- 1Laboratory of Aquatic Animal Nutrition and Feed, Fisheries College, Guangdong Ocean University, Zhanjiang, China
- 2School of Life Sciences, Institute of Aquatic Economic Animals, Sun Yat-sen University, Guangzhou, China
This study mainly evaluated the low dietary fish-meal (FM) on growth performance, immune competence and metabolomics response of juvenile Pacific white shrimp, Litopenaeus vannamei reared at low salinity (7‰). Five experimental diets with graded levels (25, 20, 15, 10, and 5%) of FM were formulated. Weight gain, feed utilization and survival were decreased with the decreasing FM levels. When dietary FM decreased, glucose, cholesterol, total bile acids, and triglyceride in hemolymph decreased. Fatty acid synthesis was promoted and fatty acid lipolysis was reduced in hepatopancreas of shrimp fed low dietary FM. Endoplasmic reticulum (ER) stress related genes expression in hepatopancreas were down-regulated and in intestine were upregulated by low dietary FM. Inhibitor kappa B kinaseβ expression in intestine increased with the dietary FM levels, while mRNA levels of dorsal in hepatopancreas showed the opposite tendency. Hematoxylin and eosin (H&E) stain and transmission electron microscope analysis of intestinal samples indicated that low FM diets induced intestinal morphological damage, ER swollen and chromatin condensation. UPLC-Q/TOF-MS analysis indicated that degree of unsaturation of the fatty acid chains of phospholipids in hemolymph decreased with the decreasing dietary FM levels. Lysophospholipids and bile acids metabolism were disturbed by high levels of FM sparing in diet. These results indicated when dietary FM contents decreased, ER stress of shrimp was induced. The decreased unsaturated degree of phospholipids, decreased contents of lysophospholipids, altered lipid metabolism and ER stress may responsible for the impaired growth performance and health of shrimp fed a low FM diet.
Introduction
Fish meal (FM) is a critical ingredient in aquatic feeds due to its balanced nutritional composition (Bendiksen et al., 2011; Olsen and Hasan, 2012; Xie et al., 2014). While the supply of FM was short recently, which make FM to be a limiting factor for the sustainable development of aquaculture. Litopenaeus vannamei has been one of the biggest consumers of FM (Tacon and Metian, 2008; Olsen and Hasan, 2012), the past 20 years witnessed plentiful research about FM replacement in L. vannamei, which showed that FM could be partial substituted without deleterious effects (Amaya et al., 2007; Ye et al., 2011; Yue et al., 2012; Kuhn et al., 2016). Excessive substitution of FM generally led to poor growth performance, which may because the nutrient-sensing and metabolism of fish and shrimp are changed when fed a low FM diet (Wang et al., 2016; Xu et al., 2016). Besides growth retardation, shrimp health were impaired when fed a low FM diet (Wu et al., 2009; Xie et al., 2016, 2020), figure out the potential mechanism would be helpful in further reducing FM in aquafeeds, not only in the feed of L. vannamei, but also in the feeds of many carnivorous fish. The wide nutritional differences between FM and other ingredients make it hard to find the key metabolism pathways and biomarkers which responsible for the negative effects brought by low dietary FM.
Metabolomics is to analyze a complete set of low molecule metabolites in biological samples such as plasma, urine and cell (Johnson et al., 2016). Because metabolites are hypersensitive to exogenous factors such as diet, metabolomics has become a strong discovery tool in nutrition research (Putri et al., 2013). Usually, metabolomics studies of biofluids and tissues are widely performed to evaluate the influences of dietary composition on metabolism and health of human and animals (He et al., 2008). In aquaculture nutrition research, metabolomics had been applied to evaluate nutritional requirements and ingredients utilization, and tend to be a strong technology to detect the complex nutrient utilization differences and identify the metabolic biomarkers (Jin et al., 2015; Li et al., 2017b; Ma et al., 2017; Wei et al., 2017; Alfaro and Young, 2018; Xie et al., 2018).
The commonly used metabolomics platforms are gas chromatography couple with mass spectrometry (GC-MS), liquid chromatography couple with mass spectrometry (LC-MS), and NMR spectroscopy (Rezzi et al., 2013). Recently, applying high pressure resistant column, ultra-performance liquid chromatography coupled with quadrupole time of flight mass spectrometry (UPLC-Q/TOF-MS) has demonstrated significant advantages in measurement time, resolution, throughput, sensitivity and specificity, and shown to be a powerful analytical technology for metabolomics research (Qian et al., 2017; Rindlisbacher et al., 2018).
Like other animals, crustaceans have different lipid classes which including fatty acids, triacylglycerides, phospholipids and sterols (Sinha et al., 2018). Lipids are not only serve as the major energy source and the carrier of fat-soluble vitamins, but also act as the components of cell membranes and the precursors of many important metabolites, therefore lipids are critical to the growth, reproduction, osmoregulation, membrane fluidity, and immune response of animals (González-Félix et al., 2002; Arts and Kohler, 2009; Xu et al., 2018).
In this study, metabolomics concept was employed to evaluate the relationship between growth performance, lipid metabolism, immune-related genes and histoarchitecture of gut of L. vannamei fed different dietary FM levels.
Materials and Methods
Diet Preparation
Five experimental diets were formulated with graded levels of FM (25, 20, 15, 10, and 5%), FM was replaced by chicken meal, wheat gluten, soy protein concentrate and blood meal. Diet formulation and proximate composition are given in Table 1, amino acid and fatty acid composition of the diets are given in Supplementary Table 1 and Supplementary Table 2, respectively. Crystalline amino acids were precoated with carboxymethyl cellulose to prevent leaching loss (Xie et al., 2014). The pellets (1.2 mm diameter) were cold-extruded using a pelletizer (South China University of Technology, Guangdong, China), then heated for 60 min in an electric oven (70°C), and air-dried to approximately 10% moisture, finally stored at -20°C before used (Xie et al., 2018).
Shrimp and Experimental Conditions
Juvenile L. vannamei were purchased from a commercial hatchery (Xiangzhou district, Zhuhai, China). Shrimp were acclimated for 3 weeks and fed a commercial shrimp diet (43.67% crude protein, 8.17% crude lipid, Haida Group, Guangdong, China). Prior to the experiment, 600 shrimp (0.3 g) were randomly assigned into 20 tanks (cylindrical fiberglass, 300 L), and fed one of the five diets in quadruplicate for 8 weeks. Shrimp were fed three times per day at 8:00, 13:00, and 18:00 to apparent satiation. Uneaten feed was collected an hour after the feeding by siphoning in order to determine feed efficiency.
Throughout the feeding trial, temperatures of water was 27.23 ± 1.41°C, the salinity was 7.02 ± 0.21‰, pH was 7.81 ± 0.07, the ammonia nitrogen was 0.03 ± 0.01 mg L–1, and dissolved oxygen was 6.88 ± 0.25 mg L–1. The parameters of water quality were collected twice weekly using the commercial kits (Sangpu, Beijing, China). The photoperiod was natural during the husbandry trial (28th, April-22th, June).
Sampling and Chemical Assays
At the end of feeding phase, shrimp were overnight fasted, shrimp from each tank were weighed and counted. Hemolymph from 10 shrimp per tank were taken from the pericardial cavity using a 1-ml sterile syringe (no anti-coagulant was used), pooled and mashed, then centrifuged (8000 rpm, 4°C, 10 min). The supernatant was collected and stored at -80°C. Intestine and hepatopancreas from two shrimp per tank were immediately frozen and stored at -80°C for RNA extraction.
Moisture, crude lipid, crude protein, and ash contents in the diets were determined using standard methods (AOAC, 1995). Fatty acid compositions of diets were determined by China National Analytical Center. Lipids were extracted by a mixture of chloroform and methanol (2:1, v/v), then solvents was evaporated and fatty acids was saponificated by potassium hydrate. Fatty acids methyl esters were separated and quanitified by a gas chromatograph (GC 7820A, Agilent, United States) equipmented with a detector flame ionization (FID) and a HP-88 column (long × inner diameter × film thickness: 30 m × 0.25 mm × 0.2 μm) with hydrogen as carrier gas. The temperature gradient was from 100°C to 180°C at 10°C/min and then to 200°C at 1°C/min and finally to 230°C at 4°C/min, each sample was running for 49.5 min.
Hemolymph Biochemical Index Assays
Concentrations of glucose (GLU), triglyceride (TG), total bile acid (TBA), and cholesterol (CHO) in hemolymph were determined by glucose oxidase-peroxidase method (Sharp, 1972), glycerol-3-phosphate oxidase-peroxidasemethod (Kohlmeier, 1986), 3α-hydroxysteroid dehydrogenase method (Bruusgaard et al., 1977) and cholesterol oxidase-peroxidase method (Allain et al., 1974), respectively. These indexes were measured following the instructions of the detection kits (Nanjing Jiancheng Bioengineering Institute, China), through monitoring the absorbance changes at 505, 510, 405, and 510 nm in a microplate reader (TECAN infinite M200, Switzerland), respectively.
Intestinal Histology Analysis
In each tank, the similar part of midgut from three shrimp were firstly fixed in Bouin’s solution (within 24 h), secondly transfered in 70% ethanol. Then samples were dehydrated in a graded series of ethyl alcohol and embedded in paraffin. Sections were stained with hematoxylin/eosin and observed under a microscope (Nikon Ni-U, Japan) at the magnification of 100×.
Another three midgut per tank were collected for transmission eletron microscopy (TEM) examination. The method was described by Zhang et al. (2012) and Xie et al. (2020), briefly, samples were fixed in 2.5% glutaraldehyde solution (4°C), then fixed in 1% osmium tetroxide (OsO4) for 1 h, dehydrated in a graded series of ethyl alcohol, finally embedded with resin. Ultrathin sections (90 nm) were placed on copper grids and were stained with saturated uranyl acetate solution for 30 min, rinsed with distilled water and poststained with lead citrate for 30 min. Ultrathin sections were screened and observed with a TEM (FEI Tecnai G2 20, Holland).
RNA Extraction and qRT PCR Analysis
The methods of RNA extraction, cDNA synthesis and qRT PCR were described as Xie et al. (2019). Total RNA was extracted from hepatopancreas and intestine using Trizol® reagent (Invitrogen, United States). Agarose gel electrophoresis was used to assess RNA integrity, and concentration and purity of RNA was measured by spectrophotometric analysis (A260:A280 nm). cDNA was synthesized using a PrimeScriptTM RT reagent kit with gDNA Eraser (Takara, Japan).
Real-time PCR for the genes were measured on the LightCycler 480 (Roche Applied Science, Basel Switzerland) using SYBR® Premix Ex TaqTM II (Takara, Japan). The efficiency of the primers were close to 100% (Supplementary Table 3).
Normalization of qRT PCR data was done by geometric averaging of tworeference genes (β-actin and elongation factor1α) (Vandesompele et al., 2002). Each sample were runned three technical replications.
UPLC-Q/TOF-MS Analysis
Thirty six shrimp hemolymph samples for all treatments were analyzed by UPLC-Q/TOF-MS in this research to study the lipid profiles in FM25, FM15 and FM5, 12 for each treatment. The method was described by Xie et al. (2020). Briefly, 400 μL acetonitrile/methanol (9:1) was mixed with 100 μL hemolymph and vortexed for 30 s. The mixture was centrifuged at 13, 000 g and 4°C for 10 min, 200 μL supernatant was used for UPLC-Q/TOF-MS analysis. Equal amounts of all samples were pooled as a QC sample for UPLC-Q/TOF-MS system conditioning and quality control.
UPLC-Q/TOF-MS was performed on an ACQUITY UPLC system (Waters, Manchester, United Kingdom) coupled with a G2-Si HDMS QTOF mass spectrometer (Waters, Manchester, United Kingdom). Chromatographic separation was carried out at 40°C on an ACQUITY HSS T3 1-class column (2.1 × 100 mm, 1.8 μm, Waters). The method of the UPLC-Q/TOF-MS analysis was same as Xie et al. (2020).
Peak detection and alignment of the mass dada were performed by Waters Masslynx v4.1, then all data were normalized to the summed total ion intensity per chromatogram. Four thousand twenty-five and three thousand two hundred thirty-three features were detected in positive and negative modes, respectively. All the features with CVs >30% were removed. Principal component analysis (PCA) and orthogonal partial least squares discriminant analysis (OPLS-DA) were performed by SIMCA-P (Version 13.0, Umetrics, Sweden).
The significantly different metabolites were selected based on variable influence on projection (VIP) values obtained from the OPLS-DA model, P-values from one way ANOVA analysis and the maximum fold change. The metabolites with VIP values larger than 1.0, P-values less than 0.05 and fold change larger than 1.5 were regarded as significantly different metabolites.
Metabolite indentification was performed using Progenesis QI (Waters, Non-linear Dynamics, Newcastle, United Kingdom). The Human Metabolome databased (HMDB), LipidMaps and Chemspider were used for MS1 identification, and theoretical fragments were used for MS/MS identification. The score (total 60) of identified metabolites obtained from QI larger than 40 was regarded as acceptable, otherwise would be regarded as uncertain.
Pheatmap package based on R software (version 3.7.2) was used for heatmap and cluster analysis.
Calculations and Statistical Analysis
The parameters were calculated as follows:
Percent weight gain (WG,%) = 100 × (final body weight - initial body weight)/initial body weight
Survival (%) = 100 × (final number of shrimp)/(initial number of shrimp)
Specific growth ratio (SGR,% day–1) = 100 × (Ln final body weight - Ln initial body weight)/t
Feed conversion ration (FCR) = feed consumed (g, dry weight)/weight gain (g, wet weight)
Protein efficiency ratio (PER) = weight gain (g, wet weight)/protein intake (g, dry weight)
Protein productive value (PPV,%) = 100 × protein gain (g)/protein intake (g)
All the data were presented as the means with SEM and were statistically analyzed by SPSS 19.0 (SPSS, Chicago, IL, United States). Data were first tested for normality (Shapiro-Wilk test) and homogeneity (Levene’s test). Normal distribution data were analyzed by one-way ANOVA. When data showed significant differences (P < 0.05), they were further compared using Duncan’s multiple range test., Non-parameter Kruskal-Wallis test was applied when data did not have a homogeneous variance, followed by all pairwise multiple comparisons if there were significant differences (P < 0.05).
Results
Effect of Dietary FM Levels on Growth Performance of White Shrimp
WG, survival, SGR, FCR, PER, and PPV were significantly affected by dietary FM levels (P < 0.05) (Figure 1). WG, SGR, FCR, PER, and PPV were lower in shrimp fed diets contained 10 and 5% FM, survival was significantly decreased when dietary FM levels were less than 20% (Figure 1). After dietary FM decreased from 25 to 5%, FCR increased 14.79%, WG, SGR, PER, PPV and survival decreased 26.55, 10.25, 11.94, 10.67, and 46.09%, respectively. All the parameters were significantly correlated to FM levels in the diet.
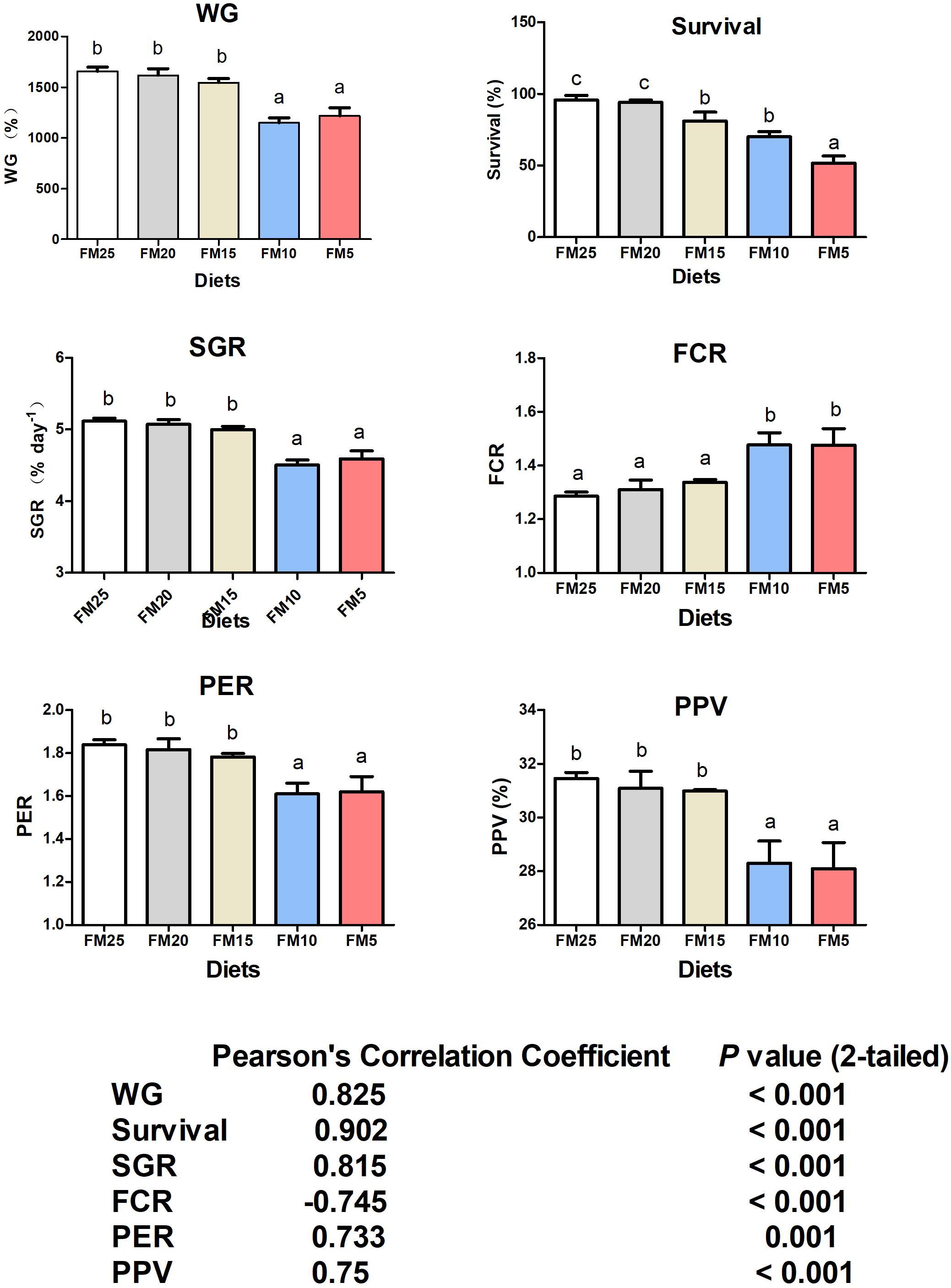
Figure 1. The effect of fish meal levels on growth performance and feed utilization of white shrimp. Data are means with SEM. Mean values with different letters denote significantly differences (P < 0.05). WG, weight gain; SGR, specific growth ratio; PER, protein efficiency ratio; FCR, feed conversion ration; PPV, protein productive value.
Glucose and Lipid Metabolism Were Affected by FM Replacement
Effect of FM replacement on hemolymph biochemical indexes of L. vannamei was displayed in Figure 2. Glucose was significantly lower in shrimp fed diets with FM levels less than 20% compared to those fed FM25 and FM20 (P < 0.05). Triglyceride and cholesterol showed similar tendency with glucose, higher in shrimp fed FM25 and FM20 compared to shrimp fed other diets (P < 0.05). Total bile acid (TBA) was higher in shrimp fed FM25 and FM20 than those fed FM5 (P < 0.05).
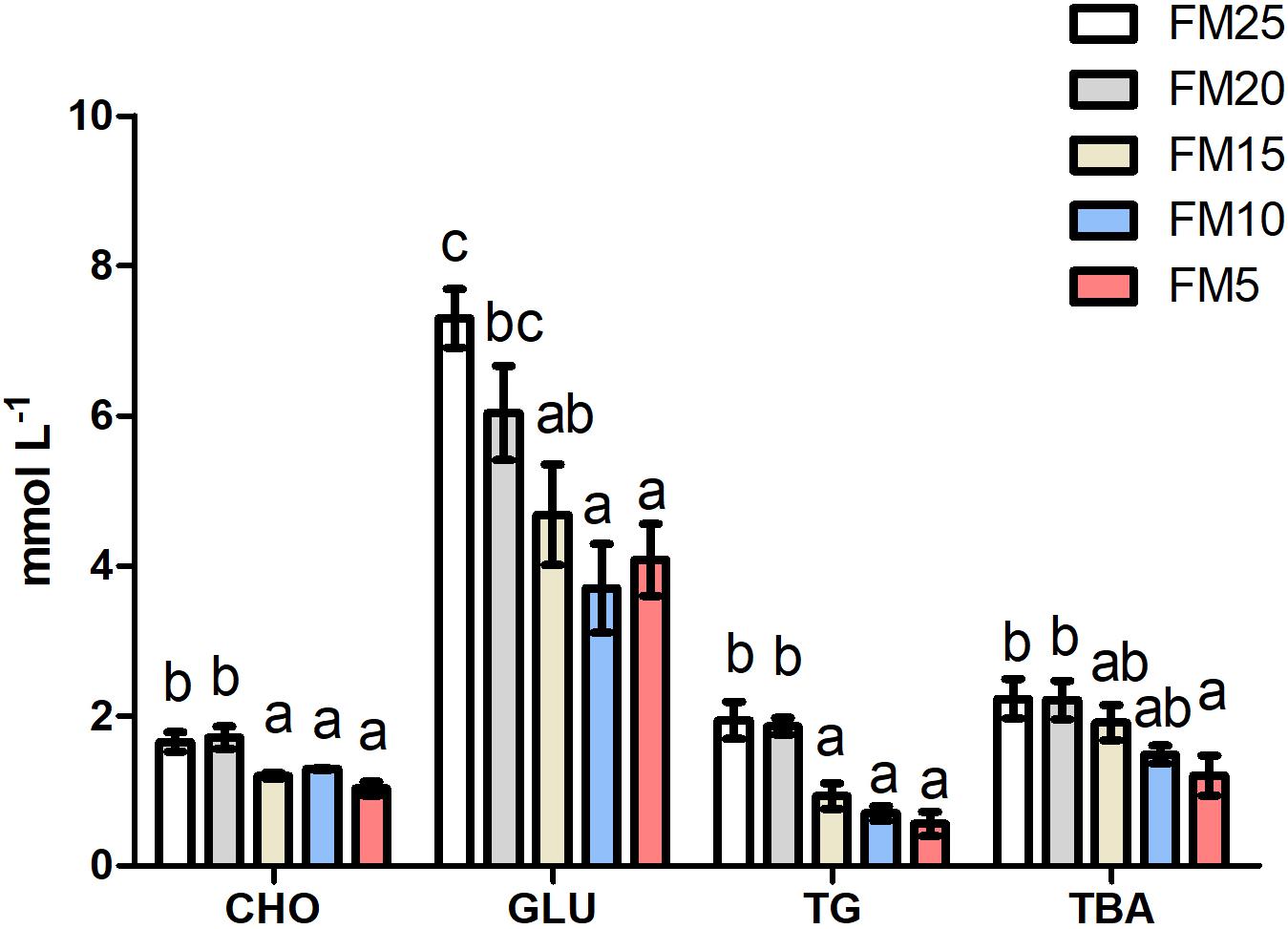
Figure 2. The effect of fish meal levels on hemolymph parameters of white shrimp. GLU, glucose; TG, triglyceride; CHO, cholesterol; TBA, total bile acid. Data are means with SEM. Mean values with different letters denote significantly differences (P < 0.05).
The gene expression of fatty acid synthetase (fas) was increased with the decreasing dietary FM levels (P < 0.05) (Figure 3), while the expression of carnitine palmitoyltransferase 1 (cpt-1) and denosine monophosphate activated protein kinase (ampk) showed opposite tendency, which were higher in shrimp fed high dietary FM than shrimp fed other diets (P < 0.05). There were no differences in the mRNA levels of pyrubate kinase (pk), glucose transporter 2 (glut2) and arginine kinase (ak) among the five groups (P > 0.05).
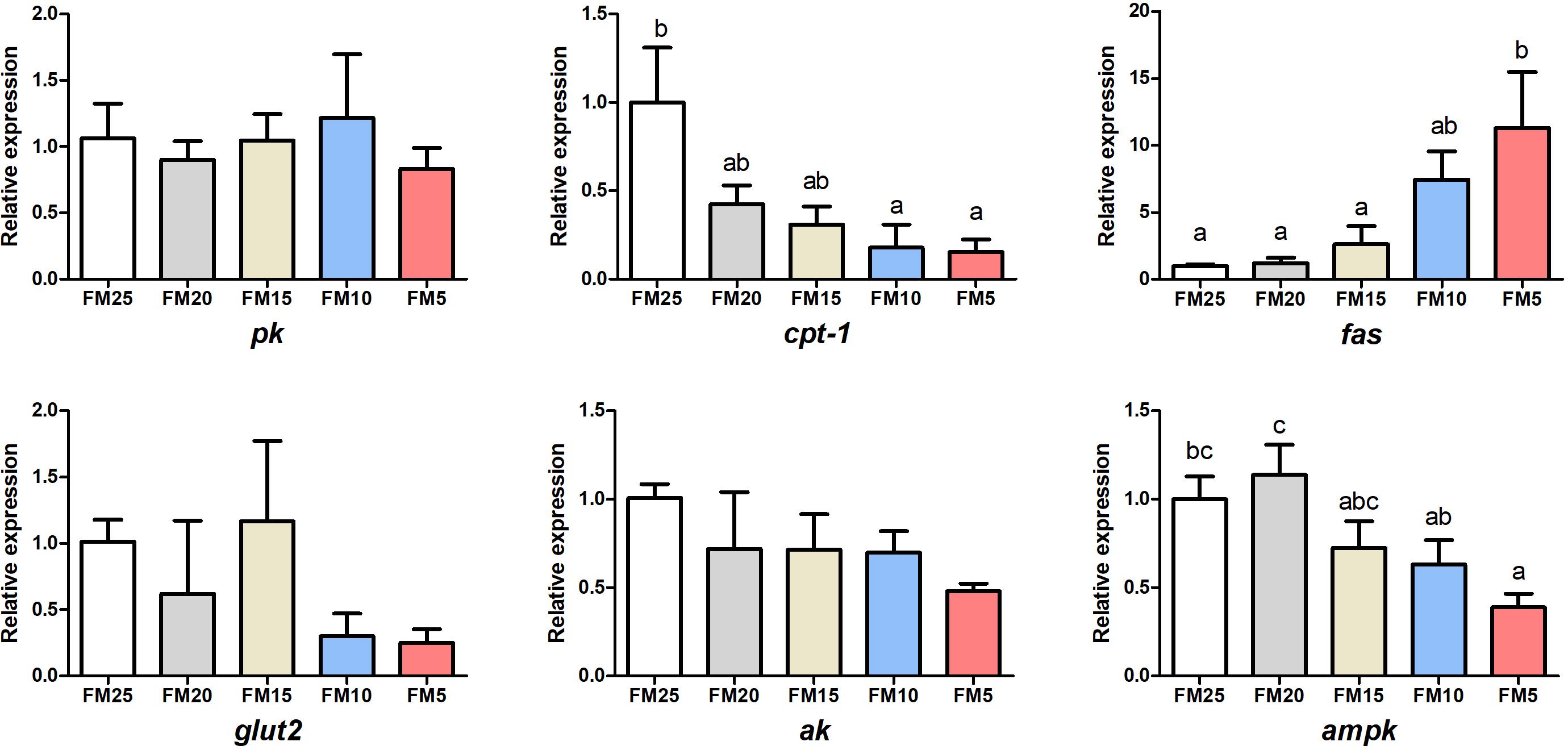
Figure 3. The effect of fish meal levels on energy metabolism of white shrimp. Pk, pyruvate kinase; ak, arginine kinase; cpt-1, carnitine palmitoyltransferase 1; fas, fatty acid synthetase; ampk, denosine monophosphate activated. Data are means with SEM. Mean values with different letters denote significantly differences (P < 0.05).
Low FM Diet Induced Endoplasmic Reticulum Stress (ERS) of Shrimp
Activating transcription factor 4 (atf4), extracellular signal-regulated kinase (erk) and eukaryotic initiation factor 2 alpha (eif2α) mRNA levels in hepatopancreas were decreased with the decreasing dietary FM levels (Figure 4) (P < 0.05), while the expression of these three genes in intestine showed the opposite tendency as the hepatopancreas. Erk expression was lower in shrimp fed FM25 compared to those fed FM5, and atf4 mRNA levels were lower in shrimp fed FM25 and FM20 compared to those fed diet contains lowest FM (P < 0.05).
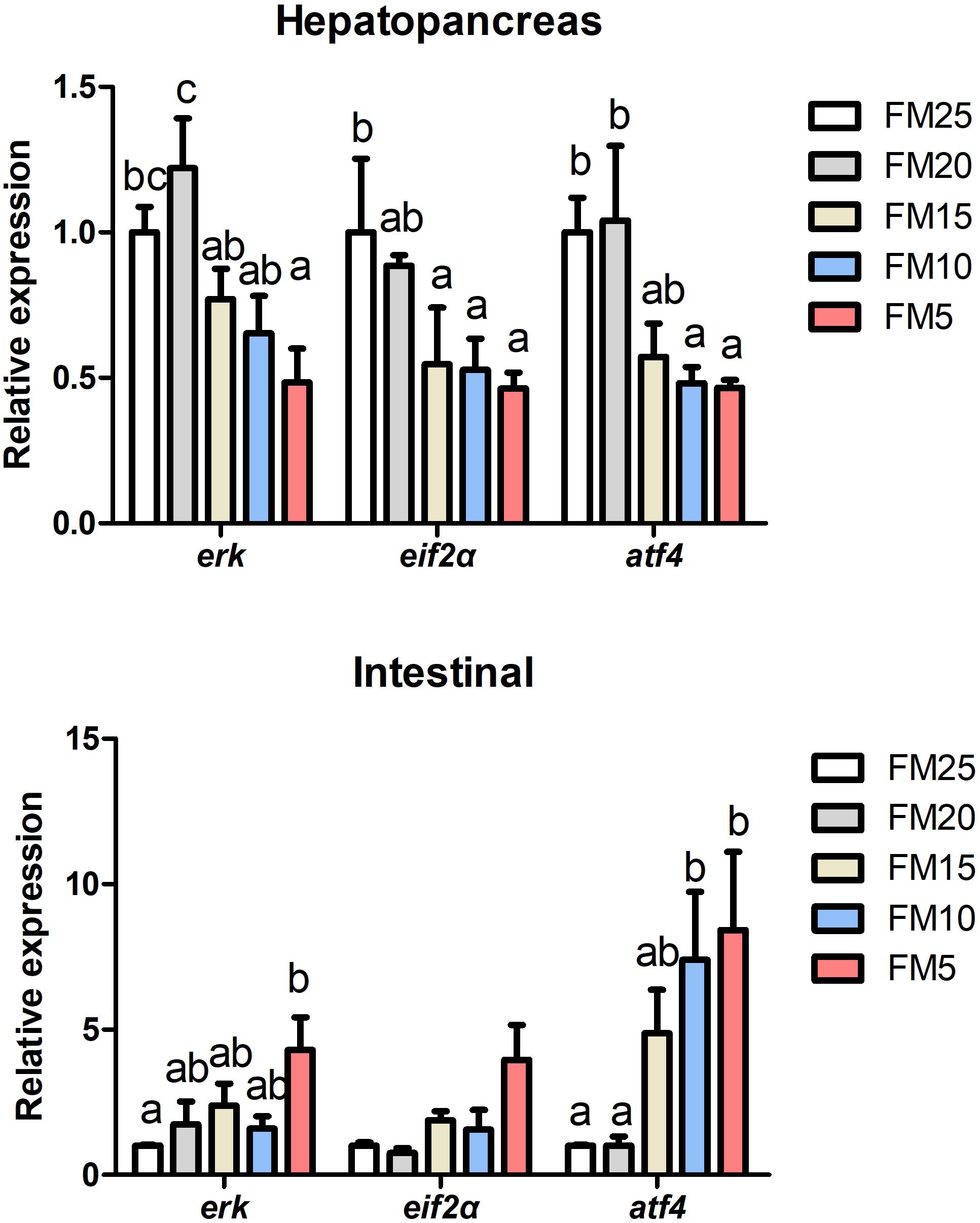
Figure 4. The effect of fish meal levels on mRNA expression of ER stress related genes of white shrimp. eif2α, eukaryotic initiation factor 2 alpha; atf4, activating transcription factor 4; erk, extracellular signal-regulated kinase. Data are means with SEM. Mean values with different letters denote significantly differences (P < 0.05).
Low FM Diets Impaired Immune Ability of Shrimp and Induced Intestinal Damage
Dorsal mRNA levels in hepatopancreas were significantly affected by FM levels in the diets, the highest dorsal expression was in shrimp fed FM25 and lowest was in shrimp fed FM15 (P < 0.05) (Figure 5), there were no differences in the expression of ikkβ and relish among all the treatments (P > 0.05). Ikkβ mRNA expression in intestine was increased with the decreasing dietary FM levels (P < 0.05), there were no differences in the expression of dorsal and relish among the five groups (P > 0.05).
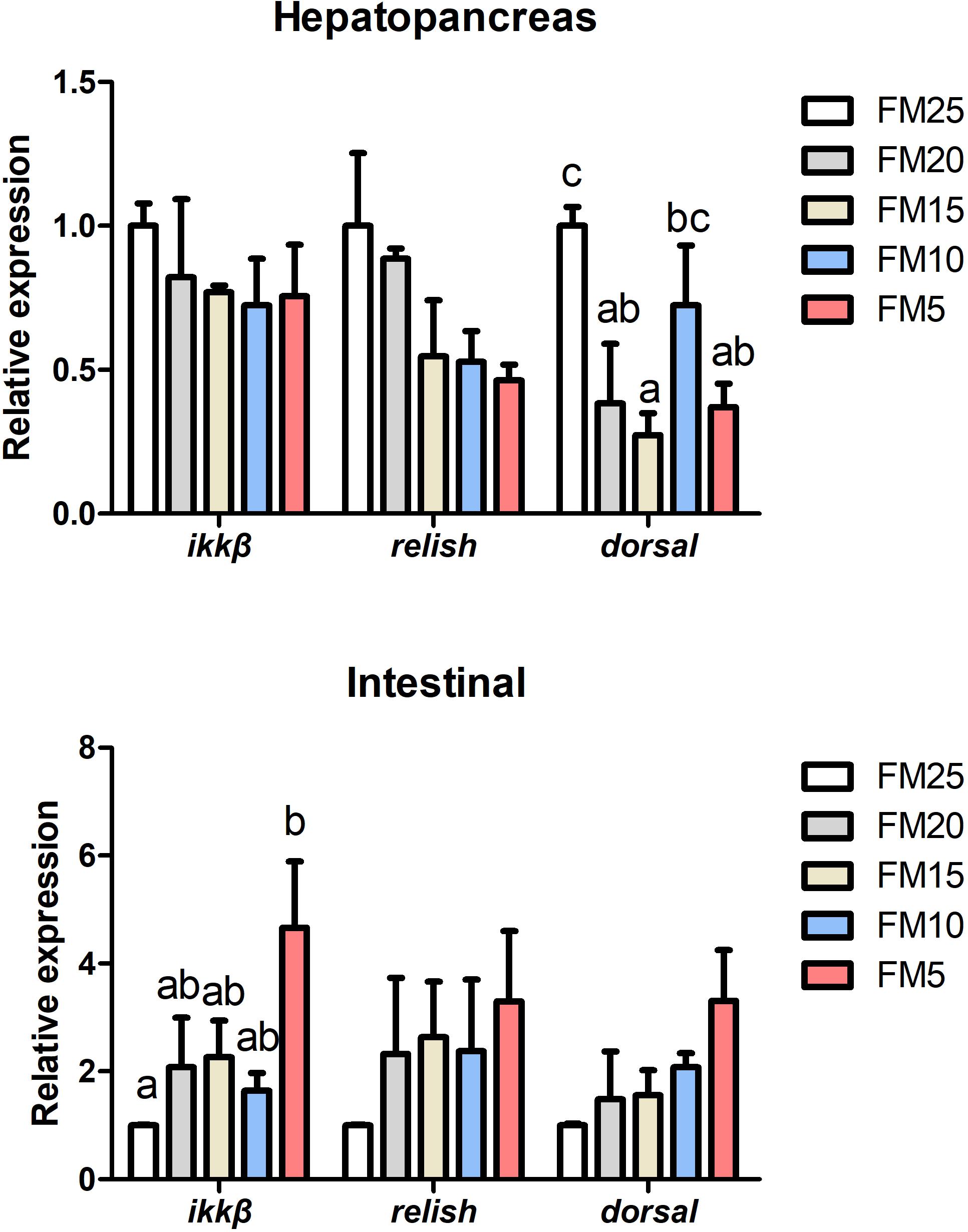
Figure 5. The effect of fish meal levels on mRNA expression of NF-kB pathway related genes of white shrimp. Ikkβ, inhibitor of nuclear factor kappa-B kinase β. Data are means with SEM. Mean values with different letters denote significantly differences (P < 0.05).
Intestinal HE stain showed that intestinal mucosal folds were clearly impaired in shrimp fed the low FM diets (FM15 and FM5) (Figure 6). TEM results showed that the intestine suffered more serious damage in shrimp fed the low FM diets (Figure 6), ER was swollen in shrimp fed the FM15 and FM5. The outside nuclear membrane was partly separated from the inside nuclear membrane in shrimp fed FM15 diet, and they were totally separated in shrimp fed FM5 diet. The apoptosis bodies were formed in shrimp fed the FM5 diet.
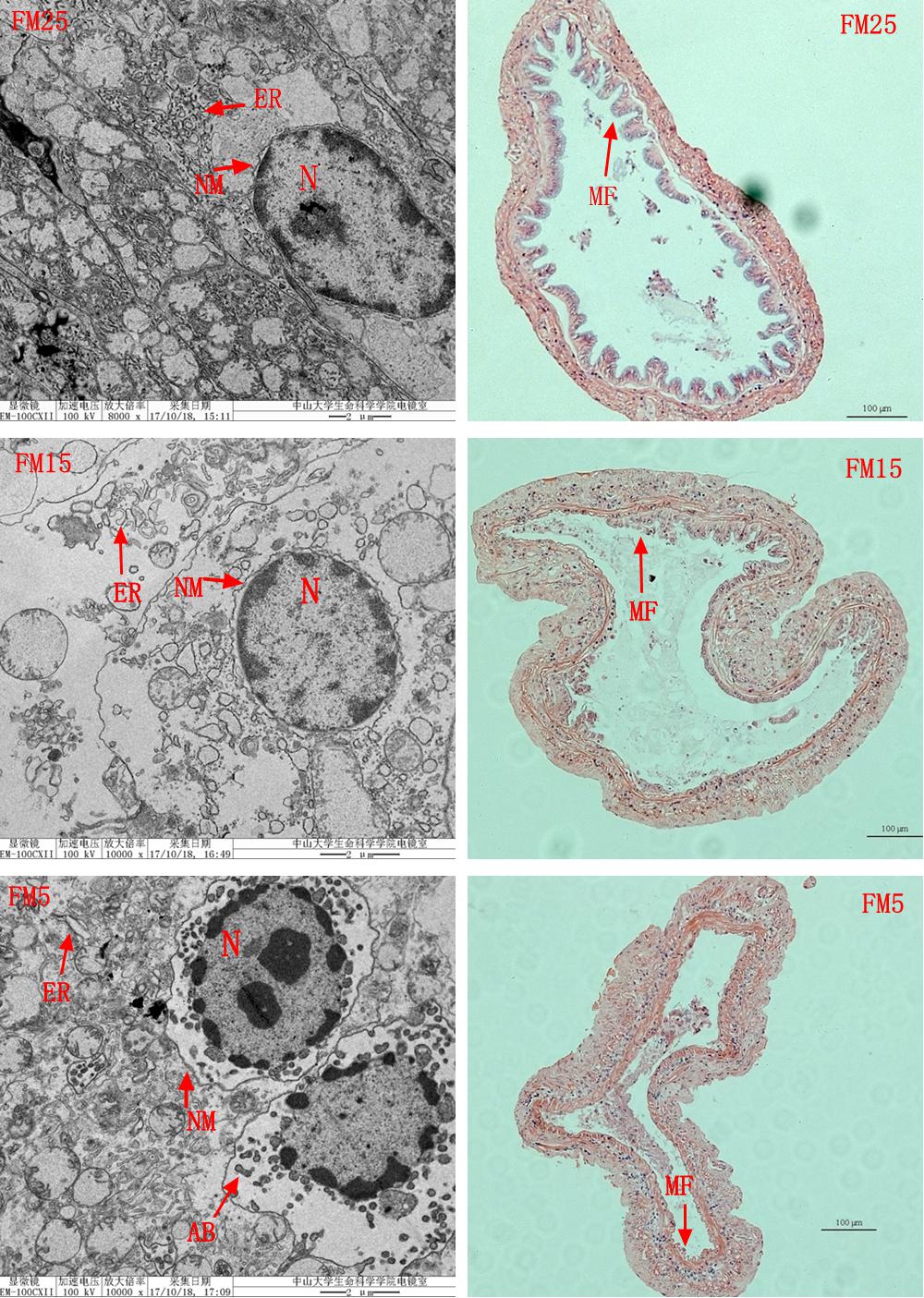
Figure 6. The effect of fish meal levels on intestinal morphology of white shrimp. ER, endoplasmic reticulum; N, cell nucleus; NM, nucleus membrane; AB, apoptosis bodies; MF, mucosal folds. Intestinal HE stain (the right part) showed that the intestinal MF were clearly impaired in shrimp fed the low FM diets (FM15 and FM5). TEM analysis (the left part) showed the ER was swollen in shrimp fed the FM15 or FM5. The outside NM was partly separated from the inside NM in shrimp fed the FM15, and they were totally separated in shrimp fed the FM5. The apoptosis bodies were formed in shrimp fed the FM5 diet.
Multivariate Analysis and Identification of Significant Different Metabolites
In this study, PCA analysis showed a clear cluster in ESI+ (R2X = 0.808, Q2 = 0.708) and ESI- (R2X = 0.84, Q2 = 0.668) modes (Figures 7A,B). OPLS-DA analysis clearly distinguished the different groups in ESI+ (R2X = 0.816, R2Y = 0.979, Q2 = 0.884) and ESI- (R2X = 0.627, R2Y = 0.969, Q2 = 0.927) modes (Figures 7C,D), showed a goodness of fit (R2) and predictive ability (Q2) in this model. Features with VIP values larger than 1.0, P-values less than 0.05 and fold change larger than 1.5 were regarded as significantly different features. Altogether, there were 545 significant features satisfying the criterion (309 in ESI+ and 236 in ESI-), 74 in ESI+ and 137 in ESI- were identified, 26 in ESI+ and 67 in ESI- were regarded as credible. In total, 93 significant different metabolites were identified in this study (Table 2).
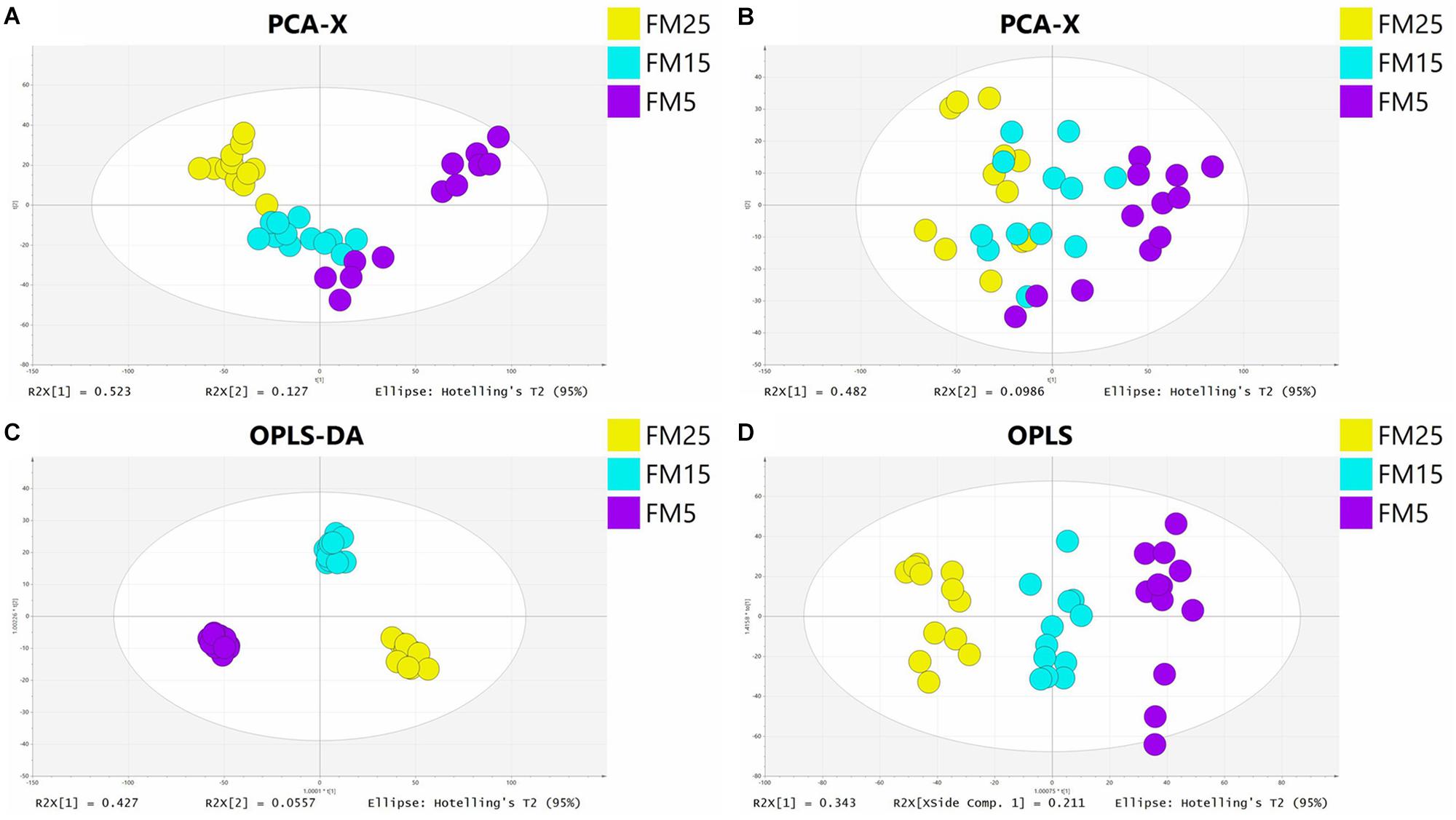
Figure 7. The principal component analysis (PCA) score plot in ESI+ (A) and ESI− (B) models and orthogonal partial least squares discriminant analysis (OPLS-DA) score plot in ESI+ (C) and ESI− (D) models.
Analysis of the Significantly Different Metabolites
Heatmap based on the significantly different metabolites showed clear difference between shrimp fed the FM25, FM15 and FM5 (Figure 8). Most of these metabolites were lipids, especially phospholipids, including phosphatidylcholine (PC), phosphatidyl ethanolamine (PE), Phosphatidyl serine (PS), Phosphatidyl inositols (PI), phosphatidic acid (PA), sphingolipid, fatty acids, and sterol.
Heatmap based on the lipids with more than five unsaturated bond showed clear differences in three groups (Figure 9), shrimp fed diet with high dietary FM tend to have more polyunsaturated lipids in hemolymph. LysoPCs were higher in hemolymph of shrimp fed high dietary FM (Figure 10), while saturated and monosaturated lipids were tend to be higher in hemolymph of shrimp fed low dietary FM.
Cholesterol and bile acid metabolism were also affected by dietary FM levels in this study (Figure 11). Taurohyocholic acid and 7-Ketodeoxycholic acid were decreased with the decreasing dietary FM levels (P < 0.05), while the contents of 7alpha-Hydroxy-3-oxo-5alpha-cholan-24-oic acid and 3alpha,7alpha,12alpha-Trihydroxy-5beta-23E-cholestan-26-oic acid were increased with the decreasing dietary FM levels (P < 0.05).
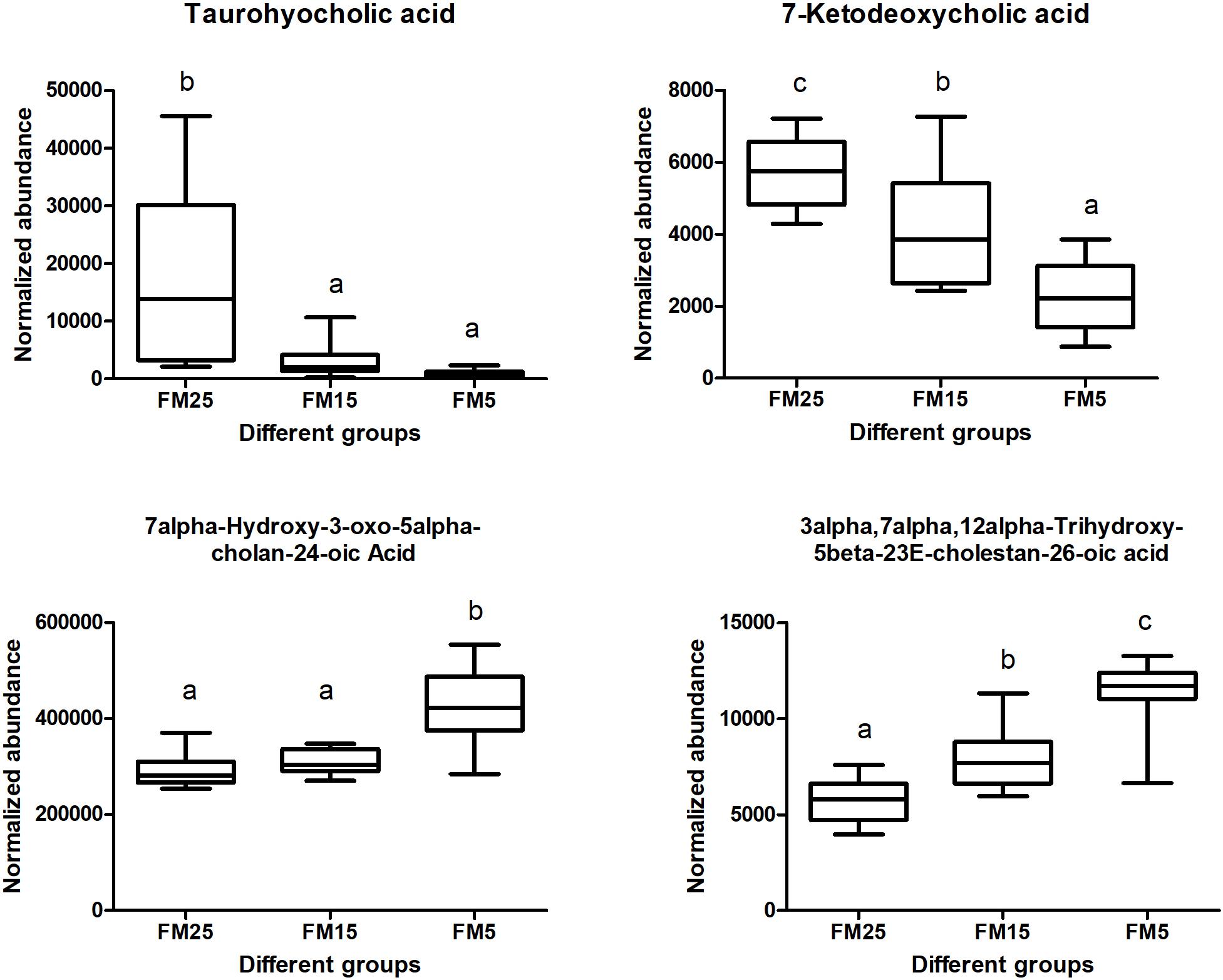
Figure 11. Effect of dietary FM on bile acids contents in hemolymph of white shrimp. Values with different letters denote significantly differences (P < 0.05).
Discussion
In this study, micro-balanced low FM diets were formulated, phytase and immunoenhancer were supplemented to enhance the digestive and immune ability of white shrimp. Our results revealed that growth performance and feed utilization of L. vannamei reared at low salinity was significantly reduced when dietary FM levels were lower than 15%. FM replacement is complex and largely depend on the diet formulation, shrimp quality and the environment. The previous research indicated that minimum FM level in the diet of white shrimp varies widely from 0 to 20%, but most of the research were conducted in sea water, because the nutritional requirement and physiology of white shrimp would be changed at low salinity (Li et al., 2017a), it was hard to compared with our result. Notably, survival of shrimp in this study was decreased with the decreasing FM levels in the diets, which was not observed in most of FM replacement research. The decreased survival in shrimp fed a low FM diet indicated that the low dietary FM harmed the health of shrimp. The impaired intestinal structure and intestinal epithelial cell subcellular structure also proved the above viewpoint. So that the impaired health of shrimp might be the primary problem after dietary FM decreased, but what’s the potential mechanism involved in this process?
Low dietary FM induced ER stress in this study. ER is the primary subcellular organelle of eukaryotic cells, and is the main place for protein folding and maturation, also for lipid synthesis (Tam et al., 2012). Conditions interfering with the function of the ER are collectively called ER stress, which is induced by excessive protein traffic or the accumulation of unfolded protein (Li et al., 2013; Liang et al., 2016). One of the pathway involved in ER stress is double stranded RNA-activated protein kinase like ER kinase (PERK)-eIF2α-ATF4 pathway (Tam et al., 2012). The expression of eif2α, erk and atf4 mRNAs in hepatopancreas and intestine were significantly affected by dietary FM levels. Ammonia stress and WSSV infection were found to induce the ER stress in white shrimp (Li et al., 2013; Liang et al., 2016; Yuan et al., 2017), while diet induced ER stress of white shrimp was still not reported. ER stress also was observed when rainbow trout was fed plant based diet, the potential reason may be the imbalance of amino acid (Skiba-Cassy et al., 2016). The presence of anti-nutritional factors (gossypol) also induced the ER stress in turbot (Bian et al., 2016). ER stress might be induced by a series of factors, in this study, the amino acids were balanced among all the groups, we were not sure whether the presence of anti-nutritional factors in plant proteins (SPC and wheat gluten) or other factors induced the ER stress.
ER stress was usually coupled with immune response (Tam et al., 2012). NF-κB is a key regulator for immune and inflammatory responses, and the recent research suggested that NF-κB was activated by ER stress during the inflammation (Kitamura, 2011). NF-κB is normally held inactive by its inhibitor, IκBα, and IκBα kinase (IKK) phosphorylate IκBα leading to its degradation and NF-κB activation (Ko et al., 2017). IKK is required for maximum activation of NF-κB in response to ER stress (Tam et al., 2012). In this study, ikkβ mRNA expression increased in intestine after FM was replaced, which may induced by the ER stress. Dorsal and Relish are the NF-κB family proteins in white shrimp (Qiu et al., 2014), in this study dorsal mRNA expression in liver was decreased with the decreasing FM levels, indicated that NF-κB in liver also affected by dietary FM level, and showed the same tendency with ER stress. The development of ER stress and inflammation finally induced intestinal damage in this study, plant proteins were found to have negative effect on the intestinal histology of fish and shrimp in many research, which may caused by the presence of anti-nutritional factors or the imbalance of nutrients (Navarrete et al., 2013; Bansemer et al., 2015). The present study not only observed the impaired intestinal brush border after FM replacement, but also found that reduced the FM in the diet would impaired the subcellular structure of white shrimp (the swollen ER and damaged nucleus).
Low FM impaired the anti-oxidative ability and immune response of white shrimp in our present and previous research (Xie et al., 2016). Although white shrimp is an euryhaline animal, the innate immunity of the white shrimp was weakened by the low salinity farming in many research (Wang and Chen, 2005; Li et al., 2007, 2010). The low salinity farming condition may worsen the low FM stress, and induced lower survival rate in this study. Salinity has a strong influence on energy metabolism, the energy demand of white shrimp would increase at low salinity (Rosas et al., 2002; Ye et al., 2009). In this study, the glucose and lipid metabolism of white shrimp were disturbed by FM replacement. Glucose, TG and TBA in hemolymph were decreased with the decreasing dietary FM, the fatty acid oxidation was decreased and fatty acid synthesis was enhanced in hepatopancreas after dietary FM was decreased. The present results indicated that under low salinity farming condition, shrimp fed the low FM diets may expand more energy on immune response.
Lipids are not only serve as one of the main fuels to supply the energy requirement of animals, also play important role in cell proliferation, immunity, apoptosis and inflammation (Arts and Kohler, 2009). Docosahexaenoic acid (DHA), one of the most important long chain ployunsaturated fatty acids (LC-PUFA), is able to inhibit a number of aspects of inflammation, derivatives of DHA such as resolvins, maresins and protectins also involved in the inflammatory process such as cytokines production and lymphocyte activity (Ye et al., 2010; Zhao et al., 2015). In this study, the DHA in hemolymph decreased with the decreasing dietary FM levels, which indicated shrimp fed the low FM diet consumed more DHA in the immune response. Besides that, lipids also structurally important, as the main component of cellular membranes (Jeromson et al., 2015). Under the stress condition, cell membranes composition and fluidity were associated with the immune response in many studies (Arts and Kohler, 2009). The activity of many membrane-bound proteins were directly regulated by the physical state of membrane lipids, such as ion channels, sensor proteins and receptor-associated protein kinases (Litman et al., 2001; Turner et al., 2003). This fluidity is largely dependent on lipid composition, the addition of fatty acid chain length and acyl chain doubled bonds is generally assumed to increased the membrane fluidity (Stillwell and Wassall, 2003; Maulucci et al., 2016). In this study, the degree of unsaturation of phospholipids in hemolymph of shrimp was decreasing with the decreased dietary FM levels. Most of the phospholipids with five or more double bonds clearly decreased with the decreasing dietary FM levels, especially for the phospholipids incorporated with DHA and EPA. Since LC-PUFA (especially DHA and EPA) are highly flexible, the decreased levels of them in phospholipids would impaired the membrane function, affect the membrane biophysical properties such as fluidity, flexibility and thickness (O’Shea et al., 2009; Kitson et al., 2016). On the other hand, most of phospholipids with 1 or 0 double bonds increased with the decreasing dietary FM levels. The present results clearly indicated the unsaturation degree of fatty acids of phospholipids was decreased in shrimp fed the low FM diet, which means the function of cell membrane in hemolymph was affected by dietary FM levels. The decreased unsaturation degree of fatty acids of phospholipids usually affect the immune receptor in membrane and then induced the impair of following immune response, this might be one of the main reason that health status was affected in shrimp fed a low FM diet. In this study, ER was swollen in shrimp fed a low FM diet, which may also induced by the decreased unsaturation degree of membrane lipids.
lysoPCs (LPC) and lysoPEs (LPE) are derived from PC and PE, they are the most prominent lysoglycerophospholipids and are considered to be inflammatory lipids involved in several immune-mediated disease (Rindlisbacher et al., 2018), and also participate in energy metabolism and storage, cell signaling and other biological processes (Bai et al., 2014). Some research showed that LPC and LPE are increased in inflammation associated diseases, and they exert their effects through different signaling pathways such as PKC, NF-κB and ERK (Zeng et al., 2017). While in other studies, some of the LPC and LPE were decreased in the inflammation condition (Bai et al., 2014; Zhou et al., 2014; Qian et al., 2017; Wang et al., 2017). In this study, several LPC and LPE were decreased with the decreasing dietary FM levels, which indicated the relationship of inflammation and the levels of LPC and LPE may dependent on species and the inflammation stage. In this study, the energy metabolism was affected by dietary FM levels, the disturbed energy metabolism may decreased the LPC and LPE in hemolymph.
Besides the phospholipids metabolism, the bile acid metabolism also affected by dietary FM levels. Bile acids are critical to lipid digestion, cholesterol metabolism and other lipid related pathways (Staley et al., 2017). Disturbance of the bile acid pool may result in a variety of disease states. Taurohyocholic acid is a conjugated primary bile acid (Joyce et al., 2014), in this study, TBA and taurohyocholic acid were decreased with the decreasing dietary FM levels, indicated the lipid digestion was affected, which may related to the impaired lipid metabolism in shrimp fed the low FM diets. 7-Ketodeoxycholic acid is a secondary bile acid and is regarded as toxic bile acid (Chen et al., 2016), while it was decreased with the decreasing dietary FM levels. 7alpha-Hydroxy-3-oxo-5alpha-cholan-24-oic acid and 3alpha,7alpha,12alpha-Trihydroxy-5beta-23E-cholestan-26-oic acid two free primary bile acids, they are the precursor of two cytotoxicity bile acids deoxycholic acid and lithocholic acid (Chen et al., 2016), their increase in hemplymph may associated with the inflammation in shrimp fed low FM diets. These results clearly indicated the bile acids metabolism was affected by dietary FM levels, which may closely linked to the impaired lipid metabolism and inflammation in shrimp fed a low FM diets.
Conclusion
In conclusion, at the low salinity farming condition, low dietary FM would decrease the growth performance and feed utilization of shrimp, also affect the health status of shrimp. The poor growth performance and unhealthy status of shrimp fed a low FM diet may mainly caused by the decreased unsaturated degree of membrane phospholipids, decreased contents of lysophospholipid and the disturbed lipid metabolism.
Data Availability Statement
The raw data supporting the conclusions of this article will be made available by the authors, without undue reservation, to any qualified researcher.
Author Contributions
SX and LT designed the experiments. SX carried out the experiments and wrote the manuscript. JN, YL, and BT reviewed and revised the manuscript. All authors contributed to the article and approved the submitted version.
Funding
This work was supported by the fund of the National Key R&D Program of China (2019YFD0900200), the Guangdong Basic and Applied Basic Research Foundation (2019A1515011970), and the China Agriculture Research Systerm-48 (CARS 48).
Conflict of Interest
The authors declare that the research was conducted in the absence of any commercial or financial relationships that could be construed as a potential conflict of interest.
Supplementary Material
The Supplementary Material for this article can be found online at: https://www.frontiersin.org/articles/10.3389/fphys.2020.01024/full#supplementary-material
References
Alfaro, A. C., and Young, T. (2018). Showcasing metabolomic applications in aquaculture: a review. Rev. Aquacult. 10, 135–152. doi: 10.1111/raq.12152
Allain, C. C., Poon, L. S., Chan, C. S. G., Richmond, W. Y., and Fu, P. C. (1974). Enzymatic determination of total serum cholesterol. Clin. Chem. 20, 470–475.
Amaya, E. A., Davis, D. A., and Rouse, D. B. (2007). Replacement of fish meal in practical diets for the Pacific white shrimp (Litopenaeus vannamei) reared under pond conditions. Aquaculture 262, 393–401. doi: 10.1016/j.aquaculture.2006.11.015
AOAC (1995). Official Methods of Analysis of Official Analytical Chemists International, 16th Edn. Arlington, VA: Association of Official Analytical Chemists.
Arts, M. T., and Kohler, C. C. (2009). Health and condition in fish: the influence of lipids on membrane competency and immune response. Lipids Aquat. Ecosys. 38, 237–256. doi: 10.1007/978-0-387-89366-2_10
Bai, Y., Zhang, H., Sun, X., Sun, C., and Ren, L. (2014). Biomarker identification and pathway analysis by serum metabolomics of childhood acute lymphoblastic leukemia. Clin. Chim. Acta 436, 207–216. doi: 10.1016/j.cca.2014.05.022
Bansemer, M. S., Forder, R. E. A., Howarth, G. S., Suitor, G. M., Bowyer, J., and Stone, D. A. J. (2015). The effect of dietary soybean meal and soy protein concentrate on the intestinal mucus layer and development of subacute enteritis in Yellowtail Kingfish (Seriola lalandi) at suboptimal water temperature. Aquacult. Nutr. 21, 300–310. doi: 10.1111/anu.12160
Bendiksen, E. Å, Johnsen, C. A., Olsen, H. J., and Jobling, M. (2011). Sustainable aquafeeds: progress towards reduced reliance upon marine ingredients in diets for farmed Atlantic salmon (Salmo salar L.). Aquaculture 314, 132–139. doi: 10.1016/j.aquaculture.2011.01.040
Bian, F., Jiang, H., Man, M., Mai, K., Zhou, H., Xu, W., et al. (2016). Dietary gossypol suppressed postprandial TOR signaling and elevated ER stress pathways in turbot (Scophthalmus maximus L.). Am. J. Physiol.-Endoc. M. 312, E37–E47. doi: 10.1152/ajpendo.00285.2016
Bruusgaard, A., Sørensen, H., Gilhuus-Moe, C. C., and Skålhegg, B. A. (1977). Bile acid determination with different prepa-rations of 3α-hydroxysteroid dehydrogenase. Clin. Chim. Acta 77, 387–395. doi: 10.1016/0009-8981(77)90245-5
Chen, H., Cao, G., Chen, D., Wang, M., Vaziri, N. D., Zhang, Z., et al. (2016). Metabolomics insights into activated redox signaling and lipid metabolism dysfunction in chronic kidney disease progression. Redox Biology 10, 168–178. doi: 10.1016/j.redox.2016.09.014
González-Félix, M. L., Gatlin, D. M., Lawrence, A. L., and Perez-Velazquez, M. (2002). Effect of various dietary lipid levels on quantitative essential fatty acid requirements of juvenile pacific white shrimp Litopenaeus vannamei. J. World Aquacult. Soc. 33, 330–340. doi: 10.1111/j.1749-7345.2002.tb00509.x
He, Q., Kong, X., Wu, G., Ren, P., Tang, H., Hao, F., et al. (2008). Metabolomic analysis of the response of growing pigs to dietary l-arginine supplementation. Amino Acids 37:199. doi: 10.1007/s00726-008-0192-199
Jeromson, S., Gallagher, J. I., Galloway, D. S., and Hamilton, L. D. (2015). Omega-3 Fatty Acids and Skeletal Muscle Health. Marine Drugs 13, 6977–7004. doi: 10.3390/md13116977
Jin, Y., Tian, L., Xie, S., Guo, D., Yang, H., Liang, G., et al. (2015). Interactions between dietary protein levels, growth performance, feed utilization, gene expression and metabolic products in juvenile grass carp (Ctenopharyngodon idella). Aquaculture 437, 75–83. doi: 10.1016/j.aquaculture.2014.11.031
Johnson, C. H., Ivanisevic, J., and Siuzdak, G. (2016). Metabolomics: beyond biomarkers and towards mechanisms. Nat. Rev. Mol. Cell Biol. 17, 451–459. doi: 10.1038/nrm.2016.25
Joyce, S. A., MacSharry, J., Casey, P. G., Kinsella, M., Murphy, E. F., Shanahan, F., et al. (2014). Regulation of host weight gain and lipid metabolism by bacterial bile acid modification in the gut. Proc. Natl. Acad. Sci. U.S.A. 111:7421. doi: 10.1073/pnas.1323599111
Kitamura, M. (2011). Control of NF-κB and inflammation by the unfolded protein response. Int. Rev. Immunol. 30, 4–15. doi: 10.3109/08830185.2010.522281
Kitson, A. P., Metherel, A. H., Chen, C. T., Domenichiello, A. F., Trépanier, M., Berger, A., et al. (2016). Effect of dietary docosahexaenoic acid (DHA) in phospholipids or triglycerides on brain DHA uptake and accretion. J. Nutr. Biochem. 33, 91–102. doi: 10.1016/j.jnutbio.2016.02.009
Ko, E., Cho, S., Kwon, S., Eom, C., Jeong, M. S., Lee, W., et al. (2017). The roles of NF-κB and ROS in regulation of pro-inflammatory mediators of inflammation induction in LPS-stimulated zebrafish embryos. Fish Shellfish Immun. 68, 525–529. doi: 10.1016/j.fsi.2017.07.041
Kohlmeier, M. (1986). Direct enzymic measurement of glycerides in serum and in lipoprotein fractions. Clin. Chem. 32, 63–66. doi: 10.1093/clinchem/32.1.63
Kuhn, D. D., Lawrence, A. L., Crockett, J., and Taylor, D. (2016). Evaluation of bioflocs derived from confectionary food effluent water as a replacement feed ingredient for fishmeal or soy meal for shrimp. Aquaculture 454, 66–71. doi: 10.1016/j.aquaculture.2015.12.009
Li, C., Yeh, S., and Chen, J. (2010). Innate immunity of the white shrimp Litopenaeus vannamei weakened by the combination of a Vibrio alginolyticus injection and low-salinity stress. Fish Shellfish Immun. 28, 121–127. doi: 10.1016/j.fsi.2009.10.003
Li, E., Chen, L., Zeng, C., Chen, X., Yu, N., Lai, Q., et al. (2007). Growth, body composition, respiration and ambient ammonia nitrogen tolerance of the juvenile white shrimp, Litopenaeus vannamei, at different salinities. Aquaculture 265, 385–390. doi: 10.1016/j.aquaculture.2007.02.018
Li, E., Wang, X., Chen, K., Xu, C., Qin, J. G., and Chen, L. (2017a). Physiological change and nutritional requirement of Pacific white shrimp Litopenaeus vannamei at low salinity. Rev. Aquacult. 9, 57–75. doi: 10.1111/raq.12104
Li, T., Li, E., Suo, Y., Xu, Z., Jia, Y., Qin, J. G., et al. (2017b). Energy metabolism and metabolomics response of Pacific white shrimp Litopenaeus vannamei to sulfide toxicity. Aquat. Toxicol. 183, 28–37. doi: 10.1016/j.aquatox.2016.12.010
Li, X., Pang, L., Chen, Y., Weng, S., Yue, H., Zhang, Z., et al. (2013). Activating Transcription Factor 4 and X Box Binding Protein 1 of Litopenaeus vannamei Transcriptional Regulated White Spot Syndrome Virus Genes Wsv023 and Wsv083. PLoS One 8:e62603. doi: 10.1371/journal.pone.0062603
Liang, Z., Liu, R., Zhao, D., Wang, L., Sun, M., Wang, M., et al. (2016). Ammonia exposure induces oxidative stress, endoplasmic reticulum stress and apoptosis in hepatopancreas of pacific white shrimp (Litopenaeus vannamei). Fish Shellfish Immun. 54, 523–528. doi: 10.1016/j.fsi.2016.05.009
Litman, B. J., Niu, S., Polozova, A., and Mitchell, D. C. (2001). The role of docosahexaenoic acid containing phospholipids in modulating G protein-coupled signaling pathways. J. Mol. Neurosci. 16, 237–242. doi: 10.1385/jmn:16:2-3:237
Ma, Q., Chen, Q., Shen, Z., Li, D., Han, T., Qin, J., et al. (2017). The metabolomics responses of Chinese mitten-hand crab (Eriocheir sinensis) to different dietary oils. Aquaculture 479, 188–199. doi: 10.1016/j.aquaculture.2017.05.032
Maulucci, G., Cohen, O., Daniel, B., Sansone, A., Petropoulou, P. I, Filou, S., et al. (2016). Fatty acid-related modulations of membrane fluidity in cells: detection and implications. Free Radic. Res. 50, S40–S50. doi: 10.1080/10715762.2016.1231403
Navarrete, P., Fuentes, P., De la Fuente, L., Barros, L., Magne, F., Opazo, R., et al. (2013). Short-term effects of dietary soybean meal and lactic acid bacteria on the intestinal morphology and microbiota of Atlantic salmon (Salmo salar). Aquacult. Nutr. 19, 827–836. doi: 10.1111/anu.12047
Olsen, R. L., and Hasan, M. R. (2012). A limited supply of fishmeal: impact on future increases in global aquaculture production. Trends Food Sci. Tech. 27, 120–128. doi: 10.1016/j.tifs.2012.06.003
O’Shea, K. M., Khairallah, R. J., Sparagna, G. C., Xu, W., Hecker, P. A., Robillard-Frayne, I., et al. (2009). Dietary ω-3 fatty acids alter cardiac mitochondrial phospholipid composition and delay Ca2+-induced permeability transition. J. Mol. Cell. Cardiol. 47, 819–827. doi: 10.1016/j.yjmcc.2009.08.014
Putri, S. P., Nakayama, Y., Matsuda, F., Uchikata, T., Kobayashi, S., Matsubara, A., et al. (2013). Current metabolomics: practical applications. J. Biosci. Bioeng. 115, 579–589. doi: 10.1016/j.jbiosc.2012.12.007
Qian, Y., Peng, Y., Shang, E., Zhao, M., Yan, L., Zhu, Z., et al. (2017). Metabolic profiling of the hepatotoxicity and nephrotoxicity of Ginkgolic acids in rats using ultra-performance liquid chromatography-high-definition mass spectrometry. Chem.-Biol. Interact. 273, 11–17. doi: 10.1016/j.cbi.2017.05.020
Qiu, W., Zhang, S., Chen, Y., Wang, P., Xu, X., Li, C., et al. (2014). Litopenaeus vannamei NF-κB is required for WSSV replication. Dev. Comp. Immunol. 45, 156–162. doi: 10.1016/j.dci.2014.02.016
Rezzi, S., Collino, S., Goulet, L., and Martin, F. (2013). Metabonomic approaches to nutrient metabolism and future molecular nutrition. TrAC Trends Anal. Chem. 52, 112–119. doi: 10.1016/j.trac.2013.09.004
Rindlisbacher, B., Schmid, C., Geiser, T., Bovet, C., and Funke-Chambour, M. (2018). Serum metabolic profiling identified a distinct metabolic signature in patients with idiopathic pulmonary fibrosis – a potential biomarker role for LysoPC. Resp. Res. 19:7. doi: 10.1186/s12931-018-0714-712
Rosas, C., Cuzon, G., Gaxiola, G., Pascual, C., Taboada, G., Arena, L., et al. (2002). An energetic and conceptual model of the physiological role of dietary carbohydrates and salinity on Litopenaeus vannamei juveniles. J. Exp. Mar. Biol. Ecol. 268, 47–67. doi: 10.1016/S0022-0981(01)00370-377
Sharp, P. (1972). Interference in glucoseoxidase-peroxidase blood glucose methods. Clin. Chim. Acta 40, 115–120. doi: 10.1016/0009-8981(72)90257-4
Sinha, A. K., Romano, N., Allen, K. M., Bowman, B. A., Thompson, K. R., and Tidwell, J. H. (2018). Metabolism and Nutritive Role of Cholesterol in the Growth, Gonadal Development, and Reproduction of Crustaceans AU - Kumar, Vikas. Rev. Fish. Sci. Aquacult. 26, 254–273. doi: 10.1080/23308249.2018.1429384
Skiba-Cassy, S., Geurden, I., Panserat, S., and Seiliez, I. (2016). Dietary methionine imbalance alters the transcriptional regulation of genes involved in glucose, lipid and amino acid metabolism in the liver of rainbow trout (Oncorhynchus mykiss). Aquaculture 454, 56–65. doi: 10.1016/j.aquaculture.2015.12.015
Staley, C., Weingarden, A. R., Khoruts, A., and Sadowsky, M. J. (2017). Interaction of gut microbiota with bile acid metabolism and its influence on disease states. Appl. Microbiol. Biot. 101, 47–64. doi: 10.1007/s00253-016-8006-8006
Stillwell, W., and Wassall, S. R. (2003). Docosahexaenoic acid: membrane properties of a unique fatty acid. Chem. Phys. Lipids 126, 1–27. doi: 10.1016/S0009-3084(03)00101-104
Tacon, A. G., and Metian, M. (2008). Global overview on the use of fish meal and fish oil in industrially compounded aquafeeds: trends and future prospects. Aquaculture 285, 146–158. doi: 10.1016/j.aquaculture.2008.08.015
Tam, A. B., Mercado, E. L., Hoffmann, A., and Niwa, M. (2012). ER Stress Activates NF-κB by Integrating Functions of Basal IKK Activity, IRE1 and PERK. PLoS One 7:e45078. doi: 10.1371/journal.pone.0045078
Turner, N., Else, P. L., and Hulbert, A. J. (2003). Docosahexaenoic acid (DHA) content of membranes determines molecular activity of the sodium pump: implications for disease states and metabolism. Naturwissenschaften 90, 521–523. doi: 10.1007/s00114-003-0470-z
Vandesompele, J., De Preter, K., Pattyn, F., Poppe, B., Van Roy, N., De Paepe, A., et al. (2002). Accurate nor-malization of real-time quantitative RT-PCR data by geometric averaging of multiple internal control genes. Genome Biol. 3, h31–h34. doi: 10.1186/gb-2002-3-7-research0034
Wang, H., Zhou, J., Liu, Q. Z., Wang, L. L., and Shang, J. (2017). Simvastatin and Bezafibrate ameliorate Emotional disorder Induced by High fat diet in C57BL/6 mice. Sci. Rep. 7:2335. doi: 10.1038/s41598-017-02576-2575
Wang, L., and Chen, J. (2005). The immune response of white shrimp Litopenaeus vannamei and its susceptibility to Vibrio alginolyticus at different salinity levels. Fish Shellfish Immun. 18, 269–278. doi: 10.1016/j.fsi.2004.07.008
Wang, Q., He, G., Mai, K., Xu, W., and Zhou, H. (2016). Fishmeal replacement by mixed plant proteins and maggot meal on growth performance, target of rapamycin signalling and metabolism in juvenile turbot (Scophthalmus maximus L.). Aquacult. Nutr. 22, 752–758. doi: 10.1111/anu.12296
Wei, Y., Liang, M., Mai, K., Zheng, K., and Xu, H. (2017). The effect of ultrafiltered fish protein hydrolysate levels on the liver and muscle metabolic profile of juvenile turbot (Scophthalmus maximus L.) by 1H NMR-based metabolomics studies. Aquac. Res. 48, 3515–3527. doi: 10.1111/are.13178
Wu, D., Ye, Y., and Zhang, B. (2009). Effects of cotton seed meal and rapeseed meal on growth performance, non-specific immune indexes and body compositions of Litopenaeus vannamei [J]. China Feed 23:012.
Xie, S., Liu, Y., Zeng, S., Niu, J., and Tian, L. (2016). Partial replacement of fish-meal by soy protein concentrate and soybean meal based protein blend for juvenile Pacific white shrimp. Litopenaeus vannamei. Aquaculture 464, 296–302. doi: 10.1016/j.aquaculture.2016.07.002
Xie, S., Niu, J., Zhou, W., Liu, Y., and Tian, L. (2018). Developing a low fishmeal diet for juvenile Pacific white shrimp, Litopenaeus vannamei, using the nutritional value of FM as the reference profile. Aquacult. Nutr. 24, 1184–1197. doi: 10.1111/anu.12657
Xie, S., Tian, L., Jin, Y., Yang, H., Liang, G., and Liu, Y. (2014). Effect of glycine supplementation on growth performance, body composition and salinity stress of juvenile Pacific white shrimp, Litopenaeus vannamei fed low fishmeal diet. Aquaculture 418, 159–164. doi: 10.1016/j.aquaculture.2013.10.023
Xie, S., Wei, D., Tan, B., Liu, Y., Tian, L., and Niu, J. (2020). Schizochytrium limacinum Supplementation in a Low Fish-Meal Diet Improved Immune Response and Intestinal Health of Juvenile Penaeus monodon. Front. Physiol. 11:613. doi: 10.3389/fphys.2020.00613
Xie, S., Wei, D., Yin, P., Zheng, L., Guo, T., Liu, Y., et al. (2019). Dietary replacement of fish-meal impaired protein synthesis and immune response of juvenile Pacific white shrimp, Litopenaeus vannamei at low salinity. Comp. Biochem. Physiol. Part B 228, 26–33. doi: 10.1016/j.cbpb.2018.11.002
Xu, C., Li, E., Liu, Y., Wang, S., Wang, X., Chen, K., et al. (2018). Effect of dietary lipid level on growth, lipid metabolism and health status of the Pacific white shrimp Litopenaeus vannamei at two salinities. Aquacult. Nutr. 24, 204–214. doi: 10.1111/anu.12548
Xu, D., He, G., Mai, K., Zhou, H., Xu, W., and Song, F. (2016). Postprandial nutrient-sensing and metabolic responses after partial dietary fishmeal replacement by soyabean meal in turbot (Scophthalmus maximus L.). Brit. J. Nutr. 115, 379–388. doi: 10.1017/S0007114515004535
Ye, J. D., Wang, K., Li, F. D., Sun, Y. Z., and Liu, X. H. (2011). Incorporation of a mixture of meat and bone meal, poultry by-product meal, blood meal and corn gluten meal as a replacement for fish meal in practical diets of Pacific white shrimp Litopenaeus vannamei at two dietary protein levels. Aquacult. Nutr. 17, 337–347. doi: 10.1111/j.1365-2095.2010.00768.x
Ye, L., Jiang, S., Zhu, X., Yang, Q., Wen, W., and Wu, K. (2009). Effects of salinity on growth and energy budget of juvenile Penaeus monodon. Aquaculture 290, 140–144. doi: 10.1016/j.aquaculture.2009.01.028
Ye, S., Tan, L., Ma, J., Shi, Q., and Li, J. (2010). Polyunsaturated docosahexaenoic acid suppresses oxidative stress induced endothelial cell calcium influx by altering lipid composition in membrane caveolar rafts. Prostaglandins Leukotrienes Essential Fatty Acids 83, 37–43. doi: 10.1016/j.plefa.2010.02.002
Yuan, K., He, H., Zhang, C., Li, X., Weng, S., He, J., et al. (2017). Litopenaeus vannamei activating transcription factor 6 alpha gene involvement in ER-stress response and white spot symptom virus infection. Fish Shellfish Immun. 70, 129–139. doi: 10.1016/j.fsi.2017.09.013
Yue, Y. R., Liu, Y. J., Tian, L. X., Gan, L., Yang, H. J., and Liang, G. Y. (2012). Effects of replacing fish meal with soybean meal and peanut meal on growth, feed utilization and haemolymph indexes for juvenile white shrimp Litopenaeus vannamei. Boone. Aquac. Res. 43, 1687–1696. doi: 10.1111/j.1365-2109.2011.02976.x
Zeng, C., Wen, B., Hou, G., Lei, L., Mei, Z., Jia, X., et al. (2017). Lipidomics profiling reveals the role of glycerophospholipid metabolism in psoriasis. GigaScience 6, 1–11.
Zhang, J., Liu, Y., Tian, L., Yang, H., Liang, G., and Xu, D. (2012). Effects of dietary mannan oligosaccharide on growth performance, gut morphology and stress tolerance of juvenile Pacific white shrimp, Litopenaeus vannamei. Fish Shellfish Immun. 33, 1027–1032. doi: 10.1016/j.fsi.2012.05.001
Zhao, J., Shi, P., Sun, Y., Sun, J., Dong, J., Wang, H., et al. (2015). DHA protects against experimental colitis in IL-10-deficient mice associated with the modulation of intestinal epithelial barrier function. Brit. J. Nutr. 114, 181–188. doi: 10.1017/S0007114515001294
Keywords: endoplasmic reticulum stress, immune response, lipid metabolism, metabolomics, sterol
Citation: Xie S, Liu Y, Tian L, Niu J and Tan B (2020) Low Dietary Fish Meal Induced Endoplasmic Reticulum Stress and Impaired Phospholipids Metabolism in Juvenile Pacific White Shrimp, Litopenaeus vannamei. Front. Physiol. 11:1024. doi: 10.3389/fphys.2020.01024
Received: 14 May 2020; Accepted: 27 July 2020;
Published: 18 August 2020.
Edited by:
Enric Gisbert, Institute of Agrifood Research and Technology (IRTA), SpainReviewed by:
Mansour Torfi Mozanzadeh, South Iran Aquaculture Research Center, IranGuangxu Liu, Zhejiang University, China
Copyright © 2020 Xie, Liu, Tian, Niu and Tan. This is an open-access article distributed under the terms of the Creative Commons Attribution License (CC BY). The use, distribution or reproduction in other forums is permitted, provided the original author(s) and the copyright owner(s) are credited and that the original publication in this journal is cited, in accordance with accepted academic practice. No use, distribution or reproduction is permitted which does not comply with these terms.
*Correspondence: Shiwei Xie, eHN3enNkeEAxNjMuY29t; Jin Niu, bml1ajNAbWFpbC5zeXN1LmVkdS5jb20=