- 1Department of Sport, Health and Exercise Science, Faculty of Health Science, University of Hull, Hull, United Kingdom
- 2Mathematics and Physical Science, Faculty of Science and Engineering, University of Hull, Hull, United Kingdom
Introduction: Investigate the effectiveness of short-term heat acclimation (STHA), over 5-days (permissive dehydration), on an intermittent sprint exercise protocol (HST) with females. Controlling for menstrual cycle phase.
Materials and Methods: Ten, moderately trained, females (Mean [SD]; age 22.6 [2.7] y; stature 165.3 [6.2] cm; body mass 61.5 [8.7] kg; 43.9 [8.6] mL⋅kg–1⋅min–1) participated. The HST (31.0°C; 50%RH) was 9 × 5 min (45-min) of intermittent exercise, based on exercise intensities of female soccer players, using a motorized treadmill and Wattbike. Participants completed HST1 vs. HST2 as a control (C) trial. Followed by 90 min, STHA (no fluid intake), for five consecutive days in 39.5°C; 60%RH, using controlled-hyperthermia (∼rectal temperature [Tre] 38.5°C). The HST3 occurred within 1 week after STHA. The HST2 vs HST3 trials were in the luteal phase, using self-reported menstrual questionnaire and plasma 17β-estradiol.
Results: Pre (HST2) vs post (HST3) STHA there was a reduction at 45-min in Tre by 0.20°C (95%CI −0.30 to −0.10°C; d = 0.77); (−0.50; −0.90 to −0.10°C; d = 0.80); and (−0.25; −0.35 to −0.15°C; d = 0.92). Cardiac frequency reduced at 45-min (−8; −16 to −1 b⋅min–1; d = 1.11) and %PV increased (7.0; −0.4 to 14.5%: d = 1.27). Mean power output increased across all nine maximal sprints by 56W (−26 to 139W; d = 0.69; n = 9). There was limited difference (P > 0.05) for these measures in HST1 vs HST2 C trial.
Discussion: Short-term heat acclimation (5-days) using controlled-hyperthermia, leads to physiological adaptation during intermittent exercise in the heat, in moderately trained females when controlling for menstrual cycle phase.
Introduction
The worldwide popularity of football results in competitive matches being held in a whole host of environmental conditions, some of which can be in excess of 30°C with high levels of RH (Ozgunen et al., 2010). The 2016 Olympic Games in Brazil and the future 2020 Olympics in Tokyo, Japan (Gerrett et al., 2019) are examples of this.
It has been reported that the menstrual cycle plays a significant role in athletic performance in the heat (Avellini et al., 1979; Tenaglia et al., 1999; Janse de Jonge et al., 2012). However, research is limited and recent evidence has been contradictory. It has demonstrated little effect of the menstrual cycle (Sunderland and Nevill, 2003), oral contraceptive pill (OCP) usage (Lei et al., 2017) and in trained females who have smaller fluctuations in hormonal response (Lei and Mundel, 2018). However, the possible attenuation of endurance performance during heat stress has been reported (Avellini et al., 1979; Tenaglia et al., 1999; Constantini et al., 2005; Janse de Jonge et al., 2012) but this is not a universal finding (Kolka and Stephenson, 1997; Sunderland and Nevill, 2003; Lei et al., 2017). Secondly, there is limited research on fluid handling across the menstrual cycle with OCP usage (Stachenfeld et al., 1999; Stachenfeld, 2008). This supports the need for a robust research design in the present study. It is recognized that resting core temperature and the temperature threshold for the activation of the thermoregulatory responses, cutaneous vasodilation and sweating in females is elevated during the luteal phase of the menstrual cycle (Stachenfeld et al., 2000; Sunderland and Nevill, 2003). Especially, in those taking the OCP (Charkoudian and Johnson, 1997; Rogers and Baker, 1997) which can rise by ∼0.2–0.6°C in the early follicular phase (Stephenson and Kolka, 1985; Kolka and Stephenson, 1989; Grucza et al., 1993; Rogers and Baker, 1997). Many women feel that the menstrual cycle status negatively affects athletic performance (Wilson et al., 1991; Bruinvels et al., 2016) but in reality it has been reported that most physical aspects of athletic performance are not hindered by the menstrual cycle (Lebrun et al., 1995; Constantini et al., 2005; Wiecek et al., 2016; Tounsi et al., 2017). In summary, research on how the menstrual cycle phase affects exercise performance in unacclimated females in the heat is limited and contradictory. Furthermore, the limited information on female sex hormones, OCP and fluid regulation by Stachenfeld and colleagues (Stachenfeld et al., 1999; Stachenfeld, 2008) supports the research design of the present study.
There are fundamentally three models in which active heat acclimation can be achieved; (i) constant work-rate, (ii) self-regulated exercise and (iii) the controlled hyperthermia or isothermic technique (Taylor and Cotter, 2006). The controlled hyperthermia technique has been postulated to provide greater heat adaptation than the constant work-rate and self-regulated work-rate methodologies. On the basis that core temperature elevation is a key consideration for successful heat acclimation associated with high skin temperature and sweating response, as exercise alone is not a sufficient stimulus for adaptation (Hessemer et al., 1986). In contrast, there has been evidence to demonstrate isothermic and fixed intensity heat acclimation methods induce similar heat adaptation in the short and long-term. However, it is suggested controlled-hyperthermia is a more efficient and practical method for heat adaptation, especially for athletes tapering before competition (Gibson et al., 2015). The addition of a permissive dehydration stimulus, that is restricting fluid intake during acclimation has received recent attention in the literature (Akerman et al., 2016) and with a female cohort (Kirby et al., 2019). However, the benefits of permissive dehydration has not been reported universally (Neal et al., 2016). In our previous work using a male cohort (Garrett et al., 2014), permissive dehydration during acclimation has been shown to improve the fluid regulatory mechanisms by improving the reabsorption of water and Na+ resulting in PV expansion. This indicated that the adaptive response may be enhanced rather than impaired by dehydration acclimation and this work had a euhydration control. Furthermore, we have demonstrated that the use of permissive dehydration with STHA (5-days) has been shown to provide heat adaptation for moderately (Garrett et al., 2009) and highly trained (Garrett et al., 2012) male athletes. From a practical perspective it provides a very light exercise load that minimizes both additional exercise strain and the disruption of quality training during the tapering period for competition (Garrett et al., 2011).
Most heat acclimation protocols have been carried out using male cohorts. Furthermore, the limited research that has been conducted using female participants, to the author’s knowledge only three have used the controlled hyperthermia technique. Mee et al. (2015) reported that in response to STHA of 5-days with permissive dehydration, there was limited physiological change. Improvements in cardiovascular stability, lower core temperature and sudomotor adaptation required a longer, medium-term heat acclimation (MTHA) of 10-days (Mee et al., 2015). Similarly, Kirby et al. (2019) determined nine-, but not 4-days heat acclimation with permissive dehydration, improves self-paced endurance performance in females (Kirby et al., 2019). Daanen and Herweijer (2015) demonstrated limited responses to STHA of 3-days in a younger versus an older female population (Daanen and Herweijer, 2015). However, these studies may not give us all the information we need given their research designs have mixed OCP and non-users. Furthermore, they have not controlled for menstrual cycle phase.
The aims of the present study are to evaluate the physiological and performance effects of a STHA (5-day) protocol, using the controlled hyperthermia technique with no fluid intake, on an intermittent HST with a female cohort and controlling for menstrual cycle phase. It is hypothesized that STHA will improve thermoregulation in a cohort of young, moderately trained female game players when controlling for menstrual cycle phase.
Materials and Methods
Experimental Design and Overview
Ten moderately trained female participants undertook a 5-day STHA regime, with no fluid replenishment during each daily acclimation session. Participants’ thermoregulatory, cardiovascular and fluid-regulatory status were measured at rest and in response to an intermittent, exercising HST, administered the week before and after the 2nd day after the STHA regime to ensure 1 day of rest. Participants were asked to refrain from strenuous exercise immediately before and 24 h prior to each HST, as it has been demonstrated that lower resting core temperature contributes to reduced physiological strain during acclimation (Kampmann et al., 2008). A general overview of the STHA protocol for the moderately trained females is shown in Figure 1.
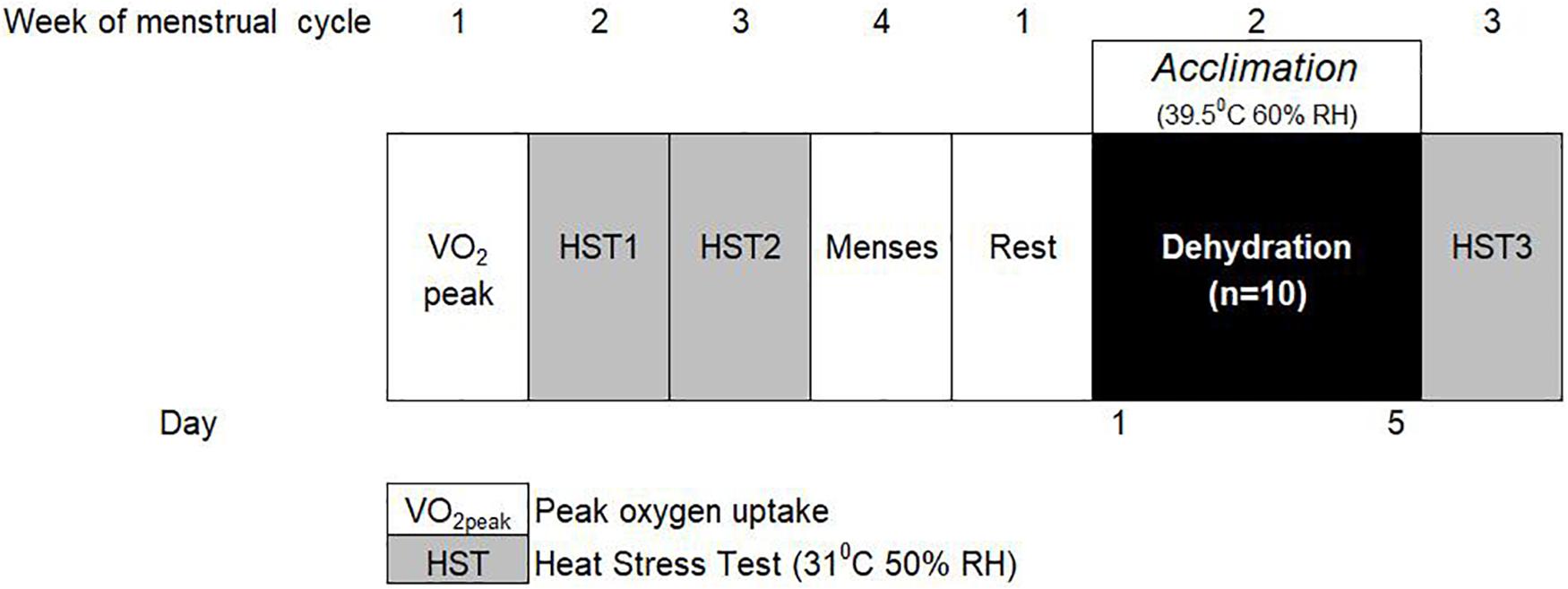
Figure 1. Schematic model of the short-term heat acclimation (STHA) protocol for moderately trained females.
Participants
Ten, moderately trained, females (Mean [SD]; age 22.6 [2.7] years; stature 165.3 [6.2] cm; body mass 61.5 [8.7] kg; cardiac output 5.5 [1.3] L; and 43.9 [8.6] mL⋅kg–1⋅min–1) participated. They were games players and oral contraceptive pill users (combined). Participants completed pre-exercise medical questionnaires and informed consent to participate in the study. All participants were in good health. The study had ethical approval (No. 1516177) from the University of Hull ethics committee following the World Health Organization declaration of Helsinki guidelines.
Protocol
Experimental Standardization
All participants were fully informed of all experimental procedures (orally and written). Prior to experimental testing participants completed pre-exercise medical questionnaires and informed consents. Each female participant used a monophasic, oral contraceptive pill (OCP) and dose of hormone concentration differed between individuals depending on their specific medication. All participants were previously unacclimated to the heat and this study was completed outside the British summertime to minimize seasonal acclimatization effects. To minimize circadian rhythm affects HSTs and acclimations occurred at the same time of day. Participants were asked to refrain from strenuous exercise for 24 h prior to HSTs and using a food diary follow a consistent food intake. They were asked to refrain from caffeine and alcohol consumption 12 h before all testing procedures.
As a methodological control participants completed HST2 and HST3 in the same phase of their menstrual cycle (luteal phase), in the active pill portion of the OCP. This was reported by menstrual cycle questionnaire. This detailed the start of the menstrual cycle, premenstrual symptoms and contraceptive medication. This was confirmed by baseline measurement of plasma 17β-estradiol (Table 1).

Table 1. Mean ± SD plasma 17β-estradiol in HST2 (pre-) and HST3 (post-) STHA in the luteal phases of the menstrual cycle.
Short-Term Heat Acclimation (STHA)
The STHA protocol consisted of five consecutive day’s heat exposure (39.5°C; 60% RH) for 90 min a day, using the controlled hyperthermia technique (Garrett et al., 2009), with permissive dehydration (Garrett et al., 2014). Participants cycled (Monark 824E, Monark Exercise AB, Varberg, Sweden) against a self-selected resistance at 60 rpm attaining a Tre 38.5°C, as quickly as possible and maintained for the 90 min of exposure by regular adjustment of workload. However, an initial workload of 60 watts for the first 5 min duration was the same for all participants at the start of each day’s trial. Elevation of Tre to the same point during heat exposures was to increase workload progressively during the week. The fluid-regulatory hormone aldosterone [aldosterone]p, electrolytes (Na+, K+, and Cl−), proteins (total protein [TP]p; Albumin [alb]p), cortisol [cortisol]p and percentage change in plasma volume (%PV) were measured at rest and end of acclimation bouts on day 1 and day 5. Time (minutes) time to a Tre of 38.5°C and work (J) was recorded on each day of acclimation.
Urinary measures
Urine samples were obtained before and after day 1 and day 5 acclimation. Using fresh urine samples, urine specific gravity and urine color were measured using a calibrated refractometer (Uricon-N, Urine specific gravity refractometer, Atago Co., Tokyo, Japan) and urine color chart (Armstrong et al., 1994, 1998), respectively. Urine volume was recorded and urine osmolality was analyzed after the experiment.
Blood measures
Plasma for the measurement of the fluid regulatory hormone aldosterone (200 μl) was stored using chilled K-EDTA tubes (1.6 mg⋅ml–1). Measurement of aldosterone and cortisol used the Coat-A Count aldosterone procedure. The intra-assay coefficient of variation for aldosterone and cortisol was 8.8 and 12.1%, respectively, for duplicate measures. All samples for a given individual were analyzed within the same assay. Plasma Na+, K+, and Cl− was analyzed using duplicate colorometric analysis (Cobas Mira Plus, New Jersey, United States).
Changes in the concentration of hemoglobin [Hb] and haematocrit [Hct] were used to determine the relative change in plasma volume described by Dill and Costill (1974). Venous blood samples (5 mL) were taken from an antecubital vein (Vacutainer Precision Glide 21-gauge needle, Becton Dickinson Vacutainer Systems) by phlebotomy without stasis and immediately analyzed – in sexplicate – for [Hb] (Willoughby et al., 2002), (Model OSM3, Radiometer, Copenhagen, Denmark), and [Hct] (using a Hawksley Microhematocrit centrifuge [Sussex, United Kingdom] and a Micro-capillary reader [Damon/IEC Division, Mass, United States]). The percentage change in plasma volume was analyzed from day 1 to 5 of the acclimation regime, during HSTs and calculated using a mathematical equation (Dill and Costill, 1974).
Aerobic Fitness Testing and Cardiac Output
Participants performed an incremental ramp exercise test to volitional exhaustion on a treadmill (h/p/Cosmos, Model Pulsar 3p, Traunstein, Germany), for determination of peak oxygen uptake () and velocities for individualisation of the HSTs. This procedure involved a starting velocity of 5 km/hr with workload increments of 0.1 km/hr every 6 s (1 km/hr/min), until volitional exhaustion. Breath by breath expired air was collected via a metabolic cart system (Cortex Metalyzer 3B, Cortex Biophysic, Leipzig, Germany). Participants RPE (Borg, 1982) and fc (Polar FS1, Polar Electro, OY, Finland) were recorded every minute. All participants were given verbal encouragement in the latter stages of the incremental test.
Baseline cardiac output was measured using a breath-by-breath cardiac output analyser (Innocor, Innovision, Odense, Denmark). Prior to measurement, calibration of the cardiac output analyser was completed. Each participant had a fresh mouthpiece connected to a bacterial filter (Innovision, Odense, Denmark). A nose clip (Innovision, Odense, Denmark) was then placed over the participant’s nose to prevent any expired air escaping. Participants were instructed to breathe in synchronization (∼5 breaths, ∼15 s) with the on-screen demonstration until measurement was complete.
Heat Stress Test (HST)
The HST took place in an environmental chamber (Type SSR 60-20H, Design Environment, Gwent, United Kingdom) set to ambient temperature of 31°C; 50% RH. Pre-exercise urine and blood measure (%PV) were taken prior to entering the chamber. The HST consisted of 9 × 5 min blocks of intermittent exercise on a treadmill (Pulsar 3p, h/p/Cosmos, Traunstein, Germany) and cycle ergometer (Wattbike Ltd., Nottingham, United Kingdom). Each 5-min block consisted of intermittent treadmill running; standing (recovery), walking (50% HRmax), jogging (60% HRmax), low- (70% HRmax), moderate- (85% HRmax) and high-intensity (95% HRmax), ending with a 6-s maximal cycle ergometer sprint. Treadmill velocity changed every 5–11 s and percentage time spent at each velocity was adapted from collegiate level football match play characteristics (Vescovi and Favero, 2014). Sprint characteristics; peak power output (PPO) and mean power output (MPO) were used as performance measures.
Prior to acclimation, as a control trial, two HST’s (HST1 vs. HST2) were performed and separated by 1 week. The post acclimation HST3 was performed within a week (5 ± 2 days) of the final acclimation day to prevent the decay of acclimation (Garrett et al., 2009) and we acknowledge there may be some variability in the magnitude of response (Waldron et al., 2019). To control for menstrual cycle phase the HST2 (Pre-) and HST3 (Post-) STHA trials were performed in week three of the menstrual cycle (luteal phase), in the active pill portion of the OCP for all participants. Baseline measures of plasma 17β-estradiol were taken prior to HST2 and HST3 trials in the luteal phase of the menstrual cycle and there was no difference (P = 0.87) observed (Table 1).
Body temperature
Core body temperature was measured using a rectal thermistor (U thermistor, Grant Instruments Ltd., Cambridge, United Kingdom) was self-inserted to a depth of 10 cm beyond the anal sphincter. Skin temperature was measured using skin thermistors (Type EUS-U-V5-V2, Grant Instruments Ltd., Cambridge, United Kingdom) placed on four, left sided, sites: chest, bicep, thigh, and calf, secured using micropore tape. Mean skin temperature () (Ramanathan, 1964) and mean body temperature () (Sawka et al., 1996) were measured. Temperature data was recorded at 1-min intervals on a portable data logger (2020 series data logger, Grant Instruments Ltd., Cambridge, United Kingdom).
Data analysis
Sample size was based upon results from the previous limited research on females (Mee et al., 2015; Kirby et al., 2019), using our permissive dehydration protocol during STHA (Garrett et al., 2014). Where a statistical difference was observed in primary outcomes. The stress response of dependent measures in STHA and HSTs were analyzed for normal distribution by using the Shapiro–Wilk and the Brown–Forsythe test determined equal variance. All data were normally distributed and two-way repeated measures ANOVA was used to determine main effects between day 1 and 5 acclimation, pre vs post HSTs and interaction over time for all dependent measures. Pairwise multiple comparison procedures were analyzed using post-hoc Bonferroni correction t-tests when appropriate. The change in thermal markers on day 1 and day 5 of acclimation were analyzed using paired t-test analysis. Work output from day 1 to day 5 of acclimation was analyzed using one-way analysis of variance ANOVA, with repeated measures and Bonferroni correction t-tests to isolate differences between days. Data is reported for ten moderately trained females unless otherwise stated. Where appropriate data is reported as mean differences ± SD with 95% confidence intervals (95% CI) and the magnitude of effect using Cohen’s d effect sizes (where 0.2–0.59 small; 0.6–1.19 moderate; 1.2–1.99 large; 2.0–4.0 very large).
Results
All ten participants completed the 5-day STHA protocol and three HSTs (HST1; HST2; HST3). The HST1 versus HST2 was a control trial taken 1 week apart with no intervention. The HST2 versus HST3 with the STHA intervention took place in week 3 of the menstrual cycle (luteal phase) for all ten participants. Due to issues with venepuncture measures blood parameters were analyzed for eight participants only. Similarly, eight participants had baseline plasma 17β-estradiol measured before HST2 and HST3 trials in the luteal phase.
Acclimation
Thermal Stress and Strain
Thermal stress and strain from days 1 to 5 of heat acclimation are presented in Table 2 and work completed in Figure 2.
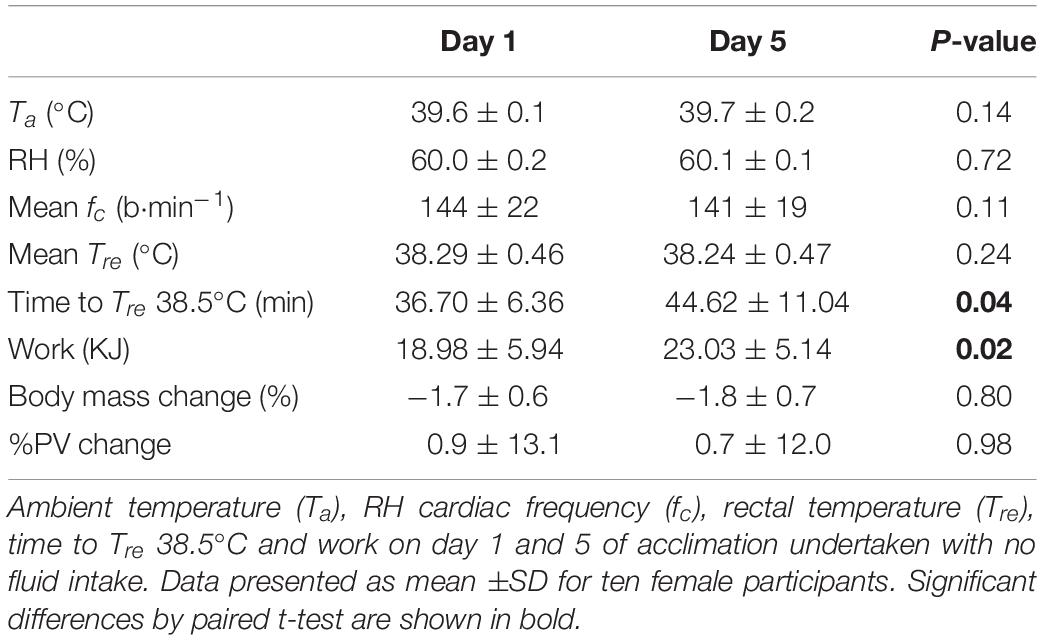
Table 2. Thermal stress and strain on the first (Day 1) and last day (Day 5) of short-term heat acclimation (STHA) for ten moderately trained females.
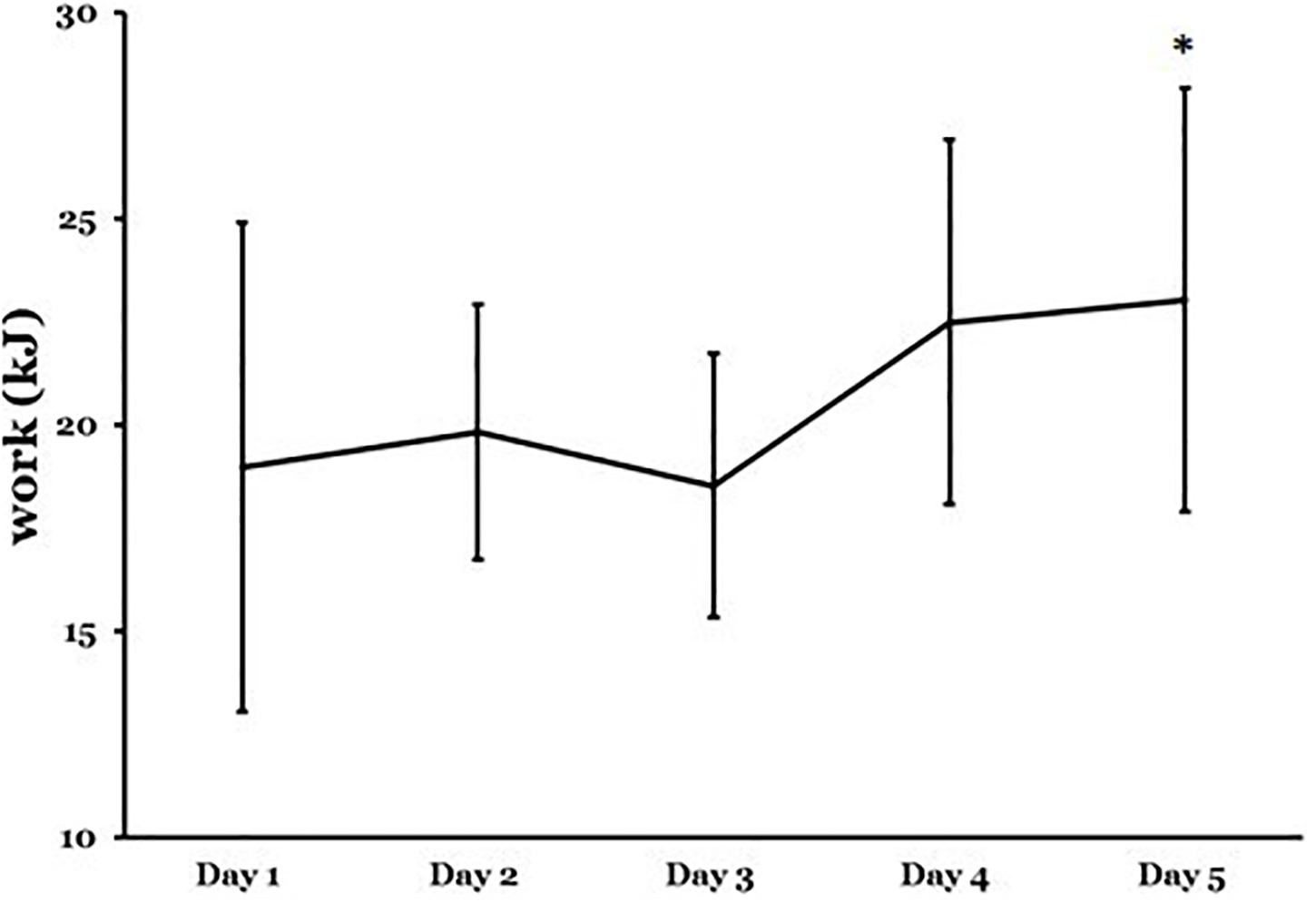
Figure 2. Work output on the first day (Day 1) to the last day (Day 5) of acclimation after 90-min heat exposure. Data are mean ±SD are for ten moderately trained females. Significant difference ∗p < 0.05; Day 1 to the last day of acclimation analyzed using one-way analysis of variance (ANOVA) with repeated measures and Bonferroni correction t-tests to isolate differences between days.
Measures of ambient temperature (Ta) and RH indicated that the thermal stress was similar on day 1 and 5 of acclimation. Similarly, the thermal strain was consistent between days illustrated by mean cardiac frequency (fc) and rectal temperature (Tre) responses. Time to 38.5°C was longer on day 5 cf. day 1 (Table 2; P = 0.04). Therefore, less work was performed on day 1 cf. day 5 (Table 2 and Figure 2; P = 0.02).
Urinary Measures
To determine hydration status urine color (coloru), urine osmolality (osmu), urine specific gravity (SGu) and body mass were measured at rest and 90 min, on day 1 and 5 of acclimation (Table 3). There was no main effect (P > 0.05) and interaction across time (P > 0.05) for coloru, osmu, SGu and body mass on day 1 and 5 of STHA.

Table 3. Urinary measures of hydration (coloru, osmu, SGu) and nude body mass, at rest and end-exercise, on day 1 and 5 of short-term heat acclimation.
Blood Measurements
Blood measures and percentage change on the first day (Day 1) to the last day (Day 5) of acclimation after 90-min heat exposure are presented in Table 4.
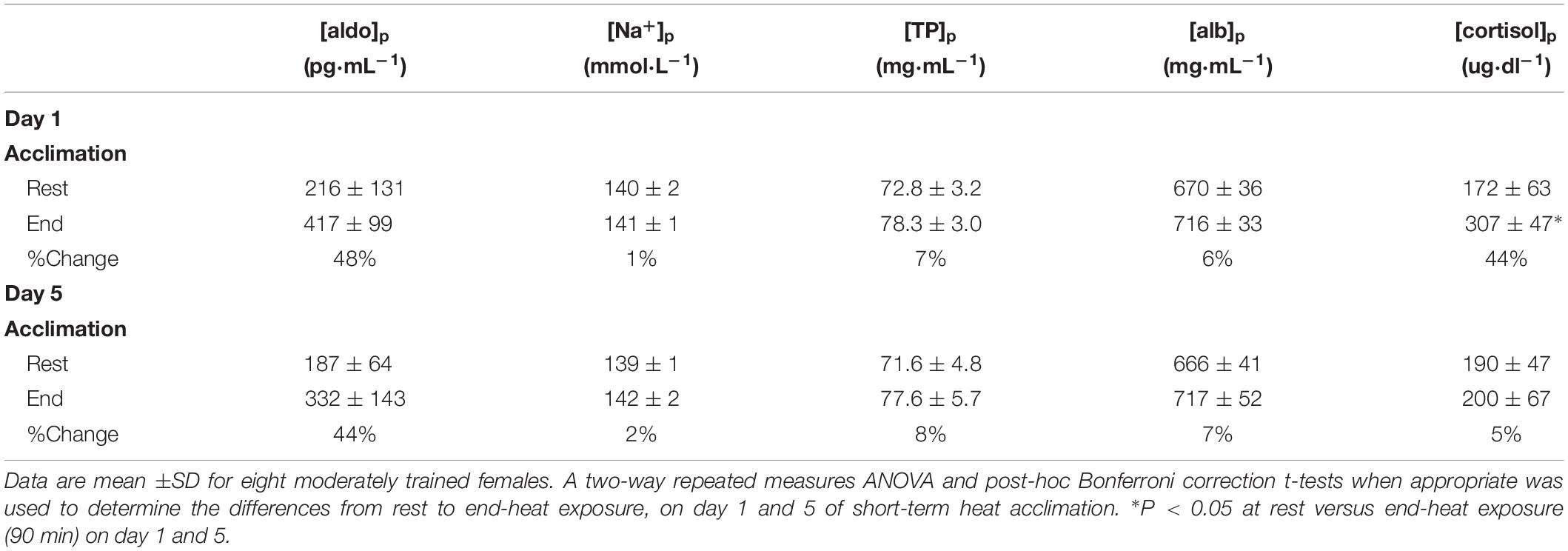
Table 4. Blood measures and percentage change from rest to end-exposure on the first day (Day 1) versus the last day (Day 5) of acclimation after 90-min heat exposure.
There was no main effect for [aldo]p between day 1 and 5 (F = 1.583; P = 0.25) or interaction across time (F = 0.755; P = 0.41). Similarly, there was no main effect between day 1 and 5 for [Na+]p (F = 1.106; P = 0.32) or interaction across time (F = 0.983; P = 0.36). The measure [TP]p demonstrated no main effect between day 1 and 5 (F = 0.93; P = 0.37) or interaction across time (F = 0.198; P = 0.67). There was no main effect between day 1 and 5 (F = 0.0618; P = 0.81) for [alb]p or interaction across time (F = 0.50; P = 0.50). In contrast, there was a significant main effect between day 1 and 5 for [cortisol]p (F = 15.303; P = 0.01), and interaction across time (F = 10.775; P = 0.02). Bonferroni-corrected post-hoc comparisons showed a significant difference between pre and post measures within day 1 (P = 0.002) but not on day 5 (P = 0.57).
Heat Stress Test
Measurements were taken at rest and across the 45 min in HSTs. Data is presented for ten female participants unless otherwise stated.
Control Study
The HST1 versus HST2 was a control trial taken 1 week apart with no intervention. There was a limited change for Tre, , , fc, and %PV (P > 0.05). Similarly, in the sprint performance test the PPO and MPO demonstrated limited change (P > 0.05).
Intervention Study
The HST2 trial took place 1 week before the STHA (5-days), with no fluid intake intervention. The post HST3 occurred within 7-days of the last acclimation.
Body temperatures
Figure 3 presents mean ± SD rectal temperature (Tre), mean skin temperature () and mean body temperature (), pre- to post acclimation in hot conditions (31°C; 50% RH; n = 10).
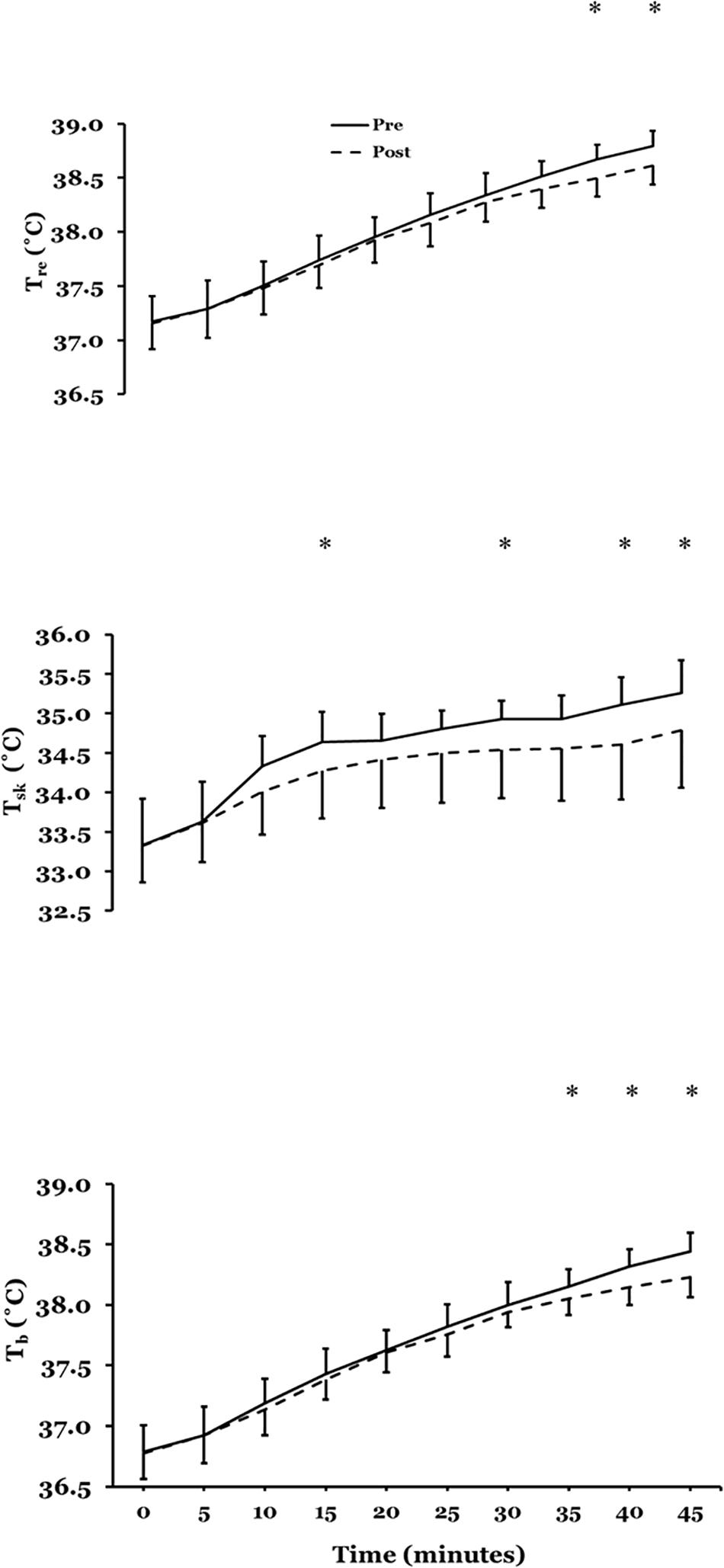
Figure 3. Mean ± SD rectal temperature (Tre) (upper plate), mean skin temperature () (mid plate) and mean body temperature () (lower plate), pre- to post acclimation in hot conditions (31°C; 50% RH; n = 10). ∗P < 0.05 post-hoc Bonferroni correction t-tests.
There was no main effect for Tre(F = 1.411; P = 0.27) after STHA but there was a significant interaction across time (F = 2.991; P = 0.004). Bonferroni-corrected post-hoc comparisons showed a significant mean difference at 40 (P = 0.01) and 45 min (P = 0.007). At 45 min Tre reduced by 0.20°C (95%CI −0.30 to −0.10°C; d = 0.77: Moderate). There was a significant main effect for (F = 5.252; P = 0.05) after STHA and interaction across time (F = 4.689; P = 0.001). Bonferroni-corrected post-hoc comparisons showed a significant mean difference at 15 (P = 0.043), 30 (P = 0.03); 35 (P = 0.004); 40 (P = 0.009) and 45 min (P = 0.009). The reduction in at 45 min was −0.50 (−0.90 to −0.10°C; d = 0.80: Moderate). There was no main effect for (F = 4.419; P = 0.07) after STHA but there was a significant interaction across time (F = 3.942; P = 0.001). Bonferroni-corrected post-hoc comparisons showed a significant mean difference at 35 (P = 0.01), 40 (P = 0.002) and 45 min (P = 0.001). At 45 min reduced by −0.25 (−0.35 to −0.15°C; d = 0.92: Moderate).
Cardiac frequency and percentage change in plasma volume (%PV)
There was a significant main effect for cardiac frequency (F = 7.702; P = 0.02) after STHA and interaction across time (F = 2.485; P = 0.02). Bonferroni-corrected post-hoc comparisons showed a significant mean difference at rest (P = 0.001), 5 (P = 0.04) and 45 min (P = 0.003). Cardiac frequency reduced at rest (−13; −18 to −7 b⋅min–1; d = 1.04: Moderate) and at 45-min (−8; −16 to −1 b⋅min–1; d = 1.11: Moderate). There was an increase in %PV from baseline post STHA by 7.0% (−0.4 to 14.5%, d = 1.27: Large).
Psychophysiological
There was a significant main effect for thermal comfort (F = 27.156; P = 0.001) after STHA and interaction across time (F = 3.378; P = 0.001). Bonferroni-corrected post-hoc comparisons showed a significant mean difference from 10 to 45 min (P < 0.05). Thermal comfort reduced at 45 min by −1 (−1.5 to −0.5 units; d = 0.89: Moderate). There was a significant main effect for thermal sensation (F = 19.462; P = 0.002) after STHA and interaction across time (F = 4.533; P = 0.001). Bonferroni-corrected post-hoc comparisons showed a significant mean difference at 0 to 10 and 20 to 45 min (P < 0.05). Thermal sensation reduced at 45 min by −1 (−1.5 to −0.0 units; d = 0.67: Moderate). There was a significant main effect for RPE (F = 5.831; P = 0.04) after STHA and interaction effect across time (F = 2.853; P = 0.006). Bonferroni-corrected post-hoc comparisons showed a significant mean difference 20 (P = 0.04), 25 (P = 0.04) 30 (P = 0.02), 40 (P = 0.01) and 45 min (P = 0.01). RPE decreased at 45 min by −2 (−4 to 0 units; d = 0.70: Moderate).
Repeated sprint performance
The PPO and MPO were measured across all nine, 6-s maximal sprints in the 45-min protocol (Figure 4).
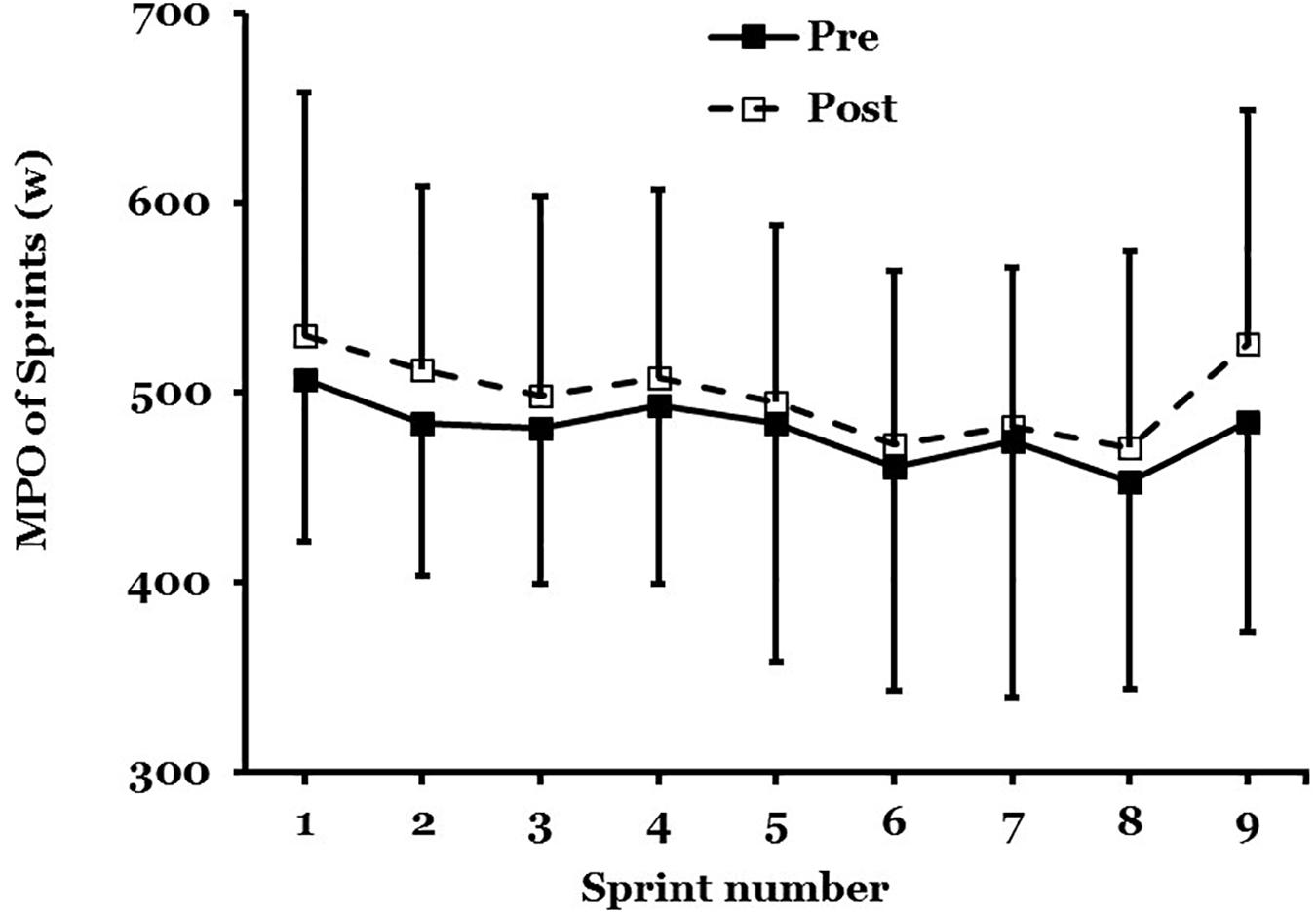
Figure 4. Mean ± SD of mean power output (MPO) in maximal sprint performance, pre- to post acclimation in hot conditions (31°C; 50% RH; n = 9).
There was no main effect for PPO (F = 1.458; P = 0.26) after STHA or significant interaction effect across all nine sprints (F = 0.397; P = 0.91). There was not a main effect for MPO (F = 3.064; P = 0.12) after STHA but the interaction across all nine sprints was close to significance (F = 0.296; P = 0.06). The MPO increased across all nine maximal sprints by 56W (−26 to 139W; d = 0.69: Moderate; n = 9).
Discussion
Effectiveness of Short-Term Heat Acclimation
The adaptations from short-term (5-d) heat acclimation with no fluid intake during acclimation, using the controlled hyperthermia technique, reduced exercising cardiovascular strain in females controlling for menstrual cycle phase. The cardiovascular stability was due to increased heat loss rather than lower heat content (∼resting core temperature), at the time of day of testing HSTs. This concurs with our previous work that has used no fluid intake during acclimation but with moderately (Garrett et al., 2009) and highly trained males (Garrett et al., 2012). However, it is in contrast with the limited work on STHA with females, using controlled-hyperthermia with permissive dehydration (Mee et al., 2015; Kirby et al., 2019).
Adaptation to Exercise in the Heat and Menstrual Cycle Phase
The post acclimation HST3 was performed within a week of the final acclimation day to prevent the decay of acclimation (Garrett et al., 2009). It has long been recognized that the menstrual cycle plays a significant role in athletic performance (Avellini et al., 1979; Tenaglia et al., 1999; Janse de Jonge et al., 2012). Therefore, to control for menstrual cycle phase the HST2 (Pre-) and HST3 (Post-) STHA trials were performed in week 3 of menstrual cycle (luteal phase), with all participants using oral contraceptive pills (combined). This was determined by menstrual cycle questionnaire and baseline measures of plasma 17β-estradiol. This was measured prior to HST2 and HST3 intervention trials and there was no statistical difference observed (Table 1). It has previously been reported that heat adaptation in females is not affected by menstrual cycle phase (Lei et al., 2017; Lei and Mundel, 2018) or the use of oral contraceptive pill (Armstrong et al., 2005). However, menstrual cycle phase and the associated changes in female sex hormones can influence core temperature (Inoue et al., 2005), the overall thermoregulatory set point range (Charkoudian and Stachenfeld, 2016) but a limited effect on whole body heat loss has been reported (Notley et al., 2018).
The present results using a female cohort and controlling for menstrual cycle phase, undergoing STHA of daily controlled hyperthermia with no fluid intake, demonstrated that the participants experienced adaptation to the heat. This was indicated by the characteristic features of acclimation. A decrease in Tre by −0.2°C (Figure 3; top panel), by −0.5°C (Figure 3; mid panel) and by −0.25 (Figure 3; lower panel) was observed. Similar body temperature measures have previously been reported by the author, using the hyperthermia-control technique but with male participants (Garrett et al., 2009, 2012, 2014). In contrast, the research group of Mee et al. (2015) reported that the employment of the controlled hyperthermia model with permissive dehydration successfully attenuated Tre during a 30-min run in the heat after 5 days in males (−0.39 ± 0.36°C) but not in females (−0.07 ± 0.18°C). Yet after a further 5 days of acclimation the females Tre response was similar by 0.48 ± 0.27°C (Mee et al., 2015). This indicates that a female population requires a longer-term intervention than the 5-days STHA we employed (Mee et al., 2015). Similarly, Kirby et al. (2019) determined nine-, but not 4-days heat acclimation improves self-paced endurance performance in females, using hyperthermia control with permissive dehydration (Kirby et al., 2019). However, this was over a shorter 4-day STHA. Furthermore, in these two studies there were method differences with the present work. The exercise protocols were short in duration (∼30 min), self-paced/fixed speed trials and importantly menstrual OCP phase was not controlled.
End-exercise fc decreased by 8 b⋅min–1. Increased cardiovascular stability is recognized as one of the most rapidly occurring adaptations to the heat (Garrett et al., 2009, 2011). Furthermore, a 7.0% PV expansion from baseline was observed in this study. Previous research suggests intravascular fluid expansion resulting from such increases in colloid-osmotic pressure (Senay et al., 1976; Senay, 1979) explains a majority of PV expansion (Goto et al., 2010). Therefore, this suggests that the PV expansion may have contributed to the greater cardiovascular stability that was observed. This is similar to the findings of Pethick et al. (2018) who demonstrated induced plasma volume expansion in a female cohort following 5-days high-intensity heat acclimation (Pethick et al., 2018).
Repeated Sprint Performance
This study demonstrated that an increase in MPO was close to significance across all nine maximal sprints after STHA of 5-days (Figure 4). This improvement in intermittent performance is supported by Sunderland et al. (2008) who developed a heat acclimation protocol (4-days) for female team sports (Sunderland et al., 2008). They reported a reduced rate of rise in rectal temperature and a 33% improvement in distance run during a repeated shuttle run performance test after STHA in a female cohort. From a practical perspective, an improvement in sprint performance is a valuable asset in team sport situations. Work-rate during team sports matches are largely determined by the oppositions playing style of the opposing team and individuals (Ozgunen et al., 2010), the ability to maintain repeated sprint performance can determine when a games player gets to the ball first and outrun the opposition.
Fluid Regulation Response to Repeated Heat Stress
In the present study, participants experienced the same thermal load and this is the basis of using the controlled hyperthermia technique for heat acclimation. Individual’s experienced a mild hypohydration of ∼2% body mass (Table 2). This is similar to the imposed hypohydration administered by Judelson and colleagues, who reported a modification in the hormonal and metabolic response to resistance exercise, influencing the post-exercise circulatory milieu (Judelson et al., 2008). The research design of this study is supported by recommendations from earlier work with females on sex hormones and fluid regulation by the Stachenfeld research group (Stachenfeld et al., 1999; Stachenfeld, 2008).
Fluid Regulatory Hormones, Electrolytes and Plasma Volume Expansion
In the present study, after 90-min exercise [aldo]p did not significantly increase across acclimation bouts (Table 4) and this is in contrast to what has previously been reported (Judelson et al., 2008). The principal effects of aldosterone are the retention of Na+ and therefore water from the urine output to maintain extracellular fluid volume and thus blood volume. However, in the present study, an exercise-induced response of increased [Na+]p was not clearly evident after the no fluid intake acclimation regime (Table 4). Therefore, this is in contrast with previous findings (Brandenberger et al., 1989; Francesconi et al., 1993; Allsopp et al., 1998) who reported a strong relationship between increased Na+ with [aldo]p response. In the present study, using the ΔPV (Dill and Costill, 1974) technique, there was an acclimation induced increase in resting %PV across HSTs by 7.0 ± 6% in the present study. This is similar to Pethick et al. (2018) who successfully induced plasma volume expansion in a female cohort, following 5-days high-intensity heat acclimation (Pethick et al., 2018).
Stress Hormone Response
In the present study the time to reach 38.5°C significantly increased (21.6%) from day 1 to 5 resulting in an associated increase in work (21.3%) (Table 2). Mean time to reach 38.5°C has been shown to be longer during STHA, using the controlled hyperthermia technique for females (51 ± 7 min), in comparison with males (48 ± 9 min) (Mee et al., 2015). Similarly, in the present study, a much larger % difference in time to 38.5°C was observed in comparison with previous studies using male cohorts with the same protocol (Garrett et al., 2009, 2012, 2014; Neal et al., 2015). The stress hormone, cortisol, significantly increased during acclimation on day 1 but this response was not observed on day 5. Despite a greater time to 38.5°C and more work being completed, hence, indicating a heat adaptive response (Table 4). This agrees with previous observations on male cohorts suggesting heat acclimation reduces cortisol levels during exercise in the heat (Francesconi et al., 1983; Armstrong et al., 1989) but such findings are not universal (Finberg and Berlyne, 1977; Sunderland et al., 2008).
Limitations and Future Directions
In order to standardize menstrual cycle phase each female participant used a monophasic, oral contraceptive pill (OCP) but a potential limitation was that the dose of hormone concentration differed between individuals depending on their specific medication.
For future directions, information is limited on the physiological mechanisms of fluid regulation in females, following STHA. Therefore, a comparison of euhydration versus dehydration STHA, may provide a greater understanding of this area. To the authors knowledge, our earlier work (Garrett et al., 2014) is the only study to have done this but with male participants.
Conclusion
In summary, this work has established the effectiveness of STHA for 5 days, using the controlled-hyperthermia technique with no fluid intake (Garrett et al., 2009, 2012, 2014), on intermittent activity in hot environments with a female cohort, controlling for menstrual cycle phase. The current research suggests these methods of heat acclimation in a female cohort enhances thermoregulation and cardiovascular stability during intermittent exercise in the heat. These improvements may provide protection from exertional heat related illnesses associated with exercise performance. This work adds to the limited body of literature available and this is particularly important given the 2020 Olympics will be held in the hot and humid conditions of Tokyo in Japan.
Data Availability Statement
The datasets generated for this study are available on request to the corresponding author.
Ethics Statement
The studies involving human participants were reviewed and approved by the University of Hull Ethics Committee. The patients/participants provided their written informed consent to participate in this study.
Author Contributions
AG conceived and designed the research. JG, ED, VB, and JS conducted the experiments. JB, HJ, DG-S, and RB contributed to the blood handling and analysis. AG and GA analyzed the data. AG and JG wrote the manuscript. All authors read and approved the manuscript.
Funding
This work was supported by the João Havelange Research Scholarship (FIFA) and Sport, Health and Exercise Science at the University of Hull, United Kingdom.
Conflict of Interest
The authors declare that the research was conducted in the absence of any commercial or financial relationships that could be construed as a potential conflict of interest.
Acknowledgments
Special thanks to the participants in this study and the technical expertise provided.
Abbreviations
fc, cardiac frequency (b ⋅ min–1); , cardiac output (L ⋅ min–1); HST, heat stress test; , maximum oxygen uptake (L ⋅ min–1or mL ⋅ kg–1 ⋅ min–1); MPO, mean power output (W); , mean body temperature (°C); , mean skin temperature (°C); , oxygen consumption (L ⋅ min–1 or mL ⋅ kg–1 ⋅ min–1); , peak oxygen uptake (L ⋅ min–1or mL ⋅ kg–1 ⋅ min–1); PPO, peak power output (W); %PV, percentage plasma volume (%); [alb]p, plasma albumin (mg ⋅ mL–1); [aldo]p, plasma aldosterone (pg ⋅ mL–1); [cortisol]p, plasma cortisol (ug ⋅ dl–1); [Na+]p, plasma sodium (mmol ⋅ L–1); TPp, plasma total protein (mg ⋅ mL–1); Tre, rectal temperature (°C); RH, relative humidity (%); STHA, short-term heat acclimation; coloru, urine color (units); osmu, urine osmolality (mOsm/kg); SGu, urine specific gravity (units).
References
Akerman, A. P., Tipton, M., Minson, C. T., and Cotter, J. D. (2016). Heat stress and dehydration in adapting for performance: good, bad, both, or neither? Temperature 3, 412–436. doi: 10.1080/23328940.2016.1216255
Allsopp, A. J., Sutherland, R., Wood, P., and Wooton, S. A. (1998). The effect of sodium balance on sweat sodium secretion and plasma aldosterone concentration. Eur. J. Appl. Physiol. 78, 516–521. doi: 10.1007/s004210050454
Armstrong, L. E., Francesconi, R. P., Kraemer, W. J., Leva, N., De Luca, J. P., and Hubbard, R. W. (1989). Plasma cortisol, renin, and aldosterone during an intense heat acclimation program. Int. J. Sports Med. 10, 38–42. doi: 10.1055/s-2007-1024871
Armstrong, L. E., Herrera Soto, J. A., Hacker, F. T., Casa, D. J., Kavouras, S. A., and Maresh, C. M. (1998). Urinary indices during dehydration, exercise, and rehydration. Int. J. Sport Nutr. 8, 345–355. doi: 10.1123/ijsn.8.4.345
Armstrong, L. E., Maresh, C. M., Castellani, J. W., Bergeron, M. F., Kenefick, R. W., LaGasse, K. E., et al. (1994). Urinary indices of hydration status. Int. J. Sport Nutr. 4, 265–279. doi: 10.1123/ijsn.4.3.265
Armstrong, L. E., Maresh, C. M., Keith, N. R., Elliott, T. A., Van Heest, J. L., Scheet, T. P., et al. (2005). Heat acclimation and physical training adaptations of young women using different contraceptive pills. Am. J. Physiol. Endocrinol. Metab. 288, E868–E875.
Avellini, B. A., Kamon, E., and Krajewski, J. T. (1979). Physiological responses of physically fit men and women to acclimation to humid heat. DTIC Doc. 49, 254–261. doi: 10.1152/jappl.1980.49.2.254
Borg, G. A. (1982). Psychophysical bases of perceived exertion. Med. Sci. Sports Exerc. 14, 377–381.
Brandenberger, G., Candas, V., Follenius, M., and Kahn, J. M. (1989). The influence of initial state of hydration on endocrine responses to exercise in the heat. Eur. J. Appl. Physiol. 58, 674–679. doi: 10.1007/bf00418516
Bruinvels, G., Burden, R., Brown, N., Richards, T., and Pedlar, C. (2016). The Prevalence and Impact of Heavy Menstrual Bleeding (Menorrhagia) in Elite and Non-Elite Athletes. PLoS One 11:e0149881. doi: 10.1371/journal.pone.0149881
Charkoudian, N., and Johnson, J. M. (1997). Modification of active cutaneous vasodilation by oral contraceptive hormones. J. Appl. Physiol. 83, 2012–2018. doi: 10.1152/jappl.1997.83.6.2012
Charkoudian, N., and Stachenfeld, N. (2016). Sex hormone effects on autonomic mechanisms of thermoregulation in humans. Auton. Neurosci. 196, 75–80. doi: 10.1016/j.autneu.2015.11.004
Constantini, N. W., Dubnov, G., and Lebrun, C. M. (2005). The menstrual cycle and sport performance. Clin. Sports Med. 24:e51-82.
Daanen, H. A., and Herweijer, J. A. (2015). effectiveness of an indoor preparation program to increase thermal resilience in elderly for heat waves. Build. Environ. 83, 115–119. doi: 10.1016/j.buildenv.2014.04.010
Dill, D. B., and Costill, D. L. (1974). Calculation of percentage changes in volumes of blood, plasma, and red cells in dehydration. J. Appl. Physiol. 37, 247–248. doi: 10.1152/jappl.1974.37.2.247
Finberg, J. P., and Berlyne, G. M. (1977). Modification of renin and aldosterone response to heat by acclimatization in man. J. Appl. Physiol. Respir. Environ. Exerc. Physiol. 42, 554–558. doi: 10.1152/jappl.1977.42.4.554
Francesconi, R. N., Amstrong, L. E., Leva, N. M., Moore, R. J., Szlyk, P. C., Matthew, W. T., et al. (1993). Endocrinological Responses to Dietary Salt Restriction During Heat Acclimation. Nutritional Needs in Hot Environments. Washington, D.C: National Academy Press, 259–275.
Francesconi, R. P., Sawka, M. N., and Pandolf, K. B. (1983). Hypohydration and heat acclimation: plasma renin and aldosterone during exercise. J. Appl. Physiol. Respir. Environ. Exerc. Physiol. 55, 1790–1794. doi: 10.1152/jappl.1983.55.6.1790
Garrett, A. T., Creasy, R., Rehrer, N. J., Patterson, M. J., and Cotter, J. D. (2012). Effectiveness of short-term heat acclimation for highly trained athletes. Eur. J. Appl. Physiol. 112, 1827–1837. doi: 10.1007/s00421-011-2153-3
Garrett, A. T., Goosens, N. G., Rehrer, N. J., Patterson, M. J., and Cotter, J. D. (2009). Induction and decay of short-term heat acclimation. Eur. J. Appl. Physiol. 107, 659–670. doi: 10.1007/s00421-009-1182-7
Garrett, A. T., Goosens, N. G., Rehrer, N. J., Patterson, M. J., Harrison, J., Sammut, I., et al. (2014). Short-term heat acclimation is effective and may be enhanced rather than impaired by dehydration. Am. J. Hum. Biol. 26, 311–320. doi: 10.1002/ajhb.22509
Garrett, A. T., Rehrer, N. J., and Patterson, M. J. (2011). Induction and decay of short-term heat acclimation in moderately and highly trained athletes. Sports Med. 41, 757–771. doi: 10.2165/11587320-000000000-00000
Gerrett, N., Kingma, B. R. M., Sluijter, R., and Daanen, H. A. M. (2019). Ambient conditions prior to Tokyo 2020 olympic and paralympic games: considerations for acclimation or acclimatization strategies. Front. Physiol. 10:414. doi: 10.3389/fphys.2019.00414
Gibson, O. R., Mee, J. A., Tuttle, J. A., Taylor, L., Watt, P. W., and Maxwell, N. S. (2015). Isothermic and fixed intensity heat acclimation methods induce similar heat adaptation following short and long-term timescales. J. Therm. Biol. 4, 55–65. doi: 10.1016/j.jtherbio.2015.02.005
Goto, M., Okazaki, K., Kamijo, Y., Ikegawa, S., Masuki, S., Miyagawa, K., et al. (2010). Protein and carbohydrate supplementation during 5-day aerobic training enhanced plasma volume expansion and thermoregulatory adaptation in young men. J. Appl. Physiol. 109, 1247–1255. doi: 10.1152/japplphysiol.00577.2010
Grucza, R., Pekkarinen, H., Titov, E. K., Kononoff, A., and Hänninen, O. (1993). Influence of the menstrual cycle and oral contraceptives on thermoregulatory responses to exercise in young women. Eur. J. Appl. Physiol. Occup. Physiol. 67, 279–285. doi: 10.1007/bf00864229
Hessemer, V., Zeh, A., and Brück, K. (1986). Effects of passive heat adaptation and moderate sweatless conditioning on responses to cold and heat. Eur. J. Appl. Physiol. Occup. Physiol. 55, 281–289. doi: 10.1007/bf02343800
Inoue, Y., Tanaka, Y., Omori, K., Kuwahara, T., Ogura, Y., and Ueda, H. (2005). Sex-and menstrual cycle-related differences in sweating and cutaneous blood flow in response to passive heat exposure. Eur. J. Appl. Physiol. 94, 232–332.
Janse de Jonge, X. A., Thompson, M. W., Chuter, V. H., Silk, L. N., and Thom, J. M. (2012). Exercise performance over the menstrual cycle in temperate and hot, humid conditions. Med. Sci. Sports Exerc. 44, 2190–2198. doi: 10.1249/MSS.0b013e3182656f13
Judelson, D. A., Maresh, C. M., Yamamoto, L. M., Farrell, M. J., Armstrong, L. E., Kraemer, W. J., et al. (2008). Effect of hydration state on resistance exercise-induced endocrine markers of anabolism, catabolism, and metabolism. J. Appl. Physiol. 105, 816–824. doi: 10.1152/japplphysiol.01010.2007
Kampmann, B., Brode, P., Schutte, M., and Griefahn, B. (2008). Lowering of resting core temperature during acclimation is influenced by exercise stimulus. Eur. J. Appl. Physiol. 104, 321–327. doi: 10.1007/s00421-007-0658-6
Kirby, N. V., Lucas, S. J. E., and Lucas, R. A. I. (2019). Nine-, but not four-days heat acclimation improves self-paced endurance performance in females. Front. Physiol. 10:539. doi: 10.3389/fphys.2019.00539
Kolka, M. A., and Stephenson, L. A. (1989). Control of sweating during the human menstrual cycle. Eur. J. Appl. Physiol. Occup. Physiol. 58, 890–895. doi: 10.1007/bf02332224
Kolka, M. A., and Stephenson, L. A. (1997). Interaction of menstrual cycle phase, clothing resistance and exercise on thermoregulation in women. J. Therm. Biol. 22, 137–141. doi: 10.1016/s0306-4565(97)00003-x
Lebrun, C. M., McKenzie, D. C., Prior, J. C., and Taunton, J. E. (1995). Effects of menstrual cycle phase on athletic performance. Med. Sci. Sports Exerc. 27, 437–444.
Lei, T. H., and Mundel, T. (2018). Humid heat stress affects trained female athletes more than does their menstrual phase. Temp. Vol. 5, 202–204. doi: 10.1080/23328940.2018.1436394
Lei, T.-H., Stannard, S. R., Perry, B. G., Schlader, Z. J., Cotter, J. D., and Mu, T. (2017). Influence of menstrual phase and arid vs. humid heat stress on autonomic and behavioural thermoregulation during exercise in trained but unacclimated women. J. Physiol. 595, 2823–2837. doi: 10.1113/JP273176
Mee, J. A., Gibson, O. R., Doust, J., and Maxwell, N. S. (2015). A comparison of males and females’ temporal patterning to short- and long-term heat acclimation. Scand. J. Med. Sci. Sports 25(Suppl. 1), 250–258. doi: 10.1111/sms.12417
Neal, R. A., Corbett, J., Massey, H. C., and Tipton, M. J. (2015). Effect of short-term heat acclimation with permissive dehydration on thermoregulation and temperate exercise performance. Scand. J. Med. Sci. Sports 26, 875–884. doi: 10.1111/sms.12526
Neal, R. A., Massey, H. C., Tipton, M. J., Young, J. S., and Corbett, J. (2016). Effect of permissive dehydration on induction and decay of heat acclimation, and temperate exercise performance. Front. Physiol. 7:564. doi: 10.3389/fphys.2016.00564
Notley, S. R., Dervis, S. M., Poirier, M. P., and Kenny, G. P. (2018). Menstrual cycle phase does not modulate whole-body heat loss during exercise in hot dry conditions. J. Appl. Physiol. 126, 286–293. doi: 10.1152/japplphysiol.00735.2018
Ozgunen, K. T., Kurdak, S. S., Maughan, R. J., Zeren, C., Korkmaz, S., Yazici, Z., et al. (2010). Effect of hot environmental conditions on physical activity patterns and temperature response of football players. Scand. J. Med. Sci. Sports 20(Suppl. 3), 140–147. doi: 10.1111/j.1600-0838.2010.01219.x
Pethick, W. A., Stellingwerff, T., Lacroix, M. A., Bergstrom, C., and Meylan, C. M. (2018). The effect of a team sport-specific heat acclimation protocol on plasma volume in elite female soccer players. Sci. Med. Footb. 2, 16–22. doi: 10.1080/24733938.2017.1384559
Ramanathan, N. L. (1964). A New weighting system for mean surface temperature of the human body. J. Appl. Physiol. 19, 531–533. doi: 10.1152/jappl.1964.19.3.531
Rogers, S. M., and Baker, M. A. (1997). Thermoregulation during exercise in women who are taking oral contraceptives. Eur. J. Appl. Physiol. Occup. Physiol. 75, 34–38. doi: 10.1007/s004210050123
Sawka, M. N., Wenger, C. B., and Pandolf, K. B. (1996). “Thermoregulatory responses to acute exercise–heat stress and heat acclimation,” in Handbook of Physiology, Section 4: Environmental Physiology, eds M. J. Fregly, and C. M. Blatteis, (New York, NY: Oxford University Press), 157–185.
Senay, L. C. (1979). Effects of exercise in the heat on body fluid distribution. Med. Sci. Sports 11, 42–48.
Senay, L. C., Mitchell, D., and Wyndham, C. H. (1976). Acclimatization in a hot, humid environment: body fluid adjustments. J. Appl. Physiol. 40, 786–796. doi: 10.1152/jappl.1976.40.5.786
Stachenfeld, N. S. (2008). Sex hormone effects on body fluid regulation. Exerc. Sport Sci. Rev. 36, 152–159. doi: 10.1097/JES.0b013e31817be928
Stachenfeld, N. S., Silva, C., and Keefe, D. L. (2000). Estrogen modifies the temperature effects of progesterone. J. Appl. Physiol. 88, 1643–1649. doi: 10.1152/jappl.2000.88.5.1643
Stachenfeld, N. S., Silva, C., Keefe, D. L., Kokoszka, C. A., and Nadel, E. R. (1999). Effects of oral contraceptives on body fluid regulation. J. Appl. Physiol. 87, 1016–1025. doi: 10.1152/jappl.1999.87.3.1016
Stephenson, L. A., and Kolka, M. A. (1985). Menstrual cycle phase and time of day alter reference signal controlling arm blood flow and sweating. Am. J. Physiol. 249(2 Pt 2), R186–R191.
Sunderland, C., Morris, J. G., and Nevill, M. E. (2008). A heat acclimation protocol for team sports. Br. J. Sports Med. 42, 327–333. doi: 10.1136/bjsm.2007.034207
Sunderland, C., and Nevill, M. (2003). Effect of the menstrual cycle on performance of intermittent, high-intensity shuttle running in a hot environment. Eur. J. Appl. Physiol. 88, 345–352. doi: 10.1007/s00421-002-0722-1
Taylor, N. A. S., and Cotter, J. D. (2006). Heat adaptation: guidelines for the optimisation of human performance. Int. Sport Med. J. 7, 33–57.
Tenaglia, S. A., McLellan, T. M., and Klentrou, P. P. (1999). Influence of menstrual cycle and oral contraceptives on tolerance to uncompensable heat stress. Eur. J. Appl. Physiol. Occup. Physiol. 80, 76–83. doi: 10.1007/s004210050561
Tounsi, M., Jaafar, H., Aloui, A., and Souissi, N. (2017). Soccer-related performance in eumenorrheic Tunisian high-level soccer players: effects of menstrual cycle phase and moment of day. J. Sports Med. Phys. Fitness 58, 497–502. doi: 10.23736/S0022-4707.17.06958-4
Vescovi, J. D., and Favero, T. G. (2014). Motion characteristics of women’s college soccer matches: Female Athletes in Motion (FAiM) study. Int. J. Sports Physiol. Perform. 9, 405–414. doi: 10.1123/IJSPP.2013-0526
Waldron, M., Jeffries, O., Tallent, J., Patterson, S., and Nevola, V. (2019). The time course of adaptations in thermoneutral maximal oxygen consumption following heat acclimation. Eur. J. Appl. Physiol. 119, 2391–2399. doi: 10.1007/s00421-019-04218-2
Wiecek, M., Szymura, J., Maciejczyk, M., Cempla, J., and Szygula, Z. (2016). Effect of sex and menstrual cycle in women on starting speed, anaerobic endurance and muscle power. Physiol. Int. 103, 127–132. doi: 10.1556/036.103.2016.1.13
Willoughby, D. S., Priest, J. W., and Nelson, M. (2002). Expression of the stress proteins, Ubiquitin, heat shock protein 72, and Myofibrillar protein content after 12 weeks of leg cycling in persons with spinal cord injury. Arch. Phys. Med. Rehabil. 83, 649–654. doi: 10.1053/apmr.2002.31184
Keywords: female, menstrual cycle, dehydration, fluid-regulation, plasma volume
Citation: Garrett AT, Dodd E, Biddlecombe V, Gleadall-Siddall D, Burke R, Shaw J, Bray J, Jones H, Abt G and Gritt J (2019) Effectiveness of Short-Term Heat Acclimation on Intermittent Sprint Performance With Moderately Trained Females Controlling for Menstrual Cycle Phase. Front. Physiol. 10:1458. doi: 10.3389/fphys.2019.01458
Received: 19 July 2019; Accepted: 12 November 2019;
Published: 29 November 2019.
Edited by:
François Billaut, Laval University, CanadaReviewed by:
Oliver R. Gibson, Brunel University London, United KingdomToby Mündel, Massey University, New Zealand
Copyright © 2019 Garrett, Dodd, Biddlecombe, Gleadall-Siddall, Burke, Shaw, Bray, Jones, Abt and Gritt. This is an open-access article distributed under the terms of the Creative Commons Attribution License (CC BY). The use, distribution or reproduction in other forums is permitted, provided the original author(s) and the copyright owner(s) are credited and that the original publication in this journal is cited, in accordance with accepted academic practice. No use, distribution or reproduction is permitted which does not comply with these terms.
*Correspondence: Andrew T. Garrett, a.garrett@hull.ac.uk