- 1Department of Fisheries Biology and Genetics, Patuakhali Science and Technology University, Patuakhali, Bangladesh
- 2School of Molecular and Life Sciences, Curtin University, Bentley, WA, Australia
- 3Department of Marine Fisheries and Oceanography, Patuakhali Science and Technology University, Patuakhali, Bangladesh
A feeding trial was carried out to evaluate the effects of substitution of fishmeal (FM) by dietary poultry by-product meal, fermented by Lactobacillus casei and Saccharomyces cerevisiae on growth, intestinal health, microbial composition, immune related cytokines and disease resistance of freshwater crayfish, marron (Cherax cainii) against Vibrio mimicus. Two isonitrogenous and isocaloric diets were formulated by replacing FM protein with fermented poultry by-product meal (FPBM) protein at 0% (Control) and 75% (FPBM), and fed marron for 70 days. The results indicated no significant difference (P > 0.05) in final body weights between two groups of marron, whilst intestinal microvilli number per fold was increased in marron fed FPBM than the control. The 16S rRNA sequences revealed an increased number of Lactobacillus and Streptococcus, and decreased number of Aeromonas at genus level in the distal intestine of marron fed FPBM. Marron fed FPBM showed up-regulated expression of IL-8, IL-10, and IL-17F genes in the distal intestine. Significantly (P < 0.05) increased lysozyme and phagocytic activity, and higher survival was found in marron fed FPBM following a bacterial challenge with Vibrio mimicus. Therefore, it is concluded that FPBM is beneficial to marron in terms of microbial community, immune-related cytokines and disease resistance against V. mimicus.
Introduction
Aquaculture, one of the fastest growing food-producing sectors in the world, produced 80 million tonnes of edible fish valued at USD 231.6 billion in 2016, with crustacean aquaculture contributing 7.9 million tonnes with a value estimated at USD 57.1 billion (FAO, 2018). Mass production through intensive farming practices may possibly result in the prevalence of pathogens and subsequent disease infestation (Thanasaksiri et al., 2019). As a result, utilization of drugs and antibiotics to control pathogens and disease outbreaks is now common practice in aquaculture. Therefore, several issues such as the development of antibiotic resistant bacteria and the accumulation of antibiotic residues in fish tissue with the potential to be transferred to humans, has resulted in many countries banning the utilization of these drugs in aquaculture production (Pourmozaffar et al., 2019). Hence it has now become an research imperative to develop functional aqua-diets through advanced approaches like fermentation, supplementation of immune-stimulant, natural therapeutics, probiotics, and micronutrients, not only to improve the growth performance, but also, to enhance immune response and disease resistance against invading pathogens (Siddik et al., 2019b).
Microbial and yeast fermented aqua-diets have been used in fish farming for decades with much research evaluating potential on a variety of finfish (Kader et al., 2012; Siddik et al., 2019a, b) and crustaceans (Ding et al., 2015; Elshopakey et al., 2018). Previous studies have reported a range of possible functional properties in fermented products, including probiotic, antimicrobial and antioxidant properties, and the degradation of anti-nutritive compounds (Chettri and Tamang, 2014; Hill et al., 2014). Elshopakey et al. (2018) reported that dietary administration of yeast and Lactobacillus fermented vegetable product in the diet of kuruma shrimp, Marsupenaeus japonicas, elevated the innate immune response in terms of total haemocyte count, phagocytosis, bactericidal activity and increased resistance against Vibrio parahaemolyticus. Antimicrobial peptides derived from fermentation can also upregulate the immune and stress-related gene expression. For instance, dietary incorporation of 25% fermented soybean meal in the diet of freshwater prawn, Macrobrachium nipponense significantly upregulated the mRNA levels of the superoxide dismutase [Cu-Zn] gene (Ding et al., 2015).
The intestine of animals is a complex microbial ecosystem harboring a dynamic consortium of microorganisms, which can play pivotal roles in host health with multiple functions, including absorbing nutrients, improving energy production, and balancing the immune response (Hooper et al., 2002; Bates et al., 2006; Semova et al., 2012). Previous studies have found that ingestions of microbial fermented diets modulated the gut microbes of animals with the most common changes in stomach and small intestine due to fermentation reported to be an increase in the concentration of lactic acid bacteria and numbers of yeast cells (Canibe and Jensen, 2003; Missotten et al., 2015). These microbiological changes can act to protect the animals from enteropathogens (Missotten et al., 2015). Ashayerizadeh et al. (2017) reported that fermented rapeseed meal reduced the colonization of Salmonella enterica serovar in the gut and internal organs of broiler chicks, potentially protecting against Salmonella infections.
It has been reported that a omnivorous freshwater Australian crayfish, marron (Cherax cainii) can be successfully cultured using poultry by-product meal (PBM), feather meal, lupin meal, or meat and bone meal as the main protein source (Saputra et al., 2019). In that study, it was found that PBM as an alternative protein source to FM increased the total haemocyte count, microvillus length and susceptibility to high temperature (30°C) exposure (Saputra et al., 2019). In addition, PBM supplemented with black soldier fly (Hermetia illucens) meal revealed a higher bacterial activity and gene function correlated to biosynthesis of protein and energy in marron (Foysal et al., 2019a, b). However, little is known pertaining to the dietary effects of fermented PBM on the health status and intestinal microbiota of marron. Thus, the present study aimed to evaluate the effects of dietary inclusion of FPBM on the growth, immune response, intestinal microbiota and disease resistance of marron against Vibrio mimicus.
Materials and Methods
Ethics Statement
Laboratory experiment with crustacean does not require specific permits in Australia. The specimen collection site is not privately owned or protected in any way, and the study did not involve endangered or protected species. The experimental was conducted under university protocols to avoid animal suffering.
Experimental Feeds
All feed ingredients used in this study were purchased from Specialty Feeds Pty. Ltd., Western Australia and ground into a fine powder of less than100-mesh particle size. The raw PBM was fermented by Baker’s yeast, Saccharomyces cerevisiae and Lactobacillus casei following a technique described in our earlier study (Siddik et al., 2019a). The amino acid compositions of raw and fermented poultry by-product meal (FPBM) are presented in Table 1. Two isonitrogenous (30.0% protein) and isoenergetic (19.0 MJ Kg–1) diets were produced with FM replaced by FPBM at a level of 0% (control) and 75%. All pre-weighed, dry ingredients were mixed and homogenized in a Hobart mixer with distilled water. The dough of the mixed ingredients was then processed with a pellet extruder to produce 3 mm pellets. The resulting pellets were air dried in the oven at 60°C for approximately 30 h and then stored at 4°C until use for feeding trials. Experimental diet ingredients and compositions are presented in Table 2.
Experimental System, Design, and Marron
Marron were purchased from Blue Ridge Marron Farm, Manjimup, Western Australia (34°12′21.8″S and 116°00′59.4″E) and shipped to the Curtin Aquatic Research Laboratory (CARL). Marron were acclimated in culture conditions for 1 week facilitated with constant aeration and recirculating filtered freshwater, and fed with a basal marron diet (29.0% protein, 8.0% fats, and 8.9% ash) at a rate of 1% body weight twice daily. After acclimation, 60 marron (pool initial weight 94.40 ± 2.07 g) were randomly stocked into twelve 250 L plastic cylindrical tanks (800 mm diameter, 500 mm high) where each diet was fed in six replicated group. Each tank was stocked with five marron and each marron was kept individually in a modified 750 mL plastic cage to provide shelter and avoid cannibalism during molting. All tanks were equipped with recirculating biological filtration system and an automatic submersible heater (Sonpar, Model: HA-100, China) that was set to 24°C to maintain constant temperature. Marron were fed test diets at the rate of 1% body weight for 70 days, and uneaten feed and faces were removed through siphoning every early morning. Water physico-chemical parameters, including temperature, pH and dissolved oxygen were measured daily using a portable multiparameter meter (YSI, United States), and total ammonia and nitrite were measured daily with chemical test kits (Aquarium Pharmaceuticals, Mars Fishcare North America, Inc., Chalfont, PA, United States). The measured water quality was as follows: water temperature 24.3 ± 1.8°C, pH 7.4–8.1, dissolved oxygen >5 mg L–1, ammonia ≤0.02 mg L–1 and nitrite ≤0.2 mg L–1. At the end of the feeding trial, marron were individually weighed to calculate final body weight (FBW), and specific growth rate (SGR) was estimated by the following formula:
Intestinal Micromorphology
Six marron from each control and FPBM groups (one from each tank) were sampled to dissect and extract the mid intestine for scanning electron microscopy (SEM) analysis. The SEM samples were prepared following the method described in Saputra et al. (2019). In short, the collected samples were soaked in 3% glutaraldehyde in 0.1 M cacodylate buffer overnight. The samples were then washed in cacodylate buffer and distilled water for three consecutive changes in each for 5 min, respectively. The samples were immersed in 2% OsO4 for 2 h followed by three washes in distilled water for 5 min, and then dehydrated using a series of 50, 75, and 95% ethanol solutions for 5 min before three final washes in 100% ethanol for 5 min. The samples were dried by washing in a series of 50%, 75% and 100% (twice) hexamethyldisilizane (HMDS) in ethanol solutions for 5 min. All processed samples were dried at room temperature and mounted on a stub using carbon tape, coated with gold and viewed under a pressure scanning electron microscope (SEM, model Phillips XL 30, FEI, Hillsboro, United States). The SEM images were analyzed to assess the length and number of microvilli by measuring and counting them on each slide using digital imaging software (Adobe Photoshop CC 2015, Adobe System Incorporated, United States).
Intestinal Microbiome Profiling and Analysis
At the termination of the feeding trial, distal intestinal samples from three randomly selected marron from each dietary group were collected under sterile conditions. Approximately 200 mg of distal intestinal content of marron were transferred into 1.5 mL eppendorf tubes and processed for DNA extraction using DNeasy Blood and Tissue Kit (Qiagen, Crawley, United Kindom). Bacterial DNA was extracted following manufacturer’s protocols and quantified by a NanoDrop Spectrophotometer (Thermo-Fisher Scientific, Waltham, MA, United States). The extracted DNA samples were diluted to a final concentration of 30 ng/μL. PCR was performed to amplify V3-V4 region of the bacterial 16S rRNA. PCR reactions were carried out in a total volume of 50 μL of master mixture, containing Hot Start Taq 2X Master Mix (New England BioLabs Inc., Ipswich, MA, United States) (25 μL), template DNA (2 μL), V3-V4 sequencing primers (1 μL each), and nuclease-free water (21 μL). A total of 30 cycles of reactions were performed in a BioRad S100 Gradient Thermal Cycler (Bio-Rad Laboratories, Inc., Foster City, CA, United States). Amplified PCR products were then separated by gel electrophoresis (Bio-Rad Laboratories Inc., Hercules, CA, United States) and visualized under gel doc (FujiFilm LAS–4000 Image Analyzer, Boston Inc., Foster City, CA, United States). According to the Illumina standard protocol (Part # 15044223 Rev. B), the 16s rRNA PCR amplicon of each sample was barcoded via a secondary PCR. The V3 kit (600 cycles, Part # MS-102-3003) was used to sequence the samples up to 30,000 reads on an Illumina MiSeq platforms (Illumina Inc., San Diego, CA, United States) at the Harry Perkins Institute of Medical Research, Western Australia.
FastQC pipelines were used to check the initial quality of 16S rRNA sequences (Andrews, 2010) and QIIME (v1.6.1) pipeline was used to analyze the 16S rRNA sequence data (Kuczynski et al., 2011). The sickle program was used for quality trimming of reads and following trimming, reads with a length of <200 bp were deleted (Joshi and Fass, 2011). Merging of short reads (>100 but ≤200) was done by MefFiT pipeline (Parikh et al., 2016) and then multiple sequence alignment (MSA) was performed using PASTA algorithm (Mirarab et al., 2015). The operational taxonomic units (OTUs) were picked at 97% similarity using open reference workflow from the SILVA database (SILVA1.32 release) (Quast et al., 2013). The raw FASTQ files have been deposited in the National Centre for Biotechnology Information (NCBI) BioProject, with the accession number PRJNA521663.
Principal coordinate analysis (PCoA) was performed to visualize the clustering of samples for two different treatment groups based on related abundance (weighted). The Shannon, Simpson, and Fisher alpha diversity index were calculated in “Rstudio” using “vegan” package (Oksanen et al., 2018). Chao1 diversity index was calculated using formula Schao1 = Sobs + (n1), where Sobs = number of observed phyla/genus, n1 = number of singletons (phyla/genus captured once), n2 = number of doubletons (phyla/genus captured twice) (Militon et al., 2010). A Venn diagram for bacterial abundance regarding bacterial diversity at genus level was generated using FunRich (v3.1.3). The raw FASTQ files are currently available at National Centre for Biotechnology Information (NCBI) BioProject, with the accession number PRJNA521663.
Cytokines Gene Expression Analysis
Intestinal samples from three marron from each control and FPBM groups were collected under aseptic conditions to analyse five selected genes from the cytokine family related to immunity (IL-1β, IL-8, IL-10, IL-17F, and TNF-α). The primer information for the selected genes are provided in Table 3. For RNA extraction, intestinal tissue samples were immediately stored in RNA Later (Sigma-Aldrich, Darmstadt, Germany) according to manufacturer’s instructions until future use. The samples were then thawed, dried, homogenized and ground into a fine powder. Total RNA from approximately 5 mg of intestinal tissue sample was extracted using RNeasy Mini Kit (Qiagen, Hilden, Germany) and extracted RNA was treated with RNase free DNase-I (Qiagen, Hilden, Germany) to remove residual DNA related contamination. Isolated RNA quality was determined in a gel electrophoresis and quantity was confirmed in a NanoDrop spectrophotometer 2000c (Thermo Fisher Scientific, United States). The cDNA library was generated from 1 μg of RNA and synthesized by Omnicript RT kit (Qiagen, Hilden, Germany) following the manufacturer’s protocols. The qRT-PCR was performed using PowerUpTM Cyber Green Master Mix (Thermo Scientific, Waltham, MA, United States) with 7500 Real-Time PCR System (Applied Biosystems, Foster City, CA, United States) to measure the relative expression level of ten selected genes of the intestine tissue. The relative expression level of each gene was measured using the 2–ΔΔCT method, after normalization against the β-actin reference gene (Foysal et al., 2019b).
Immunological Indices
Total haemocyte count (THC) and differential haemocyte count (DHC) were estimated following the established protocol for marron (Nugroho and Fotedar, 2013). The phagocytic rate (PR) of marron was assessed according to the established protocol as described by Chen et al. (2005) and Hooper et al. (2014). Briefly, 40 μL of haemolymph was smeared on a Poly-L-Lysine glass slide (Thermofisher Scientific, United States) and incubated to make the haemolymph dry. 0.0125 g of Zymosan powder was diluted in 25 mL of sterile seawater to prepare Zymosan (Sigma-Aldrich, Z4250) working solution. Then, dried samples on the glass slide were treated with 40 μL Zymosan working solution and air dried. To incubate the cells for staining, the glass slide were placed in Giemsa solution for 20 min.
Lysozyme (LYZ) activity was determined by the turbidimetric method described in Siddik et al. (2018). Briefly, 5 mg of Micrococus lyso was mixed with 20 mL of PBS to prepare a bacterial suspension and stirred for 5 min. 0.3 mL of haemolymph from each marron was withdrawn using a 23-gauge needle containing a similar amount of antiquagulent, dispensed into an Eppendorf tube and mixed. 100 μL of haemolymph was pipetted and placed into a 96-well plate in duplicate. After 15 min, 100 μL of bacterial suspension was added to the wells and the mixture was incubated at 25°C. The sample was then placed in Spectrophotometer at 450 nm and absorbance was monitored every 2 min for a total of 20 min. The results were expressed as Unit mL–1.
Challenge With Vibrio mimicus
At the end of the feeding trial, 15 average sized marron from each dietary group of control and FPBM were subjected to a bacterial challenge. For this, each dietary group had three replicates with a density of five marron per tank. The trial was conducted for 7 days with Vibrio mimicus. The challenge bacteria was obtained from the Department of Agriculture, Western Australia, and was grown in trypticase soy broth culture medium at 24°C for 24 h, after which the broth containing the contents were centrifuged for 15 min at 5000 × g and the precipitate was resuspended in phosphate-buffered saline (pH 7.2). The experimental infection was performed through the base of the fifth thoracic leg of marron by injecting with 30 μL of V. mimicus suspended in phosphate-buffered saline at a concentration of 3.2 × 106 CFU/ml as LD50 (Nugroho and Fotedar, 2013). During the challenged period marron were fed once daily with the same experimental diet that was assigned before the challenge. The immunological parameters in terms of THC, DHC, PR, and LYZ in marron were analyzed before the V. mimicus injection and at 24 h after the injection. The survival of marron were counted at the end of the challenge trial.
Statistical Analysis
Normality for all data were checked by Kolomogorow-Smirnov test. Two-tailed student t-test was applied to determine the significant difference between treatments at 5% level of significance. Kaplan–Meier, a non-parametric statistic with log rank test was used to test the probability of surviving in a given time period. The correlation analysis between the microbial community and marron immune indices was conducted using “microbiomeseq,” “phyloseq,” and “ggplot2” packages in “Rstudio.” The cycle threshold (CT) values of qRT-PCR for respective genes were analyzed using the 2–△△CT method by considering reference gene (β-actin) as a control.
Results
Growth Performance
At the end of the 70 days growth trial, the mean final body weight (FBW) of marron fed FPBM (113.10 g ± 3.54) was not significantly higher than the FBW of marron fed the control diet (105.3 g ± 3.39) (P > 0.05). Similarly, SGR was also not significantly affected by the test diets (Figure 1).
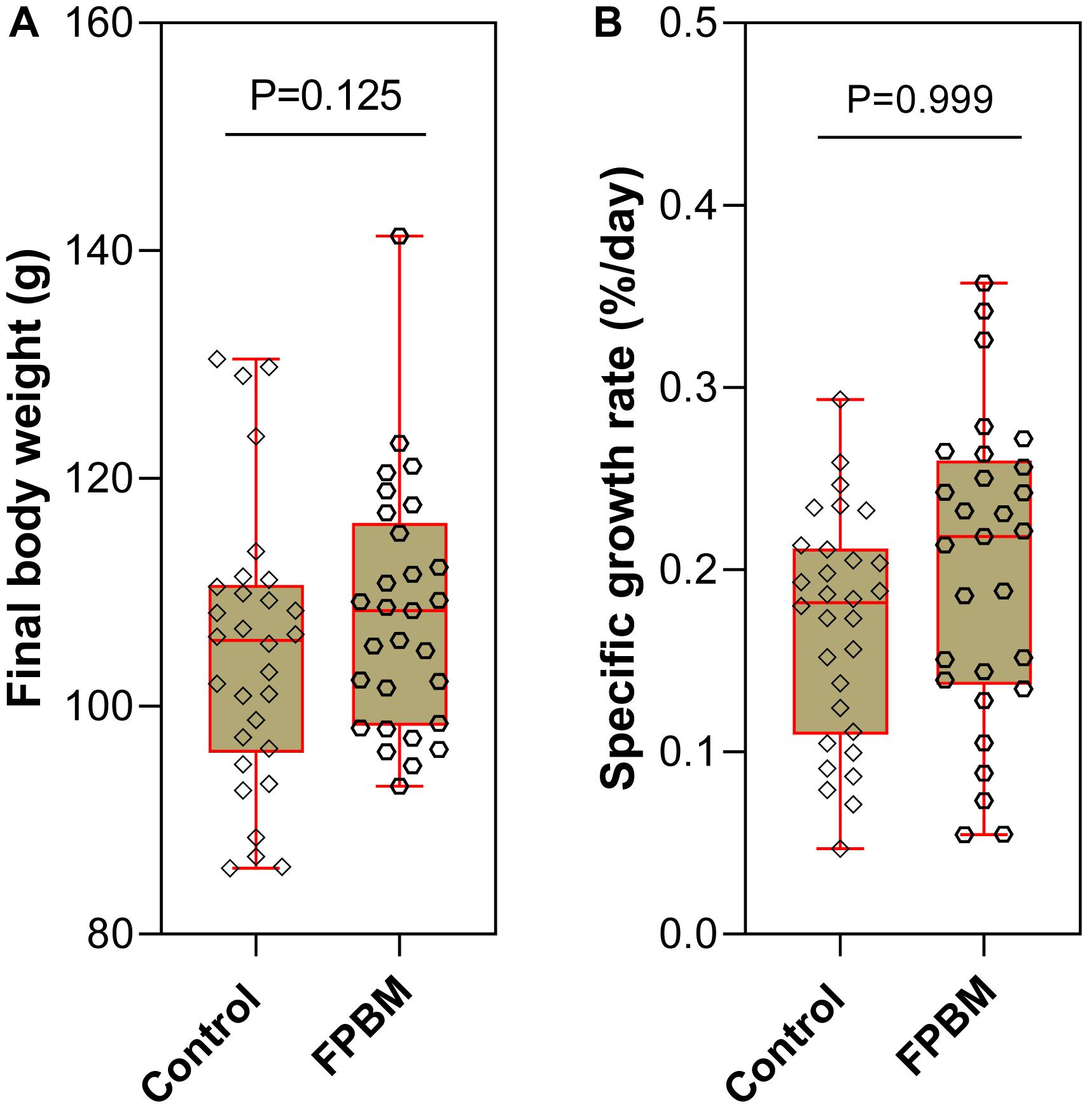
Figure 1. Growth performance (A) final body weight and (B) specific growth rate of marron fed control and fermented poultry by-products meal (FPBM) for 70 days. Statistical significance: unpaired two-tailed t test, P < 0.05. The line within each box represents the median. Boxplots show values obtained, whiskers indicate minimum and maximum of the dataset.
Intestinal Micromorphology
The visual images of distal intestinal surface of marron fed control and FPBM diets are shown in Figures 2A,B, respectively. The length of microvilli for marron fed FPBM was not significantly (P > 0.05) different than the control with the size range from 2.75 to 3.78 μm (Figure 2C). However, the microvilli number per group/fold was significantly (P < 0.05) higher in the FPBM than the control group with the number ranging from 4 to 9 per group in marron (Figure 2D).
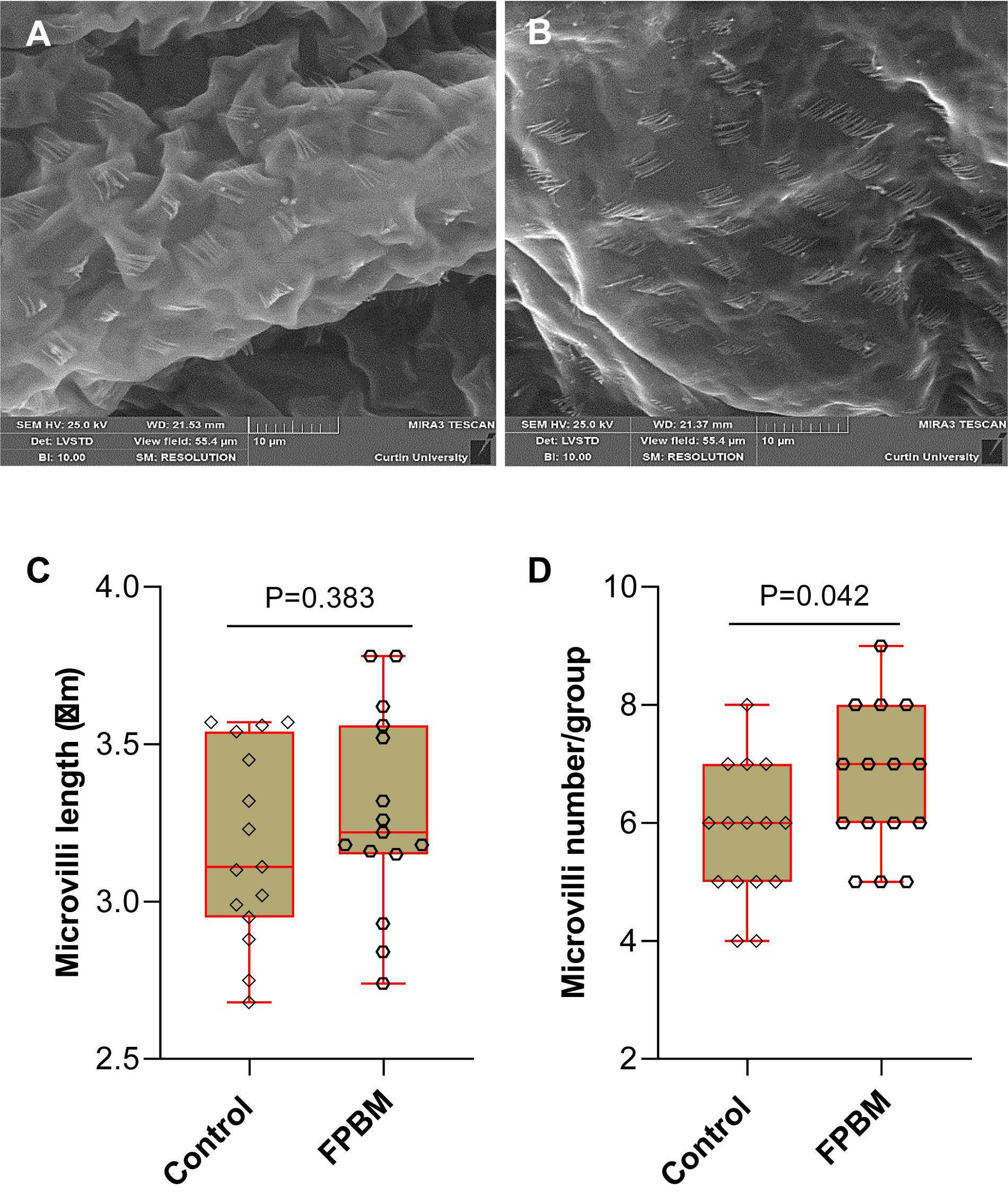
Figure 2. Scanning electron microscopy (SEM) of marron fed control (A) and FPBM diet (B). Microvilli length (C) and microvilli number per group (D) were compared in the distal intestine of marron fed control and fermented poultry by-products meal (FPBM) diets. Statistical significance: unpaired two-tailed t test, P < 0.05. The line within each box represents the median. Boxplots show values obtained, whiskers indicate minimum and maximum of the dataset. 5000 × magnification; scale bars: 10 μm.
Intestinal Microbiota
To evaluate the effects of dietary inclusion of FPBM on marron intestinal microbiota, samples from control and FPBM treated groups were analyzed using 16S rRNA Illumina MiSeq (V3 and V4 regions). Altogether 225,266 high quality sequence reads was obtained after filtering quality and trimming the length. A total of 66,963 OTUs harbored in the intestine of marron (13904 in control and 53059 in FPBM), which mapped to 14 phyla and 119 genus at 97% similarity cut-off value. The microbial profile at the phylum and genus level with relative abundance of more than 1% are presented in Figures 3A,B. Irrespective of test diets, the dominant phyla in all tested samples were Proteobacteria Firmicutes, Tenericutes, Fusobacteria, Actinobacteria, and Bacteroidetes. However, FPBM significantly influenced the Firmicutes (28.84%), when compared to the control (0.12%) (P < 0.05). At the genus level, Aeromonas abundance significantly decreased in the FPBM treated group (4.65%) when compared to the group which received the control diet (37.77%) whereas Lactobacillus and Streptococcus were only found in FPBM group (0.15 and 18.58%) against none of these two species in the control group. PCoA based on weighted Unifrac distance revealed a clear clustering of samples according to diet, indicating the influence of diets on the intestinal microbial communities between the two groups (Figure 3C). The Venn diagram depicts that a total of 59 OTUs was shared by two groups, while the number of unique OTUs for control and FPBM groups was 56 and 135, respectively (Figure 3D). However, all bacterial diversity indices including Shannon, Simpson and Fishers alpha were not significantly influenced by diets with the exception of Chao 1, which was significantly augmented in FPBM treated groups (Figures 3E–H).
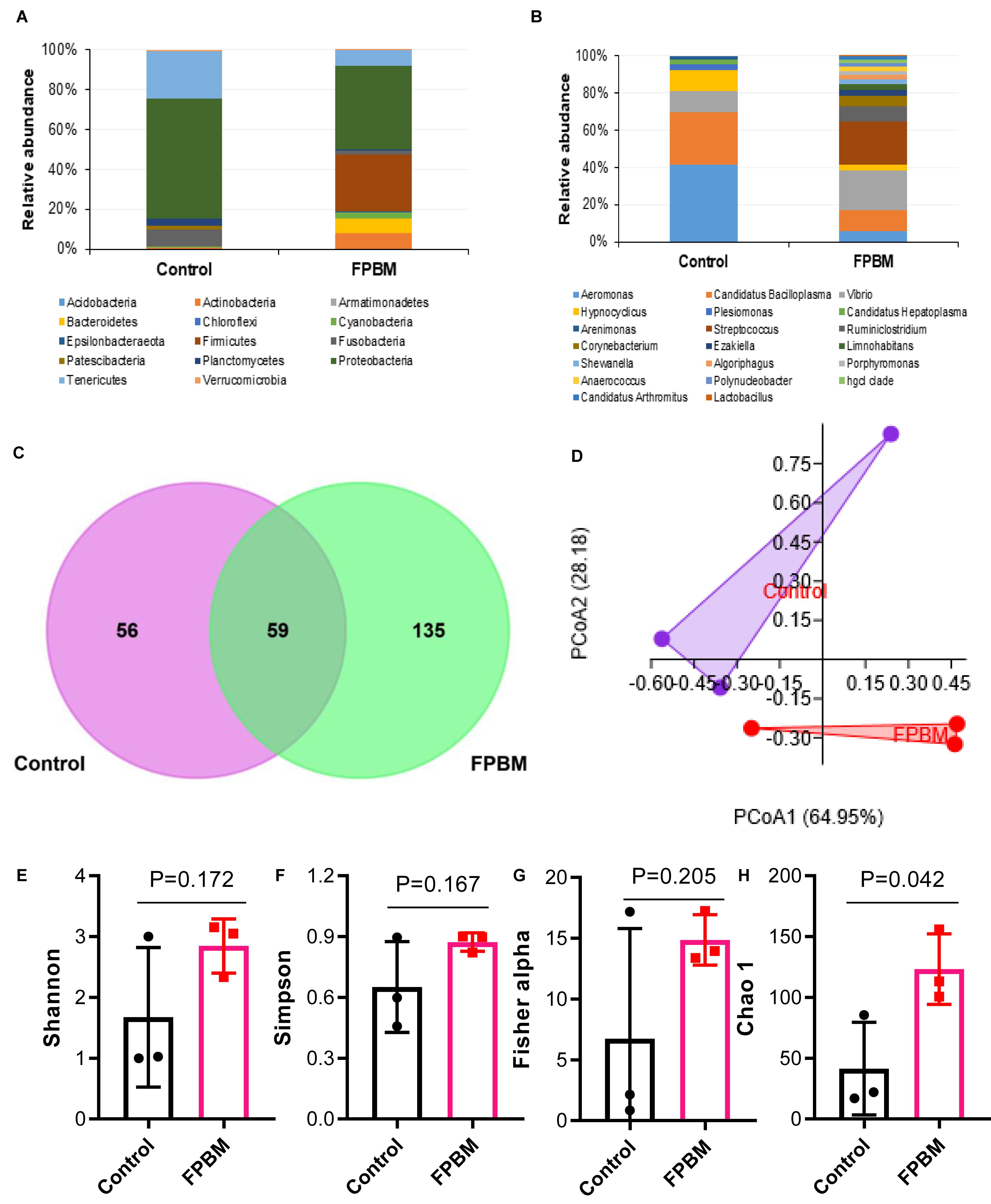
Figure 3. Relative abundance (%) of bacteria at (A) phylum, and (B) genus level in the distal intestine of marron fed control and FPBM diets where only bacteria with overall abundance of ≥1% were reported in the figure. Venn diagram (C) representing the unique and shared OTU’s between control and FPBM treated groups. Principle coordinate analysis (PCoA) (D) of intestinal microbial communities according to diets. Diversity indices of (E) Shannon, (F) Simpson, (G) Fisher alpha, and (H) Chao 1 between control and FPBM. P-value indicates significant deference in different diversity indices between the treatments as determined by two-tailed student t test. Data are presented as mean ± SEM, significant at P < 0.05.
Expression of Cytokines Gene
The effects of FPBM on pro-inflammatory and inflammatory cytokines mRNA expression level are presented in Figure 4. Marron fed FPBM had significantly higher IL-1β, IL-8, IL-10, and IL-17F expression when compared to the control, while TNF-α expression was not significantly impacted by the test diets.
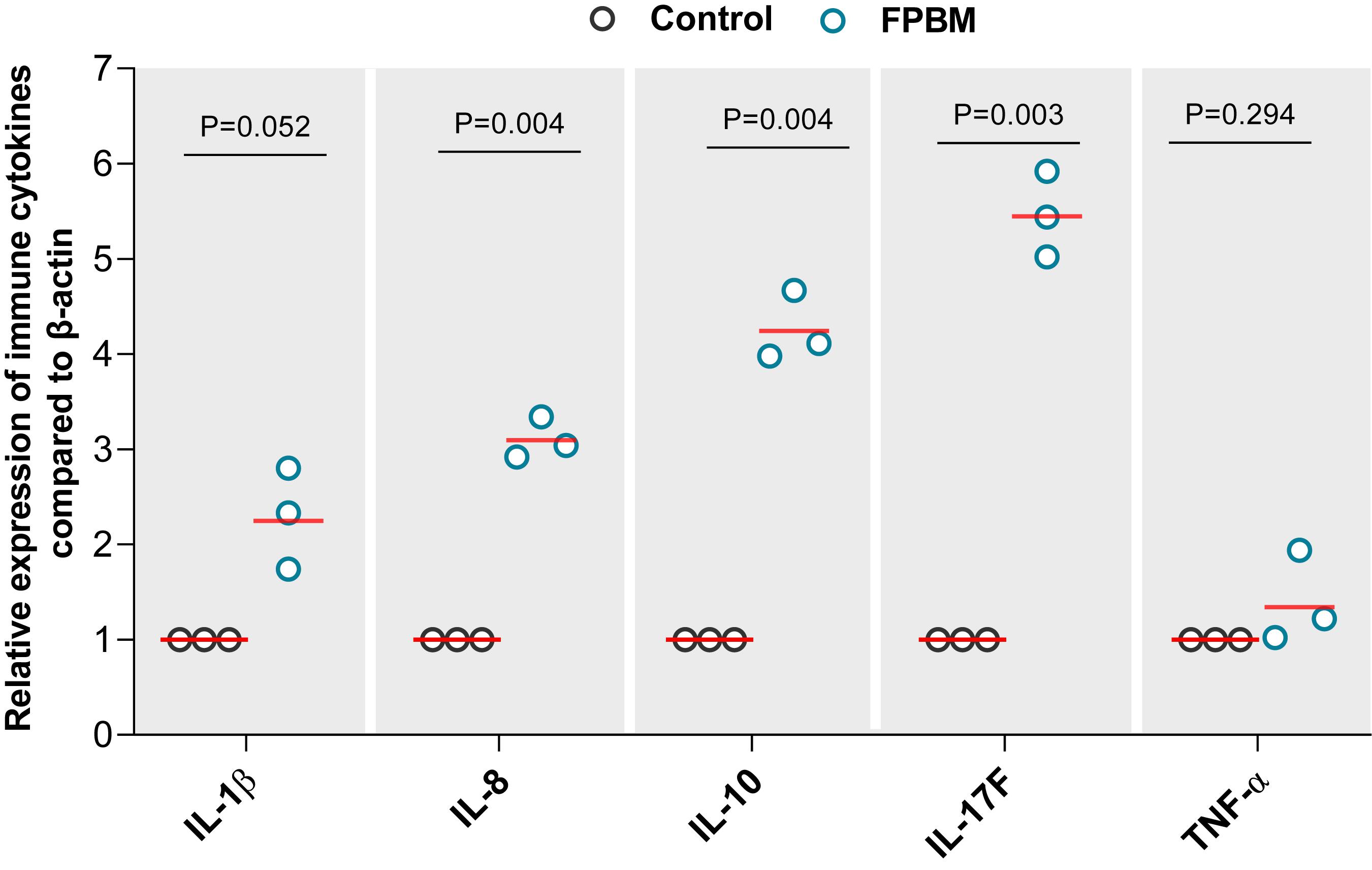
Figure 4. The effects of 70 days feeding with FPBM on the interleukin 1 beta (IL-1β), interleukin 8 (IL-8), interleukin 10 (IL-10), interleukin 17F (IL-17F) and tumor necrosis factor alpha (TNF-α) in the intestine of marron. P-value indicates significant deference between the treatments as determined by two-tailed student t test. P-value represents difference between experimental treatments. Significant difference at P < 0.05.
Immune Response
Immunological parameters of marron after 70 days of feeding trial and challenged with V. mimicus are shown in Figure 5. All parameters including THC (A), DHC (B) and LYZ (D), except PR (C) were significantly influenced in marron fed the FPBM diet when compared to the control. After 7 days of challenge, THC (Figure 5E) and DHC (Figure 5F) were significantly increased in the post challenge marron fed both control and FPBM diets when compared to the pre-challenge marron. In contrast, no significant difference was observed in PR (Figure 5G) between pre- and post-challenge in the control, while PR increased significantly in post challenge marron fed the FPBM. In agreement with the PR result, a similar trend was observed in LYZ (Figure 5H).
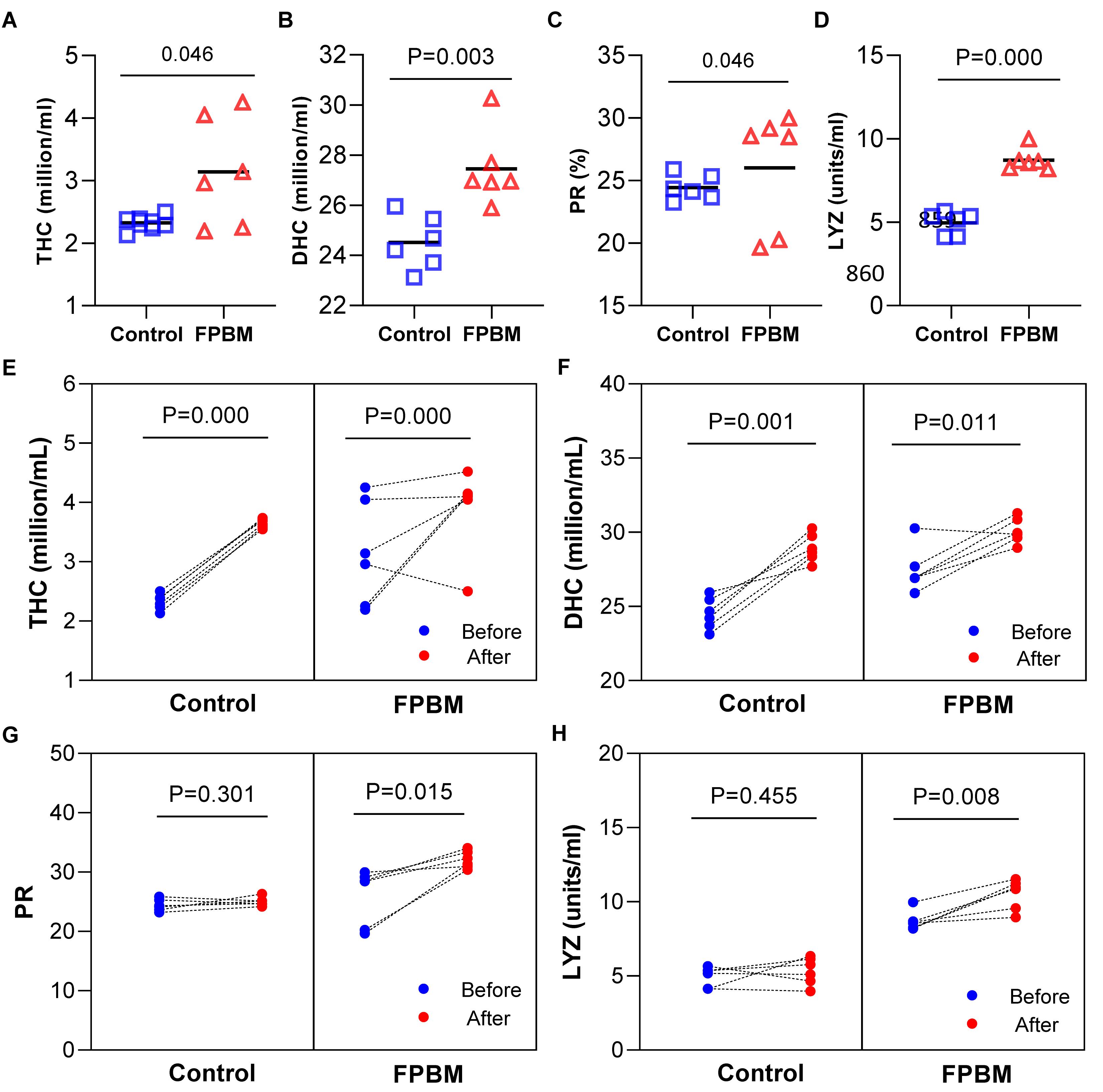
Figure 5. Immunological indices of marron fed control and FPBM diets. (A) Total haemocyte count (THC), (B) differential haemocyte count (DHC), (C) phagocytic rate (PR), and (D) lysozyme activity (LYZ) after growth trial for 70 days. Responses of marron before and after being challenged with Vibrio mimicus for 7 days (E) THC, (F) DHC, (G) PR, and (H) LYZ. P-value represent differences between experimental treatments. Significant difference at P < 0.05.
Correlation Between Immune Indices and Bacterial Community
The Pearson’s correlation plot between microbial community abundance and immune indices of marron is presented in Figure 6, revealing that presence of Porphyromonas and Aeromonas was negatively correlated with DHC and THC, respectively, in the FPBM treated group. The LYZ and PR were not correlated with bacterial communities in the control or FPBM groups.
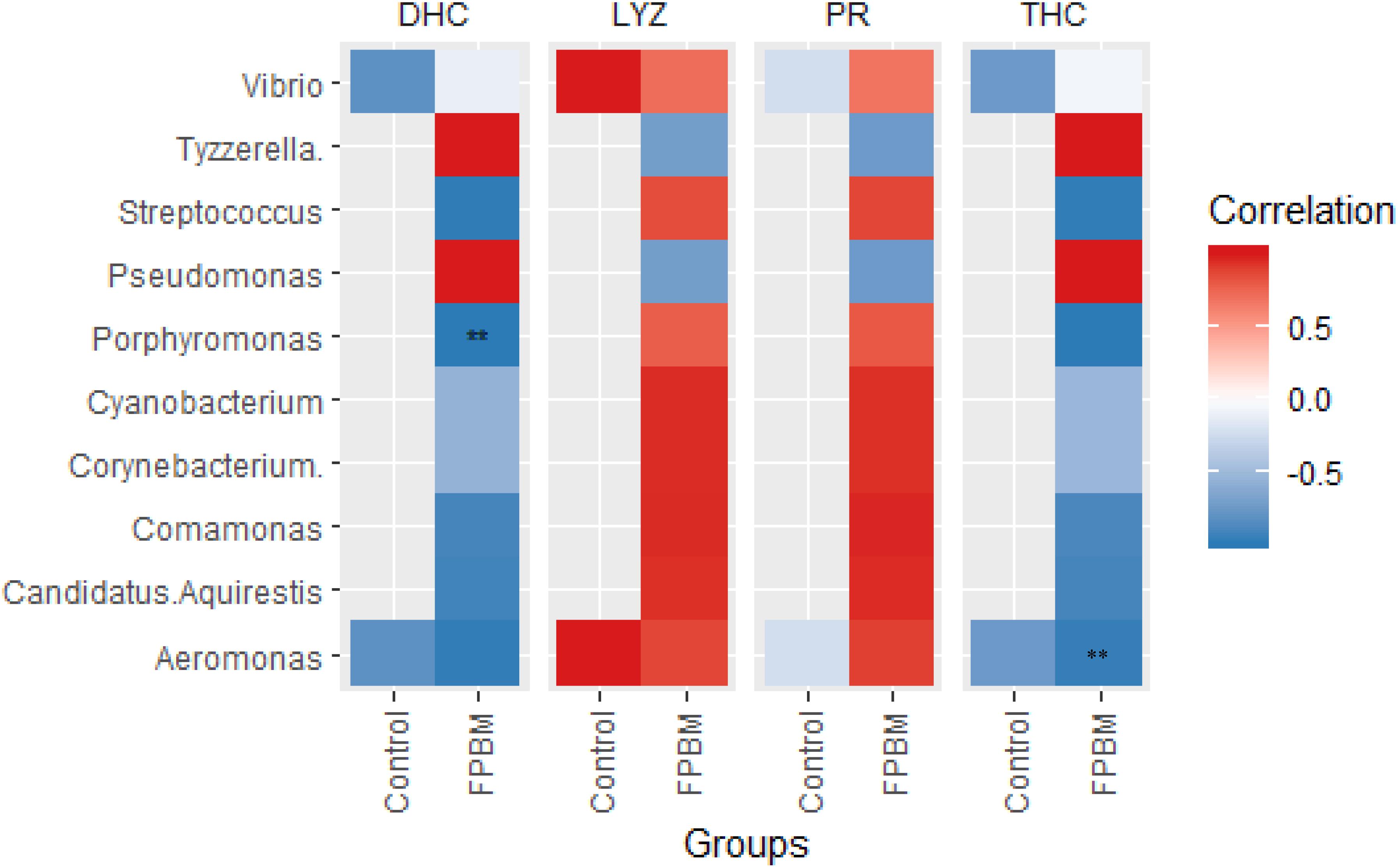
Figure 6. Pearson correlation plot showing the relationship among the microbial community at genus level and immune indices of marron. ** indicates significantly different at P < 0.05.
V. mimicus Injection Challenge Test
Kaplan-Meier survival curves with significant log-rank P-value [χ2 (1) = 5.74, P = 0.017] of marron fed control and FPBM diets are presented in Figure 7. Marron offered FPBM showed a significantly higher survival rate [χ2 (1) = 4.48, P = 0.034] following the challenge of V. mimicus for 7 days when compared to the control.
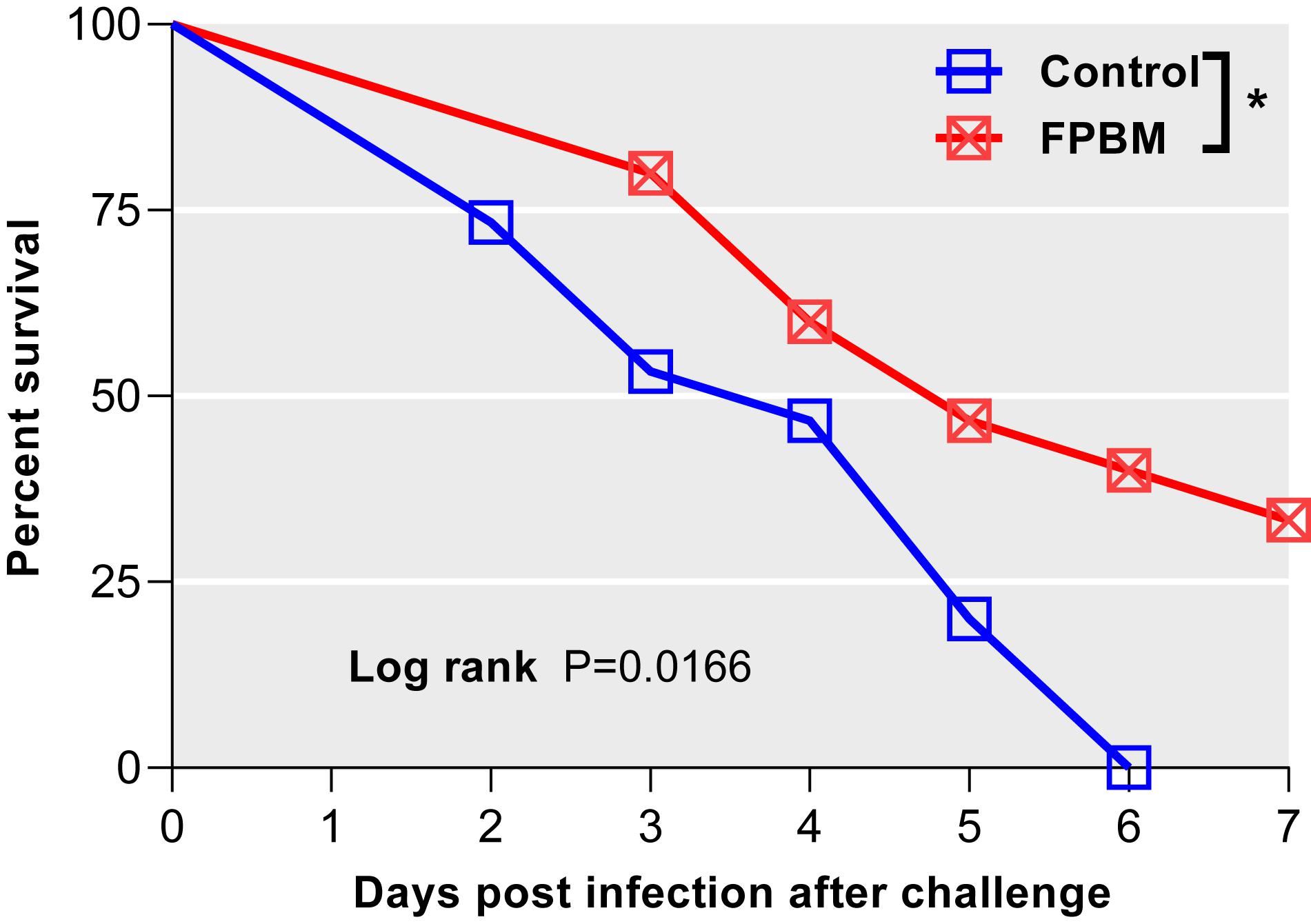
Figure 7. Comparison of survival of marron after V. mimicus challenge where n = 15 for each treatment. Kaplan-Meier plots with log rank test reveals that infection both in control and fermented poultry by-products meal (FPBM) started at 2 days post challenge (dpc). Marron fed FPBM showed significantly higher post challenge survival compared to control treated groups. *Indicates significant difference for survival at P < 0.05.
Discussion
Feeding marron with FPBM had no influence on FBW and SGR in the present study. This result is in agreement with a previous study on marron fed PBM supplemented with black soldier fry insect meal and found no variations in growth performance (Foysal et al., 2019a). Past studies have also demonstrated that FM ingredients can be replaced completely by using other protein sources without negative effects on growth but compromising biochemical and immunological responses of marron (Fotedar, 2004; Saputra et al., 2019). Whilst there is little literature about the application of fermented animal protein source diets in crustacean aquaculture, there are some studies with fermented plant source diets. Ding et al., 2015 suggested that inclusion of 25% fermented soy bean meal improved the FBW of freshwater prawn, Macrobrachium nipponense and that further increasing the inclusion levels up to 100% did not affect the growth performance. A study on white shrimp, Litopenaeus vannamei fed 25% fermented soybean meal for 12 weeks found no difference in growth performance when compared to the shrimp fed the FM based control (Lin and Mui, 2017). The discrepancies amongst the findings of these studies might be attributed to a number of factors such as the raw material, fermentation conditions including the specific types of microorganisms, temperature and feed to water ratio (Canibe and Jensen, 2012; Missotten et al., 2015) and substrate quality and quantity including compositional aspects of carbohydrates, fibers, protein, amino acids and vitamins (Niba et al., 2009). As an example, the crude protein content of soybean meal was increased after fermented with Bacillus subtilisor and Aspergillus oryzae (Teng et al., 2012), whereas, the content of protein and lipid was unchanged in soybean meal fermented by Bacillus spp. (Yamamoto et al., 2004).
It is generally assumed that longer intestinal microvillus size and density are associated with higher absorptive efficiency, leading to better nutrient utilization and improved immune function. Dimitroglou et al. (2009) stated that good intestinal health is of great importance in improving the health status of the mucosal epithelium as well as increasing the ability to prevent invading pathogens. In the present study, higher numbers of microvilli per intestinal fold were found in marron fed with FPBM. This may be due to the presence of yeast and bacteria in FPBM which may act as probiotics and could be responsible for improved nutrient absorption and utilization leading to an enhanced immune response. Lin and Mui (2017) claimed that soybean meal fermented with Lactobacillus spp. prevented the suppression of immune function triggered by soybean meal in white shrimp, Litopenaeus vannamei. However, the results of our previous research indicated that replacing FM with non-fermented PBM leads to no significant variation in microvilli number in the midgut of marron (Saputra et al., 2019).
Recently, manipulation of gut microbiota of farmed aquatic animals through dietary modulation an emerging area for aquaculture advancement. Gut microbiota play an important role in crustaceans by participating in a variety of physiological functions including feed digestion, reduction of the prevalence of pathogenic bacteria and/or synthesis of the amino acids, vitamins and trace elements (Merrifield and Ringø, 2014; Mente et al., 2016; Gao et al., 2019). A limited number of studies have been conducted on characterization of the gut microbiota of crustaceans and hence, the present study employed 16sRNA Illumina sequencing, a modern technique, for the first time to investigate the intestinal microbiota of marron post feeding with FPBM. The results showed that regardless of the diet, Actinobacteria, Bacteroidetes, Proteobacteria, Tenericutes, Firmicutes and Fusobacteria were the predominant phylum in the intestine of marron, which is in line with previous studies in other crustaceans, such as Litopenaeus vannamei (Xiong et al., 2015; Zhang et al., 2016) and Peneous monodon (Rungrassamee et al., 2013). However, in the present study, the abundance of Firmicutes (including Lactobacillus spp. and Streptococcus spp.) significantly increased in the intestine of marron fed FPBM. Similarly, Firmicutes phylum, namely under the genera of lactic acid bacteria (LAB), such as Lactobacillus, Lactococcus, and Pediococcus were enriched significantly in the intestinal content of Atlantic Salmon, Salmo salar fed fermented soybean meal in comparison with fish fed either a non-fermented or FM-based diet (Catalán et al., 2018). Many strains of Firmicutes are potent probiotics due to their beneficial effects on metabolism, digestion (by releasing different enzymes), adjustment of the intestinal environment and regulation of intestinal mucosal immunity of aquatic animals (Smriga et al., 2010). Fermented products also contain a diverse group of prebiotic compounds attracting and stimulating the growth of probiotics in terms of LAB and glucans (Swain et al., 2014). In the present study, Aeromonas was decreased in the group fed with the FPBM based diet when compared with the group fed the control diet. Aeromonas is a pathogenic group of bacteria that causes infection in fish and crustaceans (Birkbeck and Ringø, 2005; Ringo et al., 2007; Chen et al., 2018). LAB used in the present study can produce lactic acid which may reduce the pH of the intestinal content, resulting in the inhibition of the proliferation of pathogenic bacteria (Parvez et al., 2006). Pearson’s correlation revealed the negative association between DHC and Porphyromonas in the FPBM treated group. Although the effect of Porphyromonas in marron is not well understood, this pathogenic bacteria genus in humans causes chronic inflammatory disease (Mysak et al., 2014). It can be suggested that the proliferation of beneficial bacteria and reduction of the prevalence of pathogenic bacteria in the FPBM diet, as shown in this study demonstrates the potential beneficial effects of fermentation bacteria and products on the gut health of marron.
Many secondary metabolites ranging from antibiotics to peptides have been produced during the process of fermentation (Hayes and García-Vaquero, 2016), which are very much effective at elevating innate and adaptive immunity (Tsai et al., 2012). Oral administration of Lactobacillus sakei K101 obtained from fermented dairy product and Lactobacillus plantarum K55-5 obtained from fermented vegetable significantly increased cytokines such as L-10, IL-12, IFN-γ, and TNF-α in mouse spleen and blood (Lee et al., 2016). Elshopakey et al. (2018) reported an elevated level of immune related cytokines encompassing antimicrobial peptides (AMPs) and toll-like receptors (TLR) in the intestine of kuruma shrimp, Marsupenaeus japonicas fed 5.5 and 55 g fermented vegetable product (FVP). Similarly in the present study, pro-inflammatory cytokines including TNF-α, IL-1β, IL-8, and IL-17F demonstrated higher gene expression in the intestine of marron fed FPBM. These cytokines regulate many biological activities including immune response and maintenance of homeostasis of the immune system, bactericidal activities and lysozyme synthesis (Giri et al., 2015), and enhancement of host defense mechanism against bacteria and fungi (Moulton, 2016). IL-10, an important anti-inflammatory having both immunosuppressive and immunostimulatory properties, can enhance B-cell mediated functions, proliferation and antibody production (Deng and Tsao, 2013). IL-10 responses vary with different stages of inflammation, indicative of its major role in recovering from inflammation (Foysal et al., 2019b). For instance, it has been reported that the expression level of anti-inflammatory cytokines increase aligned with overexpression of pro-inflammatory genes to neutralize inflammation (Foysal et al., 2019a). In this study, the expression level of IL-10 was significantly higher in marron fed FPBM than in the group fed the control diet.
In crustacean haemocytes, antimicrobial effector cells play an important role in regulating physiological and immunological functions by acting in both adoption of the cellular immunity and supply of the humoral immunity (Liu et al., 2009; Hong et al., 2017; Saputra et al., 2019). The THC and DHC in the circulatory system of crustaceans may be easily affected by intrinsic factors and extrinsic factors including heavy metals, pathogens and pesticides (Cheng and Chen, 2000; Yao et al., 2008). Elevation of these two haemocytes parameters may induce immune competence against environmental stress (Burgos-Aceves et al., 2018; Nedaei et al., 2019) and increase resistant in response to opportunistic pathogens (Cheng and Chen, 2001; Pagano et al., 2017). In the present study, THC and DHC increased significantly in marron fed FPBM when compared to the control after 70 days and were also elevated in both control and FPBM treated groups after challenge with V. mimicus. These findings are in agreement with Elshopakey et al. (2018), who reported elevated level of THC in kuruma shrimp, Marsupenaeus japonicas when fed with fermented vegetable product.
Lysozyme, an important component of innate immune system and antimicrobial enzyme is present in phagocytic cells, and may act as an opsonin by lysing the peptidoglycans in Gram-positive bacterial cell walls (Nilojan et al., 2017). Many studies have reported elevated levels of lysozyme expression following bacterial infection (Tyagi et al., 2007; Yao et al., 2008). In this study, the lysozyme levels of marron that received FPBM were significantly elevated following 7 days challenged with V. mimicus when compared to levels before challenge. Similar to the LYZ result, post challenge marron that was fed FPBM revealed significantly higher PR than the pre challenge groups. In agreement with some previous studies (Kumar et al., 2014; Elshopakey et al., 2018), the present results proved the immunomodulatory effects of FPBM on marron by elevating the level of THC, DHC, PR, and LYZ.
Enhancement of disease resistance through the modulation of nutrition has recently become an aquaculture research priority as a strategy to increase immune response and therefore to reduce the dependence on chemotherapeutics (Pohlenz and Gatlin, 2014; Elshopakey et al., 2018). Fermentation has been reported to release bioactive peptides by the action of proteolytic enzymes produced by fermented microorganisms and these peptides have been attributed to have therapeutic benefits including immunomodulatory (Lin and Mui, 2017), antioxidant (Sanjukta et al., 2015) and antimicrobial activity (Eom et al., 2014). Antimicrobial peptides (AMPs) derived from fermentation have been considered as one of the major humoral immune effectors, which can act against both gram-positive and gram-negative bacteria, viruses, fungi and protozoans (Brown and Hancock, 2006). Three antimicrobial peptides derived from Bacillus subtilis E20-fermented soybean meal were shown to have antimicrobial activities against Vibrio infection in shrimp, while no antimicrobial activities were found in un-fermented soybean meal fed shrimp (Cheng et al., 2017). In this study, a significantly higher survival rate following V. mimicus challenge was observed in marron fed FPBM than the marron offered the control diet. Similarly, fermented soybean product in the diet of white leg shrimp, Penaeus vannamei significantly increased the survival rate against Vibrio harveyi when compared with control shrimp given diet without these product (Yatip et al., 2018). However, mortality of freshwater prawn, Macrobrachium nipponense against Aeromonas hydrophila was not significantly different between the control and diets with up to 50% of fermented soybean meal although it increased significantly in 75 and 100% FSM treated groups (Ding et al., 2015).
Conclusion
The results demonstrated that FPBM was immunologically favorable to marron, indicated by the elevated immune response and immune related cytokines, as well as increased numbers of beneficial microbiota. The abundance of Firmicutes bacterial communities suggest that positive effects on the gut microbial composition is possible to obtain through the manipulation of aqua-diets, and therefore, on the physiological condition of the host. Dietary inclusion of FPBM also significantly enhanced the disease resistance against V. mimicus, as well as enhancing the immune response in post challenge marron, indicating an immunomodulating competency of FPBM. Further studies are required to explain the mode of action of fermented product addition on intestinal microbiota and disease resistance, which may lead to the development of a novel functional feed for marron and its sustainable aquaculture production.
Data Availability Statement
The datasets in this study can be found in the BioProject database, https://www.ncbi.nlm.nih.gov/bioproject/PRJNA521663 (accession: PRJNA521663).
Author Contributions
MS and RF were involved in designing the experiment and proofreading the manuscript. MS and MC were involved in conducting the experiment, analyzed the data, and wrote the manuscript. MF was involved in all in vitro and in vivo analyses of the study. AN helped in innate immune response and electron microscopy analysis. JH helped in the experimental design and proofreading the manuscript.
Funding
This work was supported through the Research Training Program Scholarship, Australia to MS (No. 17405029-Curtin).
Conflict of Interest
The authors declare that the research was conducted in the absence of any commercial or financial relationships that could be construed as a potential conflict of interest.
Acknowledgments
We sincerely thank Rowan Kleindienst for his assistance on various aspects of the project. We also grateful to Dr. Chin-Yen Tay and Binit Lamichhane for support of the Helicobacter Research Laboratory, University of Western Australia.
References
Andrews, S. (2010). FastQC: A Quality Control Tool for High Throughput Sequence Data. Available at: http://www.bioinformatics.babraham.ac.uk/projects/fastqc (accessed July 15, 2019).
Ashayerizadeh, A., Dastar, B., Shams Shargh, M., Sadeghi Mahoonak, A., and Zerehdaran, S. (2017). Fermented rapeseed meal is effective in controlling Salmonella enterica serovar Typhimurium infection and improving growth performance in broiler chicks. Vet. Microbiol. 201, 93–102. doi: 10.1016/j.vetmic.2017.01.007
Bates, J. M., Mittge, E., Kuhlman, J., Baden, K. N., Cheesman, S. E., and Guillemin, K. (2006). Distinct signals from the microbiota promote different aspects of zebrafish gut differentiation. Dev. Biol. 297, 374–386. doi: 10.1016/j.ydbio.2006.05.006
Birkbeck, T. H., and Ringø, E. (2005). Microbial Ecology of the Growing Animal. Edinburgh: Elsevier, 208–234.
Brown, K. L., and Hancock, R. E. (2006). Cationic host defense (antimicrobial) peptides. Curr. Opin. Immunol. 18, 24–30. doi: 10.1016/j.coi.2005.11.004
Burgos-Aceves, M. A., Cohen, A., Smith, Y., and Faggio, C. (2018). MicroRNAs and their role on fish oxidative stress during xenobiotic environmental exposures. Ecotoxicol. Environ. Saf. 148, 995–1000. doi: 10.1016/j.ecoenv.2017.12.001
Canibe, N., and Jensen, B. B. (2003). Fermented and nonfermented liquid feed to growing pigs: effect on aspects of gastrointestinal ecology and growth performance. J. Anim. Sci. 81, 2019–2031. doi: 10.2527/2003.8182019x
Canibe, N., and Jensen, B. B. (2012). Fermented liquid feed—microbial and nutritional aspects and impact on enteric diseases in pigs. Anim. Feed Sci. Technol. 173, 17–40. doi: 10.1016/j.anifeedsci.2011.12.021
Catalán, N., Villasante, A., Wacyk, J., Ramírez, C., and Romero, J. (2018). Fermented soybean meal increases lactic acid bacteria in gut microbiota of Atlantic salmon (Salmo salar). Probiotics Antimicrob. Proteins 10, 566–576. doi: 10.1007/s12602-017-9366-7
Chen, H., Mai, K., Zhang, W., Liufu, Z., Xu, W., and Tan, B. (2005). Effects of dietary pyridoxine on immune responses in abalone, Haliotis discus hannai Ino. Fish Shellfish Immunol. 19, 241–252. doi: 10.1016/j.fsi.2004.12.006
Chen, L., Hu, C., Lok-Shun Lai, N., Zhang, W., Hua, J., Lam, P. K. S., et al. (2018). Acute exposure to PBDEs at an environmentally realistic concentration causes abrupt changes in the gut microbiota and host health of zebrafish. Environ. Pollut. 240, 17–26. doi: 10.1016/j.envpol.2018.04.062
Cheng, A. C., Lin, H. L., Shiu, Y. L., Tyan, Y. C., and Liu, C. H. (2017). Isolation and characterization of antimicrobial peptides derived from Bacillus subtilis E20-fermented soybean meal and its use for preventing Vibrio infection in shrimp aquaculture. Fish Shellfish Immunol. 67, 270–279. doi: 10.1016/j.fsi.2017.06.006
Cheng, W., and Chen, J. C. (2000). Effects of pH, temperature and salinity on immune parameters of the freshwater prawn Macrobrachium rosenbergii. Fish Shellfish Immunol. 10, 387–391. doi: 10.1006/fsim.2000.0264
Cheng, W., and Chen, J. C. (2001). Effects of intrinsic and extrinsic factors on the haemocyte profile of the prawn, Macrobrachium rosenbergii. Fish Shellfish Immunol. 11, 53–63. doi: 10.1006/fsim.2000.0293
Chettri, R., and Tamang, J. (2014). Functional properties of Tungrymbai and Bekang, naturally fermented soybean foods of North East India. Int. J. Fermented Foods 3, 85–104. doi: 10.5958/2321-712X.2014.01311.8
Deng, Y., and Tsao, B. P. (2013). “Chapter 4 - genetics of human SLE,” in Dubois’ Lupus Erythematosus and Related Syndromes, 8th Edn, eds D. J. Wallace, and B. H. Hahn (Philadelphia, PA: W.B. Saunders), 35–45. doi: 10.1016/b978-1-4377-1893-5.00004-2
Dimitroglou, A., Merrifield, D. L., Moate, R., Davies, S. J., Spring, P., Sweetman, J., et al. (2009). Dietary mannan oligosaccharide supplementation modulates intestinal microbial ecology and improves gut morphology of rainbow trout, Oncorhynchus mykiss (Walbaum). J. Anim. Sci. 87, 3226–3234. doi: 10.2527/jas.2008-1428
Ding, Z., Zhang, Y., Ye, J., Du, Z., and Kong, Y. (2015). An evaluation of replacing fish meal with fermented soybean meal in the diet of Macrobrachium nipponense: growth, nonspecific immunity, and resistance to Aeromonas hydrophila. Fish Shellfish Immunol. 44, 295–301. doi: 10.1016/j.fsi.2015.02.024
Elshopakey, G. E., Risha, E. F., Abdalla, O. A., Okamura, Y., Harada, S., Kishida, S., et al. (2018). Efficacy of dietary fermented vegetable product on immune response, up-regulation of immune-related genes and protection of kuruma shrimp (Marsupenaeus japonicus) against Vibrio parahaemolyticus. Aquaculture 497, 431–439. doi: 10.1016/j.aquaculture.2018.08.013
Eom, J. S., Lee, S. Y., and Choi, H. S. (2014). Bacillus subtilis HJ18-4 from traditional fermented soybean food inhibits Bacillus cereus growth and toxin-related genes. J. Food Sci. 79, M2279–M2287. doi: 10.1111/1750-3841.12569
FAO, (2018). Global World Aquaculture Production Food and Agriculture Organization of the United Nations. Available at: http://www.fao.org/fishery/en (accessed October 10, 2018).
Fotedar, R. (2004). Effect of dietary protein and lipid source on the growth, survival, condition indices, and body composition of marron, Cherax tenuimanus (Smith). Aquaculture 230, 439–455. doi: 10.1016/s0044-8486(03)00418-6
Foysal, M. J., Fotedar, R., Tay, C. Y., and Gupta, S. K. (2019a). Dietary supplementation of black soldier fly (Hermetica illucens) meal modulates gut microbiota, innate immune response and health status of marron (Cherax cainii, Austin 2002) fed poultry-by-product and fishmeal based diets. PeerJ 7:e6891. doi: 10.7717/peerj.6891
Foysal, M. J., Nguyen, T. T. T., Chaklader, M. R., Siddik, M. A. B., Tay, C.-Y., Fotedar, R., et al. (2019b). Marked variations in gut microbiota and some innate immune responses of fresh water crayfish, marron (Cherax cainii, Austin 2002) fed dietary supplementation of Clostridium butyricum. PeerJ 7:e7553. doi: 10.7717/peerj.7553
Gao, S., Pan, L., Huang, F., Song, M., Tian, C., and Zhang, M. (2019). Metagenomic insights into the structure and function of intestinal microbiota of the farmed Pacific white shrimp (Litopenaeus vannamei). Aquaculture 499, 109–118. doi: 10.1016/j.aquaculture.2018.09.026
Giri, S. S., Sen, S. S., Chi, C., Kim, H. J., Yun, S., Park, S. C., et al. (2015). Effect of cellular products of potential probiotic bacteria on the immune response of Labeo rohita and susceptibility to Aeromonas hydrophila infection. Fish Shellfish Immunol. 46, 716–722. doi: 10.1016/j.fsi.2015.08.012
Hayes, M., and García-Vaquero, M. (2016). “Bioactive compounds from fermented food products,” in Novel Food Fermentation Technologies. Food Engineering Series, eds K. Ojha, and B. Tiwari (Cham: Springer).
Hill, C., Guarner, F., Reid, G., Gibson, G. R., Merenstein, D. J., Pot, B., et al. (2014). Expert consensus document. The international scientific association for probiotics and prebiotics consensus statement on the scope and appropriate use of the term probiotic. Nat. Rev. Gastroenterol. Hepatol. 11, 506–514. doi: 10.1038/nrgastro.2014.66
Hong, Y., Yang, X., Yan, G., Huang, Y., Zuo, F., Shen, Y., et al. (2017). Effects of glyphosate on immune responses and haemocyte DNA damage of Chinese mitten crab, Eriocheir sinensis. Fish Shellfish Immunol. 71, 19–27. doi: 10.1016/j.fsi.2017.09.062
Hooper, C., Day, R., Slocombe, R., Benkendorff, K., Handlinger, J., and Goulias, J. (2014). Effects of severe heat stress on immune function, biochemistry and histopathology in farmed Australian abalone (hybrid Haliotis laevigata × Haliotis rubra). Aquaculture 432, 26–37. doi: 10.1016/j.aquaculture.2014.03.032
Hooper, L. V., Midtvedt, T., and Gordon, J. I. (2002). How host-microbial interactions shape the nutrient environment of the mammalian intestine. Annu. Rev. Nutr. 22, 283–307.
Jin, M., Xiong, J., Zhou, Q.-C., Yuan, Y., Wang, X.-X., and Sun, P. (2018). Dietary yeast hydrolysate and brewer’s yeast supplementation could enhance growth performance, innate immunity capacity and ammonia nitrogen stress resistance ability of Pacific white shrimp (Litopenaeus vannamei). Fish Shellfish Immunol. 82, 121–129. doi: 10.1016/j.fsi.2018.08.020
Joshi, N. A., and Fass, J. N. (2011). Sickle: A Sliding-Window, Adaptive, Quality-Based Trimming Tool for FastQ Files (Version 1.33)[Software].
Kader, M. A., Shunsuke, K., Manabu, I., Saichiro, Y., Mahbuba, B., Binh, T. H., et al. (2012). Can fermented soybean meal and squid by-product blend be used as fishmeal replacements for Japanese flounder (Paralichthys olivaceus)? Aquac. Res. 43, 1427–1438. doi: 10.1111/j.1365-2109.2011.02945.x
Kuczynski, J., Stombaugh, J., Walters, W. A., González, A., Caporaso, J. G., and Knight, R. (2011). Using QIIME to analyze 16S rRNA gene sequences from microbial communities. Curr. Protoc. Bioinformatics 36, 10.7.1–10.7.20.
Kumar, K., Yong-Hyun, Y., Gunhyun, P., Jeong-Yeol, L., Gwangyeol, Y., and Sungchul, C. B. (2014). Evaluation of the efficacy of fermented by-product of mushroom, Pleurotus ostreatus, as a fish meal replacer in juvenile amur catfish, Silurus asotus: effects on growth, serological characteristics and immune responses. Asian Australas. J. Anim. Sci. 27, 1478–1486. doi: 10.5713/ajas.2014.14038
Lee, Y. D., Hong, Y. F., Jeon, B., Jung, B. J., Chung, D. K., and Kim, H. (2016). Differential cytokine regulatory effect of three lactobacillus strains isolated from fermented foods. J. Microbiol. Biotechnol. 26, 1517–1526. doi: 10.4014/jmb.1601.01044
Lin, Y. H., and Mui, J. J. (2017). Comparison of dietary inclusion of commercial and fermented soybean meal on oxidative status and non-specific immune responses in white shrimp, Litopenaeus vannamei. Fish Shellfish Immunol. 63, 208–212. doi: 10.1016/j.fsi.2017.02.011
Liu, J., Pan, L. Q., Zhang, L., Miao, J., and Wang, J. (2009). Immune responses, ROS generation and the haemocyte damage of scallop Chlamys farreri exposed to Aroclor 1254. Fish Shellfish Immunol. 26, 422–428. doi: 10.1016/j.fsi.2009.01.002
Mente, E., Gannon, A. T., Nikouli, E., Hammer, H., and Kormas, K. A. (2016). Gut microbial communities associated with the molting stages of the giant freshwater prawn Macrobrachium rosenbergii. Aquaculture 463, 181–188. doi: 10.1016/j.aquaculture.2016.05.045
Merrifield, D. E., and Ringø, E. E. (2014). Aquaculture Nutrition: Gut Health, Probiotics, and Prebiotics, eds M. Daniel, and R. Einar (Chichester: Wiley Blackwell).
Militon, C., Boucher, D., Vachelard, C., Perchet, G., Barra, V., Troquet, J., et al. (2010). Bacterial community changes during bioremediation of aliphatic hydrocarbon-contaminated soil. FEMS Microbiol. Ecol. 74, 669–681. doi: 10.1111/j.1574-6941.2010.00982.x
Mirarab, S., Nguyen, N., Guo, S., Wang, L.-S., Kim, J., and Warnow, T. (2015). PASTA: ultra-large multiple sequence alignment for nucleotide and amino-acid sequences. J. Comput. Biol. 22, 377–386. doi: 10.1089/cmb.2014.0156
Missotten, J. A. M., Michiels, J., Degroote, J., and Smet, S. D. (2015). Fermented liquid feed for pigs: an ancient technique for the future. J. Anim. Sci. Biotechnol. 6:4. doi: 10.1186/2049-1891-6-4
Moulton, V. R. (2016). “Chapter 17 - cytokines,” in Systemic Lupus Erythematosus, ed. G. C. Tsokos (Boston, MA: Academic Press), 137–141.
Mysak, J., Podzimek, S., Sommerova, P., Lyuya-Mi, Y., Bartova, J., Janatova, T., et al. (2014). Porphyromonas gingivalis: major periodontopathic pathogen overview. J. Immunol. Res. 2014:476068. doi: 10.1155/2014/476068
Nedaei, S., Noori, A., Valipour, A., Khanipour, A. A., and Hoseinifar, S. H. (2019). Effects of dietary galactooligosaccharide enriched commercial prebiotic on growth performance, innate immune response, stress resistance, intestinal microbiota and digestive enzyme activity in Narrow clawed crayfish (Astacus leptodactylus Eschscholtz, 1823). Aquaculture 499, 80–89. doi: 10.1016/j.aquaculture.2018.08.076
Niba, A. T., Beal, J. D., Kudi, A. C., and Brooks, P. H. (2009). Bacterial fermentation in the gastrointestinal tract of non-ruminants: influence of fermented feeds and fermentable carbohydrates. Trop. Anim. Health Prod. 41, 1393–1407. doi: 10.1007/s11250-009-9327-6
Nilojan, J., Bathige, S., Kugapreethan, R., Thulasitha, W. S., Nam, B. H., and Lee, J. (2017). Molecular, transcriptional and functional insights into duplicated goose-type lysozymes from Sebastes schlegelii and their potential immunological role. Fish Shellfish Immunol. 67, 66–77. doi: 10.1016/j.fsi.2017.05.037
Nugroho, R. A., and Fotedar, R. (2013). Dietary organic selenium improves growth, survival and resistance to Vibrio mimicus in cultured marron, Cherax cainii (Austin, 2002). Fish Shellfish Immunol. 35, 79–85. doi: 10.1016/j.fsi.2013.04.011
Oksanen, A. J., Blanchet, F. G., Kindt, R., Legen, P., Minchin, P. R., Hara, R. B. O., et al. (2018). Package “vegan.” [Software]. Available at: https://cran.r-project.org (accessed December 12, 2019).
Pagano, M., Porcino, C., Briglia, M., Fiorino, E., Vazzana, M., Silvestro, S., et al. (2017). The influence of exposure of cadmium chloride and zinc chloride on haemolymph and digestive gland cells from Mytilus galloprovincialis. Int. J. Environ. Res. 11, 207–216. doi: 10.1007/s41742-017-0020-8
Parikh, H., Koparde, V., Bradley, S., Buck, G., and Sheth, N. (2016). MeFiT: merging and filtering tool for illumina paired-end reads for 16S rRNA amplicon sequencing. BMC Bioinformatics 17:491. doi: 10.1186/s12859-016-1358-1
Parvez, S., Malik, K. A., Ah Kang, S., and Kim, H. Y. (2006). Probiotics and their fermented food products are beneficial for health. J. Appl. Microbiol. 100, 1171–1185. doi: 10.1111/j.1365-2672.2006.02963.x
Pohlenz, C., and Gatlin, D. M. (2014). Interrelationships between fish nutrition and health. Aquaculture 431, 111–117. doi: 10.1016/j.aquaculture.2014.02.008
Pourmozaffar, S., Hajimoradloo, A., Paknejad, H., and Rameshi, H. (2019). Effect of dietary supplementation with apple cider vinegar and propionic acid on hemolymph chemistry, intestinal microbiota and histological structure of hepatopancreas in white shrimp, Litopenaeus vannamei. Fish Shellfish Immunol. 86, 900–905. doi: 10.1016/j.fsi.2018.12.019
Quast, C., Pruesse, E., Yilmaz, P., Gerken, J., Schweer, T., Yarza, P., et al. (2013). The SILVA ribosomal RNA gene database project: improved data processing and web-based tools. Nucleic Acids Res. 41, D590–D596. doi: 10.1093/nar/gks1219
Ringo, E., Myklebust, R., Mayhew, T., and Olsen, R. (2007). Bacterial translocation and pathogenesis in the digestive tract of larvae and fry. Aquaculture 268, 251–264. doi: 10.1016/j.aquaculture.2007.04.047
Rungrassamee, W., Klanchui, A., Chaiyapechara, S., Maibunkaew, S., Tangphatsornruang, S., Jiravanichpaisal, P., et al. (2013). Bacterial population in intestines of the black tiger shrimp (Penaeus monodon) under different growth stages. PLoS One 8:e60802. doi: 10.1371/journal.pone.0060802
Sanjukta, S., Rai, A. K., Muhammed, A., Jeyaram, K., and Talukdar, N. C. (2015). Enhancement of antioxidant properties of two soybean varieties of Sikkim Himalayan region by proteolytic Bacillus subtilis fermentation. J. Funct. Foods 14, 650–658. doi: 10.1016/j.jff.2015.02.033
Saputra, I., Fotedar, R., Gupta, S. K., Siddik, M. A. B., and Foysal, M. J. (2019). Effects of different dietary protein sources on the immunological and physiological responses of marron, Cherax cainii (Austin and Ryan, 2002) and its susceptibility to high temperature exposure. Fish Shellfish Immunol. 88, 567–577. doi: 10.1016/j.fsi.2019.03.012
Semova, I., Carten, J. D., Stombaugh, J., Mackey, L. C., Knight, R., Farber, S. A., et al. (2012). Microbiota regulate intestinal absorption and metabolism of fatty acids in the zebrafish. Cell Host Microbe 12, 277–288. doi: 10.1016/j.chom.2012.08.003
Siddik, M. A. B., Chungu, P., Fotedar, R., and Howieson, J. (2019a). Bioprocessed poultry by-product meals on growth, gut health and fatty acid synthesis of juvenile barramundi, Lates calcarifer (Bloch). PLoS One 14:e0215025. doi: 10.1371/journal.pone.0215025
Siddik, M. A. B., Howieson, J., and Fotedar, R. (2019b). Beneficial effects of tuna hydrolysate in poultry by-product meal diets on growth, immune response, intestinal health and disease resistance to Vibrio harveyi in juvenile barramundi, Lates calcarifer. Fish Shellfish Immunol. 89, 61–70. doi: 10.1016/j.fsi.2019.03.042
Siddik, M. A. B., Howieson, J., Partridge, G. J., Fotedar, R., and Gholipourkanani, H. (2018). Dietary tuna hydrolysate modulates growth performance, immune response, intestinal morphology and resistance to Streptococcus iniae in juvenile barramundi, Lates calcarifer. Sci. Rep. 8:15942. doi: 10.1038/s41598-018-34182-4
Smriga, S., Sandin, S. A., and Azam, F. (2010). Abundance, diversity, and activity of microbial assemblages associated with coral reef fish guts and feces. FEMS Microbiol. Ecol. 73, 31–42. doi: 10.1111/j.1574-6941.2010.00879.x
Swain, M. R., Anandharaj, M., Ray, R. C., and Praveen Rani, R. (2014). Fermented fruits and vegetables of Asia: a potential source of probiotics. Biotechnol. Res. Int. 2014:250424. doi: 10.1155/2014/250424
Teng, D., Gao, M., Yang, Y., Liu, B., Tian, Z., and Wang, J. (2012). Bio-modification of soybean meal with Bacillus subtilis or Aspergillus oryzae. Biocatal. Agric. Biotechnol. 1, 32–38. doi: 10.1016/j.bcab.2011.08.005
Thanasaksiri, K., Takano, R., Fukuda, K., Chaweepack, T., and Wongtavatchai, J. (2019). Identification of infectious spleen and kidney necrosis virus from farmed barramundi Lates calcarifer in Thailand and study of its pathogenicity. Aquaculture 500, 188–191. doi: 10.1016/j.aquaculture.2018.09.035
Tsai, Y. T., Cheng, P. C., and Pan, T. M. (2012). The immunomodulatory effects of lactic acid bacteria for improving immune functions and benefits. Appl. Microbiol. Biotechnol. 96, 853–862. doi: 10.1007/s00253-012-4407-3
Tyagi, A., Khushiramani, R., Karunasagar, I., and Karunasagar, I. (2007). Antivibrio activity of recombinant lysozyme expressed from black tiger shrimp, Penaeus monodon. Aquaculture 272, 246–253. doi: 10.1016/j.aquaculture.2007.08.055
Xiong, J., Wang, K., Wu, J., Qiuqian, L., Yang, K., Qian, Y., et al. (2015). Changes in intestinal bacterial communities are closely associated with shrimp disease severity. Appl. Microbiol. Biotechnol. 99, 6911–6919. doi: 10.1007/s00253-015-6632-z
Yamamoto, M., Saleh, F., and Hayashi, K. (2004). A fermentation method to dry and convert Shochu distillery by-product to a source of protein and enzymes. J. Poult. Sci. 41, 275–280. doi: 10.2141/jpsa.41.275
Yao, C.-L., Wu, C.-G., Xiang, J.-H., Li, F., Wang, Z.-Y., and Han, X. (2008). The lysosome and lysozyme response in Chinese shrimp Fenneropenaeus chinensis to Vibrio anguillarum and laminarin stimulation. J. Exp. Mar. Biol. Ecol. 363, 124–129. doi: 10.1016/j.jembe.2008.06.035
Yatip, P., Nitin Chandra Teja, D., Flegel, T. W., and Soowannayan, C. (2018). Extract from the fermented soybean product Natto inhibits Vibrio biofilm formation and reduces shrimp mortality from Vibrio harveyi infection. Fish Shellfish Immunol. 72, 348–355. doi: 10.1016/j.fsi.2017.11.008
Zhang, J., Chen, L., Dong, H., Duan, Y., Li, Z., Wen, G., et al. (2016). Artificial substrates in zero-water-exchange culture system regulate the rearing performance of Pacific white shrimp Litopenaeus vannamei (Boone, 1931) under the winter indoor condition. Aquac. Res. 47, 91–100. doi: 10.1111/are.12473
Keywords: crayfish, marron, fermentation, poultry by-product, cytokines, intestinal microbiome, Cherax cainii
Citation: Siddik MAB, Fotedar R, Chaklader MR, Foysal MJ, Nahar A and Howieson J (2020) Fermented Animal Source Protein as Substitution of Fishmeal on Intestinal Microbiota, Immune-Related Cytokines and Resistance to Vibrio mimicus in Freshwater Crayfish (Cherax cainii). Front. Physiol. 10:1635. doi: 10.3389/fphys.2019.01635
Received: 13 October 2019; Accepted: 27 December 2019;
Published: 31 January 2020.
Edited by:
Ignacio Ruiz-Jarabo, University of Cádiz, SpainCopyright © 2020 Siddik, Fotedar, Chaklader, Foysal, Nahar and Howieson. This is an open-access article distributed under the terms of the Creative Commons Attribution License (CC BY). The use, distribution or reproduction in other forums is permitted, provided the original author(s) and the copyright owner(s) are credited and that the original publication in this journal is cited, in accordance with accepted academic practice. No use, distribution or reproduction is permitted which does not comply with these terms.
*Correspondence: Muhammad A. B. Siddik, m.siddik@postgrad.curtin.edu.au; siddik@pstu.ac.bd
†Present address: Md Javed Foysal, Department of Genetic Engineering and Biotechnology, Shahjalal University of Science and Technology, Sylhet, Bangladesh