- 1INSERM U1273, ESPCI Paris, CNRS FRE 2031, Physics for Medicine Paris, PSL Research University, Paris, France
- 2Centre de Référence des Maladies Vasculaires Rares, Hôpital Européen Georges-Pompidou, Assistance Publique – Hôpitaux de Paris (APHP), Paris, France
- 3INSERM U970 PARCC, Paris Descartes University – Sorbonne Paris Cité University, Paris, France
- 4Service d’Anatomie Pathologique, Hôpital Européen Georges-Pompidou, Assistance Publique – Hôpitaux de Paris (APHP), Paris, France
- 5Service de Radiologie Cardiovasculaire, Hôpital Européen Georges-Pompidou, Assistance Publique – Hôpitaux de Paris (APHP), Paris, France
Purpose of the Review: Bicuspid aortic valve (BAV) is associated with a significant risk of development of aneurysm and dissection of the ascending thoracic aorta. Development of what is called BAV associated aortopathy is particularly heterogeneous with an uncertain prognosis and with no prognostic biomarkers except for the aortic diameter. This situation leads to an important variability of the therapeutic strategy of this aortopathy. By reviewing the literature on aortic stiffness in the case of BAV, we aimed at evaluating its potential prognostic role in the development of aortic dilatation.
Recent Findings: Studies evaluating aortic stiffness, with ultrasound or magnetic resonance imaging, converge toward the description of an increased segmental aortic stiffness in BAV patients regardless of age, diameter or aortic level, from the root to the arch. Even though there is a lack of longitudinal studies evaluating the progression of aortic dilatation, new data have recently shown the potential prognostic role of the maximal rate of systolic distension of the aortic wall with magnetic resonance imaging.
Summary: Although the use of aortic distensibility calculation is a simple evaluation of stiffness that could be easily transposed in daily practice, its interpretation remains uncertain. New arterial stiffening indicators seem more promising but need a stronger validation.
Introduction
Even though Bicuspid Aortic Valve (BAV) is the most common cardiac malformation with a prevalence estimated between 0.5 and 2% of the general population (Ward, 2000; Hoffman and Kaplan, 2002), the aortic prognosis in the case of BAV is still debated and remains to be the main question not yet answered in the literature. Medical and surgical management of what is called BAV associated aortopathy (BAV-AA) remains elusive with important variations in the recommendations due to the difficulty to predict the risk of acute aortic events (Borger et al., 2004). BAV-AA is difficult to estimate due to different thresholds used to define aortic dilatation (diameter over 35 or 40 mm, or normalized ratio to reference values), and age of the study population. For example, prevalence of aortic dilatation >40 mm in the Olmsted County cohort was 15% for BAV patients of 32 ± 20 years (Michelena et al., 2008), and 9.8% in the Toronto cohort with a population age of 35 ± 16 years (Tzemos et al., 2008). After a 9-year-follow up or 10 ± 6-year-follow up, aortic dilatation rate raised to 49% in the Olmsted County cohort and to 20% in the Toronto cohort, respectively. Della Corte et al. even reported a rate of 83% of aortic dilatation, while using a normalized ratio > 1.1 to define dilated patients (Della Corte et al., 2007).
Impact of BAV-AA on morbidity and mortality is related to the risk of acute aortic events, including dissection and aneurysm rupture. In the case of BAV, a higher aortic dissection risk has been suggested by numerous pathological studies (Gore and Seiwert, 1952; Larson and Edwards, 1984; Roberts and Roberts, 1991). The exact prevalence of aortic dissection remains poorly defined, ranging from 1 to 13% of BAV among aortic dissections (Ward, 2000). Among young people, BAV is one of the most important risk factors, representing 9% of all aortic dissections (Januzzi et al., 2004). However, even though BAV patients may have a relative risk of dissection 9 times greater than patients with normal tricuspid aortic valve (TAV), results of the literature converge toward a very moderate absolute risk of acute aortic event. Incidence of aortic dissection in the case of BAV was indeed estimated to 1.5/100,000 patient-years by Michelena et al. (2011) and at 18/100,000 patient-years in a recent Chinese cohort by Li et al. (2017), much lower than the 2/100 patient-years in the case of Marfan syndrome (Groth et al., 2017).
In daily practice, the risk for aortic dissection in BAV is assessed by the measure of the aortic diameter, the only morphological marker used to take therapeutic decisions, according to the most recent guidelines (Erbel et al., 2014). Aortic diameter threshold for surgical intervention was determined at 55 mm in the absence of coarctation, high blood pressure, or suspected family form of aortic dissection.
Aortic dilatation is a recognized risk factor for aortic dissection (Davies et al., 2006), but it doesn’t account for everything. Indeed, among the aortic dissections reported by the IRAD registry (not restricted to BAV), 40% of dissections occurred with an aortic diameter below 50 mm (Pape et al., 2007). Moreover, Davies et al. (2007) showed that despite a higher rate of aortic growth in BAV patients compared to controls (0.19 vs. 0.13 cm/year, p = 0.01), the incidence of rupture and dissection were similar. Because of early changes in the extracellular matrix, the elastic properties, especially the stiffness, of the aortic wall appear to be modified early in the case of BAV. This review focuses on the ascending aortic biomechanical properties in BAV. We aimed at gathering the whole set of results on the study of the biomechanical properties of the aortic wall, in order to highlight the interest of stiffness assessment with the different imaging modalities available.
Structural Changes
Histology
Histological analyses of the ascending aortic wall of BAV patients have been widely described as non-specific abnormalities. They include loss of vascular smooth muscle cells (vSMC), loss and fragmentation of elastin fibers and/or Mucoid Extracellular Matrix Accumulation (MEMA) (Figure 1). They include loss of vSMC, loss and fragmentation of elastin fibers and/or MEMA, corresponding to an accumulation of mucoid substance (Figure 1; Halushka et al., 2016). MEMA corresponds to the former denomination of multiple terms, such as Erdheim’s cystic degeneration, cystic medial necrosis, medionecrosis, and cystonecrosis (Larson and Edwards, 1984; Bonderman et al., 1999; Bauer et al., 2002). Even though these non-specific abnormalities are present in a wide range of aortic pathologies, in the case of BAV patients, they occur at an early stage in the absence of aortic dilatation (Bonderman et al., 1999). There are, however, a marked heterogeneity of lesions observed in BAV patients (De Sa et al., 1999; Parai et al., 1999; Bauer et al., 2002; Leone et al., 2012; Waters et al., 2017). The pathophysiological process leading to the medial layer remodeling in the case of BAV is poorly understood. Two assumptions have been formulated and are not necessarily conflicting: on the one hand, a constitutive aortic wall alteration due to genetic predisposition, on the other hand an acquired aortic wall alteration due to the modified amplitude and direction of the flow wall shear stress, generated by the BAV (Girdauskas et al., 2011; Sievers and Sievers, 2011; Piatti et al., 2017; Liu et al., 2018). The presence of matrix degeneration is thus emphasized in areas with high shear stress (Gore and Seiwert, 1952; Gosline et al., 2002).
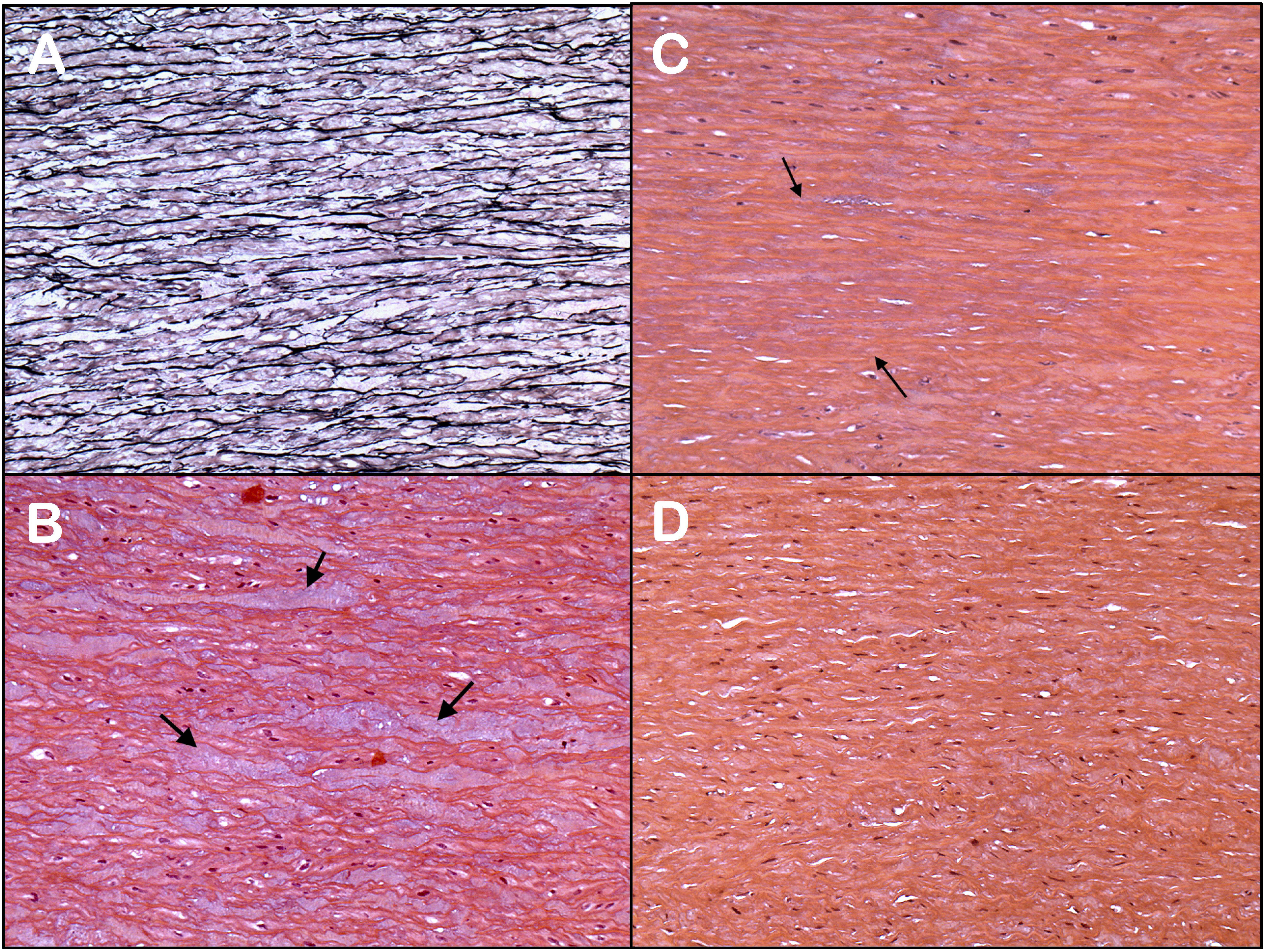
Figure 1. Different histological features observed in aortas of BAV patients (A–C) and the normal aorta (D): (A) Fragmentation and loss of the elastin fibers (limited to 1 or 2 lamellar units; mild grade) (Elastic stain; Original X10). (B) Inter-lamellar degeneration with mucoid replacement (MEMA) (H&E stain; Original X10). (C) Moderate loss of nuclei of vascular smooth muscle cells (involving 4 to 10 lamellar units; area between arrows) (H&E stain; Original X10). (D) Normal ascending thoracic aorta. The media shows no mucoid accumulation in the extracellular matrix or loss of smooth muscle cells (H&E stain; Original X10).
Elastic fragmentation and loss, vSMC loss, and MEMA are also found in a great proportion of other aortopathies, such as Marfan or Loeys-Dietz syndrome (Davies et al., 2007). The comparison between BAV and Marfan syndrome is justified due to common histological features affecting elastin fibers, vSMC, and due to an increased risk of aortic dissection in both pathologies. Grewal et al. (2016) underlined the common features of aortic wall involvement, with an alteration of fibrillin 1 expression and a lower differentiation of vSMC. However, in case of Marfan syndrome, histological abnormalities of the medial layer are diffuse and more severe (Della Corte et al., 2006; Waters et al., 2017). Marfan syndrome is clinically characterized by an earlier aortic dilation and a major risk of acute aortic event, much higher than in BAV (Detaint et al., 2014). Lastly, the presence of elastin fibers loss, vSMC loss, and MEMA, in BAV patients proves to be a non-specific marker of aortic fragility, without prejudging the causal genetic or haemodynamic origin.
Immunohistochemistry
Immunohistochemical studies confirm the early loss of elastin fibers. By analyzing aortic aneurysms of BAV patients, Nataatmadja (2003) found a reduction of fibrillin, fibronectin, and tenascin in the extracellular matrix (Fedak et al., 2003; Nataatmadja, 2003). An overall content reduction of elastin fibers was also observed by Blunder et al. (2012). Changes in extracellular matrix content may be associated with either a modification of its synthesis or an increased expression of matrix metalloproteases (MMP). In the case of BAV, studies converge toward an increase in pro-MMP-2 expression in the ascending aorta, without modification of the MMP-9 level (Fedak et al., 2003; Lemaire et al., 2005). Moreover, the activity of MMP-2 seems to be correlated with the presence of important haemodynamic wall stress (Guzzardi et al., 2015).
Micro-Architectural Analysis
Beyond the analysis by optical microscopy, few studies evaluated the aortic wall of BAV patients using electron microscopic analyses. Niwa et al. (2001) presented some analysis of a few BAV patients’ aorta. Their results are consistent with light microscopy, showing heterogeneous elastin fibers fragmentation and a further increase in collagen (Niwa et al., 2001). At the cellular level, Maleki et al. (2016) used electron microscopy to evaluate intimal and medial cells in the case of BAV, and found cell abnormalities evoking a defect of cellular maturation. In addition to the fine cell evaluation, composition and organization of the extracellular matrix can be assessed with advanced techniques of multiphoton microscopy. Results converge toward a different orientation of elastin and collagen fibers in the case of BAV, proof of the early parietal remodeling (Phillippi et al., 2014). A decrease in elastin fibers radial orientation was found specifically in regions were aorta was dilated in BAV patients (Tsamis et al., 2016). In dilated aortas from BAV patients vs. TAV patients, the elastin fibers architecture was more disrupted when the collagen beams still remained organized.
All the histological changes presented converge toward an early non-inflammatory modification of the aortic wall architecture in BAV. As changes in elastic and collagen bundles are associated with a modified biomechanical behavior of the aorta (Roach and Burton, 1957), the second part of this review focuses on the results of aortic stiffness studies.
Stiffness Evaluation of the Aorta of Bav Patients
The histological alterations observed overlap with a qualitative and quantitative early alterations of the extracellular matrix. At a physiological pressure level, elastin fibers are very distensible (Wolinsky and Glagov, 1964), with an elastic modulus of 1.1 MPa for a stress below 1 MPa (Gosline et al., 2002). BAV histological features include MEMA, elastin fiber fragmentation, loss of smooth muscle cells, and augmentation of collagen fibers (de Sa et al., 1999). Longobardo et al. (2017) found an increase of elastin degradation, evaluated by the measurement of elastin soluble fragments, in the case of BAV associated with an increased aortic stiffness. The search for early markers of abnormalities of the thoracic aorta might be helpful to guide the management of the patients carrying BAV before the appearance of an aortic dilatation. In order to assess the aortic elastic properties, the local Young’s modulus (E) can be calculated, either invasively on a test-bed or non-invasively with two major indicators: distensibility and pulse wave velocity (PWV) following these formulas:
E: Young’s modulus (Pa); dD: diastolic aortic diameter (m); h: wall thickness (m); Dist: distensibility (Pa−1); ρ: wall viscosity (Pa.s); PWV: pulse wave velocity (m.s−1).
Ex vivo Mechanical Testing
The simplest evaluation of the local Young’s modulus of a tissue is to measure directly the elongation of an ex vivo portion by controlling the stress, as developed by Roach and Burton (1957). Pham et al. (2013) compared biomechanical properties by load-controlled biaxial testing of aorta specimens from patients with BAV to patients without BAV, who had all been operated for an ascending aortic aneurism. Mean aortic diameter [50.1 mm (BAV) vs. 49.4 mm (non-BAV)] and age [55.0 years (BAV) vs. 59.5 (non-BAV)] did not differ. Aortic specimens of BAV patients were significantly stiffer in the longitudinal direction at 60 N.m−1 membrane tension, and a similar trend, although non significant, was observed at 120 N.m−1 and in the circumferential direction. Histological analyses of the specimens retrieved features that are usually found in aortic wall in the case of BAV, including a lower proportion of elastin fibers. The increased aortic stiffness in BAV patients is in agreement with previous studies with different testing conditions (Choudhury et al., 2009; Duprey et al., 2010).
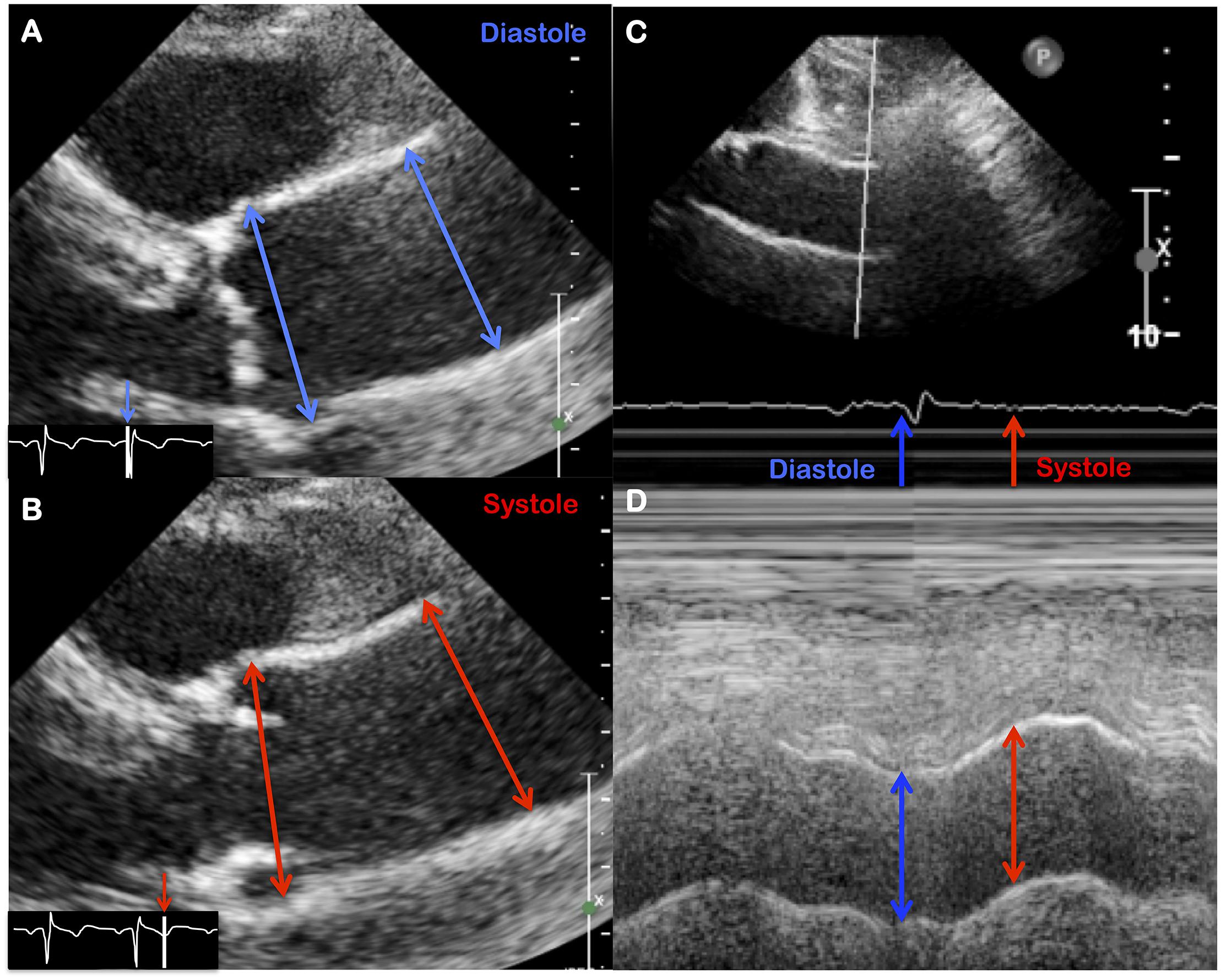
Figure 2. Evaluation of aortic strain using echocardiographic features, either with B mode (A,B) or with M mode (C,D).
A higher macroscopic strength of aortic specimens is also found in BAV patients. Forsell et al. (2014) compared biomechanical properties by displacement-controlled uniaxial testing of aorta specimens from patients with BAV to patients without BAV, who had all been operated for an ascending aortic aneurism. Mean aortic diameters [59 mm (BAV) vs. 53 mm (non-BAV)] and ages [60 years (BAV) vs. 59 (non-BAV)] did not differ. Aortic strength of BAV patients was two times stronger (0.99 ± 0.46 vs. 0.49 ± 0.21 MPa; p < 0.001; Forsell et al., 2014). This difference was primarily explained by higher collagen stiffness in BAV patients. The increased aortic strength in BAV patients is in agreement with previous studies (Okamoto et al., 2002; Pichamuthu et al., 2013).
Although these in vitro tests do not have an extension in clinical practice, they highlight the increase in aortic stiffness and strength under fixed conditions at a tissue length scale and show the challenge of an accurate evaluation at the organ length scale.
Ultrasonic Stiffness Evaluation
Evaluation of the ascending aorta by trans-thoracic ultrasound seems the simplest, most widely used and low-cost approach. Aortic distensibility and stiffness index can be easily calculated with time-motion (M-mode) or brightness (B-mode) acquisitions (Figure 2), as follows (Hirai et al., 1989; Nistri et al., 2008):
Dist: distensibility (mmHg−1); sD: systolic aortic diameter (mm); dD: diastolic aortic diameter (mm); SBP: systolic blood pressure (mmHg); DBP: diastolic blood pressure (mmHg); SI: stiffness index (no unit).
The wall stress quantification is only evaluated by the measurement of brachial blood pressure. Due to the restricted field of view, the evaluation is limited to the first centimeters of the ascending aorta. Despite these limitations, evaluation of aortic distensibility allowed a wide screening of BAV patients and can be performed during the usual follow-up of these patients. Thus, ultrasonic data represent the core data of stiffness evaluation in BAV associated aortopathy with distensibility analysis in numerous clinical series.
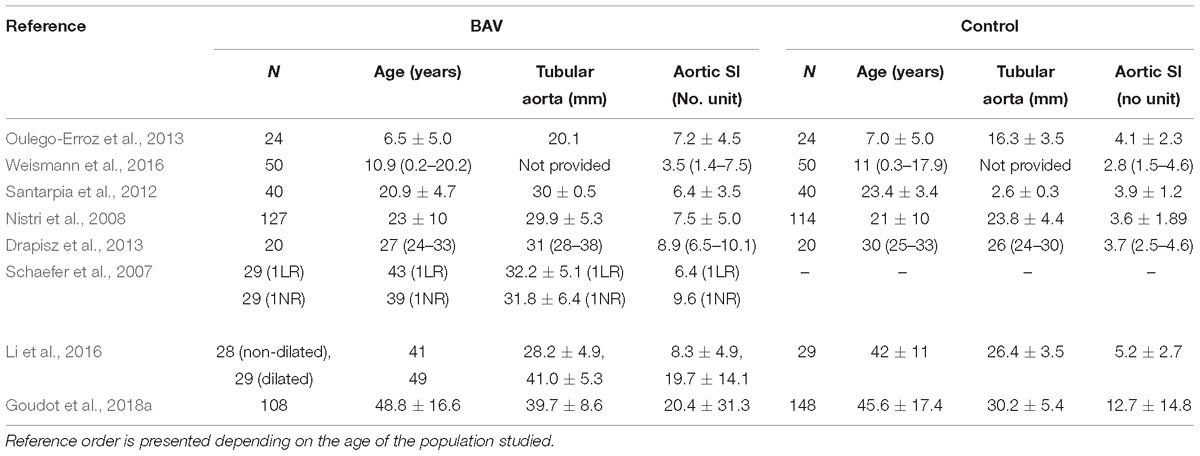
Table 1. Results of ultrasonic stiffness evaluation (stiffness index, SI) at the tubular aortic level.
Overall, ultrasonic evaluation studies converge toward an increase in aortic stiffness and a larger aortic diameter, concordant with ex vivo studies (Schaefer et al., 2007; Nistri et al., 2008; Santarpia et al., 2012; Oulego-Erroz et al., 2013; Li et al., 2016). Aortic stiffness increases early, independently of the valvular function and of the initial dilatation level. Three series evaluated young BAV patients (mean age of 6.5 years in Oulego-Erroz et al., 2013 and 8.3 years in Pees and Michel-behnke, 2012 and 10.9 years in Weismann et al., 2016) and revealed a 62, 29, and 25% increase in aortic stiffness, respectively, as compared to controls, in the absence of aortic dilatation (Pees and Michel-behnke, 2012; Oulego-Erroz et al., 2013; Weismann et al., 2016). These studies on young patients emphasize the early increase in stiffness prior to the onset of aortic dilation. This aortic stiffness increase persists in adulthood, as demonstrated by Nistri et al. (2008) with a young adult population of 127 patients with an average age of 23 years, and is then maintained throughout life, confirmed by studies of older populations which were compared to TAV controls in each case (Santarpia et al., 2012; de Wit et al., 2013; Drapisz et al., 2013; Lee et al., 2015; Li et al., 2016). In those studies, the influence of the function or the morphotype of the valve seemed limited. Schaefer et al. (2007), however, found that BAV with 1-LR morphotype was associated with a stiffer aorta, compared to 1-NR type, but only at the sinus of Valsalva level (Schaefer et al., 2007). Ultrasound evaluation can also provide an assessment of segment circumferential stiffness along with the aortic axis (sinus of Valsalva, tubular aorta, aortic arch). This approach may have a particular interest as BAV associated aortopathy presents a segmental histological injury. We recently evaluated the segmental stiffness of the ascending aorta of BAV patients. Although all aortic segments had a higher stiffness in the case of BAV, only the sinus of Valsalva remained more stuff, regardless of the aortic dilatation (Goudot et al., 2018a). These results are consistent with the previous data on an intrinsic involvement of the sinus of Valsalva in BAV (Azadani et al., 2012; Yassine et al., 2017) and highlight the need to distinguish stiffness according to the different aortic segments.
The hypothesis of a genetic background leading to the formation of a BAV and the associated aortopathy is supported by study results on syndromic forms of BAV (Turner-, Williams-Beuren-, Shone-, DiGeorge- syndromes). They represent a distinct group of BAV patients, with a high prevalence of other associated cardiac and aortic abnormalities, including ventricular septal defect and aortic coarctation. In these patients, the genetic background of BAV and cardiac or aortic defect is clear. Assessment of aortic distensibility in a series of women with Turner syndrome, particularly affected by acute aortic events (Matura et al., 2007), revealed an early increase in their ascending aorta stiffness (De Groote et al., 2017).
To our knowledge, no prognostic study has yet stated the aortic stiffness index as a predictive marker of further aortic dilatation. Although stiffness deserves attention because it precedes aortic dilatation, ultrasonic evaluation (either with M or B-mode) suffers from a great variability of values, between the BAV and TAV patient groups (Table 1), but also from poorly standardized measurements, operator dependency, and the need for coupling with an accurate measurement of central pressure. For these reasons, other morphological markers of aortic stiffness, with a minor variability depending on the operator, are required. For example, aortic wall longitudinal strain measurement with speckle tracking imaging may be a promising technique. Aortic speckle tracking was developed to obtain the longitudinal and circumferential deformation of the aortic wall (Oishi et al., 2008). With the advantage of a simple implementation, only based on cardiac ultrasound loops, 2D evaluation remains the main limitation, although 3D is under development (Karatolios et al., 2013). In its 2D use, however, absolute mean difference ± SD between longitudinal strain measurements, within one observer and between two observers, was low at 1.5 and 1.1%, respectively (Longobardo et al., 2017).
Stiffness Assessment With Magnetic Resonance Imaging (MRI)
The development of MRI in cardiovascular pathologies now provides a powerful tool to assess the stiffness of the entire thoracic aorta, not only with the local evaluation, based on distensibility, but also the regional stiffness with the PWV measurement.
Following ultrasound studies, aortic distensibility using MRI has been initially investigated by Grotenhuis et al. (2007) at the level of the sinotubular junction with 2D sequences. In 20 BAV patients as compared with 20 matched controls, a reduced root distensibility (3.1 ± 1.2.10-3 mmHg−1 vs. 5.6 ± 3.2.10-3 mmHg−1, p < 0.01) was consistent with the higher concomitant PWV measures (5.6 ± 1.3 m/s vs. 4.5 ± 1.1 m/s, p < 0.01), converging toward a higher aortic stiffness in the case of BAV (Grotenhuis et al., 2007).
This observation was confirmed by Burris et al. in 65 young BAV patients (mean age of 28 years) with a tendency for a higher PWV (6.53 vs. 3.51 m/s, p = 0.1) but with a large variation among BAV patients (SD of 5.88 m/s). More recently, the PWV can be measured from the 4D flow MRI sequences, allowing a reconstruction of the entire aortic arch (Guala et al., 2018). However, there are several limitations to measuring PWV with MRI: sequences require a long acquisition time (10 min of total scan time Guala et al., 2018), and are generally post-processed afterward. Lastly, it is a flow evaluation, without direct evaluation of the behavior of the aortic wall.
Pulse wave velocity appears as a complex biomarker, as it can be measured with very different approaches (Time to foot, time to peak upslope, Fourier analysis, cross correlation or center of mass method). Thus, variable results could be obtained in healthy adults, depending on which part of the waveform is analyzed, as reported by Dyverfeldt et al. (2014). For example, in the Bland–Altman analysis, the bias was 2.21 ± 1.1 m/s between the cross correlation and the time to foot method.
To better differentiate TAV and BAV patients, new biomarkers of aortic stiffening based on aortic strain analysis over the cardiac cycle have been proposed by Aquaro et al. (2011). In this study, 2 indexes, the Maximal Rate of Systolic Distension (MRSD) and the Maximal Rate of Diastolic Recoil (MRDR) have been tested in a BAV population. These measurements are accessible from a cross-section made on the ascending tubular aorta, perpendicular to the axis of the vessel. The circumferential aortic wall was tracked continuously over the cardiac cycle during a single acquisition with a temporal resolution of about 1 ms. They correspond to the dynamic change of the aortic diameters. However, a limitation of these indicators is the transverse evaluation, that may be affected by longitudinal movements of the aorta during the cardiac cycle. Both of these new biomarkers have proven to be much lower in BAV-patients than in controls, even in the absence of thoracic aortic dilatation. Moreover, receiver operating characteristic curve analysis of MRSD distinguished BAV from controls with 100% sensitivity and 95% specificity. In a longitudinal study with a short follow-up of 17 months, the same authors showed that MRSD could predict an increase of aortic diameter with 93.7% specificity and 75.6% sensitivity, with a cut-off value of MRSD ≤ 6 (Aquaro et al., 2017).
Global Evaluation of Aortic Stiffness: Carotid-Femoral PWV (cfPWV)
Apart from the usual imaging techniques of the ascending aorta, PWV has been first developed and validated with non-invasive measures of the pulse wave at two sites of the arterial tree. Carotid-femoral PWV, calculated by applanation tonometry gives access to a global aortic stiffness. Although this technology is not widespread in daily practice, a large validation of its use and prognosis relevance has already been achieved in non-BAV patients (Laurent et al., 2001; Reference Values for Arterial Stiffness’ Collaboration, 2010). Measurement of the cfPWV has the disadvantage of an average stiffness evaluation over the whole aorta. In case of BAV, only the ascending thoracic aorta seems to be affected by structural changes. Results of the carotid-femoral PWV should thus be interpreted with caution, considering that it is an average stiffness including regions of unequal stiffness.
Tzemos et al. (2010) showed a moderate increase in regional stiffness using cfPWV in a BAV population with aortic dilatation: 9.3 (9.0–10.0) cm.s−1 in BAV (mean age 31 years, n = 16) vs. 7.0 (6.9–7.2) cm.s−1 in controls. Results in BAV patients with non-dilated aorta were similar to controls with a mean PWV of 7.0 (6.9–7.4) cm.s−1. Moreover Warner et al. and Shim et al. did not find any difference of cfPWV between non-dilated BAV patients and controls (Grotenhuis et al., 2007; Shim et al., 2011). Compared to the increased cfPWV demonstrated in Marfan syndrome (Salvi et al., 2018), these results converge toward a more localized alteration of the elastin fibers among large vessels. However, interpretation of cfPWV in BAV patients has to be taken with caution due to the limited number of patients included (16 and 10 BAV patients, respectively).
Clinical Relevance of Aortic Stiffness Indicators
Arterial wall with increased stiffness may be due to an extracellular matrix remodeling, mainly driven by a decrease in the proportion of elastin fibers, as presented above. A stiffer aorta presents a lower deformation capacity when facing the different sources of stress (longitudinal, axial, circumferential stress), and therefore presents a higher risk of dissection or rupture. Even though, from a biomechanical point of view, arterial rupture appears when blood pressure exceeds the intrinsic wall resistance, the prognostic impact of stiffness evaluation in BAV faces many inconsistencies related to the ill-defined pathophysiology of acute aortic events. The rate of acute aortic events in BAV patients is indeed lower than their increased aortic stiffness would suggest. Among BAV patients, imaging studies and in vitro studies of the aortic properties often emphasized the segmental and particularly heterogeneous characteristics of BAV associated aortopathy. As a consequence, only a small but highly impacted aortic wall segment with altered matrix could be the starting point of a dissection, which then spreads to the entire aorta.
Thus, only prospective studies evaluating stiffness indicators on the subsequent development of aortic aneurysm and acute aortic events could validate these biomarkers as prognostic ones. There is currently very limited data on this topic. Only MRSD, measured with MRI and recently validated by Aquaro et al. (2017) as an aortic dilatation marker, gave a prognostic value among BAV patients (Nollen et al., 2004; Aquaro et al., 2017).
However, designing a follow-up study to evaluate predictive risk markers of aortic dissection in BAV is complex and uncertain due to the unexpected low incidence of aortic dissection in BAV patients. Moreover, it would need inclusion of numerous BAV patients as homogeneous as possible. The development of regional stiffness indicators in association with a better evaluation of the wall stress will also allow a better characterization of the different BAV forms.
Analysis of Arterial Stiffening: Toward New Tissue Biomarkers of Aortic Stiffening?
The main objective of this review was to stimulate future directions for the evaluation of aortopathy associated with BAV. Methods for assessing arterial stiffness (mainly distensibility and PWV) showed a stiffness difference between BAV and normal patients at the thoracic aorta level. However, analysis of the raw data showed a significant overlap of the stiffness values, and a significant amount of variability in the measurements with conventional imaging without any truly prognostic role. Interestingly, the MRSD, recently validated as a prognostic tool, is more than a simple stiffness indicator. The peak of distensibility variation appears indeed to be independent on the blood pressure, and to correspond to the dynamic aortic behavior when pressure rises. Switching from the evaluation of the arterial stiffness to the evaluation of the arterial stiffening over the cardiac may bring a new insight into the inner wall properties, as suggested by recent studies (Mirault et al., 2015; Gavish and Izzo, 2016; Goudot et al., 2018b). Arterial stiffness, strongly influenced by the blood pressure level, undergoes a continuous variation during the cardiac cycle. The arterial stiffening is a physiological condition allowing a constant adaptation of the artery to different levels of stress, regardless of its initial stiffness. This parameter is thereby less dependent on the blood pressure level.
In an evaluation of carotid arterial stiffening on patients suffering from vascular Ehlers–Danlos syndrome (vEDS), responsible for arterial ruptures due to defectuous collagen type III in the arterial wall matrix, Mirault et al. demonstrated that basal carotid stiffness was not different in vEDS patients vs. controls, whereas the stiffening over the cardiac cycle was reduced in the vEDS group (Mirault et al., 2015). In this study, the use of ultrafast ultrasonic imaging, evaluating different components of the pulse wave at different times during the cardiac cycle, could measure the arterial stiffening over the cardiac cycle, opening the field to new non-invasive parameters. However, there are currently very few tools to evaluate the arterial stiffening in daily practice. Even though new arterial stiffening biomarkers appear to be a promising tool in aortic wall assessment, the development of dynamic imaging with both MRI and ultrasound imaging requires more validation among the most achievable homogeneity in BAV-patient cohorts.
Conclusion
Regardless of age, presence of valvular dysfunction, sporadic, genetic or syndromic forms of BAV patients, early and segmental changes in the aortic wall result in an excess of wall stiffness as compared to patients with a normal TAV. These results are consistent with the histological aspect of BAV associated aortopathy with marked lesions of elastin fibers destruction and mucoid material replacement. Aortic stiffness markers require a better reliability and long-term evaluation to conclude on their useful prognostic roles. Standardization of stiffness parameters, coupling to flow data, and perhaps evaluation of aortic stiffening could allow clinical parameters to be developed in the near future for prognostic purposes on the risk of dilatation and possibly acute aortic event.
Author Contributions
GG and TM wrote the manuscript. PB proofread the histology part of the manuscript. GS proofread the part of the manuscript concerning the evaluation of the aorta by magnetic resonance imaging. MP proofread the part of the manuscript concerning the biomechanical properties of the aorta as well as the ultrasound evaluation. EM performed the final approval of the version to be published and agreed to be accountable for all aspects of the work in ensuring that questions related to the accuracy and integrity of any part of the work are appropriately investigated and resolved.
Conflict of Interest Statement
The authors declare that the research was conducted in the absence of any commercial or financial relationships that could be construed as a potential conflict of interest.
References
Aquaro, G. D., Ait-ali, L., and Basso, M. L. (2011). Elastic properties of aortic wall in patients with bicuspid aortic. AJC 108, 81–87. doi: 10.1016/j.amjcard.2011.03.005
Aquaro, G. D., Briatico Vangosa, A., Toia, P., Barison, A., Ait-Ali, L., Midiri, M., et al. (2017). Aortic elasticity indices by magnetic resonance predict progression of ascending aorta dilation. Eur. Radiol. 27, 1395–1403. doi: 10.1007/s00330-016-4501-5
Azadani, A. N., Chitsaz, S., Matthews, P. B., Jaussaud, N., Leung, J., Tsinman, T., et al. (2012). Comparison of mechanical properties of human ascending aorta and aortic sinuses. Ann. Thorac. Surg. 93, 87–94. doi: 10.1016/j.athoracsur.2011.08.002
Bauer, M., Pasic, M., Meyer, R., Goetze, N., Bauer, U., Siniawski, H., et al. (2002). Morphometric analysis of aortic media in patients with bicuspid and tricuspid aortic valve. Ann. Thorac. Surg. 74, 58–62. doi: 10.1016/S0003-4975(02)03650-0
Blunder, S., Messner, B., Aschacher, T., Zeller, I., Türkcan, A., Wiedemann, D., et al. (2012). Characteristics of TAV- and BAV-associated thoracic aortic aneurysms—Smooth muscle cell biology, expression profiling, and histological analyses. Atherosclerosis 220, 355–361. doi: 10.1016/j.atherosclerosis.2011.11.035
Bonderman, D., Gharehbaghi-Schnell, E., Wollenek, G., Maurer, G., Baumgartner, H., and Lang, I. M. (1999). Mechanisms underlying aortic dilatation in congenital aortic valve malformation. Circulation 99, 2138–2143. doi: 10.1161/01.CIR.99.16.2138
Borger, M. A., Preston, M., Ivanov, J., Fedak, P. W. M., Davierwala, P., Armstrong, S., et al. (2004). Should the ascending aorta be replaced more frequently in patients with bicuspid aortic valve disease? J. Thorac. Cardiovasc. Surg. 128, 677–683. doi: 10.1016/j.jtcvs.2004.07.009
Choudhury, N., Bouchot, O., Rouleau, L., Tremblay, D., Cartier, R., Butany, J., et al. (2009). Local mechanical and structural properties of healthy and diseased human ascending aorta tissue. Cardiovasc. Pathol. 18, 83–91. doi: 10.1016/j.carpath.2008.01.001
Davies, R. R., Gallo, A., Coady, M. A., Tellides, G., Botta, D. M., Burke, B., et al. (2006). Novel measurement of relative aortic size predicts rupture of thoracic aortic aneurysms. Ann. Thorac. Surg. 81, 169–177. doi: 10.1016/j.athoracsur.2005.06.026
Davies, R. R., Kaple, R. K., Mandapati, D., Gallo, A., Botta, D. M., Elefteriades, J. A., et al. (2007). Natural history of ascending aortic aneurysms in the setting of an unreplaced bicuspid aortic valve. Ann. Thorac. Surg. 83, 1338–1344. doi: 10.1016/j.athoracsur.2006.10.074
De Groote, K., Devos, D., Van Herck, K., De Wolf, D., Van der Straaten, S., Rietzschel, E., et al. (2017). Increased aortic stiffness in prepubertal girls with Turner syndrome. J. Cardiol. 69, 201–207. doi: 10.1016/j.jjcc.2016.03.006
de Sa, M., Moshkovitz, Y., Butany, J., and David, T. E. (1999). Histologic abnormalities of the ascending aorta and pulmonary trunk in patients with bicuspid aortic valve disease: clinical relevance to the ross procedure. J. Thorac. Cardiovasc. Surg. 118, 588–594. doi: 10.1016/S0022-5223(99)70002-4
De Sa, M., Moshkovitz, Y., Butany, J., David, T. E., Robicsek, F., Gardner, T. J., et al. (1999). Histologic abnormalities of the ascending aorta and pulmonary trunk in patients with bicuspid aortic valve disease: clinical relevance to the Ross procedure. J. Thorac. Cardiovasc. Surg. 118, 588–596. doi: 10.1016/S0022-5223(99)70002-4
de Wit, A., Vis, K., and Jeremy, R. W. (2013). Aortic stiffness in heritable aortopathies: relationship to aneurysm growth rate. Hear. Lung Circ. 22, 3–11. doi: 10.1016/j.hlc.2012.08.049
Della Corte, A., Bancone, C., Quarto, C., Dialetto, G., Covino, F. E., Scardone, M., et al. (2007). Predictors of ascending aortic dilatation with bicuspid aortic valve: a wide spectrum of disease expression. Eur. J. Cardiothorac. Surg. 31, 397–404; discussion 404–405. doi: 10.1016/j.ejcts.2006.12.006
Della Corte, A., De Santo, L. S., Montagnani, S., Quarto, C., Romano, G., Amarelli, C., et al. (2006). Spatial patterns of matrix protein expression in dilated ascending aorta with aortic regurgitation: congenital bicuspid valve versus Marfan’s syndrome. J. Heart Valve Dis. 15, 20–277; discussion 27.
Detaint, D., Michelena, H. I., Nkomo, V. T., Vahanian, A., Jondeau, G., and Sarano, M. E. (2014). Aortic dilatation patterns and rates in adults with bicuspid aortic valves: a comparative study with Marfan syndrome and degenerative aortopathy. Heart 100, 126–134. doi: 10.1136/heartjnl-2013-304920
Drapisz, S., Góralczyk, T., Jamka-Miszalski, T., Olszowska, M., and Undas, A. (2013). Nonstenotic bicuspid aortic valve is associated with elevated plasma asymmetric dimethylarginine. J. Cardiovasc. Med. 14, 446–452. doi: 10.2459/JCM.0b013e3283588dfa
Duprey, A., Khanafer, K., Schlicht, M., Avril, S., Williams, D., and Berguer, R. (2010). In vitro characterisation of physiological and maximum elastic modulus of ascending thoracic aortic aneurysms using uniaxial tensile testing. Eur. J. Vasc. Endovasc. Surg. 39, 700-707. doi: 10.1016/j.ejvs.2010.02.015
Dyverfeldt, P., Ebbers, T., and Länne, T. (2014). Pulse wave velocity with 4D flow MRI: systematic differences and age-related regional vascular stiffness. Magn. Reson. Imaging 32, 1266–1271. doi: 10.1016/j.mri.2014.08.021
Erbel, R., Aboyans, V., Boileau, C., Bossone, E., Di Bartolomeo, R., Eggebrecht, H., et al. (2014). 2014 ESC guidelines on the diagnosis and treatment of aortic diseases. Eur. Heart J. 35, 2873–2926. doi: 10.1093/eurheartj/ehu281
Fedak, P. W. M., De Sa, M. P. L., Verma, S., Nili, N., Kazemian, P., Butany, J., et al. (2003). Vascular matrix remodeling in patients with bicuspid aortic valve malformations: implications for aortic dilatation. J. Thorac. Cardiovasc. Surg. 126, 797–806. doi: 10.1016/S0022-5223(03)00398-2
Forsell, C., Björck, H. M., Eriksson, P., Franco-Cereceda, A., and Gasser, T. C. (2014). Biomechanical properties of the thoracic aneurysmal wall: differences between bicuspid aortic valve and tricuspid aortic valve patients. Ann. Thorac. Surg. 98, 65–71. doi: 10.1016/j.athoracsur.2014.04.042
Gavish, B., and Izzo, J. L. Jr. (2016). Arterial stiffness: going a step beyond. Am. J. Hypertens. 29, 1223–1233. doi: 10.1093/ajh/hpw061
Girdauskas, E., Borger, M. A., Secknus, M.-A., Girdauskas, G., and Kuntze, T. (2011). Is aortopathy in bicuspid aortic valve disease a congenital defect or a result of abnormal hemodynamics? A critical reappraisal of a one-sided argument. Eur. J. Cardiothorac. Surg. 39, 809–814. doi: 10.1016/j.ejcts.2011.01.001
Gore, I., and Seiwert, V. J. (1952). Dissecting aneurysm of the aorta; pathological aspects; an analysis of eighty-five fatal cases. AMA Arch. Pathol. 53, 121–141.
Gosline, J., Lillie, M., Carrington, E., Guerette, P., Ortlepp, C., and Savage, K. (2002). Elastic proteins: biological roles and mechanical properties. Philos. Trans. R. Soc. Lond. B Biol. Sci. 357, 121–132. doi: 10.1098/rstb.2001.1022
Goudot, G., Mirault, T., Rossi, A., Zarka, S., Albuisson, J., Achouh, P., et al. (2018a). Segmental aortic stiffness in bicuspid aortic valve patients compared to first-degree relatives. Heart 105, 130–136. doi: 10.1136/heartjnl-2018-313232
Goudot, G., Papadacci, C., Dizier, B., Baudrie, V., and Ferreira, I. (2018b). Arterial stiffening with ultrafast ultrasound imaging gives new insight into arterial phenotype of vascular ehlers-danlos mouse models. Ultraschall Med. 1–10. doi: 10.1055/a-0599-0841
Grewal, N., Franken, R., Mulder, B. J. M., Lindeman, J. H. N., Jongbloed, M. R. M., Deruiter, M. C., et al. (2016). Histopathology of aortic complications in bicuspid aortic valve versus Marfan syndrome: relevance for therapy? Heart Vessels 31, 795–806. doi: 10.1007/s00380-015-0703-z
Grotenhuis, H. B., Ottenkamp, J., Westenberg, J. J. M., Bax, J. J., Kroft, L. J. M., and de Roos, A. (2007). Reduced aortic elasticity and dilatation are associated with aortic regurgitation and left ventricular hypertrophy in nonstenotic bicuspid aortic valve patients. J. Am. Coll. Cardiol. 49, 1660–1665. doi: 10.1016/j.jacc.2006.12.044
Groth, K. A., Stochholm, K., Hove, H., Kyhl, K., Gregersen, P. A., Vejlstrup, N., et al. (2017). Aortic events in a nationwide Marfan syndrome cohort. Clin. Res. Cardiol. 106, 105–112. doi: 10.1007/s00392-016-1028-3
Guala, A., Rodriguez-Palomares, J., Dux-Santoy, L., Teixido-Tura, G., Maldonado, G., Galian, L., et al. (2018). Influence of aortic dilation on the regional aortic stiffness of bicuspid aortic valve assessed by 4-dimensional flow cardiac magnetic resonance: comparison with marfan syndrome and degenerative aortic Aneurysm. JACC Cardiovasc. Imaging doi: 10.1016/j.jcmg.2018.03.017 [Epub ahead of print].
Guzzardi, D. G., Barker, A. J., Van Ooij, P., Malaisrie, S. C., Puthumana, J. J., Belke, D. D., et al. (2015). Valve-related hemodynamics mediate human bicuspid aortopathy: insights from wall shear stress mapping. J. Am. Coll. Cardiol. 66, 892–900. doi: 10.1016/j.jacc.2015.06.1310
Halushka, M. K., Angelini, A., Bartoloni, G., Basso, C., Batoroeva, L., Bruneval, P., et al. (2016). Consensus statement on surgical pathology of the aorta from the society for cardiovascular pathology and the association for european cardiovascular pathology: II. noninflammatory degenerative diseases - nomenclature and diagnostic criteria. Cardiovasc. Pathol. 25, 247–257. doi: 10.1016/j.carpath.2016.03.002
Hirai, T., Sasayama, S., Kawasaki, T., and Yagi, S. (1989). Stiffness of systemic arteries in patients with myocardial infarction. A noninvasive method to predict severity of coronary atherosclerosis. Circulation 80, 78–86. doi: 10.1161/01.CIR.80.1.78
Hoffman, J. I. E., and Kaplan, S. (2002). The incidence of congenital heart disease. J. Am. Coll. Cardiol. 39, 1890–1900. doi: 10.1016/S0735-1097(02)01886-7
Januzzi, J. L., Isselbacher, E. M., Fattori, R., Cooper, J. V., Smith, D. E., Fang, J., et al. (2004). Characterizing the young patient with aortic dissection: results from the international registry of aortic dissection (IRAD). J. Am. Coll. Cardiol. 43, 665–669. doi: 10.1016/j.jacc.2003.08.054
Karatolios, K., Wittek, A., Nwe, T. H., Bihari, P., Shelke, A., Josef, D., et al. (2013). Method for aortic wall strain measurement with three-dimensional ultrasound speckle tracking and fitted finite element analysis. Ann. Thorac. Surg. 96, 1664–1671. doi: 10.1016/j.athoracsur.2013.06.037
Larson, E. W., and Edwards, W. D. (1984). Risk factors for aortic dissection: a necropsy study of 161 cases. Am. J. Cardiol. 53, 849–855. doi: 10.1016/0002-9149(84)90418-1
Laurent, S., Boutouyrie, P., Asmar, R., Gautier, I., Laloux, B., Guize, L., et al. (2001). Aortic stiffness is an independent predictor of all-cause and cardiovascular mortality in hypertensive patients. Hypertension 37, 1236–1241. doi: 10.1161/01.HYP.37.5.1236
Lee, S. Y., Shim, C. Y., Hong, G., and Seo, J. (2015). Association of aortic phenotypes and mechanical function with left ventricular diastolic function in subjects with normally functioning bicuspid aortic valves and comparison to subjects with tricuspid aortic valves. Am. J. Cardiol. 116, 1547–1554. doi: 10.1016/j.amjcard.2015.08.017
Lemaire, S. A., Wang, X., Wilks, J. A., Carter, S. A., Wen, S., Won, T., et al. (2005). Matrix metalloproteinases in ascending aortic aneurysms: bicuspid versus trileaflet aortic valves. J. Surg. Res. 123, 40–48. doi: 10.1016/j.jss.2004.06.007
Leone, O., Biagini, E., Pacini, D., Zagnoni, S., Ferlito, M., Graziosi, M., et al. (2012). The elusive link between aortic wall histology and echocardiographic anatomy in bicuspid aortic valve: implications for prophylactic surgery. Eur. J. Cardiothorac. Surg. 41, 322–327. doi: 10.1016/j.ejcts.2011.05.064
Li, Y., Deng, Y.-B., Bi, X.-J., Liu, Y.-N., Zhang, J., and Li, L. (2016). Evaluation of myocardial strain and artery elasticity using speckle tracking echocardiography and high-resolution ultrasound in patients with bicuspid aortic valve. Int. J. Cardiovasc. Imaging 32, 1063–1069. doi: 10.1007/s10554-016-0876-2
Li, Y., Wei, X., Zhao, Z., Liao, Y., He, J., Xiong, T., et al. (2017). Prevalence and complications of bicuspid aortic valve in chinese according to echocardiographic database. Am. J. Cardiol. 120, 287–291. doi: 10.1016/j.amjcard.2017.04.025
Liu, J., Shar, J. A., and Sucosky, P. (2018). Wall shear stress directional abnormalities in BAV aortas: toward a new hemodynamic predictor of aortopathy? Front. Physiol. 9:993. doi: 10.3389/fphys.2018.00993
Longobardo, L., Carerj, M. L., Pizzino, G., Bitto, A., Piccione, M. C., Zucco, M., et al. (2017). Impairment of elastic properties of the aorta in bicuspid aortic valve: relationship between biomolecular and aortic strain patterns. Eur. Heart J. Cardiovasc. Imaging 19, 879–887. doi: 10.1093/ehjci/jex224
Maleki, S., Kjellqvist, S., Paloschi, V., Magné, J., Branca, R. M. M., Du, L., et al. (2016). Mesenchymal state of intimal cells may explain higher propensity to ascending aortic aneurysm in bicuspid aortic valves. Sci. Rep. 6:35712. doi: 10.1038/srep35712
Matura, L. A., Matura, L. A., Ho, V. B., Rosing, D. R., and Bondy, C. A. (2007). Aortic dilatation and dissection in turner aortic dilatation and dissection in turner syndrome. Circulation 116, 1663–1670. doi: 10.1161/CIRCULATIONAHA.106.685487
Michelena, H. I., Desjardins, V. A., Avierinos, J. F., Russo, A., Nkomo, V. T., Sundt, T. M., et al. (2008). Natural history of asymptomatic patients with normally functioning or minimally dysfunctional bicuspid aortic valve in the community. Circulation 117, 2776–2784. doi: 10.1161/CIRCULATIONAHA.107.740878
Michelena, H. I., Khanna, A. D., Mahoney, D., Margaryan, E., Topilsky, Y., Suri, R. M., et al. (2011). Incidence of aortic complications in patients with bicuspid aortic valves. JAMA 306, 1104–1112. doi: 10.1001/jama.2011.1286
Mirault, T., Pernot, M., Frank, M., Couade, M., Niarra, R., Azizi, M., et al. (2015). Carotid stiffness change over the cardiac cycle by ultrafast ultrasound imaging in healthy volunteers and vascular Ehlers-Danlos syndrome. J. Hypertens. 33, 1890–1896; discussion 1896. doi: 10.1097/HJH.0000000000000617
Nataatmadja, M. (2003). Abnormal extracellular matrix protein transport associated with increased apoptosis of vascular smooth muscle cells in marfan syndrome and bicuspid aortic valve thoracic aortic aneurysm. Circulation 108, II329–II334. doi: 10.1161/01.cir.0000087660.82721.15
Nistri, S., Grande-Allen, J., Noale, M., Basso, C., Siviero, P., Maggi, S., et al. (2008). Aortic elasticity and size in bicuspid aortic valve syndrome. Eur. Heart J. 29, 472–479. doi: 10.1093/eurheartj/ehm528
Niwa, K., Perloff, J. K., Bhuta, S. M., Laks, H., Drinkwater, D. C., Child, J. S., et al. (2001). Structural abnormalities of great arterial walls in congenital heart disease light and electron microscopic analyses. Circulation 103, 393–400. doi: 10.1161/01.cir.103.3.393
Nollen, G. J., Groenink, M., Tijssen, J. G. P., Van Der Wall, E. E., and Mulder, B. J. M. (2004). Aortic stiffness and diameter predict progressive aortic dilatation in patients with Marfan syndrome. Eur. Heart J. 25, 1146–1152. doi: 10.1016/j.ehj.2004.04.033
Oishi, Y., Mizuguchi, Y., Miyoshi, H., Iuchi, A., Nagase, N., and Oki, T. (2008). A novel approach to assess aortic stiffness related to changes in aging using a two-dimensional strain imaging. Echocardiography 25, 941–945. doi: 10.1111/j.1540-8175.2008.00725.x
Okamoto, R. J., Wagenseil, J. E., DeLong, W. R., Peterson, S. J., Kouchoukos, N. T., and Sundt, T. M. (2002). Mechanical properties of dilated human ascending aorta. Ann. Biomed. Eng. 30, 624–635. doi: 10.1114/1.1484220
Oulego-Erroz, I., Alonso-Quintela, P., Mora-Matilla, M., Minaya, S. G., and De Armentia, S. L. L. (2013). Ascending aorta elasticity in children with isolated bicuspid aortic valve. Int. J. Cardiol. 168, 1143–1146. doi: 10.1016/j.ijcard.2012.11.080
Pape, L. A., Tsai, T. T., Isselbacher, E. M., Oh, J. K., O’Gara, P. T., Evangelista, A., et al. (2007). Aortic diameter ≥ 5.5 cm is not a good predictor of type A aortic dissection: observations from the International Registry of Acute Aortic Dissection (IRAD). Circulation 116, 1120–1127. doi: 10.1161/CIRCULATIONAHA.107.702720
Parai, J. L., Masters, R. G., Walley, V. M., Stinson, W. A., and Veinot, J. P. (1999). Aortic medial changes associated with bicuspid aortic valve: myth or reality? Can. J. Cardiol. 15, 1233–1238.
Pees, C., and Michel-behnke, I. (2012). Morphology of the bicuspid aortic valve and elasticity of the adjacent aorta in children. AJC 110, 1354–1360. doi: 10.1016/j.amjcard.2012.06.043
Pham, T., Caitlin, M., Elefteriades, J., and Sun, W. (2013). Biomechanical characterization of ascending aortic aneurysm with concomitant bicuspid aortic valve and bovine aortic arch. Acta Biomater. 9, 7927–7936. doi: 10.1016/j.actbio.2013.04.021
Phillippi, J. A., Green, B. R., Eskay, M. A., Kotlarczyk, M. P., Hill, M. R., Robertson, A. M., et al. (2014). Mechanism of aortic medial matrix remodeling is distinct in patients with bicuspid aortic valve. J. Thorac. Cardiovasc. Surg. 147, 1056–1064. doi: 10.1016/j.jtcvs.2013.04.028
Piatti, F., Sturla, F., Bissell, M. M., Pirola, S., Lombardi, M., Nesteruk, I., et al. (2017). 4D Flow Analysis of BAV-related fluid-dynamic alterations: evidences of wall shear stress alterations in absence of clinically-relevant aortic anatomical remodeling. Front. Physiol. 8:441. doi: 10.3389/fphys.2017.00441
Pichamuthu, J. E., Phillippi, J. A., Cleary, D. A., Chew, D. W., Hempel, J., Vorp, D. A., et al. (2013). Differential tensile strength and collagen composition in ascending aortic aneurysms by aortic valve phenotype. Ann. Thorac. Surg. 96, 2147–2154. doi: 10.1016/j.athoracsur.2013.07.001.Differential
Reference Values for Arterial Stiffness’ Collaboration (2010). Determinants of pulse wave velocity in healthy people and in the presence of cardiovascular risk factors: “establishing normal and reference values. Eur. Heart J. 31, 2338–2350. doi: 10.1093/eurheartj/ehq165
Roach, M. R., and Burton, A. C. (1957). The reason for the shape of the distensibility curves of arteries. Can. J. Biochem. Physiol. 35, 681–690. doi: 10.1139/y57-080
Roberts, C. S., and Roberts, W. C. (1991). Dissection of the aorta associated with congenital malformation of the aortic valve. J. Am. Coll. Cardiol. 17, 712–716. doi: 10.1016/S0735-1097(10)80188-3
Salvi, P., Grillo, A., Marelli, S., Gao, L., Salvi, L., Viecca, M., et al. (2018). Aortic dilatation in Marfan syndrome. J. Hypertens. 36, 77–84. doi: 10.1097/HJH.0000000000001512
Santarpia, G., Scognamiglio, G., Di Salvo, G., D’Alto, M., Sarubbi, B., Romeo, E., et al. (2012). Aortic and left ventricular remodeling in patients with bicuspid aortic valve without significant valvular dysfunction: a prospective study. Int. J. Cardiol. 158, 347–352. doi: 10.1016/j.ijcard.2011.01.046
Schaefer, B. M., Lewin, M. B., Stout, K. K., Byers, P. H., and Otto, C. M. (2007). Usefulness of bicuspid aortic valve phenotype to predict elastic properties of the ascending aorta. Am. J. Cardiol. 99, 686–690. doi: 10.1016/j.amjcard.2006.09.118
Shim, C. Y., Cho, I. J., Yang, W. I., Kang, M. K., Park, S., Ha, J. W., et al. (2011). Central aortic stiffness and its association with ascending aorta dilation in subjects with a bicuspid aortic valve. J. Am. Soc. Echocardiogr. 24, 847–852. doi: 10.1016/j.echo.2011.04.017
Sievers, H. H., and Sievers, H. L. (2011). Aortopathy in bicuspid aortic valve disease - genes or hemodynamics? or Scylla and Charybdis? Eur. J. Cardiothorac. Surg. 39, 803–804. doi: 10.1016/j.ejcts.2011.02.007
Tsamis, A., Phillippi, J. A., Koch, R. G., Chan, P. G., Krawiec, J. T., D’Amore, A., et al. (2016). Extracellular matrix fiber microarchitecture is region-specific in bicuspid aortic valve-associated ascending aortopathy. J. Thorac. Cardiovasc. Surg. 151, 1718–1728.e5. doi: 10.1016/j.jtcvs.2016.02.019
Tzemos, N., Lyseggen, E., Silversides, C., Jamorski, M., Tong, J. H., Harvey, P., et al. (2010). Endothelial function, carotid-femoral stiffness, and plasma matrix metalloproteinase-2 in men with bicuspid aortic valve and dilated aorta. J. Am. Coll. Cardiol. 55, 660–668. doi: 10.1016/j.jacc.2009.08.080
Tzemos, N., Therrien, J., Yip, J., Thanassoulis, G., Tremblay, S., Jamorski, M. T., et al. (2008). Outcomes in adults with bicuspid aortic valves. JAMA 300, 1317–1325. doi: 10.1001/jama.300.11.1317
Ward, C. (2000). Clinical significance of the bicuspid aortic valve. Heart 83, 81–85. doi: 10.1136/heart.83.1.81
Waters, K. M., Rooper, L. M., Guajardo, A., and Halushka, M. K. (2017). Histopathologic differences partially distinguish syndromic aortic diseases. Cardiovasc. Pathol. 30, 6–11. doi: 10.1016/j.carpath.2017.05.008
Weismann, C. G., Lombardi, K. C., Grell, B. S., Northrup, V., and Sugeng, L. (2016). Aortic stiffness and left ventricular diastolic function in children with well-functioning bicuspid aortic valves. Eur. Hear. J. Cardiovasc. Imaging 17, 225–230. doi: 10.1093/ehjci/jev151
Wolinsky, H., and Glagov, S. (1964). Structural basis for the static mechanical properties of the aortic media. Circ. Res. 14, 400–413. doi: 10.1161/01.RES.14.5.400
Keywords: bicuspid aortic valve, thoracic aorta, arterial stiffness, Mucoid Extracellular Matrix Accumulation, ultrasound diagnosis, carotid artery disease
Citation: Goudot G, Mirault T, Bruneval P, Soulat G, Pernot M and Messas E (2019) Aortic Wall Elastic Properties in Case of Bicuspid Aortic Valve. Front. Physiol. 10:299. doi: 10.3389/fphys.2019.00299
Received: 02 December 2018; Accepted: 06 March 2019;
Published: 10 April 2019.
Edited by:
Qiyin Fang, McMaster University, CanadaReviewed by:
Qianyu Guo, Tianjin First Central Hospital, ChinaEmiliano Votta, Polytechnic University of Milan, Italy
Copyright © 2019 Goudot, Mirault, Bruneval, Soulat, Pernot and Messas. This is an open-access article distributed under the terms of the Creative Commons Attribution License (CC BY). The use, distribution or reproduction in other forums is permitted, provided the original author(s) and the copyright owner(s) are credited and that the original publication in this journal is cited, in accordance with accepted academic practice. No use, distribution or reproduction is permitted which does not comply with these terms.
*Correspondence: Emmanuel Messas, emmanuel.messas@aphp.fr