- 1Helsinki Institute of Life Science, Institute of Biotechnology, University of Helsinki, Helsinki, Finland
- 2Orthodontics, Department of Oral and Maxillofacial Diseases, University of Helsinki, Helsinki, Finland
- 3Departamento de Desarrollo y Reparación Cardiovascular, Centro Nacional de Investigaciones Cardiovasculares (CNIC), Madrid, Spain
- 4The Institute for Neurosciences of Montpellier, Inserm UMR1051, University of Montpellier, Saint Eloi Hospital, Montpellier, France
- 5Orthodontics, Oral and Maxillofacial Diseases, Helsinki University Hospital, Helsinki, Finland
MEIS1 is a key developmental regulator of several organs and participates in stem cell maintenance in different niches. However, despite the murine continuously growing incisor being a well described model for the study of adult stem cells, Meis1 has not been investigated in a dental context. Here, we uncover that Meis1 expression in the tooth is confined to the epithelial compartment. Its expression arises during morphogenesis and becomes restricted to the mouse incisor epithelial stem cell niche, the labial cervical loop. Meis1 is specifically expressed by Sox2+ stem cells, which give rise to all dental epithelial cell lineages. Also, we have found that Meis1 in the incisor is coexpressed with potential binding partner Pbx1 during both embryonic and adult stages. Interestingly, Meis2 is present in different areas of the forming tooth and it is not expressed by dental epithelial stem cells, suggesting different roles for these two largely homologous genes. Additionally, we have established the expression patterns of Meis1 and Meis2 during tongue, hair, salivary gland and palate formation. Finally, analysis of Meis1-null allele mice indicated that, similarly, to SOX2, MEIS1 is not essential for tooth initiation, but might have a role during adult incisor renewal.
Introduction
Similar to human teeth, enamel cannot be regenerated in murine molars, as enamel-producing ameloblasts are lost after tooth eruption into the oral cavity. In contrast, in the mouse incisor, epithelial stem cells located in the labial cervical loop continuously produce ameloblasts and enable constant enamel renewal. This unceasing tooth growth is compensated by abrasion from gnawing. Murine dental epithelial stem cells express the transcription factor SOX2 (Juuri et al., 2012). SOX2 is required to maintain the pluripotent state of cells during early fetal development and it regulates the development of various tissues (Avilion et al., 2003; Pevny and Nicolis, 2010). Sox2 is expressed throughout dental morphogenesis, and it marks progenitor cells that contribute to the successional molar tooth and epithelial stem cells in the incisor (Juuri et al., 2012, 2013). Loss of Sox2 expression during dental morphogenesis leads to aberrant tooth shape, incorrect cell differentiation and, in the most extreme cases, tooth regression. In the adult incisor, lack of Sox2 delays epithelial stem cell renewal and may trigger cellular plasticity events in order to restore a Sox2-expressing cell population (Sun et al., 2016; Sanz-Navarro et al., 2018).
Meis1 gene expression is enriched in the transcriptome of Sox2+ dental epithelial cells, compared to that of murine embryonic stem cells (Sanz-Navarro et al., 2018). Meis1 is a homeobox-containing transcription factor belonging to the Three Amino Acid Loop Extension-homeodomain (TALE) superclass and, together with Meis2 and Meis3, form the Meis subfamily (Longobardi et al., 2014). Meis1 is a key regulator of the development of several organs and participates in the maintenance of stem cells (Mercader et al., 1999; Hisa et al., 2004; Unnisa et al., 2012; Okumura et al., 2014; Marcos et al., 2015). Furthermore, altered expression of this gene has been linked to cancer, including leukemia and neuroblastoma (Moskow et al., 1995; Geerts et al., 2003; Argiropoulos et al., 2007). MEIS1 often acts in cooperation with HOX proteins and PBX transcription factors, however, it can also act independently. For example, in the murine embryonic trunk, MEIS1 cooperates with HOX/PBX proteins, while the role of MEIS1 in eye formation is HOX/PBX-independent (Penkov et al., 2013; Longobardi et al., 2014; Marcos et al., 2015).
To date, despite the importance of homeobox genes in tooth development (Ramanathan et al., 2018), Meis genes have not been described in the dental context. Here, we analyse the expression pattern of Meis1 in the forming tooth and in the renewing mouse incisor. Additionally, we compare Meis1 expression relative to Meis2 and Sox2, and demonstrate that Meis1 and Sox2 are coexpressed in the incisor labial cervical loop. Finally, we show evidence that similarly, to SOX2, MEIS1 is not necessary for tooth initiation.
Materials and Methods
Mouse Lines
Wild type mice used for this study were from the outbred NMRI strain. Meis1ECFP/ECFP were used as Meis1 knockout (MeisKO) (González-Lázaro et al., 2014). A total of five MeisKO embryos from four different litters were analyzed in this study, with their corresponding control littermates. The number of teeth analyzed in each experiment is specified in the text.
The stage of the embryos was determined according to the day of the vaginal plug, which was set as embryonic day 0.5 (E0.5). Postnatal day was determined from the day of birth (P0). All aspects of mouse care and experimental protocols were approved by the University of Helsinki, and the Southern Finland Council on Animal Welfare and Ethics and in accordance to the Spanish bioethical regulations for laboratory animals.
Tissue Processing
Murine tissues were fixed in 4% paraformaldehyde (PFA) overnight at 4°C, E18.5 and adult samples were decalcified by incubating them for 2 weeks in 0.5M EDTA pH 7.5. Tissues were dehydrated in an ethanol series and embedded in paraffin. Paraffin blocks were processed into 5 μm-thick sections using a microtome.
Histology
For histological analysis, samples were rehydrated using an ethanol series of decreasing concentration, incubated 2 min in haematoxylin, 30 s in eosin, and further dehydrated in an ethanol series, following xylene treatment and mounting. Samples were imaged with light microscopy (BX61, Olympus).
RNAscope in situ Hybridization
Duplex RNAscope in situ hybridization (Advanced Cell Diagnostics) is a robust and sensitive method to detect single RNA molecules (Wang et al., 2012). We performed the experiments as previously described (Sanz-Navarro et al., 2018). In single-gene in situ RNAscope transcripts appear in red. In duplex in situ RNAscope, Channel 1 probes (C1) appear in blue color, while channel 2 probes (C2) are revealed in red. Meis1-C2 (436361-C2), Meis2-C1 (436371), Sox2-C1 (401041) and Pbx1-C1 (435171) probes were purchased from Advanced Cell Diagnostics. Images were obtained using a bright field microscope (BX61, Olympus). Borders of the oral and dental epithelium were drawn using an image processor.
Immunohistochemistry
Samples were deparaffinised, rehydrated and permeabilised as previously described (Sanz-Navarro et al., 2018). Anti-MEIS1 primary antibody was used at a dilution of 1:1000 (rabbit, Abcam, ab19867), anti-SOX2 at 1:200 (goat, Santa Cruz, SC-17320) and anti-KI67 at 1:200 (rabbit, Abcam, ab16667). Secondary antibodies Alexa488 donkey anti-goat (Invitrogen) and Alexa568 goat anti-rabbit (Invitrogen) were used at 1:400. Samples were counterstained by incubating with Hoechst (1:1000, Invitrogen). Results were imaged by means of fluorescent microscopy (BX61, Olympus) and images were processed using Adobe Photoshop.
Micro Computed Tomography (Micro-CT Scan)
Lower jaws from E14 MeisKO and control littermates were fixed in 4% PFA overnight at 4°C and incubated in a solution of 0.3% phosphotungstic acid (PTA) in 70% ethanol for 6 days. Samples were embedded into 1% low-melting point agarose and scanned with a micro-CT scanner (Bruker SKYSCAN). Reconstructions of the teeth were prepared with Avizo software (Thermo Fisher Scientific).
Results
Meis1 Is Expressed in the Dental Epithelium
Meis1 expression has been discovered in several developing organs, however, its presence has never been characterized during the development of ectodermal organs. Thus, we analyzed Meis1 expression in the developing molar and incisor teeth with RNAscope in situ hybridization. Four technical replicates were used for each stage.
Even though tooth induction events are similar for all dental types, morphogenesis of the murine molar and incisor diverges shortly after (Figure 1). For one, while the molar grows vertically, after embryonic day 14.0 (E14.0) the incisor rotates anterio-posteriorly and extends longitudinally. As the incisor elongates, the cervical loops, two bulge-like structures located at the proximal end of the tooth, become asymmetrical. The labial cervical loop enlarges and becomes the epithelial stem cell niche. Dental epithelial stem cells in the labial cervical loop give rise to rapidly dividing progenitor cells that will migrate distally toward the distal tip of the incisor and differentiate into ameloblasts (Thesleff and Tummers, 2008; Jussila and Thesleff, 2012).
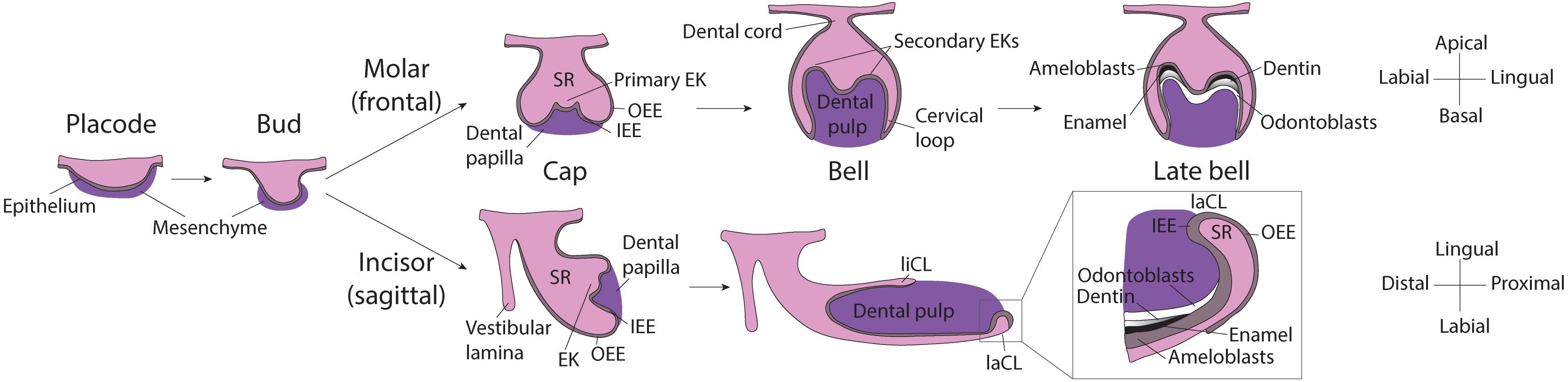
Figure 1. Scheme of murine molar and incisor development. Placode stage: At E12.5, interactions between the oral epithelium and the underlying mesenchyme cause the formation of the dental placode. Bud stage: At E13.5 the dental epithelium proliferates into the mesenchyme and buds. Cap stage: At E14.5 the primary enamel knot forms at the tip of the bud and orchestrates the proliferation of the surrounding epithelium. In the molar, the dental epithelium grows downward, while in the incisor it grows in the longitudinal axis, the expansion is led by cervical loops at the base of the tooth. The enamel organ and is composed of: stellate reticulum, stratum intermedium and enamel epithelium (inner and outer). Molar bell and late bell stages: In molars, at bell stage, secondary EKs mark the sites where the cusps will form. Mineralization starts from the crown and, when it reaches the cervical loops, the tooth starts to erupt and root formation starts. By this time, all enamel-producing cells are lost. Incisor bell and late bell stages: During bell stage, the incisor becomes asymmetrically patterned in the labial-lingual axis, the larger labial cervical loop contains stem cells that continuously generate enamel-producing ameloblasts. Enamel is deposited only on the labial side of the incisor while the lingual side is covered by dentin and cementum. EK, Enamel knot; OEE, Outer enamel epithelium; IEE, Inner enamel epithelium; SR, Stellate reticulum; liCL, lingual cervical loop; laCL, labial cervical loop.
In the course of early molar formation, a negligible amount of Meis1 transcripts was detected in the dental placode and oral epithelium (Figures 2A,A’). This expression pattern was maintained during bud stage (Figures 2B,B’). During cap and bell stages, Meis1 was expressed at the cervical loops (Figures 2C–D’).
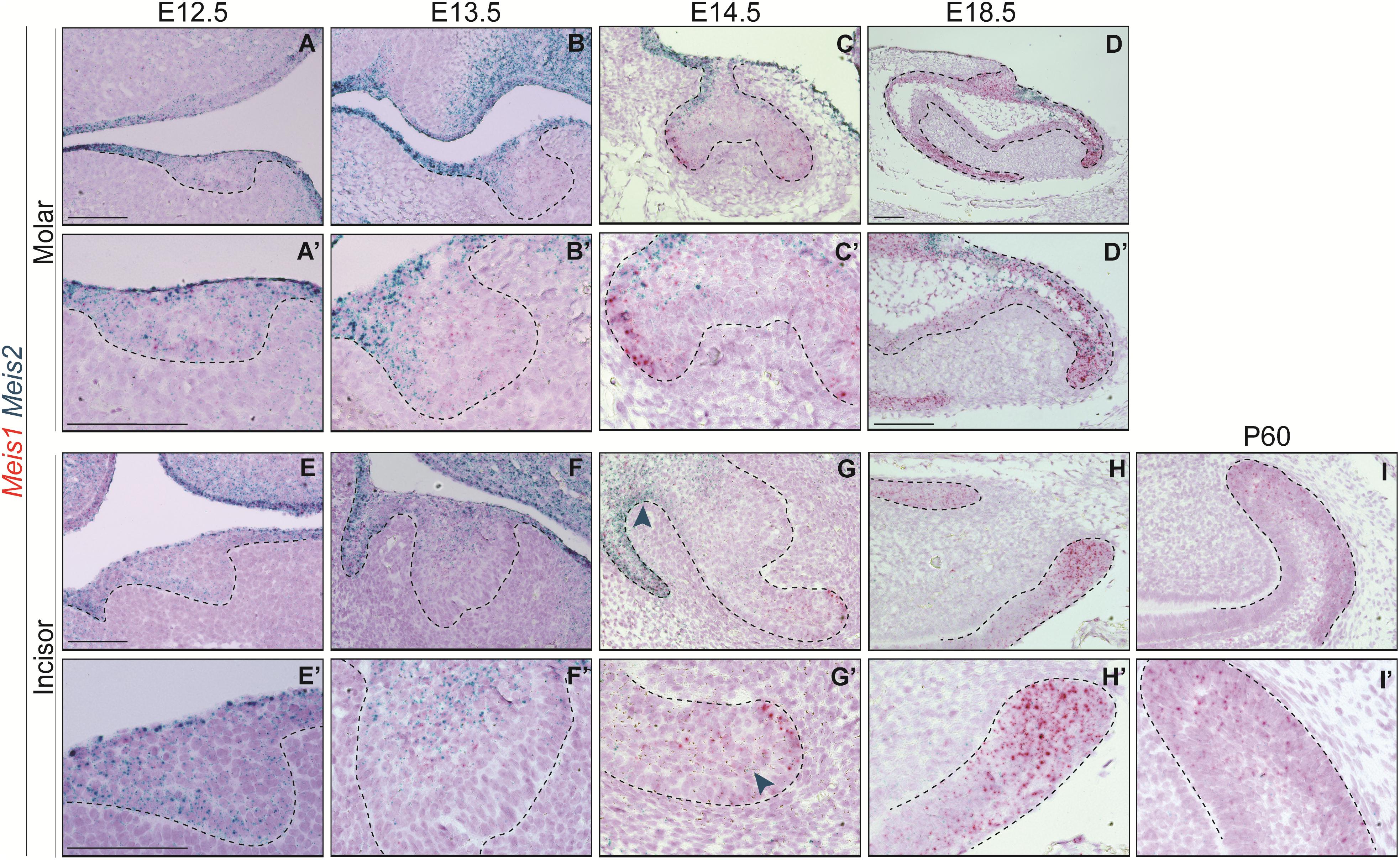
Figure 2. Meis1 and Meis2 expression patterns in molar and incisor formation. Frontal (A–C,E) and sagittal (D,F–I) sections of E12.5–E18.5 wild type mice. Meis1 is lowly expressed in the oral and dental epithelium at placode and bud stages (A,B,E,F). Its expression increases and becomes restricted to the cervical loops during cap and bell stages (C,D,G,H). Meis2 is strongly expressed in the oral epithelium and in the dental placode (A’,E’). Meis2 expression is restricted to the dental cord and vestibular lamina during the bud and cap stages (B’,C’,F’,G’). During bell stage, Meis2 is present in the proximal fraction of the molar (D’) but not in the incisor labial cervical loop (H’). In the adult incisor (P60), Meis1 is specifically expressed in the labial cervical loop, while Meis2 transcripts are no longer detected in the organ (I’). Scale bars 100 μm. Dashed line labels epithelial tissue.
During the early stages of incisor morphogenesis, Meis1 was almost undetectable in the dental epithelium (Figures 2E–F’). By cap stage, Meis1 expression localized to the labial cervical loop (Figures 2G,G’), and its expression increased by bell stage (Figures 2H,H’). At E18.5, a few transcripts were present in the lingual cervical loop (Figure 2H). During incisor renewal (P60), Meis1 was strongly expressed in the incisor stem cell niche (Figures 2I,I’). However, the lingual cervical loop is Meis1-negative (data not shown). Also, Meis1 is expressed in the vestibular lamina throughout embryonic development. The vestibular lamina originates from the oral epithelium and generates the oral vestibule, a band located between the dentition and the lips and cheeks (Hovorakova et al., 2016). Correspondingly, MEIS1 protein was detected in the cells of the vestibular lamina and the forming labial cervical loop at E14.5 (Figure 3A). During postnatal and adult stages, majority of cells in the labial cervical loop expressed MEIS1 (Figures 3B,C). Additionally, a number of epithelial transit amplifying cells are MEIS1-positive at P3.
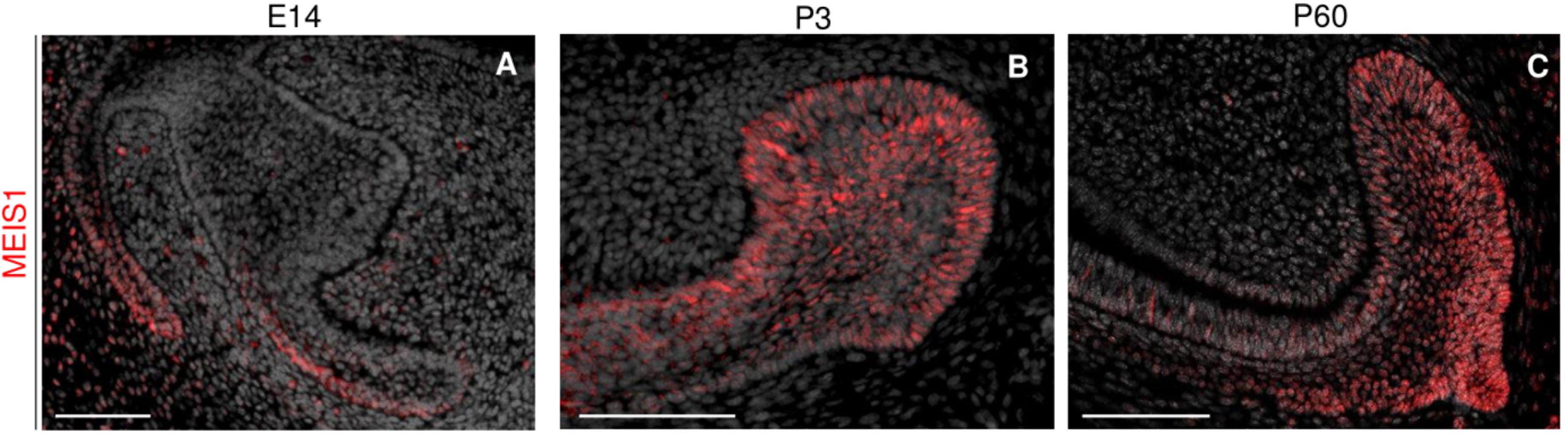
Figure 3. Immunodetection of MEIS1 in the forming and adult incisor. MEIS1 protein expression is detected in the incisor labial cervical loop around E14.5 (A). MEIS1 expression is detected in the labial cervical loop and transit amplifying cells during postnatal day three (B). During adult (P60) incisor renewal, MEIS1 specifically marks the labial cervical loop (C). Scale bars 100 μm.
Mechanistically, MEIS1 regulates transcription by binding the DNA as a dimer, and MEIS1/PBX1 complexes are commonly found (Capellini et al., 2011; Longobardi et al., 2014). Moreover, PBX1 has been proposed to play a role in tooth patterning (Schnabel et al., 2001). We found that Pbx1 and Meis1 were expressed in the same cells, as Pbx1 was ubiquitously expressed throughout the tissue at all developmental stages (Figures 4A–D).
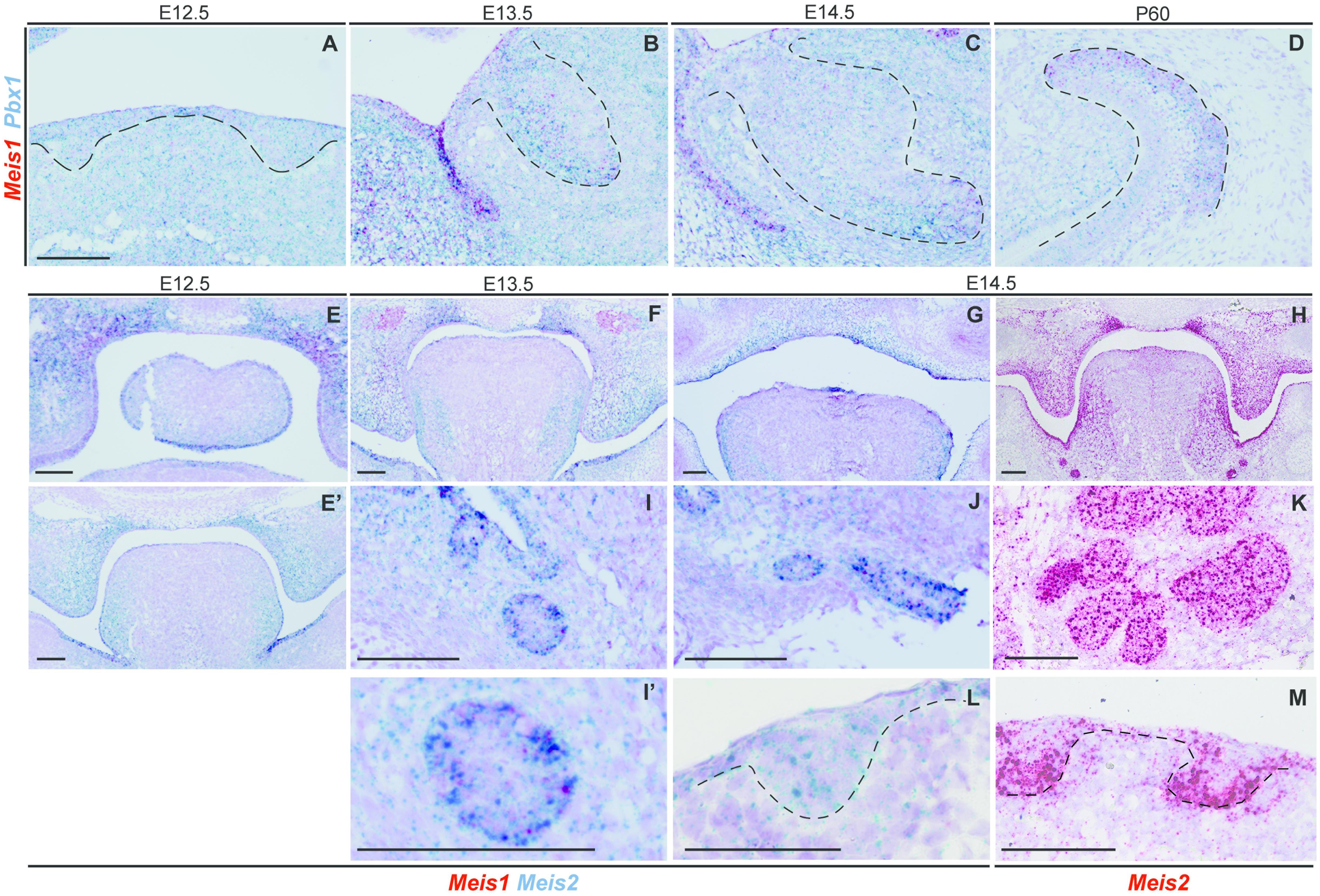
Figure 4. Expression of Pbx1 in the forming incisor and Meis1/2 expression patterns in embryonic palate, submandibular gland and hair follicle. Incisor cells expressing Meis1 in the incisor also express Pbx1, as the latter is ubiquitously expressed thorough the epithelial and mesenchymal compartments during all stages of incisor development (A–D). At E12.5 Meis1 is expressed in the frontal region of the palate, both in the mesenchymal and epithelial compartments, Meis2 is also expressed in the palatal region and in the tongue (E); Meis1 is not expressed in the posterior region of the oral cavity and Meis2 marks the mesenchymal compartment of the palatal shelves and the tongue (E’). A similar pattern is present at the posterior region of the oral cavity at E13.5 (F). At E14.5 Meis2 is expressed in both epithelial and mesenchymal compartments of the fused palate, as detected using Duplex RNAscope (G) and red-channel RNAscope (H) detection kits. Meis1 and Meis2 are expressed by the forming submandibular gland (I–K), while the hair follicle only expresses Meis2 at E14.5 (L,M). Scale bars 100 μm. Dashed line labels epithelial tissue.
Meis1 and Meis2 Are Expressed in Different Regions of the Developing Tooth
MEIS1 and MEIS2 proteins have a similar sequence (83% identity) and an almost identical homeodomain (Oulad-Abdelghani et al., 1997). It has been proposed that they could have a redundant function during ocular lens development and neural crest cell specification (Machon et al., 2015; Antosova et al., 2016). Also, in the developing vascular system, conditional overexpression of Meis2 can compensate for Meis1 deficiency (Marcos et al., 2015). Therefore we were interested to see whether these two genes were coexpressed during tooth development. Four technical replicates were used for each stage.
At E12.5, Meis2 was expressed in the oral epithelium and the molar placode, especially in the uppermost suprabasal cells (Figures 2A,A’). At the molar bud stage, Meis2 was expressed in the oral epithelium and in the area where the dental cord will form (Figure 2B). Similarly, during cap stage, Meis2 was expressed in the buccal side of the dental cord, the epithelium that connects the enamel organ to the oral surface (Figures 2C,C’). At E18.5, Meis2 partially overlapped with Meis1, as it was expressed in the posterior region of the molar, including the cervical loop (Figures 2D,D’).
Similarly, Meis2 was weakly expressed in the incisor placode and the surrounding oral epithelium at E12.5 (Figures 2E,E’). During bud stage, Meis2 was expressed in the apical region of the incisor and the vestibular lamina (Figures 2F,F’). At E14.5, Meis2 expression was mostly confined to the vestibular lamina and dental cord (Figure 2G). Meis2 expression decreased during tooth morphogenesis, and it was undetectable in the incisor labial cervical loop during late embryogenesis and adult stages (Figures 2H,H’ and data not shown).
Altogether, Meis2 was more strongly expressed during early tooth formation and was mostly restricted to the oral epithelium, vestibular lamina, and dental cord regions. In contrast, Meis1 expression appeared in the vestibular lamina and cervical loops later during development. Hence, these two genes were expressed in the forming tooth with minimal overlap.
Meis1 and Meis2 Are Differentially Expressed in the Forming Palate, Tongue, Hair Follicle and Submandibular Gland
Besides teeth, Meis1 and Meis2 were expressed in other tissues of the developing head, including the palate. Four technical replicates were used for each analyzed stage.
At E12.5 Meis1 was expressed in the frontal region of the palate (Figure 4E). Both frontal and posterior palate expressed Meis2 in the epithelium and, more strongly, in the mesenchyme (Figures 4E,E’). Meis2 expression was strong throughout palatal development, as it marked the palatal shelves at E13.5 (Figure 4F) and the fused palate at E14.5 (Figures 4G,H). Also, during development, Meis2 was expressed in the tongue mesenchyme and in the ventro-lateral region of the epithelium (Figures 4G,H).
Ectodermal organs, including teeth, have a very similar early development, and only after bud stage each organ undergoes particular morphogenesis. Thus, we investigated Meis1 and Meis2 expression in developing salivary glands and hair. The submandibular salivary glands expressed Meis1 and, more strongly, Meis2 during development (Figures 4I,K). Meis1 expression was restricted to the epithelial fraction of the organ, while Meis2 transcripts were identified also in the surrounding mesenchyme. The epithelial compartment of the forming hair follicle strongly expressed Meis2, while a few Meis2 transcripts were expressed by the underlying mesenchyme (Figures 4L,M).
Meis1 and Sox2 Are Coexpressed in the Cervical Loops During Tooth Development
As Meis1 expression was restricted to the labial cervical loop after E14.5, we wanted to compare its expression with that of Sox2, a known marker for dental epithelial stem cells (Juuri et al., 2012). In a previous report, we analyzed the transcriptome of Sox2+ cells at E14.5 and during adult renewal (P60), and compared them to the transcriptome of naïve murine embryonic stem cells (mESCs) (Sanz-Navarro et al., 2018). In silico analysis of these results (deposited at Gene Expression Omnibus, GSE104808) showed that Meis1 expression is 29.62 fold higher in E14.5 dental Sox2+ cells than in mESCs (Pvalue = 0.00003, ANOVA), similar to that of Pitx2 (29.56 fold, Pvalue = 0.012, ANOVA), a known regulator of the incisor stem cell niche (Sun et al., 2016). Similarly, when comparing adult Sox2+ dental cells and mESCs, Meis1 was 7.34 fold enriched in the adult Sox2+ cells (Pvalue = 0.017, ANOVA). Hence, Meis1 was 3.93 fold enriched in embryonic Sox2+ cells, compared to the adult dental epithelial cells (Pvalue = 0.019, ANOVA). Altogether these results indicated that at least some Sox2+ cells from the dental epithelium express Meis1. To corroborate the results obtained with the transcriptomic analysis, we analyzed the spatial expression of these two genes by means of in situ hybridization. Four technical replicates were used for each analyzed stage.
In accordance with previous reports, Sox2 was expressed in the oral epithelium and molar placode (Juuri et al., 2012, 2013). During early morphogenesis Sox2 expression was mostly restricted to the lingual side of the molar epithelium (Figures 5A–C’), and at E14.5 Sox2 and Meis1 transcripts partially overlapped (Figures 5C,C’). During late bell stage, Sox2 expression was confined to the cervical loops, where Meis1 transcripts were found (Figures 5D,D’). During incisor placode and bud stages, Meis1 expression was low and scattered (Figures 5E–F’) and by E14.5 both Meis1 and Sox2 expression localized to the labial cervical loop (Figures 5G,G’). This pattern was maintained during late incisor morphogenesis (Figures 5H,H’). These results indicated a similar expression pattern between Meis1 and Sox2 during late stages of incisor and molar morphogenesis.
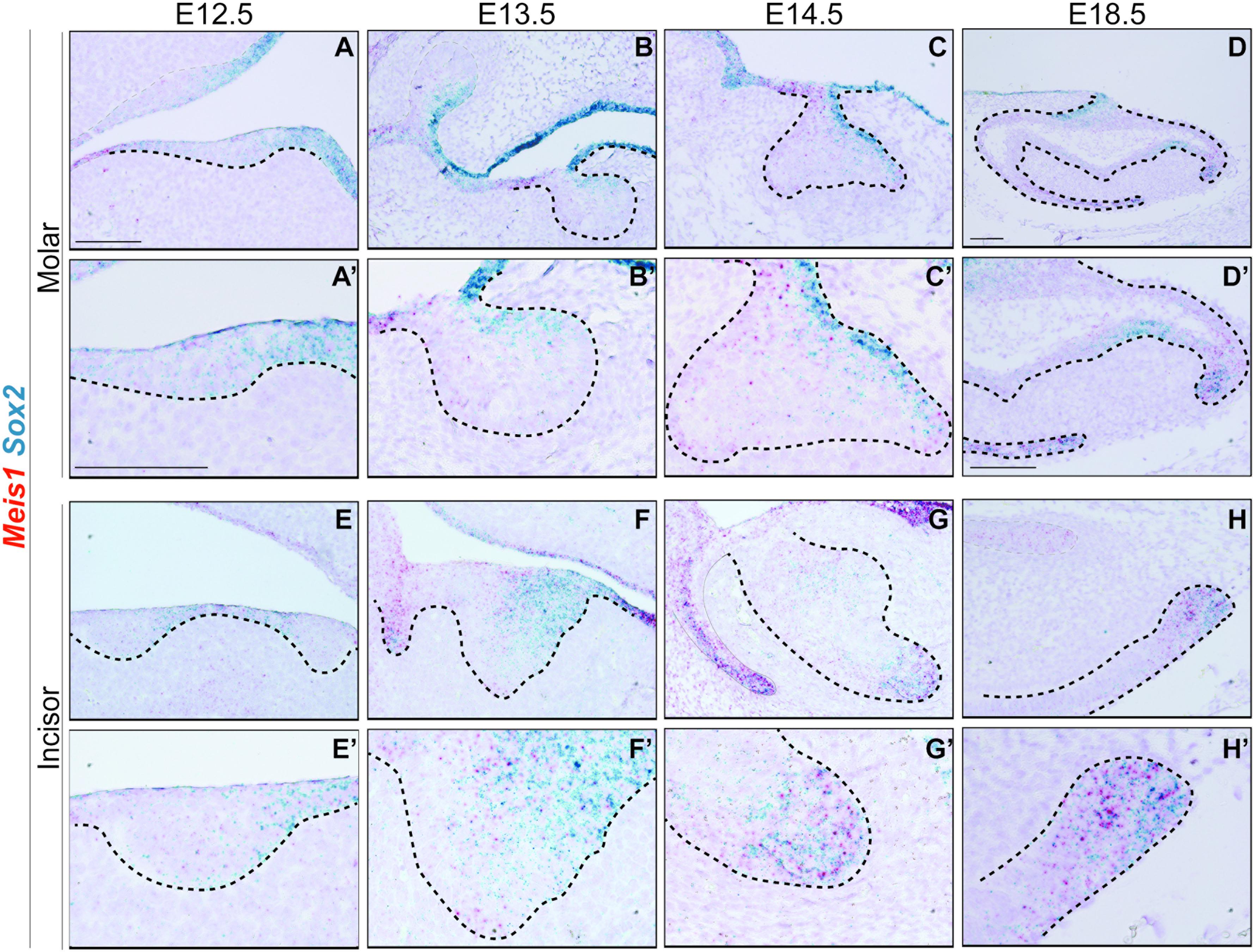
Figure 5. Meis1 and Sox2 expression patterns in developing molar and incisor. Frontal (A–C,E) and sagittal (D,F–H) sections of E12.5-E18.5 wild type mice. Sox2 is expressed in the dental placode, mostly on the lingual side, its expression partially coincides with that of Meis1 (A’,E’). Meis1 and Sox2 expression partially overlaps in the dental epithelium at the bud stage. (B’,F’). During cap stage, Meis1 and Sox2 are expressed in the developing cervical loops. Some cells from the vestibular lamina and the labial cervical loop express both MEIS1 and SOX2 (C’,G’). Toward the end of tooth morphogenesis Meis1 expression is confined to the cervical loops, in a fashion similar to that of Sox2 (D’–H’). Scale bars 100 μm. Dashed line labels epithelial tissue.
Meis1 and Sox2 Mark the Same Cells in the Adult Incisor Labial Cervical Loop
We have discovered that after birth MEIS1 expression was maintained in the labial cervical loop, where SOX2 expression has been previously described (Juuri et al., 2012). Thus, we analyzed Meis1 and Sox2 expression both at the transcriptional and protein levels to determine if they mark the same cell population. Four technical replicates were used in the in situ RNAscope analysis and three biological replicates in the immunodetection.
At P60, during incisor renewal, both Meis1 and Sox2 were expressed in the enamel epithelium and stellate reticulum of the labial cervical loop (Figure 6A). At the cell-level, we observed that a number of cells within the labial cervical loop expressed both genes (Figures 6A’,A”). MEIS1 and SOX2 protein expression completely overlapped in the adult incisor labial cervical loop (Figure 6B). Hence, adult Sox2+ stem cells also expressed Meis1.
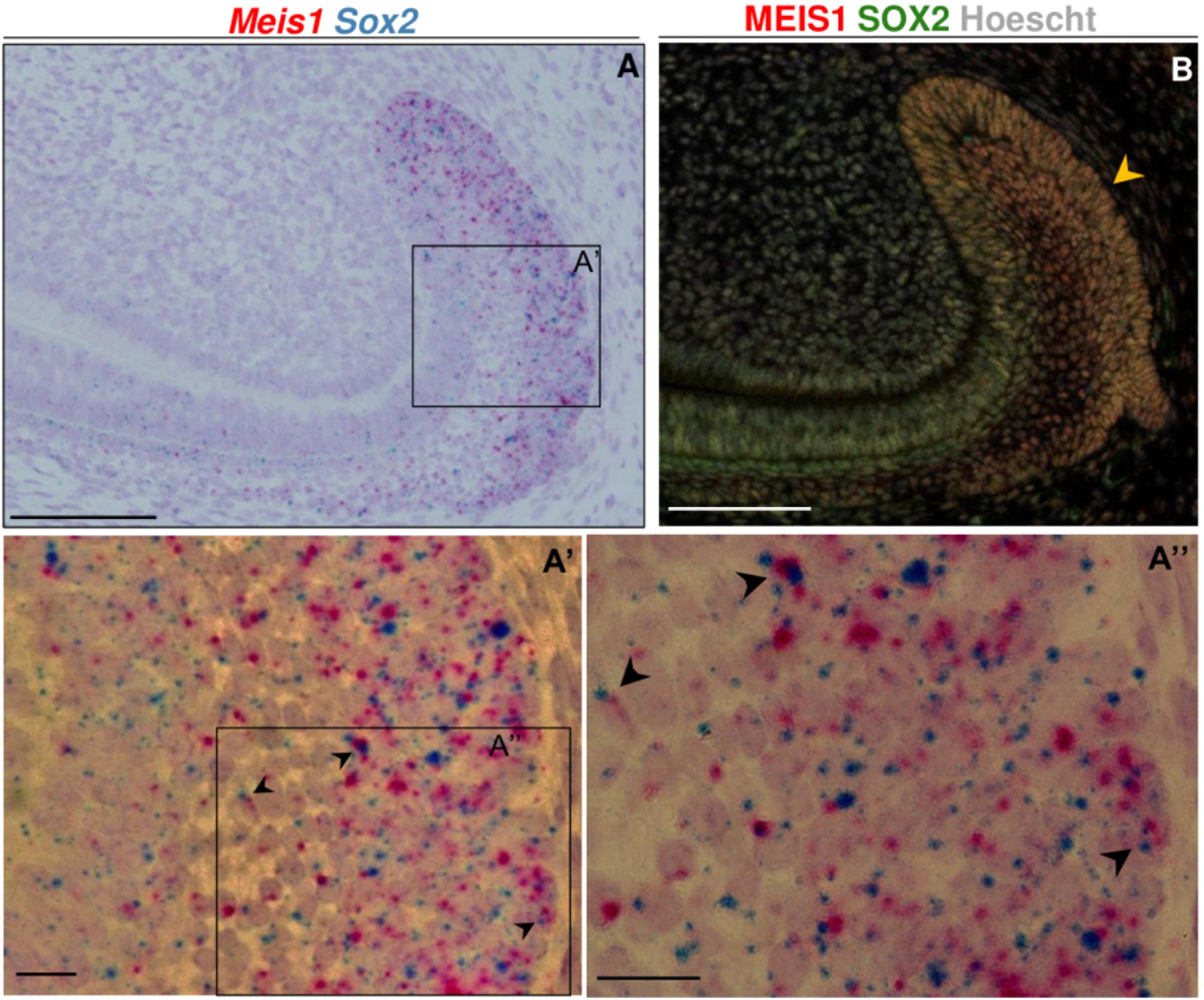
Figure 6. Meis1 and Sox2 expression in the adult incisor labial cervical loop. Meis1 and Sox2 are coexpressed in the incisor labial cervical loop (A–A”). Black arrowheads point at cells expressing both Meis1 and Sox2 transcripts. In the adult labial cervical loop MEIS1 and SOX2 mark the same cells (yellow arrowhead) (B). Scalebars (A,B), 100 μm; (A’,A”), 10 μm.
Meis1 Is Not Essential for Tooth Initiation nor for Early Sox2 Expression
In order to comprehend the role of Meis1 during tooth formation, and its relationship with Sox2 expression, we utilized Meis1-null allele embryos (Meis1ECFP/ECFP, Meis1KO) (González-Lázaro et al., 2014).
Histological analysis of Meis1KO teeth indicated the presence of developing teeth at E14.5 (N = 4) (Figures 7A–D). 3D reconstructions of the incisors of E14.5 embryos confirmed that the morphology of the Meis1KO teeth was normal (N = 4) (Figures 7E,F). Also, palatal morphology was normal at E14.5 (data not shown). By means of in situ RNAscope we confirmed that Meis1 expression was completely absent from E14.5 Meis1KO teeth and found that Sox2 expression patterns was not altered in the absence of Meis1 (N = 3, four technical replicates) (Figures 7G–J). Also, cell proliferation (KI67+ cells) and Meis2 expression pattern remained normal (Supplementary Figure S1).
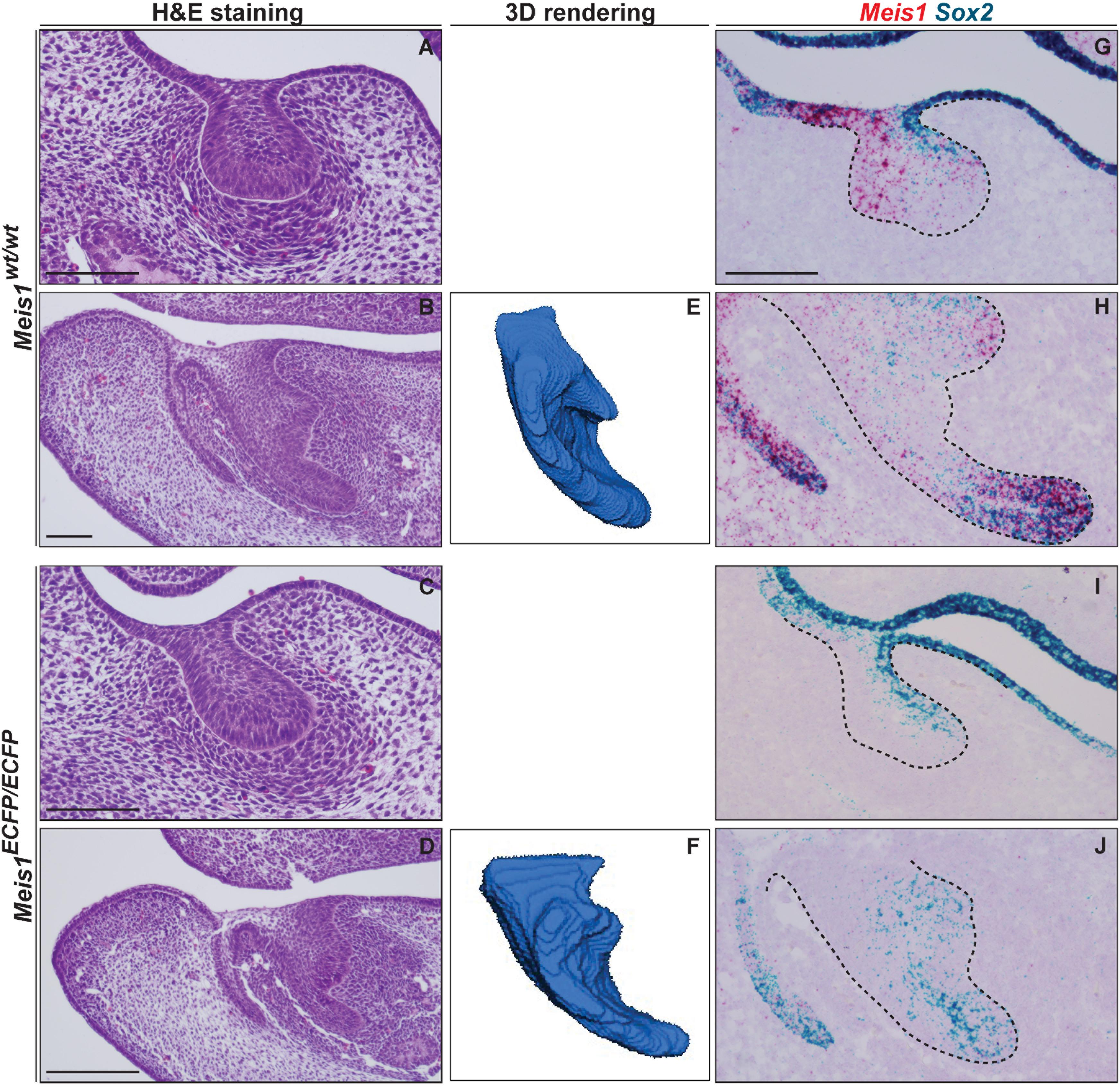
Figure 7. Tooth formation in Meis1KO embryos. Histological analysis show the presence of normal looking lower incisors and molars in Meis1KO embryos, similar to control littermates (A–D). 3D reconstructions from micro-CT scans confirm that the forming incisors have a normal morphology (E, F). RNAscope in situ hybridization shows the complete absence of Meis1 expression in Meis1KO embryos, Sox2 expression pattern remains unaltered in the absence of Meis1 expression (G–J). Scale bars 100 μm. Dashed line labels epithelial tissue.
These results suggested that Meis1 and Meis2 did not have a redundant function in the developing tooth, and that lack of phenotype at this stage was caused by the fact that Meis1 was only expressed in the cervical loops of the forming teeth at E14.5. Hence, Meis1 was dispensable during the early stages of tooth development and for early Sox2 expression. In vivo analysis of late developmental stages was not possible using Meis1-null allele mice, since they die by E14.5 (Azcoitia et al., 2005; González-Lázaro et al., 2014).
Discussion
A complex network of genes regulate tooth morphogenesis and dental epithelial stem cell formation and maintenance (Hu et al., 2014; Krivanek et al., 2017). In this report, we have for the first time characterized the expression patterns of Meis genes in the forming tooth and in the renewing incisor.
During early tooth morphogenesis Meis1 is faintly expressed throughout the dental epithelium. By E14.5, Meis1 expression increases in the forming cervical loops of the molar and in the incisor labial cervical loop, where it is maintained during postnatal stages. Meis2 is also expressed during early morphogenesis, but its expression disappears from the tooth as development progresses. Altogether, Meis1 and Meis2 expression shows minimal overlap in the tooth and absence of Meis1 does not induce a change in the expression of Meis2. This suggests a different role for these two genes, in contrast to previous research (Machon et al., 2015; Marcos et al., 2015; Antosova et al., 2016). Similarly, the differential function of Meis1 and Meis2 in the oral cavity is supported by the fact that, while absence of Meis2 expression leads to palatal abnormalities (Machon et al., 2015), we have not observed any palatal phenotype in the Meis1KO embryos at E14.5.
Meis1 and Meis2 are also differentially expressed in other ectodermal organs, such as hair, where only Meis2 is expressed at E14.5. However, Meis1 has been linked to the maintenance of murine epidermal hair stem cells (Okumura et al., 2014), which suggests that Meis1 expression may appear later during hair development, in a fashion similar to the incisor.
Besides preventing epidermal stem cell differentiation in the hair bulge, Meis1 regulates long-term haematopoietic stem cells (Unnisa et al., 2012; Okumura et al., 2014). Also, Meis1 expression has been linked to long cell cycles and reduced cell proliferation in olfactory epithelium progenitors and cardiomyocytes (Tucker et al., 2010; Mahmoud et al., 2013). In the adult mouse incisor, we have shown that Meis1 expression is restricted to the epithelial stem cell niche, and that it marks the Sox2+ cells. Previously, we had shown that Sox2 is important for cell differentiation and stem cell maintenance during tooth formation and adult incisor renewal (Sun et al., 2016; Sanz-Navarro et al., 2018). Thus, it is possible that Meis1 is involved in the regulation of the dental epithelial stem cells during postnatal stages.
It is possible that MEIS1 and SOX2 are modulating each other’s expression in the dental context, as Meis1 has been associated with the regulation of Sox2 in different systems. In human oesophageal squamous carcinoma, MEIS1 can suppress SOX2 expression, while in NT2/D1 cells (neuroepithelial precursors-like cell line), MEIS1 up-regulates SOX3, another member of the SoxB1 family with overlapping functions with Sox2 (Mojsin and Stevanovic, 2010; Rad et al., 2016; Adikusuma et al., 2017). Contrarily, in the olfactory epithelium, SOX2 downregulates MEIS1, while Meis1 overexpression does not affect Sox2 (Tucker et al., 2010). Moreover, MEIS1 binding sites have been described in Sox2 enhancer regions (Marcos et al., 2015). To better understand this relationship, loss-of-function approaches would be necessary. In this study we have found a largely normal morphology in Meis1-null teeth at E14.5. The lack of an abnormal phenotype at this stage correlates with the late appearance of Meis1 expression during tooth morphogenesis. However, the fact that Meis1 is specifically expressed by Sox2+ cells and the importance of MEIS1 in the regulation of other stem cell niches (Unnisa et al., 2012; Okumura et al., 2014; Miller et al., 2016; Wang et al., 2018) suggest a role for MEIS1 during later stages of incisor development or during adult renewal. The use of conditional mouse mutants during postnatal stages could lead to understand the role of MEIS1 in the incisor labial cervical loop.
To exert its function, MEIS1 binds the DNA as a dimer, like other TALE factors, and its preferred partner is PBX1 (Penkov et al., 2013; Longobardi et al., 2014). We have found ubiquitous Pbx1 expression in the head of embryonic mice and partially overlapping with Meis1 expression. Pbx1 gene expression is broader than the previously reported protein pattern (Schnabel et al., 2001). This indicates that these transcripts might be exposed to extensive post-transcriptional regulation or that low levels of protein expression could not be detected. Thus, it is possible that MEIS1 and PBX1 are expressed in the same cells of the tooth and that are interacting to regulate cell behavior. However, further research is necessary in order to conclude the exact role of Meis1 in the dental context, and its association with PBX1.
Limitations of the Study
The early death of the Meis1KO embryos is a limitation of this study as it prevents the analysis of ameloblastic differentiation and enamel formation. Conditional loss-of-functions mouse models with longer lifespans in which Meis1 would be specifically ablated in the dental epithelium would be a valuable tool to study the later stages of tooth morphogenesis. Alternatively, ex vivo culture of Meis1KO E14.5 teeth or ex vivo gene silencing strategies could be employed. Hence, further research is necessary to unravel the exact role of Meis1 in the maintenance of dental epithelial stem cells.
Conclusion
In this study, we have shown that Meis1 and Meis2 are expressed in different areas of the tooth, tongue, palate, hair follicle and submandibular salivary gland during morphogenesis. In the tooth, Meis1 expression gets restricted to the cervical loops as development progresses, whereas MEIS1 and SOX2 are coexpressed in the incisor labial cervical loop during adult incisor renewal. Given the data as a whole, MEIS1 is a putative marker of dental epithelial stem cells. Altogether, Meis1 is an interesting candidate for future studies in the fields of dental stem cells and tooth development.
Data Availability
The datasets generated for this study are available on request to the corresponding author.
Author Contributions
MS-N designed and performed all experiments, analyzed the data, and wrote the manuscript. ID, MT, TM, and FM provided reagents, edited the manuscript, and designed the experiments. DR designed the experiments, analyzed the data, and wrote the manuscript.
Funding
This study was funded by Minerva Foundation (Selma and Maja-Lisa Selander’s fund) and FINDOS to MS-N, the Academy of Finland to FM and University of Helsinki Research Foundation (Y1014SL009) to DR.
Conflict of Interest Statement
The authors declare that the research was conducted in the absence of any commercial or financial relationships that could be construed as a potential conflict of interest.
Acknowledgments
The authors thank Mona Christensen, Anne Kivimäki, and Rakibul Hasan for assistance and Dr. Ondrej Machon for discussions.
Supplementary Material
The Supplementary Material for this article can be found online at: https://www.frontiersin.org/articles/10.3389/fphys.2019.00249/full#supplementary-material
References
Adikusuma, F., Pederick, D., McAninch, D., Hughes, J., and Thomas, P. (2017). Functional equivalence of the sox2 and sox3 transcription factors in the developing mouse brain and testes. Genetics 206, 1495–1503. doi: 10.1534/genetics.117.202549
Antosova, B., Smolikova, J., Klimova, L., Lachova, J., Bendova, M., Kozmikova, I., et al. (2016). The gene regulatory network of lens induction is wired through meis-dependent shadow enhancers of pax6. PLoS Genet. 12:e1006441. doi: 10.1371/journal.pgen.1006441
Argiropoulos, B., Yung, E., and Humphries, R. K. (2007). Unraveling the crucial roles of meis1 in leukemogenesis and normal hematopoiesis. Genes Dev. 21, 2845–2849. doi: 10.1101/gad.1619407
Avilion, A. A., Nicolis, S. K., Pevny, L. H., Perez, L., Vivian, N., and Lovell-Badge, R. (2003). Multipotent cell lineages in early mouse development depend on SOX2 function. Genes Dev. 17, 126–140. doi: 10.1101/gad.224503
Azcoitia, V., Aracil, M., Martínez-A, C., and Torres, M. (2005). The homeodomain protein Meis1 is essential for definitive hematopoiesis and vascular patterning in the mouse embryo. Dev. Biol. 280, 307–320. doi: 10.1016/j.ydbio.2005.01.004
Capellini, T. D., Zappavigna, V., and Selleri, L. (2011). Pbx homeodomain proteins: TALEnted regulators of limb patterning and outgrowth. Dev. Dyn. 240, 1063–1086. doi: 10.1002/dvdy.22605
Geerts, D., Schilderink, N., Jorritsma, G., and Versteeg, R. (2003). The role of the MEIS homeobox genes in neuroblastoma. Cancer Lett. 197, 87–92. doi: 10.1016/S0304-3835(03)00087-9
González-Lázaro, M., Roselló-Díez, A., Delgado, I., Carramolino, L., Sanguino, M. A., Giovinazzo, G., et al. (2014). Two new targeted alleles for the comprehensive analysis of M eis1 functions in the mouse. Genesis 52, 967–975. doi: 10.1002/dvg.22833
Hisa, T., Spence, S. E., Rachel, R. A., Fujita, M., Nakamura, T., Ward, J. M., et al. (2004). Hematopoietic, angiogenic and eye defects in meis1 mutant animals. EMBO J. 23, 450–459. doi: 10.1038/sj.emboj.7600038
Hovorakova, M., Lochovska, K., Zahradnicek, O., Domonkosova Tibenska, K., Dornhoferova, M., Horakova-Smrckova, L., et al. (2016). One odontogenic cell-population contributes to the development of the mouse incisors and of the oral vestibule. PLoS One 11:e0162523. doi: 10.1371/journal.pone.0162523
Hu, J. K.-H., Mushegyan, V., and Klein, O. D. (2014). On the cutting edge of organ renewal: identification, regulation, and evolution of incisor stem cells. Genesis 52, 79–92. doi: 10.1002/dvg.22732
Jussila, M., and Thesleff, I. (2012). Signaling networks regulating tooth organogenesis and regeneration, and the specification of dental mesenchymal and epithelial cell lineages. Cold Spring Harb. Perspect. Biol. 4:a008425. doi: 10.1101/cshperspect.a008425
Juuri, E., Jussila, M., Seidel, K., Holmes, S., Wu, P., Richman, J., et al. (2013). Sox2 marks epithelial competence to generate teeth in mammals and reptiles. Development 140, 1424–1432. doi: 10.1242/dev.089599
Juuri, E., Saito, K., Ahtiainen, L., Seidel, K., Tummers, M., Hochedlinger, K., et al. (2012). Sox2+ stem cells contribute to all epithelial lineages of the tooth via Sfrp5+ progenitors. Dev. Cell 23, 317–328. doi: 10.1016/j.devcel.2012.05.012
Krivanek, J., Adameyko, I., and Fried, K. (2017). Heterogeneity and developmental connections between cell types inhabiting teeth. Front. Physiol. 8:376. doi: 10.3389/fphys.2017.00376
Longobardi, E., Penkov, D., Mateos, D., De Florian, G., Torres, M., and Blasi, F. (2014). Biochemistry of the tale transcription factors PREP, MEIS, and PBX in vertebrates. Dev. Dyn. 243, 59–75. doi: 10.1002/dvdy.24016
Machon, O., Masek, J., Machonova, O., Krauss, S., and Kozmik, Z. (2015). Meis2 is essential for cranial and cardiac neural crest development. BMC Dev. Biol. 15:40. doi: 10.1186/s12861-015-0093-6
Mahmoud, A. I., Kocabas, F., Muralidhar, S. A., Kimura, W., Koura, A. S., Thet, S., et al. (2013). Meis1 regulates postnatal cardiomyocyte cell cycle arrest. Nature 497, 249–253. doi: 10.1038/nature12054
Marcos, S., González-Lázaro, M., Beccari, L., Carramolino, L., Martin-Bermejo, M. J., Amarie, O., et al. (2015). Meis1 coordinates a network of genes implicated in eye development and microphthalmia. Development 142, 3009–3020. doi: 10.1242/dev.122176
Mercader, N., Leonardo, E., Azpiazu, N., Serrano, A., Morata, G., Martínez, C., et al. (1999). Conserved regulation of proximodistal limb axis development by Meis1/Hth. Nature 402, 425–429. doi: 10.1038/46580
Miller, M. E., Rosten, P., Lemieux, M. E., Lai, C., and Humphries, R. K. (2016). Meis1 is required for adult mouse erythropoiesis, megakaryopoiesis and hematopoietic stem cell expansion. PLoS One 11:e0151584. doi: 10.1371/journal.pone.0151584
Mojsin, M., and Stevanovic, M. (2010). PBX1 and MEIS1 up-regulate SOX3 gene expression by direct interaction with a consensus binding site within the basal promoter region. Biochem. J. 425, 107–116. doi: 10.1042/BJ20090694
Moskow, J. J., Bullrich, F., Huebner, K., Daar, I. O., and Buchberg, A. M. (1995). Meis1, a PBX1-related homeobox gene involved in myeloid leukemia in BXH-2 mice. Mol. Cell. Biol. 15, 5434–5443. doi: 10.1128/MCB.15.10.5434
Okumura, K., Saito, M., Isogai, E., Aoto, Y., Hachiya, T., Sakakibara, Y., et al. (2014). Meis1 regulates epidermal stem cells and is required for skin tumorigenesis. PLoS One 9:e102111. doi: 10.1371/journal.pone.0102111
Oulad-Abdelghani, M., Chazaud, C., Bouillet, P., Sapin, V., Chambon, P., and Dollé, P. (1997). Meis2, a novel mousePbx-related homeobox gene induced by retinoic acid during differentiation of P19 embryonal carcinoma cells. Dev. Dyn. 210, 173–183. doi: 10.1002/(SICI)1097-0177(199710)210:2<173::AID-AJA9>3.0.CO;2-D
Penkov, D., San Martín, D. M., Fernandez-Díaz, L. C., Rosselló, C. A., Torroja, C., Sánchez-Cabo, F., et al. (2013). Analysis of the DNA-binding profile and function of tale homeoproteins reveals their specialization and specific interactions with hox genes/proteins. Cell Rep. 3, 1321–1333. doi: 10.1016/J.CELREP.2013.03.029
Pevny, L. H., and Nicolis, S. K. (2010). Sox2 roles in neural stem cells. Int. J. Biochem. Cell Biol. 42, 421–424. doi: 10.1016/J.BIOCEL.2009.08.018
Rad, A., Farshchian, M., Forghanifard, M. M., Matin, M. M., Bahrami, A. R., Geerts, D., et al. (2016). Predicting the molecular role of MEIS1 in esophageal squamous cell carcinoma. Tumor Biol. 37, 1715–1725. doi: 10.1007/s13277-015-3780-9
Ramanathan, A., Srijaya, T. C., Sukumaran, P., Zain, R. B., and Abu Kasim, N. H. (2018). Homeobox genes and tooth development: understanding the biological pathways and applications in regenerative dental science. Arch. Oral Biol. 85, 23–39. doi: 10.1016/J.ARCHORALBIO.2017.09.033
Sanz-Navarro, M., Seidel, K., Sun, Z., Bertonnier-Brouty, L., Amendt, B. A., Klein, O. D., et al. (2018). Plasticity within the niche ensures the maintenance of a Sox2+ stem cell population in the mouse incisor. Development 145:dev155929. doi: 10.1242/dev.155929
Schnabel, C. A., Selleri, L., Jacobs, Y., Warnke, R., and Cleary, M. L. (2001). Expression of Pbx1b during mammalian organogenesis. Mech. Dev. 100, 131–135. doi: 10.1016/S0925-4773(00)00516-5
Sun, Z., Yu, W., Sanz Navarro, M., Sweat, M., Eliason, S., Sharp, T., et al. (2016). Sox2 and Lef-1 interact with Pitx2 to regulate incisor development and stem cell renewal. Development 143, 4115–4126. doi: 10.1242/dev.138883
Thesleff, I., and Tummers, M. (2008). Tooth Organogenesis and Regeneration. Cambridge MA: Harvard Stem Cell Institute.
Tucker, E. S., Lehtinen, M. K., Maynard, T., Zirlinger, M., Dulac, C., Rawson, N., et al. (2010). Proliferative and transcriptional identity of distinct classes of neural precursors in the mammalian olfactory epithelium. Development 137, 2471–2481. doi: 10.1242/dev.049718
Unnisa, Z., Clark, J. P., Roychoudhury, J., Thomas, E., Tessarollo, L., Copeland, N. G., et al. (2012). Meis1 preserves hematopoietic stem cells in mice by limiting oxidative stress. Blood 120, 4973–4981. doi: 10.1182/blood-2012-06-435800
Wang, F., Flanagan, J., Su, N., Wang, L.-C., Bui, S., Nielson, A., et al. (2012). RNAscope: a novel in situ RNA analysis platform for formalin-fixed, paraffin-embedded tissues. J. Mol. Diagn. 14, 22–29. doi: 10.1016/j.jmoldx.2011.08.002
Keywords: mouse incisor, tooth development, palate, tongue, ectodermal organ, stem cells, SOX2, MEIS1
Citation: Sanz-Navarro M, Delgado I, Torres M, Mustonen T, Michon F and Rice DP (2019) Dental Epithelial Stem Cells Express the Developmental Regulator Meis1. Front. Physiol. 10:249. doi: 10.3389/fphys.2019.00249
Received: 22 October 2018; Accepted: 25 February 2019;
Published: 12 March 2019.
Edited by:
Giovanna Orsini, Polytechnical University of Marche, ItalyReviewed by:
Zhi Chen, Wuhan University, ChinaMarianna Bei, Harvard Medical School, United States
Michel Goldberg, Institut National de la Santé et de la Recherche Médicale (INSERM), France
Ralf Kist, Newcastle University, United Kingdom
Copyright © 2019 Sanz-Navarro, Delgado, Torres, Mustonen, Michon and Rice. This is an open-access article distributed under the terms of the Creative Commons Attribution License (CC BY). The use, distribution or reproduction in other forums is permitted, provided the original author(s) and the copyright owner(s) are credited and that the original publication in this journal is cited, in accordance with accepted academic practice. No use, distribution or reproduction is permitted which does not comply with these terms.
*Correspondence: David P. Rice, david.rice@helsinki.fi