- 1Developmental Programming Research Group, The Liggins Institute, The University of Auckland, Auckland, New Zealand
- 2Saban Research Institute, Children’s Hospital Los Angeles, Los Angeles, CA, United States
- 3UCD School of Public Health, Physiotherapy and Sports Science, University College Dublin, Dublin, Ireland
- 4Conway Institute/Institute of Food and Health, University College Dublin, Dublin, Ireland
Background: The global incidence of obesity continues to rise, increasing the prevalence of metabolic diseases such as insulin resistance, dyslipidemia, and type 2 diabetes mellitus. Low-grade chronic inflammation, associated with the obese state, also contributes to the development of these metabolic comorbidities. Interleukin-1-receptor-1 (IL-1R1), a pro-inflammatory mediator, bridges the metabolic and inflammatory systems. In young male mice, deficiency of IL-1R1 (IL-1R1−/−) paired with a high-fat diet (HFD) offered beneficial metabolic effects, however in female mice, the same pairing led to metabolic dysfunction. Therefore, we examined the contribution of maternal HFD in combination with IL1R1−/− to metabolic health in adult offspring.
Methods: Female C57BL/6 and IL-1R1−/− mice were randomly assigned to a control diet (10% kcal from fat) or HFD (45% kcal from fat) 10 days prior to mating and throughout gestation and lactation. Male and female offspring were housed in same-sex pairs post-weaning and maintained on control diets until 16 weeks old. At 15 weeks, an oral glucose tolerance test (OGTT) was performed to assess glucose tolerance. Histological analysis was carried out to assess adipocyte size and gene expression of adipogenic and inflammatory markers were examined.
Results: IL-1R1−/− contributed to increased body weight in male and female adult offspring, irrespective of maternal diet. IL-1R1−/− and maternal HFD increased adipocyte size in the gonadal fat depot of female, but not male offspring. In female offspring, there was reduced expression of genes involved in adipogenesis and lipid metabolism in response to IL1R1−/− and maternal HFD. While there was an increase in inflammatory gene expression in response to maternal HFD, this appeared to be reversed in IL1R1−/− female offspring. In male offspring, there was no significant impact on adipogenic or lipid metabolism pathways. There was an increase in inflammatory gene expression in IL1R1−/− male offspring from HFD-fed mothers.
Conclusion: This study suggests that IL-1R1 plays a complex and important role in the metabolic health of offspring, impacting adipogenesis, lipogenesis, and inflammation in a sex-specific manner.
Introduction
Obesity is quickly becoming the most common chronic health condition globally and is instrumental in the development of co-morbidities such as dyslipidemia, insulin resistance (IR), and type 2 diabetes (Blüher, 2019). Low-grade chronic inflammation is strongly associated with obesity and is thought to be one of the major contributory factors in progression to overt metabolic disease (Saltiel and Olefsky, 2017). There is now clear evidence that exposure to maternal dietary, emotional, and environmental stressors during gestation can predispose offspring to obesity and metabolic dysfunction in later life (Reynolds et al., 2017; McGowan and Matthews, 2018). However, there is limited knowledge around the impact of metabolic inflammation during pregnancy and its effects on the next generation (Segovia et al., 2017).
There has been a robust effort over recent years to understand the molecular mediators involved in metabolic inflammation. Interleukin (IL)-1β has been continuously implicated as a major driving force in the pathogenesis of metabolic disease (Jager et al., 2007; Koenen et al., 2011). Previous studies have demonstrated that deletion of IL-1R1 signaling prevents the onset of IR in a mouse model of diet-induced obesity (McGillicuddy et al., 2011; Finucane et al., 2015). It is notable that this protection is reserved exclusively to younger male mice with detrimental metabolic effects of IL-1R1 depletion observed in older male mice (García et al., 2006; McGillicuddy et al., 2013).
IL-1 signaling during pregnancy has been associated with deleterious effects including increased activation and expression observed in woman with gestational diabetes mellitus [GDM; Katra et al. (2016)] and pre-eclampsia (Siljee et al., 2013). While IL-1 signaling has a functional role during normal pregnancy, particularly in the induction of labor, increased concentrations as a result of infection or obesity-induced meta-inflammation can alter the hormonal balance which is required for maintenance of normal pregnancy (Allport et al., 2001; Terzidou et al., 2006). Additionally, several groups have identified an association between single nucleotide polymorphisms in genes related to IL-1 signaling and increased risk for pregnancy complications (Li et al., 2014; Nair et al., 2014). However, a recent study by our group has identified that IL-1 signaling may be important for maintaining metabolic health in female mice, with depletion resulting in increased adipocyte size and metabolic dysregulation pre, during, and post pregnancy (Plows et al., 2019). A fine balance exists between the inflammatory milieu and metabolic health and is likely altered in response to factors such as age and sex.
Early life-exposure to inflammatory agents such as bacterial lipotoxin results in programming of immune function (Mandal et al., 2013). However, the effect of maternal “meta-inflammation” resulting from obesity and high fat (HF) consumption has not been comprehensively examined within the developmental programming of obesity and metabolic disease paradigm. This study therefore examined the effect of a maternal HF diet during pregnancy and the potential contribution of IL-1 signaling to metabolic programming in adult offspring with a focus on adipose tissue effects.
Materials and Methods
Animal Procedures
All animal procedures were approved by the AgResearch Animal Ethics Committee in accordance with the New Zealand Animal Welfare Act, 1999. IL1R1−/− (B6.129S7-IL1R1tm1lmx/J) and C57BL/6J breeding pairs were imported from the Jackson Laboratory, USA, and housed in a animal containment facility at AgResearch, Ruakura, Waikato, New Zealand (22°C, lights on at 06:00 h, off at 18:00 h, humidity at 40–45%, and woodchip bedding). Third-generation female pups were given free access to standard laboratory chow until 10 weeks of age, at which time they were randomly assigned to receive either purified control diet (CD; Research Diets Inc., New Brunswick, USA; #D12450H, 20% kcal from protein, 70% kcal carbohydrate, and 10% kcal fat) or matched (for protein and micronutrient content) HF diet (HFD, Research Diets Inc.; #D12451, 20% kcal from protein, 35% kcal carbohydrate, and 45% kcal fat). This generated four groups (n = 8/group) in a balanced experimental design: (1) C57CD (C57BL/6J fed a CD), (2) C57HF (C57BL/6J fed a HFD), (3) IL1CD (IL1R1−/− fed a CD), and (4) IL1HF (IL1R1−/− fed a HFD). Mice began diets 10 days before mating and throughout pregnancy and lactation. At postnatal day 2 (PD2), litters were weighed and sexed using anogenital distance and were reduced to three males and three females to ensure standardized nutrition until weaning. Weaning occurred at PD21 (n = 6–8 litters/group). Offspring were housed in same-sex sibling groups and weighed weekly from week 3 to week 16. An oral glucose tolerance test (OGTT) was carried out 1 week prior to cull.
Oral Glucose Tolerance Test (OGTT)
Offspring were fasted for 6 h and then weighed. The tip of the tail (<1 mm) was cut and the resulting blood was read by glucometer (Optimum Freestyle Neo, Abbott Laboratories, Alameda, CA, USA). Two grams per kilogram of D-glucose (Sigma-Aldrich, NZ) was orally gavaged, and blood glucose concentrations were measured at 0, 15, 30, 60, 90, and 120 min.
Tissue Collection
Mice were fasted for 6 h and culled by cervical dislocation. A cardiac puncture was performed immediately following death and blood was collected in an EDTA coated tube and centrifuged at 2,000 rpm for 10 min at 4°C. Resulting plasma was stored at −20°C. Organs were dissected, weighed, and snap-frozen in liquid nitrogen and then stored at −80°C or fixed in neutral-buffered formalin for histological analysis.
Plasma Analysis
Fasting plasma insulin, leptin, and testosterone concentrations were analyzed using commercial mouse-specific ELISAs (UltraSensitive Mouse Insulin ELISA #90080; Mouse Leptin ELISA #90030; Mouse Testosterone ELISA 80552; Crystal Chem., Chicago, IL, USA), according to manufacturers’ instructions.
Histological Analysis
Gonadal adipose tissue samples were fixed in 10% NBF and were paraffin embedded and sectioned (10 μm) using a Leica RM 2135 rotary microtome (Leica Instruments, Nussloch, Germany). Hematoxylin and eosin (H&E) staining was performed, and sections were mounted using DPX mountant. Slides were visualized under a light microscope, and images captured with NIS Elements-D software (Nikon 800, Tokyo, Japan). Four representative images were taken from each section by an individual blinded to the study groups. A minimum of 100 adipocytes per animal were analyzed. Any cells that were not fully visible were not included in the analysis. Cells that were 150 μm or under were excluded to ensure that stromal vascular cells were not analyzed. Images were analyzed using ImageJ to determine average adipocyte size and adipocyte size distribution per sample.
Gene Expression Analysis
RNA was extracted from gonadal adipose tissue using TRI Reagent and stored at −80°C. Single-stranded cDNA was prepared using RT2 First Strand Kit (SABioscience; Qiagen, Hilden, Germany). The expression of 84 genes relevant to IR was analyzed using the Mouse Insulin Resistance RT2 Profiler PCR Array (SABioscience). mRNA expression was quantified by real-time PCR (RT-PCR) on a LightCycler® 480 SYBR Green I Master (Roche Diagnostics, Auckland, New Zealand). To control between-sample variability, mRNA levels were normalized to the geometric mean of a panel of housekeeping genes for each sample by subtracting the Cycle threshold (Ct) of controls from the Ct for the gene of interest producing a ΔCt value. The ΔCt for each treatment sample was compared to the mean ΔCt for control samples using the relative quantification 2-(ΔΔCt) method to determine fold change (Livak and Schmittgen, 2001).
Statistical Analysis
Statistical analysis was performed using SPSS 24 (IBM, Armonk, NY, USA). Repeated measure ANOVA was performed for the OGTT and weight trajectory data. All other data were analyzed by two-way factorial ANOVA, with genotype and maternal diet as factors. Outliers were assessed as any value greater than 1.5 box-lengths from the edge of each group’s boxplot, and were subsequently winsorized. The Shapiro–Wilk’s test was then performed to assess normality of the data, and Levene’s test was used to assess homogeneity of variances. In the event that one or more groups were not normally distributed and/or homogenous, the data were suitably transformed. Bonferroni post hoc tests were performed for multiple comparisons testing between groups when ANOVA found a significant effect. Differences between groups were considered significant at p < 0.05. Data are presented as mean ± SEM and graphed using Prism 6 software (GraphPad Software Inc., La Jolla, USA).
Results
IL-1R1−/− Increased Glucose Concentrations and Body Weight Irrespective of Maternal Diet in Female and Male Offspring
IL-1R1−/− induced an increase in the body weight of female and male offspring by 16 weeks of age irrespective of maternal diet. This was associated with a significant increase in fasting plasma glucose and gonadal fat mass (Table 1). There was a reduction in OGTT area under the curve (AUC) in female IL-1HF offspring compared to C57CD and C57HF groups creating an overall genotype effect (Figures 1A,B). In male offspring, there was a significant increase in OGTT AUC in IL-1R1−/− compared to C57BL/6 groups (Figures 1D,E). Maternal diet did not have an effect on weight, fasting glucose, OGTT, or fat mass in male and female offspring. However, there was a maternal diet effect on insulin concentrations in both female and male offspring. In females, C57HF had significantly increased insulin compared to all other groups (Figure 1C). Testosterone concentrations followed a similar pattern with significantly increased concentrations in C57HF compared to all other groups (Table 1). In male offspring, there was a significant increase in insulin concentrations in C57HF compared to C57CD and IL1HF groups and an interaction effect with an increase in IL1CD compared to C57CD and a decrease in IL1HF compared to C57HF (Figure 1F). There was a significant increase in IL1CD testosterone concentrations compared to all other groups (Table 1). There was no significant effect on leptin concentrations between groups (Table 1).
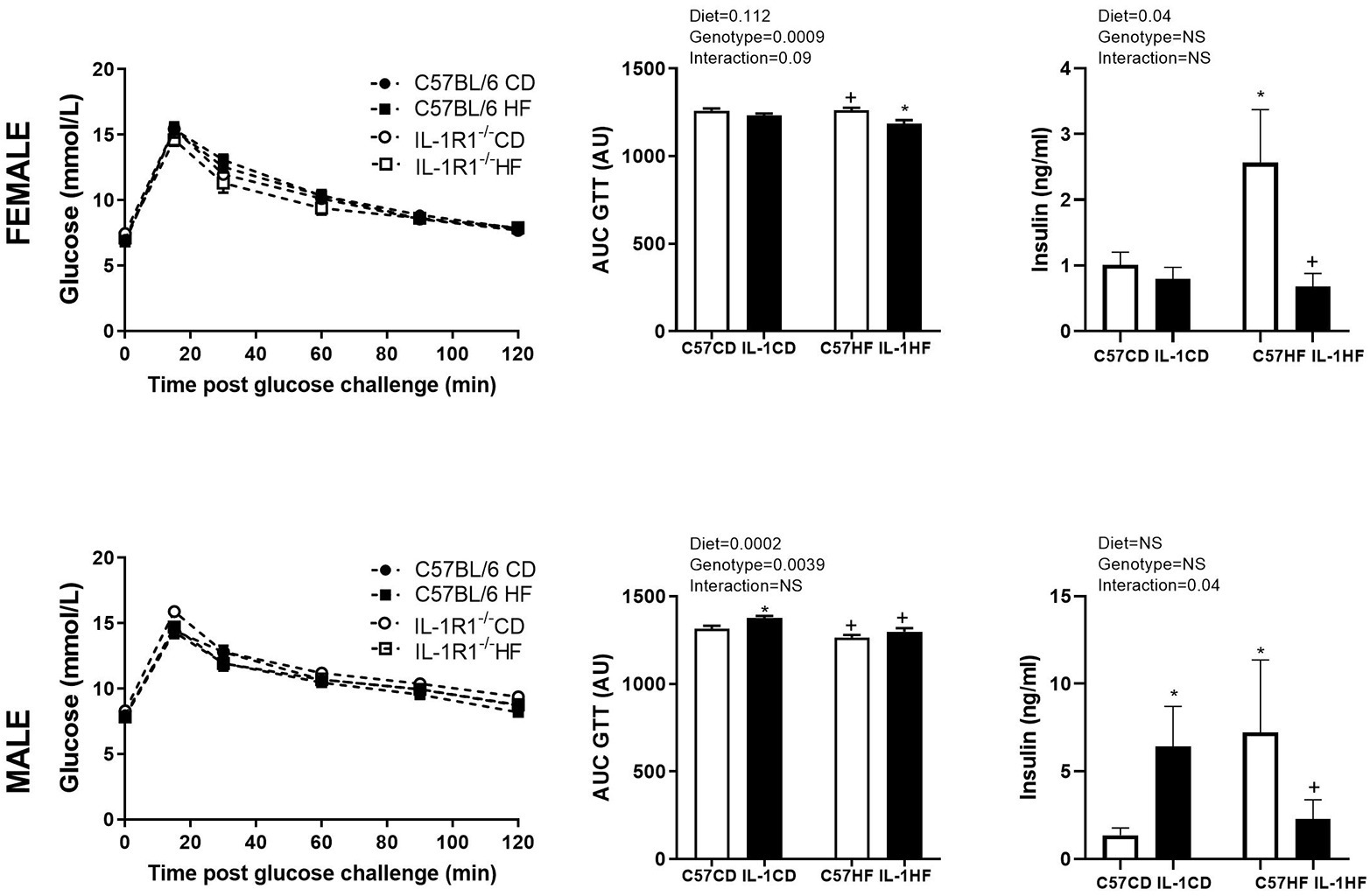
Figure 1. Impact of IL-1R1−/− and maternal high-fat diet (HFD) on offspring glucose tolerance and plasma insulin concentrations. Oral glucose tolerance test (OGTT) (2 g/kg) at 12 weeks in 6 h fasted female (A) and male (D) offspring. Area under the OGTT curve in female (B) and male (E) offspring. Insulin concentrations as measured by ELISA in female (C) and male offspring (F). Data are expressed as mean ± SEM. N = 6–8 litters/group. *p < 0.05 with respect to C57CD. +p < 0.05 with respect to C57HF.
Adipose Tissue Morphology and Adipogenic Gene Expression in Response to Maternal Diet and IL-1R1−/− Genotype
Maternal diet and IL-1R1−/− resulted in increased average adipocyte size in the gonadal fat pad of female offspring. All groups were significantly increased compared to C57CD. Further, there was a reduction in average adipocyte size between IL1CD and IL1HF groups (Figures 2A,B). When adipocyte size was delineated by size distribution, there was a clear shift to the right in the distribution curve in C57HF, IL1CD, and IL1HF groups compared to C57CD, with a reduction in smaller adipocytes and increased numbers of hypertrophic adipocytes (Figure 2C).
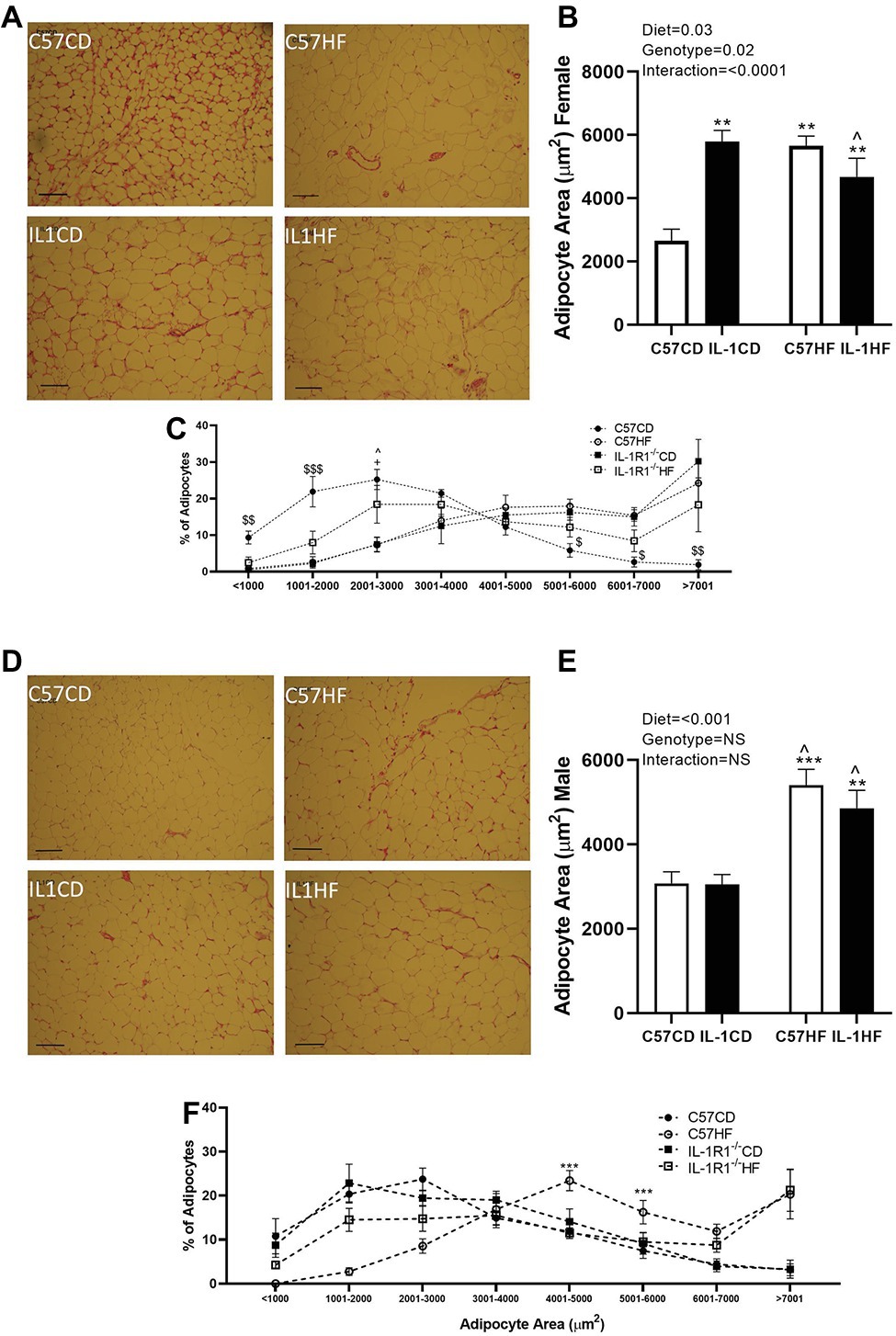
Figure 2. The effect of IL-1R1−/− and maternal HFD on offspring adipocyte size and distribution. Representative gonadal adipose tissue sections stained by hematoxylin and eosin (scale represents 200 μm) in female (A) and male (D) offspring. Mean adipocyte area analyzed by ImageJ. Four representative images were blindly analyzed per animal in female (B) and male (E) offspring. Adipocyte area distribution in female (C) and male (F) offspring. Data are expressed as mean ± SEM. N = 6–8 litters/group. **p < 0.01, ***p < 0.001 with respect to C57CD. +p < 0.05 with respect to C57HF. ^p < 0.05 with respect to IL1CD. $p < 0.05, $$p < 0.01, $$$p < 0.01, C57CD compared to all other groups.
There was a clear effect of maternal diet on adipocyte size in the gonadal fat pad of male offspring although there was no genotype effect (Figures 2D,E). The distribution of adipocyte size showed that C57HF and IL1HF had an increased percentage of adipocytes in the hypertrophic size category (Figure 2F).
In line with increased adipocyte size in female offspring, there was a reduction in the gene expression of a number of markers related to adipogenesis. While significance was not reached, there was a trend towards decreased peroxisome profilerator activated receptor gamma (Pparg) and resistin (Retn) expression in relation to maternal diet and genotype (Figures 3A,C). PPAR co-activator 1 alpha (Ppargc1) was significantly reduced with maternal diet with expression reduced in all groups compared to C57CD (Figure 3B). Insulin-like growth factor (Igf1) was increased with maternal diet (Figure 3D). There was a significant reduction in hormone sensitive lipase (Lipe) in IL-1R1−/− groups irrespective of maternal diet (Figure 3E). Stearoyl Co-A desaturase (Scd1) expression was significantly increased in C57HF compared to all other groups (Figure 3F).
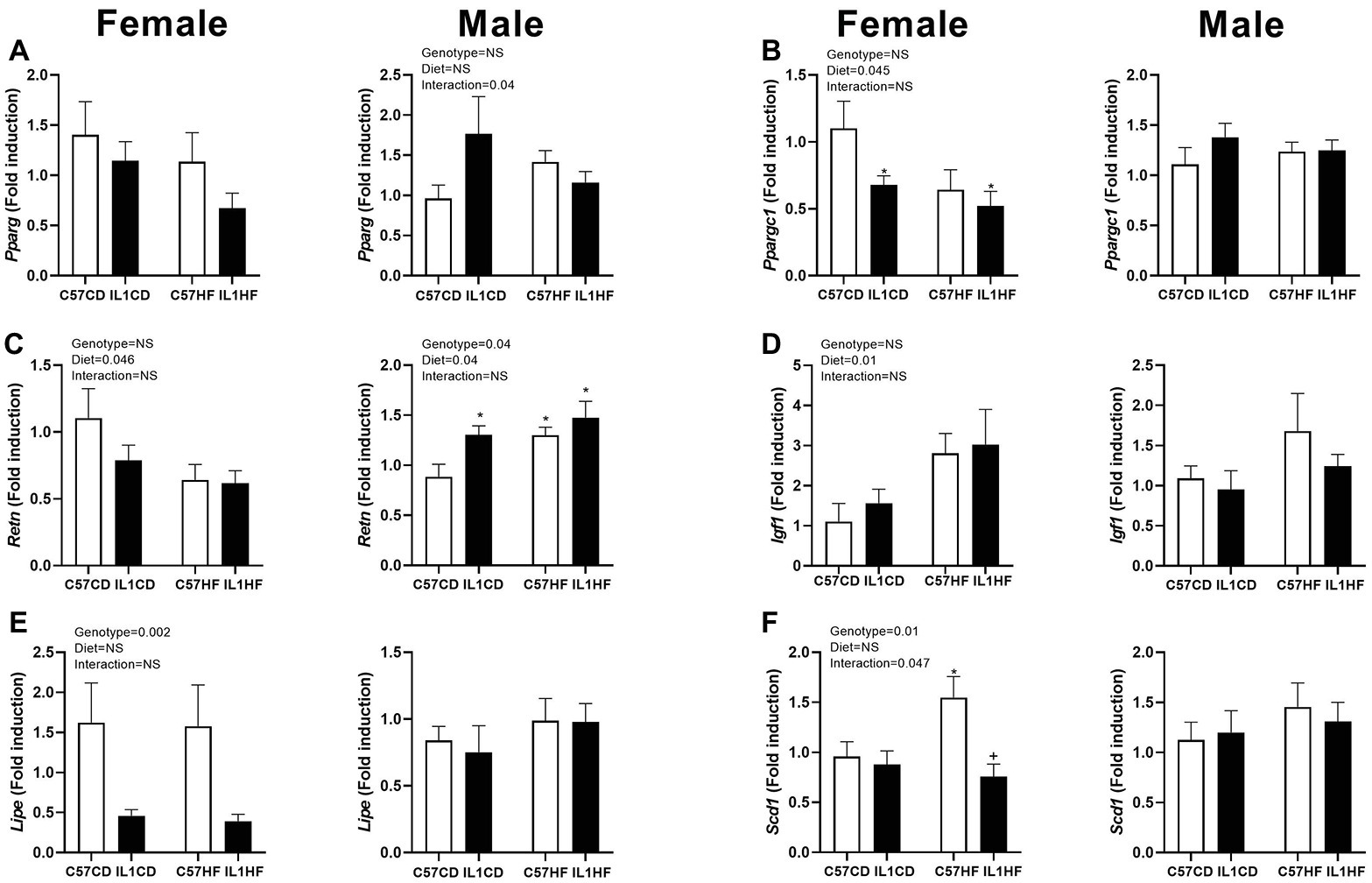
Figure 3. Gene expression of adipogenic markers in adipose tissue. Adipose tissue gene expression of markers related to adipogenesis. (A) Pparg, (B) Ppargc1, (C) Retn, (D) Igf1, (E) Lipe, and (F) Scd1. Data are expressed as mean ± SEM. N = 6–8 litters/group. *p < 0.05 with respect to C57CD. +p < 0.05 with respect to C57HF.
In the male offspring, IL1CD had increased Pparg expression compared to other groups creating an interaction effect (Figure 3A). Retn expression was increased in all groups compared to C57CD (Figure 3C). There was no significant difference between groups with Ppargc1, Igf1, Lipe, or Scd1 (Figures 3B,D–F).
IL-1R1−/− Genotype Alters Lipid Metabolism Gene Expression in Gonadal Adipose Tissue of Female but not Male Offspring
In the female offspring, there was a significant reduction in acetyl co-A carboxylase 2 (Acacb) gene expression in IL1CD compared to C57CD creating an overall genotype effect (Figure 4A). Long chain fatty acid Co-A ligase 1 (Acsl1) expression was reduced in IL-1R1−/− groups irrespective of maternal diet (Figure 4B). There was a significant increase in the leptin receptor (Lepr) expression in IL1CD group creating an overall genotype effect (Figure 4E). Oxidized low-density lipoprotein receptor 1 (Olr1) was reduced in IL1CD and IL1HF compared to C57CD, creating an overall genotype effect (Figure 4G).
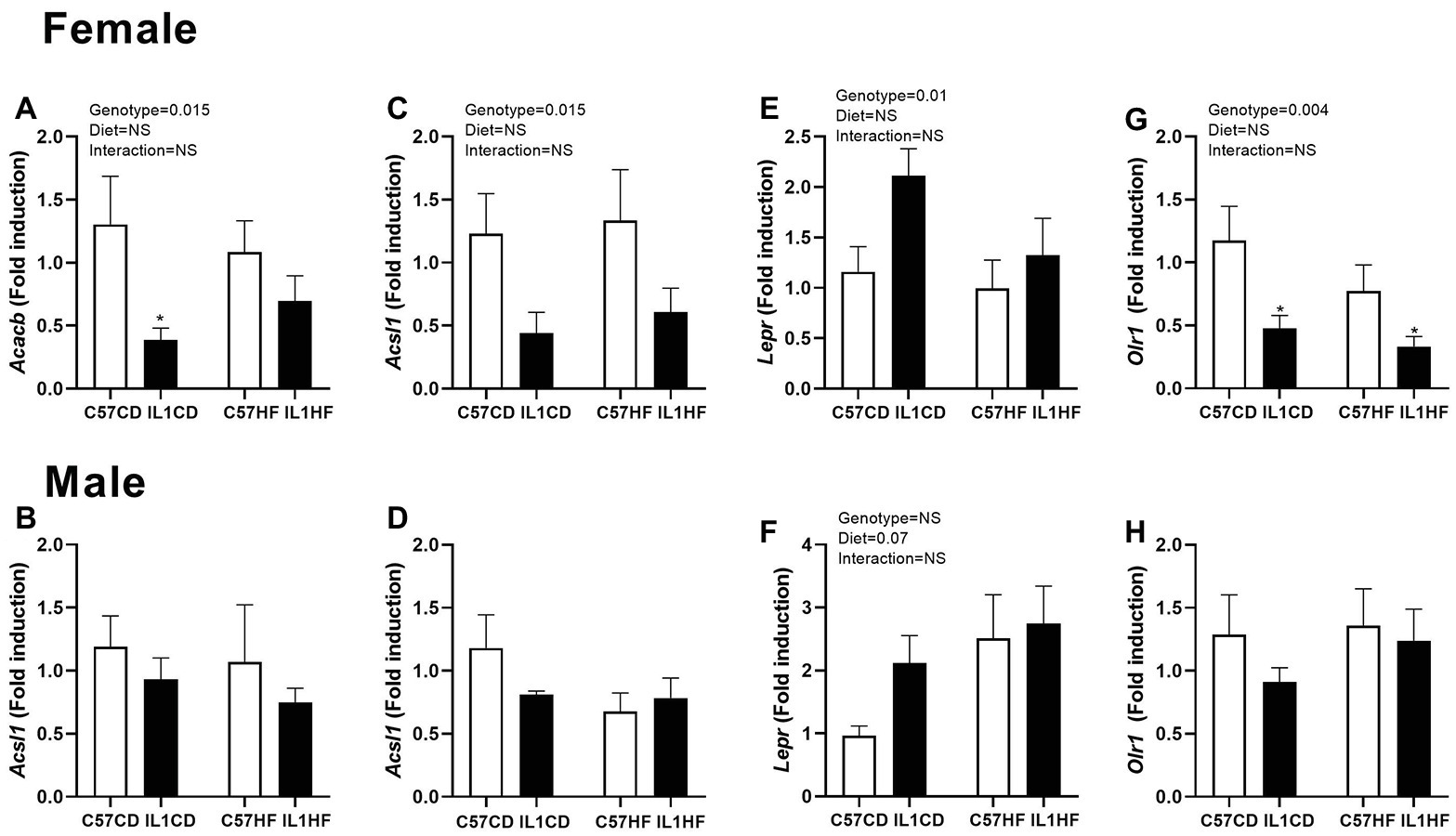
Figure 4. Gene expression of lipid metabolism markers in adipose tissue. Adipose tissue gene expression of markers related to adipogenesis. Acacb in female (A) and male (B) offspring, Acsl1 in female (C) and male (D) offspring, Lepr in female (E) and male (F) offspring, and Olr1 in female (G) and male (H) offspring. Data are expressed as mean ± SEM. N = 6–8 litters/group. *p < 0.05 with respect to C57CD.
There were no significant effects of either maternal diet or genotype on lipid metabolism markers in the male offspring (Figures 4B,D,F,H).
Differential Inflammatory Gene Expression Profiles in Female and Male Offspring in Response to Maternal Diet and Genotype
In the female offspring, there was a significant increase in the monocyte chemotactic protein 5 gene (Ccl12) and CC chemokine receptor (Ccr4) in C57HF compared to all other groups (Figures 5A,C). There was a reduction in tumor necrosis factor (Tnf) expression in IL-1R1−/− groups irrespective of maternal diet (Figure 5E). There was no difference between groups for mammalian target of rapamycin (mTor), toll-like receptor 4 (Tlr4), and Tnf receptor 2 (Tnfrsf1b) (Figures 5G,I,K).
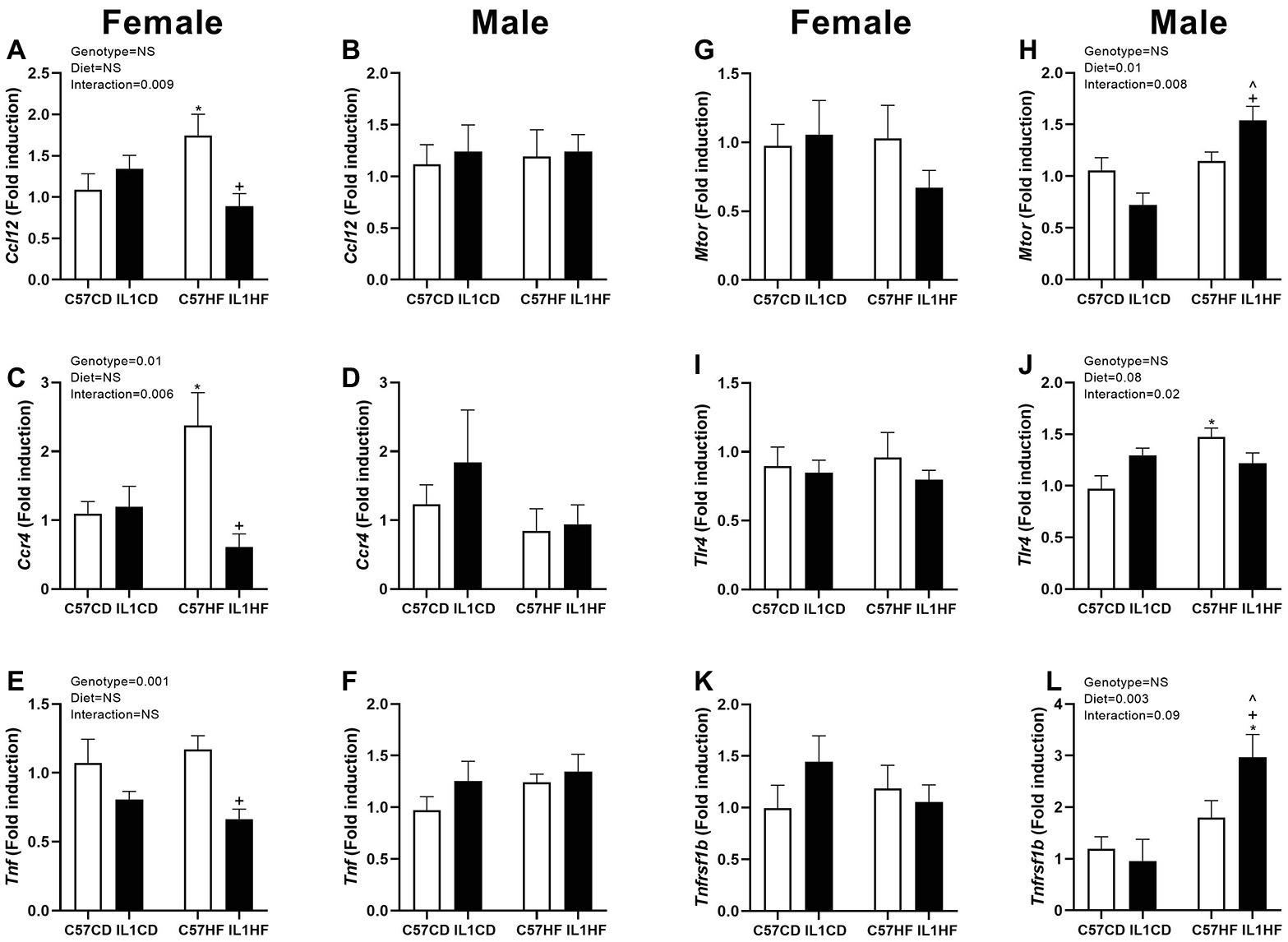
Figure 5. Adipose tissue gene expression of inflammatory markers in female and male offspring. Adipose tissue gene expression of markers related to inflammation. Ccl12 in female (A) and male (B) offspring, Ccr4 in female (C) and male (D) offspring, Tnf in female (E) and male (F) offspring, mTor in female (G) and male (H) offspring, Tlr4 in female (I) and male (J) offspring, and Tnfrsf1b in female (K) and male (L) offspring. Data are expressed as mean ± SEM. N = 6–8 litters/group. *p < 0.05 with respect to C57CD. +p < 0.05 with respect to C57HF. ^p < 0.05 with respect to IL1CD.
In the male offspring, there was no difference between groups for Ccl12, Ccr4, and Tnf (Figures 5B,D,F). mTor and Tnfrsf1b expressions were significantly increased in the IL1HF group contributing to an overall maternal diet effect. There was a decrease in IL1CD compared to all other groups creating an interaction effect (Figure 5H), however, this only reached significance with mTor (Figures 5H,L). Tlr4 was increased in the C57HF group compared to C57CD. This was partially normalized in the IL1HF group contributing to an interaction effect (Figure 5J).
Discussion
It is now well-accepted that maternal dietary stressors during pregnancy can have a marked impact on the risk of obesity and cardiometabolic disease in offspring during later life (Reynolds et al., 2017; Chang et al., 2019). Furthermore, the critical windows where early life nutrition and environment influence later metabolic health coincide with the development of the immune system. The IL-1 signaling pathway represents a point of intersection between metabolic and inflammatory systems, with previous work demonstrating a protective metabolic effect of IL-1R1 depletion in young male mice exposed to diet-induced obesity (McGillicuddy et al., 2011). However, it is clear that the role of this pathway in metabolic processes is complex, with evidence that older male mice become spontaneously obese and insulin resistant (García et al., 2006; McGillicuddy et al., 2013). A recent study from our group has also highlighted the detrimental effect of IL-1R1 depletion in nulliparous, pregnant, and postpartum female mice (Plows et al., 2019). Here, we demonstrated a reduction in weight gain and decreased kilocalorie consumption over pregnancy in IL-1R1−/− mice where there was no change in glucose tolerance and an increase in adipocyte size resulting in decreased adipose tissue insulin sensitivity. Given the complexity of this pathway in terms of metabolic regulation, we examined its impact in relation to metabolic programming of offspring adiposity and glucose tolerance. There were minimal changes in glucose tolerance, with protective effects of IL-1R1−/− on maternal HFD-induced programming of insulin concentration in both sexes. Both IL-1R1−/− and maternal HFD had a considerable negative impact on adipocyte size as well as lipid metabolism and adipogenic gene expression in female but not male offspring despite beneficial effects of IL-1R1−/− in inflammatory pathways.
In the current study, female offspring on an IL-1R1−/− genetic background remained heavier and had an increased fat mass. Despite this, maternal HFD-induced increases in insulin secretion were prevented with IL-1R1 depletion, accompanied by a moderate improvement in glucose tolerance. Following a similar trend to insulin, IL-1R1 depletion prevented elevation of maternal-HFD induced testosterone concentrations in female offspring. To our knowledge, there are no data examining the impact of IL-1R1 signaling on testosterone production. However, it is possible that elevated testosterone concentrations in the maternal HFD diet fed control animals may contribute to adverse metabolic outcomes such as hyperinsulinemia. Not only is there evidence implicating testosterone in the pathogenesis of insulin resistance in females (Corbould, 2008) but there are also studies which have demonstrated a developmental origin for polycystic ovary syndrome, characterized by increased testosterone and metabolic dysfunction (Franks, 2012).
There was no effect of maternal HFD on glucose tolerance, weight gain, or testosterone concentrations in male offspring. However, there was a programming effect on insulin concentrations. Despite preventing hyperinsulinemia, male offspring with an IL-1R1−/− background had increased, albeit modestly, glucose intolerance. This was accompanied by a significant increase in weight and fat mass. There is evidence that IL-1R1−/− mice become spontaneously obese and glucose intolerant as they age (García et al., 2006; McGillicuddy et al., 2013). These animals were culled at 16 weeks of age, this may represent a time-point at which this transition to a detrimental metabolic phenotype begins. Given the increase in fat mass in the IL1HF group, it is unlikely that IL-1R1 signaling represents a valid target for the effects of maternal HFD diet on developmental programming of obesity.
In line with overall increases in weight and fat mass, it is unsurprising to see an overall increase in adipocyte size in response to both maternal HFD and IL-1R1−/− background in female offspring. Maternal HFD preempted an increase in adipocyte size irrespective of genotype in male offspring. There is significant evidence showing that adipose tissue dysfunction is related to the enlargement of adipocytes. The ability of adipocytes to differentiate from preadipocytes has a huge impact on the health and function of the adipose tissue. When these adipogenic processes are disrupted, adipocyte hypertrophy occurs. This reduces the capacity of the adipose tissue to respond to insulin contributing to systemic metabolic dysfunction. There are a number of studies which demonstrate that adipocyte hypertrophy in the absence of obesity can predict later type 2 diabetes (Weyer et al., 2000; Hammarstedt et al., 2012). The converse of this is also true where severely obese individuals with smaller adipocytes remain insulin sensitive (Klöting et al., 2010).
A panel of genes relating to adipogenic processes were examined to determine the relationship between adiposity and metabolic health in response to maternal HFD and IL1R1−/−. There was a significant reduction of Ppargc1, which encodes PGC-1alpha, a master regulator of mitochondrial biogenesis, in response to both IL-1R1−/− genotype and maternal HFD in female but not male offspring. PGC-1alpha acts as a co-activator for PPARγ and is critical for adipogenesis and the regulation of fatty acid oxidation pathways (Liang and Ward, 2006). Reduction of Ppargc1 in both morbidly obese patients (Semple et al., 2004) and rodents fed a HFD (Kleiner et al., 2012) is associated with significant metabolic dysregulation. It is therefore likely that reduction in this co-activator prevents adipocyte expansion resulting in hypertrophy, thus limiting the capacity to both store and efficiently process fatty acids. In the current study, we also see a negative correlation between Ppargc1 and Igf1 in the female offspring. While IGF1 is a known regulator of growth and differentiation, there is evidence that as adipocytes undergo hypertrophy, there is a reduction in IGF1 resulting in recruitment of macrophages to the adipose tissue, and these immune cells then produce IGF1 to compensate for the reduction in adipocytes (Chang et al., 2016).
Hormone sensitive lipase (HSL) is encoded by the gene Lipe and is involved in the mobilization of fat stores through the hydrolysis of lipids to fatty acids. There is evidence that knockout of HSL results in increased adipocyte size in rodents (Osuga et al., 2000) and a loss of function mutation in humans was associated with increased risk for metabolic dysfunction (Albert et al., 2014). These studies underscore the importance of HSL in adipocyte function. The current study demonstrates a significant reduction in Lipe in female IL1R1−/− mice irrespective of genotype. There is also a decrease in Acacb, which encodes the protein ACC2, a rate limiting step in fatty acid uptake and oxidation in the mitochondria, in female IL1R1−/− groups irrespective of maternal diet. Depletion of this protein improves overall metabolic health in mice fed a HFD (Takagi et al., 2018). Furthermore, there was a decrease in expression of Acsl1, which encodes a protein which mediates the conversion of long-chain fatty acids to fatty acyl-CoA esters producing important substrates for mitochondrial fatty acid biosynthesis, in IL1R1−/− groups irrespective of genotype. Recent reports have demonstrated that Acsl1 depletion dampens insulin stimulated glucose uptake and fatty acid efflux in 3t3-L1 adipocytes (Lobo et al., 2009). Overall, these changes in lipid metabolism may inhibit the efficiency of the adipose tissue to mobilize and efficiently utilize lipids which in the short term may reduce the flux of fatty acids to peripheral tissues but may have long term effect via the enlargement of adipocytes and overall lipid metabolic health.
Female offspring also exhibited alterations in the expression of several genes involved in immune cell chemotaxis. Ccl12 encodes MCP-5, which promotes macrophage recruitment to the adipose tissue and promotes IR in response to HFD (Kim et al., 2014). The current study demonstrated an increase in Ccl12 gene expression in response to maternal HFD, and IL1R1−/− prevented this increase. There is some evidence that Ccl12 is increased in response to prenatal exposure to HFD. However, these studies focus on behavioral changes in the offspring and there appears to be a paucity of information on Ccl12 in relation to metabolic health in the offspring. We also see an increase in the expression chemokine receptor Ccr4 in response to maternal HFD in C57BL/6 but not IL1R1−/− mice. This receptor binds MCP-1, a key protein in the recruitment of macrophages to the adipose tissue, and subsequent IR in response to diet-induced obesity (Kanda et al., 2006). Our results are perhaps not surprising given that MCP-1 is involved in the regulation of the IL-1 pathway (Gavrilin et al., 2000). Furthermore, a role for this protein has been shown in response to maternal HFD, where offspring from mothers fed a HFD had increased MCP-1 which was associated with improved glucose tolerance (Murabayashi et al., 2013). There is also evidence of an IL1R1−/− mediated reduction in the gene expression of Tnf, a potent cytokine known to have a negative influence on metabolic health (Hotamisligil, 1999). It is possible that the moderate benefits in glucose tolerance observed in female offspring, despite adipocyte hypertrophy, may be mediated by alterations in immune signaling.
While the impact of maternal diet and IL1R1−/− on lipid metabolism and chemokine expression is limited in males, there is evidence of alterations in several genes which influence metabolic inflammatory processes. mTOR is a critical regulator of cellular metabolism and plays an important role in adipogenesis, lipid metabolism, and inflammatory processes (Mao and Zhang, 2018). Hyper-activation of mTOR signaling has the capacity to increase fat mass and reduce glucose tolerance in mice (Robitaille et al., 2013). It is therefore possible that the increase in adipocyte size in male offspring from HFD-fed mothers may be linked to increased mTOR expression. We also observed a significant increase in Tnfrsfb, a receptor for TNF, in the adipose tissue of male offspring exposed to prenatal HFD, independent of genotype. Given the important role of TNF in metabolic dysfunction and adipose tissue biology, it is possible that increases in this receptor may compensate for the lack of IL-1 signaling and thereby influence the glucose intolerance observed in male offspring.
There are several limitations to this current study. Firstly, the knockout model used represents a whole body knockout of the IL1R1 receptor. This may be problematic when interpreting results in specific tissues as the overall phenotype observed may be due to a complex interplay of different organ systems. It would therefore be useful to develop an adipose tissue specific IL1R1−/− to examine the specific contribution of adipose tissue inflammation in the developmental programming paradigm. Another limitation is the lack of littermate controls. While animals came from the same source and IL1R1−/− were maintained on a C57Bl/6 background, there is the possibility that artifactual variation might be introduced between the C57Bl/6 control group and the IL1R1−/− group. This may result from factors such as differences in microbiome composition, and genetic drift etc. and may ultimately skew the results of the study. It is possible that some of the detrimental effects observed in the IL1R1−/− group are due to compensation in other inflammatory mediators such as TNF. We had a limited capacity to conduct comprehensive analysis of circulating plasma proteins. This limited our capacity to uncover any changes in inflammatory profile of these animals. We did examine the inflammatory profile in adipose tissue via PCR analysis, a high correlation between RNA and protein concentrations in key inflammatory proteins.
Conclusion
Previous work from our group demonstrated that IL-1R1−/− resulted in metabolic dysregulation during pregnancy and demonstrated features associated with gestational diabetes including significant adipocyte hypertrophy and evidence of adipose tissue IR (Plows et al., 2019). We sought to expand this work by examining whether IL-1 signaling impacts long-term outcomes in offspring exposed to a HFD in utero. Our results show that while there was little impact on glucose tolerance between groups, both IL1R1 knockout and maternal HFD resulted in adipocyte hypertrophy and altered gene expression in pathways relating to adipogenesis, lipid metabolism, and inflammation in a sex-specific manner. This furthers the understanding of diet-induced metabolic inflammation in relation to in utero exposures and lends support to the importance of studying both male and female subjects. There are clear beneficial effects of depletion of the IL-1 signaling pathway in females, particularly in relation to inflammation which are not seen in males. This may in part explain why males are more susceptible to metabolic dysregulation following in utero exposure to HFD. This work highlights the complexity of IL-1 signaling in terms of metabolic health and the importance of cardiometabolic outcomes in response to in utero exposures.
Data Availability Statement
The raw data supporting the conclusions of this article will be made available by the authors, without undue reservation, to any qualified researcher.
Ethics Statement
The animal study was reviewed and approved by AgResearch Animal Ethics Committee in accordance with the New Zealand Animal Welfare Act, 1999.
Author Contributions
PBC collected data, performed analysis, and contributed to the writing of the manuscript. JP and FR performed analysis and edited the manuscript. RP and TG collected data. JS and MV reviewed the manuscript. CR designed the study, collected data, and wrote the manuscript. All authors have read and approved the manuscript.
Funding
This work was funded by the Health Research Council of New Zealand (Emerging Researcher grant). CR is supported by an HRC Sir Charles Hercus Health Research Fellowship.
Conflict of Interest
The authors declare that the research was conducted in the absence of any commercial or financial relationships that could be construed as a potential conflict of interest.
Acknowledgments
The authors would like to thank Robert Smith and Ric Broadhurst from AgResearch for their expert animal assistance. They would also like to thank Satya Amirapu, who prepared the histological samples for visualizing.
References
Albert, J. S., Yerges-Armstrong, L. M., Horenstein, R. B., Pollin, T. I., Sreenivasan, U. T., Chai, S., et al. (2014). Null mutation in hormone-sensitive lipase gene and risk of type 2 diabetes. N. Engl. J. Med. 370, 2307–2315. doi: 10.1056/NEJMoa1315496
Allport, V. C., Pieber, D., Slater, D. M., Newton, R., White, J. O., and Bennett, P. R. (2001). Human labour is associated with nuclear factor-κB activity which mediates cyclo-oxygenase-2 expression and is involved with the ‘functional progesterone withdrawal’. Mol. Hum. Reprod. 7, 581–586. doi: 10.1093/molehr/7.6.581
Blüher, M. (2019). Obesity: global epidemiology and pathogenesis. Nat. Rev. Endocrinol. 15, 288–298. doi: 10.1038/s41574-019-0176-8
Chang, E., Hafner, H., Varghese, M., Griffin, C., Clemente, J., Islam, M., et al. (2019). Programming effects of maternal and gestational obesity on offspring metabolism and metabolic inflammation. Sci. Rep. 9, 1–15. doi: 10.1038/s41598-019-52583-x
Chang, H. R., Kim, H. J., Xu, X., and Ferrante, A. W. (2016). Macrophage and adipocyte IGF1 maintain adipose tissue homeostasis during metabolic stresses. Obesity 24, 172–183. doi: 10.1002/oby.21354
Corbould, A. (2008). Effects of androgens on insulin action in women: is androgen excess a component of female metabolic syndrome? Diabetes Metab. Res. Rev. 24, 520–532. doi: 10.1002/dmrr.872
Finucane, O. M., Lyons, C. L., Murphy, A. M., Reynolds, C. M., Klinger, R., Healy, N. P., et al. (2015). Monounsaturated fatty acid–enriched high-fat diets impede adipose NLRP3 inflammasome–mediated IL-1β secretion and insulin resistance despite obesity. Diabetes 64, 2116–2128. doi: 10.2337/db14-1098
Franks, S. (2012). Animal models and the developmental origins of polycystic ovary syndrome: increasing evidence for the role of and rogens in programming reproductive and metabolic dysfunction. Endocrinology 153, 2536–2538. doi: 10.1210/en.2012-1366
García, M. C., Wernstedt, I., Berndtsson, A., Enge, M., Bell, M., Hultgren, O., et al. (2006). Mature-onset obesity in interleukin-1 receptor I knockout mice. Diabetes 55, 1205–1213. doi: 10.2337/db05-1304
Gavrilin, M. A., Deucher, M. F., Boeckman, F., and Kolattukudy, P. E. (2000). Monocyte chemotactic protein 1 upregulates IL-1beta expression in human monocytes. Biochem. Biophys. Res. Commun. 277, 37–42. doi: 10.1006/bbrc.2000.3619
Hammarstedt, A., Graham, T. E., and Kahn, B. B. (2012). Adipose tissue dysregulation and reduced insulin sensitivity in non-obese individuals with enlarged abdominal adipose cells. Diabetol. Metab. Syndr. 4:42. doi: 10.1186/1758-5996-4-42
Hotamisligil, G. S. (1999). The role of TNFalpha and TNF receptors in obesity and insulin resistance. J. Intern. Med. 245, 621–625. doi: 10.1046/j.1365-2796.1999.00490.x
Jager, J., Grémeaux, T., Cormont, M., Le Marchand-Brustel, Y., and Tanti, J. -F. (2007). Interleukin-1β-induced insulin resistance in adipocytes through down-regulation of insulin receptor substrate-1 expression. Endocrinology 148, 241–251. doi: 10.1210/en.2006-0692
Kanda, H., Tateya, S., Tamori, Y., Kotani, K., Hiasa, K., Kitazawa, R., et al. (2006). MCP-1 contributes to macrophage infiltration into adipose tissue, insulin resistance, and hepatic steatosis in obesity. J. Clin. Invest. 116, 1494–1505. doi: 10.1172/JCI26498
Katra, P., Dereke, J., Nilsson, C., and Hillman, M. (2016). Plasma levels of the interleukin-1-receptor antagonist are lower in women with gestational diabetes mellitus and are particularly associated with postpartum development of type 2 diabetes. PLoS One 11:e0155701. doi: 10.1371/journal.pone.0155701
Kim, D., Kim, J., Yoon, J. H., Ghim, J., Yea, K., Song, P., et al. (2014). CXCL12 secreted from adipose tissue recruits macrophages and induces insulin resistance in mice. Diabetologia 57, 1456–1465. doi: 10.1007/s00125-014-3237-5
Kleiner, S., Mepani, R. J., Laznik, D., Ye, L., Jurczak, M. J., Jornayvaz, F. R., et al. (2012). Development of insulin resistance in mice lacking PGC-1α in adipose tissues. Proc. Natl. Acad. Sci. U. S. A. 109, 9635–9640. doi: 10.1073/pnas.1207287109
Klöting, N., Fasshauer, M., Dietrich, A., Kovacs, P., Schön, M. R., Kern, M., et al. (2010). Insulin-sensitive obesity. Am. J. Physiol. Endocrinol. Metab. 299, E506–E515. doi: 10.1152/ajpendo.00586.2009
Koenen, T. B., Stienstra, R., van Tits, L. J., de Graaf, J., Stalenhoef, A. F. H., Joosten, L. A. B., et al. (2011). Hyperglycemia activates caspase-1 and TXNIP-mediated IL-1β transcription in human adipose tissue. Diabetes 60, 517–524. doi: 10.2337/db10-0266
Li, J., Liu, M., Zong, J., Tan, P., Wang, J., Wang, X., et al. (2014). Genetic variations in IL1A and IL1RN are associated with the risk of preeclampsia in Chinese Han population. Sci. Rep. 4:5250. doi: 10.1038/srep05250
Liang, H., and Ward, W. F. (2006). PGC-1α: a key regulator of energy metabolism. Adv. Physiol. Educ. 30, 145–151. doi: 10.1152/advan.00052.2006
Livak, K. J., and Schmittgen, T. D. (2001). Analysis of relative gene expression data using real-time quantitative PCR and the 2(−Delta Delta C(T)) method. Methods 25, 402–408. doi: 10.1006/meth.2001.1262
Lobo, S., Wiczer, B. M., and Bernlohr, D. A. (2009). Functional analysis of long-chain acyl-CoA synthetase 1 in 3T3-L1 adipocytes. J. Biol. Chem. 284, 18347–18356. doi: 10.1074/jbc.M109.017244
Mandal, M., Donnelly, R., Elkabes, S., Zhang, P., Davini, D., David, B. T., et al. (2013). Maternal immune stimulation during pregnancy shapes the immunological phenotype of offspring. Brain Behav. Immun. 33, 33–45. doi: 10.1016/j.bbi.2013.04.012
Mao, Z., and Zhang, W. (2018). Role of mTOR in glucose and lipid metabolism. Int. J. Mol. Sci. 19:E2043. doi: 10.3390/ijms19072043
McGillicuddy, F. C., Harford, K. A., Reynolds, C. M., Oliver, E., Claessens, M., Mills, K. H. G., et al. (2011). Lack of interleukin-1 receptor I (IL-1RI) protects mice from high-fat diet–induced adipose tissue inflammation coincident with improved glucose homeostasis. Diabetes 60, 1688–1698. doi: 10.2337/db10-1278
McGillicuddy, F. C., Reynolds, C. M., Finucane, O., Coleman, E., Harford, K. A., Grant, C., et al. (2013). Long-term exposure to a high-fat diet results in the development of glucose intolerance and insulin resistance in interleukin-1 receptor I-deficient mice. Am. J. Physiol. Endocrinol. Metab. 305, E834–E844. doi: 10.1152/ajpendo.00297.2013
McGowan, P. O., and Matthews, S. G. (2018). Prenatal stress, glucocorticoids, and developmental programming of the stress response. Endocrinology 159, 69–82. doi: 10.1210/en.2017-00896
Murabayashi, N., Sugiyama, T., Zhang, L., Kamimoto, Y., Umekawa, T., Ma, N., et al. (2013). Maternal high-fat diets cause insulin resistance through inflammatory changes in fetal adipose tissue. Eur. J. Obstet. Gynecol. Reprod. Biol. 169, 39–44. doi: 10.1016/j.ejogrb.2013.02.003
Nair, R. R., Khanna, A., and Singh, K. (2014). Association of interleukin 1 receptor antagonist (IL1RN) gene polymorphism with recurrent pregnancy loss risk in the North Indian population and a meta-analysis. Mol. Biol. Rep. 41, 5719–5727. doi: 10.1007/s11033-014-3443-8
Osuga, J., Ishibashi, S., Oka, T., Yagyu, H., Tozawa, R., Fujimoto, A., et al. (2000). Targeted disruption of hormone-sensitive lipase results in male sterility and adipocyte hypertrophy, but not in obesity. Proc. Natl. Acad. Sci. U. S. A. 97, 787–792. doi: 10.1073/pnas.97.2.787
Plows, J. F., Vickers, M. H., Ganapathy, T. P., Bridge-Comer, P. E., Stanley, J. L., and Reynolds, C. M. (2019). Interleukin-1 receptor-1 deficiency impairs metabolic function in pregnant and non-pregnant female mice. Mol. Nutr. Food Res. e1900770. doi: 10.1002/mnfr.201900770 [Epub ahead of print]
Reynolds, C. M., Segovia, S. A., and Vickers, M. H. (2017). Experimental models of maternal obesity and neuroendocrine programming of metabolic disorders in offspring. Front. Endocrinol. 8:245. doi: 10.3389/fendo.2017.00245
Robitaille, A. M., Christen, S., Shimobayashi, M., Cornu, M., Fava, L. L., Moes, S., et al. (2013). Quantitative phosphoproteomics reveal mTORC1 activates de novo pyrimidine synthesis. Science 339, 1320–1323. doi: 10.1126/science.1228771
Saltiel, A. R., and Olefsky, J. M. (2017). Inflammatory mechanisms linking obesity and metabolic disease. J. Clin. Invest. 127, 1–4. doi: 10.1172/JCI92035
Segovia, S. A., Vickers, M. H., and Reynolds, C. M. (2017). The impact of maternal obesity on inflammatory processes and consequences for later offspring health outcomes. J. Dev. Orig. Health Dis. 8, 529–540. doi: 10.1017/S2040174417000204
Semple, R. K., Crowley, V. C., Sewter, C. P., Laudes, M., Christodoulides, C., Considine, R. V., et al. (2004). Expression of the thermogenic nuclear hormone receptor coactivator PGC-1alpha is reduced in the adipose tissue of morbidly obese subjects. Int. J. Obes. Relat. Metab. Disord. 28, 176–179. doi: 10.1038/sj.ijo.0802482
Siljee, J. E., Wortelboer, E. J., Koster, M. P. H., Imholz, S., Rodenburg, W., Visser, G. H. A., et al. (2013). Identification of interleukin-1 beta, but no other inflammatory proteins, as an early onset pre-eclampsia biomarker in first trimester serum by bead-based multiplexed immunoassays. Prenat. Diagn. 33, 1183–1188. doi: 10.1002/pd.4219
Takagi, H., Ikehara, T., Kashiwagi, Y., Hashimoto, K., Nanchi, I., Shimazaki, A., et al. (2018). ACC2 deletion enhances IMCL reduction along with acetyl-CoA metabolism and improves insulin sensitivity in male mice. Endocrinology 159, 3007–3019. doi: 10.1210/en.2018-00338
Terzidou, V., Lee, Y., Lindström, T., Johnson, M., Thornton, S., and Bennett, P. R. (2006). Regulation of the human oxytocin receptor by nuclear factor-κB and CCAAT/enhancer-binding protein-β. J. Clin. Endocrinol. Metab. 91, 2317–2326. doi: 10.1210/jc.2005-2649
Keywords: developmental programming, adipose tissue, inflammation, IL-1R1, high fat diet, maternal diet
Citation: Bridge-Comer PE, Plows JF, Ramzan F, Patel R, Ganapathy TP, Stanley JL, Vickers MH and Reynolds CM (2020) Interleukin 1 Receptor 1 Knockout and Maternal High Fat Diet Exposure Induces Sex-Specific Effects on Adipose Tissue Adipogenic and Inflammatory Gene Expression in Adult Mouse Offspring. Front. Physiol. 11:601. doi: 10.3389/fphys.2020.00601
Edited by:
Gina Galli, The University of Manchester, United KingdomReviewed by:
Jonatan Dereke, Lund University, SwedenEmily Camm, University of Cambridge, United Kingdom
Copyright © 2020 Bridge-Comer, Plows, Ramzan, Patel, Ganapathy, Stanley, Vickers and Reynolds. This is an open-access article distributed under the terms of the Creative Commons Attribution License (CC BY). The use, distribution or reproduction in other forums is permitted, provided the original author(s) and the copyright owner(s) are credited and that the original publication in this journal is cited, in accordance with accepted academic practice. No use, distribution or reproduction is permitted which does not comply with these terms.
*Correspondence: Clare M. Reynolds, Yy5yZXlub2xkc0BhdWNrbGFuZC5hYy5ueg==