- 1Department of Exercise Physiology, Faculty of Physical Education and Sport Sciences, Kharazmi University, Tehran, Iran
- 2College of Health and Human Sciences, Charles Darwin University, Darwin, NT, Australia
- 3Faculty of Health and Sport Science, Eastern Institute of Technology, Napier, New Zealand
- 4Department of Exercise Physiology, Sports Medicine Research Center, Sport Sciences Research Institute, Tehran, Iran
- 5Institute of Primary Care, University of Zurich, Zurich, Switzerland
- 6Exercise Physiology Laboratory, Nikaia, Greece
- 7Medbase St. Gallen Am Vadianplatz, St. Gallen, Switzerland
The purpose of this study was to compare the effects of different combinations of blood flow restriction (BFR) pressure and exercise intensity on aerobic, anaerobic, and muscle strength adaptations in physically active collegiate women. Thirty-two women (age 22.8 ± 2.9 years; body mass index 22.3 ± 2.7 kg/m2) were randomly assigned into four experimental training groups: (a) increasing BFR pressure with constant exercise intensity (IP-CE), (b) constant partial BFR pressure with increasing exercise intensity (CPp-IE), (c) constant complete BFR pressure with increasing exercise intensity (CPC-IE), and (d) increasing BFR pressure with increasing exercise intensity (IP-IE). The participants completed 12 training sessions comprised of repeated bouts of 2 min running on a treadmill with BFR interspersed by 1-min recovery without BFR. Participants completed a series of tests to assess muscle strength, aerobic, and anaerobic performances. Muscle strength, anaerobic power, and aerobic parameters including maximum oxygen consumption (VO2max), time to fatigue (TTF), velocity at VO2max (vVO2max), and running economy (RE) improved in all groups (p ≤ 0.01). The CPC-IE group outscored the other groups in muscle strength, RE, and TTF (p < 0.05). In summary, participants with complete occlusion experienced the greatest improvements in muscle strength, aerobic, and anaerobic parameters possibly due to increased oxygen deficiency and higher metabolic stress.
Introduction
Endurance training improves performance capacity via targeted muscular and cardiovascular adaptations such as an increase in oxidative enzymes, capillary density, glycogen content, and increases in stroke volume and cardiac hypertrophy (Nadel, 1985). Former research suggests that training adaptations maybe further enhanced by the addition of ischemia to working muscles (Sundberg, 1994). Sundberg (1994) applied 50 mmHg restrictive pressure on one leg during exercise for 4 weeks while the contralateral leg was trained without occlusion, and the results indicated a greater peak oxygen uptake and TTF in the ischemic condition. Thus, the application of exogenous hypoxia may be a potent stimulant for physical adaptation.
Blood flow restriction reduces arterial blood flow to working muscles while also occluding venous return. In BFR conditions, active muscles encounter a ischemia state which imposes a greater metabolic stress on working muscles (Tanimoto et al., 2005). The additional metabolic stress of BFR and especially venous occlusion increases muscle cell swelling (Loenneke et al., 2012), activates intra-cellular anabolic pathways (Abe et al., 2005), and recruits fast-twitch fibers (Yasuda et al., 2009), which are thought to be involved in muscular adaptation. The adaptations to exercise with BFR depend on several factors including the pressure of occlusion (i.e., partial or complete), the type of occlusion (i.e., continuous or intermittent), the intensity of exercise (i.e., low, moderate, or high), and the volume of exercise with BFR.
The pressure of occlusion used in previous studies varied from partial (∼160 mmHg) to complete (∼240–300 mmHg) occlusion (Abe et al., 2010a; Park et al., 2010), although it depend on the limb size and the cuff size (Mattocks et al., 2018). To identify an optimum occlusion pressure, Loenneke et al. (2015) studied the effect of different occlusion pressure and training intensity in young males. The results showed greater muscle activation at higher degrees of occlusion (i.e., 50% arterial occlusion). Also, muscle activation was greater at the higher training intensity (i.e., 30% 1RM compared to 20% 1RM). Interestingly, any additional increase in occlusion pressure higher than 50% arterial occlusion did not result in any greater muscle activation, and neither pressure nor training load affected muscle torque development (Loenneke et al., 2015). However, these findings are inconsistent with other research showing a positive effect of higher degrees of occlusion and reporting a sevenfold increase in growth hormone levels in response to a training session with complete occlusion (Pierce et al., 2006) and a fourfold increase in response to partial occlusion compare to non-BFR groups (Reeves et al., 2006).
In addition to occlusion pressure, training intensity is an important factor affecting adaptations in ischemic conditions. Training with BFR is proposed as an effective training paradigm to enhance both aerobic power (Abe et al., 2010a) and muscle strength (Abe et al., 2006; de Oliveira et al., 2016) without the need for training at high intensity. Hence most researchers have studied the effect of low-intensity resistance or aerobic training with BFR. Abe et al. (2010a) compared the effect of 15 min cycling with BFR at 40% VO2max with 45 min cycling without BFR at the same intensity for 8 weeks in young men. Their results showed that cycling with BFR (160–210 mmHg) significantly improved VO2max, time to exhaustion (TTE), strength, and muscle cross-sectional area (CSA) compared with cycling without BFR. Similarly, Park et al. (2010) reported ∼12% improvements in maximum aerobic capacity and 2.5% increase in anaerobic capacity after 2 weeks of walk training with BFR in collegiate basketball players. Other researchers have also reported an improvement in VO2max, onset blood lactate accumulation (OBLA), maximal power output, and muscle strength in BFR group in response to low-intensity aerobic training (Abe et al., 2010a, b; Park et al., 2010; de Oliveira et al., 2016). These adaptations were similar to those seen with high-intensity aerobic interval training without BFR (Laursen and Jenkins, 2002).
A few studies have examined the effects of high-intensity training in combination with BFR. Following 6 (Keramidas et al., 2012) and 4 weeks (Paton et al., 2017) of high-intensity training, there were similar improvements at VO2max between the BFR and the non-BFR group, but there were significant improvement at the RE and TTE in BFR group compared to non-BFR group in a former study (Paton et al., 2017). Therefore, it appears that higher intensity training with BFR could also result in additional benefits. However, training at a higher intensity with complete occlusion may be difficult for many individuals because this combination results in higher rate of perceived exertion (RPE) during training (Loenneke et al., 2013; Amani-shalamzari et al., 2019).
Previous studies have investigated the effects of various degrees of BFR or training intensities separately. However, in BFR training, the magnitude of pressure (partial or complete occlusion) and exercise intensity are important variables, which determine physiological adaptations. No previous study has investigated the combination of these variables on physiological and performance measures. It was hypothesized that various combinations of cuff pressure and training intensities result in different aerobic and anaerobic adaptations. Therefore, this study aimed to investigate the effects of various combinations of occlusion pressure, and exercise intensity on performance in physically active collegiate women.
Materials and Methods
Experimental Design
This study was a randomized controlled trial and examined the effects of four training protocols with BFR on aerobic and anaerobic adaptations. The protocols were developed using a combination of different occlusion pressure and training intensity. The protocols were chosen based on the most common variations in pressures and exercise intensities used in previous studies. We also took an applied approach and chose to use those methods that were practicable for athletes and coaches to perform during training. The dependent variables in this study included: body composition, aerobic and anaerobic capacity, TTE, vVO2max, isometric leg strength, and RE, which were measured before and after the training intervention.
Participants
Thirty-two active collegiate women met the inclusion criteria and volunteered to participate in this study (Table 1). Eligibility criteria included physically active women aged between 18 and 30 years without orthopedic, neuromuscular disorder, or metabolic and cardiovascular diseases. All experimental procedures were approved by the Ethics committee of Sport Sciences Research Institute of Iran with code IR.SSRI.REC.1397.326 and were conducted in accordance with the Declaration of Helsinki. The researcher explained the risks and benefits of the study to participants and obtained written informed consent prior to initial assessments.
Familiarization and Training Programs
Participants were familiarized with assessments and training protocols and then the next week anthropometric characteristics, VO2max, isometric leg strength, TTE, and anaerobic performance were assessed in three sessions interspersed by 48 h of recovery (Figure 1).
Upon completion of the initial assessment, the participants were divided into four homogeneous groups (n = 8) based on aerobic and anaerobic power, then randomly assigned into one of the following four groups: IP-CE, CPP-IE, CPC-IE, and IP-IE. The intervention period was 4 weeks for all groups and consisted of three sessions per week for 4 weeks (i.e., a total of 12 training sessions). The training protocol for all groups was the same and comprised of bouts of 2 min running on a treadmill with BFR interspersed by 1 min of recovery without BFR. The IP-CE, CPP-IE, and CPC-IE groups completed 10 sets of training in each session, while the IP-IE group completed 10, 8, 6, and 5 bouts in weeks 1, 2, 3, and 4, respectively. This reduction in training bouts was to account for difficulties in training at high intensity with a complete occlusion. Two 5 × 120 cm pneumatic cuffs (Ghamat Pooyan, Tehran, Iran) were worn by participants on the most proximal portion of both thighs. The cuffs were inflated to the target pressure during the exercise and deflated in the break periods. The final pressure was set to a percentage of arterial occlusion estimated from thigh circumference (Loenneke et al., 2015). The thigh circumference was measured and the partial BFR pressure (∼50% estimated arterial occlusion) and complete occlusion pressure proportional to the thigh circumference were selected. These BFR pressures remained constant in CPP-IE and CPC-IE groups until the end of the training protocol (Table 2). However, the BFR gradually increased in IP-CE and IP-IE groups. A polar telemetry system (Polar RC3, Polar Electro Oy, Kempele, Finland) was used to measure heart rate during all training sessions. The Borg scale (CR-20) was also used to determine RPE during training (Borg, 1998).
Test Procedures
Participants attended an Exercise and Sport Science laboratory to complete fitness tests 1 week before and after the training intervention. A schematic view of the testing timeline is presented in Figure 1. Anthropometric measurements included body mass (digital weighing scales, Seca 769, Germany), body height (stadiometer, Seca 213, Germany), and percentage body fat (InBody S10, Biospace Co. Ltd., Seoul, Korea) and were obtained 1 week prior to the intervention period. On the same day, VO2max was measured using an incremental running test on a motorized treadmill (Pulsar® 3p, h/p/Cosmos, Nussdorf-Traunstein, Germany). The test started at 10 km h–1 for 3 min followed by increments of 1 km h–1 every 1 min at 1% inclination until voluntary exhaustion (Milioni et al., 2016). The maximum oxygen uptake and produced carbon dioxide were analyzed breath-by-breath using a calibrated ergospirometer (MetaLyzer3B, CORTEX, Leipzig, Germany). vVO2max was defined as the minimum velocity at which VO2max was initially achieved (McLaughlin et al., 2010). In addition, a measure of RE was identified using the mean absolute oxygen cost (l min–1) measured during the final 2 min of the 10-km/h running stage. TTF was measured 48 h after the VO2max-test. After a 5 min warm-up including walking and jogging on a treadmill, participants started the TTF test on a treadmill at a speed corresponding to 60% of their vVO2max, and then progressively increased to 100% vVO2max over 30–45 s period. At this moment, a stopwatch was used to record the time (the start of vVO2max) to exhaustion (Billat et al., 1996). The test was terminated when a participant could not maintain the running speed and reached exhaustion. On the same day, 2 h before TTF test, participants performed an isometric leg muscle strength test using a digital dynamometer (Backmuscle0033, Danesh Salar Iranian, Tehran, Iran) to obtain back and leg strength. Participants stood upright on the base of the dynamometer and adjusted the length of the chain so that the angles of their knees were approximately 110 degrees. With straight arms and without changing the angle of their back, participants pulled the chain steadily with maximum strength. On the following day, participants performed a 30-s Wingate test on a stationary bicycle ergometer (Monark Model 894E, Monark, Vansbro, Sweden) to measure peak power (PP), average power (AP), and minimum power (MP). After cycling for 5 min at 60 revolutions per min (rpm) for warm-up, participants increased the pedaling rate to reach the maximum cadence, and manually dropped the basket that was holding the load equivalent to 0.075 kg kg body mass–1. The researchers encouraged participants verbally for the duration of the test.
Statistical Analysis
Data were analyzed using the statistical package of Social Sciences (SPSS, IBM, v19) and presented in mean ± standard deviation. A one-way analysis of covariance (ANCOVA) was performed to analyze differences between the values of post-test between groups, while the baseline values were considered as co-variants. First, the assumptions of ANCOVA included the normality of data (Shapiro–Wilk test), homogeneity of variance (box plot), and homogeneity of regression slopes was confirmed. To determine differences between groups, when a significant difference was found, Bonferroni post hoc tests were performed. Paired sample tests were performed to analyze differences within groups. Effect size (ES) was also calculated to examine the magnitude of differences in the dependent measures within groups, with 0.2 considered as a small ES, 0.5 as a moderate ES, and >0.8 as a large ES (Batterham and Hopkins, 2006). The level of significance was set at p ≤ 0.05 for all analysis.
Results
Aerobic Parameters
Changes in aerobic and anaerobic fitness, RE, TTF, and effect sizes are presented in Table 3. VO2max increased significantly in all groups from pre-to-post intervention (p < 0.05). However, the percentage change was different between groups (IP-CE: 9.6 ± 2.0%; CPP-IE: 11.2 ± 5.5%; CPC-IE: 14.8 ± 4.9%; IP-IE: 8.4 ± 2.4%). Bonferroni post hoc analysis revealed a significant difference between CPC-IE with IP-IE group (p = 0.01).
There was a significant difference between the groups in RE (p = 0.02). Bonferroni post hoc tests revealed a significant difference between the CPC-IE group with IP-CE (p = 0.04) and IP-IE (p = 0.04) groups. The RE decreased significantly from pre-to-post intervention for IP-CE (−5.6 ± 5.2%; CPP-IE: −9.6 ± 7.4%; CPC-IE: −17.6 ± 6.1%). However, this decrease for IP-IE was not statistically significant (−6.3 ± 6.6%).
In addition, TTF increased significantly in all groups, IP-CE: 22.1 ± 5.4%; CPP-IE: 18.2 ± 9.9%; CPC-IE: 30.3 ± 7.6%; and IP-IE: 21.3 ± 8.5%. Bonferroni post hoc analysis showed a significant difference between CPC-IE and CPP-IE group (p = 0.04).
The vVO2max also increased significantly in all groups (IP-CE: 14.6 ± 6.9%; CPP-IE: 12.4 ± 9.7%; CPC-IE: 18.3 ± 2.9%; and IP-IE: 15.9 ± 6.8%). However, there was no significant difference between groups (p = 0.21).
Anaerobic Parameters
The PP increased significantly in all groups (IP-CE: 21.3 ± 5.5%; CPP-IE: 17.5 ± 9.7%; CPC-IE: 28.1 ± 4.3%; and IP-IE: 13.5 ± 10.0%). Moreover, there was a significant difference between CPC-IE with IP-IE group (p = 0.02). In addition, AP showed a significant increase in all groups from pre-training to post-training (IP-CE: 15.1 ± 6.8%; CPP-IE: 10.3 ± 6.1%; CPC-IE: 22.6 ± 4.9%; and IP-IE: 13.9 ± 8.6%). The difference between CPC-IE group with CPP-IE and IP-IE groups was also significant (p < 0.05).
The MP increased significantly in all groups (IP-CE: 4.9 ± 2.7%; CPP-IE: 6.9 ± 3.9%; CPC-IE: 16.9 ± 9.2%; and IP-IE: 7.4 ± 3.6%). The results of CPC-IE group were significantly different from all other groups (p < 0.05).
Strength
Muscle strength increased significantly in all training conditions (IP-CE: 18.8 ± 7.9%; CPP-IE: 20.3 ± 6.3%; CPC-IE: 31.0 ± 6.2%; and IP-IE: 20.5 ± 2.7%). The strength of CPC-IE group was also significantly different from the other three groups (p < 0.05).
RPE and Heart Rate
Similar to heart rate responses, RPE was greater in the CPC-IE group than in the other groups during all training sessions (Figure 2). RPE values for the CPC-IE group were “very hard” (18.0 ± 0.4) and for the other groups rated as “hard” (IP-CE: 16.2 ± 0.5; CPP-IE: 16.4 ± 0.4; IP-IE: 16.8 ± 0.6). In most training sessions, the mean heart rate was higher in the CPC-IE group than in the other groups. During the last week, mean heart rate in CPC-IE and IP-IE was the same but higher than in the other two groups (Figure 2).
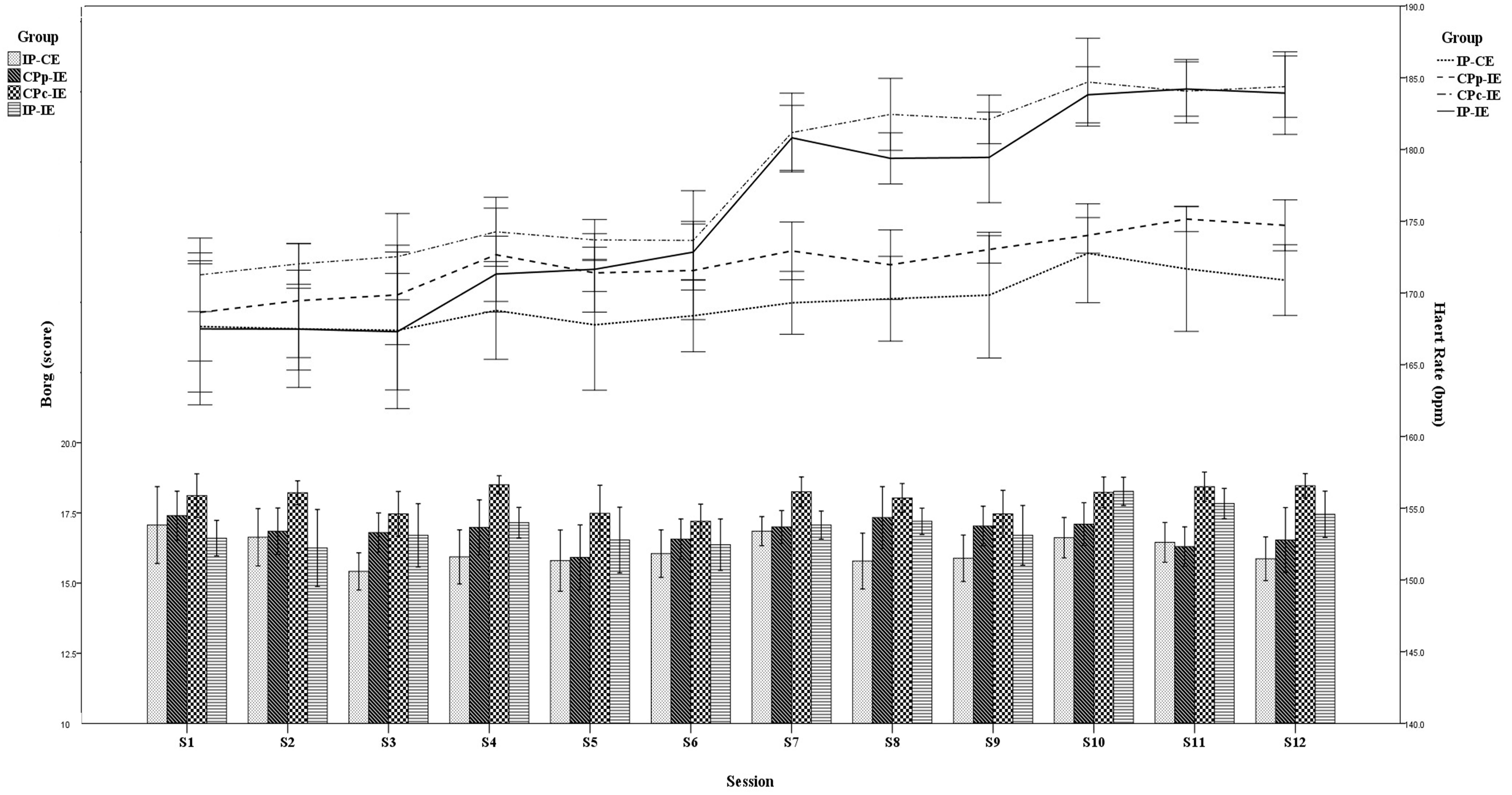
Figure 2. Heart rate responses and the rate of perceived exertion (RPE) for each group in each training session. IP-CE, increasing BFR pressure with constant exercise intensity; CPP-IE, constant partial BFR pressure with increasing exercise intensity; CPC-IE, constant complete BFR pressure with increasing exercise intensity; IP-IE, increasing BFR pressure with increasing exercise intensity.
Discussion
Previous researchers have examined the efficacy of aerobic training with BFR, and because of methodological difference that included various combinations of exercise loads and occlusion pressures, reported inconsistent and contradictory findings (Sundberg, 1994; Abe et al., 2006; Park et al., 2010). This study attempted to identify an optimal combination of occlusion and exercise intensity to maximize aerobic and anaerobic adaptations. The results showed greater improvements in strength, aerobic, and anaerobic parameters in response to complete occlusion and progressive training.
The improvement in VO2max and aerobic parameters such as vVO2max and TTF in this study is in agreement with those of previous studies that investigated the effect of low-intensity aerobic exercise in combination with BFR (Abe et al., 2010a, b; Park et al., 2010). Significant improvements in aerobic capacity have been reported in response to 4 weeks of training with 50 mmHg external pressure (Sundberg, 1994), 2 weeks training with 200 mmHg (Park et al., 2010), 4 weeks training with 140–200 mmHg (de Oliveira et al., 2016). These results suggest an interrelationship between the training duration and the degree of BFR, in which training programs with lower training durations could be effective if a higher degree of BFR is imposed and vice versa.
Maximum oxygen consumption is identified by cardiac output and arteriovenous oxygen difference. Hemodynamic parameters such as stroke volume, heart rate, and cardiac output are possible mechanisms that could have contributed to the increased aerobic capacity in this study. Although these factors were not measured in this study, Park et al. (2010) attributed the improvements in aerobic power in response to walking with BFR to hemodynamic parameters. Furthermore, the increased fluid shear stress caused by ischemia and reperfusion between repetitions in this study could have been a strong stimulator for up-regulation of expression of angiogenesis-related factors (Hudlicka and Brown, 2009), which has been shown to improve aerobic power (Hudlicka and Brown, 2009).
Running economy is related to oxygen consumption in running at a specific velocity. The results of this study showed that RE can significantly improve in response to progressive exercise intensity. Thus, exercise intensity appears to be a key factor in improving RE. Chen et al. (2009) compared RE in response to training at 70, 80, and 90% VO2max and reported a greater improvement in response to training at a higher intensity (the lactate threshold) compared to low intensity (Chen et al., 2009). Also, an improved RE in response to high-intensity training (80% peak running velocity) and BFR was reported by Paton et al. (2017). Researchers attributed this improvement to intra-muscle adaptations such as the activity of oxidative enzymes and capillary density of involved muscles (Esbjornsson et al., 1993; Paton et al., 2017). In this study, there were significant differences between the RE in CPC-IE with IP-CE and IP-IE groups. It seems that in addition to exercise intensity, training volume could also affect the magnitude of change in RE. The training volume of the IP-IE group was reduced over time (from 10 repetitions in the first week to 5 repetitions in the last week). Therefore, to improve the RE, training volume may also need to be considered.
In addition to improvement in aerobic parameters, significant increases with large ES were observed in TTF in all groups. An interesting finding was the larger percentage of change from pre-intervention to post-intervention in groups that experience complete occlusion either from the beginning or toward the end of the training intervention. This finding may suggest that greater cuff pressure may result in greater improvements.
Time to fatigue was defined as the duration of running from vVO2max to exhaustion (Messonnier et al., 2002). The energy required for this period is provided by both aerobic and anaerobic energy system (Bertuzzi et al., 2012), and the performance during this time relies on anaerobic capacity and lactate clearance capabilities (Messonnier et al., 2002). It is likely that participants of this study have developed greater anaerobic capacity and lactate clearance ability, and these adaptations may have contributed to TTF improvement. Although these variables were not measured in this study, previous studies have demonstrated that training with BFR results in a significant increase in the rate of lactate clearance during incremental exercise (de Oliveira et al., 2016). In addition, it has been reported that training with BFR is associated with increased recruitment of type 2 muscle fibers and a corresponding increase in lactate production (Takano et al., 2005). Thus, increased lactate tolerance with BFR may be associated with a TTF increase. Also, it is possible that muscle buffering capacity have increased in all groups, especially in groups that cuff pressure either was at a maximum or was increasing. In these groups, accumulation of metabolic by-products was associated with more buffering capacity.
The Wingate anaerobic test is considered as the gold standard for assessment of anaerobic power. In this study, significant increases in anaerobic parameters (peak, average, and minimum power) were observed in all groups. This observed improvements in anaerobic parameters in our study were similar to those reported in response to resistance training with BFR (Sundberg, 1994; Burgomaster et al., 2003), and aerobic training with BFR (Park et al., 2010). Based on the results of this study, it seems there was a relationship between the magnitude of BFR and improvements in anaerobic power, so that the least improvement in anaerobic parameters was seen in the CPP-IE group where the cuff pressure remained low throughout the intervention period. The pressure of cuff in the other three groups reached the complete occlusion during the intervention, and the differences between these three groups were significantly higher than of those observed in CPP-IE group. The difference in PP between CPC-IE group with IP-IE group could be attributed to the lower exercise volume in the IP-IE group; although the higher cuff pressure in this group was a plausible reason for the observed non-significant difference with the other two groups. The metabolic stress with BFR training plays an important role in initiating muscle adaptations (Suga et al., 2012; Teixeira et al., 2018). Dependence on anaerobic metabolism and disturbed oxygen delivery during BFR training could result in increased muscle glycogen stores (Sundberg, 1994; Burgomaster et al., 2003) which would improve anaerobic power especially in CPC-IE group that trained with the highest metabolic stress.
An important finding of this study was a significant increase in muscle strength in all groups that was reinforced by large effect sizes. Furthermore, CPC-IE group showed a significantly larger gain in muscle strength compared to other three groups. Few researches reported an increased muscle strength with short-term low-intensity training (de Oliveira et al., 2016) and walking (Abe et al., 2006, 2010b) with BFR. The mechanisms of strength gain in response to training with BFR are not fully understood. Abe et al. (2006) observed an increase in CSA of thigh muscles in response to 3 weeks of twice-daily KAATSU-walking in young men. A significant increase in muscle strength in all groups in this study could possibly be due to an increase in CSA. However, a limitation of this study was the lack of information about structural changes of the involved muscles.
Another possibility for the observed increase in muscle strength could be the recruitment of fast twitch muscle fibers. Previous researchers have highlighted the effect of oxygen deficiency on the recruitment of fast twitch motor units (type II muscle fibers) to generate force and strength adaptations (Moritani et al., 1992; Abe et al., 2010b; de Oliveira et al., 2016). It seems that BFR which is associated with the release of metabolic by-products could recruit more fast-twitch motor units to generate force, and thus induces a favorable milieu for improving muscle strength and anaerobic power (Moore et al., 2004).
We acknowledge several limitations with this study. First, instead of the gold standard method, such as Doppler, we estimated arterial occlusion from the thigh circumference which is a method based on findings of previous research (Loenneke et al., 2015). Second, the sample size was small because few female students from a large statistical community volunteered to participate in the study and met the inclusion criteria.
Conclusion
In conclusion, this study showed that training intensity and magnitude of BFR might alter aerobic, anaerobic, and muscular performance in physically active women. Our findings demonstrated improvements in all aerobic and anaerobic variables in all groups with a trend for greater gains in strength, aerobic, and anaerobic parameters in response to progressively periodized intensity and complete occlusion.
Ethics Statement
All procedures performed in studies involving human participants were in accordance with the ethical standards of Sport Sciences Research Institute of Iran with code IR.SSRI.REC.1397.326 and with the 1964 Helsinki declaration and its later amendments or comparable ethical standards. This article does not contain any studies with animals performed by any of the authors.
Author Contributions
SA-S, HR, and CP conceived the study. SR and SA-S conducted the experiments. SA-S, DG, CP, and MB analyzed the study. SA-S, HR, DG, CP, MB, TR, PN, and BK interpreted the data for the study. All authors made substantial contributions to the design of the work, drafted the work or revised it critically for important intellectual content, provided final approval of the version to be published, and agreed to be accountable for all aspects of the work in ensuring that questions related to the accuracy or integrity of any part of the work are appropriately investigated and resolved.
Conflict of Interest Statement
The authors declare that the research was conducted in the absence of any commercial or financial relationships that could be construed as a potential conflict of interest.
Acknowledgments
The authors would like to acknowledge the students who participated in this study.
Abbreviations
ANCOVA, analysis of covariance; AP, average power; BFR, blood flow restriction; CPC-IE, constant complete BFR pressure with increasing exercise intensity; CPP-IE, constant partial BFR pressure with increasing exercise intensity; CSA, cross-sectional area; ES, effect size; IP-CE, increasing BFR pressure with constant exercise intensity; IP-IE, increasing BFR pressure with increasing exercise intensity; MP, minimum power; OBLA, onset blood lactate accumulation; PP, peak power; RE, running economy; RPE, rate perceived exertion; TTE, time to exhaustion; TTF, time to fatigue; VO2max, maximum oxygen consumption; vVO2max, velocity at VO2max.
References
Abe, T., Fujita, S., Nakajima, T., Sakamaki, M., Ozaki, H., Ogasawara, R., et al. (2010a). Effects of low-intensity cycle training with restricted leg blood flow on thigh muscle volume and VO2max in young men. J. Sports Sci. Med. 9, 452–458.
Abe, T., Sakamaki, M., Fujita, S., Ozaki, H., Sugaya, M., Sato, Y., et al. (2010b). Effects of low-intensity walk training with restricted leg blood flow on muscle strength and aerobic capacity in older adults. J. Geriatr. Phys. Ther. 33, 34–40.
Abe, T., Kearns, C. F., and Sato, Y. (2006). Muscle size and strength are increased following walk training with restricted venous blood flow from the leg muscle, Kaatsu-walk training. J. Appl. Physiol. 100, 1460–1466. doi: 10.1152/japplphysiol.01267.2005
Abe, T., Yasuda, T., Midorikawa, T., Sato, Y., Kearns, C. F., Inoue, K., et al. (2005). Skeletal muscle size and circulating IGF-1 are increased after two weeks of twice daily “KAATSU” resistance training. Int. J. Kaatsu. Train. Res. 1, 6–12. doi: 10.3806/ijktr.1.6
Amani-shalamzari, S., Farhani, F., Rajabi, H., Abbasi, A., Sarikhani, A., Paton, C., et al. (2019). Blood flow restriction during futsal training increases muscle activation and strength. Front. Physiol. 10:614. doi: 10.3389/fphys.2019.00614
Batterham, A. M., and Hopkins, W. G. (2006). Making meaningful inferences about magnitudes. Int. J Sports Physiol. Perform 1, 50–57. doi: 10.1123/ijspp.1.1.50
Bertuzzi, R., Bueno, S., Pasqua, L. A., Acquesta, F. M., Batista, M. B., Roschel, H., et al. (2012). Bioenergetics and neuromuscular determinants of the time to exhaustion at velocity corresponding to VO2max in recreational long-distance runners. J. Strength Cond. Res. 26, 2096–2102. doi: 10.1519/JSC.0b013e31823b8721
Billat, V. L., Hill, D. W., Pinoteau, J., Petit, B., and Koralsztein, J. P. (1996). Effect of protocol on determination of velocity at VO2 max and on its time to exhaustion. Arch. Physiol. Biochem. 104, 313–321. doi: 10.1076/apab.104.3.313.12908
Burgomaster, K. A., Moore, D. R., Schofield, L. M., Phillips, S. M., Sale, D. G., and Gibala, M. J. (2003). Resistance training with vascular occlusion: metabolic adaptations in human muscle. Med. Sci. Sports Exerc. 35, 1203–1208. doi: 10.1249/01.mss.0000074458.71025.71
Chen, T. C., Nosaka, K., Lin, M. J., Chen, H. L., and Wu, C. J. (2009). Changes in running economy at different intensities following downhill running. J. Sports Sci. 27, 1137–1144. doi: 10.1080/02640410903062027
de Oliveira, M. F., Caputo, F., Corvino, R. B., and Denadai, B. S. (2016). Short-term low-intensity blood flow restricted interval training improves both aerobic fitness and muscle strength. Scand. J. Med. Sci. Sports 26, 1017–1025. doi: 10.1111/sms.12540
Esbjornsson, M., Jansson, E., Sundberg, C. J., Sylven, C., Eiken, O., Nygren, A., et al. (1993). Muscle fibre types and enzyme activities after training with local leg ischaemia in man. Acta Physiol. Scand. 148, 233–241. doi: 10.1111/j.1748-1716.1993.tb09554.x
Hudlicka, O., and Brown, M. D. (2009). Adaptation of skeletal muscle microvasculature to increased or decreased blood flow: role of shear stress, nitric oxide and vascular endothelial growth factor. J. Vasc. Res. 46, 504–512. doi: 10.1159/000226127
Keramidas, M. E., Kounalakis, S. N., and Geladas, N. D. (2012). The effect of interval training combined with thigh cuffs pressure on maximal and submaximal exercise performance. Clin. Physiol. Funct. Imaging 32, 205–213. doi: 10.1111/j.1475-097X.2011.01078.x
Laursen, P. B., and Jenkins, D. G. (2002). The scientific basis for high-intensity interval training: optimising training programmes and maximising performance in highly trained endurance athletes. Sports Med. 32, 53–73. doi: 10.2165/00007256-200232010-00003
Loenneke, J. P., Fahs, C. A., Rossow, L. M., Abe, T., and Bemben, M. G. (2012). The anabolic benefits of venous blood flow restriction training may be induced by muscle cell swelling. Med. Hypotheses 78, 151–154. doi: 10.1016/j.mehy.2011.10.014
Loenneke, J. P., Kim, D., Fahs, C. A., Thiebaud, R. S., Abe, T., Larson, R. D., et al. (2015). Effects of exercise with and without different degrees of blood flow restriction on torque and muscle activation. Muscle Nerve 51, 713–721. doi: 10.1002/mus.24448
Loenneke, J. P., Thiebaud, R. S., Fahs, C. A., Rossow, L. M., Abe, T., and Bemben, M. G. (2013). Blood flow restriction does not result in prolonged decrements in torque. Eur. J. Appl. Physiol. 113, 923–931. doi: 10.1007/s00421-012-2502-x
Mattocks, K. T., Jessee, M. B., Mouser, J. G., Dankel, S. J., Buckner, S. L., Bell, Z. W., et al. (2018). The application of blood flow restriction: lessons from the laboratory. Curr. Sports Med. Rep. 17, 129–134. doi: 10.1249/JSR.0000000000000473
McLaughlin, J. E., Howley, E. T., Bassett, D. R. Jr., Thompson, D. L., and Fitzhugh, E. C. (2010). Test of the classic model for predicting endurance running performance. Med. Sci. Sports Exerc. 42, 991–997. doi: 10.1249/MSS.0b013e3181c0669d
Messonnier, L., Freund, H., Denis, C., Dormois, D., Dufour, A. B., and Lacour, J. R. (2002). Time to exhaustion at VO(2)max is related to the lactate exchange and removal abilities. Int. J. Sports Med. 23, 433–438. doi: 10.1055/s-2002-33740
Milioni, F., Vieira, L. H., Barbieri, R. A., Zagatto, A. M., Nordsborg, N. B., Barbieri, F. A., et al. (2016). Futsal match-related fatigue affects running performance and neuromuscular parameters but not finishing kick speed or accuracy. Front. Physiol. 7:518. doi: 10.3389/fphys.2016.00518
Moore, D. R., Burgomaster, K. A., Schofield, L. M., Gibala, M. J., Sale, D. G., and Phillips, S. M. (2004). Neuromuscular adaptations in human muscle following low intensity resistance training with vascular occlusion. Eur. J. Appl. Physiol. 92, 399–406. doi: 10.1007/s00421-004-1072-y
Moritani, T., Sherman, W. M., Shibata, M., Matsumoto, T., and Shinohara, M. (1992). Oxygen availability and motor unit activity in humans. Eur. J. Appl. Physiol. Occup. Physiol. 64, 552–556.
Nadel, E. R. (1985). Physiological adaptations to aerobic training: the increase in blood volume induced by aerobic conditioning is a critical factor in improving performance and promoting resistance to fatigue. Am. Sci. 73, 334–343.
Park, S., Kim, J. K., Choi, H. M., Kim, H. G., Beekley, M. D., and Nho, H. (2010). Increase in maximal oxygen uptake following 2-week walk training with blood flow occlusion in athletes. Eur. J. Appl. Physiol. 109, 591–600. doi: 10.1007/s00421-010-1377-y
Paton, C. D., Addis, S. M., and Taylor, L. A. (2017). The effects of muscle blood flow restriction during running training on measures of aerobic capacity and run time to exhaustion. Eur. J. Appl. Physiol. 117, 2579–2585. doi: 10.1007/s00421-017-3745-3
Pierce, J. R., Clark, B. C., Ploutz-Snyder, L. L., and Kanaley, J. A. (2006). Growth hormone and muscle function responses to skeletal muscle ischemia. J. Appl. Physiol. 101, 1588–1595. doi: 10.1152/japplphysiol.00585.2006
Reeves, G. V., Kraemer, R. R., Hollander, D. B., Clavier, J., Thomas, C., Francois, M., et al. (2006). Comparison of hormone responses following light resistance exercise with partial vascular occlusion and moderately difficult resistance exercise without occlusion. J. Appl. Physiol. 101, 1616–1622. doi: 10.1152/japplphysiol.00440.2006
Suga, T., Okita, K., Takada, S., Omokawa, M., Kadoguchi, T., Yokota, T., et al. (2012). Effect of multiple set on intramuscular metabolic stress during low-intensity resistance exercise with blood flow restriction. Eur. J. Appl. Physiol. 112, 3915–3920. doi: 10.1007/s00421-012-2377-x
Sundberg, C. J. (1994). Exercise and training during graded leg ischaemia in healthy man with special reference to effects on skeletal muscle. Acta. Physiol. Scand Suppl. 615, 1–50.
Takano, H., Morita, T., Iida, H., Asada, K., Kato, M., Uno, K., et al. (2005). Hemodynamic and hormonal responses to a short-term low-intensity resistance exercise with the reduction of muscle blood flow. Eur. J. Appl. Physiol. 95, 65–73. doi: 10.1007/s00421-005-1389-1
Tanimoto, M., Madarame, H., and Ishii, N. (2005). Muscle oxygenation and plasma growth hormone concentration during and after resistance exercise: comparison between “KAATSU” and other types of regimen. Int. J. Kaatsu Train. Res. 1, 51–56. doi: 10.3806/ijktr.1.51
Teixeira, E. L., Barroso, R., Silva-Batista, C., Laurentino, G. C., Loenneke, J. P., Roschel, H., et al. (2018). Blood flow restriction increases metabolic stress but decreases muscle activation during high-load resistance exercise. Muscle Nerve 57, 107–111. doi: 10.1002/mus.25616
Keywords: occlusion, VO2max, time to fatigue, running economy, strength
Citation: Amani-Shalamzari S, Rajabi S, Rajabi H, Gahreman DE, Paton C, Bayati M, Rosemann T, Nikolaidis PT and Knechtle B (2019) Effects of Blood Flow Restriction and Exercise Intensity on Aerobic, Anaerobic, and Muscle Strength Adaptations in Physically Active Collegiate Women. Front. Physiol. 10:810. doi: 10.3389/fphys.2019.00810
Received: 27 January 2019; Accepted: 07 June 2019;
Published: 26 June 2019.
Edited by:
Urs Granacher, Universität Potsdam, GermanyReviewed by:
Brendan Richard Scott, Murdoch University, AustraliaMichael Behringer, Goethe-Universität Frankfurt am Main, Germany
Copyright © 2019 Amani-Shalamzari, Rajabi, Rajabi, Gahreman, Paton, Bayati, Rosemann, Nikolaidis and Knechtle. This is an open-access article distributed under the terms of the Creative Commons Attribution License (CC BY). The use, distribution or reproduction in other forums is permitted, provided the original author(s) and the copyright owner(s) are credited and that the original publication in this journal is cited, in accordance with accepted academic practice. No use, distribution or reproduction is permitted which does not comply with these terms.
*Correspondence: Sadegh Amani-Shalamzari, YW1hbmlfc2FkZWdoQGtodS5hYy5pcg==; Beat Knechtle, YmVhdC5rbmVjaHRsZUBoaXNwZWVkLmNo