- Laboratory of Cardiac Cellular Physiology, Department of Biotechnology and Bioscience, University of Milano-Bicocca, Milan, Italy
Introduction: Increases in action potential duration (APD), genetic or acquired, and arrhythmias are often associated; nonetheless, the relationship between the two phenomena is inconstant, suggesting coexisting factors. β-adrenergic activation increases sarcoplasmic reticulum (SR) Ca2+-content; angiotensin II (ATII) may increase cytosolic Ca2+ and ROS production, all actions stimulating RyRs opening. Here we test how APD interacts with β-adrenergic and AT-receptor stimulation in facilitating spontaneous Ca2+ release events (SCR).
Methods: Under “action potential (AP) clamp”, guinea-pig cardiomyocytes (CMs) were driven with long (200 ms), normal (150 ms), and short (100 ms) AP waveforms at a CL of 500 ms; in a subset of CMs, all the 3 waveforms could be tested within the same cell. SCR were detected as inward current transients (ITI) following repolarization; ITI incidence and repetition within the same cycle were measured under increasing isoprenaline concentration ([ISO]) alone, or plus 100 nM ATII (30 min incubation+superfusion).
Results: ITI incidence and repetition increased with [ISO]; at longer APs the [ISO]-response curve was shifted upward and ITI coupling interval was reduced. ATII increased ITI incidence more at low [ISO] and under normal (as compared to long) APs. Efficacy of AP shortening in suppressing ITI decreased in ATII-treated myocytes and at higher [ISO].
Conclusions: AP prolongation sensitized the SR to the destabilizing actions of ISO and ATII. Summation of ISO, ATII and AP duration effects had a “saturating” effect on SCR incidence, thus suggesting convergence on a common factor (RyRs stability) “reset” by the occurrence of spontaneous Ca2+ release events.
Introduction
Prolonged ventricular repolarization, whether caused by myocardial remodeling (Nattel et al., 2007), genetic defects (Schwartz et al., 2001), or drugs (Yang et al., 2001), may reduce myocardial electrical stability. Prolonged action potential duration (APD) may be a reporter of reduced “repolarization reserve,” but it can also be causally linked to arrhythmogenesis through several mechanisms. Along with purely electrophysiological consequences (e.g., increased repolarization heterogeneity) (Winter and Shattock, 2016), APD prolongation affects the sarcolemmal Ca2+ influx/efflux balance (Bers, 2001), thus it represents a stress-condition for intracellular Ca2+ homeostasis requiring robust compensatory mechanisms. This might be critical under conditions, such as heart failure, in which intracellular Ca2+ homeostasis is primarily impaired; indeed, “triggered activity” is common in the failing myocardium (Pogwizd, 1995).
On the other hand, prolonged repolarization per se may be insufficient to induce arrhythmias, which might require the concomitance of multiple factors. This may be true also for primarily electrical disorders, such as genetic (Napolitano et al., 2015) and drug-induced (Redfern et al., 2003) repolarization abnormalities. After the early identification of sympathetic activation as a powerful triggering mechanism (Malliani et al., 1980), relatively little attention has been devoted to other biological variables that might theoretically converge with prolonged repolarization in reducing electrical stability. Their identification might pave the way to risk stratification and development of relatively simple preventive measures.
Myocardial and systemic production of angiotensin II (ATII) is increased in response to stress. Beside its well-known role in long-term structural remodeling of the failing heart, ATII has a couple of actions at the cellular level that might acutely compromise the compensations required to cope with prolonged repolarization.
AT-1 receptors (AT1R) stimulation by ATII induces Ca2+ release from the sarcoplasmic reticulum (SR) through inositol 1,4,5-trisphospate-receptor channels (IP3R) and IP3R are functionally coupled to RYR2 in atrial myocytes (Kockskamper et al., 2008; Wullschleger et al., 2017). Although, in ventricular myocytes IP3R are less expressed (Garcia and Boehning, 2017), IP3R activation (by endothelin 1) has been reported to enhance CICR in rabbit ventricular myocytes (Domeier et al., 2008). Secondly, AT1R stimulation activates production of radical O2 species (ROS) by sarcolemmal NADPH-oxydases (Kawai et al., 2017); peroxydation destabilizes RyR2 closed state and is well-known to facilitate spontaneous Ca2+ release events (SCR) and related arrhythmias (Prosser et al., 2013).
While an antiarrhythmic effect of ATII antagonism has been reported (Garg et al., 2006), acute proarrhythmia does not stand out as a direct consequence of increased ATII signaling, but what happens if the functional reserve provided by Ca2+-homeostatic mechanisms is concomitantly decreased by prolonged repolarization?
Based on the above information, we hypothesize that stimulation of ATII receptors, may facilitate the occurrence of SCR events induced by APD prolongation and β-adrenergic stimulation. The aim of this study was to test such hypothesis.
The practical interest in this question also lies in the availability of widely used drugs limiting ATII cellular effects (AT1R antagonists), which might then be considered as complements in the prevention of ventricular arrhythmias under conditions of prolonged repolarization.
Materials and Methods
The investigation conforms to the Guide of the Care and Use of Laboratory Animals published by the US National Institute of Health (NIH publication No, 85-23). This study was reviewed and approved by the Animal Care Committee endorsed by University Milano-Bicocca.
Cell Isolation
Dunkin-Hartley guinea pigs left ventricular cardiomyocytes were isolated by using a retrograde coronary perfusion method previously published (Zaza et al., 1998), with minor modifications. Rod-shaped, Ca2+-tolerant myocytes were used within 12 h from dissociation. No measure was taken to dissociate myocytes selectively from either the left or the right ventricle.
Action Potential Clamp Recordings
Measurements were performed during superfusion with Tyrode's solution at 36.5°C. Pre-recorded guinea-pig action potential (AP) waveforms were applied in V-clamp mode, as previously described (Sala et al., 2017), at a cycle length of 2 Hz. Three AP waveforms (obtained from previous I-clamp recordings under appropriate conditions) with different APD90 (APD measured at 90% of repolarization) values were used: 200 ms (Long AP), 150 ms (Normal AP), and 100 ms (Short AP). APD90 in the Long AP is 40% longer than in Normal AP, a change proportionally compatible with repolarization abnormalities seen in the clinical setting (Schwartz et al., 1995) (it would simulate a QTc change from e.g., 370–518 ms). According to the experimental protocol, the different AP waveforms were applied to different myocytes (group comparison) or within the same myocyte (internal control). Because of their design, internal control experiments involved application of both AP waveforms in a fixed sequence, thus requiring to exclude the dependence of ITI properties on the sequence itself. This was ruled out in preliminary experiments by applying Long-Short-Long AP sequences within the same myocyte. Characteristics of AP waveforms are summarized in Table S1.
Total membrane current (Im) during the AP-clamp cycle (Figure 1A and Figure S1) was recorded. Because the AP waveforms were not recorded within the same myocyte, Im during the AP was not null as expected under proper “self” AP-clamp conditions (Zaza et al., 1997); nonetheless, changes in the balance between Im inward and outward components could still be used as a gross estimate of interventions effect on systolic currents.
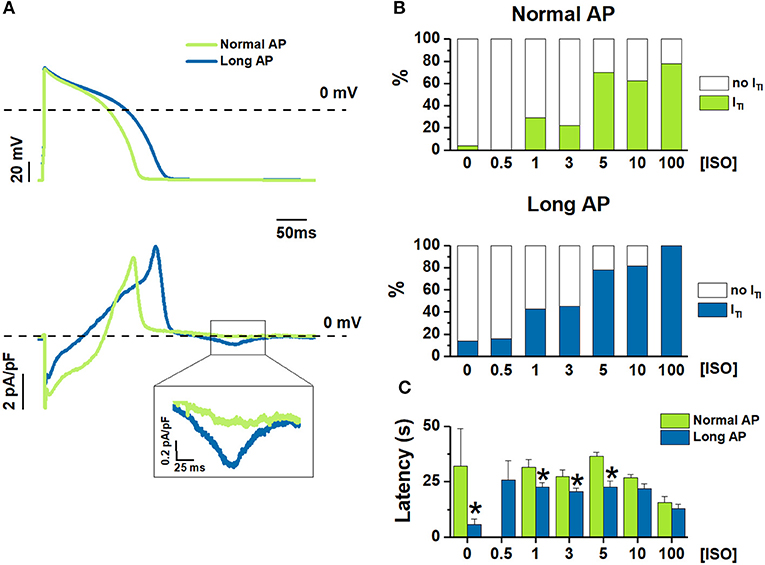
Figure 1. [ISO]-dependency effect on ITI incidence under Normal AP and Long AP. (A) Examples of Long AP and Normal AP waveforms used as a command in AP clamp (top) and the corresponding Im (bottom) during 1nM ISO. (B) ITI incidence, and (C) latency before ITI onset from starting ISO challenge at different ISO concentrations (nM). N > 10 for all data points. *P < 0.05 vs. Normal AP.
SCR events were detected as the occurrence of diastolic inward current transients (ITI), known to result from activation of Na+/Ca2+ exchange by intracellular Ca2+ transients. This method was preferred to direct recording of intracellular Ca2+ because free from the contaminating effect of Ca2+ buffering by fluorescent Ca2+ probes; furthermore, Im is sensitive to sub-sarcolemmal Ca2+ changes, which are relevant to membrane electrophysiology but poorly detected by epifluorescence measurements. Based on the baseline variance of diastolic current, ITI events were defined ITI exceeding a threshold of 15 pA; their incidence was defined as the percentage of myocytes in which at least 1 event was observed. Peak ITI amplitude (pA/pF) and ITI integral (QTI, in μC), which reports the charge moved by Na+/Ca2+ exchange, was used to quantify the magnitude of SCR events. Other parameters considered were ITI coupling interval after the preceding AP (CI in ms) and ITI latency (in sec) after the beginning of ISO infusion.
β-adrenergic stimulation was achieved by exposure to increasing isoprenaline concentrations [ISO]; ATII-receptors were stimulated by 30 min preincubation in 100 nM ATII, which was also added to the superfusate.
Whether the effect of ATII was due to modulation of IP3R was tested by (5 min) exposure to 2 μM 2-aminoethyl diphenylborinate (2APB), an antagonist of IP3R. Nifedipine 5 μM (NIFE) was used to compare 2APB effect on Im to that of L type Ca2+ current (ICaL) blockade.
Experimental Solutions
Tyrode's solution contained (in mM): 154 NaCl, 4 KCl, 2 CaCl2, 1 MgCl2, 5 HEPES-NaOH, 5.5 D-glucose, adjusted to pH 7.35 with NaOH. Myocytes were patch-clamped with borosilicate glass pipettes containing (mM): K+-aspartate 110, KCl 23, MgCl2 3, HEPES KOH 5, EGTA KOH 1, GTP Na+-salt 0.4, ATP Na+-salt 5, creatine phosphate Na+-salt 5, CaCl2 0.4 (calculated free-Ca2+ = 10−7 M), adjusted to pH 7.3.
ISO and ATII were dissolved in water, NIFE in ethanol, and 2APB in DMSO. When used, compound solvents (ethanol or DMSO) were added to all solutions at the same final concentration, which never exceed 0.1%. All chemicals were purchased from Sigma.
Statistical Analysis
Student's paired or unpaired t-test was applied to compare means of continuous variables (latency, amplitudes etc). Difference between categorical variables (expressed as incidence %) was tested by chi-square (group comparison) or McNemar analysis (internal control) applied to absolute numbers; GLM regression for binomial data (R statistical package) was used to compare vectors of categorical variables (incidence in ISO dose-response curves). Statistical significance was defined as P < 0.05. Sample size (n, number of cells) is specified for each experimental condition in the respective figure legend.
Categorical variables are expressed in percentages while the continuous variables are expressed as average ± standard error of the mean.
Results
Effect of APD Prolongation on ISO-Induced ITI Events
The experimental protocol was optimized to test the effect of multiple [ISO] in each myocyte; because of limitations inherent to preparation stability, the effect of changing APD on the [ISO] vector had to be tested by comparing myocyte groups. Thus, the incidence of ITI events was compared between myocytes clamped with either the Normal AP or the Long AP (group comparison) at baseline and during exposure to increasing ISO concentrations ([ISO]) (Figure 1).
ITI incidence dose-dependently increased with [ISO] under both AP waveforms (Figure 1B); the Long APD moved the ISO concentration-response curve to significantly higher ITI incidences (p < 0.05 at binomial GLM regression) and reduced the threshold concentration for ISO effect (lower vs. upper panel in Figure 1B). As compared to Normal AP, Long AP shortened the time of ITI appearance after the beginning of ISO (or control) solution challenge; however, this effect became smaller as [ISO] was increased (Figure 1C).
Effect of Adding ATII
The above experiments were repeated in cells treated with 100 nM ATII (ATII) and compared with untreated cells (no ATII) (Figure 2).
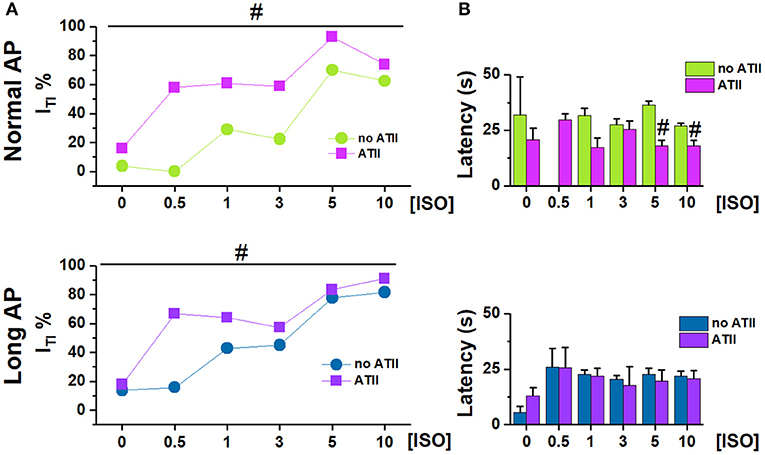
Figure 2. Effect of adding ATII on ITI incidence under Normal AP and Long AP. (A) ITI incidence and (B) latency at different ISO concentrations (nM) in untreated (noATII) and ATII- treated (ATII) CMs. N > 20 for all groups. #p < 0.05 vs. ISO group.
ATII alone [[ISO] = 0] had a very small effect on ITI incidence under Normal AP (p < 0.05) and failed to change ITI significantly under Long AP (Figure 2A). Within each AP waveform, ATII moved the ISO concentration-response curve to significantly higher ITI incidences. ATII effect was present with both AP waveforms, (p < 0.05 at binomial GLM regression), but it was smaller and limited to low [ISO] during the Long AP (Figure 2A). ATII tended to shorten the time of ITI appearance (latency) after the beginning of ISO challenge, but did so only under the Normal AP (Figure 2B).
Effect of APD Changes on ITI Occurrence and Properties
This set of experiments was designed to test whether APD shortening would effectively modify ITI events induced by receptor stimulation. To this end, each myocyte in which ITI events occurred during the Long AP (incidence = 100% by default) was subsequently clamped with the Normal AP (internal control). This was repeated in myocyte groups, each exposed to a different [ISO] alone, or in the presence of ATII (Figures 3, 4). ITI incidence and properties were measured.
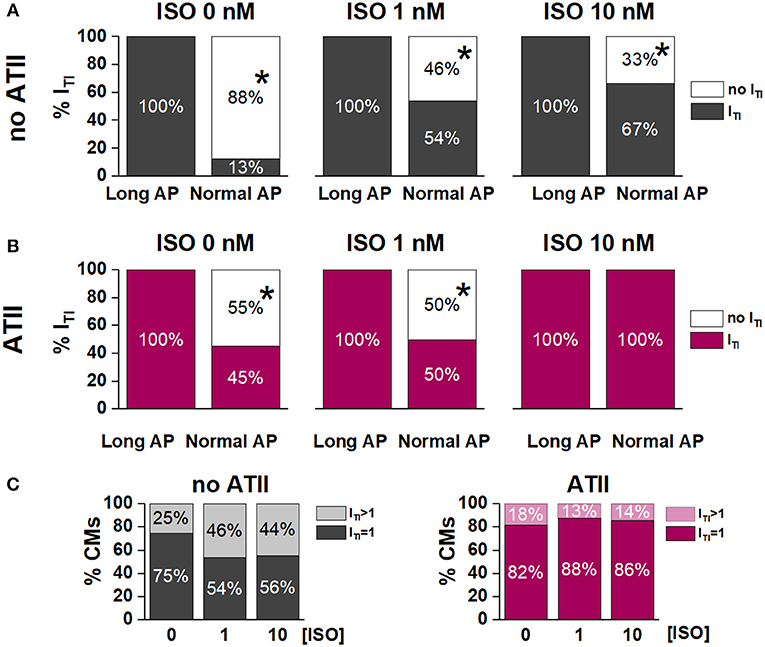
Figure 3. Effect of APD shortening on ITI incidence within the same cardiomyocyte in the absence (no ATII) (A) or presence of ATII (ATII) (B). (C) Percent of cardiomyocytes with 1 ITI event or >1 ITI events in the absence (no ATII) or presence (ATII) of ATII (Long AP; 0, 1,10 nM ISO). CMs: cardiomyocytes. N > 8 for all groups. *p < 0.05 vs. Long AP.
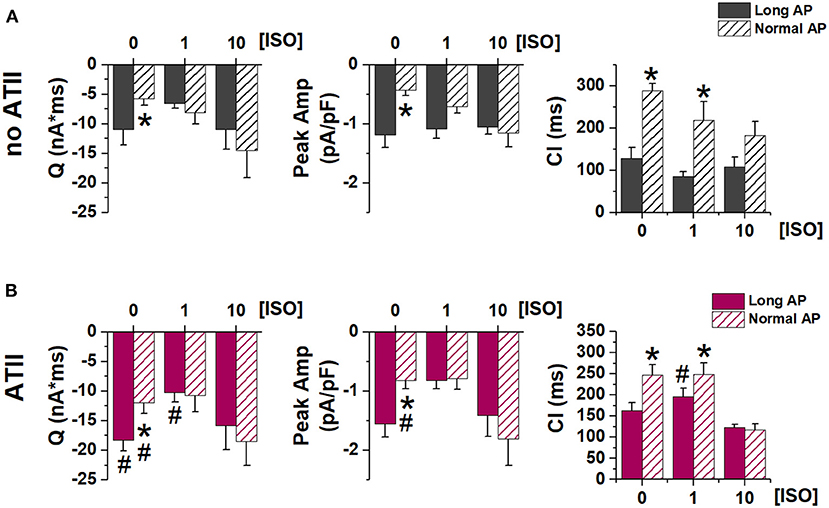
Figure 4. Effect of APD shortening on ITI properties. Statistics (mean ± S.E.) of ITI charge (Q), peak ITI amplitude (Peak Amp) and coupling interval (CI) (A) in the absence of ATII (no ATII); (B) in the presence of ATII (ATII). N > 8 for all groups. *p < 0.05 vs. Long AP; #p < 0.05 vs. no ATII CMs.
Without ATII, (Figure 3A), switching from Long AP to Normal AP caused ITI to cease within several beats in 88% of the cells in basal conditions, in 46% under 1 nM ISO and in 33% under 10 nM ISO (p < 0.05 for ΔAPD90 differences along the [ISO] vector). In ATII-treated myocytes, APD shortening terminated ITI in 55% of cells under basal conditions and termination rate decreased with increasing [ISO] (Figure 3B). The effect of APD shortening on ITI incidence was not significantly modified by ATII.
In the absence of ATII, occurrence of two ITI events within the same cycle was not significantly affected by ISO. Surprisingly, ATII did not increase the occurrence of double ITI events and, if anything, tended to reduce it (Figure 3C).
Similarly, ITI properties were affected by APD shortening (in the direction of smaller SCR with greater latency) only in the absence of β-adrenergic stimulation. ATII increased ITI magnitude (QTI and peak amplitude), but the effect of APD shortening was preserved (Figure 4).
To test whether APD affected ITI occurrence only if increased above its normal value, in a subset of myocytes the Short AP waveform was also tested (again by internal control) (Figure 5). ITI incidence and properties were incrementally modified by AP waveforms of shorter duration, suggesting that SR stability depends on APD in a continuous fashion. High [ISO] minimized the effect of APD shortening.
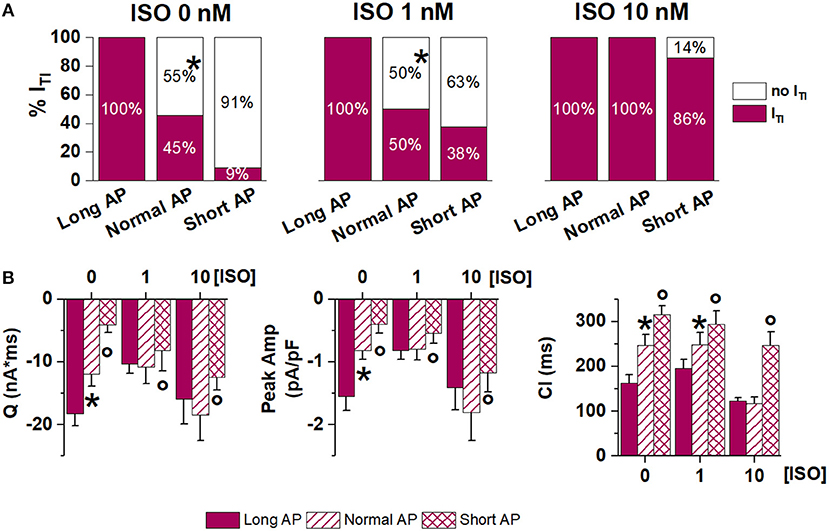
Figure 5. Effect of APD shortening within the same ATII-treated cardiomyocyte (Long AP, Normal AP and Short AP waveforms). (A) ITI incidence in basal condition (ISO 0) and in 1 nM and 10 nM ISO. (B) statistics (mean ± S.E.) of ITI charge (Q), peak ITI amplitude (Peak Amp) and coupling interval (CI). N > 8 for all groups *p < 0.05 vs. Long AP; °p < 0.05 vs. Normal AP.
Overall, internal control experiments confirmed that APD shortening may counter the arrhythmogenic effect of receptor stimulation, but its impact was reduced at high levels of neurohumoral stimulation.
IP3R Contribution to ATII Effect
The contribution of IP3 signaling to ITI facilitation by ATII was investigated by blocking IP3R (by 2APB) (Figure 6). To this end ITI incidence was measured, during Normal and Long APs, in the presence of 1 nM or 10 nM ISO + ATII only and after adding 2APB to the superfusate.
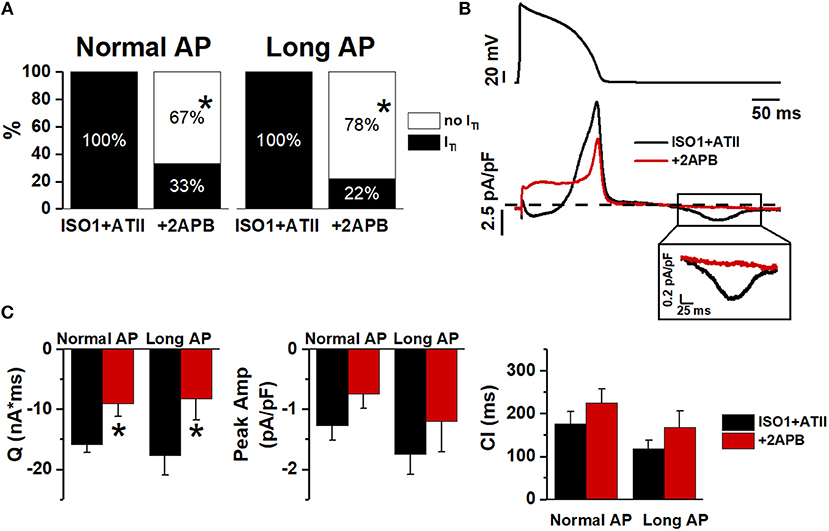
Figure 6. Effect of 2APB on ITI induced by ISO+ATII. (A) ITI incidence in ISO1+ATII alone and with 2APB. (B) Examples of Normal AP (top) and Im corresponding (bottom). (C) statistic (mean ± S.E.) of ITI charge (Q); peak ITI amplitude (Peak Amp) and coupling interval (CI). N = 9 for both groups. *p > 0.05 vs. ISO1+ATII.
With 1 nM ISO, 2APB similarly reduced ITI incidence during Normal APD and Long APD (Figure 6A); the same was true for ITI charge (Q) (Figure 6C). When [ISO] was increased to 10 nM 2APB still reduced ITI incidence during Normal AP (by 60%) but had a much smaller effect during the Long AP (Figure S2).
This would suggest that IP3R stimulation significantly contributed to ATII-induced increment in SCR events; however, a substantial outward shift of Im during the AP (Figure 6B) opens the possibility of decreased Ca2+ influx as the mechanism for ITI suppression. To test the hypothesis of direct ICaL blockade by 2APB, we compared the kinetics of its action on Im during the AP plateau to that of a saturating concentration of NIFE (Figure S3). The much slower kinetics of 2APB argues against direct ICaL blockade by 2APB.
Discussion
The main findings of the present study were: ISO concentration-dependently induced SCR events and APD prolongation enhanced ISO effect; ATII increased SCR incidence preferentially when it was still far from 100%, i.e., with normal APD and in the lower range of [ISO].
While APD prolongation typically facilitates EADs through a mechanism at least partially independent of SCR (January and Riddle, 1989; Song et al., 2015), its association with SCR is less straightforward. An increase in intracellular Ca2+ content (both on the cytosolic and luminal sides of SR membrane) facilitates SCR (Egdell et al., 2000; Venetucci et al., 2008). Because of net charge transport by the Na+/Ca2+ exchanger, Ca2+ extrusion from the cell is favored at diastolic potentials; thus, assuming a constant cycle length (CL), APD prolongation can increase intracellular Ca2+ content simply by decreasing the proportion of the cycle occupied by diastole (Bers, 2001). Accordingly, modeling studies indicate that the amplitude and prematurity of DADs is expectedly related to APD because of APD-dependency of SR Ca2+ load (Song et al., 2015). Nonetheless, blockade of a repolarizing current (IKs) in canine myocytes prolonged ventricular APD (by ~15%) but failed to induce DADs unless associated with β-adrenergic stimulation (Burashnikov and Antzelevitch, 2000). In the present study, APD prolongation (by 40%) alone slightly increased ITI incidence also in the absence of ISO; this effect was smaller than that previously reported in rabbit myocytes using more pronounced APD prolongation (Wu et al., 1999). APD prolongation had a much larger effect in the presence of very low (nanomolar) ISO concentrations (Figure 1). This suggests that APD prolongation by amounts more relevant to the clinical setting may be relatively inconsequential on its own but becomes a powerful co-factor in the induction of SCR by adrenergic stimulation.
In the present experiments, APD was changed at a constant cycle length, thus reciprocally affecting diastolic interval. This implies that membrane “duty cycle” (the ratio between systolic and diastolic times), rather than APD itself, was considered. This approach was motivated by the consideration that repolarization abnormalities are defined as changes of the rate-corrected QT interval, which implies that they are not accompanied by a commensurate change in cycle length. Furthermore, from a mechanistic standpoint, the sarcolemmal Ca2+ flux balance depends on membrane duty cycle, rather than on APD alone (Bers, 2001). Nonetheless, it is fair to stress that the present data may not describe the effect of changes in APD alone (i.e., with constant diastolic interval).
In the presence of normal APD, ATII significantly facilitated ITI induction at all levels of β-adrenergic stimulation (Figure 2). ATII has been reported to exert “enhanced antagonism” of ICaL activation by β1-AR, reflecting inhibition of pre-activated adenylate cyclase (Ai et al., 1998). Thus, ITI facilitation by ATII in the context of β1-AR activation may look contradictory. However, it should be noted that, in the present experiments, ITI facilitation by ATII prevailed at low ISO concentrations, which failed to induce ITI events on their own (Figure 2). Overall, this may suggest that ATII facilitated ITI events by mechanisms other than those triggered by β1-AR activation, but converging with them to impair SR stability.
ATII activates membrane phospholipase C (PLC) resulting in production of IP3 and diacylglycerol. SR Ca2+ release through IP3R channels is central in smooth muscle physiology and significantly contributes to intracellular Ca2+ dynamics of atrial myocytes (Li et al., 2005; Wullschleger et al., 2017). Expression of IP3R in ventricular myocytes is lower, largely perinuclear and likely devoted to excitation-transcription coupling (Lipp et al., 2000; Wu et al., 2006; Domeier et al., 2008); therefore, their role in intracellular Ca2+ dynamics is debated. Nevertheless, IP3-dependent enhancement of excitation-contraction coupling has been observed in rabbit ventricular myocytes (Domeier et al., 2008). Functional crosstalk between neighboring IP3R and RyR2 channels, caused by local Ca2+ diffusion and amounting to direct activation of Ca2+ release units, has been demonstrated in atrial myocytes (Wullschleger et al., 2017). In ventricular myocytes (including human ones), enhanced IP3R-mediated SR Ca2+ leak has been reported not to affect RyR2 function directly, but rather secondary to APD prolongation by the increment in Na+/Ca2+ exchanger current (INCX) (Signore et al., 2013). Consistent with this interpretation, IP3R overexpression in a transgenic mouse model substantially enhanced SR Ca2+ leak without affecting the incidence of organized SCR events (Ca2+ sparks or puffs) (Blanch and Egger, 2018).
IP3R activation likely contributed to SCR facilitation by ATII in the present experiments, as suggested by the effect of 2APB (Figure 6). 2APB also shifted the current balance during the AP in the outward direction, a change compatible with an increased contribution of INCX during IP3R activation (Signore et al., 2013). Direct ICaL blockade by 2APB is unlikely because of the slow kinetics of 2APB effect (Figure 6) and of failure 2APB alone to affect Ca2+ dynamics in ventricular myocytes (Peppiatt et al., 2003). The present results suggest that IP3R activation, possibly through an increment of cytosolic Ca2+, may indeed facilitate Ca2+ waves under the conditions generated by APD prolongation and β-adrenergic stimulation.
Albeit not investigated in the present work, activation of ROS production by ATII is a further mechanism potentially contributing to RyR2 destabilization (Prosser et al., 2013).
Seen in the context of previous findings, the present results suggest that, albeit potentially inconsequential on its own, ATII may substantially facilitate SCR induction by β-adrenergic stimulation in ventricular myocytes.
Apparently in contrast with the hypothesis of a facilitatory role of APD prolongation, when APD was prolonged, ATII effect was smaller and limited to very low ISO concentrations (Figure 2). However, this can be viewed as “saturation” of the mechanisms responsible for SCR by APD prolongation (i.e., intracellular Ca2+ load) and strong β-adrenergic stimulation. Notably, even at saturation, ITI incidence did not achieve 100% and events per cycle were limited to one or two. Such pattern is expected from convergence of the three co-factors (APD, ISO and ATII) on a common mechanism (RyR2 facilitation) with a “ceiling” effect. The ceiling can be conceivably caused by the SCR itself, which resets SR Ca2+ content (Venetucci et al., 2008). Considering that ATII facilitated SCR, we expected it to increase the occurrence of double ITI events within a cycle. The finding that the opposite was true can be tentatively interpreted by considering that ATII increased the magnitude of the first SCR event (Q in Figure 4); by causing larger SR Ca2+ depletion, this might reduce the probability of a second SCR event.
The relevance of APD on ITI induction is highlighted by the ability of APD shortening to abrogate ITI once it was induced by either ISO alone or ISO plus ATII (Figure 5). Even when ITI persisted after AP normalization, its charge and amplitude were reduced, indicating that APD may affect the amount of Ca2+ released by the SCR and, with it, the likelihood of triggering a propagated beat. In keeping with the convergence of factors, the ability of APD normalization to affect ITI occurrence and properties was reduced at high ISO concentrations and, further, in the presence of ATII.
Conclusion
From the data obtained it is possible to conclude that ATII may act as a co-factor to facilitate the occurrence of SCR in the presence of AP prolongation and β-adrenergic stimulation.
Limitations
Among small animals, guinea-pigs have an action potential contour which is closest to the human one; this justifies their adoption as experimental model. However, as compared to canine (and, possibly, human) myocytes, guinea-pig ones do not express ITO (Nakajima et al., 2002) and have a prominent sustained ICaL, which likely supports a more positive action potential plateau (Sala et al., 2017). This might exaggerate the impact of changes in APD in facilitating SCR events.
While ITI (measured under V-clamp) is a suitable reporter of SCR, the direct cause of arrhythmogenesis by SCR are DADs. Albeit ITI is the cause of DAD, their features do not necessarily coincide because V-clamp prevents the positive feed-back loop between depolarization and further current activation; moreover, the amplitude of DADs caused by a given ITI amplitude depends on membrane diastolic resistance and, thus, on the currents determining it (mostly IK1). While absence of positive feed-back would lead to underestimate DADs amplitude, the effect of the interventions tested on diastolic membrane resistance is unknown.
Pratical Implications
ATII signaling is part of an homeostatic control system central to physiological adaptations; furthermore, ATII is also synthetized locally (cardiomyocytes, fibroblasts, vascular cells) in response to stress (Varagic and Frohlich, 2002); its production and its receptors (AT1R) are upregulated in cardiac disease (Szczepanska-Sadowska et al., 2018). Therefore, the extent of ATII signaling may well vary among subjects and, within a subject, in response to physiological and pathological states. The present results in cardiomyocytes suggest that ATII signaling might act as a variable co-factor in determining the impact of repolarization abnormalities on SR stability and the resulting arrhythmias. Thus, ATII antagonism might have an antiarrhythmic significance beyond prevention of myocardial remodeling, potentially extended to genetic and drug-induced conditions of prolonged repolarization. Considering that myocardial ATII production may be independent of the angiotensin converting enzyme (ACE) (Varagic and Frohlich, 2002), direct antagonism of AT1 receptors should be considered as the logical intervention.
Author Contributions
CR planned, performed, analyzed the majority of experiments and wrote the first manuscript draft; BB and JB contributed to part of the experiments and to data analysis. AZ supervised the study and contributed to the manuscript final version.
Funding
This work was funded by Fondo di Ateneo per la Ricerca (FAR 2018) to AZ.
Conflict of Interest Statement
The authors declare that the research was conducted in the absence of any commercial or financial relationships that could be construed as a potential conflict of interest.
Supplementary Material
The Supplementary Material for this article can be found online at: https://www.frontiersin.org/articles/10.3389/fphys.2018.01893/full#supplementary-material
References
Ai, T., Horie, M., Obayashi, K., and Sasayama, S. (1998). Accentuated antagonism by angiotensin II on guinea-pig cardiac L-type Ca-currents enhanced by β-adrenergic stimulation. Pflügers Archiv. 436, 168–174.
Bers, D. M. (2001). Excitation-Contraction Coupling and Cardiac Contractile Force. Boston, MA: Kluwer Academic Publishers.
Blanch, I. Salvador. J., and Egger, M. (2018). Obstruction of ventricular Ca2+ -dependent arrhythmogenicity by inositol 1,4,5-trisphosphate-triggered sarcoplasmic reticulum Ca2+ release. J. Physiol. 596, 4323–4340. doi: 10.1113/JP276319
Burashnikov, A., and Antzelevitch, C. (2000). Block of I(Ks) does not induce early afterdepolarization activity but promotes beta-adrenergic agonist-induced delayed afterdepolarization activity. J. Cardiovasc. Electrophysiol. 11, 458–465. doi: 10.1111/j.1540-8167.2000.tb00342.x
Domeier, T. L., Zima, A. V., Maxwell, J. T., Huke, S., Mignery, G. A., and Blatter, L. A. (2008). IP3 receptor-dependent Ca2+ release modulates excitation-contraction coupling in rabbit ventricular myocytes. Am. J. Physiol. Heart Circ. Physiol. 294, H596–H604. doi: 10.1152/ajpheart.01155.2007
Egdell, R. M., De Souza, A. I., and MacLeod, K. T. (2000). Relative importance of SR load and cytoplasmic calcium concentration in the genesis of aftercontractions in cardiac myocytes. Cardiovasc. Res. 47, 769–777. doi: 10.1016/s0008-6363(00)00147-4
Garcia, M. I., and Boehning, D. (2017). Cardiac inositol 1, 4, 5-trisphosphate receptors. Biochim. Biophys. Acta (BBA)-Mol. Cell Res. 1864, 907–914. doi: 10.1016/j.bbamcr.2016.11.017
Garg, S., Narula, J., Marelli, C., and Cesario, D. (2006). Role of angiotensin receptor blockers in the prevention and treatment of arrhythmias. Am. J. Cardiol. 97, 921–925. doi: 10.1016/j.amjcard.2005.10.028
January, C. T., and Riddle, J. M. (1989). Early afterdepolarizations: mechanism of induction and block. A role for L-type Ca2+ current. Circ. Res. 64, 977–990.
Kawai, T., Forrester, S. J., O'Brien, S., Baggett, A., Rizzo, V., and Eguchi, S. (2017). AT1 receptor signaling pathways in the cardiovascular system. Pharmacol. Res. 125 (Pt A), 4–13. doi: 10.1016/j.phrs.2017.05.008
Kockskämper, J., Zima, A. V., Roderick, H. L., Pieske, B., Blatter, L. A., and Bootman, M. D. (2008). Emerging roles of inositol 1,4,5-trisphosphate signaling in cardiac myocytes. J. Mol. Cell Cardiol. 45, 128–147. doi: 10.1016/j.yjmcc.2008.05.014
Li, X., Zima, A. V., Sheikh, F., Blatter, L. A., and Chen, J. (2005). Endothelin-1-induced arrhythmogenic Ca2+ signaling is abolished in atrial myocytes of inositol-1,4,5-trisphosphate(IP3)-receptor type 2-deficient mice. Circ. Res. 96, 1274–1281. doi: 10.1161/01.RES.0000172556.05576.4c
Lipp, P., Laine, M., Tovey, S. C., Burrell, K. M., Berridge, M. J., Li, W., et al. (2000). Functional InsP3 receptors that may modulate excitation-contraction coupling in the heart. Curr. Biol. 10, 939–942. doi: 10.1016/s0960-9822(00)00624-2
Malliani, A., Schwartz, P. J., and Zanchetti, A. (1980). Neural mechanisms in life-threatening arrhythmias. Am. Heart J. 100, 705–715.
Nakajima, I., Watanabe, H., Iino, K., Saito, T., and Miura, M. (2002). Ca2+ overload evokes a transient outward current in guinea-pig ventricular myocytes. Circ. J. 66, 87–92. doi: 10.1253/circj.66.87
Napolitano, C., Novelli, V., Francis, M. D., and Priori, S. G. (2015). Genetic modulators of the phenotype in the long QT syndrome: state of the art and clinical impact. Curr. Opin. Genet. Dev. 33, 17–24. doi: 10.1016/j.gde.2015.06.009
Nattel, S., Maguy, A., Le Bouter, S., and Yeh, Y. H. (2007). Arrhythmogenic ion-channel remodeling in the heart: heart failure, myocardial infarction, and atrial fibrillation. Physiol. Rev. 87, 425–456. doi: 10.1152/physrev.00014.2006
Peppiatt, C. M., Collins, T. J., Mackenzie, L., Conway, S. J., Holmes, A. B., Bootman, M. D., et al. (2003). 2-Aminoethoxydiphenyl borate (2-APB) antagonises inositol 1, 4, 5-trisphosphate-induced calcium release, inhibits calcium pumps and has a use-dependent and slowly reversible action on store-operated calcium entry channels. Cell Calcium 34, 97–108. doi: 10.1016/s0143-4160(03)00026-5
Pogwizd, S. M. (1995). Nonreentrant mechanism underlying spontaneous ventricular arrhythmias in a model of nonischemic heart failure in rabbits. Circulation 192, 1034–1048.
Prosser, B. L., Khairallah, R. J., Ziman, A. P., Ward, C. W., and Lederer, W. J. (2013). X-ROS signaling in the heart and skeletal muscle: stretch-dependent local ROS regulates [Ca2+]i. J. Mol. Cell Cardiol. 58, 172–181. doi: 10.1016/j.yjmcc.2012.11.011
Redfern, W. S., Carlsson, L., Davis, A. S., Lynch, W. G., MacKenzie, I., Palethorpe, S., et al. (2003). Relationships between preclinical cardiac electrophysiology, clinical QT interval prolongation and torsade de pointes for a broad range of drugs: evidence for a provisional safety margin in drug development. Cardiovasc. Res. 58, 32–45. doi: 10.1016/s0008-6363(02)00846-5
Sala, L., Hegyi, B., Bartolucci, C., Altomare, C., Rocchetti, M., Váczi, K., et al. (2017). Action potential contour contributes to species differences in repolarization response to β-adrenergic stimulation. Europace 20, 1543–1552. doi: 10.1093/europace/eux236
Schwartz, P. J., Priori, S. G., Locati, E. H., Napolitano, C., Cantù, F., Towbin, J. A., et al. (1995). Long QT syndrome patients with mutations of the SCN5A and HERG genes have differential responses to Na+ channel blockade and to increases in heart rate. Circulation 92, 3381–3386. doi: 10.1161/01.Cir.92.12.3381
Schwartz, P. J., Priori, S. G., Spazzolini, C., Moss, A. J., Vincent, G. M., Napolitano, C., et al. (2001). Genotype-phenotype correlation in the long-QT syndrome: gene-specific triggers for life-threatening arrhythmias. Circulation 103, 89–95. doi: 10.1161/01.cir.103.1.89
Signore, S., Sorrentino, A., Ferreira-Martins, J., Kannappan, R., Shafaie, M., Del Ben, F., et al. (2013). Inositol 1, 4, 5-trisphosphate receptors and human left ventricular myocytes. Circulation 128, 1286–1297. doi: 10.1161/CIRCULATIONAHA.113.002764
Song, Z., Ko, C. Y., Nivala, M., Weiss, J. N., and Qu, Z. (2015). Calcium-voltage coupling in the genesis of early and delayed afterdepolarizations in cardiac myocytes. Biophys. J. 108, 1908–1921. doi: 10.1016/j.bpj.2015.03.011
Szczepanska-Sadowska, E., Czarzasta, K., and Cudnoch-Jedrzejewska, A. (2018). Dysregulation of the renin-angiotensin system and the vasopressinergic system interactions in cardiovascular disorders. Curr. Hypertens. Rep. 20:19. doi: 10.1007/s11906-018-0823-9
Varagic, J., and Frohlich, E. D. (2002). Local cardiac renin–angiotensin system: hypertension and cardiac failure. J. Mol. Cell. Cardiol. 34, 1435–1442. doi: 10.1006/jmcc.2002.2075
Venetucci, L. A., Trafford, A. W., O'Neill, S. C., and Eisner, D. A. (2008). The sarcoplasmic reticulum and arrhythmogenic calcium release. Cardiovasc. Res. 77, 285–292. doi: 10.1093/cvr/cvm009
Winter, J., and Shattock, M. J. (2016). Geometrical considerations in cardiac electrophysiology and arrhythmogenesis. Europace 18, 320–331. doi: 10.1093/europace/euv307
Wu, X., Zhang, T., Bossuyt, J., Li, X., McKinsey, T. A., Dedman, J. R., et al. (2006). Local InsP3-dependent perinuclear Ca2+ signaling in cardiac myocyte excitation-transcription coupling. J. Clin. Invest. 116, 675–682. doi: 10.1172/JCI27374
Wu, Y., Roden, D. M., and Anderson, M. E. (1999). Calmodulin kinase inhibition prevents development of the arrhythmogenic transient inward current. Circ. Res. 84, 906–912.
Wullschleger, M., Blanch, J., and Egger, M. (2017). Functional local crosstalk of inositol 1,4,5-trisphosphate receptor- and ryanodine receptor-dependent Ca2+ release in atrial cardiomyocytes. Cardiovasc. Res. 113, 542–552. doi: 10.1093/cvr/cvx020
Yang, T., Snyders, D., and Roden, D. M. (2001). Drug block of I(kr): model systems and relevance to human arrhythmias. J. Cardiovasc. Pharmacol. 38, 737–744. doi: 10.1097/00005344-200111000-00010
Zaza, A., Micheletti, M., Brioschi, A., and Rocchetti, M. (1997). Ionic currents during sustained pacemaker activity in rabbit sino-atrial myocytes. J. Physiol. 505, 677–688.
Keywords: action potential duration, angiotensin II, SR stability, arrhythmias, repolarization reserve, β-adrenergic activation
Citation: Ronchi C, Badone B, Bernardi J and Zaza A (2019) Action Potential Prolongation, β-Adrenergic Stimulation, and Angiotensin II as Co-factors in Sarcoplasmic Reticulum Instability. Front. Physiol. 9:1893. doi: 10.3389/fphys.2018.01893
Received: 06 September 2018; Accepted: 14 December 2018;
Published: 09 January 2019.
Edited by:
Daniel M. Johnson, University of Birmingham, United KingdomReviewed by:
Andrew F. James, University of Bristol, United KingdomSenka Holzer, Medical University of Graz, Austria
Copyright © 2019 Ronchi, Badone, Bernardi and Zaza. This is an open-access article distributed under the terms of the Creative Commons Attribution License (CC BY). The use, distribution or reproduction in other forums is permitted, provided the original author(s) and the copyright owner(s) are credited and that the original publication in this journal is cited, in accordance with accepted academic practice. No use, distribution or reproduction is permitted which does not comply with these terms.
*Correspondence: Antonio Zaza, YW50b25pby56YXphQHVuaW1pYi5pdA==