- 1Max Planck Institute for Chemical Ecology, Jena, Germany
- 2UMR Evolution, Génomes, Comportement, Ecologie, CNRS, IRD, Univ Paris-Sud, Université Paris-Saclay, Gif-sur-Yvette, France
- 3AgroParisTech, Université Paris-Saclay, Paris, France
Herbivorous insects mainly rely on their sense of taste to decode the chemical composition of potential hosts in close range. Beetles for example contact and scan leaves with their tarsi, mouthparts and antennal tips, i.e., appendages equipped with gustatory sensilla, among other sensillum types. Gustatory neurons residing in such uniporous sensilla detect mainly non-volatile compounds that contribute to the behavioral distinction between edible and toxic plants. However, the identification of gustatory sensilla is challenging, because an appendage often possesses many sensilla of distinct morphological and physiological types. Using the specialized poplar leaf beetle (Chrysomela populi, Chrysomelidae), here we show that cuticular autofluorescence scanning combined with electron microscopy facilitates the identification of antennal gustatory sensilla and their differentiation into two subtypes. The gustatory function of sensilla chaetica was confirmed by single sensillum tip-recordings using sucrose, salicin and salt. Sucrose and salicin were found at higher concentrations in methanolic leaf extracts of poplar (Populus nigra) as host plant compared to willow (Salix viminalis) as control, and were found to stimulate feeding in feeding choice assays. These compounds may thus contribute to the observed preference for poplar over willow leaves. Moreover, these gustatory cues benefited the beetle’s performance since weight gain was significantly higher when C. populi were reared on leaves of poplar compared to willow. Overall, our approach facilitates the identification of insect gustatory sensilla by taking advantage of their distinct fluorescent properties. This study also shows that a specialist beetle selects the plant species that provides optimal development, which is partly by sensing some of its characteristic non-volatile metabolites via antennal gustatory sensilla.
Introduction
Plant-feeding insects often judge the suitability of potential hosts as food source or oviposition site by sensing various phytochemicals (Van Naters and Carlson, 2006; Agnihotri et al., 2016). While the olfactory system serves in attraction at a distance by identifying mainly volatiles, the gustatory system acts in close range by decoding mainly non-volatile phytochemicals (Chapman, 2003). Thus, gustation (taste, contact chemosensation) is used by phytophagous insects to determine host quality and initiate feeding (Ômura, 2018).
Upon arrival at the leaf surface, chrysomelid leaf beetles contact and scan leaves by extensive antennation, palpating and test biting, which constitutes a critical stage to final plant acceptance and rejection (Mitchell et al., 1999). The antennae, mouthparts and tarsi are equipped with contact gustatory sensilla, i.e., tiny cuticular, hair-like structures that house gustatory receptor neurons (GRNs) (Hallem et al., 2006; Zhang et al., 2016; Faucheux and Kundrata, 2017). Morphologically, insect gustatory sensilla have an apical pore (Isidoro et al., 1998; Hallberg and Hansson, 1999) allowing non-volatile phytochemicals to enter and dissolve into the aqueous sensillum lymph to reach and stimulate GRNs, ultimately leading to behavioral responses. However, since one appendage usually possesses a high number and different types of sensilla each comprising different sensory functions (e.g., chemo-, mechano-, hygro- thermosensory) (Hallberg and Hansson, 1999), the identification of taste sensilla is often challenging. Usually, scanning or transmission electron microscopy is used to identify putative pores and their position on each sensillum (Faucheux and Kundrata, 2017; Zhang et al., 2017). Taking advantage of distinct fluorescent properties of different sensilla types may provide the potential to distinguish among them by using fluorescence microscopy, which has so far mainly been tested on tarsal setae of ladybird beetles (Peisker et al., 2013). Nevertheless, additional electrophysiological recording analyses are required for functional characterisation of gustatory sensilla (e.g., Sollai et al., 2017).
Suitable host plants are chemically characterized by possessing compounds that act as behavioral stimulants and lacking those that act as behavioral deterrents (Ômura, 2018). Whereas plant primary metabolites such sucrose are often detected by antennal GRNs and thus stimulate feeding of different insect species, plant secondary metabolites are usually deterrent, growth inhibiting or even toxic to generalist insect herbivores (Isidoro et al., 1998; Chapman, 2003; Osier and Lindroth, 2006; Jørgensen et al., 2007; Alabi et al., 2014). Interestingly, the same secondary metabolites often stimulate feeding of specialists that have overcome and may even exploit host chemical defense (Bernays et al., 2000; Robert et al., 2012; Gray et al., 2015; Müller et al., 2015; Sollai et al., 2017). This pattern has been found for different insect species in case of salicinoids, which are phenolic glucosides typically found in Salicaceae plants (Fernandez and Hilker, 2007; Boeckler et al., 2011; Julkunen-Tiitto and Virjamo, 2016). One such intriguing example is the specialized poplar leaf beetle Chrysomela populi that specifically feeds on leaves of poplar species (Populus ssp.), an economically important tree known to contain various salicinoids (Gruppe et al., 1999; Boeckler et al., 2011). C. populi sequesters salicin for use in its own defense (Pasteels et al., 1983; Burse et al., 2009); however, it is unclear if and how non-volatile host salicinoids are tasted by the beetle, and if host primary metabolites such as sucrose or glucose play an additional role in host identification.
Here we take advantage of the distinct fluorescent properties of the different sensilla (sub)types on the antennae of a specialized phytophagous beetle and combine confocal laser scanning and electron microscopy, which facilitates identification of gustatory sensilla. The gustatory function of these sensilla chaetica was confirmed by electrophysiological tip recordings using sugars such as sucrose and salicin. Finally, we show that adult C. populi select a certain plant species which serves optimal weight gain and is partly due to tasting some of the host’s characteristic metabolites.
Materials and Methods
Biological Material
Poplar leaf beetles (C. populi, Linnaeus, Chrysomelidae, Coleoptera, Insecta) were collected in the north of Jena close to Dornburg-Camburg (+51°00′52′′, +11°38′17′′), Germany and reared in a climate chamber provided with fresh twigs of poplar under a 16 h:8 h light:dark cycle at 20°C. For experiments, beetles between three and 15 days old were used. Poplar (Populus nigra) and willow (Salix viminalis) plants, both species belonging to the Salicaceae family, were grown in the institute’s green house. Leaves of P. nigra are usually considered as main host of C. populi, whereas S. viminalis leaves are preferred by other leaf beetle species such as Chrysomela saliceti, Weise and Plagiodera versicolora (Laicharting) (Gök and Çilbiroğlu, 2005; La Spina et al., 2010).
Fluorescence Imaging
Antennae were dissected from cold anesthetized adults of C. populi (n = 4), briefly cleaned in an Ultrasonic Cleaner (VWRTM) and mounted in glycerol (G9012 Sigma-Aldrich) in two cover slips, allowing microscopic observation of the distal antennal tip from both sides. Autofluorescence scanning images were acquired on a confocal laser scanning microscope (LSM 880, Zeiss, Oberkochen, Germany) using a 40×/1.2 W C-Apochromat. Excitation of cuticular autofluorescence was conducted by a 405 nm laser diode and the fitting main beam splitter 405. Emission was detected in Lambda mode between 410 and 695 nm to discriminate between high and low concentrations of resilin or chitin, respectively. Approximately 300 scans at a thickness of 0.5 μm were employed to image the terminal (9th) flagellomere of the antennae from both sides. Maximum intensity projections were used for visualization of the entire image stacks. Images were processed using ZEN software (Zeiss) and ImageJ (Schneider et al., 2012).
Electron Microscopy
Scanning electron microscopy was employed to identify morphological details of antennal sensilla, e.g., an apical pore. Three days old beetles were fixed with 2.5% (v/v) glutaraldehyde in cacodylate buffer for 60 min. Afterwards the samples were washed three times for 10 min with cacodylate buffer and dehydrated in ascending ethanol concentrations (30, 50, 70, 90, and 100%) for 20 min each. Subsequently, the samples were critical-point dried using liquid CO2 and sputter coated with platinum (thickness approximately 8 nm) using a CCU-010 sputter coater (safematic GmbH, Bad Ragaz, Switzerland) to avoid surface charging. Finally, the specimens were investigated with a field emission scanning electron microscope LEO-1530 Gemini (Carl Zeiss Microscopy GmbH, Jena, Germany) at 8–12 kV.
Electrophysiological Recordings
Electrophysiological recordings were obtained from GRNs in s. chaetica subtypes 1 and 2 found on the terminal flagellomere of adult C. populi beetles using single sensillum tip recordings (Hodgson et al., 1955). Live beetles were immobilized on a microscope slide using adhesive pads (UHU® Patafix, Bolton Adhesives). The recording electrode (a thin-walled borosilicate glass capillary, World Precision Instruments) was pulled in a two-step electrode puller (PP-830, Narishige Group, Japan) to a tip diameter of approximately 5 μm. The recording electrode containing the test solution was placed over single sensilla during 2–3 s using a motorized piezo-micromanipulator. The following tastants, all freshly dissolved in 30 mM KCl and obtained from Sigma-Aldrich, were used: sucrose (1, 100 and 300 mM; n = 9 insects), fructose (100 and 300 mM; n = 4 insects) and salicin (100 mM, n = 2); as positive control 30 mM KCl were used (n = 3). Each stimulus was applied twice, i.e., with a time interval of 30 s to avoid adaptation. The grounded reference electrode was a 1 mm diameter silver chloride wire placed into the beetle’s abdomen and moved carefully as close as possible to the head and antennae. The recording electrode was connected to a TastePROBE amplifier [10×, Syntech (Marion-Poll and Van Der Pers, 1996)] and the signals were further amplified and filtered (high pass 10 Hz, low pass 3000 Hz) using a CyberAmp 320 amplifier (Axon Instruments, Burlingame, CA, United States). Signals were digitized and analyzed by counting all spikes occurring during the first second of recording using dbWave (Marion-Poll, 1996).
Metabolite Extraction, Identification and Quantification
For metabolite extraction, 1 g fresh leaf from P. nigra (n = 6 plants) or S. viminalis (n = 3 plants) was ground in 3 ml aqueous 80% MeOH using clean mortar and pestle under the addition of liquid nitrogen and acid washed sand (silicon dioxide, 18649 Supelco, Sigma-Aldrich) to maximize homogenisation of plant tissue. After centrifugation at 15,000 g for 5 min at 4°C to pellet debris, the supernatant was filtered through a 0,22 μm polyvinylidene fluoride membrane (Durapore®, GVWP04700, Merck) by an additional centrifugation step and used for analysis via high pressure liquid chromatography coupled to mass spectrometry (LC-MS) and gas chromatography coupled to mass spectrometry (GC-MS). In the latter case, the filtered supernatant was evaporated in a vacuum centrifuge (EppendorfTM Concentrator plus F-45-48-1) at 45°C for 30 min and derivatized by adding 50 μl N-Methyl-N-(trimethylsilyl) trifluoroacetamide and pyridine at 60°C for 30 min. The sample was diluted 1:2 with dichloromethane. LC-MS was carried using an Agilent HP1100 HPLC system equipped with a Purospher® STAR RP-18 endcapped (5 μm) column coupled to a FinniganTM LTQTM (Thermo Electron Corporation, Dreieich, Germany) mass spectrometer operated in the atmospheric pressure chemical ionization mode with a vaporizer temperature of 450°C. Samples were analyzed by injection of 5 μl. The gradient elution (90:10) consisted of water with 0.1% formic acid as solvent A and acetonitrile with 0.1% formic acid as solvent B; the final flow rate was 1 ml per minute at a maximal pressure of 230 bar. For identification and quantification of salicin (S0625 Sigma) via a standard curve the formic acid adduct [M + HCOOH - H]- of salicin with m/z 331 was used. GC-MS analysis was used for identification and quantification of sucrose (S0389 Sigma), glucose (G8270 Sigma) and fructose (4981 Roth). It consisted of a ThermoQuest TRACE GC 2000 connected to TRACE MS (Thermo Finnigan) equipped with a ZB5 column and a 10-m guard column (Phenomenex, Torrance, CA, United States). Mass spectra were measured in electron impact mode at 70 eV, 33–450 m/z. Volatiles were eluted under programmed conditions: 40°C (2 min isotherm), followed by heating at 10°C per min to 220°C and at 30°C per min to 280°C, using helium (1.5 ml/min) as the carrier gas. The GC injector (split ratio 1:7), transfer line, and ion source were set at 220, 280, and 200°C, respectively. For each compound, statistic differences of the concentration between poplar and willow leaves were calculated via Mann–Whitney Rank Sum test using SigmaPlot (Systat Software, Erkrath, Germany).
Feeding Choice Assays
Beetles were starved 24 h before the experiment and allowed to feed for 24 h. Feeding choice assays using leaf disks (cut with a cork borer to a diameter of 13 mm) from P. nigra and S. viminalis were carried out by placing four disks of each species in an alternating order, mounted on a needle, in a Petri dish (diameter 9 cm) with moist filter paper. One beetle was used per Petri dish (n = 5). In addition, baking wafers (wheat flour based, Kuchle Back Oblaten Round, 50 mm) were used as neutral substrate to test feeding initiation triggered by the presence of sucrose or salicin (dissolved in distilled water at 500 mM) in comparison to water controls. Therefore, 50 μl test substance were pipetted onto a wafer divided into eights and put in a plastic box (with fine mesh on lid, 20 cm × 20 cm × 6.5 cm) together with six individual beetles (n = 3). Feeding damage on the four leaf disks and wafers per arena was measured digitally using ImageJ (Schneider et al., 2012) by comparing the area before and after the experiment; values were converted into percentage terms. Statistic differences were calculated using t-test (including equal variance test) for leaf disk assays or one-way analysis of variance (ANOVA, including equal variance and Shapiro–Wilk normality test) for wafer assays using SigmaPlot 12.
Weight Gain Assays
Chrysomela populi were reared on a no-choice diet using twigs with leaves of either P. nigra or S. viminalis. Weight was measured regularly for 12 days (excluding the pupal period) from seven beetles per group from the larval to the adult stage in mg. Each individual was kept in a single plastic box (with fine mesh on lid, 10 cm × 10 cm × 6.5 cm); fresh twigs with leaves were supplied daily. Significant differences between the two groups with respect to individual weight gain was analyzed with a two-way repeated measurement analysis of variance (RM-ANOVA, including equal variance and Shapiro–Wilk normality test) using SigmaPlot 12.
Results
Sensilla Morphology
The antenna of adult C. populi consisted of a scape, a pedicel and a flagellum with 9 subsegments, i.e., flagellomeres (Figure 1A; compare also to Figure 9 in Ge et al., 2015). Sensilla chaetica on the 9th and apical flagellomere represented the longest sensilla type with a mean length of 67.8 μm ± 5.8 s.e.m. (Figure 1B). Using autofluorescence scanning, the s. chaetica could be distinguished from other sensilla types such as short sensilla basiconica or styloconic or thin-walled trichoid sensilla (Figure 1B). Moreover, there were two subtypes of s. chaetica as shown by their distinct fluorescent properties, especially in the more distal parts, resulting in blue (subtype 1) or green (subtype 2) fluorescence as indicated by lambda scanning (Figure 1C). Analysis by scanning electron microscopy showed that the blunt tips of s. chaetica subtypes 1 and 2 have different morphologies, especially with respect to their pore types (Figure 1D).
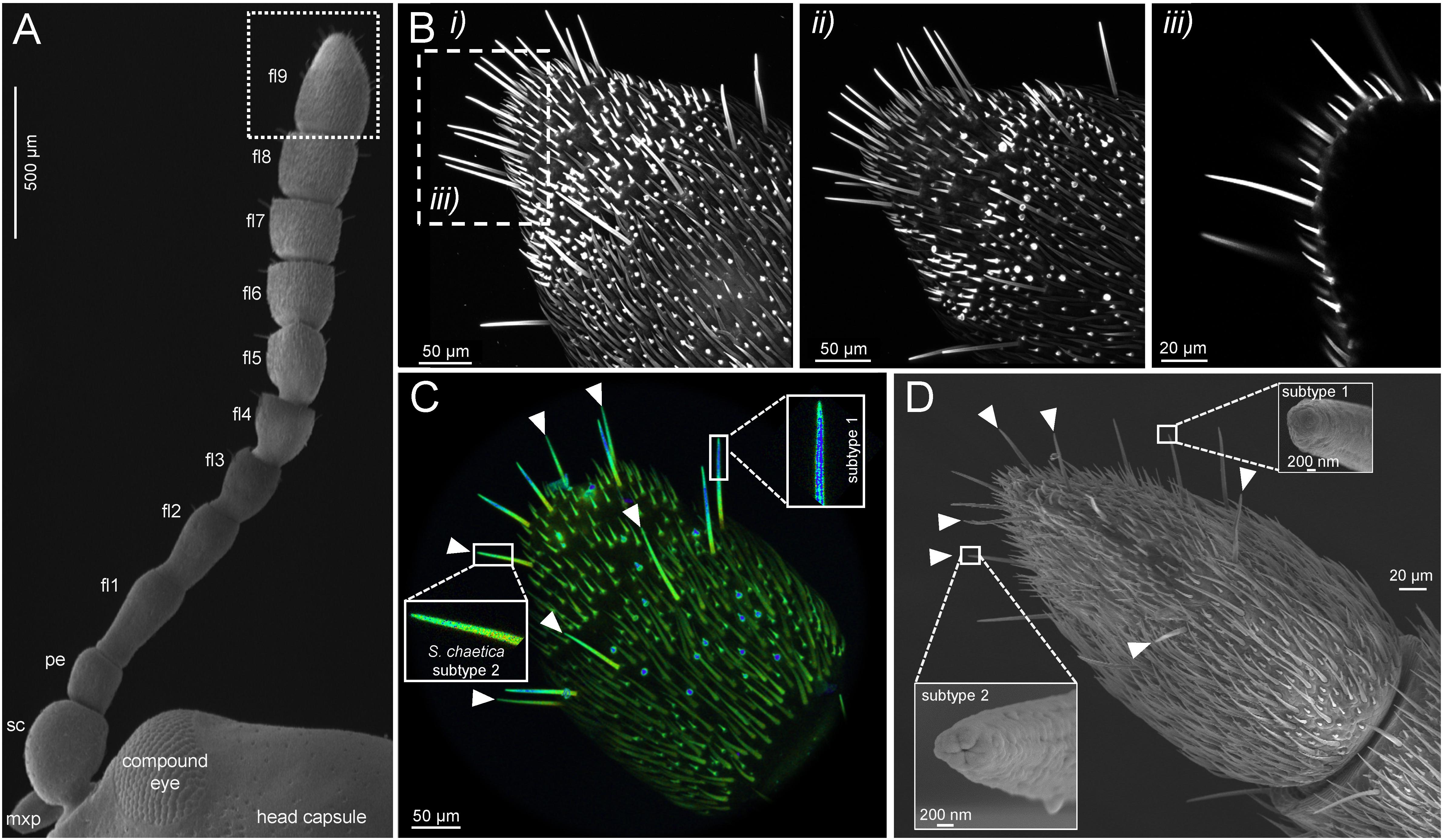
Figure 1. Distinct autofluorescent properties among different antennal sensilla types facilitate identification of gustatory sensilla chaetica. (A) The antenna of adult C. populi consists of scapus (sc), pedicellus (pe) and nine flagellomeres (fl) of which the apical flagellomere was the focus of this study (fl9, dotted frame); mxp - maxillary palp; dorsal view by scanning electron microscopy. (B) Cuticular autofluorescence scanning by confocal laser microscopy showing one stack (consisting of 300 scans at 0.5 μm thickness) from the (i) dorsal and (ii) ventral side; (iii) single scan from i) as indicated by dashed frame. (C) Lambda scan indicates two subtypes of s. chaetica due to their distinct fluorescence (subtype 1 in blue; subtype 2 in green with arrowheads). (D) Scanning electron microscopy reveals a blunt end of both s. chaetica subtypes, but different terminal pore types between subtype 1 and 2 (arrowheads).
Confirmation of the Gustatory Function of Sensilla Chaetica
Using single sensillum recordings, the spike activity of GRNs was recorded from s. chaetica subtypes 1 and 2 at the antennal tip, i.e., the apical flagellomere (Figures 1A, 2) with sucrose, fructose, salicin and KCl. Using 30 mM KCl that served as positive control and solvent for tastants, mean frequency of s. chaetica subtype 2 was 16.7 Hz ± 1.3 s.e.m. (Figure 2). Responses of s. chaetica subtype 2 using fructose at 100 and 300 mM were below this control threshold and did not increase with increasing ligand concentration, i.e., 15.3 Hz ± 2.0 s.e.m. and 15.6 Hz ± 3.2 s.e.m., respectively (Figure 2). However, when using sucrose at 1, 100, and 300 mM, spikes from GRNs in s. chaetica subtype 2 were on average 19.3 Hz (±5.0 s.e.m.), 24.3 Hz (±7.8 s.e.m.), and 35.8 Hz (±5.5 s.e.m.), respectively (Figure 2). Finally, using salicin at 100 mM, spike discharges were recorded from s. chaetica subtype 2 at 19.6 Hz (±2.3 s.e.m.). In contrast, s. chaetica subtype 1 did not respond to any of the tested tastants.
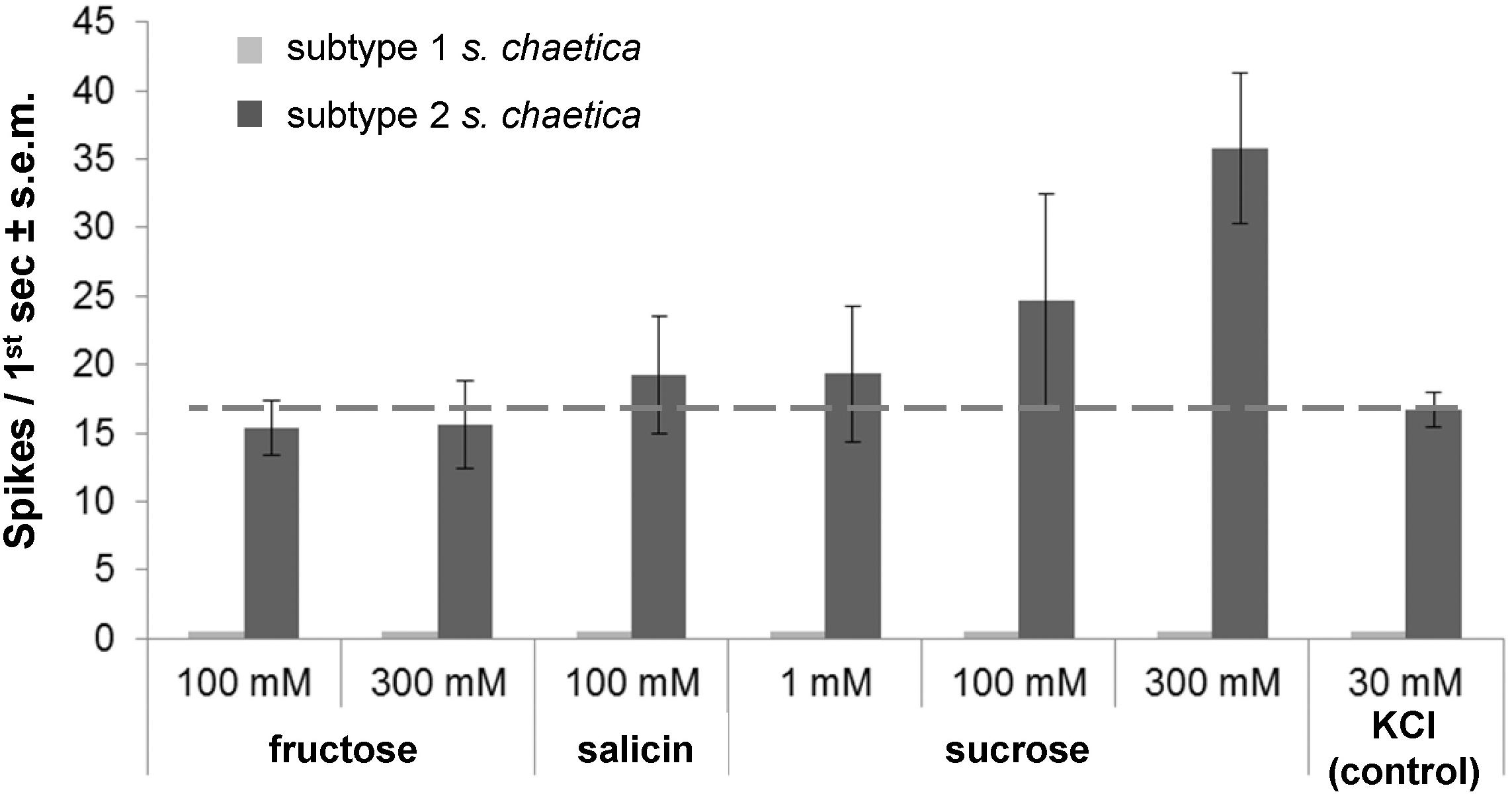
Figure 2. Gustatory receptor neurons in antennal sensilla chaetica of subtype 2 respond to salicin, sucrose and salt. Mean spike frequency toward different tastants (dissolved in 30 mM KCl) at different concentrations; 30 mM KCl as control; n ≥ 3. Subtype 1 s. chaetica did not respond to any of the tastants. Dashed line indicates background activity of gustatory neurons due to 30 mM KCl in tastant solution.
Primary and Secondary Metabolites in Leaves
Using LC-MS analysis, methanolic leaf extracts of P. nigra were found to contain 3.0 mg (±0.7 s.e.m.) of salicin per g leaf fresh mass, whereas in S. viminalis salicin was not detectable (Figure 3). Using GC-MS on the same extracts, sucrose was found to be approximately 25 times higher concentrated in P. nigra (104.1 mg ± 11.0 s.e.m. per g leaf fresh mass) than in S. viminalis (4.2 mg ± 3.0 s.e.m. per g leaf fresh mass). P. nigra leaves contained 1.6 mg (±0.02 s.e.m.) of glucose per gram leaf fresh mass, whereas glucose was not detectable in S. viminalis leaves (Figure 3). Each of these three compounds differed significantly between P. nigra and S. viminalis (P < 0.05). Fructose had the lowest concentration in P. nigra leaves (0.3 mg ± 0.2 s.e.m. per g leaf fresh mass) compared to the other saccharides tested, whereas in S. viminalis fructose was even lower concentrated (0.04 mg ± 0.01 s.e.m. per g leaf fresh mass).
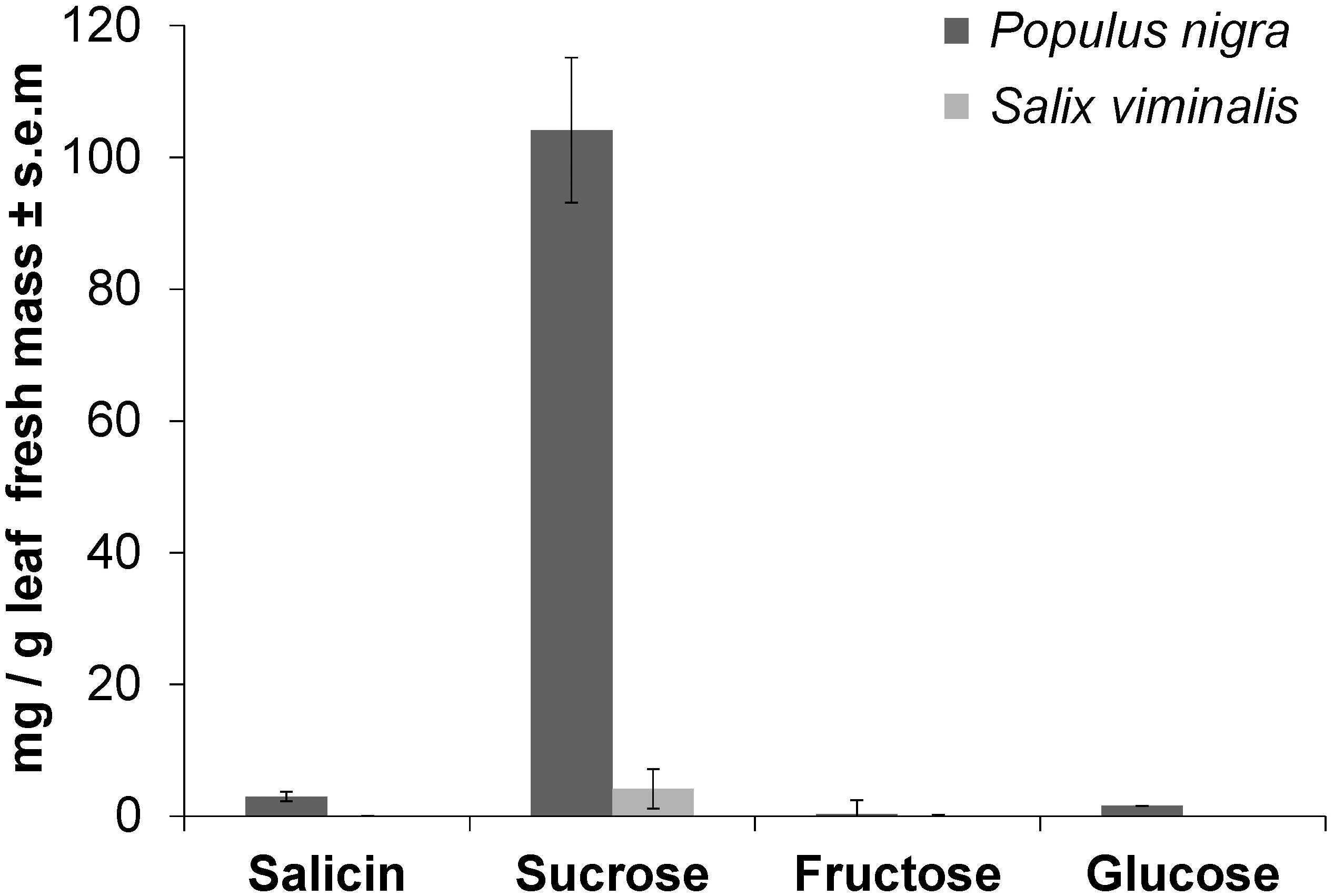
Figure 3. Sucrose and salicin are major compounds in leaves of Populus nigra, but less concentrated in Salix viminalis. Mean concentration of salicin and different saccharides in leaves of P. nigra (n = 6) or S. viminalis (n = 3) as measured by liquid chromatography or gas chromatography coupled to mass spectrometry after extracting fresh leaves in methanol. Authentic standards were used for identification and quantification.
Feeding Choice and Weight Gain Assays
Feeding choice assays using leaf disks indicated significant feeding preference of C. populi for P. nigra over S. viminalis (P < 0.005); beetles consumed on average 55.3% ± 14.4 s.e.m. of the provided P. nigra leaf material, but did not feed on S. viminalis (0% feeding damage) if given the choice between these two plant species (Supplementary Figure S1A). Feeding choice assays using baking wafers supplemented with single ligands (sucrose or salicin at 500 mM) indicated significant feeding preference for sucrose (13.0% ± 2.6 s.e.m. feeding damage) over water controls (0.5% ± 0.3 s.e.m.; P = 0.03), but not over salicin (7.1% ± 3.2 s.e.m.) (Supplementary Figure S1B). Weight gain assays of C. populi using no-choice diets with leaves of P. nigra or S. viminalis resulted in significantly higher weight gain of those beetles that were reared on P. nigra compared to S. viminalis leaves (Figure 4).
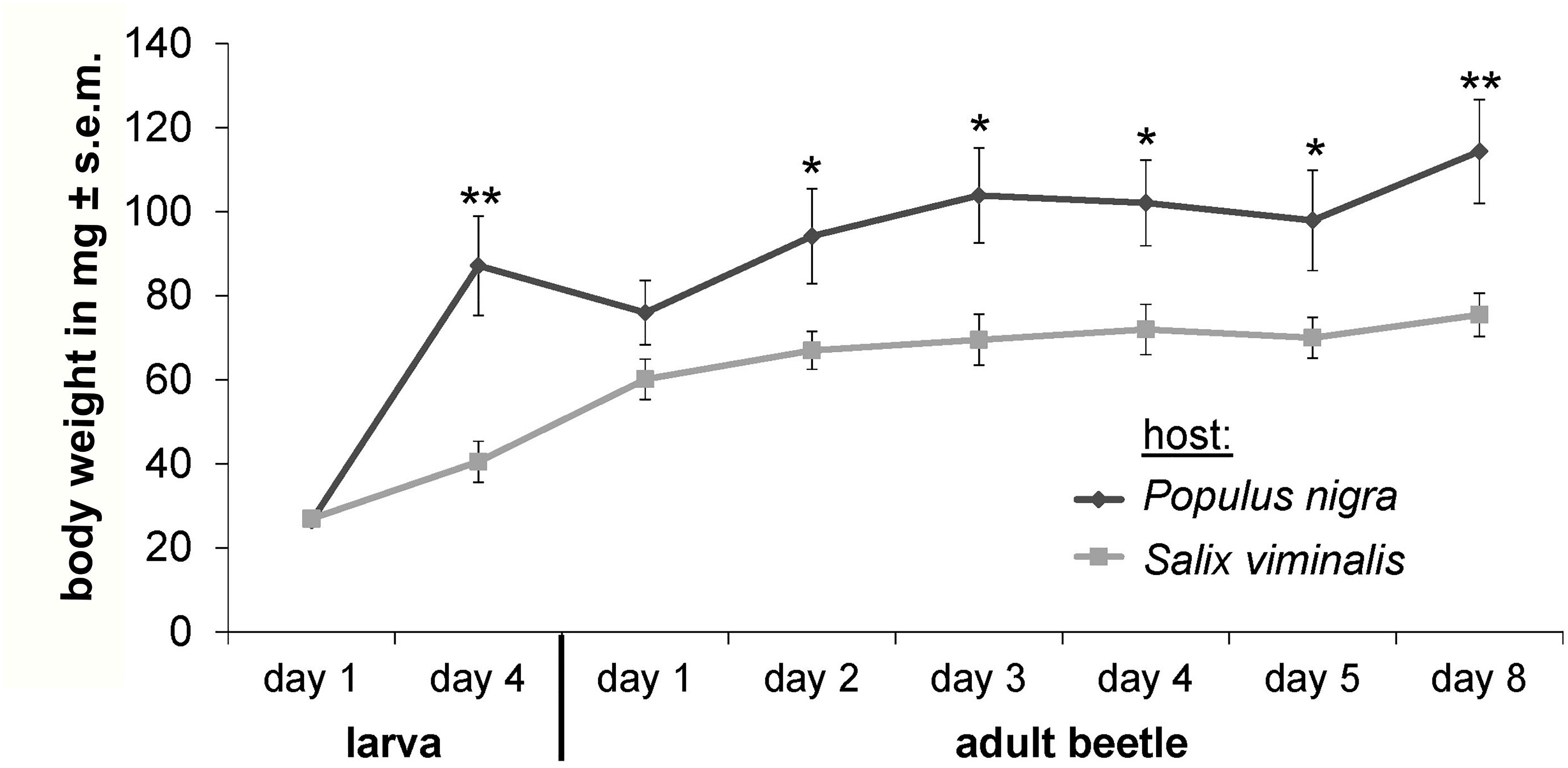
Figure 4. Weight gain of C. populi depends on host plant species. Using no-choice diet with leaves from P. nigra or S. viminalis species shows that individual poplar leaf beetles gain significantly more weight over time on P. nigra (n = 7) compared to S. viminalis (n = 7); Two Way Repeated Measurement ANOVA with pairwise multiple comparison (Holm–Sidak method). ∗P < 0.05, ∗∗P < 0.01.
Discussion
In phytophagous insects, the main role of the peripheral gustatory system is to provide information about the suitability of plants as food source or oviposition site mainly by sensing compounds that are nutritional, i.e., feeding stimulating, or potentially harmful, i.e., feeding deterrent (Sollai et al., 2014; Pentzold et al., 2017). The plant’s set of primary and secondary metabolites plays a key role in this interaction, since they modulate insect behavior in diverse ways from deterrence to attraction to addiction (Ômura, 2018; Wink, 2018). In turn, insect herbivores sense the majority of non-volatile metabolites via gustation, which requires direct contact with the plant material, finally providing accurate and reliable information about host suitability and identification (Chapman, 2003; Heisswolf et al., 2007; Bustos-Segura and Foley, 2018). In most chrysomelid beetles for example, during antennation of the leaf, gustatory sensilla would be able to detect phytochemicals, present in the surface waxes or the epidermis allowing the beetle to gain information on the suitability of the plant without biting it (Isidoro et al., 1998). However, studying insect gustation at the morphological and physiological level is often hampered by the difficulty of identifying putative gustatory sensilla, because most appendages possess many individual and distinct functional types of sensilla (Hallberg and Hansson, 1999; Faucheux and Kundrata, 2017). To facilitate identification of sensilla involved in contact chemosensation, here we take advantage of the distinct fluorescent properties among the different sensilla types present on the beetle’s antennal tip and confirm their taste responsiveness via electrophysiological recordings using sucrose and salicin. Finally, we show that behavioral choice via contact chemosensation of these cues may contribute to feeding on the host that provides optimal weight gain for poplar leaf beetles.
Autofluorescence scanning of the antennal tips from C. populi has identified sensilla of the type chaetica (Figure 1C). They are distinct from other sensilla types such as basiconica, styloconic, and trichoid sensilla usually present on a beetle’s antennae (Hallberg, 1982). For example, s. chaetica possess the highest amount of cuticular expression, thus they are long, heavy and thick-walled (Ryan, 2002). Similar to other chrysomelid beetle species, s. chaetica in C. populi were the longest antennal sensilla protruding above all other and are therefore likely involved in contact chemosensation (Isidoro et al., 1998; Zhang et al., 2013, 2016). For example, s. chaetica of the cabbage stem flea beetle Psylliodes chrysocephala present on terminal flagellomeres respond to host plant-derived taste stimuli such as the non-volatile glucobrassicin (Isidoro et al., 1998). This glucosinolate was also the most effective at stimulating feeding by P. chrysocephala (Bartlet et al., 1994). Sensilla chaetica seem to be the only contact chemosensillar type on the antennae of P. chrysocephala (Isidoro et al., 1998) although in the flour beetle Tribolium brevicornis a row of small s. basiconica on the terminal flagellomere respond to sucrose and NaCl (Alabi et al., 2014). In the lepidopteran Heliothis virescens GRNs in antennal s. chaetica respond toward sucrose, inositol, salts and bitter substances (Jørgensen et al., 2007). In general, antennal s. chaetica in C. populi were quite low in their number (14 on the terminal flagellomere) in comparison to other sensilla types, which is similar to other beetle species (Bartlet et al., 1999; Faucheux and Kundrata, 2017).
In some coleopteran species there are two to three distinct subtypes of s. chaetica on the antennae depending on the species as shown via electron microscopy (Faucheux and Kundrata, 2017; Zhang et al., 2017). Similarly, there were two subtypes of s. chaetica on the terminal antennal flagellomere of C. populi, which was revealed in this study using confocal microscopy (Figures 1B,C). Taking advantage of their distinct fluorescent properties by lambda scanning, s. chaetica of subtype 1 were identified by their pronounced blue fluorescence, especially in the more distal part, whereas subtype 2 showed green fluorescence equally distributed along the sensilla. The differences in fluorescence between the two subtypes are likely due to different amounts in resilin and chitin, respectively. For example, the adhesive tarsal setae of the ladybird beetle Coccinella septempunctata have a pronounced longitudinal material gradient with high concentrations of the elastic protein resilin (blue autofluorescence) in the tips, whereas the less flexible central and proximal parts of the setae mainly consist of other materials such as chitin indicated by green autofluorescence (Peisker et al., 2013). Therefore, subtype 1 s. chaetica in C. populi could be envisioned as being more flexible than subtype 2 due to higher proportions of resilin, whereas subtype 2 (to agree with the definition of Faucheux and Kundrata, 2017) seems stiffer due to its higher proportion of chitin. These findings further stress the potential of fluorescence and confocal microscopy for visualizing morphological details of external insect structures (Michels and Gorb, 2012) to facilitate the elucidation of distinct material compositions between sensilla subtypes. Furthermore, fluorescence microscopy as described here requires less time for sample preparation than scanning or transmission electron microscopy.
The presence of distinct subtypes of blunt-ended s. chaetica in C. populi is similar to other beetle species and usually related to a contact chemosensory function (Bartlet et al., 1999; Faucheux and Kundrata, 2017). For example, s. chaetica of subtype 3 in elm leaf beetles possess an apical pore and a blunt tip indicating gustatory function (Zhang et al., 2017). Thus, those s. chaetica in C. populi that were found to possess a clear terminal pore (subtype 2) (Figure 1D), did respond electrophysiologically to host tastants such as sucrose and salicin as well as KCl, whereas subtype 1 did not evoke these responses (Figure 2). The presence of an apical pore is usually characteristic for contact chemosensation, and required for non-volatile plant-derived compounds to enter into the sensillum, dissolve into lymph to reach and stimulate GRNs ultimately mediating behavioral responses (feeding or rejection). Recordings on s. chaetica subtype 1 may evoke physiological responses when using other compounds than those tested. Other salicinoids or condensed tannins would be candidates since they are abundantly present in poplar (Barbehenn and Constabel, 2011); this question requires future investigations. That no electrophysiological responses toward fructose could be obtained from s. chaetica subtype 2 was consistent with the apparent lack of fructose in the host plant (Figure 3). Thus, fructose is either of little or no importance for sensation in C. populi or, alternatively, it is sensed by gustatory s. chaetica on mouthparts and tarsi as putatively present in other beetles such as Tribolium castaneum (Seada and Hamza, 2018). In any case, functional characterisation of single gustatory receptor genes warrants future investigation to reveal the number of putative ligands. In contrast to fructose, sucrose and salicin were highly concentrated in P. nigra leaves (Figure 3). Correspondingly, in feeding choice assays P. nigra leaves were clearly preferred over S. viminalis leaves (Supplementary Figure S1) that contained 25 times less sucrose and lacked salicin. Also other studies indicate that C. populi prefer to feed on salicaceous species with relatively high concentrations of phenolic glucosides such as salicin in the leaves (Ikonen, 2002); similarly, adults of Chrysomela aeneicollis were stimulated to feed by salicin itself (Rank, 1992). It is conceivable that the metabolic differences between P. nigra and S. viminalis contributed to the observed significant differences in weight gain over time when C. populi beetles were reared on either plant species (Figure 4). Thus, sucrose seems a feeding stimulant, while salicin seems to contribute to feeding initiation, potentially promoting recognition of P. nigra leaves by contact and serving increased weight gain during development of C. populi.
Overall, the combined analysis of autofluorescent properties and morphological details of different sensilla types on the beetle’s antenna facilitated the identification of those sensilla with gustatory function. In case of s. chaetica subtype 2 from C. populi, beetles physiologically sensed major primary and secondary metabolites from its host plant with their antennal tip via contact chemoreception promoting taste selection of those plant species that provide a nutritional basis for optimal development. However, since the most obvious secondary compounds found in an insect’s host plant may not be the only or even the primary basis of host recognition (Mitchell et al., 1999), future experiments should take into account the structural diversity of (poplar) plant metabolites as well as additional gustatory sensilla likely present on other chemosensory appendages such as mouthparts and tarsi. This will help to elucidate which sensillum responds to which phytochemical and how this influences the insect’s host selection and feeding behavior.
Data Availability
All datasets generated for this study are included in the manuscript and/or the Supplementary Files.
Author Contributions
SP and AB contributed conception and design of the study. SP, FM-P, and VG carried out the experiments. SP wrote the first draft of the manuscript. All authors contributed to manuscript revision, read and approved the submitted version.
Funding
This project has received funding from the European Union’s Horizon 2020 Research and Innovation Programme under the Marie Skłodowska-Curie grant agreement no. 705151. Further financial support from the Max Planck Society is acknowledged.
Conflict of Interest Statement
The authors declare that the research was conducted in the absence of any commercial or financial relationships that could be construed as a potential conflict of interest.
Acknowledgments
We would like to thank Dr. Alexander Haverkamp, Johannes Krenn, and Nicole Ullrich for their assistance with single sensillum recordings. We would also like to thank PD Dr. Martin Westermann and Susanne Linde (Elektronenmikroskopisches Zentrum Jena) for their help using the scanning electron microscope.
Supplementary Material
The Supplementary Material for this article can be found online at: https://www.frontiersin.org/articles/10.3389/fphys.2019.00343/full#supplementary-material
FIGURE S1 |(A) Exemplary two-choice feeding assay using leaf disks of poplar (p) Populus nigra and willow (w) Salix viminalis indicates highly significant feeding preference of adult C. populi for poplar over willow (∗∗∗P < 0.005; t-test); n = 5. (B) Exemplary feeding choice assay using wafers soaked with sucrose or salicin at 500 mM to test feeding damage (arrowheads) by C. populi; n = 3. Significant feeding preferences were found for sucrose over water solvent controls (∗P = 0.03; one-way ANOVA); n.s., not significant.
References
Agnihotri, A., Roy, A., and Joshi, R. (2016). Gustatory receptors in Lepidoptera: chemosensation and beyond. Insect Mol. Biol. 25, 519–529. doi: 10.1111/imb.12246
Alabi, T., Marion-Poll, F., Danho, M., Mazzucchelli, G., De Pauw, E., Haubruge, E., et al. (2014). Identification of taste receptors and proteomic characterization of the antenna and legs of Tribolium brevicornis, a stored food product pest. Insect Mol. Biol. 23, 1–12. doi: 10.1111/imb.12056
Barbehenn, R. V., and Constabel, C. P. (2011). Tannins in plant–herbivore interactions. Phytochemistry 72, 1551–1565. doi: 10.1016/j.phytochem.2011.01.040
Bartlet, E., Parsons, D., Williams, I., and Clark, S. (1994). The influence of glucosinolates and sugars on feeding by the cabbage stem flea beetle, Psylliodes chrysocephala. Entomol. Exp. Appl. 73, 77–83. doi: 10.1111/j.1570-7458.1994.tb01841.x
Bartlet, E., Romani, R., Williams, I. H., and Isidoro, N. (1999). Functional anatomy of sensory structures on the antennae of Psylliodes chrysocephala L. (Coleoptera: Chrysomelidae). Int. J. Insect Morphol. Embryol. 28, 291–300. doi: 10.1016/S0020-7322(99)00032-X
Bernays, E., Oppenheim, S., Chapman, R., Kwon, H., and Gould, F. (2000). Taste sensitivity of insect herbivores to deterrents is greater in specialists than in generalists: a behavioral test of the hypothesis with two closely related caterpillars. J. Chem. Ecol. 26, 547–563. doi: 10.1023/A:1005430010314
Boeckler, G. A., Gershenzon, J., and Unsicker, S. B. (2011). Phenolic glycosides of the Salicaceae and their role as anti-herbivore defenses. Phytochemistry 72, 1497–1509. doi: 10.1016/j.phytochem.2011.01.038
Burse, A., Frick, S., Discher, S., Tolzin-Banasch, K., Kirsch, R., Strauss, A., et al. (2009). Always being well prepared for defense: the production of deterrents by juvenile Chrysomelina beetles (Chrysomelidae). Phytochemistry 70, 1899–1909. doi: 10.1016/j.phytochem.2009.08.002
Bustos-Segura, C., and Foley, W. J. (2018). Foliar terpene chemotypes and herbivory determine variation in plant volatile emissions. J. Chem. Ecol. 44, 51–61. doi: 10.1007/s10886-017-0919-8
Chapman, R. F. (2003). Contact chemoreception in feeding by phytophagous insects. Annu. Rev. Entomol. 48, 455–484. doi: 10.1146/annurev.ento.48.091801.112629
Faucheux, M. J., and Kundrata, R. (2017). Comparative antennal morphology of male Drilini with special reference to the sensilla (Coleoptera: Elateridae: Agrypninae). Zool. Anz. 266, 105–119. doi: 10.1016/j.jcz.2016.11.002
Fernandez, P., and Hilker, M. (2007). Host plant location by Chrysomelidae. Basic Appl. Ecol. 8, 97–116. doi: 10.1016/j.baae.2006.05.001
Ge, S.-Q., Hua, Y., Ren, J., Ślipiński, A., Heming, B., Beutel, R. G., et al. (2015). Transformation of head structures during the metamorphosis of Chrysomela populi (Coleoptera, Chrysomelidae). Arthropod. Syst. Phylo. 73,129–152.
Gök, A., and Çilbiroğlu, E. (2005). Studies on the abundance, biology and harmfulness of leaf beetles (Coleoptera: Chrysomelidae) in natural bush vegetation in Isparta, Turkey. J. Pest Sci. 78, 13–15. doi: 10.1007/s10340-004-0061-6
Gray, C. A., Runyon, J. B., Jenkins, M. J., and Giunta, A. D. (2015). Mountain pine beetles use volatile cues to locate host limber pine and avoid non-host great basin bristlecone pine. PLoS One 10:e0135752. doi: 10.1371/journal.pone.0135752
Gruppe, A., Fusseder, M., and Schopf, R. (1999). Short rotation plantations of aspen and balsam poplar on former arable land in Germany: defoliating insects and leaf constituents. For. Ecol. Manag. 121, 113–122. doi: 10.1016/S0378-1127(98)00560-X
Hallberg, E. (1982). Sensory organs in Ips typographus (Insecta: Coleoptera)—fine structure of antennal sensilla. Protoplasma 111, 206–214. doi: 10.1007/BF01281968
Hallberg, E., and Hansson, B. S. (1999). Arthropod sensilla: morphology and phylogenetic considerations. Microsc. Res. Tech. 47, 428–439. doi: 10.1002/(SICI)1097-0029(19991215)47:6<428::AID-JEMT6>3.0.CO;2-P
Hallem, E. A., Dahanukar, A., and Carlson, J. R. (2006). Insect odor and taste receptors. Annu. Rev. Entomol. 51, 113–135. doi: 10.1146/annurev.ento.51.051705.113646
Heisswolf, A., Gabler, D., Obermaier, E., and Müller, C. (2007). Olfactory versus contact cues in host plant recognition of a monophagous chrysomelid beetle. J. Insect Behav. 20:247. doi: 10.1007/s10905-007-9078-z
Hodgson, E., Lettvin, J., and Roeder, K. (1955). Physiology of a primary chemoreceptor unit. Science 122, 417–418. doi: 10.1126/science.122.3166.417-a
Ikonen, A. (2002). Preferences of six leaf beetle species among qualitatively different leaf age classes of three Salicaceous host species. Chemoecology 12, 23–28. doi: 10.1007/s00049-002-8323-3
Isidoro, N., Bartlet, E., Ziesmann, J., and Williams, I. (1998). Antennal contact chemosensilla in Psylliodes chrysocephala responding to cruciferous allelochemicals. Physiol. Entomol. 23, 131–138. doi: 10.1046/j.1365-3032.1998.232066.x
Jørgensen, K., Almaas, T. J., Marion-Poll, F., and Mustaparta, H. (2007). Electrophysiological characterization of responses from gustatory receptor neurons of sensilla chaetica in the moth Heliothis virescens. Chem. Sens. 32, 863–879. doi: 10.1093/chemse/bjm057
Julkunen-Tiitto, R., and Virjamo, V. (2016). “Biosynthesis and roles of Salicaceae salicylates,” in Plant Specialized Metabolism, eds G.-I. Arimura and M. Maffei (Boca Raton: CRC Press), 77–96.
La Spina, S., Gregoire, J.-C., Mertens, P., and De-Canniere, C. (2010). Impact of poplar water status on leaf-beetle (Chrysomela populi) survival and feeding. Ann. For. Sci. 67, 209–209. doi: 10.1051/forest/2009102
Marion-Poll, F. (1996). Display and analysis of electrophysiological data under windowsTM. Entomol. Exp. Appl. 80, 116–119. doi: 10.1111/j.1570-7458.1996.tb00900.x
Marion-Poll, F., and Van Der Pers, J. (1996). Un-filtered recordings from insect taste sensilla. Entomol. Exp. Appl. 80, 113–115. doi: 10.1111/j.1570-7458.1996.tb00899.x
Michels, J., and Gorb, S. (2012). Detailed three-dimensional visualization of resilin in the exoskeleton of arthropods using confocal laser scanning microscopy. J. Microsc. 245, 1–16. doi: 10.1111/j.1365-2818.2011.03523.x
Mitchell, B., Itagaki, H., and Rivet, M.-P. (1999). Peripheral and central structures involved in insect gustation. Microsc. Res. Tech. 47, 401–415. doi: 10.1002/(SICI)1097-0029(19991215)47:6<401::AID-JEMT4>3.0.CO;2-7
Müller, C., Van Loon, J., Ruschioni, S., De Nicola, G. R., Olsen, C. E., Iori, R., et al. (2015). Taste detection of the non-volatile isothiocyanate moringin results in deterrence to glucosinolate-adapted insect larvae. Phytochemistry 118, 139–148. doi: 10.1016/j.phytochem.2015.08.007
Ômura, H. (2018). “Plant secondary metabolites in host selection of butterfly,” in Chemical Ecology of Insects, ed. J. Tabata (Boca Raton: CRC Press),3–27.
Osier, T. L., and Lindroth, R. L. (2006). Genotype and environment determine allocation to and costs of resistance in quaking aspen. Oecologia 148, 293–303. doi: 10.1007/s00442-006-0373-8
Pasteels, J. M., Rowell-Rahier, M., Braekman, J. C., and Dupont, A. (1983). Salicin from host plant as precursor of salicyl aldehyde in defensive secretion of chrysomeline larvae. Physiol. Entomol. 8, 307–314. doi: 10.1111/j.1365-3032.1983.tb00362.x
Peisker, H., Michels, J., and Gorb, S. N. (2013). Evidence for a material gradient in the adhesive tarsal setae of the ladybird beetle Coccinella septempunctata. Nat. Commun. 4:1661. doi: 10.1038/ncomms2576
Pentzold, S., Burse, A., and Boland, W. (2017). Contact chemosensation of phytochemicals by insect herbivores. Nat. Prod. Rep. 34, 478–483. doi: 10.1039/c7np00002b
Rank, N. E. (1992). Host plant preference based on salicylate chemistry in a willow leaf beetle (Chrysomela aeneicollis). Oecologia 90, 95–101. doi: 10.1007/BF00317814
Robert, C. A. M., Veyrat, N., Glauser, G., Marti, G., Doyen, G. R., Villard, N., et al. (2012). A specialist root herbivore exploits defensive metabolites to locate nutritious tissues. Ecol. Lett. 15, 55–64. doi: 10.1111/j.1461-0248.2011.01708.x
Ryan, M. F. (ed.). (2002). “The chemoreceptive organs: structural aspects”, in Insect Chemoreception: Fundamental and Applied (Dordrecht: Springer), 113–139. doi: 10.1007/0-306-47581-2_4
Schneider, C. A., Rasband, W. S., and Eliceiri, K. W. (2012). NIH Image to ImageJ: 25 years of image analysis. Nat. Methods 9:671. doi: 10.1038/nmeth.2089
Seada, M. A., and Hamza, A. M. (2018). Differential morphology of the sensory sensilla of antennae, palpi, foretarsi and ovipositor of adult Tribolium castaneum (Herbst) (Coleoptera: Tenebrionidae). Ann. Agr. Sci. 63, 1–18. doi: 10.1016/j.aoas.2018.02.001
Sollai, G., Barbarossa, I. T., Masala, C., Solari, P., and Crnjar, R. (2014). Gustatory sensitivity and food acceptance in two phylogenetically closely related Papilionid species: Papilio hospiton and Papilio machaon. PLoS One 9:e100675. doi: 10.1371/journal.pone.0100675
Sollai, G., Biolchini, M., Solari, P., and Crnjar, R. (2017). Chemosensory basis of larval performance of Papilio hospiton on different host plants. J. Insect Physiol. 99, 47–57. doi: 10.1016/j.jinsphys.2017.02.007
Van Naters, W. V. D. G., and Carlson, J. R. (2006). Insects as chemosensors of humans and crops. Nature 444, 302–307. doi: 10.1038/nature05403
Wink, M. (2018). Plant secondary metabolites modulate insect behavior-steps toward addiction? Front. Physiol. 9:364. doi: 10.3389/fphys.2018.00364
Zhang, G.-H., Li, B.-L., and Li, C.-R. (2016). Morphology and distribution of antennal sensilla of female Phyllotreta striolata (Fabricius) (Coleoptera: Chrysomelidae). Microsc. Res. Tech. 79, 219–226. doi: 10.1002/jemt.22621
Zhang, J., Hou, L., Cheng, B., and Xi, J. (2017). Morphology and distribution of the antennal sensillia of the leaf beetle, Ambrostoma quadriimpressum (Coleoptera: Chrysomelidae). Trans. Am. Entomol. Soc. 143, 757–772. doi: 10.3157/061.143.0406
Keywords: contact chemosensation, sensilla chaetica, cuticular autofluorescence, gustation, herbivory, antenna, leaf beetle, Chrysomela populi
Citation: Pentzold S, Marion-Poll F, Grabe V and Burse A (2019) Autofluorescence-Based Identification and Functional Validation of Antennal Gustatory Sensilla in a Specialist Leaf Beetle. Front. Physiol. 10:343. doi: 10.3389/fphys.2019.00343
Received: 21 January 2019; Accepted: 14 March 2019;
Published: 28 March 2019.
Edited by:
Peng He, Guizhou University, ChinaReviewed by:
Letian Xu, Hubei University, ChinaDingze Mang, Tokyo University of Agriculture and Technology, Japan
Jackson Sparks, High Point University, United States
Copyright © 2019 Pentzold, Marion-Poll, Grabe and Burse. This is an open-access article distributed under the terms of the Creative Commons Attribution License (CC BY). The use, distribution or reproduction in other forums is permitted, provided the original author(s) and the copyright owner(s) are credited and that the original publication in this journal is cited, in accordance with accepted academic practice. No use, distribution or reproduction is permitted which does not comply with these terms.
*Correspondence: Stefan Pentzold, c3BlbnR6b2xkQGljZS5tcGcuZGU=