- 1Department of Surgery, Yale School of Medicine, New Haven, CT, United States
- 2Transplantation and Hepatobiliary Surgery, University of Padua, Padua, Italy
Background: The calcium-sensing receptor (CaSR) has been localized and characterized in numerous tissues throughout the body. In the mammalian gastrointestinal tract, the CaSR is known to act as a nutrient sensor and has recently been found to play a role in intestinal fluid and electrolyte balance. This study aims to demonstrate the functionality of the CaSR as a modulator of fluid secretion and absorption along the small intestine.
Methods: Small intestine regions (proximal, middle, and distal) were isolated from Sprague Dawley rats and loaded into an ex vivo intestinal perfusion device that provides independent intraluminal and extraluminal (serosa/basolateral) perfusion. The regions were perfused with 5 and 7 mM of Ca2+, both in the presence and absence of forskolin (FSK), a potent secretagogue. Control experiments were conducted with intraluminal perfusate containing standard Ringer-HEPES buffer with a physiological concentration of Ca2+ (1 mM). A second set of comparison experiments was performed with intraluminal perfusates containing AC-265347, a CaSR activator and agonist, in the presence of FSK. In all experimental conditions, the intraluminal perfusate contained fluorescein isothiocyanate (FITC)-inulin, a nonabsorbable fluorescent marker of secretion and/or absorption. Intraluminal fluorescence signal was utilized as a measure of water movement at the start of the experiment and every 15 min for 90 min.
Results: Under physiological conditions, increasing the concentration of Ca2+ in the luminal perfusate reduced intestinal fluid secretion in all regions. At a Ca2+ concentration of 7 mM, net fluid absorption was observed in all regions. In the presence of FSK, 5 mM Ca2+ significantly decreased fluid secretion and 7 mM Ca2+ abolished FSK-induced fluid secretion. Intraluminal perfusion with 5 mM Ca2+ was as effective as AC-265347, in reducing secretagogue-induced fluid hypersecretion in the proximal and middle regions.
Conclusion: This study concludes that apical CaSR is active along the small intestine. Its activation by Ca2+ and/or calcimimetics reduces fluid secretion in a dose-dependent manner, with higher Ca2+ concentrations, or application of a calcimimetic, leading to fluid absorption. We furthermore show that, in the presence of FSK, receptor activation abates FSK secretagogue-induced fluid secretion. This presents a new therapeutic target to address secretory diarrheal illnesses.
Introduction
The extracellular CaSR is a classic transmembrane GPCR that was first identified in the parathyroid gland, where it plays an important role in total body calcium (Ca2+) homeostasis (Brown et al., 1993; Brown and MacLeod, 2001). In the mammalian gastrointestinal tract, the CaSR has been identified in Meissner’s and Auerbach’s plexuses as well as the apical and basolateral membranes of fluid-absorbing villous cells and fluid-secreting crypt cells of rat and human intestines (Chattopadhyay et al., 1998; Sheinin et al., 2000; Cheng et al., 2002; Tfelt-Hansen and Brown, 2005; Cheng, 2012; Macleod, 2013). In the absorptive gut, the CaSR functions as a nutrient sensor and provides a mechanism to signal to the enteric nervous system and coordinate food delivery and gut motility in order to maximize nutrient absorption (Tang et al., 2016). It has also been implicated in intestinal ion transport and the regulation of bicarbonate secretion (Xie et al., 2014; Tang et al., 2015).
In rat colonic crypts, the CaSR has been shown to be activated by Ca2+, amino acids, polyamines, and calcimimetics, resulting in net inhibition of fluid secretion into the lumen (Cheng et al., 2004; Geibel et al., 2006). The receptor modulates fluid and electrolyte secretion in the colon, both under normal physiological conditions and following exposure to potent secretagogues such as FSK, cholera toxin and heat-stable enterotoxins (Flores and Sharp, 1976; Geibel et al., 2006; Breitwieser, 2013; Tang et al., 2015). Extensive studies have been done on the role of the CaSR in the colon, which is not the case in the small intestine. Although the CaSR has been found along the entire length of the small intestine (Cheng et al., 2002; Dufner et al., 2005; Tu and Bikle, 2013; Alfadda et al., 2014), functional data on its role in modulating salt and fluid transport in the small intestine is lacking, partly due to the difficulty of isolating and maintaining viable villi. In this study, an ex vivo perfusion device capable of providing real-time data on intestinal fluid absorption and secretion is used to examine the functional role of the CaSR on the apical surface along the length of the small intestine. We also study the functional role of activation of the CaSR in blocking cyclic nucleotide-induced intestinal fluid secretion.
Materials and Methods
Male Sprague-Dawley rats weighing between 250 and 490 g (n = 24) were acquired from Charles River Laboratories (Wilmington, MA, United States) and housed in climate-controlled and light-cycled rooms with free access to water, in accordance with Yale University Animal Care and Use Committee guidelines (Protocol #2018-10253). The rats were fasted 12–15 h prior to each experiment.
Small Intestine Procurement
Fasted rats were fully anesthetized with Isoflurane (IsoThesiaTM, 99.9%/mL) after which a midline laparotomy was performed. Cold (4°C) Ringer-HEPES buffer (Table 1) was used to irrigate the abdominal cavity to reduce endogenous metabolism and decrease ischemic damage. The entire small bowel was procured. The proximal region (jejunum) was recovered 10–12 cm distally from the stomach and the distal region (ileum) was obtained 1–2 cm proximally from the ileocecal junction. The midpoint of the residual intestinal region was identified, and the middle region was obtained by cutting off 5 cm above and 5 cm below the midpoint. Each intestinal region (proximal, middle, and distal) was approximately 10 cm in length. The lumen of the regions was flushed with cold Ringer-HEPES buffer to remove residual fecal material. The regions were then connected to perfusion chambers of an ex vivo perfusion device previously described by our group (Munoz-Abraham et al., 2015). Each chamber provided independent serosa (extraluminal) and luminal (intraluminal) perfusion to the intestinal regions at a constant temperature and flow rate (37°C and 6 mL/min).
Ex vivo Perfusion
The first set of experiments included two groups in which the intestinal regions were intraluminally perfused with Ringer-HEPES buffer solution containing different concentrations of Ca2+ (5 and 7 mM) separately. Control regions were perfused with standard Ringer-HEPES buffer containing 1 mM Ca2+, which is within the physiologic concentration of Ca2+ in the small intestine (Chattopadhyay, 2000). The second set of experiments included three groups, in which the intraluminal perfusate contained 10 μM FSK (Sigma-Aldrich, St. Louis, MO, United States) a potent secretagogue, and varying concentrations of Ca2+ (5, 7, and control 1 mM), respectively. Lastly, we compared the effect of 1, 5, and 7 mM intraluminal Ca2+ in the presence of FSK to that of 2 μM of the calcimimetic 1-Benzothiazol-2-yl-1-(2,4-dimethyl-phenyl)-ethanol (AC-265347, Sigma-Aldrich, St. Louis, MO, United States), which is an allosteric agonist of the CaSR, which is also known to reduce ionized calcium (Ma et al., 2011).
In summary, a total of seven experimental conditions were performed for each intestinal region. In each condition extraluminal perfusion was with Ringer-HEPES buffer and the intraluminal perfusates were as follows:
(1) Ringer-HEPES buffer with 5 mM Ca2+
(2) Ringer-HEPES buffer with 5 mM Ca2+ + 10 μM FSK
(3) Ringer-HEPES buffer with 7 mM Ca2+
(4) Ringer-HEPES buffer with 7 mM Ca2+ + 10 μM FSK
(5) Standard Ringer-HEPES buffer
(6) Standard Ringer-HEPES buffer + 10 μM FSK
(7) Standard Ringer-HEPES buffer + 10 μM FSK + 2 μM AC-265347
All intraluminal perfusates also contained 1 mM FITC-inulin, a non-absorbable fluorescent marker of fluid secretion and/or absorption (Munoz-Abraham et al., 2015; AlKukhun et al., 2017). All solutions were adjusted to a final osmolarity of 300 ± 5 mOsm with mannitol, at a pH of 7.4 and temperature of 37°C. The concentration of NaCl was appropriately adjusted to compensate for the increased concentration of CaCl2, thereby keeping chloride concentration constant (refer to Table 1 for composition of all perfusion solutions).
Measurement of Fluid Secretion and Absorption
Nanofluorospectrophotometry (Nanodrop 3300, Thermo Fisher Scientific Inc.) was used to measure fluorescence intensity of the intraluminal perfusate over time. The greater the fluorescence of the sampled luminal content, the less water secretion into the lumen was observed, decreases in luminal fluorescence were indicative of a decreased concentration of FITC-inulin. For each experiment, fluorescence measurements were taken at seven-time points over the course of the experiment: 0, 15, 30, 45, 60, 75, and 90 min. Five intraluminal fluid samples of 2 μL each were taken for each experimental time point; the slope and SEM were calculated and graphed. At the end of each experiment, extraluminal perfusate samples were collected to exclude the possibility of leakage due to perforation or damage to the intestinal wall. The absence of any detected FITC-inulin in the basolateral (extraluminal) perfusate ruled out leakage and confirmed preserved integrity of intestinal regions. Presence of FITC-inulin in the extraluminal perfusate warranted discarding of the intestinal region.
Data Presentation and Statistical Analysis
Intraluminal fluorescence values of FITC-inulin concentration were calculated, plotted and analyzed using GraphPad Prism 8.01 (GraphPad Software). Linear regression analysis was performed to determine slopes, which represented average secretion or absorption per minute over 90 min. Statistically significant differences were calculated using mixed-effects analysis and Tukey’s multiple comparisons test. A p value of less than 0.05 (p < 0.05) was considered statically significant.
Results
Effect of Increasing Ca2+ Concentration
In the absence of FSK in the intraluminal perfusate, all small intestinal regions exhibited basal secretion with standard Ringer-HEPES buffer containing 1 mM Ca2+ (Table 2). When the intraluminal Ca2+ concentration was increased to 5 mM, the average secretion over time significantly decreased in all intestinal regions (Figure 1). Intraluminal perfusion with 7 mM Ca2+ not only abolished fluid secretion but also caused moderate fluid absorption in all intestinal regions, reversing the typical endogenous secretion profile seen in control regions perfused with standard Ringer-HEPES (Figure 1).
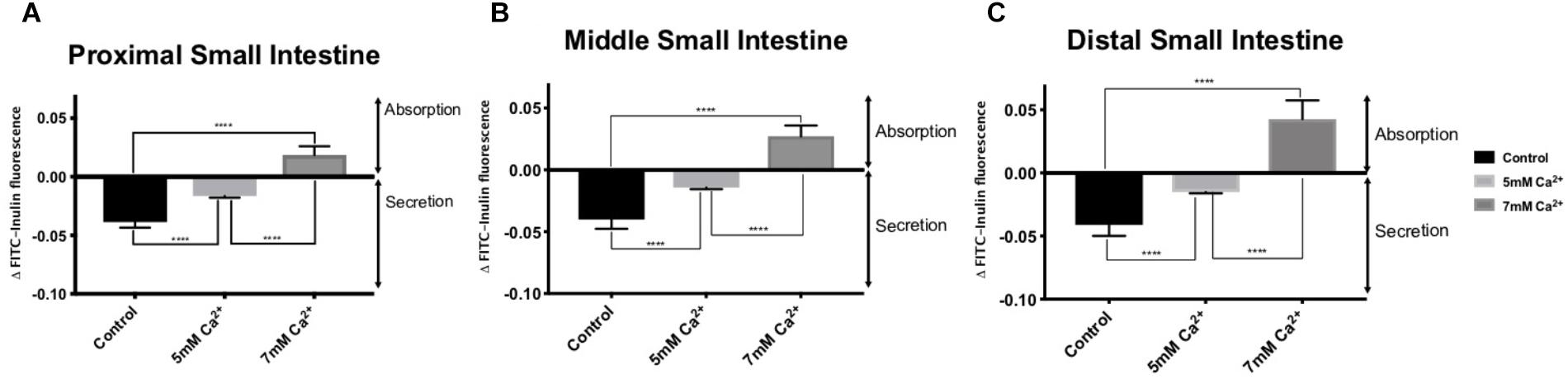
Figure 1. Effect of increasing intraluminal [Ca2+] on basal intestinal fluid secretion in the: (A) Proximal small intestine (B) Middle small intestine (C) Distal small intestine. P value < 0.0001 is represented by ∗∗∗∗.
Presence of FSK
Addition of FSK to the intraluminal perfusate dramatically increased fluid secretion in the control intestinal regions perfused with standard Ringer-HEPES (Table 3). In the presence of 5 mM Ca2+, intraluminal fluid secretion in all regions perfused with FSK significantly decreased in comparison to the controls (p < 0.0001 in all regions). As shown in Figure 2, intraluminal perfusion with 7 mM Ca2+ completely abolished FSK-induced secretion in all intestinal regions. This effect was statistically significant in comparison to control experiments (p < 0.0001 in all regions).
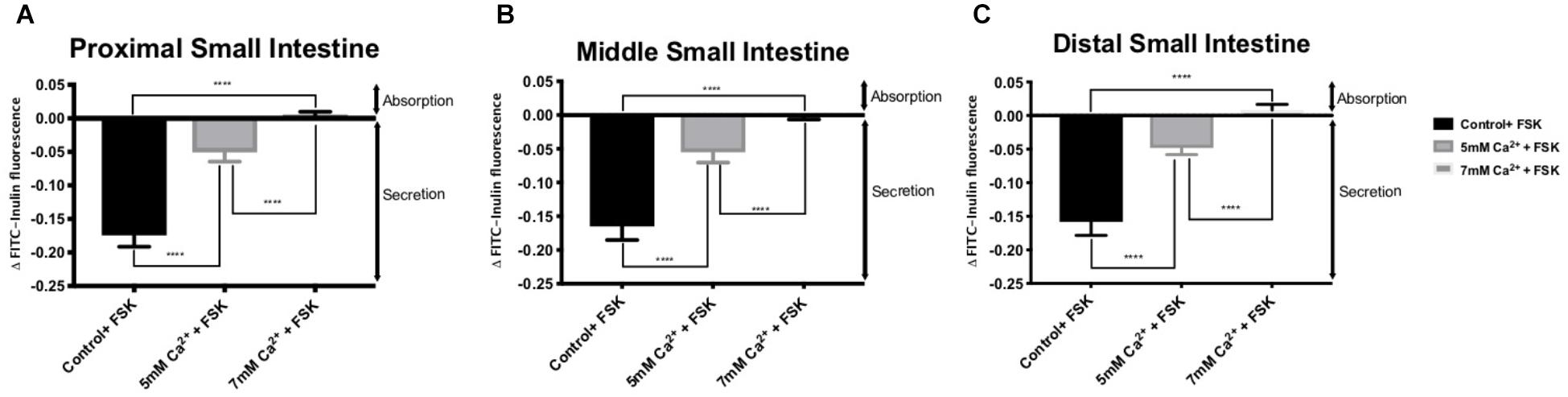
Figure 2. Intestinal fluid secretion in the presence of intraluminal 10 μM forskolin with varying [Ca2+] in the: (A) Proximal small intestine (B) Middle small intestine (C) Distal small intestine. P value < 0.0001 is represented by ∗∗∗∗.
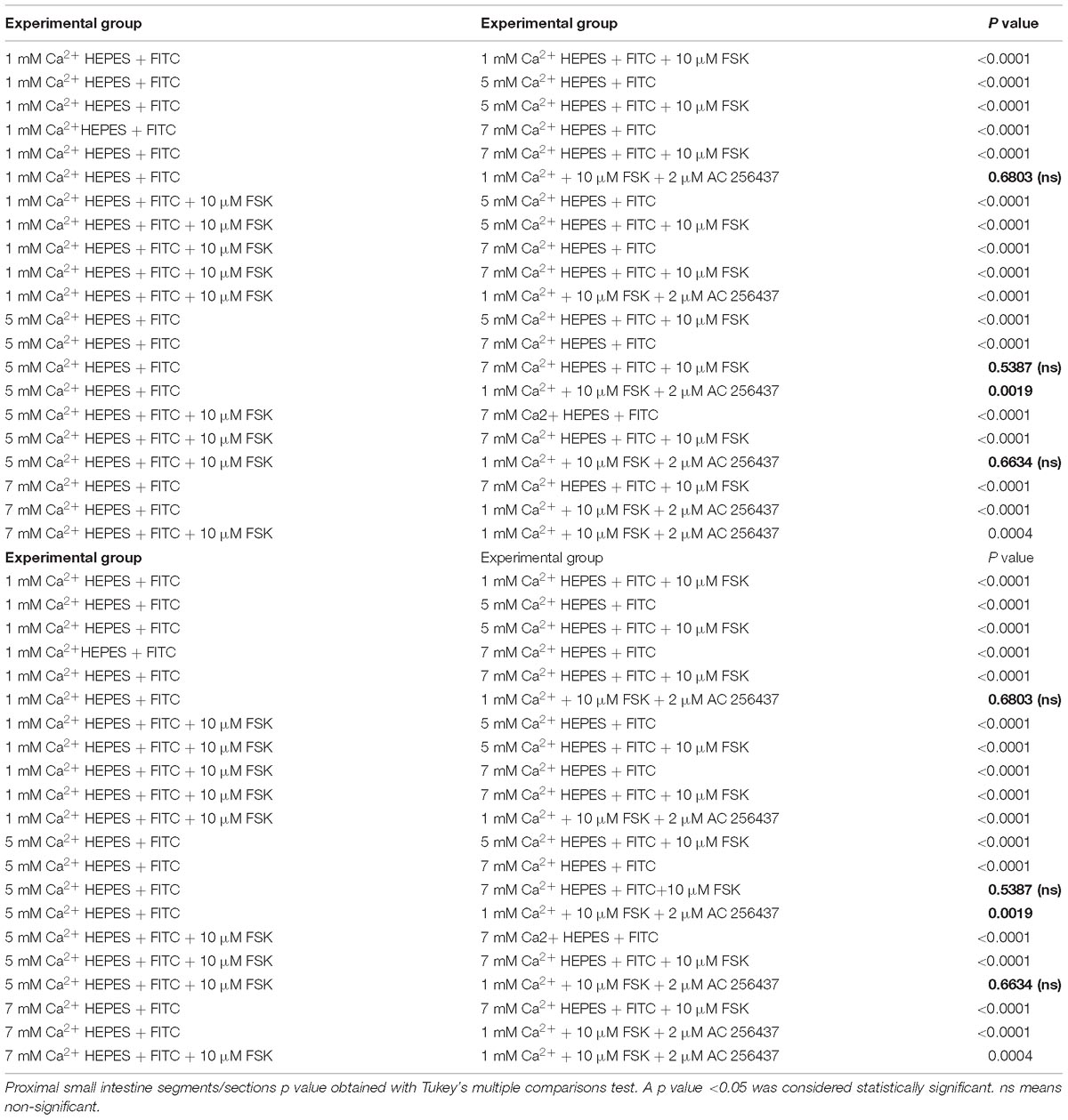
Table 4. Proximal region of small intestine. P values of ΔFluorescence/Minute with the different [Ca2+] and FSK in comparison to AC-265347 with FSK.
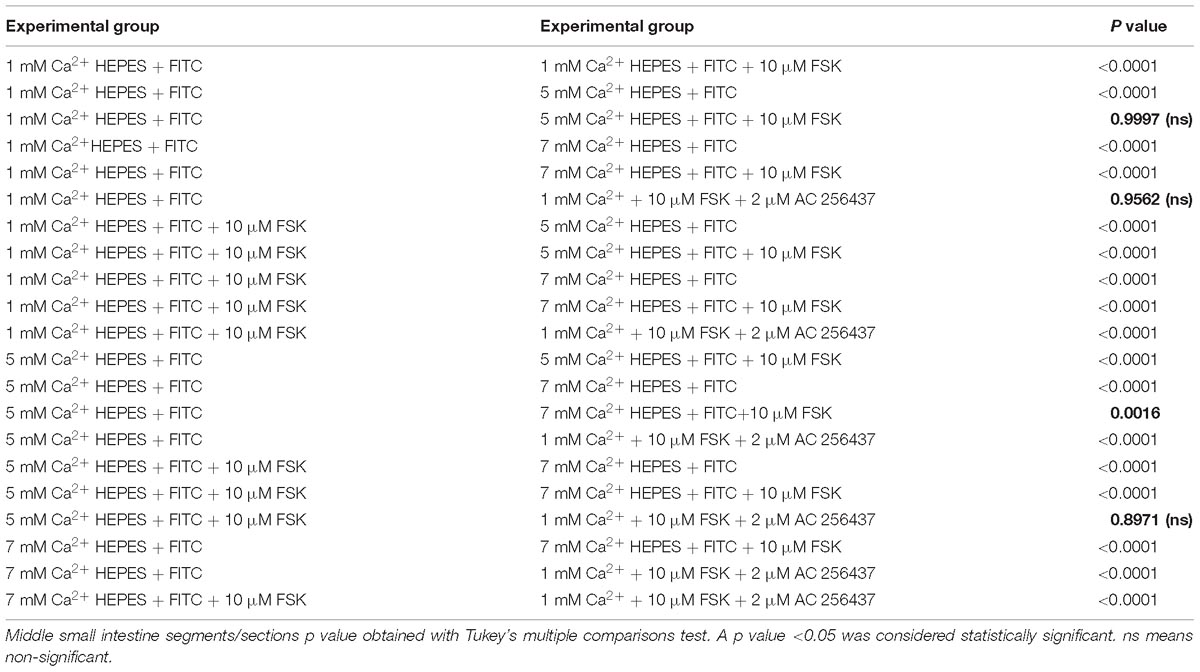
Table 5. Middle region of small intestine. P values of ΔFluorescence/Minute with the different [Ca2+] and FSK in comparison to AC-265347 with FSK.
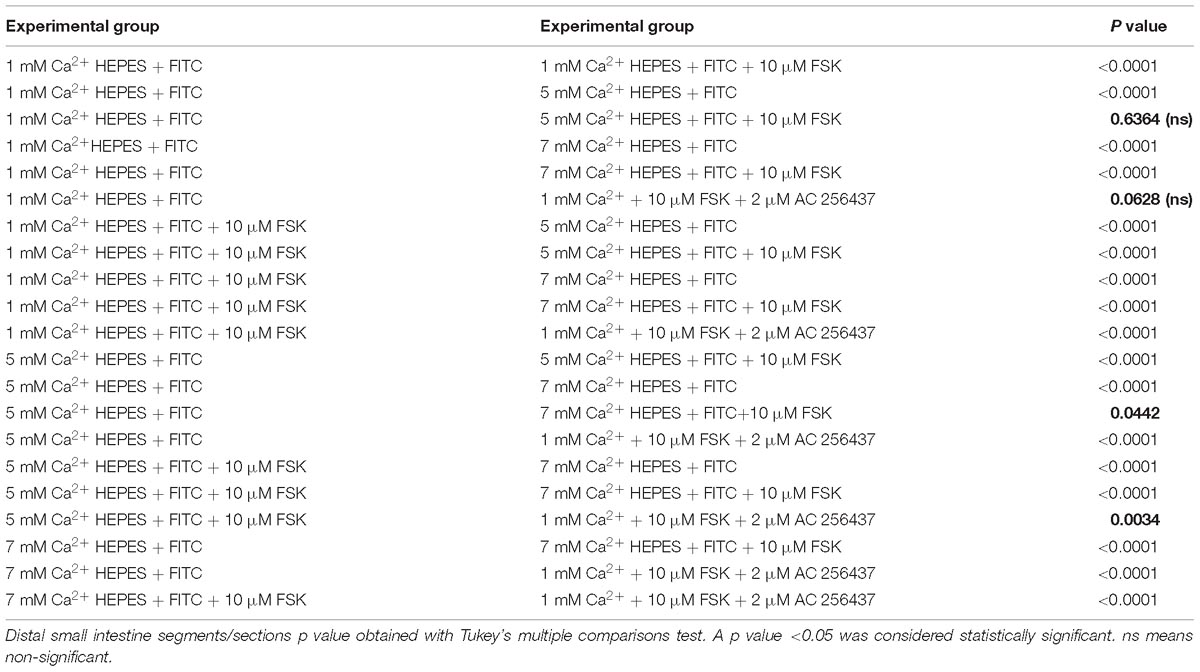
Table 6. Distal region of small intestine. P values of ΔFluorescence/Minute with the different [Ca2+] and FSK in comparison to AC-265347 with FSK.
Comparison With Calcimimetic AC-265347
In order to determine if the CaSR was being activated with Ca2+, the secretion profiles in all the intestinal regions were compared in the presence of control (1 mM of Ca2+), control with 10 μM FSK, control with 10 μM FSK and 2 μM AC-265347, and 5 mM Ca2+ with 10 μM FSK. There was no statistical significance between control and control with 10 μM FSK and 2 μM AC-265347, a CaSR agonist, in all regions as shown in Figure 3. The comparisons of the effects of 5 and 7 mM Ca2+ in abating secretagogue-induced fluid secretion to that of 2 μM AC-265347 in standard Ringer-HEPES buffer containing 1 mM Ca2+ demonstrated that intraluminal perfusion with 5 mM Ca2+ in the presence of 10 μM FSK was statistically similar to intraluminal perfusion with 2 μM AC-265347 with 1 mM Ca2+ in decreasing FSK-induced fluid secretion in the proximal and middle regions of the intestine (p = 0.6634, p = 0.8971, respectively), and more effective in the distal region (p = 0.0034). The reduction in secretion observed with 7 mM Ca2+ was larger when compared to the calcimimetic AC-265347 with 1 mM of Ca2+ in the middle and distal regions (p < 0.0001) and had a similar effect in the proximal region (p = 0.0004) as observed in Figure 4. The mean and SEM from each condition were graphed and Tukey’s multiple comparisons test provided the adjusted p value for the intestinal regions’ comparison. A detail of each comparison is shown in Tables 4–6.
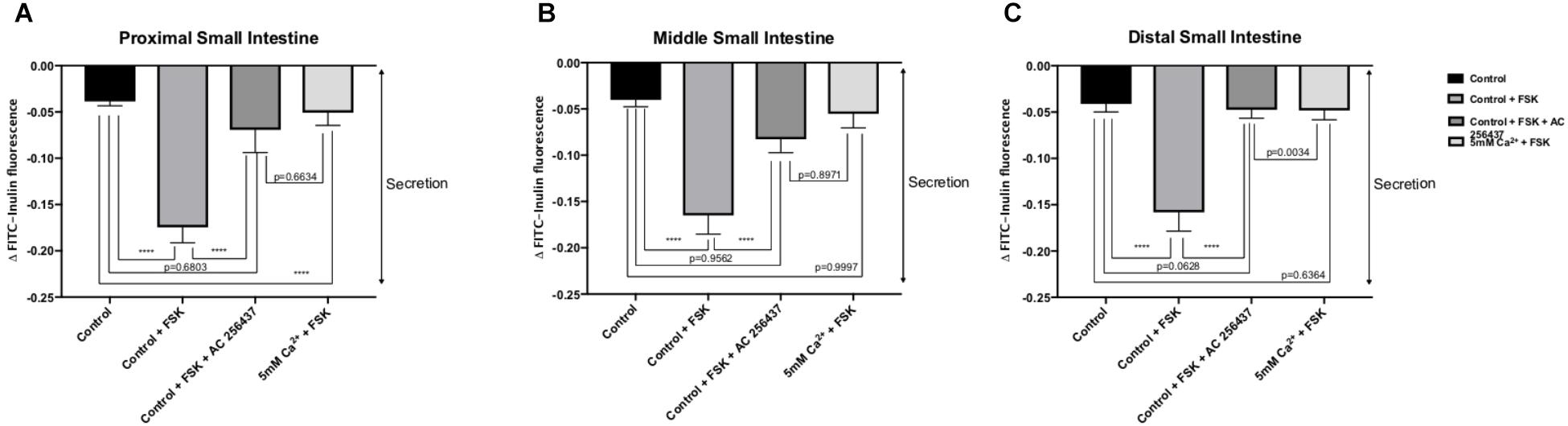
Figure 3. Comparison of the effect of intraluminal 1 mM [Ca2+] as control, 5 mM [Ca2+] and 2 μM AC-265347 with 1 mM [Ca2+] in reducing FSK-induced fluid secretion in the: (A) Proximal small intestine (B) Middle small intestine (C) Distal small intestine. P value < 0.0001 is represented by ∗∗∗∗.
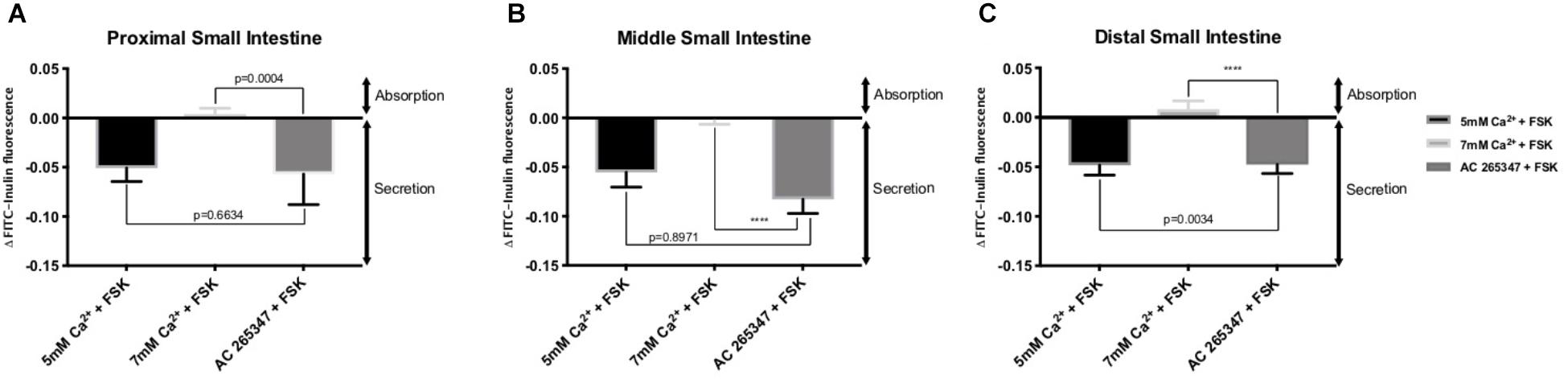
Figure 4. Comparison of the effect of intraluminal [Ca2+] and 2 μM AC-265347 in reducing FSK-induced fluid secretion in the: (A) Proximal small intestine (B) Middle small intestine (C) Distal small intestine. P value < 0.0001 is represented by ∗∗∗∗.
Discussion
This study provides evidence that activation of the CaSR by Ca2+ or a calcimimetic decreases intraluminal fluid secretion under physiological conditions. Increasing luminal calcium concentrations completely abates the secretory effect of the potent secretagogue FSK along the entire length of the rat small intestine. The receptor has been previously localized along the entire length of the mammalian gastrointestinal tract (Gama et al., 1997; Mitsuma et al., 1999; Cheng et al., 2002; Dufner et al., 2005; Kirchhoff and Geibel, 2006; Remy et al., 2007; Geibel, 2010), however, very few functional studies have been conducted on its role in fluid and electrolyte homeostasis in the small intestine. Using an ex vivo perfusion system that maintains the intestinal regions at 37°C throughout the experiment with a peristaltic flow, we can measure intestinal fluid secretion and absorption in real-time. As previously described, an increase in the fluorescence signal of FITC-inulin in the intraluminal perfusate corresponds to a decreased intraluminal fluid volume, which translates to absorption. In turn, a decrease in the fluorescence signal of FITC-inulin in the intraluminal perfusate responds to an increase in the intraluminal fluid. FITC-inulin fluorescence over time is thus indicative of intestinal luminal absorption or secretion (Munoz-Abraham et al., 2015).
The data obtained in the present study suggests that elevations in intraluminal Ca2+concentration can lead to a decrease in secretagogue induced fluid secretion and that further elevations in luminal Ca2+ concentration are associated with net fluid absorption via modulation in the CaSR located on the apical surface of the intestine. We confirm this hypothesis by application of a calcimimetic (AC-265347) with physiological Ca2+ concentration that results in decreased secretagogue induced fluid secretion comparable to a high Ca2+ perfusate. The observed effect of activating the CaSR established a dose-dependence and occurs along the entire length of the small intestine, with net fluid absorption occurring at a Ca2+ concentration of 7 mM in all intestinal regions (Figure 1). Accordingly, increasing the concentration of receptor ligand (Ca2+) increases the activity of the CaSR in modulating luminal fluid secretion, and at elevated levels of Ca2+ (7 mM) induces absorption of fluid.
Overstimulation of the secretory capacity of the intestinal tract results in secretory diarrhea, which is characterized by large stool volumes and is a leading cause of mortality amongst children and the elderly worldwide (Field, 2003; Schiller, 2009; Schiller et al., 2017). As such, there has been a search for therapies with the potential of directly inhibiting intestinal secretory mechanisms (Farthing, 2006). We thus sought to examine if activation of the CaSR by luminal Ca2+ would influence secretagogue-induced fluid secretion. FSK is a cyclic nucleotide activator that leads to an elevation of cAMP levels within intestinal epithelial cells and as such mimics the physiology of secretory diarrhea (Tang et al., 2016). As shown in Figure 2, increasing luminal Ca2+ concentration in the continued presence of FSK significantly decreases the secretory action of FSK in a dose-dependent manner. CaSR activation inhibits cyclic nucleotide activation and increases Na+-dependent absorption, thus inhibiting secretion and promoting fluid absorption. At a Ca2+ concentration of 7 mM, CaSR activation completely abolished FSK-induced fluid secretion along the small intestine. To confirm that the exposure to elevated luminal Ca2+ levels was via activation of the CaSR and not due to a non-targeted effect we exposed small intestinal segments to a physiological luminal Ca2+ concentration in the presence of the calcimimetic AC-265347 (Ma et al., 2011) (Figure 3). In the presence of FSK, intraluminal Ca2+ is just as effective as intraluminal AC-265347 in decreasing secretagogue-induced fluid secretion (Table 3 and Figure 3). In fact, 7 mM Ca2+ is significantly better than 2 μM AC-265347 in abrogating fluid secretion (Figure 4). These results confirm CaSR activation and are similar to what has been observed in the colon (Cheng, 2012; Tang et al., 2018).
The CaSR has recently provided a target for treatment of diarrheal illnesses, with the aim of activating it to induce its anti-secretory, pro-absorptive, anti-motility, and anti-inflammatory effects (Cheng, 2016). Human studies have shown that dietary Ca2+ improves resistance to enterotoxigenic Escherichia coli in young adults and ameliorates infectious diarrhea in immunocompromised children (Bovee-Oudenhoven et al., 2003; Cheng et al., 2013). Our findings corroborate these studies by providing direct evidence that the CaSR is functionally active along the entire length of the small intestine and acts as a modulator for fluid and electrolyte homeostasis. Additionally, we show that activation of the CaSR by Ca2+ leads to cessation of cyclic nucleotide-induced hypersecretion, and results in fluid absorption at higher luminal Ca2+ concentrations. These results not only provide a novel approach of Ca2+-based anti-secretory therapeutics, but also provides the possibility of correction of dehydration and nutrient absorption by increasing fluid absorption in secretory diarrheal maladies.
Author Contributions
MB was involved in conducting the experiments, statistical analysis, and writing of the manuscript. RM was involved in conducting experiments, writing, and editing of the manuscript. TL and MF were involved in conducting the experiments and writing the manuscript. GC was involved in the experimental design. VB was involved in editing of the manuscript. FD and DM were involved in experimental design, manuscript writing, and editing. JG was involved in all aspects of the experimental design, statistical analysis, manuscript writing, and editing.
Funding
The study was supported by the Charles Ohse Research Fund from the Yale Department of Surgery.
Conflict of Interest Statement
The authors declare that the research was conducted in the absence of any commercial or financial relationships that could be construed as a potential conflict of interest.
Abbreviations
AC-265347, 1-Benzothiazol-2-yl-1-(2,4-dimethyl-phenyl)-ethanol; CaSR, calcium-sensing receptor; FITC, fluorescein isothiocyanate; FSK, forskolin; GPCR, G-protein coupled receptor.
References
Alfadda, T. I., Saleh, A. M., Houillier, P., and Geibel, J. P. (2014). Calcium-sensing receptor 20 years later. Am. J. Physiol. Cell Physiol. 307, C221–C231. doi: 10.1152/ajpcell.00139.2014
AlKukhun, A., Caturegli, G., Munoz-Abraham, A. S., Judeeba, S., Patron-Lozano, R., Morotti, R., et al. (2017). Use of fluorescein isothiocyanate-inulin as a marker for intestinal ischemic injury. J. Am. Coll. Surg. 224, 1066–1073. doi: 10.1016/j.jamcollsurg.2016.12.016
Bovee-Oudenhoven, I. M., Lettink-Wissink, M. L., Van Doesburg, W., Witteman, B. J., and Van Der Meer, R. (2003). Diarrhea caused by enterotoxigenic Escherichia coli infection of humans is inhibited by dietary calcium. Gastroenterology 125, 469–476. doi: 10.1016/s0016-5085(03)00884-9
Breitwieser, G. E. (2013). The calcium sensing receptor life cycle: trafficking, cell surface expression, and degradation. Best Pract. Res. Clin. Endocrinol. Metab. 27, 303–313 doi: 10.1016/j.beem.2013.03.003
Brown, E. M., Gamba, G., Riccardi, D., Lombardi, M., Butters, R., Kifor, O., et al. (1993). Cloning and characterization of an extracellular Ca(2+)-sensing receptor from bovine parathyroid. Nature 366, 575–580. doi: 10.1038/366575a0
Brown, E. M., and MacLeod, R. J. (2001). Extracellular calcium sensing and extracellular calcium signaling. Physiol. Rev. 81, 239–297. doi: 10.1152/physrev.2001.81.1.239
Chattopadhyay, N. (2000). Biochemistry, physiology and pathophysiology of the extracellular calcium-sensing receptor. Int. J. Biochem. Cell Biol. 32, 789–804. doi: 10.1016/s1357-2725(00)00031-5
Chattopadhyay, N., Cheng, I., Rogers, K., Riccardi, D., Hall, A., Diaz, R., et al. (1998). Identification and localization of extracellular Ca(2+)-sensing receptor in rat intestine. Am. J. Physiol. 274, G122–G130.
Cheng, S. X. (2012). Calcium-sensing receptor inhibits secretagogue-induced electrolyte secretion by intestine via the enteric nervous system. Am. J. Physiol. Gastrointest. Liver Physiol. 303, G60–G70. doi: 10.1152/ajpgi.00425.2011
Cheng, S. X. (2016). Calcium-sensing receptor: a new target for therapy of diarrhea. World J. Gastroenterol. 22, 2711–2724. doi: 10.3748/wjg.v22.i9.2711
Cheng, S. X., Bai, H. X., Gonzalez-Peralta, R., Mistry, P. K., and Gorelick, F. S. (2013). Calcium ameliorates diarrhea in immune compromised children. J. Pediatr. Gastroenterol. Nutr. 56, 641–644. doi: 10.1097/mpg.0b013e3182868946
Cheng, S. X., Geibel, J. P., and Hebert, S. C. (2004). Extracellular polyamines regulate fluid secretion in rat colonic crypts via the extracellular calcium-sensing receptor. Gastroenterology 126, 148–158. doi: 10.1053/j.gastro.2003.10.064
Cheng, S. X., Okuda, M., Hall, A. E., Geibel, J. P., and Hebert, S. C. (2002). Expression of calcium-sensing receptor in rat colonic epithelium: evidence for modulation of fluid secretion. Am. J. Physiol. Gastrointest. Liver Physiol. 283, G240–G250. doi: 10.1152/ajpgi.00500.2001
Dufner, M. M., Kirchhoff, P., Remy, C., Hafner, P., Muller, M. K., Cheng, S. X., et al. (2005). The calcium-sensing receptor acts as a modulator of gastric acid secretion in freshly isolated human gastric glands. Am. J. Physiol. Gastrointest. Liver Physiol. 289, G1084–G1090.
Farthing, M. J. (2006). Antisecretory drugs for diarrheal disease. Dig. Dis. 24, 47–58. doi: 10.1159/000090308
Field, M. (2003). Intestinal ion transport and the pathophysiology of diarrhea. J. Clin. Invest. 111, 931–943. doi: 10.1172/jci18326
Flores, J., and Sharp, G. W. (1976). The activation of adenylate cyclase by cholera toxin: possible interaction with the nucleotide regulatory site. Ciba Found. Symp., 42, 89–108. doi: 10.1002/9780470720240.ch6
Gama, L., Baxendale-Cox, L. M., and Breitwieser, G. E. (1997). Ca2+-sensing receptors in intestinal epithelium. Am. J. Physiol. 273, C1168–C1175.
Geibel, J., Sritharan, K., Geibel, R., Geibel, P., Persing, J. S., Seeger, A., et al. (2006). Calcium-sensing receptor abrogates secretagogue- induced increases in intestinal net fluid secretion by enhancing cyclic nucleotide destruction. Proc. Natl. Acad. Sci. U.S.A. 103, 9390–9397. doi: 10.1073/pnas.0602996103
Kirchhoff, P., and Geibel, J. P. (2006). Role of calcium and other trace elements in the gastrointestinal physiology. World J. Gastroenterol. 12, 3229–3236.
Ma, J. N., Owens, M., Gustafsson, M., Jensen, J., Tabatabaei, A., Schmelzer, K., et al. (2011). Characterization of highly efficacious allosteric agonists of the human calcium-sensing receptor. J. Pharmacol. Exp. Ther. 337, 275–284. doi: 10.1124/jpet.110.178194
Macleod, R. J. (2013). CaSR function in the intestine: Hormone secretion, electrolyte absorption and secretion, paracrine non-canonical Wnt signaling and colonic crypt cell proliferation. Best Pract. Res. Clin. Endocrinol. Metab. 27, 385–402. doi: 10.1016/j.beem.2013.05.005
Mitsuma, T., Rhue, N., Kayama, M., Mori, Y., Adachi, K., Yokoi, Y., et al. (1999). Distribution of calcium sensing receptor in rats: an immunohistochemical study. Endocr. Regul. 33, 55–59.
Munoz-Abraham, A. S., Judeeba, S., Alkukhun, A., Alfadda, T., Patron-Lozano, R., Rodriguez-Davalos, M. I., et al. (2015). A new method to measure intestinal secretion using fluorescein isothiocyanate-inulin in small bowel of rats. J. Surg. Res. 197, 225–230. doi: 10.1016/j.jss.2015.02.049
Remy, C., Kirchhoff, P., Hafner, P., Busque, S. M., Mueller, M. K., Geibel, J. P., et al. (2007). Stimulatory pathways of the Calcium-sensing receptor on acid secretion in freshly isolated human gastric glands. Cell Physiol. Biochem. 19, 33–42. doi: 10.1159/000099190
Schiller, L. R. (2009). Diarrhea and malabsorption in the elderly. Gastroenterol. Clin. North Am. 30, 427–444. doi: 10.1016/s0889-8553(05)70189-8
Schiller, L. R., Pardi, D. S., and Sellin, J. H. (2017). Chronic diarrhea: diagnosis and management. Clin. Gastroenterol. Hepatol. 15, 182.e3–193.e3.
Sheinin, Y., Kallay, E., Wrba, F., Kriwanek, S., Peterlik, M., and Cross, H. S. (2000). Immunocytochemical localization of the extracellular calcium-sensing receptor in normal and malignant human large intestinal mucosa. J. Histochem. Cytochem. 48, 595–602.
Tang, L., Cheng, C. Y., Sun, X., Pedicone, A. J., Mohamadzadeh, M., and Cheng, S. X. (2016). The extracellular calcium-sensing receptor in the intestine: evidence for regulation of colonic absorption, secretion, motility, and immunity. Front. Physiol. 7:245. doi: 10.3389/fphys.2016.00245
Tang, L., Jiang, L., Mcintyre, M. E., Petrova, E., and Cheng, S. X. (2018). Calcimimetic acts on enteric neuronal CaSR to reverse cholera toxin-induced intestinal electrolyte secretion. Sci. Rep. 8:7851. doi: 10.1038/s41598-018-26171-4
Tang, L., Peng, M., Liu, L., Chang, W., Binder, H. J., and Cheng, S. X. (2015). Calcium-sensing receptor stimulates Cl(-)- and SCFA-dependent but inhibits cAMP-dependent HCO3(-) secretion in colon. Am. J. Physiol. Gastrointest. Liver Physiol. 308, G874–G883. doi: 10.1152/ajpgi.00341.2014
Tfelt-Hansen, J., and Brown, E. M. (2005). The calcium-sensing receptor in normal physiology and pathophysiology: a review. Crit. Rev. Clin. Lab. Sci. 42, 35–70. doi: 10.1080/10408360590886606
Tu, C. L., and Bikle, D. D. (2013). Role of the calcium-sensing receptor in calcium regulation of epidermal differentiation and function. Best Pract. Res. Clin. Endocrinol. Metab. 27, 415–427. doi: 10.1016/j.beem.2013.03.002
Keywords: CaSR, FITC-inulin, fluid homeostasis, forskolin, gastrointestinal tract
Citation: Barahona MJ, Maina RM, Lysyy T, Finotti M, Caturegli G, Baratta V, D’Amico F, Mulligan D and Geibel JP (2019) Activation of the Calcium Sensing Receptor Decreases Secretagogue-Induced Fluid Secretion in the Rat Small Intestine. Front. Physiol. 10:439. doi: 10.3389/fphys.2019.00439
Received: 16 October 2018; Accepted: 01 April 2019;
Published: 03 May 2019.
Edited by:
Richard T. Waldron, Cedars-Sinai Medical Center, United StatesReviewed by:
Mirajul Hoque Kazi, University of Maryland, United StatesJoel C. Bornstein, University of Melbourne, Australia
Copyright © 2019 Barahona, Maina, Lysyy, Finotti, Caturegli, Baratta, D’Amico, Mulligan and Geibel. This is an open-access article distributed under the terms of the Creative Commons Attribution License (CC BY). The use, distribution or reproduction in other forums is permitted, provided the original author(s) and the copyright owner(s) are credited and that the original publication in this journal is cited, in accordance with accepted academic practice. No use, distribution or reproduction is permitted which does not comply with these terms.
*Correspondence: Maria J. Barahona, maria.barahona@yale.edu John P. Geibel, john.geibel@yale.edu
†These authors have contributed equally to this work