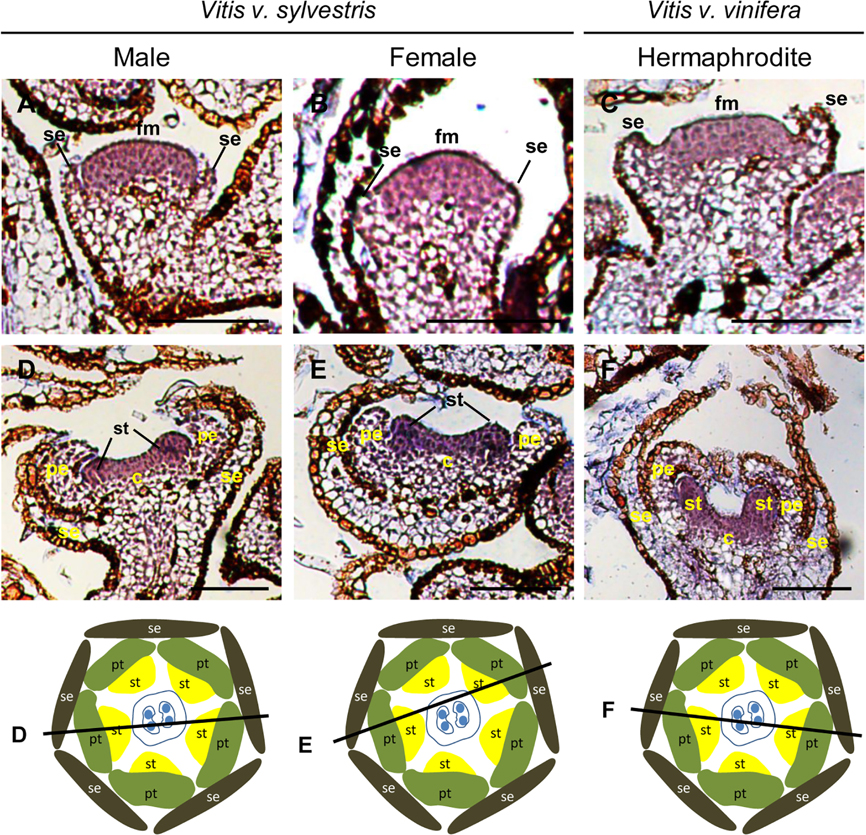
FIGURE 3. In situ hybridization of A- class gene VviAPETALA2 (VviAP2) in developing flowers. VviAP2 (VIT_207s0031g00220) expression analysis on longitudinal sections of wild and hermaphrodite Vitis inflorescences. Male: A,D; Female: B,E; Hermaphrodite (Her): C,F. VviAP2 expression is similar in the three flower types throughout flower development and is first detected in the central dome of the flower meristem (A–C) but absent in the sepal primordia. When petals and stamens start to emerge the expression is restricted to the third and four whorls (D–F) where it remains. The diagrams represent cross-sections with the corresponding figure indicated on each line. Abbreviations are as follows: fm, flower meristem; se, sepals; pe, petals; st, stamens; c, carpel. Scale bar, 100 μm.
In Vitis the expression of VviAP2 suggests (1) that this gene may not be fundamentally necessary for sepal or petal formation; (2) its transient expression during primordia initiation could be the trigger for first and second whorl identities or (3) may be part of a complex set of genes that act redundantly to establish the identity of the first and second whorl. The results obtained in Vitis seem to corroborate the results reported in A. thaliana (Krogan et al., 2012) being suggestive of a role of VviAP2 in floral meristem identify, as its mRNA accumulation is observed early in flower development (Figures 3A–C) and later may be acting synergistically with B- and C- class genes in the third and fourth whorl, respectively, to specify the reproductive organs.
Although AP1 and AP2 show a widespread role in flower meristem initiation, the association regarding their role in organ identity has been troublesome (reviewed in Litt and Kramer, 2010). The results, regarding Vitis AP1 and AP2 contribute to the mystery surrounding the role of A- function genes regarding perianth identity across the core eudicots (Theissen et al., 2000; Maes et al., 2001; Shepard and Purugganan, 2002; Smyth, 2005; Morel et al., 2017) and reinforce the intricacy of these set of genes.
B- Class Genes: VviAPETALA3, VviPISTILLATA, and VviTM6
Early in development of Arabidopsis flowers, PI is expressed in the central dome of the flower meristem (Goto and Meyerowitz, 1994). Later, the expression of AP3/PI is detected in the second and third whorls where the petals and stamens are specified (Bowman et al., 1989). TM6, considered a B-class homeotic gene (Poupin et al., 2007), was first identified in tomato and is expressed in stamens and in carpels (Pnueli et al., 1994; de Martino et al., 2006). AP3/PI are responsible for petal (along with AP2) and stamen (together with AG) identity. Mutations in B- class genes lead to sepaloid structures formed in the second whorl and carpeloid structures in the third whorl (Hill and Lord, 1989; Jack et al., 1992; Wuest et al., 2012). In Arabidopsis, the AP3 gene is expressed only after sepal identity has been established and is confined to the second and third whorl. Even in early stages of development, the AP3 mRNA is not detected in the central region of the flower meristem (Goto and Meyerowitz, 1994). In grapevine, in situ hybridization showed that VviAP3 is expressed in the carpel whorl in the three flower types. In early stages of Vitis flower development, before sepal primordia start to emerge, high levels of VviAP3 were detected in the central region of the flower meristem in the cells that will contribute to the formation of petal, stamen and carpel primordia (Figure 4, Upper panel). When sepal primordia become visible, VviAP3 is still detected in the central dome of the flower primordia (Figures 4A–C). After the emergence of the petal primordia, VviAP3 expression is present in the second and third whorls and its absence from the carpel whorl is inconclusive (Figures 4D–F). This pattern is common to the three flower types (Figures 4G–I). However, previous work, using RT-qPCR, showed that the expression of VvAP3 is restricted to petals and stamens in a hermaphrodite variety of Vitis v. vinifera (Poupin et al., 2007). Due to the sequence similarity between VviAP3 and VviTM6 (Supplementary Figure 2) and in order to avoid cross hybridization with VviTM6, it was necessary to increase the stringency of VviAP3 probe hybridization (see Material and Methods). Nevertheless, we do not rule out the possibility that some signal in the hybridization with the VviAP3 probe may have the contribution from the VviTM6 RNA. In all hybridizations with higher stringency the results were consistent and there is a strong possibility that VviAP3 transcript does not accumulate in the carpel whorl (Figure 4).
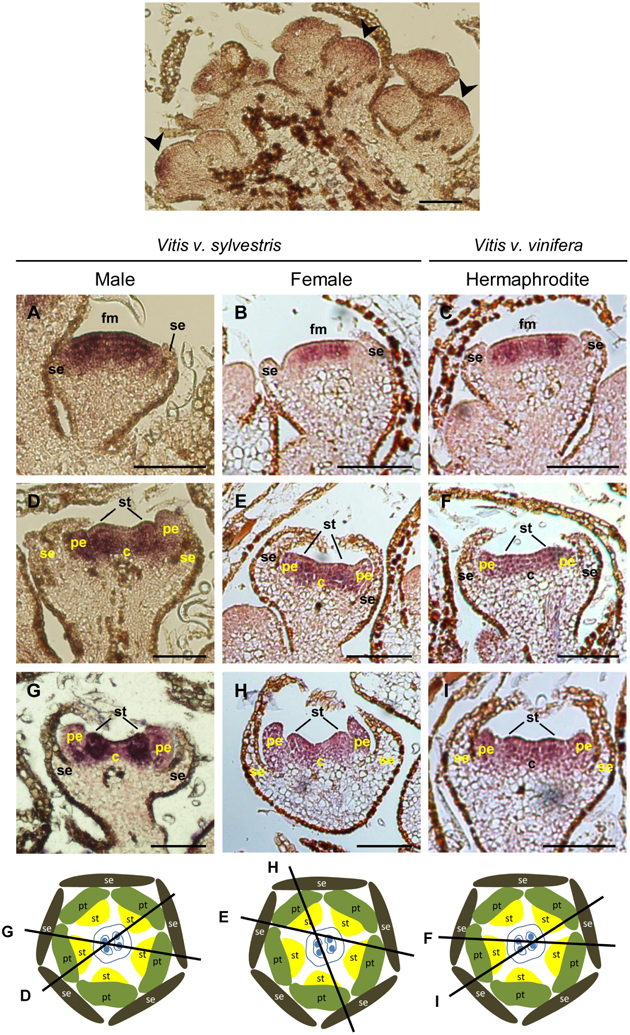
FIGURE 4. In situ hybridization of B- class gene VviAPETALA3 (VviAP3) in developing flowers. VviAP3 (VIT_18s0001g13460) expression analysis on longitudinal sections of wild and hermaphrodite Vitis inflorescences. Upper panel: in early stages of inflorescence development the expression pattern is detected in the central dome of the flower meristem (arrowhead), before sepal primordia emerge, being similar in the three flower types. Male: A,D,G; Female: B,E,H; Hermaphrodite (Her): C,F,I. VviAP3 expression is similar in the three flower types throughout flower development and is first detected in the central dome of the flower meristem (A–C). When petals emerge the expression starts to be restricted to the second and third whorl being excluded from sepals (D–I). The diagrams represent cross sections with the corresponding figure indicated on each line. Abbreviations are as follows: fm, flower meristem; se, sepals; pe, petals; st, stamens; c, carpel. Scale bar, 100 μm.
TM6 is considered a B- class homeotic gene, and was first identified as being expressed in petals, stamens and carpels of L. lycopersicum flowers (Pnueli et al., 1994; de Martino et al., 2006). In Vitis, in early stages of female, male, and hermaphrodite grapevine flower primordia with already emerging sepals, the highest levels of VviTM6 expression was detected in the center of the flower meristem (Figures 5A–C), being excluded from the sepal whorl (Figures 5A–C). After the development of petal and stamen primordia (Figures 5D–F), VviTM6 was confined to the second, third and fourth whorls in the three flower types. VviTM6 seems equally expressed in all three flower types when stamen primordia start emerging (Figures 5G–I), which does not suggest a preferential role in stamen development as seen by the analysis of the TM6 function in tomato (de Martino et al., 2006). As reported in tomato (de Martino et al., 2006), the gene silencing of TM6 by RNAi generates flowers with a compromised stamen development, however, no change in VviTM6 expression was observed between functional stamens of male and reflexed stamens of female flowers in Vitis suggesting that VviTM6 is not involved in stamen abortion. In the Petunia ap3 mutant (that lacks petals and stamens) is complemented with 35S-driven PhTM6, petal development is restored (Rijpkema et al., 2006), suggesting a role in petal development. In grapevine, the presence of VviTM6 mRNA in the three inner whorls points to its participation in the development of petals, stamens, and carpels.
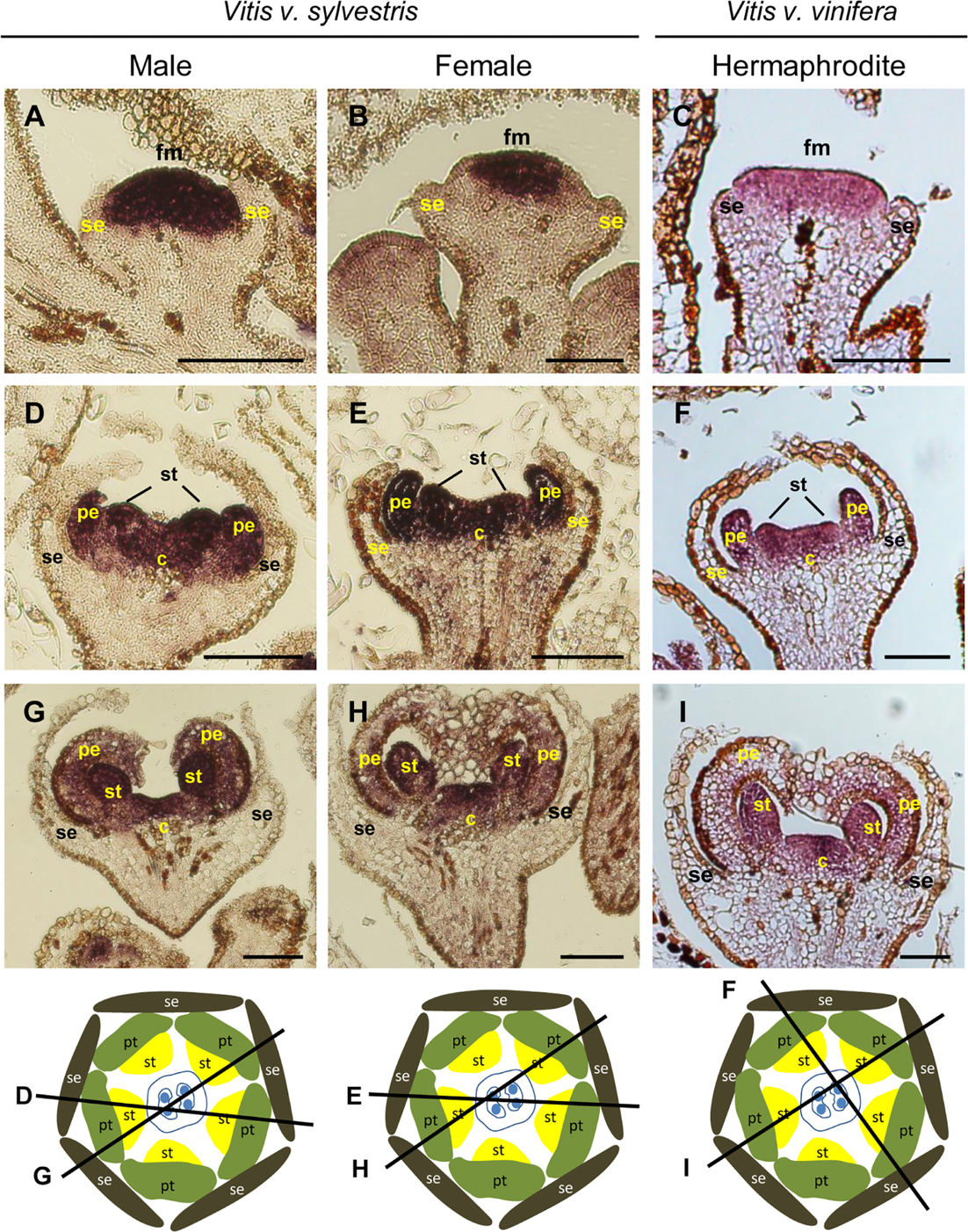
FIGURE 5. In situ hybridization of B- class gene VviTM6 in developing flowers. VviTM6 (VIT_04s0023g02820) expression analysis on longitudinal sections of wild and hermaphrodite Vitis inflorescences. Male: A,D,G; Female: B,E,H; Hermaphrodite (Her): C,F,I. VviTM6 expression is similar in the three flower types throughout flower development and is first detected in the central dome of the flower (A–C). When petals emerge VviTM6 expression is restricted to the second, third and fourth whorls (D–F) where it remains (G–I). The diagrams represent cross sections with the corresponding figure indicated on each line. Abbreviations are as follows: fm, flower meristem; se, sepals; pe, petals; st, stamens; c, carpel. Scale bar, 100 μm.
PISTILLATA in Arabidopsis is expressed in cells that will give rise to petals, stamens, and carpel primordia in early stages of flower development (Hill and Lord, 1989; Sundstrom et al., 2006), and its expression is confined to the second and third whorls only in later stages (Goto and Meyerowitz, 1994). In early stages of Vitis flower development, VviPI is expressed in the center of the flower meristem (Figure 6, Upper panel) similar to what was described for Arabidopsis (Goto and Meyerowitz, 1994). When sepal primordia start to emerge, VviPI has a high expression in the cells that will develop into petals and stamens and starts to fade from the region that will develop into carpels (Figures 6A–C). As soon as petal primordia starts to emerge, VviPI is completely excluded from the fourth whorl (Figures 6D–F) remaining confined to the second and third whorl during the later stages of development (Figures 6G–I). VviPI seems to be more expressed in stamen than in petal primordia, both in male and in female flowers. This expression pattern is similar in female and male flowers and did not follow the pattern of organ abortion founded in Silene lafolia, where at later stages of female flower development PI expression is absent from the aborted stamens (Kazama et al., 2005).
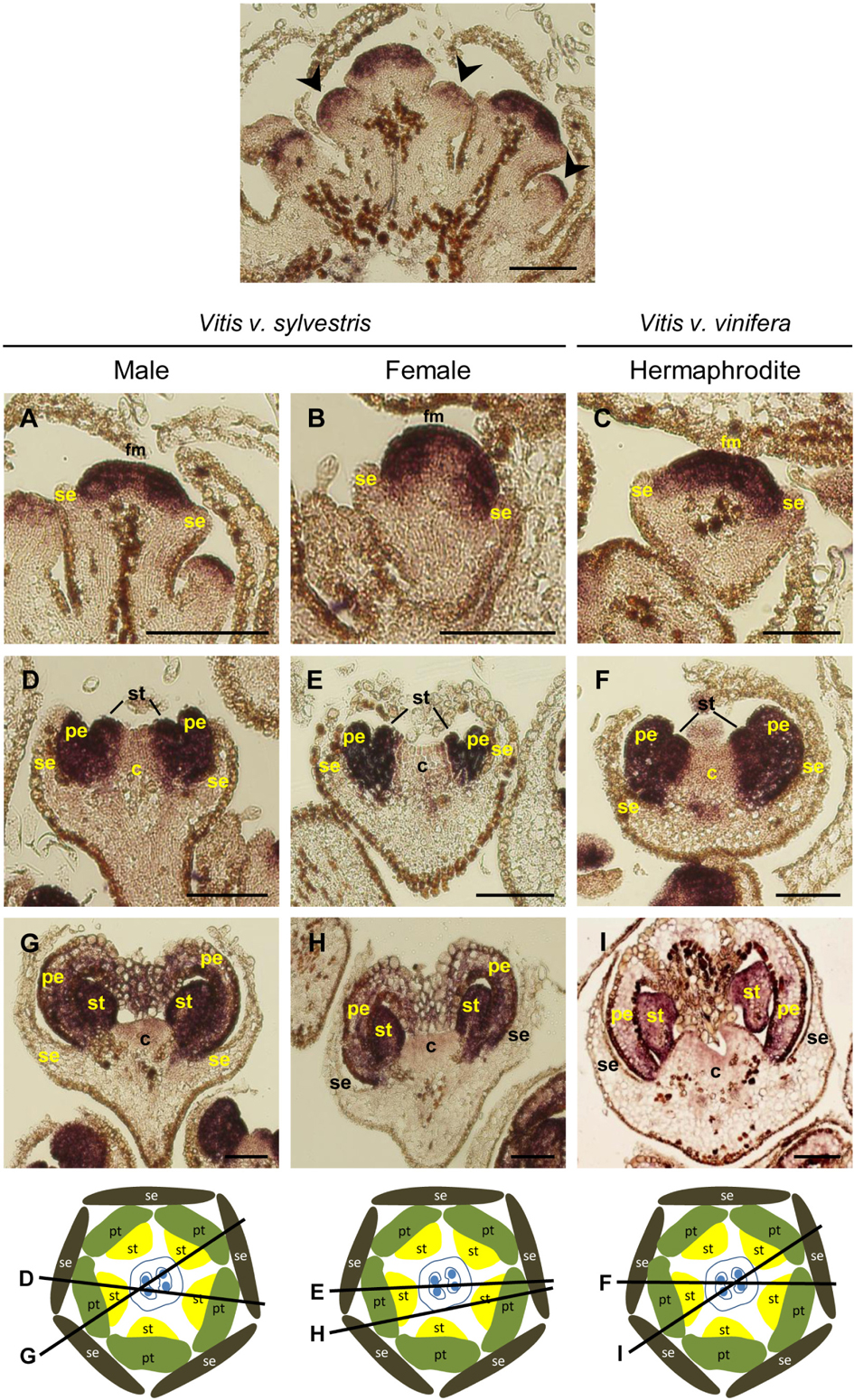
FIGURE 6. In situ hybridization of B- class gene VviPISTILLATA (VviPI) in developing flowers. VviPI (VIT_218s0001g01760) expression analysis on longitudinal sections of wild and hermaphrodite Vitis inflorescences. Upper pane: in early stages of inflorescence development the expression is detected in the central dome of the flower meristem (arrowheads), before sepal primordia emerge and is similar in the three flower types. Male: A,D,G; Female: B,E,H; Hermaphrodite (Her): C,F,I. VviPI expression is similar in the three flower types throughout flower development and is first detected in the central dome of the flower meristem (A–C). When petals start to emerge VviPI is excluded from the first and fourth whorl, but is present in the second and third whorl (D–F) where it remains (G–I). The diagrams represent cross sections with the corresponding figure indicated on each line. Abbreviations are as follows: fm, flower meristem; se, sepals; pe, petals; st, stamens; c, carpel. Scale bar, 100 μm.
Together, the expression of VviPI and VviAP3 B- class genes is similar to what was described for the B- class Arabidopsis homologs (Goto and Meyerowitz, 1994). The early dynamics of both VviAP3 and VviPI (Figures 4, 6) raises questions regarding the molecular mechanism underlying the activation of both genes in the floral meristem in Vitis. Our results suggest that the boundaries of VviAP3 and VviPI are identical during the onset of Vitis flower meristem suggesting, at least in part, that both genes could be under the regulation of identical upstream factors.
The Cadrastral Gene: VviSUPERMAN
The SUP gene establishes the boundaries between stamen and carpel whorls by acting as a cadastral factor restricting the B- class genes expression (Hiratsu et al., 2002). In sup Arabidopsis mutants, the expression of AP3/PI expands to the fourth whorl where, staminoid structures are formed instead of a carpel (Bowman et al., 1991, 1992). SUP acts by inhibiting the expression of AP3 and PI in the fourth whorl of the developing flower (Jack et al., 1992; Sakai et al., 2000). In Vitis inflorescences, the expression of VviSUPERMAN (VviSUP) is similar during flower development of the three flower types (Figure 7). The earliest expression of VviSUP is detected in the central region of the floral meristem adjacent to the boundary between the third and fourth whorls (Figures 7A–C). In later stages of floral development, VviSUP is expressed in the inner region of the developing stamen primordia (Figures 7D–F). When stamen primordia are clearly defined, VviSUP expression remains in the region adjacent to the carpel primordia (Figures 7G–I).
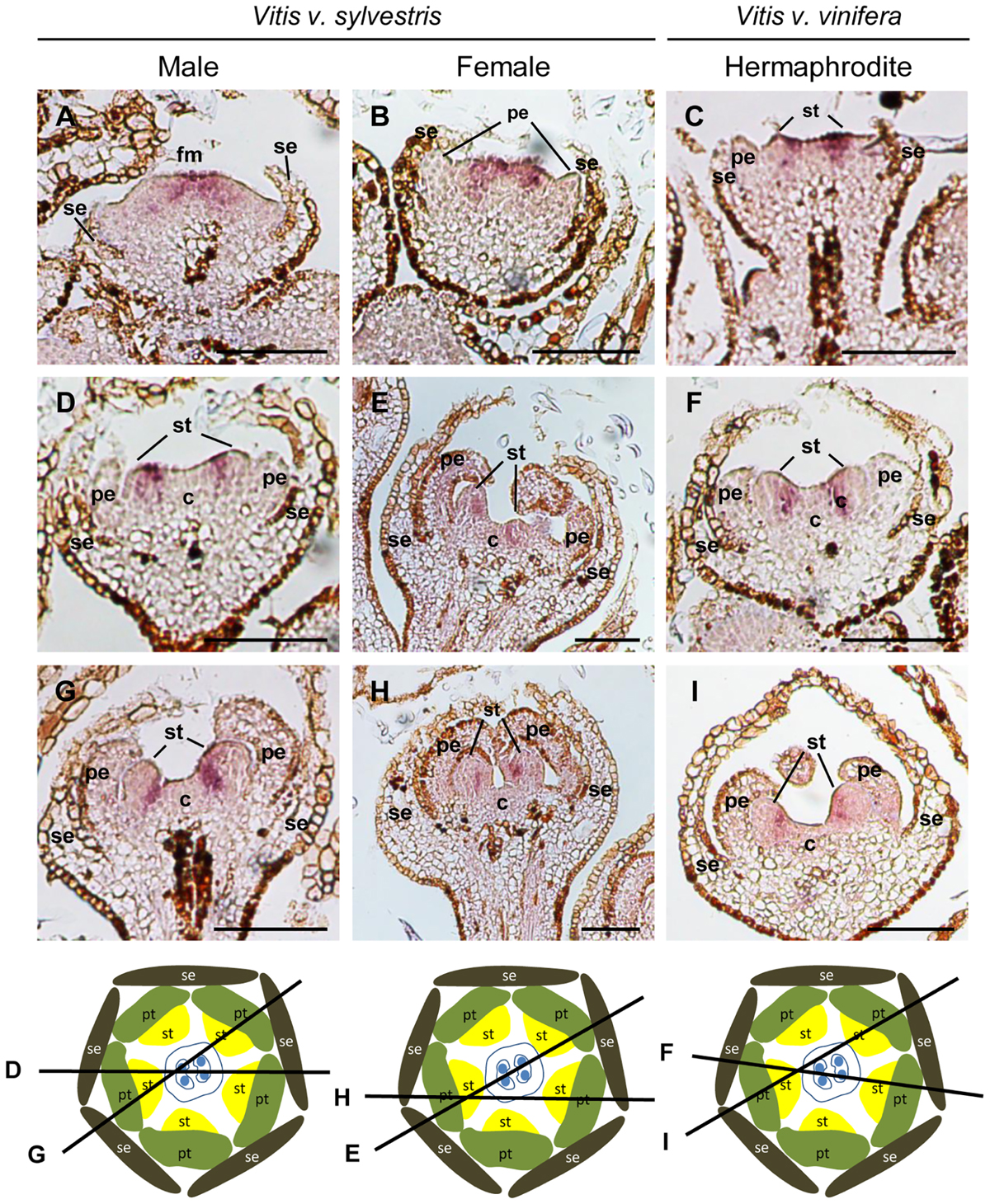
FIGURE 7. In situ hybridization of VviSUPERMAN (VviSUP) in developing flowers. VviSUP (VIT_205s0049g00070) expression analysis on longitudinal sections of wild and hermaphrodite Vitis inflorescences. Male: A,D,G; Female: B,E,H; Hermaphrodite (Her): C,F,I. VviSUP expression is similar in all three flower types throughout flower development. VviSUP is detected in early stages of flower development in the central area of the flower meristem in cells that will define the boundary between third and fourth whorl (A–C). When stamens emerge, VviSUP is well settled out in the cells that divide those two whorls (D–F) where it remains at least until sepals fuse and enclose the flower bud (G–I). The diagrams represent cross sections with the corresponding figure indicated on each line. Abbreviations are as follows: fm, flower meristem; se, sepals; pe, petals; st, stamens; c, carpel. Scale bar, 100 μm.
The in situ results obtained with VviAP3/VviPI and their regulator VviSUP show that VviAP3 expression appears first in the central region of flower meristem and is, in a later stage, followed by VviSUP expression, which goes in agreement with what has been observed in Arabidopsis mutants, in which SUP expression is detect after the initiation of AP3 expression (Sakai et al., 1995).
Our data suggest that early VviSUP expression act in a similar way to what has been described in Arabidopsis, maintaining the whorl 3 and 4 boundary after the whorl prepattern has been established. In Arabidopsis, SUP gene appears to act transiently, only required for a short period before the cells in the floral whorls undergo extensive divisions to produce organ primordia (Sakai et al., 2000), a brief expression that is sufficient to fulfill SUP function in whorl boundary maintenance (Bowman et al., 1991, 1992; Jack et al., 1992). However, in grapevine, the expression of VviSUP persists in the inner part of whorl 3 until later flower developmental stages (Figures 7G–I) suggesting that it may not be the trigger but could be involved in maintain the genetic signaling by controlling the balanced proliferation of two adjacent floral whorls.
C- Class Genes: VviAGAMOUS
AGAMOUS is a C- class homeotic MADS-box gene expressed both in the third whorl, acting together with PI and AP3 in specifying stamen identity, and in the fourth whorl, where it is responsible for carpel identify and, to some degree, ovule identity (Bowman et al., 1989, 1991). Mutant plants for AG do not have reproductive organs, and instead exhibit a phenotype described as “a flower within a flower” with the absence of stamens and carpels (Yanofsky et al., 1990). In Vitis, VviAG expression (VIT_210s0003g02070) follows a temporal and spatial pattern (identical in all the three flower types) that fits with what was proposed by the ABCDE model. VviAG is expressed in the center of the Vitis flower meristem (Figures 8A–C) and is confined to the third and fourth whorls when stamen primordia emerge (Figures 8D–F). This expression pattern is maintained throughout later stages of flower development (Figures 8G–I). A similar expression profile has been reported in the hermaphrodite Riesling variety, in which VviSHP1 is expressed in later stages of flower development (Joly et al., 2004) where it is required to control ovule identity on tissues that develop within the carpels (Pinyopich et al., 2003). In the dioecious Rumex acetosa, AG transcript accumulation decrease in the aborted organs (Ainsworth et al., 1995), however, in grapevine the similar expression profile of VviAG in all flower types (Ramos et al., 2014) may exclude the direct involvement of VviAGAMOUS in carpel abortion or the formation of reflexed stamens.
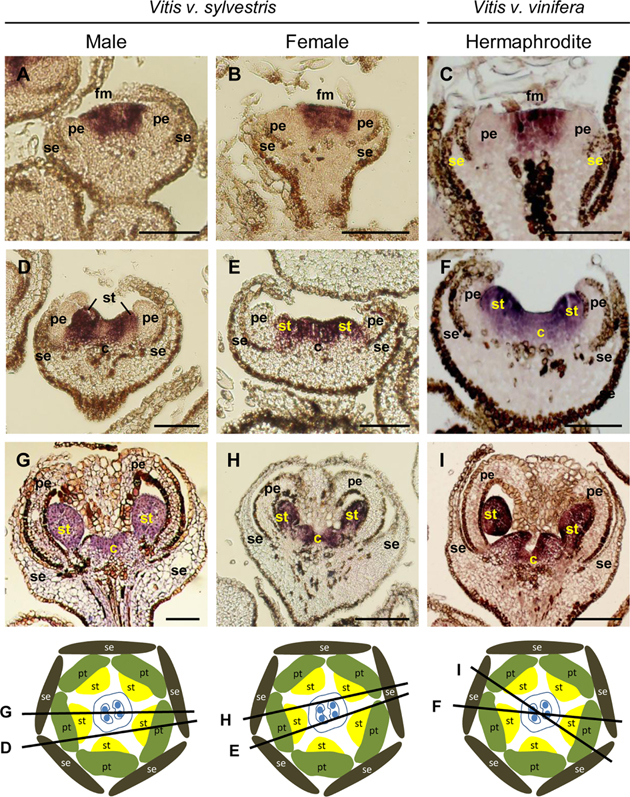
FIGURE 8. In situ hybridization of C- class gene VviAGAMOUS (VviAG) in developing flowers. VviAG (VIT_210s0003g02070) expression analysis on longitudinal sections of wild and hermaphrodite Vitis inflorescences. Male: A,D,G; Female: B,E,H; Hermaphrodite (Her): C,F,I. The location of the VviAG RNA is similar throughout flower development in the three flower types. VviAG is first detected in the central dome of the flower (A–C). When petal primordia emerge (D–F) VviAG is detected in the third and fourth whorls where it remains throughout flower development (G–I). The diagrams represent cross sections with the corresponding figure indicated on each line. Abbreviations are as follows: fm, flower meristem; se, sepals; pe, petals; st, stamens; c, carpel. Scale bar, 100 μm.
E- Class Genes: VviSEPALLATA1 and VviSEPALLATA3
The E- class SEPALLATA (SEP) play a central role in flower meristem determinacy and organ identity (Ditta et al., 2004). The SEP genes, although functionally redundant, are required for the formation of petals, stamens and carpels as the triple mutant (sep 1/2/3) has an indeterminate flower with all flower organs converted into sepals (Pelaz et al., 2000; Honma and Goto, 2001). In A. thaliana, SEP1 is expressed throughout flower development (Savidge et al., 1995). In the three Vitis flower types, VviSEP1 is expressed in the center of the flower meristem (Figures 9A–C), but excluded from the sepal primordia. When petal primordia emerge, VviSEP1 remains in the second, third and fourth whorls (Figures 9D–F) and at later developmental stages, VviSEP1 is still expressed in the third and fourth whorls but has lower expression in the petals (Figures 9G–I).
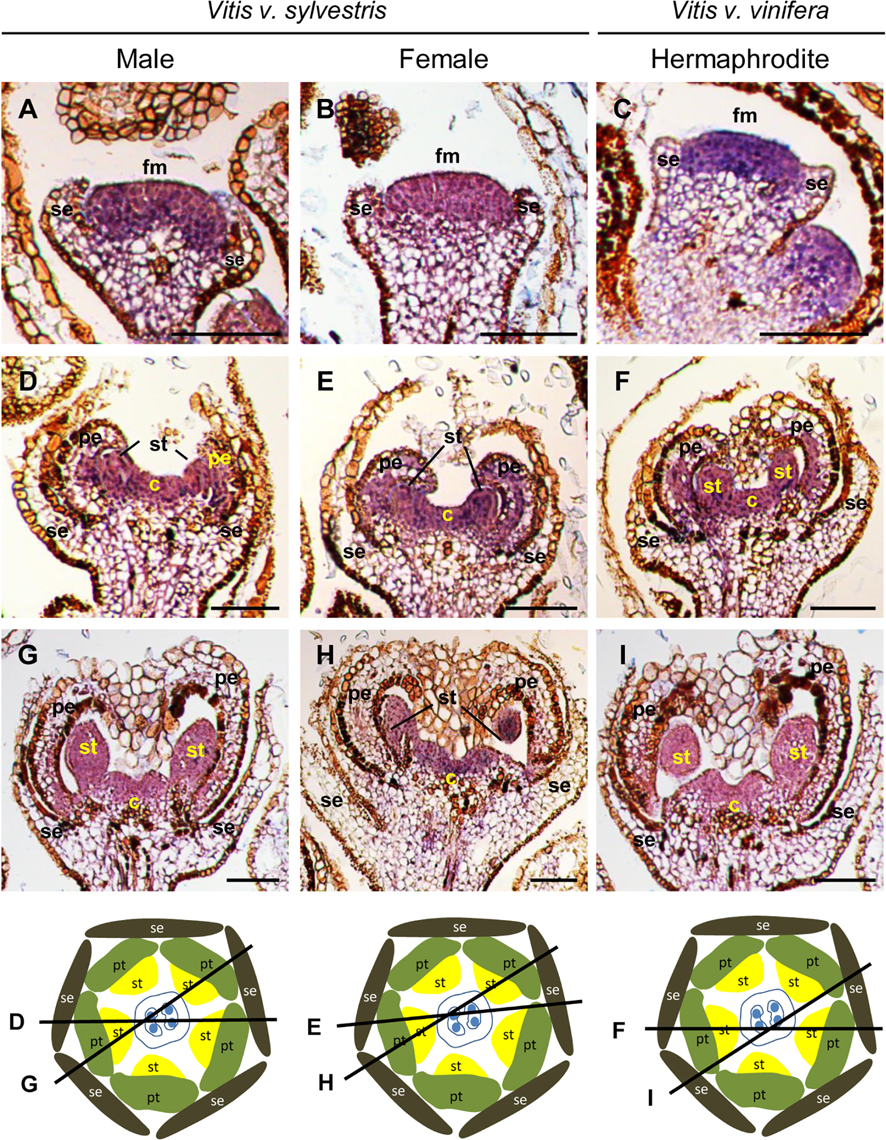
FIGURE 9. In situ hybridization of E- class gene VviSEPALLATA1 (VviSEP1) in developing flowers. VviSEP1 (VIT_214s0083g01050) expression analysis on longitudinal sections of wild and hermaphrodite Vitis inflorescences. Male: A,D,G; Female: B,E,H; Hermaphrodite (Her): C,F,I. VviSEP1 expression is similar in the three flower types throughout flower development and is first detected in the central dome of the flower (A–C). When petals start to develop the expression is restricted to the second, third and four whorls (D–F) where it remains throughout flower development but with lower expression in the second whorl (G–I). The diagrams represent cross sections with the corresponding figure indicated on each line. Abbreviations are as follows: fm, flower meristem; se, sepals; pe, petals; st, stamens; c, carpel. Scale bar, 100 μm.
SEP3 is involved in sepal, petal, stamen, carpel, and ovule development and its ectopic expression is enough to activate AtAP3 and AtAG (Pelaz et al., 2000; Favaro et al., 2003; Ditta et al., 2004). In Vitis, VviSEP3 expression is detected very early in the floral meristem in all flower types (Figures 10A–C). When petal and stamen primordia emerge, VviSEP3 is excluded from sepals (Figures 10D–F). In later stages of flower development, VviSEP3 is weakly expressed in both the base and adaxial side of petals (Figures 10G–I). No differences were found in the expression of VviSEP3 in the three flower types (Figure 10). In Arabidopsis SEP1 is expressed slightly earlier than SEP3, which is expressed in a region corresponding to the inner three whorls just before the initiation of floral primordia (Flanagan and Ma, 1994; Savidge et al., 1995; Ditta et al., 2004). In Vitis, the activities of VviSEP1/3 span the three inner whorls similar to what happens in Arabidopsis (Pelaz et al., 2000). However, and contrarily to what happens in Arabidopsis, our data show that VviSEP1 expression is spatially and temporally very similar to VviSEP3.
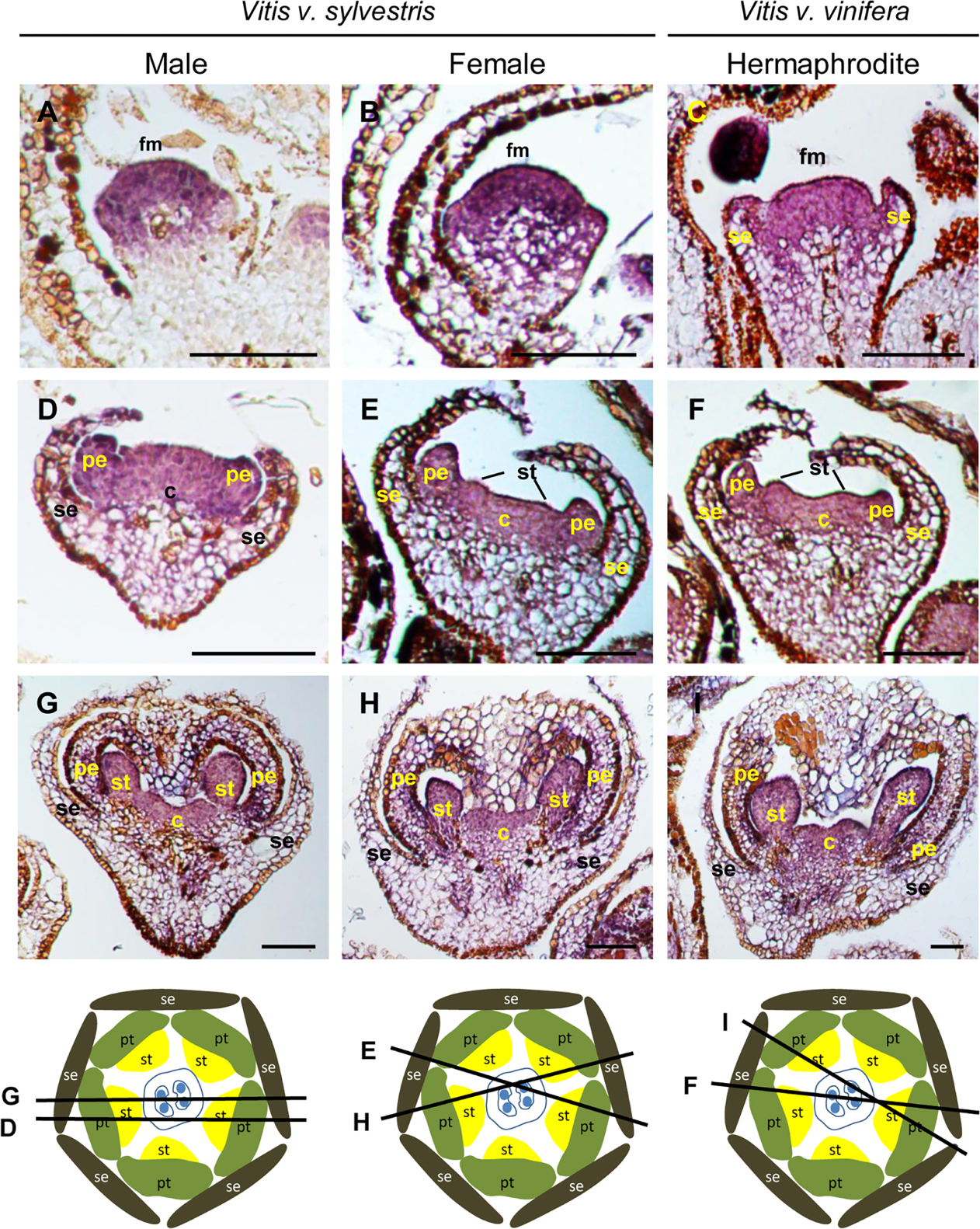
FIGURE 10. In situ hybridization of E- class gene VviSEPALLATA3 (VviSEP3) in developing flowers. VviSEP3 (VIT_01s0010g03900) expression analysis on longitudinal sections of wild and hermaphrodite Vitis inflorescences. Male: A,D,G; Female: B,E,H; Hermaphrodite (Her): C,F,I. VviSEP3 expression is similar in the three flower types throughout flower development. VviSUP3 is first detected in the central dome of the flower (A–C). When petals emerge VviSEP3 expression is excluded from the first whorl (D–F). In later development stages VviSEP3 remains in the second, third and fourth whorls (G–I). The diagrams represent cross sections with the corresponding figure indicated on each line. Abbreviations are as follows: fm, flower meristem; se, sepals; pe, petals; st, stamens; c, carpel. Scale bar, 100 μm.
Conclusion
The results of the present study reinforce the great complexity of the events and molecular cascades that occur during determination and specification of floral organ identity in Vitis. Previous results (RNA-seq and qRT-PCR) showed similar levels of ABCDE gene expression in male, female and hermaphrodite Vitis flowers (Ramos et al., 2014), suggesting that these genes may not be directly involved in sex specification in this species. However, similar levels of expression can be produced by different patterns of tissue expression and, therefore, there was still a possibility that these ABCDE genes could mediate the specification of the three different Vitis flower types.
Despite some slight differences, the homeotic genes exhibit a spatial expression profile similar in the three flower types and analogous to what has been described for Arabidopsis homologs. Additionally, none of these genes fall into the region of chromosome 2 responsible for sex determination (Fechter et al., 2014; Picq et al., 2014; Coito et al., 2017; Zhou et al., 2017). Moreover, our results show that despite these genes being involved in flower organ identity they are not directly responsible for flower type specification in Vitis. One outcome that emerges from this work concerns the identity of sepals (Figure 11A). The homeotic genes, VviAP1 and VviAP2, have no expression in the first whorl where they are supposed to regulate the formation of sepals (Figure 11A). This unexpected absence of expression raises two hypotheses: these genes may not be involved in sepal identity specification in this species or the structure known as sepals has, in fact, an identity different from canonical sepals. Additionally, their expression in flower development at initial stages also point to a role in flower meristem identity.
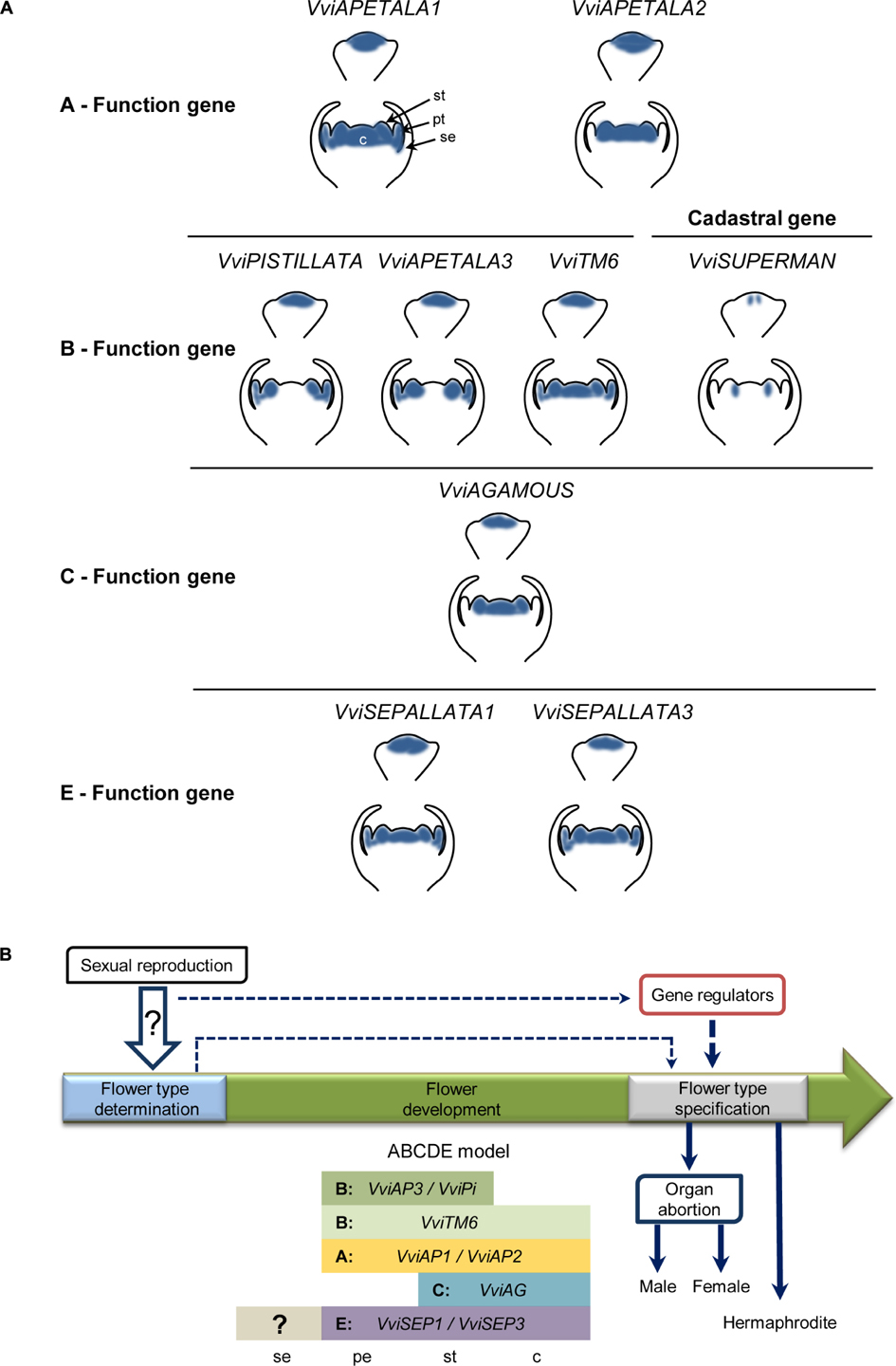
FIGURE 11. Homeotic genes expression in Vitis and their role in flower sex development. (A) Diagrams of flowering genes expression. Expression pattern of the ABCDE model genes and the cadastral gene VviSUPERMAN. Homeotic genes VviAPETALA (VviAP1) and VviAP2 (VviAP2) from the A- function have no expression in the first whorl of sepals. They are expressed in petals, stamens and carpel primordia. The genes VviAPETALA (VviAP3) and VviPISTILATA (VviPI) from the B- function form petals and stamens in the second and third whorl. VviSUPERMAN restricts B- function expression genes in flower meristems in different flower developmental stages of V. v. vinifera and a V. v. sylvestris. VviAGAMOUS (VviAG) is the only gene in the C- function responsible for carpel formation in the fourth whorl. Our data suggest that there are no antagonism between A- and C- function in Vitis. E- function VviSEPALLATA (VviSEP) genes act redundantly and are expressed in the three inner whorls and required for the correct formation of all flower organs. (B) Proposed model for flower development. The model suggests that flower determination is established at the moment of reproduction by yet unknown factors. The flower development is regulated through the onset of the ABCDE model genes until a later stage of development, as observed in this work. Downstream of homeotic genes that establish flower organ identity and initiation, other regulators might be involved in flower type specification. These regulators can either (I) act under the control of the initial unknown sexual determination factors or (II) the initial unknown sexual determination factors are directly involved in flower specification into male and female. Both scenarios can be exclusively or act together promoting organ abortion in V. v. sylvestris or a hermaphrodite flower development in V.v. vinifera. se, sepals; pe, petals; st, stamens; c, carpel.
A model for flower development, shown in Figure 11B, proposes that flower determination is established at the time of fertilization by factors still unknown (Figure 11B) present in autosomal chromosomes. The subsequent flower organ development may be regulated through the onset of the ABCDE homeotic genes that act after sex determination and upstream of flower organ abortion regulators, such as the already studied gene VviAPRT3 (Coito et al., 2017). These putative regulators can act under the control of the initial unknown sexual determination factors or each of the later may be directly controlling the flower specification promoting the abnormal development of male or female reproductive organs in later stages of flower development.
Both scenarios can be exclusive or act together leading to organ abortion as in V. v. sylvestris flowers or hermaphrodite flowers as in V. v. vinifera.
Author Contributions
MR, JLC, HS, and MMRC conceived and designed the experiments. JLC, HS, MM, and MJNR performed the experiments. HS, JLC, MJNR, MMRC, and MR analyzed the data. HS, JLC, MJNR, SA, MMRC, and MR wrote the paper. JC, JLC, and MR established Vitis vinifera sylvestris collection and collected plant tissues according phenological developmental stage.
Funding
This work was supported by the funded project PTDC/AGR-GPL/119298/2010 from Fundação para a Ciência e Tecnologia (FCT, Portugal), by UID/AGR/04129/2013 centre grant from FCT, Portugal (to LEAF), and by UID/MULTI/04046/2013 centre grant from FCT, Portugal (to BioISI), and JLC, MJNR, MMRC, and MR are supported by FCT fellowships, SFRH/BD/85824/2012, SFRH/BD/110274/2015, SFRH/BSAB/113781/2015, SFRH/BPD/64905/2009, respectively.
Conflict of Interest Statement
The authors declare that the research was conducted in the absence of any commercial or financial relationships that could be construed as a potential conflict of interest.
Acknowledgments
We are also grateful to Eng. Eiras-Dias, curator of the Portuguese Ampelographic Collection (property of Instituto Nacional de Investigação Agrária e Veterinária, Dois Portos) where sampling was performed, for the collaboration in this work allowing the access to the Vitis collection.
Supplementary Material
The Supplementary Material for this article can be found online at: https://www.frontiersin.org/articles/10.3389/fpls.2018.01029/full#supplementary-material
Footnotes
- ^http://www.genoscope.cns.fr/externe/GenomeBrowser/Vitis/entry_ggb.html
- ^http://genomes.cribi.unipd.it/grape/
- ^https://www.arabidopsis.org/
- ^https://www.ncbi.nlm.nih.gov/
- ^http://genomes.cribi.unipd.it/gb2/gbrowse/public/vitis_vinifera_v2/
- ^http://genomes.cribi.unipd.it/grape/
References
Ainsworth, C., Crossley, S., Buchanan-Wollaston, V., Thangavelu, M., and Parker, J. (1995). Male and female flowers of the dioecious plant sorrel show different patterns of MADS box gene expression. Plant Cell 7, 1583–1598. doi: 10.1105/tpc.7.10.1583
Ainsworth, C., Rahman, A., Parker, J., and Edwards, G. (2005). Intersex inflorescences of Rumex acetosa demonstrate that sex determination is unique to each flower. New Phytol. 165, 711–720. doi: 10.1111/j.1469-8137.2005.01281.x
Baggiolini, M. (1952). Les stades repères dans le developpement annuel de la vigne et leur utilisation pratique. Rev. Romande Agric. Vitic. Arbor. 8, 4–6.
Becker, A., and Theissen, G. (2003). The major clades of MADS-box genes and their role in the development and evolution of flowering plants. Mol. Phylogenet. Evol. 29, 464–489. doi: 10.1016/S1055-7903(03)00207-0
Boss, P. K., Sensi, E., Hua, C., Davies, C., and Thomas, M. R. (2002). Cloning and characterisation of grapevine (Vitis vinifera L.) MADS-box genes expressed during inflorescence and berry development. Plant Sci. 162, 887–895. doi: 10.1016/S0168-9452(02)00034-1
Boss, P. K., Vivier, M., Matsumoto, S., Dry, I. B., and Thomas, M. R. (2001). A cDNA from grapevine (Vitis vinifera L.), which shows homology to AGAMOUS and SHATTERPROOF, is not only expressed in flowers but also throughout berry development. Plant Mol. Biol. 45, 541–553. doi: 10.1023/A:1010634132156
Bowman, J. L., Alvarez, J., Weigel, D., Meyerowitz, E. M., and Smyth, D. R. (1993). Control of flower development in Arabidopsis thaliana by Apetala1 and interacting genes. Development 119, 721–743.
Bowman, J. L., Sakai, H., Jack, T., Weigel, D., Mayer, U., and Meyerowitz, E. M. (1992). Superman, a regulator of floral homeotic genes in Arabidopsis. Development 114, 599–615.
Bowman, J. L., Smyth, D. R., and Meyerowitz, E. M. (1989). Genes directing flower development in Arabidopsis. Plant Cell 1, 37–52. doi: 10.1105/tpc.1.1.37
Bowman, J. L., Smyth, D. R., and Meyerowitz, E. M. (1991). Genetic interactions among floral homeotic genes of Arabidopsis. Development 112, 1–20.
Bowman, J. L., Smyth, D. R., and Meyerowitz, E. M. (2012). The ABC model of flower development: then and now. Development 139, 4095–4098. doi: 10.1242/dev.083972
Calonje, M., Cubas, P., Martinez-Zapater, J. M., and Carmona, M. J. (2004). Floral meristem identity genes are expressed during tendril development in grapevine. Plant Physiol. 135, 1491–1501. doi: 10.1104/pp.104.040832
Camacho, C., Coulouris, G., Avagyan, V., Ma, N., Papadopoulos, J., Bealer, K., et al. (2009). BLAST plus : architecture and applications. BMC Bioinformatics 10:421. doi: 10.1186/1471-2105-10-421
Carmona, M. J., Chaib, J., Martinez-Zapater, J. M., and Thomas, M. R. (2008). A molecular genetic perspective of reproductive development in grapevine. J. Exp. Bot. 59, 2579–2596. doi: 10.1093/jxb/ern160
Castillejo, C., Romera-Branchat, M., and Pelaz, S. (2005). A new role of the Arabidopsis SEPALLATA3 gene revealed by its constitutive expression. Plant J. 43, 586–596. doi: 10.1111/j.1365-313X.2005.02476.x
Chen, X. M. (2004). A microRNA as a translational repressor of APETALA2 in Arabidopsis flower development. Science 303, 2022–2025. doi: 10.1126/science.1088060
Coen, E. S., and Meyerowitz, E. M. (1991). The war of the whorls: genetic interactions controlling flower development. Nature 353, 31–37. doi: 10.1038/353031a0
Coen, E. S., Romero, J. M., Doyle, S., Elliot, R., Murphy, G., and Carpenter, R. (1990). Floricaula: a homeotic gene required for flower development in Antirrhinum majus. Cell 63, 1311–1322. doi: 10.1016/0092-8674(90)90426-F
Coito, J. L., Ramos, M., Cunha, J. M. M., Silva, H. G., Amâncio, S., Costa, M. M. R., et al. (2017). VviAPRT3 and VviFSEX: two genes involved in sex specification able to distinguish different flower types in Vitis. Front. Plant Sci. 8:98. doi: 10.3389/fpls.2017.00098
Dalbó, M. A., Ye, G. N., Weeden, N. F., Steinkellner, H., Sefc, K. M., and Reisch, B. I. (2000). A gene controlling sex in grapevines placed on a molecular marker-based genetic map. Genome 43, 333–340. doi: 10.1139/g99-136
de Martino, G., Pan, I., Emmanuel, E., Levy, A., and Irish, V. F. (2006). Functional analyses of two tomato APETALA3 genes demonstrate diversification in their roles in regulating floral development. Plant Cell 18, 1833–1845. doi: 10.1105/tpc.106.042978
Dellaporta, L. S., and Calderon-Urrea, A. (1993). Sex determination in flowering plants. Plant Cell 5, 1241–1251. doi: 10.1105/tpc.5.10.1241
Díaz-Riquelme, J., Lijavetzky, D., Martínez-Zapater, J. M., and Carmona, M. J. (2009). Genome-wide analysis of MIKCC-type MADS box genes in grapevine. Plant Physiol. 149, 354–369. doi: 10.1104/pp.108.131052
Ditta, G., Pinyopich, A., Robles, P., Pelaz, S., and Yanofsky, M. F. (2004). The SEP4 gene of Arabidopsis thaliana functions in floral organ and meristem identity. Curr. Biol. 14, 1935–1940. doi: 10.1016/j.cub.2004.10.028
Favaro, R., Pinyopich, A., Battaglia, R., Kooiker, M., Borghi, L., Ditta, G., et al. (2003). MADS-box protein complexes control carpel and ovule development in Arabidopsis. Plant Cell 15, 2603–2611. doi: 10.1105/tpc.015123
Fechter, I., Hausmann, L., Daum, M., Sorensen, T. R., Viehover, P., Weisshaar, B., et al. (2012). Candidate genes within a 143 kb region of the flower sex locus in Vitis. Mol. Genet. Genomics 287, 247–259. doi: 10.1007/s00438-012-0674-z
Fechter, I., Hausmann, L., Zyprian, E., Daum, M., Holtgrawe, D., Weisshaar, B., et al. (2014). QTL analysis of flowering time and ripening traits suggests an impact of a genomic region on linkage group 1 in Vitis. Theor. Appl. Genet. 127, 1857–1872. doi: 10.1007/s00122-014-2310-2
Flanagan, C. A., and Ma, H. (1994). Spatially and temporally regulated expression of the MADS-box Gene Agl2 in wild-type and mutant Arabidopsis flowers. Plant Mol. Biol. 26, 581–595. doi: 10.1007/BF00013745
Goto, K., and Meyerowitz, E. M. (1994). Function and regulation of the Arabidopsis floral homeotic gene Pistillata. Genes Dev. 8, 1548–1560. doi: 10.1101/gad.8.13.1548
Hardenack, S., Ye, D., Saedler, H., and Grant, S. (1994). Comparison of MADS-box gene expression in developing male and female flowers of the dioecious pant white campion. Plant Cell 6, 1775–1787. doi: 10.1105/tpc.6.12.1775
Hileman, L. C., and Irish, V. F. (2009). More is better: the uses of developmental genetic data to reconstruct perianth evolution. Am. J. Bot. 96, 83–95. doi: 10.3732/ajb.0800066
Hill, J. P., and Lord, E. M. (1989). Floral development in Arabidopsis thaliana - a comparison of the wild-type and the homeotic Pistillata mutant. Can. J. Bot. 67, 2922–2936. doi: 10.1139/b89-375
Hiratsu, K., Ohta, M., Matsui, K., and Ohme-Takagi, M. (2002). The SUPERMAN protein is an active repressor whose carboxy-terminal repression domain is required for the development of normal flowers. FEBS Lett. 514, 351–354. doi: 10.1016/S0014-5793(02)02435-3
Honma, T., and Goto, K. (2001). Complexes of MADS-box proteins are sufficient to convert leaves into floral organs. Nature 409, 525–529. doi: 10.1038/35054083
Huala, E., and Sussex, I. M. (1992). Leafy interacts with floral homeotic genes to regulate Arabidopsis floral development. Plant Cell 4, 901–913. doi: 10.1105/tpc.4.8.901
Huijser, P., Klein, J., Lonnig, W. E., Meijer, H., Saedler, H., and Sommer, H. (1992). Bracteomania, an inflorescence anomaly, is caused by the loss of function of the mads-box gene Squamosa in Antirrhinum majus. EMBO J. 11, 1239–1249.
Husbands, A. Y., Chitwood, D. H., Plavskin, Y., and Timmermans, M. C. P. (2009). Signals and prepatterns: new insights into organ polarity in plants. Genes Dev. 23, 1986–1997. doi: 10.1101/gad.1819909
Irish, V. F., and Sussex, I. M. (1990). Function of the apetala-1 gene during Arabidopsis floral development. Plant Cell 2, 741–753. doi: 10.1105/tpc.2.8.741
Jack, T., Brockman, L. L., and Meyerowitz, E. M. (1992). The homeotic gene Apetala3 of Arabidopsis thaliana encodes a mads box and is expressed in petals and stamens. Cell 68, 683–697. doi: 10.1016/0092-8674(92)90144-2
Jofuku, K. D., Denboer, B. G. W., Vanmontagu, M., and Okamuro, J. K. (1994). Control of Arabidopsis flower and seed development by the homeotic gene Apetala2. Plant Cell 6, 1211–1225. doi: 10.1105/tpc.6.9.1211
Joly, D., Perrin, M., Gertz, C., Kronenberger, J., Demangeat, G., and Masson, J. E. (2004). Expression analysis of flowering genes from seedling-stage to vineyard life of grapevine cv. Riesling. Plant Sci. 166, 1427–1436. doi: 10.1016/j.plantsci.2003.12.041
Kazama, Y., Fujiwara, M. T., Koizumi, A., Nishihara, K., Nishiyama, R., Kifune, E., et al. (2009). A SUPERMAN-like gene is exclusively expressed in female flowers of the dioecious plant Silene latifolia. Plant Cell Physiol. 50, 1127–1141. doi: 10.1093/pcp/pcp064
Kazama, Y., Koizumi, A., Uchida, W., Ageez, A., and Kawano, S. (2005). Expression of the floral B-function gene SLM2 in female flowers of Silene latifolia infected with the smut fungus Microbotryum violaceum. Plant Cell Physiol. 46, 806–811. doi: 10.1093/pcp/pci080
Kramer, E. M., and Irish, V. F. (2000). Evolution of the petal and stamen developmental programs: evidence from comparative studies of the lower eudicots and basal angiosperms. Int. J. Plant Sci. 161, S29–S40. doi: 10.1086/317576
Krizek, B. A., and Fletcher, J. C. (2005). Molecular mechanisms of flower development: an armchair guide. Nat. Rev. Genet. 6, 688–698. doi: 10.1038/nrg1675
Krogan, N. T., Hogan, K., and Long, J. A. (2012). APETALA2 negatively regulates multiple floral organ identity genes in Arabidopsis by recruiting the co-repressor TOPLESS and the histone deacetylase HDA19. Development 139, 4180–4190. doi: 10.1242/dev.085407
Kunst, L., Klenz, J. E., Martinezzapater, J., and Haughn, G. W. (1989). Ap2 gene determines the identity of perianth organs in flowers of Arabidopsis thaliana. Plant Cell 1, 1195–1208. doi: 10.1105/tpc.1.12.1195
Licausi, F., Giorgi, F. M., Zenoni, S., Osti, F., Pezzotti, M., and Perata, P. (2010). Genomic and transcriptomic analysis of the AP2/ERF superfamily in Vitis vinifera. BMC Genomics 11:719. doi: 10.1186/1471-2164-11-719
Litt, A. (2007). An evaluation of A-function: evidence from the APETALA1 and APETALA2 gene lineages. Int. J. Plant Sci. 168, 73–91. doi: 10.1086/509662
Litt, A., and Kramer, E. M. (2010). The ABC model and the diversification of floral organ identity. Semin. Cell Dev. Biol. 21, 129–137. doi: 10.1016/j.semcdb.2009.11.019
Maes, T., Van de Steene, N., Zethof, J., Karimi, M., D’Hauw, M., Mares, G., et al. (2001). Petunia Ap2-like genes and their role in flower and seed development. Plant Cell 13, 229–244. doi: 10.1105/tpc.13.2.229
Mandel, M. A., Gustafsonbrown, C., Savidge, B., and Yanofsky, M. F. (1992). Molecular characterization of the Arabidopsis floral homeotic gene Apetala1. Nature 360, 273–277. doi: 10.1038/360273a0
Marguerit, E., Boury, C., Manicki, A., Donnart, M., Butterlin, G., Nemorin, A., et al. (2009). Genetic dissection of sex determinism, inflorescence morphology and downy mildew resistance in grapevine. Theor. Appl. Genet. 118, 1261–1278. doi: 10.1007/s00122-009-0979-4
Morel, P., Heijmans, K., Rozier, F., Zethof, J., Chamot, S., Bento, S. R., et al. (2017). Divergence of the floral A-function between an Asterid and a Rosid Species. Plant Cell 29, 1605–1621. doi: 10.1105/tpc.17.00098
O’Maoileidigh, D. S., Graciet, E., and Wellmer, F. (2014). Gene networks controlling Arabidopsis thaliana flower development. New Phytol. 201, 16–30. doi: 10.1111/nph.12444
Pelaz, S., Ditta, G. S., Baumann, E., Wisman, E., and Yanofsky, M. F. (2000). B and C floral organ identity functions require SEPALLATA MADS-box genes. Nature 405, 200–203. doi: 10.1038/35012103
Pfent, C., Pobursky, K. J., Sather, D. N., and Golenberg, E. M. (2005). Characterization of SpAPETALA3 and SpPISTILLATA, B class floral identity genes in Spinacia oleracea, and their relationship to sexual dimorphism. Dev. Genes Evol. 215, 132–142. doi: 10.1007/s00427-004-0459-4
Picq, S., Santoni, S., Lacombe, T., Latreille, M., Weber, A., Ardisson, M., et al. (2014). A small XY chromosomal region explains sex determination in wild dioecious V. vinifera and the reversal to hermaphroditism in domesticated grapevines. BMC Plant Biol. 14:229. doi: 10.1186/s12870-014-0229-z
Pinyopich, A., Ditta, G. S., Savidge, B., Liljegren, S. J., Baumann, E., Wisman, E., et al. (2003). Assessing the redundancy of MADS-box genes during carpel and ovule development. Nature 424, 85–88. doi: 10.1038/nature01741
Pnueli, L., Hareven, D., Broday, L., Hurwitz, C., and Lifschitz, E. (1994). The Tm5 mads box gene mediates organ differentiation in the 3 inner whorls of tomato flowers. Plant Cell 6, 175–186. doi: 10.1105/tpc.6.2.175
Poupin, M. J., Federici, F., Medina, C., Matus, J. T., Timmermann, T., and Arce-Johnson, P. (2007). Isolation of the three grape sub-lineages of B-class MADS-box TM6, PISTILLATA and APETALA3 genes which are differentially expressed during flower and fruit development. Gene 404, 10–24. doi: 10.1016/j.gene.2007.08.005
Ramos, M. J., Coito, J. L., Silva, H. G., Cunha, J., Costa, M. M., and Rocheta, M. (2014). Flower development and sex specification in wild grapevine. BMC Genomics 15:1095. doi: 10.1186/1471-2164-15-1095
Riaz, S., Krivanek, A. F., Xu, K., and Walker, M. A. (2006). Refined mapping of the Pierce’s disease resistance locus, PdR1, and sex on an extended genetic map of Vitis rupestris x V. arizonica. Theor. Appl. Genet. 113, 1317–1329. doi: 10.1007/s00122-006-0385-0
Rijpkema, A. S., Royaert, S., Zethof, J., van der Weerden, G., Gerats, T., and Vandenbussche, M. (2006). Analysis of the Petunia TM6 MADS box gene reveals functional divergence within the DEF/AP3 lineage. Plant Cell 18, 1819–1832. doi: 10.1105/tpc.106.042937
Rijpkema, A. S., Vandenbussche, M., Koes, R., Heijmans, K., and Gerats, T. (2010). Variations on a theme: changes in the floral ABCs in angiosperms. Semin. Cell Dev. Biol. 21, 100–107. doi: 10.1016/j.semcdb.2009.11.002
Sakai, H., Krizek, B. A., Jacobsen, S. E., and Meyerowitz, E. N. (2000). Regulation of SUP expression identifies multiple regulators involved in Arabidopsis floral meristem development. Plant Cell 12, 1607–1618. doi: 10.1105/tpc.12.9.1607
Sakai, H., Medrano, L. J., and Meyerowitz, E. M. (1995). Role of Superman in maintaining Arabidopsis floral whorl boundaries. Nature 378, 199–203. doi: 10.1038/378199a0
Sather, D. N., Jovanovic, M., and Golenberg, E. M. (2010). Functional analysis of B and C class floral organ genes in spinach demonstrates their role in sexual dimorphism. BMC Plant Biol. 10:46. doi: 10.1186/1471-2229-10-46
Savidge, B., Rounsley, S. D., and Yanofsky, M. F. (1995). Temporal relationship between the transcription of 2 Arabidopsis Mads Box genes and the floral organ identity genes. Plant Cell 7, 721–733. doi: 10.1105/tpc.7.6.721
Shepard, K. A., and Purugganan, M. D. (2002). The genetics of plant morphological evolution. Curr. Opin. Plant Biol. 5, 49–55. doi: 10.1016/S1369-5266(01)00227-8
Smyth, D. R. (2005). Morphogenesis of flowers - Our evolving view. Plant Cell 17, 330–341. doi: 10.1105/tpc.104.030353
Sreekantan, L., Torregrosa, L., Fernandez, L., and Thomas, M. R. (2006). VvMADS9, a class B MADS-box gene involved in grapevine flowering, shows different expression patterns in mutants with abnormal petal and stamen structures. Funct. Plant Biol. 33, 877–886. doi: 10.1071/FP06016
Sundstrom, J. F., Nakayama, N., Glimelius, K., and Irish, V. F. (2006). Direct regulation of the floral homeotic APETALA1 gene by APETALA3 and PISTILLATA in Arabidopsis. Plant J. 46, 593–600. doi: 10.1111/j.1365-313X.2006.02720.x
Tamura, K., Stecher, G., Peterson, D., Filipski, A., and Kumar, S. (2013). MEGA6: molecular evolutionary genetics analysis version 6.0. Mol. Biol. Evol. 30, 2725–2729. doi: 10.1093/molbev/mst197
Theissen, G. (2001). Development of floral organ identity: stories from the MADS house. Curr. Opin. Plant Biol. 4, 75–85. doi: 10.1016/S1369-5266(00)00139-4
Theissen, G., Becker, A., Di Rosa, A., Kanno, A., Kim, J. T., Munster, T., et al. (2000). A short history of MADS-box genes in plants. Plant Mol. Biol. 42, 115–149. doi: 10.1023/A:1006332105728
Vandenbussche, M., Theissen, G., Van de Peer, Y., and Gerats, T. (2003). Structural diversification and neo-functionalization during floral MADS-box gene evolution by C-terminal frameshift mutations. Nucleic Acids Res. 31, 4401–4409. doi: 10.1093/nar/gkg642
Wagner, D., Sablowski, R. W., and Meyerowitz, E. M. (1999). Transcriptional activation of APETALA1 by LEAFY. Science 285, 582–584. doi: 10.1126/science.285.5427.582
Wellmer, F., and Riechmann, J. L. (2010). Gene networks controlling the initiation of flower development. Trends Genet. 26, 519–527. doi: 10.1016/j.tig.2010.09.001
Wollmann, H., Mica, E., Todesco, M., Long, J. A., and Weigel, D. (2010). On reconciling the interactions between APETALA2, miR172 and AGAMOUS with the ABC model of flower development. Development 137, 3633–3642. doi: 10.1242/dev.036673
Wuest, S. E., O’Maoileidigh, D. S., Rae, L., Kwasniewska, K., Raganelli, A., Hanczaryk, K., et al. (2012). Molecular basis for the specification of floral organs by APETALA3 and PISTILLATA. Proc. Natl. Acad. Sci. U.S.A. 109, 13452–13457. doi: 10.1073/pnas.1207075109
Wurschum, T., Gross-Hardt, R., and Laux, T. (2006). APETALA2 regulates the stem cell niche in the Arabidopsis shoot meristem. Plant Cell 18, 295–307. doi: 10.1105/tpc.105.038398
Yanofsky, M. F., Ma, H., Bowman, J. L., Drews, G. N., Feldmann, K. A., and Meyerowitz, E. M. (1990). The protein encoded by the Arabidopsis homeotic gene Agamous resembles transcription factors. Nature 346, 35–39. doi: 10.1038/346035a0
Yant, L., Mathieu, J., Dinh, T. T., Ott, F., Lanz, C., Wollmann, H., et al. (2010). Orchestration of the floral transition and floral development in Arabidopsis by the bifunctional transcription factor APETALA2. Plant Cell 22, 2156–2170. doi: 10.1105/tpc.110.075606
Yu, D. Y., Kotilainen, M., Pollanen, E., Mehto, M., Elomaa, P., Helariutta, Y., et al. (1999). Organ identity genes and modified patterns of flower development in Gerbera hybrida (Asteraceae). Plant J. 17, 51–62. doi: 10.1046/j.1365-313X.1999.00351.x
Yu, H., Ito, T., Wellmer, F., and Meyerowitz, E. M. (2004). Repression of AGAMOUS-LIKE 24 is a crucial step in promoting flower development. Nat. Genet. 36, 157–161. doi: 10.1038/ng1286
Zanis, M. J., Soltis, P. S., Qiu, Y. L., Zimmer, E., and Soltis, D. E. (2003). Phylogenetic analyses and perianth evolution in basal angiosperms. Ann. Mo. Bot. Gard. 90, 129–150. doi: 10.2307/3298579
Zhao, J. Y., Liu, M. L., Jiang, L., Ding, L., Yan, S. S., Zhang, J., et al. (2014). Cucumber SUPERMAN has conserved function in stamen and fruit development and a distinct role in floral patterning. PLoS One 9:e86192. doi: 10.1371/journal.pone.0086192
Zhao, L., Kim, Y., Dinh, T. T., and Chen, X. (2007). miR172 regulates stem cell fate and defines the inner boundary of APETALA3 and PISTILLATA expression domain in Arabidopsis floral meristems. Plant J. 51, 840–849. doi: 10.1111/j.1365-313X.2007.03181.x
Keywords: Vitis vinifera sylvestris, in situ hybridization, homeotic genes, flower ABCDE model, development, dioecious
Citation: Coito JL, Silva H, Ramos MJN, Montez M, Cunha J, Amâncio S, Costa MMR and Rocheta M (2018) Vitis Flower Sex Specification Acts Downstream and Independently of the ABCDE Model Genes. Front. Plant Sci. 9:1029. doi: 10.3389/fpls.2018.01029
Received: 11 May 2018; Accepted: 25 June 2018;
Published: 16 July 2018.
Edited by:
Cristina Ferrandiz, Instituto de Biología Molecular y Celular de Plantas (IBMCP), SpainReviewed by:
Pablo Daniel Jenik, Franklin & Marshall College, United StatesMichael Nicolas, Centro Nacional de Biotecnología (CNB), Spain
Copyright © 2018 Coito, Silva, Ramos, Montez, Cunha, Amâncio, Costa and Rocheta. This is an open-access article distributed under the terms of the Creative Commons Attribution License (CC BY). The use, distribution or reproduction in other forums is permitted, provided the original author(s) and the copyright owner(s) are credited and that the original publication in this journal is cited, in accordance with accepted academic practice. No use, distribution or reproduction is permitted which does not comply with these terms.
*Correspondence: Margarida Rocheta, rocheta@isa.ulisboa.pt
†These authors have contributed equally to this work.