- 1Aquaculture Graduate Program, Nilton Lins University, Manaus, Brazil
- 2Laboratory of Ecophysiology and Molecular Evolution, National Institute of Amazonian Research, Manaus, Brazil
The control of abiotic parameters is fundamental for fish survival, growth and reproduction. These factors have a direct effect on sperm quality. Thus, this study evaluated the effect of different temperatures (29, 31, 33, and 35°C), pHs (4 and 8), and hypoxia (1 mgO2 L−1) on sperm motility of Colossoma macropomum (tambaqui). The results indicated a longer duration of sperm motility at 29°C (50.1 ± 2.70 s) that progressively decreased when exposed to 35°C (31.2 ± 1.31 s) and hypoxia at pH 4 (27.4 ± 1.42 s) and pH 8 (30.44 ± 1.66 s; p < 0.05), respectively. Sperm oxygen consumption increased in hypoxia at both pH (pH 4 = 61.22; pH 8 = 54.74 pmol s−1). There was an increase in the activity of glutathione-S-transferase (GST) and superoxide dismutase (SOD), as well as in lipid peroxidation levels (LPO) and DNA damage in sperm exposed to higher temperatures and hypoxia. The pH 4 and pH 8 under normoxia did not affect the quality of C. macropomum sperm. These results suggest that water warming and acidification, consequences of climate changes, significantly affect the reproduction of C. macropomum, reducing the quality of spermatozoids during fertilization.
Introduction
Climate changes are universal and are causing unprecedented environmental and life damage. Among the changes are higher temperatures, increases in precipitation rates, extreme drought, and changes in the habitat of terrestrial and aquatic species, which can result in reallocation and adaptation to survival (Isaak et al., 2012). Freshwater species face particular challenges within this scenario, worsened by increased pollution and over-exploitation of commercial fisheries, leading to declining fish populations (Wenger et al., 2011), that is, elimination of sensitive species, through direct and indirect effects on the biology of these organisms (Jeppesen et al., 2010), as well as on sperm quality and viability (Jenkins et al., 2018). Thereby, in order to minimize the impacts caused by fishing and climate change in natural environments, aquaculture is becoming increasingly important for the maintenance of fish stocks and for animal protein production. Therefore, targeted efforts to assess how fish in confined environments will be affected by the climate change in terms of reproductive efficiency are needed.
Dissolved oxygen (DO), pH, and temperature are important factors affecting survival, growth, and reproduction of fish (Caldwell and Hinshaw, 1994). In the Amazon region, these factors are influenced by diurnal variations of DO, seasonal flooding, thermal stratification, aquatic plants, and anthropogenic effects, such as eutrophication and global warming (Richards et al., 2009). The control of these factors is pivotal for the reproductive success of fish that have external fertilization (Dong et al., 2011), since sperm activity is only triggered when it comes into contact with water (Alavi and Cosson, 2006). Thus, sperm motility is strongly influenced by the activation medium (Dadras et al., 2016).
Sperm motility is a key factor determining semen quality being directly related to fertilization success, and is potentially used as a biomarker of environmental quality (Gallego et al., 2018). Negative effect of hypoxia, temperature, and pH on the spermatic quality of Porichthys notatus, Sparus aurata (Lahnsteiner and Caberlotto, 2012), and Genypterus blacodes (Dumorné et al., 2018) have been documented. The duration of sperm motility of farmedfish species is approximately 1 min; for this reason, accurate assessments using rapid and sensitive methods are needed to increase the efficiency of artificial fertilization (Kime et al., 2001; Rurangwa et al., 2004).
The motility and fertilization capacity of sperm are dependent on energy stored in the form of ATP, which in turn is strongly influenced by the oxygen concentration (Bencic et al., 1999). However, molecular oxygen, although necessary, can induce the production of reactive oxygen species (ROS), causing oxidative stress. During periods of environmental stress to which sperm are subjected at the time of activation, several antioxidant enzymes act to neutralize ROS (Dadras et al., 2016). The antioxidant capacity of semen can vary among fish species (Slowinska et al., 2013) and the formation of ROS can cause different types of damage, in particular lipid peroxidation of membranes, due to the abundance of polyunsaturated fatty acids, and DNA damage, with consequent loss of motility and decreased fertilization (Aramli and Kalbassi, 2016).
The tambaqui, Colossoma macropomum (Cuvier, 1818), is a migratory tropical fish species native from the Amazon and Orinoco basins (Araujo-Lima and Goulding, 1998), belonging to family Serrasalmidae (Mirade, 2010). The spawning of tambaqui coincides with the beginning of the flood, occurring in October/November and the species presents annual and total spawning. Mature individuals of C. macropomum have high fecundity, releasing more than one million oocytes. The fertilization is external and the species does not show parental care (Araujo-Lima and Goulding, 1998; Vieira et al., 1999). At the beginning of the flood season, individuals start their upstream migration into whitewater rivers, and when the gonads are fully developed they spawn along the grassy leaves that are being inundated with rising water. After spawning, the fish enter the floodplain areas where they feed mainly on fruits (Goulding and Carvalho, 1982;Araujo-Lima and Goulding, 1998).
This species is of great interest for researchers and producers due to its acclimatization facility, rapid growth, and great social and economic importance for the central region of the Amazon (Mendonça et al., 2009). Because it is a tropical species, C. macropomum is expected to be especially vulnerable to climate change, specifically to warming, because it has a narrower thermal sensitivity range compared to subtropical and temperate species and tends to live closer to its thermal limits (Lapointe et al., 2018). Therefore, even small temperature increases can lead to declining performance of individuals and populations (Tewksbury et al., 2008).
Considering that homeostasis of the sperm of C. macropomum is extremely important for the aquaculture industry, understanding how environmental parameters dependent on climate change affect sperm quality is critical in improving the methods and manipulations required for successful in vitro fertilization. Thus, the present study aims to investigate in vitro effects of temperature, pH and hypoxia on motility and antioxidant responses of the sperm of the Amazonian fish C. macropomum.
Materials and Methods
Semen Collection
Males of C. macropomum (n = 3, 60.4 ± 0.36 cm, 4.13 ± 0.05 kg) were selected from 600 m2 nurseries at the Center for Technology, Training and Production in Aquaculture (CTTPA; Balbina, Presidente Figueiredo, Amazonas – 1°55′54.4"S; 59°24′39.1"W). The fish were randomly selected for a light abdominal massage and in cranium-caudal direction, and those who released semen were chosen. The fish were recovered from this initial handling in 1000 L tanks with oxygen 5.54 ± 0.22 mg L−1, temperature 29.2 ± 0.07°C, and pH 7.73 ± 0.28 for a period of 6 h. Subsequently, spermiation was induced by intraperitoneal injection of crude extract of carp pituitary hormone (EBHC). The procedure was performed by injecting two boluses of EBHC. The first bolus was 0.5 mg/kg EBHC, and after an interval of 12 h, a second bolus of 1.0 mg/kg EBHC was injected. Six hours after injection of second hormone bolus, the semen was collected in graduated Falcon tubes, avoiding contact with water, blood, feces, or urine, thus preventing sperm activation. From each animal, 4 ml of semen was collected and immediately diluted 1:10 (50 μl semen: extender 450 μl) in Beltsville Thawing Solution (BTS-MINITUB®) and refrigerated at 4°C to be transported to the laboratory Ecophysiology and Molecular Evolution (LEEM) of the Brazilian National Institute for Research of the Amazon (INPA). All procedures and experimental manipulations used in this study were performed according to the Brazilian Guidelines for Animal Care and were approved by the Ethics Committee for the use of Animals of INPA, protocol number 004/2018.
Activation
For activation of sperm motility, the following treatments were tested: temperature (29, 31, 33, and 35°C), pH (4 and 8), and interaction between pH and hypoxia (1 mgO2·L−1) in controlled activator solution (distilled water-0 mOsm kg−1). The different temperatures were maintained using thermostats HOPAR model (Aquarium Heater H-606). The pHs 4 and 8 were achieved by adding HCl 1% and NaOH 1%, respectively. The pH values were then monitored using a digital pH meter (Ohaus, Starter 3100). Hypoxia was adjusted with the addition of gaseous nitrogen until the concentration of 1 mgO2 L−1. The sperm were activated in the proportion 2:20 (v:v), in a closed environment with controlled temperature (23°C). Immediately after activation, sperm were observed under an optical microscope (Leica DM500; 40x), by the same observer to avoid the subject bias of the analyzes. The motility time was monitored with the aid of a timer (s) being evaluated from the start of movement until 100% sperm immobility. To identify the percent of sperm motility, a scale from 0 to 100% was used, according to Cosson et al. (2008), where: 1 = 0–5%, 2 = 5–25%, 3 = 25–50%, 4 = 50–75%, 5 = 75–100%. For each treatment, nine replicates were performed in triplicate.
Oxygen Consumption of Sperm
Oxygen consumption of sperm was monitored using a high-resolution respirometer (Oxygraph-2 K, Oroboros Instruments) equipped with a Peltier thermostat and an electromagnetic stirrer. The treatments (temperature, hypoxia, and pH) were controlled within two 2 ml closed Oxygraph chambers. With the aid of injection cannulas, 100 μl of semen were added, immediately activated inside the chambers. Oxygen consumption was recorded immediately after activation until cessation of sperm respiration. Recordings of new samples were performed at intervals of 5 min between exposures, to the complete stabilization of the equipment. Data are expressed as pmol·s−1·cm−3.
Biochemical Analysis
For analysis of antioxidant enzymes, 200 μl of semen from each fish was activated, with five replicates in triplicate for each treatment and after motility cessation, it was immediately immersed in liquid nitrogen. The semen samples were homogenized in a buffer solution (Tris base 200 mM, ethylene diamine tetraacetic acid (EDTA) 1 mM, dichloro-diphenyl-trichlorethane (DDT) 1 mM, sucrose 500 mM, KCl 150 mM, and pH 7.6) and centrifuged at 9,000 g for 10 min at 4°C. The supernatant was used for analysis of the activity of glutathione S-transferase enzyme (GST), superoxide dismutase (SOD) and catalase (CAT), and for determination of lipid peroxidation (LPO) levels. GST activity was determined as described by Keen et al. (1976), considering absorbance changes at 340 nm using 1-chloro-2,4-dinitrobenzene (CDNB) as the substrate. GST activity was calculated as nmol of conjugate CDNB·min−1·mg−1 protein, using the molar extinction coefficient 9.6 mM·cm−1. SOD activity was quantified from the inhibition of the reduction rate of cytochrome c influenced by the xanthine/xanthine oxidase system at 550 nm (Flohé and Otting, 1984) and is expressed as U min−1·mg of protein−1. CAT activity was determined following the method of Beutler (1975) and is expressed as μmol H2O2·min−1·mg−1 protein. The inhibition rate of H2O2 decomposition was monitored by decreasing the absorbance at 240 nm. LPO levels were measured by the ferrous oxidation/xylenol orange (FOX) method as described by Jiang et al. (1991) which consists of the oxidation of Fe+2 to Fe+3 hydroperoxide in an acid medium and subsequent formation of Fe3+/xylenol orange in the presence of the stabilizer butylate hydroxytoluene (BHT). The formation of this complex was quantified by increased absorption at 560 nm and is expressed in μM cumene hydroperoxide (CHP)·mg−1 of sperm protein. Total sperm protein was determined spectrophotometrically at 595 nm, according to the colorimetric assay described by Bradford (1976) using bovine serum albumin (BSA) as standard. All assays were performed with a SpectraMax M2 spectrophotometer, Molecular Devices Inc., Sunnyvale, CA, USA.
Genotoxic Damage
To verify the DNA integrity of C. macropomum sperm cells, unicellular gel electrophoresis (comet assay) was used, according to Cabrita et al. (2005). After experimental exposures, 5 μl of semen was added to 300 μl of RPMI. Previously, the experimental units were covered with 0.5% agarose and all procedures were completed in triplicate. After agarose drying, the slides were placed in the lysis solution (2.5 M NaCl, 100 mM EDTA, 10 mM Tris, pH 10–10.5, 1% Triton X-100, and 10% DMSO) for 90 min at 4°C. The slides were then submerged in alkaline sodium hydroxide and EDTA buffer (300 mM NaOH and 1 mM EDTA, pH > 13) for 20 min. The slides were electrophoresed for 20 min at 25 V, 300 mA, and 4°C. Subsequently, the slides were neutralized (0.4 M Tris, pH 7.5 at 4°C) and stained with silver nitrate solution (5% sodium carbonate, 0.1% ammonium nitrate, 0.1% silver, 0.25% tungstosilicic acid, and 0.15% formaldehyde) for 15 min at 37°C. A total of 100 spermatozoids were randomly quantified per slide, using an optical microscope (Leica DM205) with a magnification of 100x. Damage was classified according the size of the tail that represents the degree of fragmentation of the DNA. DNA fragments of damaged nucleus migrate when exposed to an electrical field (electrophoresis). Fragments of different sizes migrate at different speeds, forming the typical figure of a comet. Thus, during the electrophoretic run, the more intense the breaks in the DNA molecule, the smaller the fragments generated and the greater the extent of migration and so the comet tail (Singh et al., 1988; Collins et al. 2008). The cells were divided into five categories, according to tail size, ranging from 0 to 4, i.e., class 0 corresponds to intact DNA, without tail; class 1, low damage index; class 2, intermediate damage; class 3, high damage; and class 4, extreme damage.
Statistical Analysis
Data are expressed as mean ± SEM. To compare the different treatments, an ANOVA one way, followed by Tukey’s post-hoc test when differences (p < 0.05) between means were detected. Data that did not meet the premises of linear models were log transformed. All analysis was performed using the R-3.5.1 software (R Core Team, 2018), with additional car package (Fox and Weisberg, 2011).
Results
Sperm Motility
The duration of C. macropomum sperm motility was 50.1 ± 2.7 s at 29°C and significantly reduced (p < 0.05) at 33°C (33.66 ± 2.58 s) and 35°C (31.22 ± 1.31 s; Figure 1). The percentage of mobile cells showed no differences at 29 and 31°C, but decreased significantly at 33 and 35°C (Figure 1). Exposure to pH 4 and pH 8 in hypoxia caused a significant reduction in motility time and percentage of mobile sperm (p < 0.05; Figure 2). Motility time and percentage of mobile sperm showed no differences when exposed to pH 4 and pH 8 in normoxia (p > 0.05; Figure 2).
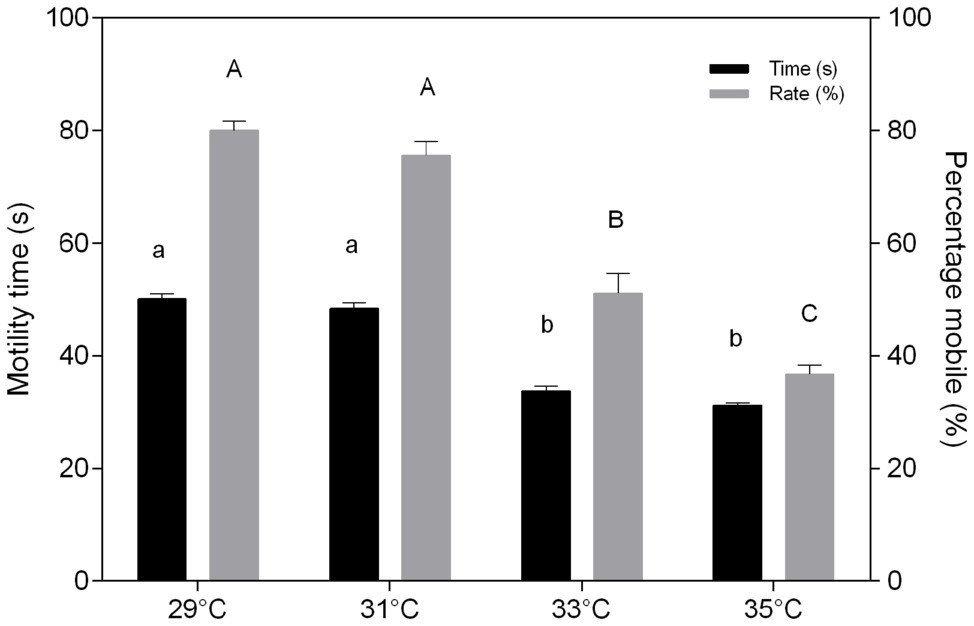
Figure 1. In vitro effect of temperature on time (s) and percentage mobile (%) of sperm of Colossoma macropomum. Data are presented as mean ± SEM. Lowercase letters indicate difference in motility time between treatments. Uppercase letters indicate differences in motility rate between treatments (p < 0.05).
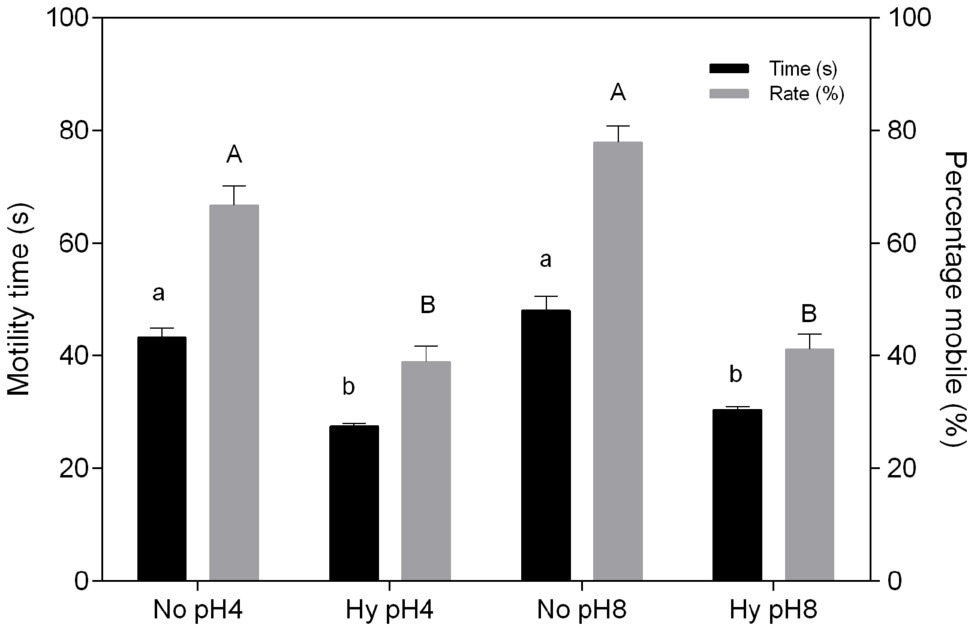
Figure 2. In vitro effect of pH and hypoxia (Hy) on time (s) and percentage mobile (%) of sperm of C. macropomum. Data are presented as mean ± SEM. Lowercase letters indicate differences in motility time between Hy and normoxia (No). Uppercase letters indicate differences in motility rate between Hy and No (p < 0.05).
Oxygen Consumption of Sperm
The oxygen consumption of sperm was not altered with increasing temperatures (p > 0.05; Figure 3A) or under different pHs within the same treatment (Figure 3B). However, exposure to hypoxia resulted in an increase in oxygen consumption at both pH 4 (61.22 pmol s−1) and pH 8 (54.74 pmol s−1) compared to normoxia at pH 4 (14.20 pmol s−1) and pH 8 (10.59 pmol s−1; p < 0.05; Figure 3B).
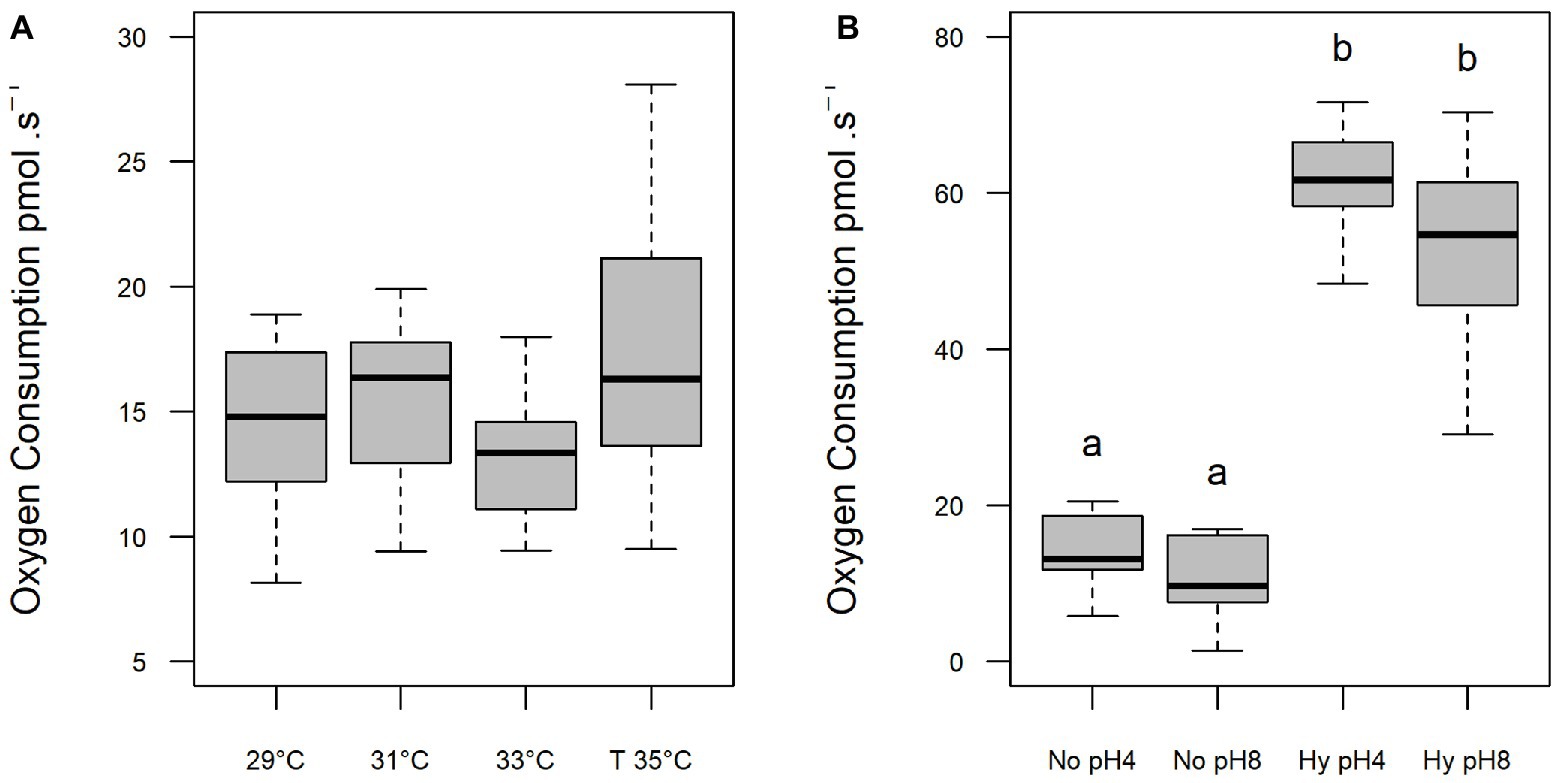
Figure 3. Oxygen consumption (pmol⋅s−1) of C. macropomum sperm activated at different in vitro temperatures (A) and at pH 4 and pH 8 in No (5.5 mgO2/L) and Hy (1 mgO2/L). (B) Lowercase letters indicate differences between treatments.
Biochemical Analysis
Activities of GST, SOD, and CAT and levels of LPO of C. macropomum sperm exposed to different temperatures are shown in Figure 4. GST activity was increased at 35°C, differing from sperm exposed to 33°C (p < 0.05), and similar to other treatments (p > 0.05; Figure 4A). SOD activity was significantly increased at 35°C compared to all other treatments (p < 0.05; Figure 4B). CAT did not differ in any of the treatments (p > 0.05; Figure 4C). Exposure to 35°C caused an increase in LPO levels compared to cells exposed to 29 and 33°C, while for sperm exposed to 33°C LPO levels were reduced compared to other groups (p < 0.05; Figure 4D). Low oxygen concentration exposure during sperm activation caused an increase of GST and SOD activities (Figures 5A,B). CAT activity at pH 8 in normoxia was equal to pH 4 normoxia (p > 0.05) and showed a similar not significant increase of sperm exposed to hypoxia at both pHs (p < 0.05; Figure 5C). Increased enzymatic activities in hypoxic environments contributed to increased LPO levels (Figure 5D). Normoxic environments at pHs 4 and 8 did not affect the activities of SOD and GST, as well as the LPO levels (p > 0.05; Figure 5).
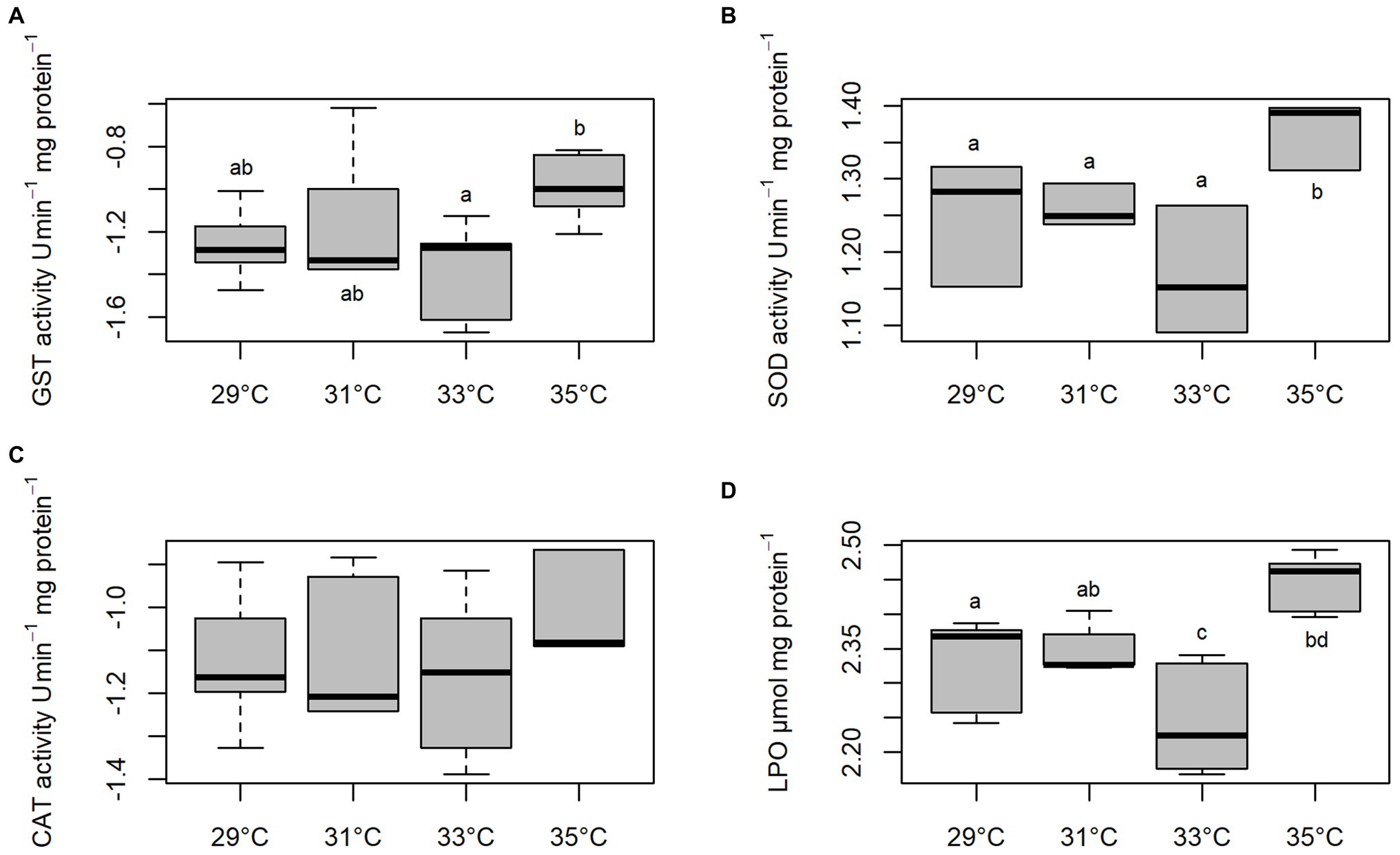
Figure 4. In vitro effect of temperature on Glutathione-S-transferase (GST), (A) superoxide dismutase (SOD), (B) catalase (CAT), (C) and lipoperoxidation (LPO) (D) levels in sperm of C. macropomum. Lowercase letters indicate differences between treatments.
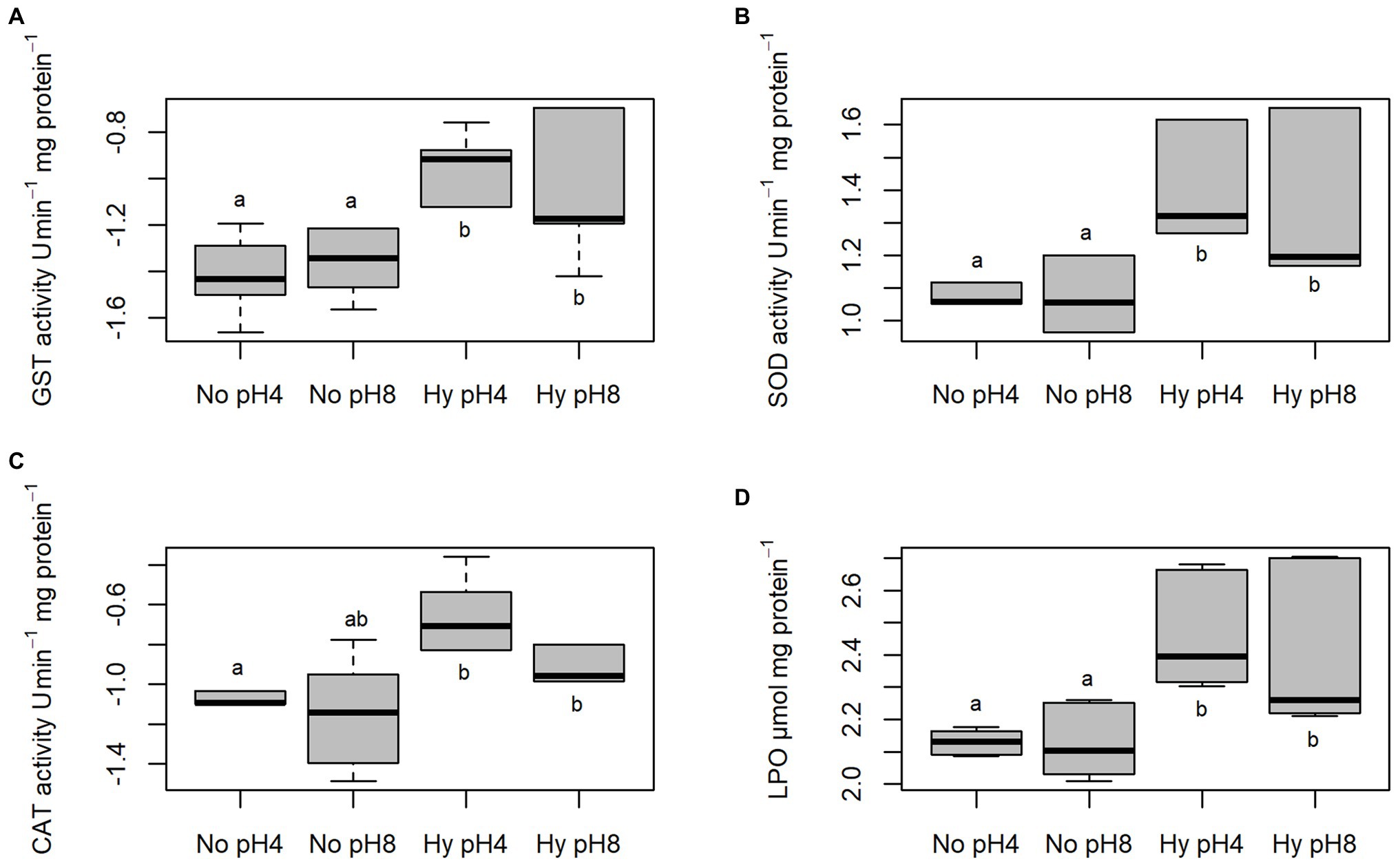
Figure 5. In vitro effect of pH in No (5.5 mgO2/L) and Hy (1 mgO2/L) on GST, (A) SOD, (B) CAT, and (C) LPO, (D) in sperm of C. macropomum. Lowercase letters indicate differences between treatments.
Genotoxic Damages
Genetic damage to sperm of C. macropomum increased at higher temperatures (33 and 35°C) compared to sperm activated at 29 and 31°C (Figure 6). At 29 and 31°C, there was a prevalence of intact DNA with about 71 and 66%, respectively. At the highest temperature, 35°C, approximately 60% of the sperm cells had extreme damage, class 4 (Figure 6). Activated spermatozoa under normoxia showed the highest rate of intact cells (pH 4 = 43%, pH 8 = 57%), but low oxygen activation medium on both pHs caused an increased classes 2, 3, and 4 damages, considered intermediate, high, and extreme, respectively (Figure 6).
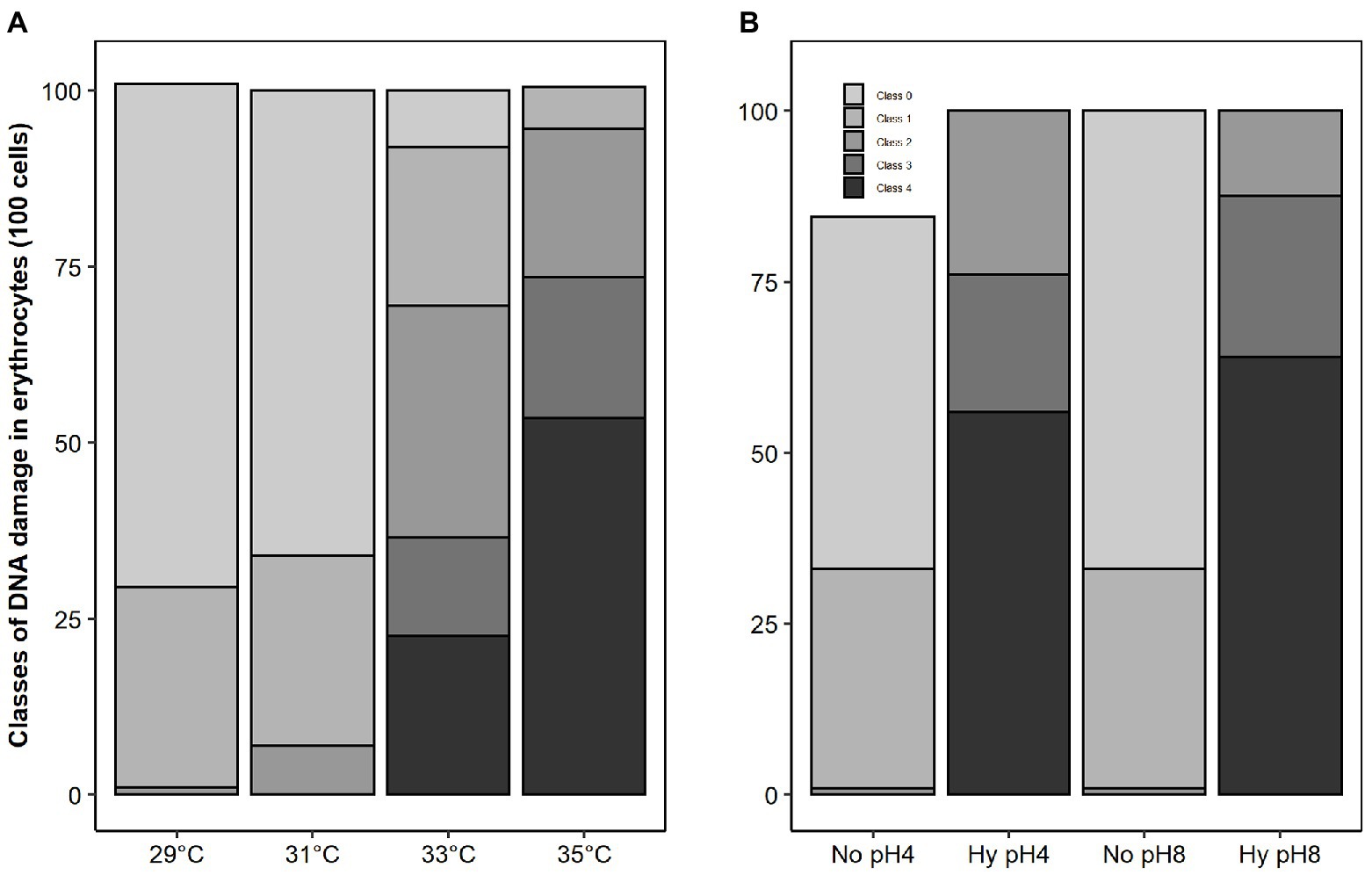
Figure 6. Frequency of DNA damage of sperm of C. macropomum exposed to different temperatures (A) and exposed to pH 4 and pH 8 in No and Hy (B) in vitro.
Discussion
Temperature Effects
Sperm motility, a critical semen characteristic, depends on activation media (Williot et al., 2000) that strongly influence the fertilization in teleosts (Bera et al., 2016). In the present study, sperm exposure to increased temperature (33 and 35°C) caused a reduction on time and percentage of mobile sperm (Figure 1) without altering the oxygen consumption (Figure 3A). These findings corroborate the report of Lahnsteiner and Caberlotto (2012) for S. aurata and Dadras et al. (2016) for Cyprinus carpio, which showed that higher temperatures caused lower percentage of mobile sperm. According to Purchase et al. (2010), fish sperm has limited energetic resources; thus, temperature increase causes a motility decrease due to the acceleration of the metabolic processes. Low temperatures reduce the intensity of flagellar movement significantly, prolonging motile duration of spermatozoids (Alavi and Cosson, 2006). In the present study, the high temperature accelerated the swimming speed of C. macropomum sperm cells, increasing the metabolic consumption of the cells and decreasing the motility time and the percentage of mobile cells. Activated sperm at low temperatures consume metabolic energies more slowly, increasing the duration of these cells, corroborating the report of Alavi and Cosson (2006), who found that low temperatures reduce the intensity of flagellar movement, significantly prolonging the mobile duration of sperm.
As a result, at lower temperatures, the consumption of ATP is reduced, which is directly related to the longer motility time (Lahnsteiner and Caberlotto, 2012). Dzyuba et al. (2019), studying the tropical species Oreochromis niloticus, showed that higher temperatures induce an increase in sperm motility velocity, which is accompanied by a reduction in motility time. The reduction in time and percentage of mobile sperm at the higher temperatures observed in the present study may be related to increased energy demands, which was not compensated for, since oxygen consumption remained constant at all temperatures. Therefore, our results suggest that semen from tambaqui has limited energy supply to deal with increased temperature, suggesting high vulnerability of this species under warming scenarios.
Another factor that may explain the lower percentage of mobile sperm observed at the higher temperature is related to the oxidative damages caused by ROS production, as observed in the present study. The exposure of sperm cells at 35°C resulted in an increase in the activity of antioxidant enzymes (SOD and GST; Figure 4); however, such increase was not enough to avoid damage to macromolecules, since there was an increase in lipoperoxidation and DNA damage (Figures 4, 6A). Oxidative stress can induce metabolic disorders that reduce sperm motility. As suggested by Aramli and Kalbassi (2016), oxidative stress causes disturbances in the sperm metabolism, which results in decreased sperm motility.
The oxidative damage observed in the present study suggests a inefficiency in the neutralization of ROS by the antioxidant defenses, since even with the increase of antioxidant enzyme activity, such as SOD, sperm presented high levels of lipoperoxidation and DNA damage. Aramli and Kalbassi (2016) also showed that the increase of the antioxidant defenses in the species Acipenser persicus was accompanied by the increase of oxidative damages. Fish sperm have characteristics that make them susceptible to damage related to oxidative stress, considering the high amount of polyunsaturated fatty acids of sperm membrane (Shaliutina et al., 2013).
Studies have shown that temperature increases potentially induce oxidative stress in different fish tissues (Wiens et al., 2017), including sperm cells, as presented in this paper with the increase in antioxidant enzymes, contributing to the increase in lipid peroxidation levels, as shown by Dadras et al. (2016) when evaluating the sperm of C. carpio, identifying that the lipid peroxidation in these cells is temperature sensitive. Lipids are probably used as a source of energy by sperm to increase motility duration (Baeza et al., 2015) that plays an important role in fertilization capacity (Gholami et al., 2011). The lipid peroxidation of these cells is associated with deterioration of membrane function, decreased fluidity, and increased non-specific permeability to ions and other transmembrane transport processes (Wong-Ekkabut et al., 2007).
Although C. macropomum sperm cells were activated at the high temperature evaluated in this experiment, they showed a significant increase in tail damage (class 4). The evaluation of fish sperm DNA damage is important for fish farming, since the loss of genetic information or the appearance of larval deformities is detrimental to future generations (Cabrita et al., 2005; Lewis and Galloway, 2009). Due to the reduced motility time of freshwater fish spermatozoid, the activation environment is critical for the successful delivery of paternal DNA to the egg (Gazo et al., 2015). However, oxidative stress is highly detrimental to DNA integrity, since this damage is not limited to the direct effect on the fragmentation of chromatin; it can also alter gene expression or induce epigenetic deregulation (Chervona and Costa, 2012). The extreme DNA damage of C. macropomum sperm caused by activation at high temperatures is similar to the results found by Lacaze et al. (2011) in assessing DNA damage of the sperm of Gammarus fossarum. The authors explain that the increase in damage is associated with an overall stress caused by increased temperature and decreased oxygen concentration. Our results corroborate the findings of De Andrade et al. (2004), who found an increase in DNA damage of Dreissena polymorpha and Netuma sp. exposed to 37°C. Despite increased DNA damage in various species of fish exposed to various stressors, Pérez-Cerezales et al. (2010) reported that DNA damaged spermatozoids are capable of performing fertilization and that the oocyte can repair this damage to some extent in Oncorhynchus mykiss. These authors stated that when the DNA fragmentation rate is high, the oocyte repair capacity is insufficient, and infertility rate increases considerably.
Effects of pH and Hypoxia
In the present study, activation media pHs (pH 4 and pH 8) did not influence the sperm quality of C. macropomum. This may be related to the adaptation of this species to large pH range that drives resilience of sperm cell to pH changes and so, possibly, increasing reproductive success. Aride et al. (2004) reported that in confined environments C. macropomum is able to tolerate large variations in pH, which was confirmed by Wood et al. (2018). Cosson (2004) showed that the pH of activating medium usually affects motility, but in small proportions. In addition, the external pH can reduce or prolong motility, but it is not the main environmental factor affecting initial motility (Cosson et al., 2008). The results found in this study are similar to the findings by PerchecPoupard et al. (1997), who showed reduced pH impact on carp sperm motility, indicating that fish that inhabit tropical/subtropical environments are possibly more resistant to pH variation. However, for species that inhabit temperate environments such as Samotrutta, variations in pH affect sperm motility (Dziewulska and Domagała, 2013).
Hypoxia exposure affected sperm quality of C. macropomum, suggesting that hypoxia, a common environmental condition in tropical waters and aquaculture, is highly detrimental to the reproduction of this tropical species. The results observed in the present study are in agreement with Wu et al. (2003), which suggested that hypoxia caused reduction of sperm motility in carp and other reproductive dysfunctions, such as the retardation of gametogenesis. Thomas et al. (2007) evaluated the formation of sperm cells of Micropogonias undulates and found that sperm production, gametogenesis and all stages of spermatogenesis were impaired in hypoxic environments.
The decrease in the time and percentage of mobile sperm of C. macropomum under hypoxic conditions was accompanied by an increase in oxygen consumption (Figure 3B). At low oxygen, sperm cells present an increase in the rate of flagellar motility, not measured in the present study, which resulted in decreased motility time due to the rapid consumption of ATP. As a result, oxygen consumption increased. Fitzpatrick et al. (2009) showed that sperm velocity of P. notatus exposed to hypoxia was positively related to oxygen consumption. Both species breed in hypoxic environments. During the breeding period, males of P. notatus are trapped in small pools facing drastic hypoxia, similar to C. macropomum in their natural environments. It is known that the mitochondria of fish sperm supply ATP demand aerobically (Dzyuba et al., 2017). According to Cosson (2010), as the ATP reserve is depleted along the spermatozoid activity, there is a gradual decrease of the flagellum movement, settling off the movement of the cell. In aquaculture, fish are commonly kept under artificial conditions, exposed to various adverse conditions, which may contribute to the establishment of hypoxic environments (Rurangwa et al., 2004). Therefore, sperm exposed in these environments consume more quickly ATP reserves, directly affecting the duration of motility and the efficiency of fertilization. In addition, recent studies suggest that changes in sperm quality may be related to changes at the molecular level, such as changes in gene expression or epigenetic factors. Wang et al. (2016) verified the occurrence of epigenetic changes in the sperm methyloma of Oryzias melastigma exposed to hypoxia, as well as differential expression of genes and proteins involved in spermatogenesis and gene silencing, which was associated with a reduction in sperm motility, even in generations that never had been exposed to hypoxic conditions.
We also observed that exposure to hypoxia caused an increase in antioxidant activity (SOD, CAT, and GST; Figure 5), which suggests an increased capacity to prevent ROS effects. However, despite the higher activity of antioxidant enzymes under hypoxia condition, there was an increase in lipoperoxidation levels and DNA damage. Thus, the oxidative damages observed in the present study may be related to loss of sperm quality under hypoxic conditions. It has been shown that hypoxia induces oxidative stress in different fish tissues (Mustafa et al., 2011). The results of our study are similar to those of Bera et al. (2016), evaluating the activity of antioxidant enzymes in the testes and ovary of C. carpio in hypoxic environments. The authors reported an increase in CAT and SOD activities compared to tissues under normoxia. Other authors report the relationship between loss of sperm quality and oxidative damage in fish sperm under different stress conditions (Dadras et al., 2016; Wiens et al., 2017). Shaliutina et al. (2013) also showed a relationship between loss of sperm quality and oxidative stress in Acipenser gueldenstaedtii and Acipenser bae. Taken together, these results suggest that tambaqui reproduction is especially sensitive to disturbances caused by exposure to hypoxia.
Conclusions
The elevation of temperature and the decrease in DO, factors associated among others to climate change, will negatively affect sperm quality of C. macropomum. No pH effects on spermatozoids of C. macropomum were observed. This study provides basic information for future research on sperm quality of tropical fish in confined environments. However, further investigations are needed to describe the mechanisms involved in metabolism and sperm motility in C. macropomum under warm hypoxic environments. In general, the present results suggest that C. macropomum sperm are vulnerable to warm hypoxic waters, conditions associated with global warming, and may impair the reproduction of the species.
Data Availability Statement
The datasets generated for this study are available on request to the corresponding author.
Ethics Statement
The animal study was reviewed and approved by Brazilian Guidelines for Animal Care and were approved by the Ethics Committee for the use of Animals of INPA, protocol number 004/2018.
Author Contributions
AV and JC designed the work. JC performed the experiment, collected data, analyzed and drafted the original version of the manuscript. SS, SB-M, and DC helped with laboratory analyzes, discussed and reviewed the manuscript and AV supervised this work, obtained financial support, discussed and revised the manuscript. All authors contributed to the article and approved the submitted version.
Funding
The Brazilian National Research Council (CNPq, 465540/2014-7), Amazonas State Research Foundation (FAPEAM, 0621187/2017) and Brazilian Coordination for the Improvement of Higher Education Personnel (CAPES) (88887.136340/2017-00) support the INCT ADAPTA. The CAPES – Finance Code 001 granted the fellowships.
Conflict of Interest
The authors declare that the research was conducted in the absence of any commercial or financial relationships that could be construed as a potential conflict of interest.
Abbreviations
ADAPTA, Adaptations of aquatic biota of the Amazon; ATP, Adenosine triphosphate; BHT, Butylate hydroxytoluene; BSA, Bovine serum albumin; BTS, Beltsville thawing solution; CAPES, Coordination for the improvement of higher education personnel; CDNB, 1-Chloro-2,4-dinitrobenzene; CHP, Cumene hydroperoxide; CNPq, Brazilian National Research Council; CTTPA, Center for Technology Training and Production in Aquaculture; DDT, Dichloro-diphenyl-trichlorethane; DMSO, Dimethyl sulfoxide; EBHC, Crude extract of carp pituitary hormone; EDTA, Ethylene diamine tetra-acetic acid; ROS, Reactive oxygen species; FAPEAM, Amazonas State Research Foundation; FOX, Ferrous oxidation/xylenol orange; GST, Glutathione S transferase; HCL, Hydrochloric acid; INPA, National Institute for Research of the Amazon; LEEM, Laboratory of Ecophysiology and Molecular Evolution; LPO, Lipoperoxidation; NaOH, Sodium hydroxide; SEM, Standard error of mean; SOD, Superoxide Dismutase.
Acknowledgments
JC, SB-M, and DC were recipients of post-grad fellowships from CAPES (finance code 001); SS was recipients of post-grad fellowships from FAPEAM; AV is the recipient of a research fellowship from CNPq (303930/2014-4). We would also like to thank the collaborators at the Balbina fish farm.
References
Alavi, S. M. H., and Cosson, J. (2006). Sperm motility in fishes. (II) Effects of ions and osmolality: a review. Cell Biol. Int. 30, 1–14. doi: 10.1016/j.cellbi.2005.06.004
Aramli, M. S., and Kalbassi, M. R. (2016). Antioxidant characteristics of Persian sturgeon (Acipenser persicus) seminal plasma did not show significant changes during in vitro storage (48 h at 4°C). Aquac. Res. 49:3235. doi: 10.1111/are.13204
Araujo-Lima, C., and Goulding, M. (1998). So fruitful a fish: ecology, conservation and aquaculture of the Amazon’s tambaqui. Environ. Conserv. 3, 279–289. doi: 10.1177/0020881797034002007
Aride, P. H. R., Roubach, R., and Val, A. L. (2004). Water pH in central Amazon and its importance for tambaqui (Colossoma macropomum) culture. World Aquac. 35:24.
Baeza, R., Mazzeo, I., Vílchez, M. C., Gallego, V., Peñaranda, D. S., Pérez, L., et al. (2015). Relationship between sperm quality parameters and the fatty acid composition of the muscle, liver and testis of European eel. Comp. Biochem. Physiol. A Mol. Integr. Physiol. 181, 79–86. doi: 10.1016/j.cbpa.2014.11.022
Bencic, D. C., Krisfalusi, M., Cloud, J. G., and Ingermann, R. L. (1999). Maintenance of steelhead trout (Oncorhynchus mykiss) sperm at different in vitro oxygen tensions alters ATP levels and cell functional characteristics. Fish Physiol. Biochem. 2, 193–200. doi: 10.1023/A:1007880426488
Bera, A., Chadha, N. K., Dasgupta, S., Sawant, P. B., and Lakra, W. S. (2016). In vivo ovarian and testicular stress responses in adult koi carp (Cyprinus carpio) under chronic hypoxia. Ecol. Environ. Conserv. 22, 1425–1433.
Beutler, E. (1975). Red cell metabolism: a manual of biochemical methods. New York, USA: Grune and Straton, 198.
Bradford, M. M. (1976). A rapid and sensitive method for the quantitation of microgram quantities of protein utilizing the principle of protein-dye binding. Anal. Biochem. 72, 248–254. doi: 10.1016/0003-2697(76)90527-3
Cabrita, E., Robles, V., Rebordinos, L., Sarasquete, C., and Herráez, M. P. (2005). Evaluation of DNA damage in rainbow trout (Oncorhynchus mykiss) and gilthead sea bream (Sparus aurata) cryopreserved sperm. Cryobiology 50, 144–153. doi: 10.1016/j.cryobiol.2004.12.003
Caldwell, C. A., and Hinshaw, J. (1994). Physiological and haematological responses in rainbow trout subjected to supplemental dissolved oxygen in fish culture. Aquaculture 126, 183–193. doi: 10.1016/0044-8486(94)90259-3
Chervona, Y., and Costa, M. (2012). The control of histone methylation and gene expression by oxidative stress, hypoxia, and metals. Free Radic. Biol. Med. 53, 1041–1047. doi: 10.1016/j.freeradbiomed.2012.07.020
Collins, A. R., Oscoz, A. A., Brunborg, G., Gaivão, I., Giovannelli, L., Kruszewski, M., et al. (2008). The comet assay: topical issues. Mutagenesis 23, 143–151. doi: 10.1093/mutage/gem051
Cosson, J. (2004). The ionic and osmotic factors controlling motility of fish spermatozoa. Aquac. Int. 12, 69–85. doi: 10.1023/B:AQUI.0000017189.44263.bc
Cosson, J. (2010). Frenetic activation of fish spermatozoa flagella entails short-term motility, portending their precocious decadence. J. Fish Biol. 76, 240–279. doi: 10.1111/j.1095-8649.2009.02504.x
Cosson, J., Groison, A. L., Suquet, M., Fauvel, C., Dreanno, C., and Billard, R. (2008). Studying sperm motility in marine fish: an overview on the state of the art. J. Appl. Ichthyol. 24, 460–486. doi: 10.1111/j.1439-0426.2008.01151.x
Dadras, H., Dzyuba, V., Cosson, J., Golpour, A., and Dzyuba, B. (2016). The in vitro effect of temperature on motility and antioxidant response of common carp Cyprinus carpio spermatozoa. J. Therm. Biol. 59, 64–68. doi: 10.1016/j.jtherbio.2016.05.003
De Andrade, V. M., De Freitas, T. R. O., and Da Silva, J. (2004). Comet assay using mullet (Mugil sp.) and sea catfish (Netuma sp.) erythrocytes for the detection of genotoxic pollutants in aquatic environment. Mutat. Res. 560, 57–67. doi: 10.1016/j.mrgentox.2004.02.006
Dong, X. Y., Qin, J. G., and Zhang, X. M. (2011). Adaptation of fish to changes in oxygen in aquaculture from hypoxia to hyperoxia. J. Fish. Aquac. 2, 23–28.
Dumorné, K., Valdebenito, I., Contreras, P., Rodríguez, P. U., Risopatron, J., Figueroa, E., et al. (2018). Effect of pH, osmolality and temperature on sperm motility of pink cusk-eel (Genypterus blacodes, (Forster, 1801)). Aquac. Reports 11, 42–46. doi: 10.1016/j.aqrep.2018.05.002
Dziewulska, K., and Domagała, J. (2013). Effect of pH and cation concentrations on spermatozoan motility of sea trout (Salmo trutta m. trutta L.). Theriogenology 79, 48–58. doi: 10.1016/j.theriogenology.2012.09.008
Dzyuba, B., Bondarenko, O., Fedorov, P., Gazo, I., Prokopchuk, G., and Cosson, J. (2017). Energetics of fish spermatozoa: the proven and the possible. Aquaculture 472, 60–72. doi: 10.1016/j.aquaculture.2016.05.038
Dzyuba, B., Legendre, M., Baroiller, J. F., and Cosson, J. (2019). Sperm motility of the Nile tilapia (Oreochromis niloticus): effects of temperature on the swimming characteristics. Anim. Reprod. Sci. 204, 65–72. doi: 10.1016/j.anireprosci.2019.01.010
Fitzpatrick, J. L., Craig, P. M., Bucking, C., Balshine, S., Wood, C. M., and McClelland, G. B. (2009). Sperm performance under hypoxic conditions in the intertidal fish Porichthys notatus. Can. J. Zool. 87, 464–469. doi: 10.1139/Z09-031
Flohé, L., and Otting, F. (1984). Superoxide dismutase assays. Methods Enzymol. 105, 93–104. doi: 10.1016/S0076-6879(84)05013-8
Gallego, V., Herranz-Jusdado, J. G., Rozenfeld, C., Pérez, L., and Asturiano, J. F. (2018). Subjective and objective assessment of fish sperm motility: when the technique and technicians matter. Fish Physiol. Biochem. 44, 1–11. doi: 10.1007/s10695-018-0505-1
Gazo, I., Shaliutina-Kolešová, A., Dietrich, M. A., Linhartová, P., Shaliutina, O., and Cosson, J. (2015). The effect of reactive oxygen species on motility parameters, DNA integrity, tyrosine phosphorylation and phosphatase activity of common carp (Cyprinus carpio L.) spermatozoa. Mol. Reprod. Dev. 82, 48–57. doi: 10.1002/mrd.22442
Gholami, H., Chamani, M., Towhidi, A., and Fazeli, M. H. (2011). Improvement of semen quality in holstein bulls during heat stress by dietary supplementation of omega-3 fatty acids. Int. J. Fertil. Steril. 4, 160–167.
Goulding, M., and Carvalho, M. L. (1982). Life history and management of the tambaqui (Colossoma macropomum, Characidae): an important Amazonian food fish. Rev. Bras. Zool. 2, 107–133. doi: 10.1590/S0101-81751982000200001
Isaak, D. J., Wollrab, S., Horan, D., and Chandler, G. (2012). Climate change effects on stream and river temperatures across the northwest U. S. from 1980–2009 and implications for salmonid fishes. Clim. Chang. 113, 499–524. doi: 10.1007/s10584-011-0326-z
Jenkins, J. A., Rosen, M. R., Draugelis-dale, R. O., Echols, K. R., Torres, L., Wieser, C. M., et al. (2018). Sperm quality biomarkers complement reproductive and endocrine parameters in investigating environmental contaminants in common carp (Cyprinus carpio) from the Lake Mead National Recreation Area. Environ. Res. 163, 149–164. doi: 10.1016/j.envres.2018.01.041
Jeppesen, E., Meerhoff, M., Holmgren, K., González-Bergonzoni, I., Teixeira-de Mello, F., Declerck, S. A. J., et al. (2010). Impacts of climate warming on lake fish community structure and potential effects on ecosystem function. Hydrobiologia 646, 73–90. doi: 10.1007/s10750-010-0171-5
Jiang, Z. Y., Woollard, A. C. S., and Wolff, S. P. (1991). Lipid hydroperoxide measurement by oxidation of Fe2+ in the presence of xylenol orange. Comparison with the TBA assay and an iodometric method. Lipids 26, 853–856. doi: 10.1007/BF02536169
Keen, J. H., Habig, W. H., and Jakoby, W. B. (1976). Mechanism for the several activities of the glutathione S transferases. J. Biol. Chem. 251, 6183–6188.
Kime, D. E., Van Look, K. J. W., McAllister, B. G., Huyskens, G., Rurangwa, E., and Ollevier, F. (2001). Computer-assisted sperm analysis (CASA) as a tool to monitor sperm quality in fish. Comp. Biochem. Physiol. C Toxicol. Pharmacol. 130, 425–433. doi: 10.1016/S1532-0456(01)00270-8
Lacaze, E., Devaux, A., Jubeaux, G., Mons, R., Gardette, M., Bony, S., et al. (2011). DNA damage in Gammarus fossarum sperm as a biomarker of genotoxic pressure: intrinsic variability and reference level. Sci. Total Environ. 409, 3230–3236. doi: 10.1016/j.scitotenv.2011.05.012
Lahnsteiner, F., and Caberlotto, S. (2012). Motility of gilthead seabream Sparus aurata spermatozoa and its relation to temperature, energy metabolism and oxidative stress. Aquaculture 370–371, 76–83. doi: 10.1016/j.aquaculture.2012.09.034
Lapointe, D., Cooperman, M. S., Chapman, L. J., Clark, T. D., Val, A. L., Ferreira, M. S., et al. (2018). Predicted impacts of climate warming on aerobic performance and upper thermal tolerance of six tropical freshwater fishes spanning three continents. Conserv. Physiol. 6, 1–19. doi: 10.1093/conphys/coy056
Lewis, C., and Galloway, T. (2009). Reproductive consequences of paternal genotoxin exposure in marine invertebrates. Environ. Sci. Technol. 43, 928–933. doi: 10.1021/es802215d
Mendonça, P. P., Ferreira, R. A., Vidal Júnior, M. V., Andrade, D. R., Santos, M. V. B., Ferreira, A. V., et al. (2009). Influência do fotoperíodo no desenvolvimento de tambaqui (Colossoma macropomum). Arch. Zootec. 58, 323–331. doi: 10.4321/S0004-05922009000300001
Mirade, J. M. (2010). Phylogeny of the family Characidae (Teleostei: Characiformes): from characters to taxonomy. Neotrop. Ichthyol. 8, 385–568. doi: 10.1590/S1679-62252010000300001
Mustafa, A. S., Al-Subiai, S. N., Davies, S. J., and Jha, A. N. (2011). Hypoxia-induced oxidative DNA damage links with higher level biological effects including specific growth rate in common carp, Cyprinus carpio L. Ecotoxicology 20, 1455–1466. doi: 10.1007/s10646-011-0702-5
PerchecPoupard, G., Gatti, J. L., Cosson, J., and Fierville, F. (1997). Involved in activation of motility of carp spermatozoa. J. Reprod. Fertil. 110, 315–327.
Pérez-Cerezales, S., Martínez-Páramo, S., Beirão, J., and Herráez, M. P. (2010). Fertilization capacity with rainbow trout DNA-damaged sperm and embryo developmental success. Reproduction 139, 989–997. doi: 10.1530/REP-10-0037
Purchase, C. F., Butts, I. A. E., Alonso-Fernández, A., and Trippel, E. A. (2010). Thermal reaction norms in sperm performance of Atlantic cod (Gadusmorhua). Can. J. Fish. Aquat. Sci. 67, 498–510. doi: 10.1139/F10-001
R Core Team, (2018). R: A language and environment for statistical computing. Vienna, Austria: Foundation R for Statistical Computing.
Richards, J. G., Farrell, A. P., and Brauner, C. J. (2009). Fish physiology: Hypoxia. 1st Edn. Vol. 27. London, UK: Academic Press.
Rurangwa, E., Kime, D. E., Ollevier, F., and Nash, J. P. (2004). The measurement of sperm motility and factors affecting sperm quality in cultured fish. Aquaculture 234, 1–28. doi: 10.1016/j.aquaculture.2003.12.006
Shaliutina, A., Hulak, M., Gazo, I., Linhartova, P., and Linhart, O. (2013). Effect of short-term storage on quality parameters, DNA integrity, and oxidative stress in Russian (Acipenser gueldenstaedtii) and Siberian (Acipenser baerii) sturgeon sperm. Anim. Reprod. Sci. 139, 127–135. doi: 10.1016/j.anireprosci.2013.03.006
Singh, N. P., McCoy, M. T., Tice, R. R., and Schneider, E. L. (1988). A simple technique for quantitation of low levels of DNA damage in individual cells. Exp. Cell Res. 175, 184–191. doi: 10.1016/0014-4827(88)90265-0
Slowinska, M., Nynca, J., Cejko, B. I., Dietrich, M. A., Horváth, Á., Urbányi, B., et al. (2013). Total antioxidant capacity of fish seminal plasma. Aquaculture 401, 101–104. doi: 10.1016/j.aquaculture.2013.03.010
Tewksbury, J., Huey, R., and Deutsch, C. (2008). Putting heat on tropical animals. Science 320, 1296–1297. doi: 10.1126/science.1159328
Thomas, P., Rahman, M. S., Khan, I. A., and Kummer, J. A. (2007). Widespread endocrine disruption and reproductive impairment in an estuarine fish population exposed to seasonal hypoxia. Proc. R. Soc. B Biol. Sci. 274, 2693–2701. doi: 10.1098/rspb.2007.0921
Vieira, E. F., Isaac, V. J., and Fabré, N. N. (1999). Biologia reprodutiva do tambaqui, Colossoma macropomm Cuvier, 1818 (Teleostei, Serrasalmidae) no baixo Amazonas, Brasil. Acta Amaz. 29, 625–638. doi: 10.1590/1809-43921999294638
Wang, S. Y., Lau, K., Lai, K., Zhang, J., Tse, A. C., Li, J., et al. (2016). Reproduction of fish. Nat. Commun. 7, 1–9. doi: 10.1038/ncomms12114
Wenger, S. J., Isaak, D. J., Dunham, J. B., Fausch, K. D., Luce, C. H., Neville, H. M., et al. (2011). Role of climate and invasive species in structuring trout distributions in the interior Columbia river. Can. J. Fish. Aquat. Sci. 68, 988–1008. doi: 10.1139/f2011-034
Wiens, L., Banh, S., Sotiri, E., Jastroch, M., Block, B. A., Brand, M. D., et al. (2017). Comparison of mitochondrial reactive oxygen species production of ectothermic and endothermic fish muscle. Front. Physiol. 8:704. doi: 10.3389/fphys.2017.00704
Williot, P., Kopeika, E. F., and Goncharov, B. F. (2000). Influence of testis state, temperature and delay in semen collection on spermatozoa motility in the cultured Siberian sturgeon (Acipenser baeri Brandt). Aquaculture 189, 53–61. doi: 10.1016/S0044-8486(00)00358-6
Wong-Ekkabut, J., Xu, Z., Triampo, W., Tang, I. M., Tieleman, D. P., and Monticelli, L. (2007). Effect of lipid peroxidation on the properties of lipid bilayers: a molecular dynamics study. Biophys. J. 93, 4225–4236. doi: 10.1529/biophysj.107.112565
Wood, C. M., Márcio, R. J. G., Ferreira, S., Braz, S., Adalberto, M., and Val, L. (2018). The physiology of the tambaqui (Colossoma macropomum) at pH 8.0. J. Comp. Physiol. B 188, 393–408. doi: 10.1007/s00360-017-1137-y
Keywords: aquaculture, antioxidant enzymes, climate change, comet assay, fish breeding, motility, tropical fish
Citation: Castro JS, Braz-Mota S, Campos DF, Souza SS and Val AL (2020) High Temperature, pH, and Hypoxia Cause Oxidative Stress and Impair the Spermatic Performance of the Amazon Fish Colossoma macropomum. Front. Physiol. 11:772. doi: 10.3389/fphys.2020.00772
Edited by:
Alexssandro Geferson Becker, Universidade Federal do Paraná, Setor Palotina, BrazilReviewed by:
Michael L. Fine, Virginia Commonwealth University, United StatesCharlene Menezes, Federal University of Santa Maria, Brazil
Copyright © 2020 Castro, Braz-Mota, Campos, Souza and Val. This is an open-access article distributed under the terms of the Creative Commons Attribution License (CC BY). The use, distribution or reproduction in other forums is permitted, provided the original author(s) and the copyright owner(s) are credited and that the original publication in this journal is cited, in accordance with accepted academic practice. No use, distribution or reproduction is permitted which does not comply with these terms.
*Correspondence: Jonatas S. Castro, am9uc2Nhc3Ryb29AZ21haWwuY29t