- 1Center of Excellence in Poultry Science, University of Arkansas, Fayetteville, AR, United States
- 2Department of Animal Physiology and Endocrinology, University of Agriculture in Krakow, Kraków, Poland
The present review summarizes the state of knowledge of endogenous opioids in birds. Endogenous opioid peptides acts in a neuromodulatory, hormonal and paracrine manner to mediate analgesic and other physiological functions. These peptides act through specific G-protein coupled receptors. Opioid receptors consist of a family of four closely-related proteins. The three types of opioid receptors are the mu (MOR or μ), delta (DOR or δ), and kappa (KOR or κ) opioid receptor proteins. The role of the fourth member of the opioid receptor family, the nociceptin or orphanin FQ receptor (ORL), is not clear. The ligands for opioid receptors are: β –endorphin (MOR), Met- enkephalin, Leu-enkephalin (DOR) and dynorphin (KOR), together with probably endomorphins 1 and 2. In spite of long history of research on endogenous opioid peptides, there are no studies of endogenous opioids per se in wild birds and few in poultry species. β-endorphin is present in all birds investigated and there is close agreement between the structures of β-endorphin in different birds. Plasma concentrations of β-endorphin are increased by ether stress in geese. There is evidence that β-endorphin plays a role in the control of luteinizing hormone release in chickens. Met-enkephalin is present in tissues such as the retina, hypothalamus, pituitary gland, and adrenals together with circulation of birds. Stresses such as crowding and withholding water increase circulating concentrations of Met-enkephalin in chickens. The structures of chicken dynorphin A and B have been deduced from cDNA. What is missing are comprehensive studies of plasma concentrations and expression of the full array of endogenous opioids in multiple avian species under different situations. Also, what is not known is the extent to which circulating or locally released or intra-cellular Met-enkephalin influence physiological process in birds. Thus, there is considerable scope for investigation of the physiology of endogenous opioids in birds.
Introduction
The ligands for opioid receptors are endogenous opioid peptides, specifically: Met- enkephalin, Leu-enkephalin, β -endorphin and dynorphin, together with probably endomorphins 1 and 2. These are the physiological signaling peptides that mimic the effects of synthetic and natural opioids such as morphine and codeine.
The opioid/orphanin gene family contains the following:
• Proenkephalin A (PENK) gene—encoding pro-enkephalin which is processed to the endogenous opioids—Met-enkephalin and Leu-enkephalin (Figure 1).
• Proopiomelanocortin (POMC) gene—encoding POMC which is processed to the endogenous opioid–β-endorphin (containing the amino-acid sequence of Met-enkephalin) together with adrenocorticotropin (ACTH) and other peptides (Figure 1).
• Prodynorphin (proenkephalin B, PDYN) gene–encoding pro-dynorphin which is processed to the endogenous opioid–dynorphin (Figure 1).
• Pronociceptin/proorphanin FQ (PNOC) gene–encoding pro-prepronociceptin which is processed to a series of peptides including nociceptin, nocistatin, and orphanin FQ2 (Figure 1).
The opioid receptors are members of the G-protein coupled receptors (GPCR) gene family and the rhodopsin-like superfamily of GPCR (reviewed Stevens, 2009):
• Delta (δ) opioid peptide receptor (DOR or DOPr or OP1 receptors) (d as in vas deferens). Endogenous ligand: Met enkephalin and Leu enkephalin together with probably β-endorphin.
• Mu (μ) opioid peptide receptor (MOR or MOPr or OP3 receptors) (m as in morphine). Endogenous ligand: β-endorphin and probably enkephalins and endomorphins 1 and 2.
• Kappa (κ) opioid peptide receptor (KOR or KOPr or OP3 receptors) (k as ketocyclazocine). Endogenous ligand: dynorphin and possibly other.
• Nociceptin or orphanin FQ receptor (ORL) or opioid receptor-like receptor OPRL1. Endogenous ligand: the neuropeptide, nociceptin or orphanin FQ (Grossman and Clement-Jones, 1983; Mansour et al., 1995; reviewed Stevens, 2009).
These endogenous opioid receptor agonists have analgesic (relieving pain) or antinociceptive (inhibiting the sensation of pain) properties. There are other peptides that bind to opioid receptors. For instance, the tetrapeptide, cytochrophin-4, is a breakdown product of cytochrome-b and influences memory formation in chicks (Freeman and Young, 2000). The carboxy-amidated tetrapeptides, endomorphins 1 and 2, have been isolated from bovine brain tissue (Zadina et al., 1999). However, the endomorphins have received little attention in avian species.
It is thought that the four opioid receptor genes and the four members of the opioid/orphanin family of genes are the result of two genome duplications leading to quadruplication of the ancestral genes; these occurring prior to the radiation of the Gnathostomes (Figure 2) (Khalap et al., 2005; Dreborg et al., 2008; Sundström et al., 2010). It has not been possible to identify pro-enkephalin genes in primitive vertebrates (Agnathans) or basal chordate (Dreborg et al., 2008; Sundström et al., 2010).
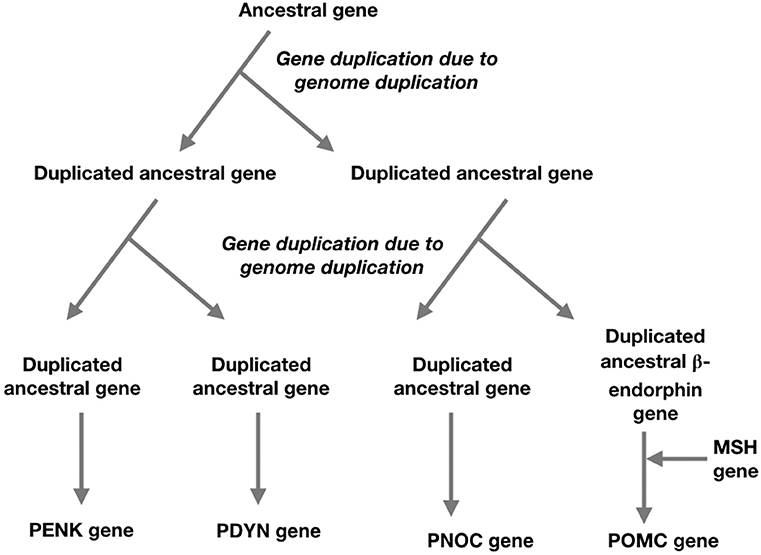
Figure 2. A schema for the evolution of the opioid/orphanin gene family based on two genome duplications and the insertion of melanocortin sequence to ancestral beta-endorphin gene (based on Sundström et al., 2010; Navarro et al., 2016).
The present review summarizes the state of knowledge of endogenous opioids in birds. In addition, areas ripe for research are covered. The communication discusses endogenous opioids in the following order: β-endorphin, Met-enkephalin and finally, products of the prodynorphin.
β-Endorphin in Birds
There is arguably more information on β-endorphin than of any other endogenous opioid in birds. This is particularly the case with the deduced structures of POMC from multiple species of birds together with mammals and reptiles.
Evolutionary Aspects of β-Endorphin
β-endorphin contains the Met-enkephalin pentapeptide motif (Figure 1). The deduced structures of β-endorphin in reptiles and birds together with evolutionary relationships are summarized in Figures 3, 4 (Shen et al., 2003). Despite the last common ancestor of reptiles and birds being about 250 million years ago, there is very close similarities between the structures of β-endorphin in reptiles and birds (Naudé et al., 2006; Shoureshi et al., 2007; Dores and Baron, 2011). A tentative structure of ancestral avian β-endorphin in the common ancestor of both birds and Crocodilia (i.e., within the Archosauromorpha, the clade includes birds, crocodyles and dinosaurs) is the following:
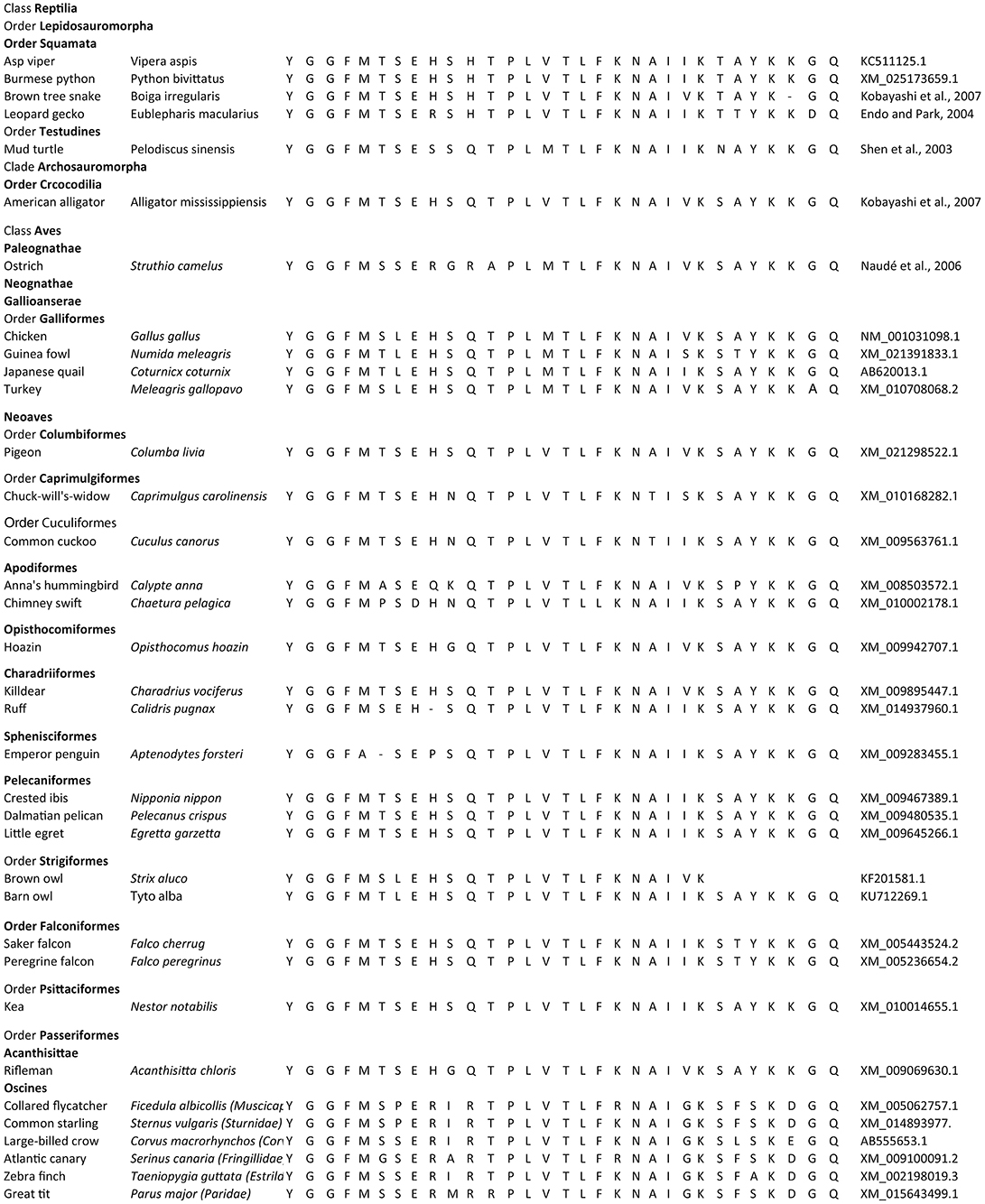
Figure 3. Evolution of β-endorphin. Comparison for the structures of β-endorphin in reptiles and birds.
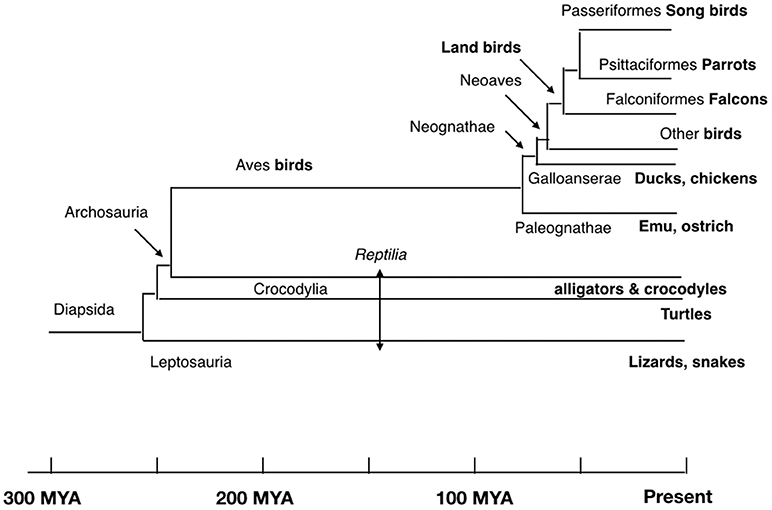
Figure 4. Evolutionary relationships of birds and reptiles showing conjectural time line (based on Hedges and Kumar, 2009; Chiari et al., 2012; Ezcurra et al., 2014).
YGGFMXSEHSQTPLVTLFKNAIVKSAYKKGQ (Where X is S or T) (Endo and Park, 2004).
This is identical to β-endorphin in both alligator and pigeon (Kobayashi et al., 2007). Within the class Aves, there are close similarities for β-endorphin. Excluding residue 6, there are two or fewer amino-acid residue differences between the ancestral form and that in multiple birds including chicken, egret, ibis, pelican, rifleman, and ruff (Figures 3, 4). This argues strongly that there is strong evolutionary pressure to maintain the structure of β-endorphin and, therefore, also of the physiological importance of β-endorphin in birds. In contrast, there are multiple differences in the Oscines (song birds) (Figures 3, 4).
Circulating Concentrations of β-Endorphin
There is limited information on circulating concentrations of β-endorphin in either wild or domesticated birds. In plasma from adult chickens, two-thirds of β-endorphin immune-reactivity measured by radioimmunoassay followed by Sephadex G-75 chromatography has an identical size as β-endorphin but one-third of β-endorphin is the same size as β-lipotropin (Hylka and Thommes, 1991).
The later research on domestic gander proved that plasma concentrations of immunoreactive β-endorphin measured by specific radioimmunoassay are about 30 pmoles · ml−1 (Barna et al., 1998a).
It might be assumed that circulating concentrations of β-endorphin would change in parallel with those of ACTH as they are both products of POMC. However, this does not seem to be necessarily the case. While, plasma concentrations of ACTH increased following castration in domestic geese, there is no effect on circulating concentrations of β-endorphin (Barna et al., 1998a). Moreover, while plasma concentrations of ACTH in domestic geese are increased by both ether stress or LPS endotoxin, plasma concentrations of β-endorphin are reported to be elevated following ether stress but not LPS endotoxin (Barna et al., 1998b). Thus, there is some evidence of independent control of synthesis and/or release and/or degradation of β-endorphin and ACTH.
Distribution of β-Endorphin and Tissue Concentrations
There are reports on the immunocytochemical studies of the regional distribution of β-endorphin containing neurons in avian brains (Bayon et al., 1980; Contijoch et al., 1993; Van Gils et al., 1994). β-endorphin as determined by radioimmunoassay has been reported to vary in the hypothalamus as follows: medial basal hypothalamus (MBH) (1.6 pmoles · mg−1) > paraventricular nucleus > pars nervosa/supra-optic nucleus > septum >> archistriatum/lobus parolfactorius (Barna et al., 1998a). Hypothalamic concentrations of β-endorphin are decreased at the time of spontaneous and progesterone induced pre-ovulatory LH surge (Contijoch et al., 1993).
β-endorphin is present in the goose anterior pituitary gland at lower concentrations than that of ACTH as follows: cephalic lobe: β-endorphin-867 pmol · lobe−1, ACTH-3596 pmol · lobe−1 (ratio 1:4.15); caudal lobe–β-endorphin-189 pmol · lobe−1, ACTH-383 pmol · lobe−1 (ratio 1:2.03) (Barna et al., 1998a).
Physiological Roles of β-Endorphin
There is evidence that β-endorphin is involved in the inhibitory control of gonadotropin releasing hormone (GnRH) release from the hypothalamus at least in chickens. β-endorphin cell bodies in the periarcuate area project to the median eminence (Contijoch et al., 1993). Intra-ventricular administration of β-endorphin blocks the pre-ovulatory LH surge (Sakurai et al., 1986). Moreover, β-endorphin depresses in vitro GnRH release from hypothalamic tissue from hens during pre-ovulatory surge of luteinizing hormone (LH) (Contijoch et al., 1993)
Met-Enkephalin in Birds
The Met-enkephalin together with Leu-enkephalin are pentapeptide endogenous opioids:
• Met-enkephalin - Tyr-Gly- Gly-Phe-Met (YGGFM)
• Leu-enkephalin - Tyr-Gly-Gly-Phe-Leu (YGGFL)
These neuropeptides were initially isolated from the brain of pigs (Hughes et al., 1975). Met- enkephalin and Leu-enkephalin are produced by proteolytic cleavage of pre-proenkephalin at paired-basic amino acid residues. Proenkephalin contains seven enkephalin motifs:
• Motifs 1-3 Met-E (Met-enkephalin)
• Motif 4 - MEAGL – octapeptide – Met-Enkephalin-Arg-Gly-Leu,
• Motif 5 - Met-E (Met-enkephalin),
• Motif 6 - Leu-E (Leu-enkephalin)
• Motif 7 MEAP - heptapeptide - Met-Enkephalin-Arg-Phe.
Evolutionary Aspects of Enkephalins
Met- and Leu-enkephalin are identical across the vertebrates (Roberts et al., 2007). There are seven enkephalin motifs in proenkephalin (Figure 1) in chickens and across the gnathostomes (jawed vertebrates). While, Met-enkephalin is identical, there are differences in both the heptapeptide (e.g., amphibian, newt - YGGFMRY and zebrafish – YGGFMGY) and octapeptide (e.g.,YGGFMRGY in the primitive mammal-platypus and amphibians; YGGFMRSV – chickens) (Bojnik et al., 2010, 2011).
The PENK gene has not been identified in invertebrates. However, invertebrate tissues have been reported to contain proteins homologous to proenkephalin (Stefano and Salzet, 1999). Immuno-reactive Met-enkephalin has been detected in, for instance, crustaceans (Jaros et al., 1985), annelids (Tasiemski et al., 2000) and tunicates (Georges and Dubois, 1984). In addition, Leu-enkephalin and delta opioid receptor immuno-reactivity are found in a mollusk, the octopus (Sha et al., 2012). Moreover, enkephalins influence the physiology of invertebrates, for instance, disrupting reproduction in insects (Kumar et al., 2013) and impacting both heart and immune functioning in molluscs (Tasiemski et al., 2000; Liu et al., 2016).
Circulating Concentrations of Met-Enkephalin
Plasma concentrations of Met-enkephalin in chickens are 28 pg · ml−1 (~50 fmoles · ml−1) (Pierzchala and Van Loon, 1990). There is a larger form of Met-enkephalin called cryptic ([Met5]–enkephalin) that found both in the circulation and in tissues; this being enzymatically cleaved (processed) to the pentapeptide (Pierzchala and Van Loon, 1990). Cryptic Met-enkephalin appears to be not only the storage of Met-enkephalin (free pentapeptide) but is also quickly processed under different stressors such as withholding of food and water, overcrowding, cold and restraint (Pierzchala-Koziec et al., 1999). Cryptic Met-enkephalin concentration in blood plasma of growing chickens was ~4.2 pmoles · ml−1 (Pierzchała-Koziec and Mazurkiewicz-Karasińska, 1997; Pierzchala-Koziec et al., Submitted). Enkephalins have short half-lives in the circulation. For instance, Leu-enkephalin is rapidly degraded by aminopeptidase M in chicken plasma with a half-life of 0.7–1.0 min in vitro (Shibanoki et al., 1991). Thus, aminopeptidases inhibitors need to be present in tubes and syringes when sampling birds.
Some stressors have been demonstrated to increase plasma concentrations of Met enkephalin in chickens. This is in addition to the stress induced activation of the hypothalamo-pituitary-adrenocortical (HPA) axis. Plasma concentrations of Met-enkephalin were increased by short term crowding in chickens with the effect being attenuated in the presence of the opioid antagonist, naltrexone (Pierzchała-Koziec et al., 1996; Pierzchala-Koziec et al., Submitted). Fasting increased plasma concentrations of Met enkephalin in female chickens but decreased those in male chickens (Pierzchała-Koziec and Mazurkiewicz-Karasińska, 1997; Pierzchala-Koziec et al., Submitted). Moreover, acute withholding of water was accompanied by increased plasma concentrations of Met-enkephalin (Pierzchala-Koziec et al., Submitted). What are not known are the effects of stress on Met-enkephalin in wild birds or the physiological relevance of increased plasma concentrations of Met-enkephalin during stress.
Control of Met-Enkephalin Release
There is very limited information on control of Met-enkephalin release. In chickens, release of Met-enkephalin from the hypothalamus and pituitary gland in vitro is depressed in tissues taken from birds subjected to water deprivation stress (Mazurkiewicz-Karasińska and Pierzchała-Koziec, 1997; Pierzchala-Koziec et al., Submitted). There are increases in release of Met-enkephalin and cryptic [Met5]- enkephalin from the retina during the night/darkness in chickens (Dowton et al., 1990).
There is evidence that endogenous opioids depress in vitro release of Met-enkephalin from the hypothalamus. In vitro release of Met-enkephalin is increased when chicken hypothalamic fragments are incubated in the presence of naltrexone (Mazurkiewicz-Karasińska and Pierzchała-Koziec, 1997; Pierzchala-Koziec et al., Submitted). There are changes in release of Met-enkephalin and cryptic [Met5]- enkephalin from the retina during the night/darkness in chickens (Dowton et al., 1990).
There is some evidence of cross talk between the HPA axis and Met-enkephalin in birds. This is supported by the report that stress increases plasma concentrations of both Met-enkephalin, and corticosterone in chickens (Pierzchala-Koziec et al., Submitted). In addition, corticotropin releasing hormone (CRH) increases release of both Met-enkephalin (Pierzchala-Koziec et al., Submitted) and ACTH in chickens (Nakayama et al., 2011). Moreover, there is evidence that glucocorticoids influence release of Met-enkephalin with dexamethasone increasing release of Met-enkephalin in vitro from either chicken hypothalamic or adrenal tissue (Pierzchala-Koziec et al., Submitted).
Distribution of Met-Enkephalin and Tissue Concentrations
Table 1 summarizes the available information on the distribution of Met-enkephalin. The distribution of enkephalin neurons has been reported in the pigeon brain (Bayon et al., 1980). Enkephalin neurons are found, for instance, in the brainstem, limbic regions, organum vasculosum hypothalamic, paleostriatum, and pituitary stalk (Bayon et al., 1980). There are marked differences in the distribution of enkephalin neurons and those containing β-endorphin (Bayon et al., 1980).
Tissue concentration of Met-enkephalin and PENK gene expression have been determined.
• Met-enkephalin concentrations in chickens: Pituitary > hypothalamus> adrenal gland > cerebellum >heart>kidney (Pierzchala-Koziec et al., Submitted).
• PENK expression: Pituitary > hypothalamus > adrenal gland > cerebellum >> heart atria > heart ventricles > kidneys (Pierzchala-Koziec et al., Submitted).
Tissue concentrations of Met-enkephalin change during avian embryonic development. There are large increases (>1,000 fold) in immuno-reactive (IR-) Met-enkephalin in the lumbar spinal cord between days 4.5 and 18 in chick of embryos (Maderdrut et al., 1986). Moreover, IR-Met-enkephalin is not observed in the circular smooth muscle until day 17 of embryonic development (Epstein et al., 1983).
Physiological Role of Met-Enkephalin
Met-enkephalin may act in neuromodulatory, and/or hormonal and/or paracrine manners. Met-enkephalin inhibits release of gonadotropin releasing hormone (GnRH) from hypothalamic tissue from male chickens in vitro with the effect being via mu opioid receptors (Stansfield and Cunningham, 1987, 1988). It is unclear whether these observations reflect an effect of Met-enkephalin per se or exogenous Met-enkephalin acting as a surrogate for other endogenous opioids. Met-enkephalin has been reported to have other effects in birds. For instance, Met-enkephalin acts as a gastric inhibitor in chickens via mu receptors (Jiménez et al., 1993).
Dynorphin in Birds
There is very limited information on dynorphin in birds. The structures of chicken dynorphins can be deduced from the cDNA:
• Dynorphin A - YGGFMRRIRPKLKWDN
• Dynorphin B - YGGFLRRQFKVTT
(based on Figure 1). An additional peptide product is α-neo-endorphin.
Circulating Concentrations of Products of the Prodynorphin
There are no reports on circulating concentrations of dynorphin A or B in birds. However, overcrowding stress was associated with increased plasma concentrations of α-neo-endorphin (Pierzchała-Koziec et al., 1996).
Distribution of Dynorphin
The distribution of dynorphin neurons in the pigeon brain has been reported (Reiner, 1986). Outside of the hypothalamus, there are neurons containing both substance P and dynorphin (Reiner, 1986).
Physiological Effects of Dynorphin
There is evidence that dynorphin plays a role in the neuroendocrine control of prolactin release in birds. Intraventricular infusion of dynorphin into the II ventricle is followed by increases in circulating concentrations of prolactin in turkeys with the effect mediated via kappa (κ) opioid receptors as demonstrated by κ opioid receptor antagonists to block the effect (Youngren et al., 1993, 1999).
Other Neuroendocrine Effects of Endogenous Opioids in Birds
There is evidence that endogenous opioids influence release of arginine vasotocin (AVT) in birds. Plasma concentrations of AVT, but not those of mesotocin, are increased by morphine and a specific mu receptor agonist in chickens (Saito et al., 1999; Sasaki et al., 2000). Moreover, AVT release is depressed by the opioid receptor antagonist, naloxone, in hypertonic saline treated chickens (Saito et al., 1999). It is not clear which endogenous opioid influences AVT release. It is reasonable to exclude dynorphin as it is not present in the pars nervosa of the chicken (Martin et al., 1992). Opioid agonists appear to inhibit whereas antagonists stimulate socio-sexual interactions in starlings (Riters, 2011). Moreover, it was suggested that enkephalin in medial preoptic area is involved in reward associated with both feeding and sexual behavior (Riters, 2011).
Conclusions and Future Directions
There are few studies of endogenous opioids in poultry species and none in wild birds. The available evidence that both β-endorphin and Met-enkephalin are present across the class Aves. The structure of β-endorphin is very similar across birds suggesting its importance. There have been some physiological studies and all support a relationship between both β-endorphin and Met-enkephalin and stress. What is missing are comprehensive studies of plasma concentrations, expression and receptors of the full array of endogenous opioids in multiple avian species under the following situations:
• Temporal changes during annual, daily/circadian and ovulatory cycles.
• Acute responses in response to activators of the HPA axis, other stressors (e.g., disease), neuropeptides and hormones.
• Interactions between the different endogenous opioids and their receptors.
There is considerable scope for investigation of the physiology of endogenous opioids in birds.
Author Contributions
KP-K and CS: conceived, drafted and wrote the review. It is based in part on research from KP-K's laboratory. CS and KP-K: re-analyzed the research and together discussed their relevance.
Conflict of Interest Statement
The authors declare that the research was conducted in the absence of any commercial or financial relationships that could be construed as a potential conflict of interest.
Acknowledgments
Financed by The National Centre of Science (PL) No: 12006406 and University of Agriculture Research Funding No:DS 3243/DAPE/2017-2018.
References
Atoji, Y., Yamamoto, Y., Komatsu, T., and Suzuki, Y. (1997). Localization of neuropeptides in endocrine cells of the chicken thymus. J. Vet. Med. Sci. 59, 601–603. doi: 10.1292/jvms.59.601
Barna, I., Koenig, J. I., and Péczely, P. (1998a). Characteristics of the proopiomelanocortin system in the outdoor-bred domestic gander. I. ACTH and beta-endorphin levels in the brain, pituitary, and plasma. Gen. Comp. Endocrinol. 109, 44–51. doi: 10.1006/gcen.1997.7006
Barna, I., Koenig, J. I., and Péczely, P. (1998b). Characteristics of the proopiomelanocortin system in the outdoor-bred domestic gander. II. Seasonal and circadian rhythmicity; effect of ether stress and lipopolysaccharide administration. Gen. Comp. Endocrinol. 109, 52–59. doi: 10.1006/gcen.1997.7002
Bayon, A., Koda, L., Battenberg, E., Azad, R., and Bloom, F. E. (1980). Regional distribution of endorphin, Met5-enkephalin and Leu5- enkephalin in the pigeon brain. Neurosci. Lett. 16, 75–80. doi: 10.1016/0304-3940(80)90104-4
Bojnik, E., Babos, F., Magyar, A., Borsodi, A., and Benyhe, S. (2010). Bioinformatic and biochemical studies on the phylogenetic variability of proenkephalin-derived octapeptides. Neuroscience 165, 542–552. doi: 10.1016/j.neuroscience.2009.10.008
Bojnik, E., Boynik, E., Corbani, M., Babos, F., Magyar, A., Borsodi, A., et al. (2011). Phylogenetic diversity and functional efficacy of the C-terminally expressed heptapeptide unit in the opioid precursor polypeptide proenkephalin A. Neuroscience 178, 56–67. doi: 10.1016/j.neuroscience.2011.01.008
Chiari, Y., Cahais, V., Galtier, N., and Delsuc, F. (2012). Phylogenomic analyses support the position of turtles as the sister group of birds and crocodiles (Archosauria). BMC Biol. 10:65. doi: 10.1186/1741-7007-10-65
Contijoch, A. M., Malamed, S., Sarkar, D. K., and Advis, J. P. (1993). Beta-endorphin regulation of LHRH release at the median eminence level: immunocytochemical and physiological evidence in hens. Neuroendocrinology 57, 365–373. doi: 10.1159/000126381
Dores, R. M., and Baron, A. J. (2011). Evolution of POMC: origin, phylogeny, posttranslational processing, and the melanocortins. Ann. N. Y. Acad. Sci. 1220, 34–48. doi: 10.1111/j.1749-6632.2010.05928.x
Dowton, M., Boelen, M. K., Morgan, I. G., and Chubb, I. W. (1990). Light inhibits the release of both [Met5] enkephalin and [Met5] enkephalin-containing peptides in chicken retina, but not their syntheses. Neuroscience 38, 187–193. doi: 10.1016/0306-4522(90)90384-G
Dreborg, S., Sundström, G., Larsson, T. A., and Larhammar, D. (2008). Evolution of vertebrate opioid receptors. Proc. Natl. Acad. Sci. U.S.A. 105, 15487–14392. doi: 10.1073/pnas.0805590105
Endo, D., and Park, M. K. (2004). Molecular characterization of the leopard gecko POMC gene and expressional change in the testis by acclimation to low temperature and with a short photoperiod. Gen. Comp. Endocrinol. 138, 70–77. doi: 10.1016/j.ygcen.2004.04.009
Epstein, M. L., Hudis, J., and Dahl, J. L. (1983). The development of peptidergic neurons in the foregut of the chick. J. Neurosci. 3, 2431–2447. doi: 10.1523/JNEUROSCI.03-12-02431.1983
Ezcurra, M. D., Scheyer, T. M., and Butler, R. J. (2014). The origin and early evolution of sauria: reassessing the permian saurian fossil record and the timing of the crocodile-lizard divergence. PLoS ONE 9:e89165. doi: 10.1371/journal.pone.0089165
Freeman, F. M., and Young, I. G. (2000). Inhibition of passive-avoidance memory formation in the day-old chick by the opioid cytochrophin-4. Learn. Mem. 7, 213–219. doi: 10.1101/lm.7.4.213
Georges, D., and Dubois, M. P. (1984). Methionine-enkephalin-like immunoreactivity in the nervous ganglion and the ovary of a protochordate, Ciona intestinalis. Cell Tiss. Res. 236, 165–170. doi: 10.1007/BF00216527
Grossman, A., and Clement-Jones, V. (1983). Opiate receptors: enkephalins and endorphins. Clin. Endocrinol. Metab. 12, 31–56. doi: 10.1016/S0300-595X(83)80028-0
Hughes, J., Smith, T. W., Kosterlitz, H. W., Fothergiil, L., Morgan, B. A., and Morris, H. R. (1975). Identification of two related pentapeptides from the brain with potent opiate agonist activity. Nature 258, 577–579. doi: 10.1038/258577a0
Hylka, V. W., and Thommes, R. C. (1991). Avian beta-endorphin: alterations in immunoreactive forms in plasma and pituitary of embryonic and adult chickens. Comp. Biochem. Physiol. C. 100, 643–648. doi: 10.1016/0742-8413(91)90054-W
Jaros, P. P., Dircksen, H., and Keller, R. (1985). Occurrence of immunoreactive enkephalins in a neurohemal organ and other nervous structures in the eyestalk of the shore crab, Carcinus maenas L. (Crustacea, Decapoda). Cell Tiss. Res. 241, 111–117. doi: 10.1007/BF00214632
Jiménez, M., Martínez, V., Goñalons, E., and Vergara, P. (1993). In vivo modulation of gastrointestinal motor activity by Met-enkephalin, morphine and encephalin analogs in chickens. Regul. Pept. 44, 71–83. doi: 10.1016/0167-0115(93)90131-Q
Khalap, A., Bagrosky, B., Lecaude, S., Youson, J., Danielson, P., and Dores, R. M. (2005). Trends in the evolution of the proenkephalin and prodynorphin genes in gnathostomes. Ann. N. Y. Acad. Sci. 1040, 22–37. doi: 10.1196/annals.1327.003
Kobayashi, Y., Sakamoto, T., Iguchi, K., Imai, Y., Hoshino, M., Lance, V. A., et al. (2007). cDNA cloning of proopiomelanocortin (POMC) and mass spectrometric identification of POMC-derived peptides from snake and alligator pituitaries. Gen. Comp. Endocrinol. 152, 73–81. doi: 10.1016/j.ygcen.2007.02.026
Kumar, S., Ganji, P. N., Song, H., von Kalm, L., and Borst, D. W. (2013). Exposure to exogenous enkephalins disrupts reproductive development in the eastern lubber grasshopper, Romalea microptera (Insecta: Orthoptera). PLoS ONE 8:10. doi: 10.1371/annotation/7c19edf3-4648-45de-8132-16092fc4bf40
Liu, Z., Wang, L., Zhou, Z., Sun, Y., Wang, M., Wang, H., et al. (2016). The simple neuroendocrine-immune regulatory network in oyster Crassostrea gigas mediates complex functions. Sci. Rep. 6:26396. doi: 10.1038/srep26396
Maderdrut, J. L., Merchenthaler, I., Sundberg, D. K., Okado, N., and Oppenheim, R. W. (1986). Distribution and development of proenkephalin-like immunoreactivity in the lumbar spinal cord of the chicken. Brain Res. 377, 29–40. doi: 10.1016/0006-8993(86)91187-X
Mansour, A., Hoversten, M. T., Taylor, L. P., Watson, S. J., and Akil, H. (1995). The cloned mu, delta and kappa receptors and their endogenous ligands: evidence for two opioid peptide recognition cores. Brain Res. 700, 89–98. doi: 10.1016/0006-8993(95)00928-J
Martin, R., McGregor, G. P., Halbinger, G., Falke, N., and Voigt, K. H. (1992). Methionine 5-enkephalin and opiate binding sites in the neurohypophysis of the bird, Gallus domesticus. Regul. Pept. 38, 33–44. doi: 10.1016/0167-0115(92)90070-B
Martínez, A., López, J., and Sesma, P. (2000). The nervous system of the chicken proventriculus: an immunocytochemical and ultrastructural study. Histochem. J. 32, 63–70. doi: 10.1023/A:1003962413417
Mazurkiewicz-Karasińska, D., and Pierzchała-Koziec, K. (1997). “The effects of dehydration on the in vitro release of Met-enkephalin from hen tissues,” in Mol.Physiol. Aspects Reg. Process. (Krakow: Organism), 247–249.
Nakayama, H., Takahashi, T., Nakagawa-Mizuyachi, K., and Kawashima, M. (2011). Effect of calcitonin on adrenocorticotropic hormone secretion stimulated by corticotropin-releasing hormone in the hen anterior pituitary. Anim. Sci. J. 82, 475–480. doi: 10.1111/j.1740-0929.2010.00850.x
Naudé, R., Oelofsen, W., Takahashi, A., Amano, M., and Kawauchi, H. (2006). Molecular cloning and characterization of preproopiomelanocortin (prePOMC) cDNA from the ostrich (Struthio camelus). Gen. Comp. Endocrinol. 146, 310–317. doi: 10.1016/j.ygcen.2005.11.018
Navarro, S., Soletto, L., Puchol, S., Rotllant, J., Soengas, J. L., and Cerdá-Reverter, J. M. (2016). POMC: an evolutionary perspective. J. Mol. Endocrinol. 56, T113–T118. doi: 10.1530/JME-15-0288
Ohmori, Y., Okada, Y., and Watanabe, T. (1997). Immunohistochemical localization of serotonin, galanin, cholecystokinin, and methionine-enkephalin in adrenal medullary cells of the chicken. Tiss. Cell 29, 199–205. doi: 10.1016/S0040-8166(97)80019-5
Ohmori, Y., Suzuki, M., and Fukuta, K. (2003). Anatomical relationship between enkephalin-containing neurones and caecum-projecting neurones in the chicken intestinal nerve. Anat. Histol. Embryol. 32, 179–182. doi: 10.1046/j.1439-0264.2003.00457.x
Pierzchala, K., and Van Loon, G. R. (1990). Plasma native and peptidase-derivable Met-enkephalin responses to restraint stress in rats. Adaptation to repeated restraint. J. Clin. Invest. 85, 861–873. doi: 10.1172/JCI114513
Pierzchała-Koziec, K., and Mazurkiewicz-Karasińska, D. (1997). “The chromatographic profile of enkephalin-like peptides in blood and tissues of hen,” in Mol.Physiol. Aspects Reg. Process. (Krakow: Organism), 286–288.
Pierzchała-Koziec, K., Niezgoda, J., Bobek, S., and Przewłocki, R. (1996). Endogenous opioids may modulate the activity of the hypothalamus-pituitary-adrenocortical axis in domestic fowl. Zentralbl. Veterinarmed. A. 43, 139–146. doi: 10.1111/j.1439-0442.1996.tb00438.x
Pierzchala-Koziec, K., Niezgoda, J., and Jacek, T. (1999). Hormonal responses to exogenous glucocorticoidds in hens pretreated with opioid delta receptor agonist and antagonist. Mol. Physiol. Aspects Reg. Proc. Org. 8, 277–278.
Reiner, A. (1986). The co-occurrence of substance P-like immunoreactivity and dynorphin-like immunoreactivity in striatopallidal and striatonigral projection neurons birds and reptiles. Brain Res. 371, 155–161. doi: 10.1016/0006-8993(86)90821-8
Riters, L. V. (2011). Pleasure seeking and birdsong. Neurosci. Biobehav. Rev. 35, 1837–1845. doi: 10.1016/j.neubiorev.2010.12.017
Roberts, E., Shoureshi, P., Kozak, K., Szynskie, L., Baron, A., Lecaude, S., et al. (2007). Tracking the evolution of the proenkephalin gene in tetrapods. Gen. Comp. Endocrinol. 153, 189–197. doi: 10.1016/j.ygcen.2007.02.023
Saito, N., Furuse, M., Sasaki, T., Arakawa, K., and Shimada, K. (1999). Effects of naloxone on neurohypophyseal peptide release by hypertonic stimulation in chicks. Gen. Comp. Endocrinol. 115:228–235. doi: 10.1006/gcen.1999.7302
Sakurai, H., Kawashima, M., Kamiyoshi, M., and Tanaka, K. (1986). Effect of serotonin and beta-endorphin on the release of luteinizing hormone in the hen (Gallus domesticus). Gen. Comp. Endocrinol. 63, 24–30. doi: 10.1016/0016-6480(86)90177-2
Sasaki, T., Shimada, K., and Saito, N. (2000). Regulation of opioid peptides on the release of arginine vasotocin in the hen. J. Exp. Zool. 286, 481–486. doi: 10.1002/(SICI)1097-010X(20000401)286:5<481::AID-JEZ5>3.0.CO;2-J
Sha, A., Sun, H., and Wang, Y. (2012). Immunohistochemical study of leucine-enkephalin and delta opioid receptor in mantles and feet of the octopus, Octopus ocellatus gray. Int. J. Pept. Res. Ther. 18, 71–76. doi: 10.1007/s10989-011-9280-x
Shen, S. T., Lu, L. M., Chen, J. R., Chien, J. T., and Yu, J. Y. (2003). Molecular cloning of proopiomelanocortin (POMC) cDNA from mud turtle, Pelodiscus sinensis. Gen. Comp. Endocrinol. 131, 192–201. doi: 10.1016/S0016-6480(03)00028-5
Shibanoki, S., Weinberger, S. B., Beniston, D., Nudelman, K. A., Schulteis, G., Bennett, E. L., et al. (1991). Hydrolysis of [Leu]enkephalin by chick plasma in vitro. J. Pharmacol. Exp. Ther. 256, 650–655.
Shoureshi, P., Baron, A., Szynskie, L., and Dores, R. M. (2007). Analyzing the evolution of beta-endorphin post-translational processing events: studies on reptiles. Gen. Comp. Endocrinol. 153, 148–154. doi: 10.1016/j.ygcen.2007.01.002
Stansfield, S. C., and Cunningham, F. J. (1987). Modulation by endogenous opioid peptides of the secretion of LHRH from cockerel (Gallus domesticus) mediobasal hypothalamic tissue. J. Endocrinol. 114, 103–110. doi: 10.1677/joe.0.1140103
Stansfield, S. C., and Cunningham, F. J. (1988). Attenuation of endogenous opioid peptide inhibition of [Gln8] luteinizing hormone-releasing hormone secretion during sexual maturation in the cockerel. Endocrinology 123, 787–794. doi: 10.1210/endo-123-2-787
Stefano, G. B., and Salzet, M. (1999). Invertebrate opioid precursors: evolutionary conservation and the significance of enzymatic processing. Int. Rev. Cytol. 187, 261–286. doi: 10.1016/S0074-7696(08)62420-1
Stevens, C. W. (2009). The evolution of vertebrate opioid receptors. Front. Biosci. 14, 1247–1269. doi: 10.2741/3306
Sundström, G., Dreborg, S., and Larhammar, D. (2010). Concomitant duplications of opioid peptide and receptor genes before the origin of jawed vertebrates. PLoS ONE 5:e10512. doi: 10.1371/journal.pone.0010512
Tasiemski, A., Verger-Bocquet, M., Cadet, M., Goumon, Y., Metz-Boutigue, M. H., Aunis, D., et al. (2000). Proenkephalin A-derived peptides in invertebrate innate immune processes. Brain Res. Mol. Brain Res. 76, 237–252. doi: 10.1016/S0169-328X(00)00005-X
Van Gils, J., Absil, P., Moons, L., Grauwels, L., Vandesdande, F., and Balthazart, J. (1994). Distribution of beta-endorphin-like immuno- reactive structures in the chicken and quail brain as demonstrated with a new homologous antibody directed against a synthetic peptide. J. Comp. Neurol. 350, 382–396. doi: 10.1002/cne.903500305
Youngren, O. M., Pitts, G. R., Chaiseha, Y., and El Halawani, M. E. (1999). An opioid pathway in the hypothalamus of the turkey that stimulates prolactin secretion. Neuroendocrinology 70, 317–323. doi: 10.1159/000054492
Youngren, O. M., Silsby, J. L., Phillips, R. E., and el Halawani, M. E. (1993). Dynorphin modulates prolactin secretion in the turkey. Gen. Comp. Endocrinol. 91, 224–231. doi: 10.1006/gcen.1993.1121
Keywords: β endorphin, Met-enkephalin, endogenous opioids, opioid receptor, stress
Citation: Scanes CG and Pierzchala-Koziec K (2018) Perspectives on Endogenous Opioids in Birds. Front. Physiol. 9:1842. doi: 10.3389/fphys.2018.01842
Received: 08 March 2018; Accepted: 06 December 2018;
Published: 21 December 2018.
Edited by:
Rocco Victor Carsia, Rowan University School of Osteopathic Medicine, United StatesReviewed by:
Takeshi Ohkubo, Ibaraki University, JapanGregoy Y. Bedecarrats, University of Guelph, Canada
Copyright © 2018 Scanes and Pierzchala-Koziec. This is an open-access article distributed under the terms of the Creative Commons Attribution License (CC BY). The use, distribution or reproduction in other forums is permitted, provided the original author(s) and the copyright owner(s) are credited and that the original publication in this journal is cited, in accordance with accepted academic practice. No use, distribution or reproduction is permitted which does not comply with these terms.
*Correspondence: Krystyna Pierzchala-Koziec, rzkoziec@cyf-kr.edu.pl