- 1Department of Poultry Science, University of Arkansas, Fayetteville, AR, United States
- 2DSM Animal Nutrition and Health, Kaiseraugst, Switzerland
Phytate forms insoluble precipitates with various cations that are recalcitrant to digestion in poultry. Dietary supplementation with exogenous phytase has been shown to improve phytate solubility and digestibility and, in turn, improve animal growth performance. Although the kinetics of phytate hydrolysis by exogenous phytase are well described in vitro, the progression of the reaction in vivo is still not well defined. The aim of the present study was, therefore, to monitor the kinetic variation of myo-inositol (myo-Ins) levels in both circulation and feather following exogenous phytase supplementation. In experiment 1, 4 week-old male broilers were individually housed with ad libitum access to water and a standard commercial diet. Birds were maintained under environmental temperature of 24°C and 30% RH. Birds were cannulated in the cutaneous ulnar vein on the right wing and remained untouched for 3 days. On the day of the experiment, birds were randomly divided into three body weight-matched groups and fed either the control diet, the control diet-supplemented with myo-Ins or Ronozyme HiPhos (0.06%, DSM Nutritional Products, Switzerland) for 10 h. In the experiment 2, birds were fed only HiPhos for 30 h. Growing feathers and blood were collected at baseline and then every 2 h for 10 h (experiment 1) and 30 h (experiment 2) post-prandially. Plasma and feather myo-Ins levels were determined by UHPLC-MS/MS. The relative expression of inositol polyphosphate-1-phosphatase (INPP1), inositol hexakisphosphate kinase 1-3 (IP6K1-3), inositol-3-phosphate synthase (ISYNA), and multiple inositol-polyphosphate phosphatase 1 (MNPP1) genes in blood and feathers was determined by real-time qPCR using 2–ΔΔCt method. Plasma and feather myo-Ins levels were significantly increased by HiPhos at 6 h to 8 h post-prandial. The mRNA abundances of INPP1, IP6K1, and ISYNA in the circulation were significantly down regulated at all periods compared to the baseline levels. IP6K2, IP6K3, and MINPP1 gene expression, however, was up regulated at 8 h post-prandial and then returned to the baseline levels. In feathers, the expression of INPP1 was induced at 8 h post-prandial and remained higher compared to the baseline. The expression of IP6K2, IP6K3, and MINPP1 was down regulated during the first 10 h and then returned to baseline levels for the rest of the post-prandial period. Taken together, our data show that phytase modulates the expression of genes associated with myo-Ins metabolism and generates release of myo-Ins in both circulation and feather at 6–10 h post-feeding. Feather myo-Ins concentration could be used as a non-invasive method to monitor phytate hydrolysis in practice.
Introduction
In plants, a large proportion of the phosphorous (P) is bound in the form of phytate, the mixed salt of phytic acid (myo-inositol hexakisphosphate; InsP6) (Plimmer and Page, 1913; Maga, 1982; Eeckhout and De Paepe, 1994; Viveros et al., 2000). Phytate-P as a percentage of total P is highest in the cereal grains wheat and corn (Viveros et al., 2000), and it is estimated that poultry diets contain approximately 2.5–4.0 g of phytate-P per kg of feed (Ravindran, 1995). Phytase and phosphatases are the enzymes responsible for the catalysis of the stepwise hydrolysis of phytate to inorganic phosphorous and inositol (with lower-phosphorylated inositol intermediates). Endogenous activity of these enzymes within the digestive tract of poultry is concentrated in the small intestine where the relatively high pH and presence of various cations renders phytate solubility low, compromising phytate-P retention (Ravindran, 1995; Maenz and Classen, 1998; Rutherfurd et al., 2004; Cowieson et al., 2006). This, significantly impairs P retention and in turn creates complex environmental pressure (Waldroup, 1999). Additionally, phytate has anti-nutritional effects, as it can interact with multiple minerals and proteins, particularly calcium, iron, and zinc, and subsequently impact absorption of these compounds (Cheryan and Rackis, 1980). Consequently, the majority of commercial poultry diets have been supplemented with exogenous phytase (Bedford, 2000) to not only improve the use and absorption of phytate-P, but to also decrease the anti-nutritive effects of this compound (Mullaney et al., 2000; Selle and Ravindran, 2007). This supplementation has beneficial effects on broiler performance, including increased body weight gain, feed efficiency (Dilger et al., 2004; Dos Santos et al., 2013), and nutrient utilization (Liu et al., 2014). Interestingly, the effects of dietary phytase can be greater than expected from increased nutrient release alone (Coweison et al., 2015). These effects may be due, in part, to the production/release of myo-inositol (myo-Ins), as it has been shown to have effects on mammalian metabolism, as it can act as an insulin memetic (Chhetri, 2019; Gonzalez-Uarquin et al., 2019). In poultry, however, the kinetics of phytate hydrolysis by exogenous phytase is still not well defined. Furthermore, information on myo-Ins absorption, transport within the body, and metabolism in poultry is sparse. Therefore, the objective of the present study was to monitor the kinetic variation of circulating myo-Ins levels following exogenous phytase supplementation. As myo-Ins has been shown to be involved in hirsutism treatment (Minozzi et al., 2008), and as feathers have been demonstrated to be a new biomonitoring tool (Jaspers et al., 2011), we extended our analysis to growing feathers.
Materials and Methods
Care and Use of Animals
This study was conducted in accordance with the National Institutes of Health recommendations guide for laboratory animal use and care. All animal care and procedures were approved by the Institutional Animal Care and Use Committee at the University of Arkansas (protocol # 16084).
Animal Procedure and Environment
Two experiments were conducted. In the first experiment, 4 week-old male broilers (Cobb 500, n = 36) were transferred to individual cages and acclimatized for 1 week to the laboratory housing conditions with ad libitum access to water and a diet that meets the usual integration energy and nutrient allowances (Table 1). Birds were maintained under environmental temperature of 24°C and 20% RH (Figure 1A). Birds were cannulated in the cutaneous ulnar vein on the right wing, using the technique of Bayer et al. (2012). Briefly, feathers were removed, and the area surrounding the vein was cleaned with 70% ethanol. Lidocaine cream (AneCream, Focus Health Group, Knoxville, TN) was topically applied to the cannulation site, followed by a subcutaneous injection of 2% lidocaine HCl to mitigate pain. A 24G, inch winged catheter (B. Braun, Germany) was inserted into the vein, patency confirmed, and fixed in place by sutures (Ethilon nylon monofilament 4-0, Ethicon, Somerville, NJ) on both sides of the cannula. A 10% heparin solution was used to maintain patency and prevent clot formation. On the day of the experiment, birds were randomly divided into three body weight-matched groups and fed either the control diet (C, Table 1) or diets supplemented with myo-Ins (Sigma-Aldrich, St. Louis, MO) at 0.30% (Myo-Ins) or Ronozyme HiPhos (0.06%, DSM Switzerland) (HiPhos). Feed and water intake were recorded hourly, and body weights were recorded at the beginning and end of the experiment (10 h). The second experiment was similar to the first one, except that two diets were used (C at the baseline vs. HiPhos) for a duration of 30 h.
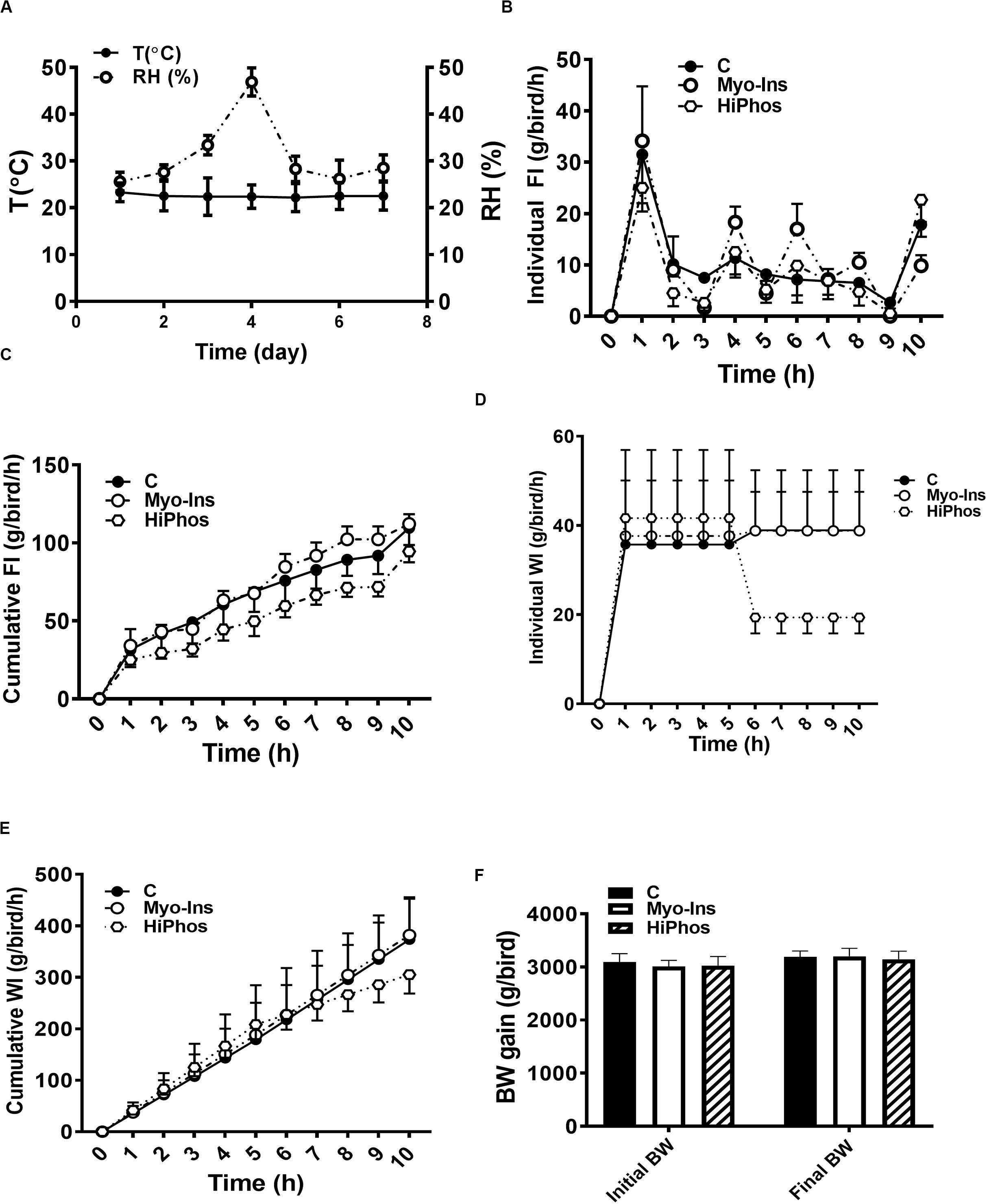
Figure 1. Effect of myo-Ins and HiPhos supplementation during experiment 1 on chicken feed intake, water intake, and body weight. (A) Environmental condition (RH and T°) of the chambers. Myo-Ins and HiPhos did not affect individual and cumulative feed intake (B,C). HiPhos decreased individual (D) and cumulative (E) water intake. There was no change in BW (F). Data are presented as mean ± SEM. BW, body weight; Myo-Ins, myo-inositol.
Sample Collection
Growing feathers and blood were collected at baseline and then every 2 h for 10 h (experiment 1) and 30 h post-prandial (experiment 2). Growing feathers were collected from the left breast tract, and the living tissues (pulp) were sectioned, rinsed in PBS, snap frozen in liquid nitrogen, and stored at −80°C until use. 250 μL of blood was collected for RNA isolation and gene expression, with the remainder placed in a heparinized tube for plasma separation. All samples were stored at −80°C until analysis.
Circulating and Feather Myo-Inositol Measurement
After addition of internal standard (Myo-Ins and Myo-Ins-C-d6), plasma protein precipitation is performed with acetonitrile. Feather myo-Ins samples were prepared in duplicate as described by Frieler et al. (2009). After centrifugation, the supernatant is injected in LC-MS/MS system with a turbo spray ionization and analyzed by ultrahigh performance liquid chromatography 1290 Agilent system coupled with API4000 quadrupole mass spectrometer from ABsciex according to the method of Leung et al. (2011). A Poroshell 120 Hilic-phase column (100 mm × 2.1 mm i.d.; particle size, 2.7 μm) was used. The mobile phase A was a solution of water/50 mM ammonium formate/Formic acid (950/50/1; v/v/v) and the mobile phase B was a solution of acetonitrile/50 mM ammonium formate/Formic acid (950/50/1; v/v/v). The system ran under gradient mode at a flow rate of 0.6 mL/min for 2.5 min: 0 to 0.3 min held at 2.0 % of A, then 0.3 to 1.5 min linear increased to 20% of A, then decreased to 2% of A in 0.1 min and held till 2.5 min at 2% of A. The retention times for myo-Ins / myo-Ins-C-d6 were at 1.3 min. The mass spectrometer was operated in ESI negative mode. The source was set as follows: Temperature at 650°c, curtain gas at 40, transfer voltage at −4500, gas1 at 80, gas2 at 60 and CAD at 12. The multiple reaction monitoring mode transitions for the ion pairs were: Q1/Q3 = 178.8/86.6 m/z for myo-Ins and 184.9/102.0 for the internal standard. Data acquisition of extracted ion chromatograms, identification, integration and quantification are performed by Analyst® software from AB Sciex. Quantification was performed by applying an external calibration. Daily performance of the system was monitored with calibration and quality control samples ran in each analytical batch.
RNA Isolation and Quantitative Real-Time PCR
Total RNA was extracted using Trizol reagent (for feather) or Trizol LS (for blood) (Life Technologies, Carlsbad, CA) according to the manufacturer’s instructions. RNA integrity and quality was assessed using 1% agarose gel electrophoresis and RNA concentrations and purity were determined for each sample by Take 3 Micro-Volume Plate using Synergy HT multi-mode micro plate reader (BioTek, Winooski, VT). RNA samples were DNase treated, reverse transcribed using qScript cDNA Synthesis Supermix (Quanta Biosciences, Gaithersburg, MD), and amplified by real-time quantitative PCR (Applied Biosystems 7500 Real Time System) with PowerUp SYBR green master mix (Life Technologies, Carlsbad, CA, United States) as previously described (Rajaei-Sharifabadi et al., 2017). The qPCR cycling conditions were 50°C for 2 min, 95°C for 10 min followed by 40 cycles of a two-step amplification program (95°C for 15 s and 58°C for 1 min). At the end of the amplification, melt curve analysis was applied using the dissociation protocol to exclude contamination with unspecific PCR products. Relative expression of the target genes was determined using the 2–ΔΔCt method, with normalization to 18 s rRNA as a housekeeping gene (Schmittgen and Livak, 2008). Oligonucleotide primer sequences specific for chicken are presented in Table 2.
Statistical Analysis
Data were analyzed by two-way repeated measures ANOVA with diet (C, Myo-Ins, and HiPhos) and time as factors. If ANOVA revealed significant effects, the means were compared by Tukey multiple range test using the Graph Pad Prism version 6.00 for Windows (Graph Pad Software, La Jolla, CA, United States), and differences were considered significant at P < 0.05.
Results
During the course of the first experiment, temperature and relative humidity averaged 24°C and 30% RH, respectively (Figure 1A). Hourly individual and cumulative feed intake was highest for the myo-Ins group and lowest for the HiPhos group (Figures 1B,C). After 5 h, water intake was lower for the phytase-fed birds (Figures 1D,E). There was no significant change in body weight over the course of the experiment (Figure 1F).
Plasma myo-Ins levels were increased in the myo-Ins group from 6 to 10 h (Figure 2A), whereas feather myo-Ins concentrations were increased by HiPhos after 6 h, and by myo-Ins supplementation only after 10 h (Figure 2B).
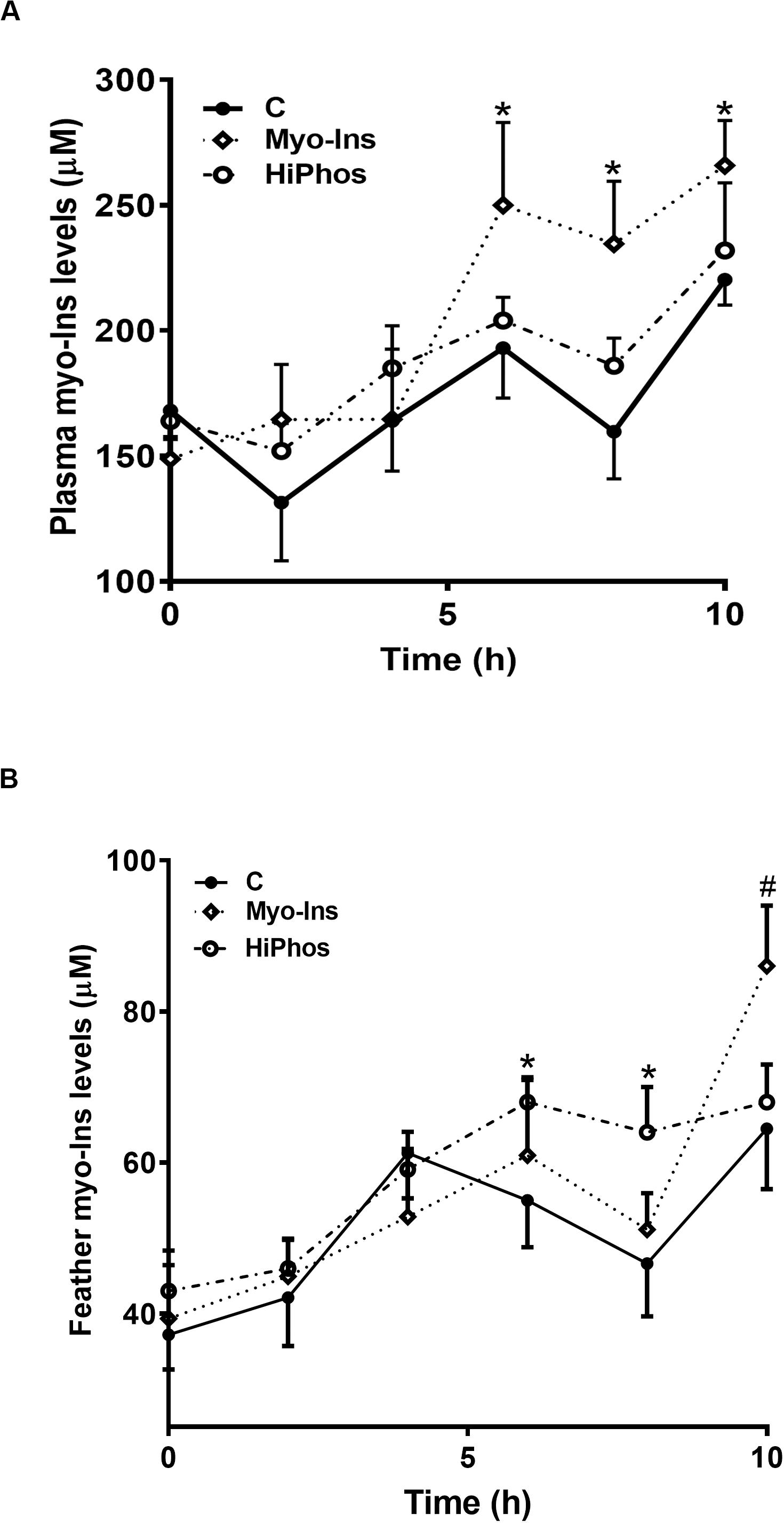
Figure 2. Effect of myo-Ins and HiPhos supplementation during experiment 1 on plasma and feather myo-Ins levels. Myo-Ins-enriched diets increased plasma myo-Ins concentrations 6–10 h post-prandial (A). HiPhos supplementation increased feather myo-Ins concentrations 6–8 h post-prandial (B). Myo-Ins supplementation increased myo-Ins concentrations in feather at 10 h post feeding. Data are presented as mean ± SEM. *Indicates significant difference compared to the control diet at P < 0.05. #Indicates significant difference compared to the control diet at P < 0.01.
In the second experiment, temperature and relative humidity averaged 20°C and 22%, respectively (Figure 3A). Individual feed and water remained relatively constant, with a peak in intake at 26 h compared to the beginning of the experiment (Figures 3B,D), and cumulative increases over time (Figures 3C,E). Body weight of birds did not significantly change over the course of the experiment (Figure 3F). A similar pattern was seen to the first experiment, whereby plasma (Figure 4A) and feather (Figure 4B) myo-Ins levels were increased after 6–10 h. In plasma, levels returned to baseline after 24 h, whereas in feather, myo-Ins levels fell below baseline after 24 h (Figures 4A,B).
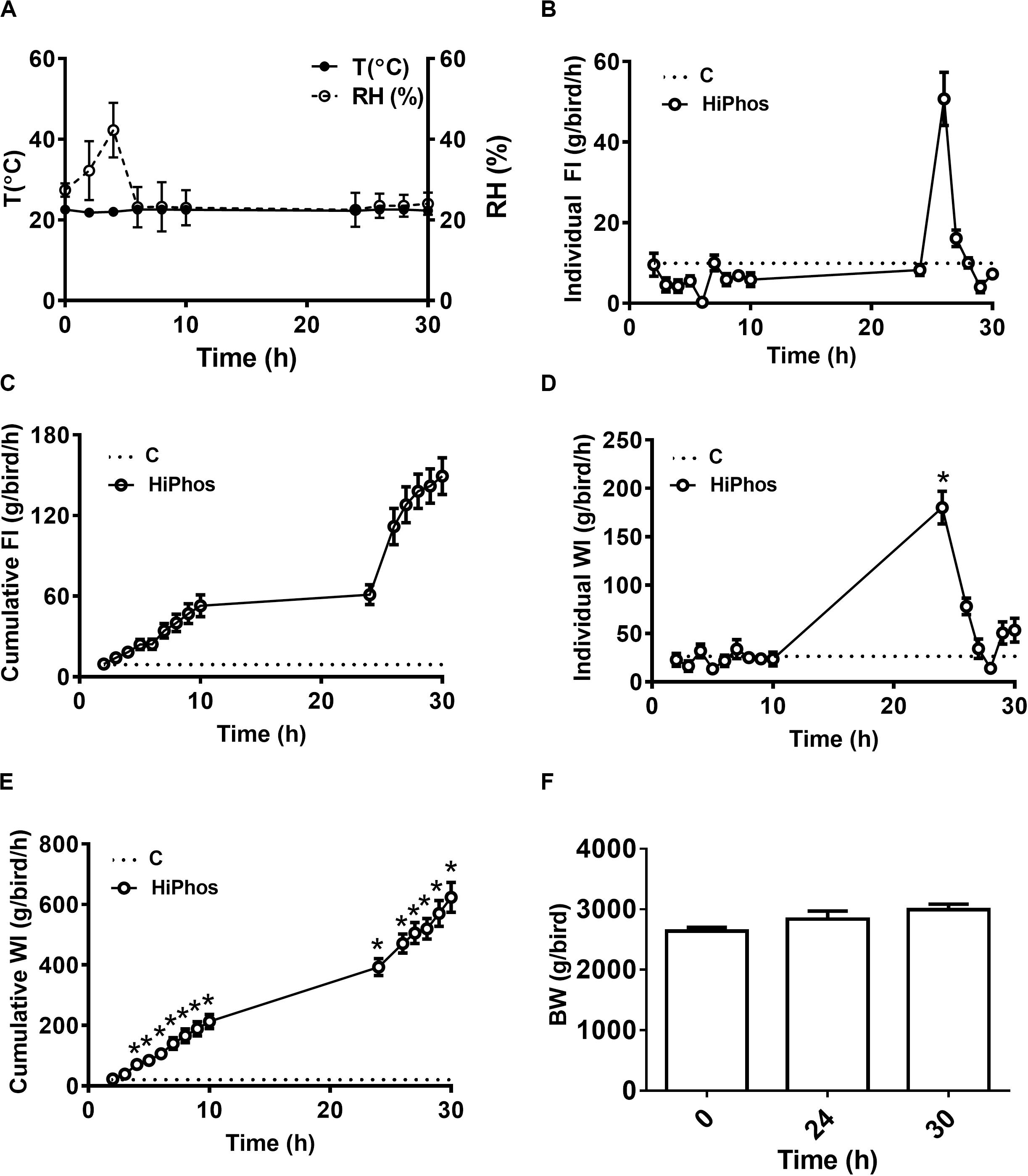
Figure 3. Effect of HiPhos supplementation during experiment 2 on feed intake, water intake, and body weight over 30 h in broilers. (A) Environmental condition (RH and T°) of the chambers. HiPhos affected individual and cumulative feed intake and individual and cumulative water intake after 24 h (B–E). There was no change in BW over time (F). Data are presented as mean ± SEM. *Indicates significant difference compared to the baseline (C, 0 h) at P < 0.05.
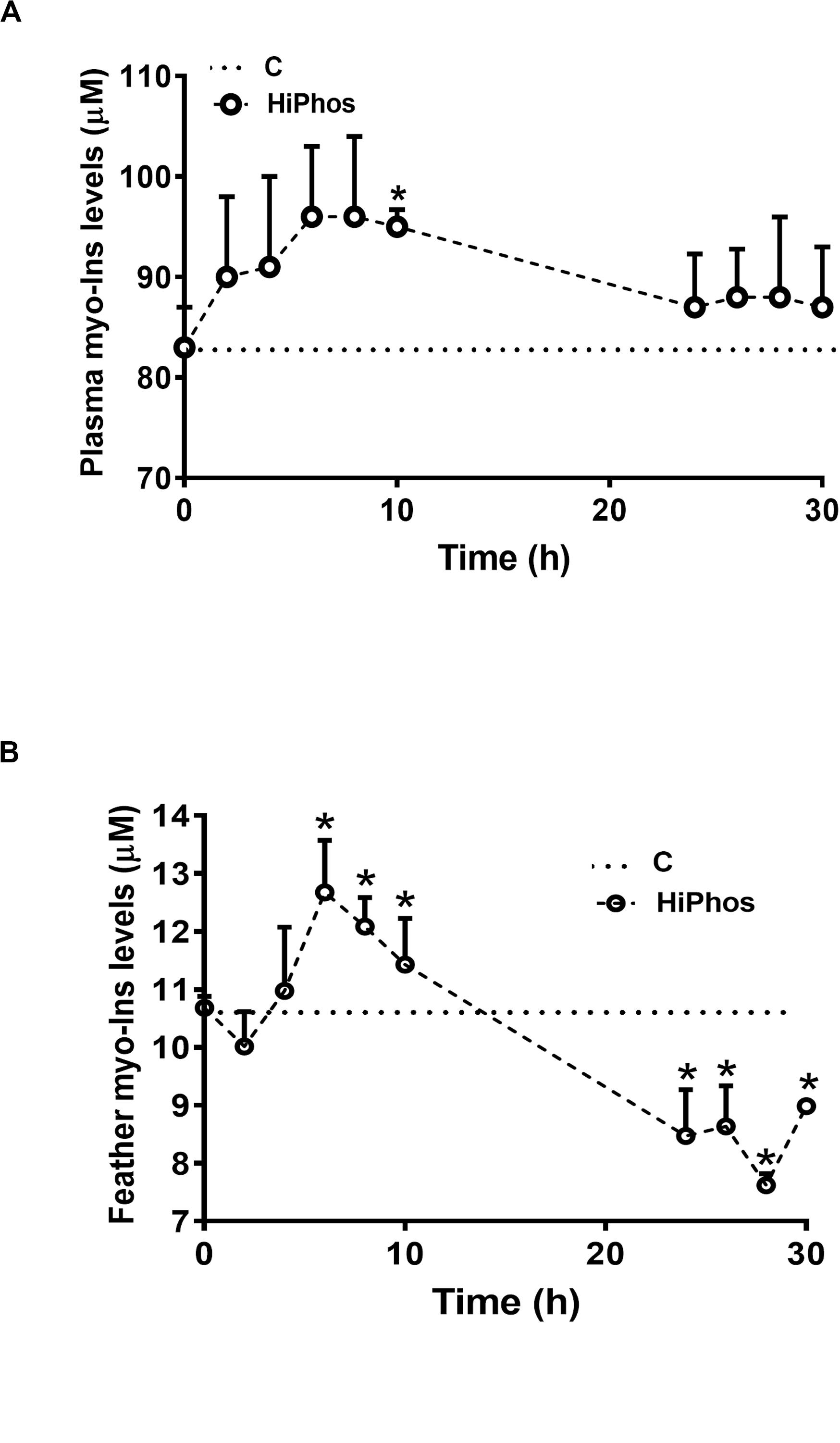
Figure 4. Effect of HiPhos supplementation during experiment 2 on plasma and feather myo-Ins levels over 30 h. HiPhos increased plasma (A) and feather (B) myo-Ins concentrations 6–10 h post-prandial. Plasma levels returned to baseline, whereas feather levels fell fellow baseline after 24 h. Data are presented as mean ± SEM. *Indicates significant difference compared to the baseline (C, 0 h) at P < 0.05.
To further investigate phytate metabolism, the gene expression of enzymes involved in phytate degradation and myo-Ins formation in circulation and feather were measured in experiment 2. In circulation, relative expression of inositol polyphosphate-1-phosphatase (INPP1), inositol hexakisphosphate kinase 1 (IP6K1), and inositol-3-phosphate synthase (ISYNA) genes were significantly down regulated at all time periods compared to baseline levels. Inositol hexakisphosphate kinase 2 (IP6K2), inositol hexakisphosphate kinase 3 (IP6K3), and multiple inositol-polyphosphate phosphatase 1 (MINPP1) gene expression, however, were up regulated at 6–8 h post-prandial and then returned to the baseline levels (Figures 5A–F). In the feather, the expression of INPP1 and IP6K1 was induced by 8 h post-prandial and remained higher compared to the baseline (Figures 6A,B). The expression of IP6K2, IP6K3, ISYNA, and MINPP1 was down regulated during the first 10 h and then up regulated for the remainder of the post-prandial period (Figures 6C–F).
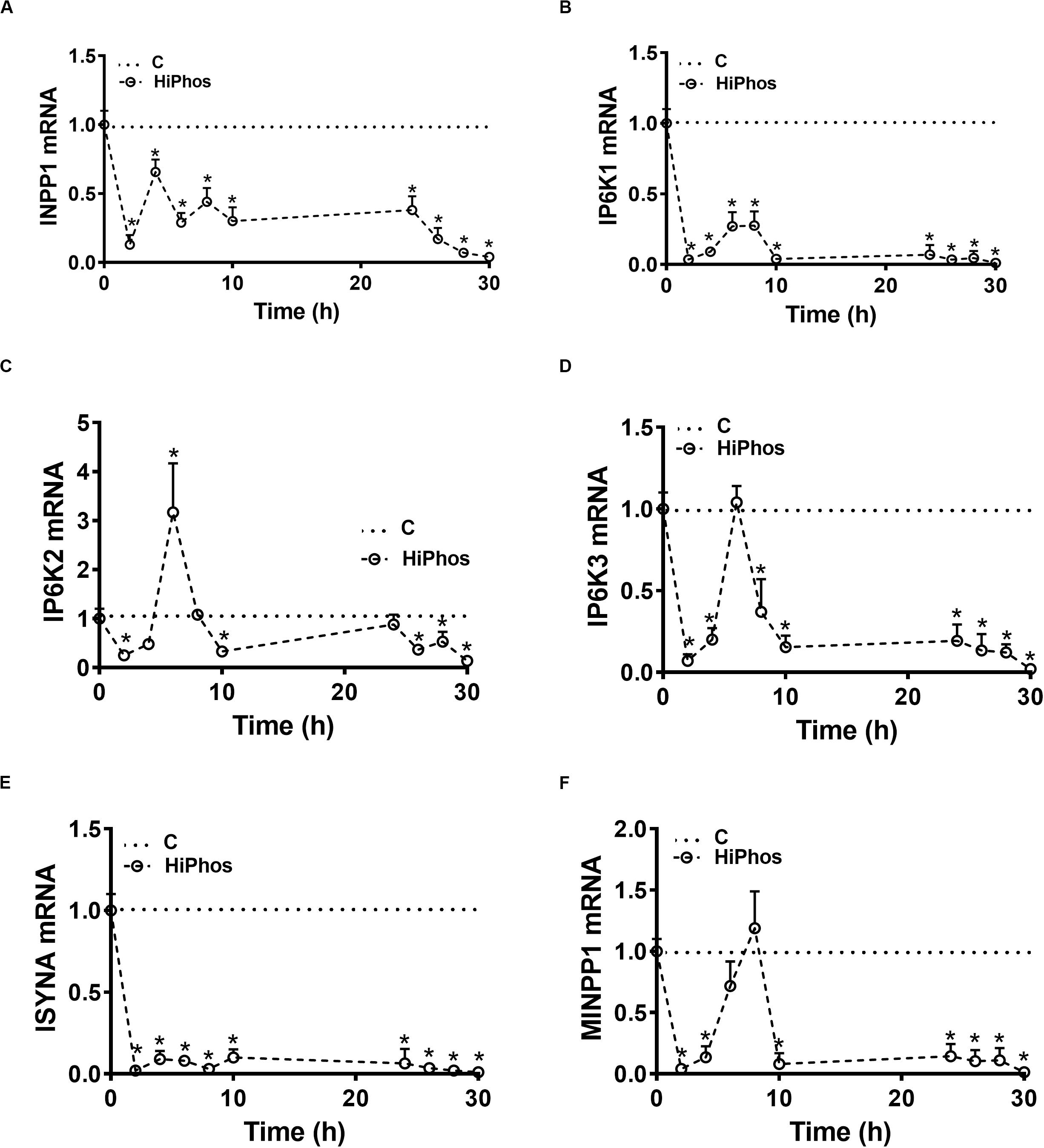
Figure 5. Effect of HiPhos supplementation during experiment 2 on gene expression of myo-inositol metabolism in whole blood. The expression of INPP1 (A), IP6K1 (B), IP6K2 (C), IP6K3 (D), ISYNA (E), and MINPP1 (F) was measured using real-time qPCR and the relative expression was determined by 2– ΔΔCt method (Schmittgen and Livak, 2008). Data are presented as mean ± SEM. *Indicates significant difference compared to the baseline (C, 0 h) at P < 0.05. INPP1, inositol polyphosphate-1-phosphatase; IP6K1-3, inositol hexakisphosphate kinase 1-3; ISYNA, inositol-3-phosphate synthase; MINPP1, multiple inositol-polyphosphate phosphatase 1.
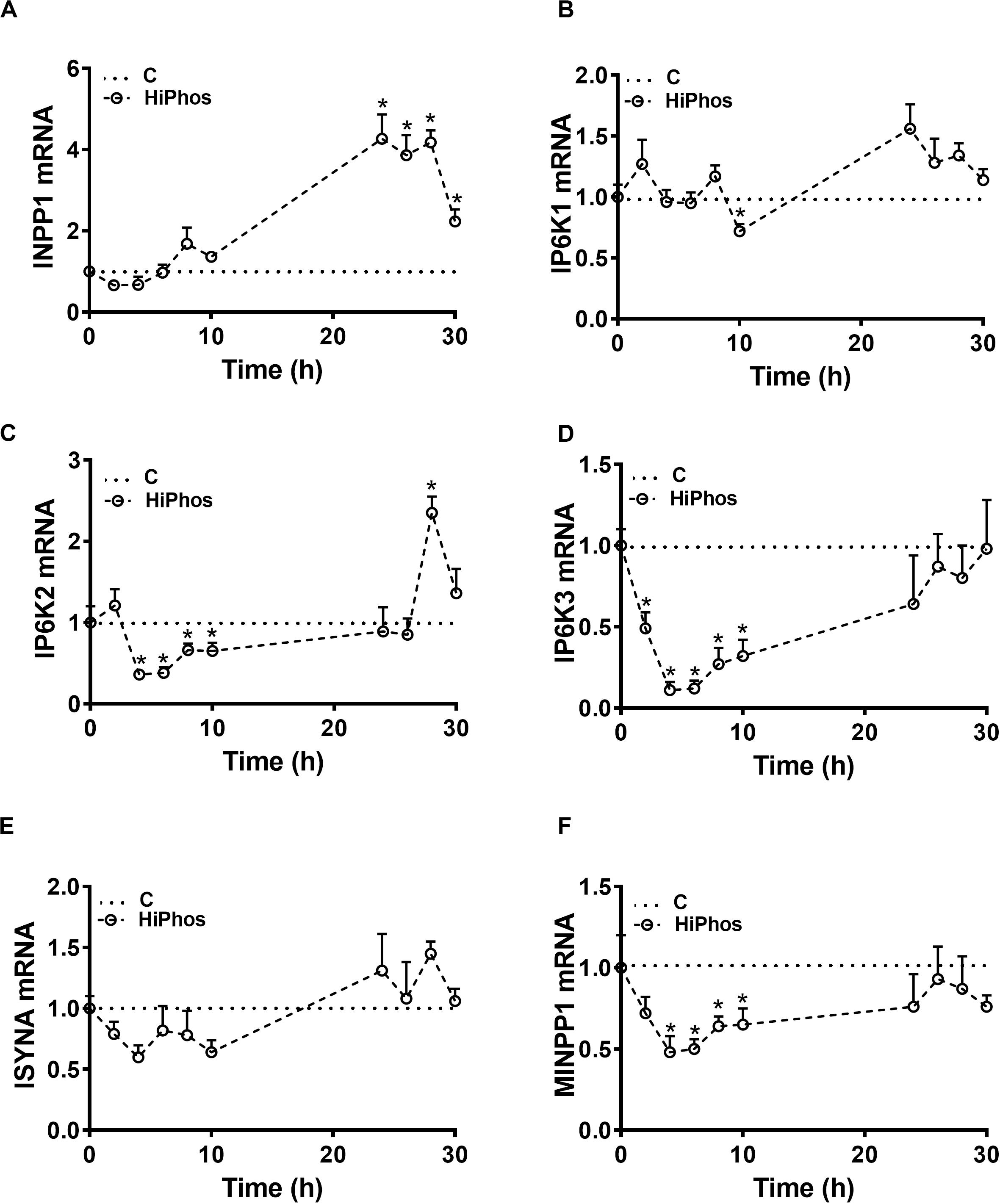
Figure 6. Effect of HiPhos supplementation during experiment 2 on gene expression of myo-inositol metabolism in feather. The expression of INPP1 (A), IP6K1 (B), IP6K2 (C), IP6K3 (D), ISYNA (E), and MINPP1 (F) was measured using real-time qPCR and the relative expression was determined by 2– ΔΔCt method (Schmittgen and Livak, 2008). Data are presented as mean ± SEM. *Indicates significant difference compared to the baseline (C, 0 h) at P < 0.05. INPP1, inositol polyphosphate-1-phosphatase; IP6K1-3, inositol hexakisphosphate kinase 1-3; ISYNA, inositol-3-phosphate synthase; MINPP1, multiple inositol-polyphosphate phosphatase 1.
Discussion
Phytate is an important anti-nutritive component in poultry feeds, and adding supplementary phytase to the diet has become broadly utilized in the poultry industry (Bedford, 2000). However, there is a paucity of information regarding the kinetics of phytate hydrolysis to myo-Ins, as well as the role of myo-Ins in poultry metabolism. Here, we were able to monitor myo-Ins kinetic variations in both circulation and feather after the addition of phytase to the diet and have shown marked increases 6 h post-prandial. The majority of dietary myo-Ins that is absorbed is likely transferred to the bloodstream (Lewin et al., 1976), making its presence in circulation a viable marker for digestion of phytate in the small intestine. Indeed, it has recently been shown that plasma myo-Ins levels are greatly increased with phytase feeding at both moderate (Schmeisser et al., 2017) and “super-dosing” levels (Coweison et al., 2015) in chicken. However, even with high levels of phytase, not all phytate is hydrolyzed to myo-Ins, as differently phosphorylated inositol phosphates can be found in the chicken digestive tract (Sommerfeld et al., 2018a, b); therefore, myo-Ins absorption cannot be assumed from the amount of phytate in feed alone, indicating the need for a more direct measurement.
In mammals, circulating myo-Ins can reach body tissues, where it has been shown to accumulate as free myo-Ins (Lewin et al., 1976; Sakamoto et al., 1993), but at varying levels, depending on the tissue, with cerebellar and cortical tissue highest in concentration and muscle and liver lowest (Battaglia et al., 1961). To date, ours is the first report of myo-Ins concentrations in any poultry tissue (Gonzalez-Uarquin et al., 2019). Here, we show that feather myo-Ins increased in a manner similar to that in plasma in the initial (first 10 h) post-prandial state, indicating its potential as a non-invasive method to temporally measure and monitor phytate and myo-Ins metabolism in chicken. Feather sampling has several advantages over other methods of monitoring responses in birds. Feather samples are quick and easy to obtain, do not require significant restraint and stress, and allow for observing the same animal over time. Feather sampling is becoming recognized in this respect, as we have recently shown feather to be advantageous for monitoring HSP70 during heat stress in chicken (Greene et al., 2019), and others have used feather to diagnose Marek’s disease (Davidson and Borenshtain, 2002; Angamuthu et al., 2012), and measure corticosterone (Carbajal et al., 2014; Weimer et al., 2018).
Dietary phytase has positive effects beyond those expected from relief of phytate anti-nutrient effects alone. These effects may be attributable to the hydrolysis of phytate to myo-Ins, which likely has numerous metabolic functions that can affect the growth performance of poultry. As the primary functions of myo-Ins are in cellular signaling pathways and as a precursor of other biological compounds, but it can be synthesized by the body, it may be considered a semi-essential nutrient that may be limited under certain physiological conditions (Gonzalez-Uarquin et al., 2019). In humans, myo-Ins has been shown to potentially reduce the symptoms of metabolic syndrome, including insulin resistance and dyslipidemia (Croze and Soulage, 2013), as well as affect other endocrine disorders such as polycystic ovary syndrome (Unfer et al., 2012), all of which relate to its role as an insulin mimetic. As modern chickens are insulin resistant (Stout et al., 1973; Scanes, 2009; Shiraishi et al., 2011), myo-Ins may be both a viable marker and treatment to help mitigate the negative consequences of this problem in both health and production parameters. Indeed, as myo-Ins may prevent ectopic fat deposition in mice (Plows et al., 2017) and deficiency has been associated with increased hepatic triglycerides in rat (Hayashi et al., 1974), it is conceivable that it may also be correlated with fat deposition in poultry. Though this has yet to be explored, Gonzalez-Uarquin et al. (2019) suggest tracking myo-Ins over the lifespan of the bird in order to monitor lipid metabolism.
Intracellular levels of myo-Ins depend on intestinal absorption, cellular synthesis, and excretion (Croze and Soulage, 2013; Dinicola et al., 2017). Intracellular myo-Ins can be formed in two ways: de novo synthesis from glucose and hydrolysis of phosphatidylinositol, phosphoinositides, and inositol phosphates (Loewus and Loewus, 1983). In this study, supplementary phytase or myo-Ins increased the concentrations of myo-Ins in circulation and feather, likely decreasing the need for further synthesis. Evidence for down regulation of both the cellular de novo and hydrolysis pathways is evident in the initial post-prandial decreases in gene expression of ISYNA and INPP1 in blood and MINPP1 in both blood and feather. Microbial phytases have varying ability to de-phosphorylate phytate, with some yielding to InsP3 (Haefner et al., 2005), and others able to yield InsP1 (Wyss et al., 1999). Interestingly however, avian MINPP1 has phytase hydrolyzing activity 100 fold greater than its mammalian counterpart (Cho et al., 2006), and is able to convert 80% of phytate to InsP1 (Cho et al., 2006).
The inositol hexakisphosphate kinases (IP6K1-3) are flexible in their function. These enzymes generate inositol pyrophosphates from InsP5, but also dephosphorylate InsP6 to InsP5 in the presence of a decreasing ATP/ADP ratio (Wundenberg et al., 2014). In both blood and feather, there was an immediate post-prandial decrease in the gene expression of IP6K1, IP6K2, and IP6K3. This may reflect the positive energy balance associated with food consumption or the reduction in need for inositol pyrophosphates. These enzymes also play roles in insulin sensitivity (Kamimura et al., 2004; Illies et al., 2007) and apoptosis (Thomas and Potter, 2014), which may help explain the physiological effects of myo-Ins.
In summary, this is the first report, to our knowledge, showing kinetic variations of myo-Ins in both circulation and feathers and suggest feather myo-Ins levels as a reliable non-invasive marker to study phytate hydrolysis in chickens. These findings can allow for better understanding of phytase usage and phytate metabolism in poultry health, disease, and productivity.
Data Availability Statement
All datasets presented in this study are included in the article/supplementary material.
Ethics Statement
The animal study was reviewed and approved by Institutional Animal Care and Use Committee at the University of Arkansas.
Author Contributions
SD conceived and designed the study. EG, BM, and SD conducted the experiments, determined gene and protein expression, and analyzed the data. JW and AC provided the HiPhos. SD and EG wrote the manuscript.
Funding
This study was supported by a grant from DSM Animal Nutrition and Health (Kaiseraugst, Switzerland) (to SD). The funder was not involved in the study design, collection, analysis, interpretation of data, the writing of this article or the decision to submit it for publication.
Conflict of Interest
The remaining authors declare that the research was conducted in the absence of any commercial or financial relationships that could be construed as a potential conflict of interest.
References
Angamuthu, R., Baskaran, S., Gopal, D. R., Devarajan, J., and Kathaperumal, K. (2012). Rapid detection of the Marek’s Disease viral genome in chicken feathers by loop-mediated isothermal amplification. J. Clin. Microbiol. 50, 961–965. doi: 10.1128/jcm.05408-11
Battaglia, F. C., Meschia, G., Blechner, J. N., and Barron, D. H. (1961). The free myo-inositol concentration of adult and fetal tissues of several species. Q. J. Exp. Physiol. Cognate Med. Sci. 46, 188–193. doi: 10.1113/expphysiol.1961.sp001532
Bayer, D., Mohan, K., Jayakumar, K., Milad, M., and Pavithra, B. (2012). Simple cannulation procedure for serial blood sampling through cutaneous ulnar vein in chickens. J. Appl. Anim. Welfare Sci. 15, 91–100. doi: 10.1080/10888705.2012.624925
Bedford, M. R. (2000). Exogenous enzymes in monogastric nutrition — their current value and future benefits. Anim. Feed Sci. Technol. 86, 1–13. doi: 10.1016/S0377-8401(00)00155-3
Carbajal, A., Tallo-Parra, O., Sabes-Alsina, M., Mular, I., and Lopez-Bejar, M. (2014). Feather corticosterone evaluated by ELISA in broilers: a potential tool to evaluate broiler welfare. Poult. Sci. 93, 2884–2886. doi: 10.3382/ps.2014-04092
Cheryan, M., and Rackis, J. J. (1980). Phytic acid interactions in food systems. Crit. Rev. Food Sci. Nutr. 13, 297–335. doi: 10.1080/10408398009527293
Chhetri, D. R. (2019). Myo-Inositol and its derivatives: their emerging role in the treatment of human dseases. Front. Pharmacol. 10:1172. doi: 10.3389/fphar.2019.01172
Cho, J., Choi, K., Darden, T., Reynolds, P. R., Petitte, J. N., and Shears, S. B. (2006). Avian multiple inositol polyphosphate phosphatase is an active phytase that can be engineered to help ameliorate the planet’s “phosphate crisis”. J. Biotechnol. 126, 248–259. doi: 10.1016/j.jbiotec.2006.04.028
Coweison, A. J., Aureli, R., Guggenbuhl, P., and Fru-Nji, F. (2015). Possible involvement of myo-inositol in the physiological response of broilers to high doses of microbial phytase. Anim. Prod. Sci. 55, 710–719.
Cowieson, A. J., Acamovic, T., and Bedford, M. R. (2006). Phytic acid and phytase: implications for protein utilization by poultry. Poult. Sci. 85, 878–885. doi: 10.1093/ps/85.5.878
Croze, M. L., and Soulage, C. O. (2013). Potential role and therapeutic interests of myo-inositol in metabolic diseases. Biochimie 95, 1811–1827. doi: 10.1016/j.biochi.2013.05.011
Davidson, I., and Borenshtain, R. (2002). The feather tips of commercial chickens are a favorable source of DNA for the amplification of Marek’s disease virus and avian leukosis virus, subgroup. J. Avian Pathol. 31, 237–240. doi: 10.1080/03079450220136549
Dilger, R. N., Onyango, E. M., Sands, J. S., and Adeola, O. (2004). Evaluation of microbial phytase in broiler diets. Poult. Sci. 83, 962–970. doi: 10.1093/ps/83.6.962
Dinicola, S., Minini, M., Unfer, V., Verna, R., Cucina, A., and Bizzarri, M. (2017). Nutritional and acquired deficiencies in inositol bioavailability. Correlations with metabolic disorders. Int. J. Mol. Sci. 18:2187. doi: 10.3390/ijms18102187
Dos Santos, T. T., Srinongkote, S., Bedford, M. R., and Walk, C. L. (2013). Effect of high phytase inclusion rates on performance of broilers fed diets not severely limited in available phosphorus. Asian Austral. J. Anim. Sci. 26, 227–232. doi: 10.5713/ajas.2012.12445
Eeckhout, W., and De Paepe, M. (1994). Total phosphorus, phytate-phosphorus and phytase activity in plant feedstuffs. Anim. Feed Sci. Technol. 47, 19–29. doi: 10.1016/0377-8401(94)90156-2
Frieler, R. A., Mitteness, D. J., Golovko, M. Y., Gienger, H. M., and Rosenberger, T. A. (2009). Quantitative determination of free glycerol and myo-inositol from plasma and tissue by high-performance liquid chromatography. J. Chrom. B 877, 3667–3672. doi: 10.1016/j.jchromb.2009.09.015
Gonzalez-Uarquin, F., Rodehutscord, M., and Huber, K. (2019). Myo-inositol: its metabolism and potential implications for poultry nutrition—a review. Poult. Sci. 99, 893–905. doi: 10.1016/j.psj.2019.10.014
Greene, E. S., Rajaei-Sharifabadi, H., and Dridi, S. (2019). Feather HSP70: a novel non-invasive molecular marker for monitoring stress induced by heat exposure in broilers. Poult. Sci. 98, 3400–3404. doi: 10.3382/ps/pez120
Haefner, S., Knietsch, A., Scholten, E., Braun, J., Lohscheidt, M., and Zelder, O. (2005). Biotechnological production and applications of phytases. Appl. Microbiol. Biotechnol. 68, 588–597. doi: 10.1007/s00253-005-0005-y
Hayashi, E., Maeda, T., and Tomita, T. (1974). The effect of myo-inositol deficiency on lipid metabolism in rats: I. The alteration of lipid metabolism in myo-inositol deficient rats. Biochim. Biophys. Acta Lipids Lipid Metab. 360, 134–145. doi: 10.1016/0005-2760(74)90163-5
Illies, C., Gromada, J., Fiume, R., Leibiger, B., Yu, J., Juhl, K., et al. (2007). Requirement of inositol pyrophosphates for full exocytotic capacity in pancreatic β cells. Science 318, 1299–1302. doi: 10.1126/science.1146824
Jaspers, V. L. B., Rodriguez, F. S., Boertmann, D., Sonne, C., Dietz, R., Rasmussen, L. M., et al. (2011). Body feathers as a potential new biomonitoring tool in raptors: a study on organohalogenated contaminants in different feather types and preen oil of West Greenland white-tailed eagles (Haliaeetus albicilla). Environ. Int. 37, 1349–1356. doi: 10.1016/j.envint.2011.06.004
Kamimura, J., Wakui, K., Kadowaki, H., Watanabe, Y., Miyake, K., Harada, N., et al. (2004). The IHPK1 gene is disrupted at the 3p21. 31 breakpoint of t (3; 9) in a family with type 2 diabetes mellitus. J. Hum. Genet. 49, 360–365. doi: 10.1007/s10038-004-0158-z
Leung, K.-Y., Mills, K., Burren, K. A., Copp, A. J., and Greene, N. D. E. (2011). Quantitative analysis of myo-inositol in urine, blood and nutritional supplements by high-performance liquid chromatography tandem mass spectrometry. J. Chrom. B 879, 2759–2763. doi: 10.1016/j.jchromb.2011.07.043
Lewin, L. M., Yannai, Y., Sulimovici, S., and Kraicer, P. F. (1976). Studies on the metabolic role of myo-inositol. Distribution of radioactive myo-inositol in the male rat. Biochem. J. 156, 375–380. doi: 10.1042/bj1560375
Liu, S. Y., Cadogan, D. J., Péron, A., Truong, H. H., and Selle, P. H. (2014). Effects of phytase supplementation on growth performance, nutrient utilization and digestive dynamics of starch and protein in broiler chickens offered maize-, sorghum- and wheat-based diets. Anim. Feed Sci. Technol. 197, 164–175. doi: 10.1016/j.anifeedsci.2014.08.005
Loewus, F. A., and Loewus, M. W. (1983). Myo-inositol: its biosynthesis and metabolism. Ann. Rev. Plant Physiol. 34, 137–161.
Maenz, D. D., and Classen, H. L. (1998). Phytase activity in the small intestinal brush border membrane of the chicken. Poult. Sci. 77, 557–563. doi: 10.1093/ps/77.4.557
Maga, J. A. (1982). Phytate: its chemistry, occurrence, food interactions, nutritional significance, and methods of analysis. J. Agr. Food Chem. 30, 1–9. doi: 10.1021/jf00109a001
Minozzi, M., D’Andrea, G., and Unfer, V. (2008). Treatment of hirsutism with myo-inositol: a prospective clinical study. Reprod. Biomed. Online 17, 579–582. doi: 10.1016/s1472-6483(10)60248-9
Mullaney, E. J., Daly, C. B., and Ullah, A. H. (2000). Advances in phytase research. Adv. Appl. Microbiol. 47, 157–199. doi: 10.1016/s0065-2164(00)47004-8
Plimmer, R. H., and Page, H. J. (1913). An investigation of phytin. Biochem. J. 7, 157–174. doi: 10.1042/bj0070157
Plows, J. F., Budin, F., Andersson, R. A. M., Mills, V. J., Mace, K., Davidge, S. T., et al. (2017). The effects of myo-inositol and B and D Vitamin supplementation in the db/+ mouse model of gestational diabetes mellitus. Nutrients 9:141. doi: 10.3390/nu9020141
Rajaei-Sharifabadi, H., Ellestad, L., Porter, T., Donoghue, A., Bottje, W. G., and Dridi, S. (2017). Noni (Morinda citrifolia) modulates the hypothalamic expression of stress- and metabolic-related genes in broilers exposed to acute heat stress. Front. Genet. 8:192. doi: 10.3389/fgene.2017.00192
Ravindran, V. (1995). Phytases in poultry nutrition. An overview. Proc. Aust. Poult. Sci. Symp. 7, 135–139.
Rutherfurd, S., Chung, T., Morel, P., and Moughan, P. (2004). Effect of microbial phytase on ileal digestibility of phytate phosphorus, total phosphorus, and amino acids in a low-phosphorus diet for broilers. Poult. Sci. 83, 61–68. doi: 10.1093/ps/83.1.61
Sakamoto, K., Vucenik, I., and Shamsuddin, A. M. (1993). [3H] Phytic acid (inositol hexaphosphate) is absorbed and distributed to various tissues in rats. J. Nutr. 123, 713–720. doi: 10.1093/jn/123.4.713
Scanes, C. G. (2009). Perspectives on the endocrinology of poultry growth and metabolism. Gen. Comp. Endocrinol. 163, 24–32. doi: 10.1016/j.ygcen.2009.04.013
Schmeisser, J., Séon, A. A., Aureli, R., Friedel, A., Guggenbuhl, P., Duval, S., et al. (2017). Exploratory transcriptomic analysis in muscle tissue of broilers fed a phytase-supplemented diet. J. Anim. Physiol. Anim. Nutr. 101, 563–575. doi: 10.1111/jpn.12482
Schmittgen, T. D., and Livak, K. J. (2008). Analyzing real-time PCR data by the comparative CT method. Nat. Protoc. 3, 1101–1108. doi: 10.1038/nprot.2008.73
Selle, P. H., and Ravindran, V. (2007). Microbial phytase in poultry nutrition. Anim. Feed Sci. Technol. 135, 1–41. doi: 10.1016/j.anifeedsci.2006.06.010
Shiraishi, J.-I., Yanagita, K., Fukumori, R., Sugino, T., Fujita, M., Kawakami, S.-I., et al. (2011). Comparisons of insulin related parameters in commercial-type chicks: evidence for insulin resistance in broiler chicks. Physiol. Behav. 103, 233–239. doi: 10.1016/j.physbeh.2011.02.008
Sommerfeld, V., Künzel, S., Schollenberger, M., Kühn, I., and Rodehutscord, M. (2018a). Influence of phytase or myo-inositol supplements on performance and phytate degradation products in the crop, ileum, and blood of broiler chickens. Poult. Sci. 97, 920–929. doi: 10.3382/ps/pex390
Sommerfeld, V., Schollenberger, M., Kühn, I., and Rodehutscord, M. (2018b). Interactive effects of phosphorus, calcium, and phytase supplements on products of phytate degradation in the digestive tract of broiler chickens. Poult. Sci. 97, 1177–1188. doi: 10.3382/ps/pex404
Stout, R. W., Buchanan, K. D., and Vallance-Owen, J. (1973). The relationship of arterial disease and glucagon metabolism in insulin-treated chickens. Atherosclerosis 18, 153–162. doi: 10.1016/0021-9150(73)90126-3
Thomas, M. P., and Potter, B. V. L. (2014). The enzymes of human diphosphoinositol polyphosphate metabolism. FEBS J. 281, 14–33. doi: 10.1111/febs.12575
Unfer, V., Carlomagno, G., Dante, G., and Facchinetti, F. (2012). Effects of myo-inositol in women with PCOS: a systematic review of randomized controlled trials. Gynecol. Endocrinol. 28, 509–515. doi: 10.3109/09513590.2011.650660
Viveros, A., Centeno, C., Brenes, A., Canales, R., and Lozano, A. (2000). Phytase and acid phosphatase activities in plant feedstuffs. J. Agri. Food Chem. 48, 4009–4013. doi: 10.1021/jf991126m
Waldroup, P. W. (1999). Nutritional approaches to reducing phosphorus excretion by poultry. Poult. Sci. 78, 683–691. doi: 10.1093/ps/78.5.683
Weimer, S. L., Wideman, R. F., Scanes, C. G., Mauromoustakos, A., Christensen, K. D., and Vizzier-Thaxton, Y. (2018). An evaluation of methods for measuring stress in broiler chickens. Poult. Sci. 97, 3381–3389. doi: 10.3382/ps/pey204
Wundenberg, T., Grabinski, N., Lin, H., and Mayr, G. W. (2014). Discovery of InsP6-kinases as InsP6-dephosphorylating enzymes provides a new mechanism of cytosolic InsP6 degradation driven by the cellular ATP/ADP ratio. Biochem. J. 462, 173–184. doi: 10.1042/BJ20130992
Keywords: phytate hydrolysis, myoinositol, phytase, gene expression, feather, broilers
Citation: Greene E, Mallmann B, Wilson JW, Cowieson AJ and Dridi S (2020) Monitoring Phytate Hydrolysis Using Serial Blood Sampling and Feather Myo-Inositol Levels in Broilers. Front. Physiol. 11:736. doi: 10.3389/fphys.2020.00736
Received: 08 April 2020; Accepted: 08 June 2020;
Published: 26 June 2020.
Edited by:
Yajun Wang, Sichuan University, ChinaReviewed by:
Sergio Luiz Vieira, Federal University of Rio Grande do Sul, BrazilVasil Pirgozliev, Harper Adams University, United Kingdom
Copyright © 2020 Greene, Mallmann, Wilson, Cowieson and Dridi. This is an open-access article distributed under the terms of the Creative Commons Attribution License (CC BY). The use, distribution or reproduction in other forums is permitted, provided the original author(s) and the copyright owner(s) are credited and that the original publication in this journal is cited, in accordance with accepted academic practice. No use, distribution or reproduction is permitted which does not comply with these terms.
*Correspondence: Sami Dridi, dridi@uark.edu
†Present address: Barbara Mallmann, Adisseo France S.A.S., CERN, Commentry, France