- 1Interdisciplinary Stem Cell Institute, University of Miami Leonard M. Miller School of Medicine, Miami, FL, United States
- 2Division of Cardiology, Department of Medicine, University of Miami Leonard M. Miller School of Medicine, Miami, FL, United States
- 3Department of Pathology, University of Miami Leonard M. Miller School of Medicine, Miami, FL, United States
- 4Vascular Biology Institute, University of Miami Leonard M. Miller School of Medicine, Miami, FL, United States
- 5Peggy and Harold Katz Family Drug Discovery Center, University of Miami Leonard M. Miller School of Medicine, Miami, FL, United States
Sepsis and pneumonia are major causes of death in the United States, and their pathophysiology includes infection with inflammation and immune dysfunction. Both sepsis and pneumonia cause cardiovascular dysfunction. The expression of Osteopontin (OPN) in cardiomyocytes of patients with sepsis or pneumonia, and its role the induced cardiac dysfunction have not been thoroughly investigated. OPN is a matricellular protein synthesized by multiple diseased tissues and cells including cardiomyocytes. Here, we studied the expression of OPN protein using immunofluorescence in human myocardial autopsy tissues from pediatric and mid age or elderly patients with sepsis and/or pneumonia. Fourteen human myocardial tissues from six pediatric patients and eight mid-age or elderly patients were studied. Immunofluorescence was used to investigate the expression of OPN in paraffin-embedded heart sections co-stained with the myocyte markers Actin Alpha 1 (ACTA1) and Myosin Light Chain 2 (MLC2). A quantitative analysis was performed to determine the number of ACTA1 and MLC2 positive cardiomyocytes that express OPN. The results showed that OPN expression was significantly increased in cardiomyocytes in the hearts from pediatric patients with sepsis and/or pneumonia (N = 3) relative to pediatric patients without sepsis/pneumonia (N = 3), or adult to elderly patients with sepsis/pneumonia (N = 5). Among the older septic hearts, higher levels of cardiomyocyte OPN expression was seen only in conjunction with severe coronary arterial occlusion. This is the first study to document increased OPN expression in cardiomyocytes of pediatric subjects with sepsis or pneumonia. Our findings highlight a potentially important role for OPN in sepsis- or pneumonia-mediated cardiac dysfunction in pediatric patients.
Introduction
Osteopontin (OPN) is a matricellular protein synthesized by a range of tissues and cell types including immunological cells (macrophages and T cells) (Lund et al., 2009), fibroblasts (Ashizawa et al., 1996; Jin et al., 2011), epithelial cells (Kato et al., 2014), osteoclasts, and osteoblasts (Qing et al., 2014), vascular smooth muscle cells (Sodhi et al., 2001), renal tubular epithelial cells (Zhang et al., 2010; Cobbs et al., 2018; Ding et al., 2018), and cardiomyocytes (Ashizawa et al., 1996; Lorenzen et al., 2015). OPN has been implicated as an important regulator of inflammation, biomineralization, cellular viability, cancer, diabetes, and renal disease (Icer and Gezmen-Karadag, 2018), and in the last few years has been getting increasing attention in the cardiovascular field (Singh et al., 2014; Li et al., 2017; Icer and Gezmen-Karadag, 2018). Under normal physiological conditions, the heart expresses low levels of OPN (Singh et al., 2014). However, OPN expression in the heart increases significantly under pathophysiological states such as myocardial infarction, hypertrophy, and heart failure in both pre-clinical (Graf et al., 1997; Trueblood et al., 2001; Li et al., 2017), and clinical studies (Graf et al., 1997; Stawowy et al., 2002; Lopez et al., 2013).
Two important conditions, sepsis (Merx and Weber, 2007; Rudiger and Singer, 2007, 2013; Hunter and Doddi, 2010) and pneumonia (Corrales-Medina et al., 2011, 2012; Singanayagam et al., 2012; Brown et al., 2014) can compromise the heart causing myocardial dysfunction. Sepsis is a systemic inflammatory response to an infection and can lead to death due to multiple organ failure, including the organs of the cardiovascular system (Hunter and Doddi, 2010). In the heart, some of the sepsis-caused alterations include contractile systolic and diastolic dysfunction (Poelaert et al., 1997; Jardin et al., 1999; Landesberg et al., 2012), arrhythmias (Leibovici et al., 2007), and right heart failure (Dhainaut et al., 1988; Parker et al., 1990). The mechanisms responsible for sepsis-caused cardiac dysfunction could be multifactorial (Merx and Weber, 2007; Liu et al., 2017). Studies have suggested roles in sepsis-caused cardiac dysfunction for endotoxins released by lysis of gram-negative bacteria together with the presence of Toll-like receptor-4 and CD14 (Suffredini et al., 1989; Tavener et al., 2004; Rudiger and Singer, 2007), inflammatory cytokines (mainly TNF-α, IL-1) (Vincent et al., 1992; Kumar et al., 1996; Hunter and Doddi, 2010), nitric oxide (Dal-Secco et al., 2017), high level of circulating catecholamine (Bocking et al., 1979; Rudiger and Singer, 2007), endothelin-1 (Shindo et al., 1998), mitochondrial dysfunction, and oxidative stress (Khadour et al., 2002; Watts et al., 2004; Matkovich et al., 2017), or altered intracellular calcium trafficking (Zhong et al., 1997; Rudiger and Singer, 2013). Few studies reported the elevated serum levels of OPN in patients with sepsis (Vaschetto et al., 2008) or the association of OPN with inflammation and mortality in a mouse model of sepsis (Fortis et al., 2015). However, the expression of OPN in cardiomyocytes of septic patients, and its role in sepsis-mediated cardiac dysfunction have not been studied.
Pneumonia is an inflammatory disease of the lung caused by bacteria, viruses, or fungi, and it is responsible for high morbidity and mortality in adults and children (Mattila et al., 2014; le Roux and Zar, 2017). Some reports have shown that pneumococcal pneumonia can lead to cardiac complications including heart failure, arrhythmias, and myocardial infarction (Musher et al., 2007; Corrales-Medina et al., 2012). Besides these studies on increased levels of OPN in the plasma of patients with interstitial (Kadota et al., 2005) and community-acquired pneumonia (Chang et al., 2016), and in the plasma and lung of mice infected with Streptococcus pneumonia (van der Windt et al., 2011), the cardiomyocyte expression of OPN and its role in pneumonia-mediated cardiac dysfunction have not been investigated.
This study quantifies the expression of OPN protein using immunofluorescence in human myocardial autopsy tissues from pediatric and elderly patients with sepsis or pneumonia.
Materials and Methods
Human Myocardial Tissue Samples
A total of 14 human myocardial and two control kidney autopsy tissues were obtained from the Department of Pathology at the University of Miami Leonard M. Miller School of Medicine. The myocardial tissues belonged to six pediatric (<2 years old) patients and eight adult or elderly (48–77 years old) patients. Table 1 lists demographic data for autopsy cases used for this study. These kidney tissues (especially the diseased one) were used as positive controls for OPN expression as it is known that renal tubular cells express high levels of OPN under pathological conditions (Ding et al., 2018).
This study was carried out in accordance with the recommendations of the University of Miami Institutional Review Board (IRB protocol # 20180439). All autopsy specimens were de-identified and there was no need for consent forms.
Staining and Image Acquisition
Immunofluorescence was used to investigate the OPN expression in paraffin-embedded sections. Samples were incubated for 45 min at 70 degrees, then dewaxed with two 5-min xylene washes and hydrated by 3-min graded ethanol washes of 100% (twice), 95%, 80%, and 70% followed by two 4-min water immersions. Antigen retrieval was then done by steaming the slides for 75 min in 1X Citrate Antigen Retrieval Buffer (Ab93678). Samples were rinsed with PBS then permeabilized with 0.2% Triton X-100 (Sigma-Aldrich) in PBS for 30 min, followed by blocking with 10% Donkey serum in TBST for 45 min. The samples were then incubated overnight at 4°C with three primary antibodies, Anti-Alpha Skeletal Muscle Actin, Alpha-Sr 1 (ACTA-1) (Abcam Ab28052; dilution 1:200), anti-hOPN (R&D AF1433; dilution 1:50), and anti-Myosin Light Chain (MLC2) (Abcam Ab92721; dilution 1:200) in blocking solution. ACTA-1 and MLC2 were used as cardiomyocyte markers. The following day the slides were washed with PBS then incubated for 1 h at room temperature with conjugated secondary antibodies Donkey anti-Mouse IgG, Alexa Fluor 568 (ThermoFisher Scientific A-10037; 1:400), Donkey anti-Goat IGG, Alexa Fluor 647 (ThermoFisher Scientific A-21447, 1:400), and Donkey anti-Rabbit IgG, Alexa Fluor 488 (ThermoFisher Scientific A-21206; 1:400) in blocking solution. DAPI was used to stain nuclei before mounting with ProLong Gold Antifade (Invitrogen P36934) and coverslips. After 2 days, the slides were scanned at 20 × magnification using the Olympus VS120–L100 Virtual Slide Microscope (Tokyo, Japan). At least five slides per specimen were stained to confirm results. Representative confocal images (shown in Figures 1–9) were captured at 40x magnification and 0.6x digital zoom using Z-stacking on a Zeiss LSM710 confocal microscope.
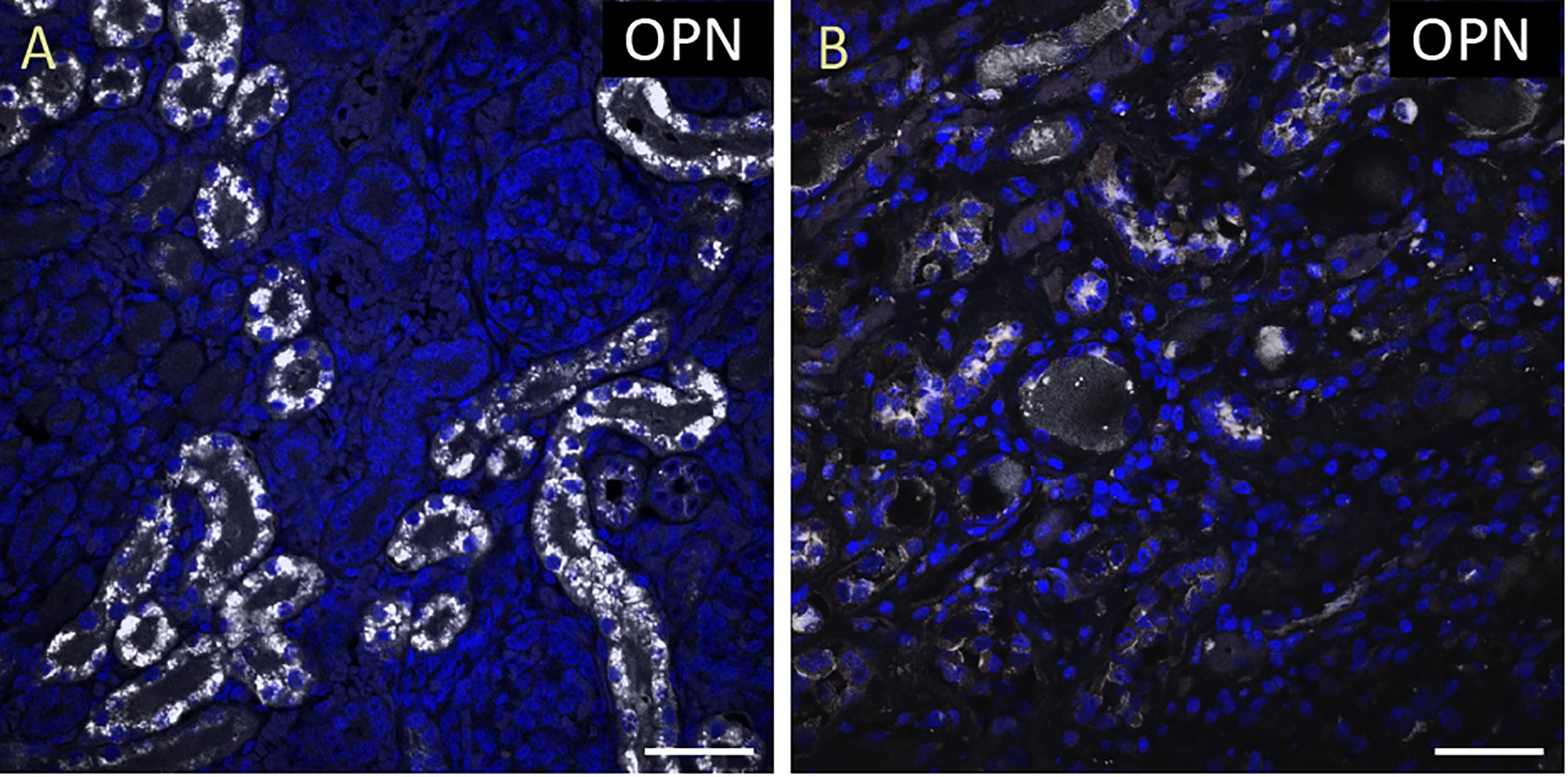
Figure 1. OPN is highly expressed in pediatric diseased kidney. (A) In the pediatric patient with enlarged right kidney, OPN (white) is highly expressed in the renal tubular epithelial cells. (B) In an elderly patient with a normal kidney, OPN (white) is moderately expressed in the renal tubular epithelial cells. Scale bar = 50 μm.
Image Analyses and Quantification of OPN Positive Myocytes
All immunostaining scanned images were analyzed using Olympus OlyVIA 2.9 software. A quantitative analysis was performed independently by two investigators to manually count the number of ACTA1 and MLC2 positive cardiomyocytes that express OPN in representative fields. Five images per sample were counted and averaged.
Results
To quantify the OPN protein expression, immunofluorescence was performed in paraffin-embedded sections of myocardial biopsies obtained from patients with different conditions (described in Table 1). All myocardial tissues in addition to two control kidney tissues were stained for OPN. As expected, the pediatric diseased kidney had a very high expression of OPN in the renal tubules (Figure 1A) and the adult healthy kidney had a moderate expression of OPN in the renal tubules (Figure 1B). All myocardial tissues were additionally co-stained with the myocyte markers ACTA1 and MLC2 to unequivocally identify the OPN positive cardiomyocytes.
From the 14 myocardial tissues analyzed, the three pediatric hearts with sepsis and/or pneumonia, showed the highest expression of OPN (Figures 2, 3, 5). Except for the heart from the old patient with sepsis and several severe coronary occlusions (Figure 4), all the other hearts from the pediatric patients and the mid-age and old patients had barely had any OPN expression in the cardiomyocytes (Figures 6–9). The microscopic images (Figures 1–9) are presented in the order of high to low expression of OPN. The quantification of the number of OPN positive cardiomyocytes in each of the fourteen myocardial tissues and in the three groups (pediatric with or without sepsis/pneumonia and elderly with sepsis/pneumonia) is shown in Figure 10.
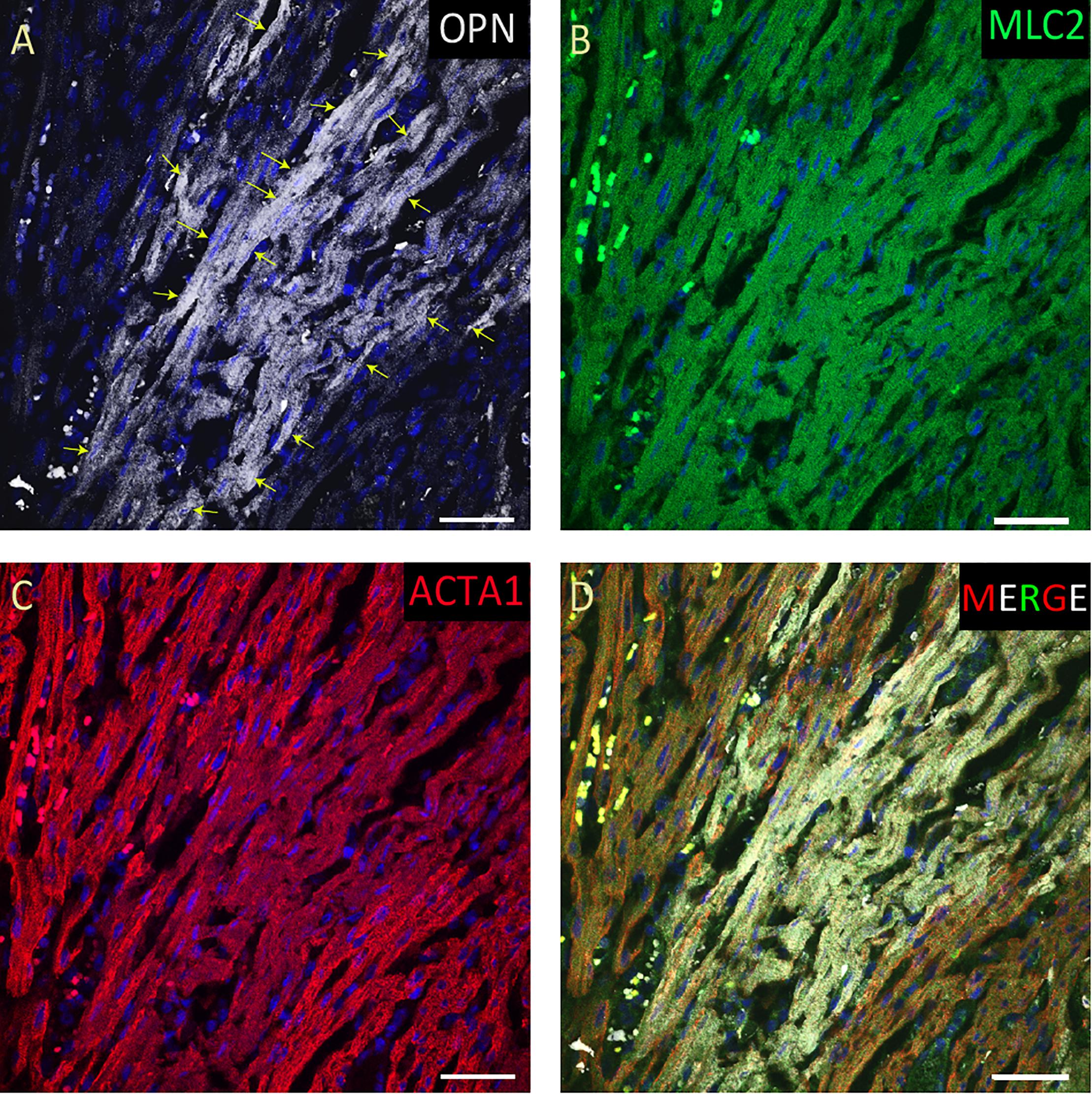
Figure 2. OPN expression is high in the cardiomyocytes from the pediatric patient with sepsis and pneumonia. In the pediatric patient with sepsis and pneumonia, OPN (white in A) is highly expressed in MLC (green in B), and ACTA1 (red in C) positive cardiomyocytes (arrows). (D) is the merged panel. Scale bar = 50 μm.
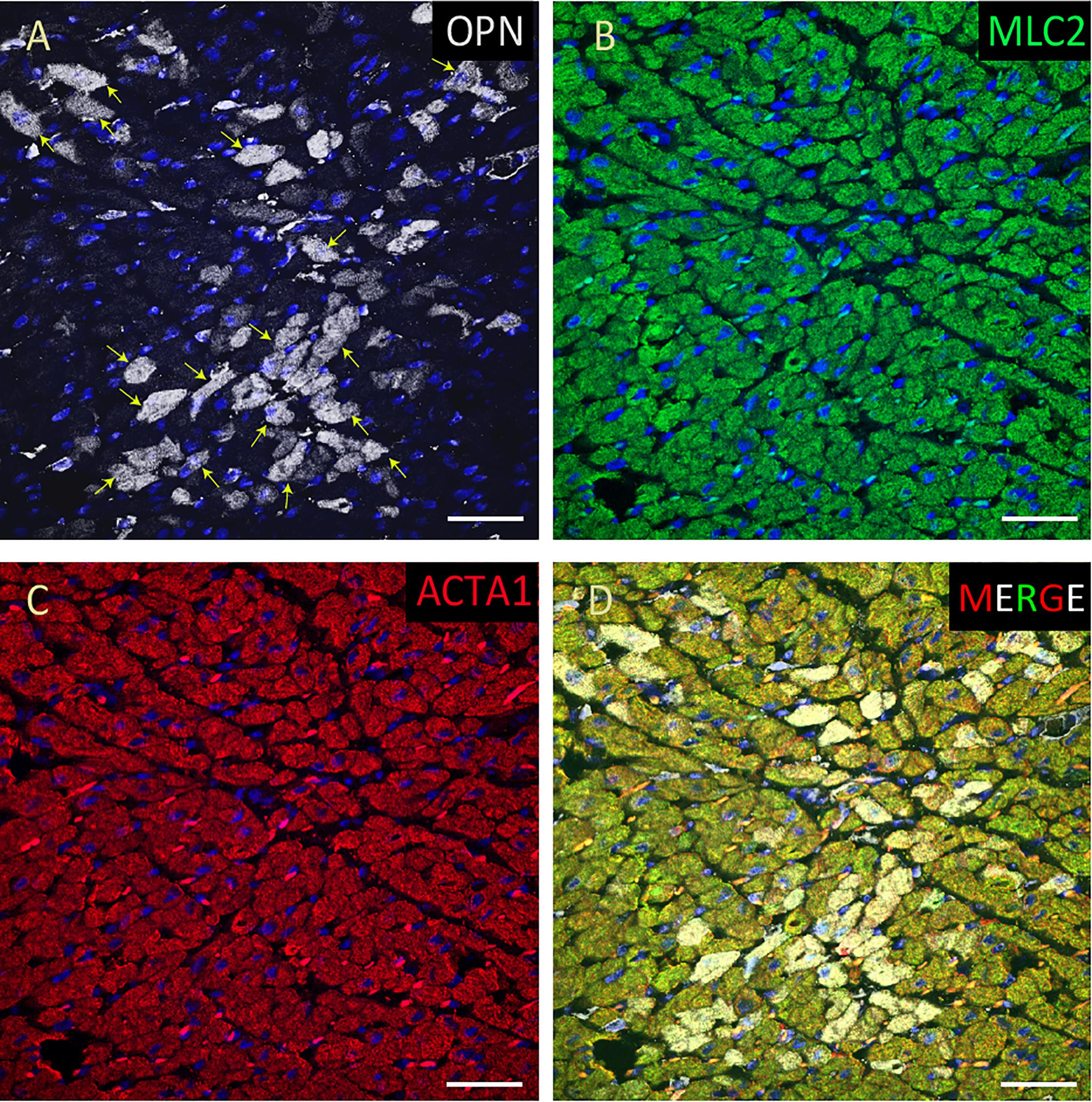
Figure 3. OPN expression is high in the cardiomyocytes from the pediatric patient with fungal pneumonia. In the pediatric patient with fungal pneumonia, OPN (white in A) is highly expressed in MLC (green in B), and ACTA1 (red in C) positive cardiomyocytes (arrows). (D) is the merged panel. Scale bar = 50 μm.
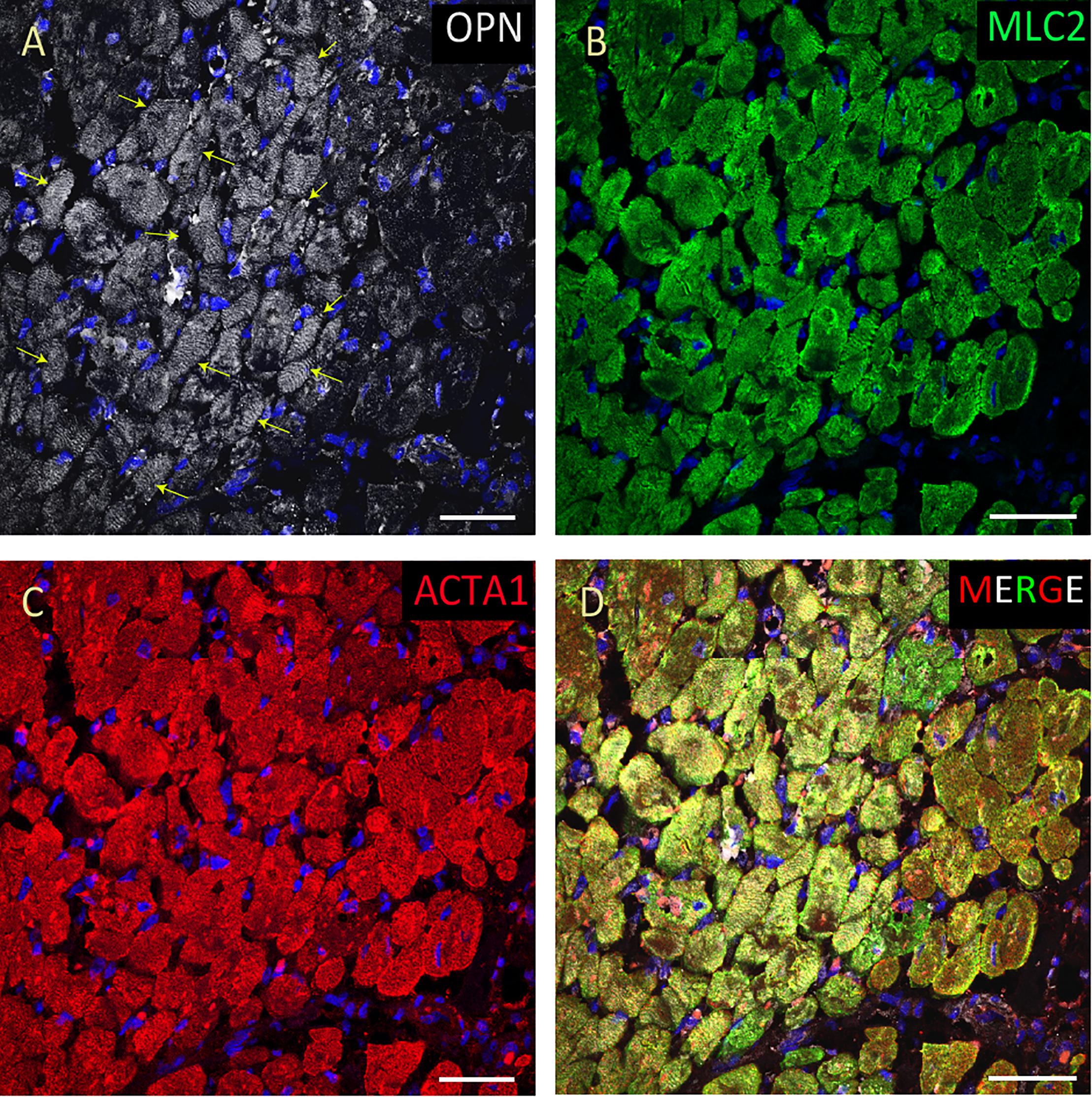
Figure 4. OPN expression is increased in the cardiomyocytes from the elderly patient with septic shock and coronary artery disease. In the elderly patient with septic shock and >75% occlusion in the left anterior descending (LAD) artery and 100% occlusion in the right circumflex artery (RCA), OPN (white in A) is highly expressed in MLC (green in B), and ACTA1 (red in C) positive cardiomyocytes (arrows). (D) is the merged panel. Scale bar = 50 μm.
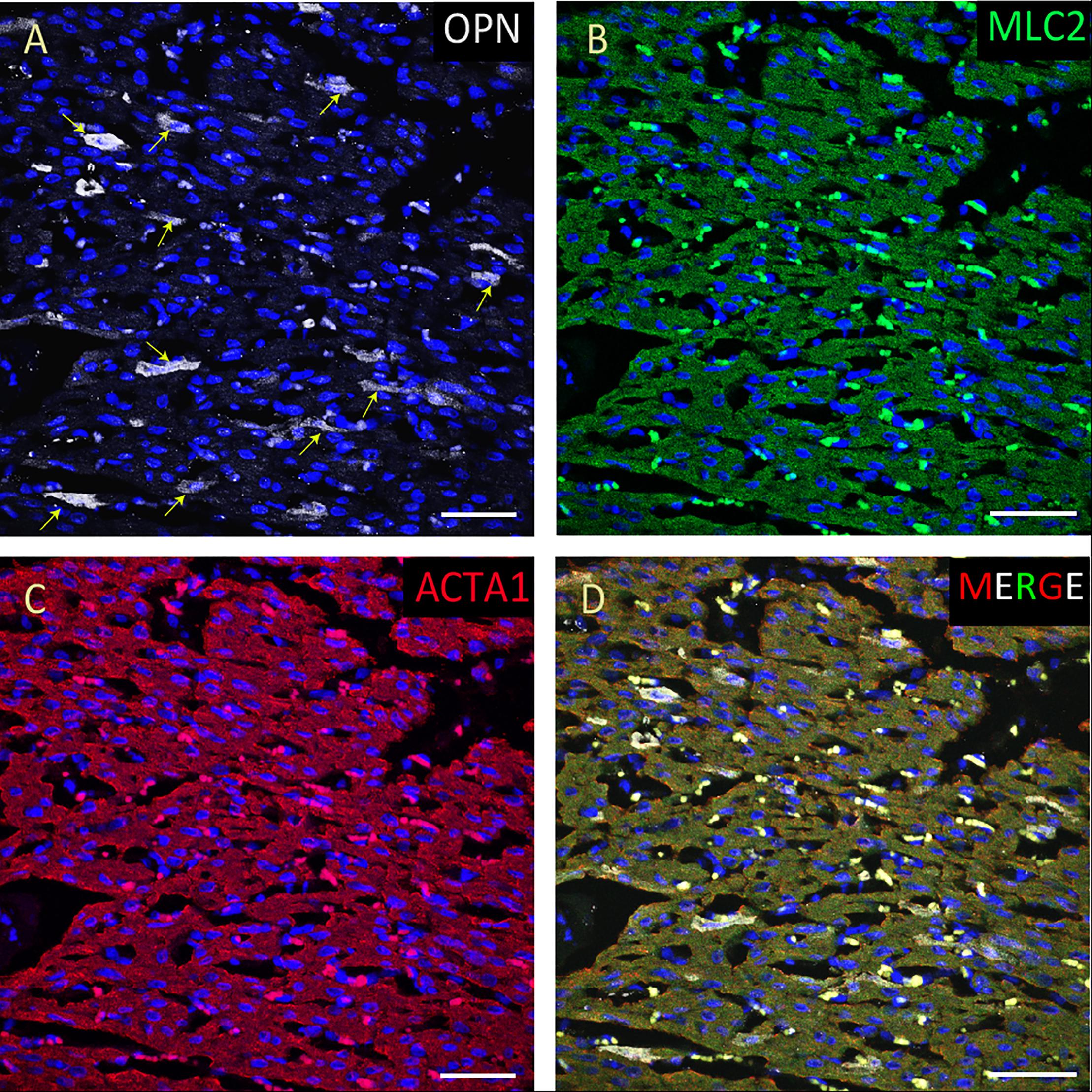
Figure 5. OPN expression is high in the cardiomyocytes from the pediatric patient with sepsis. In the pediatric patient with sepsis, OPN (white in A) is highly expressed in MLC (green in B), and ACTA1 (red in C) positive cardiomyocytes (arrows). (D) is the merged panel. Scale bar = 50 μm.
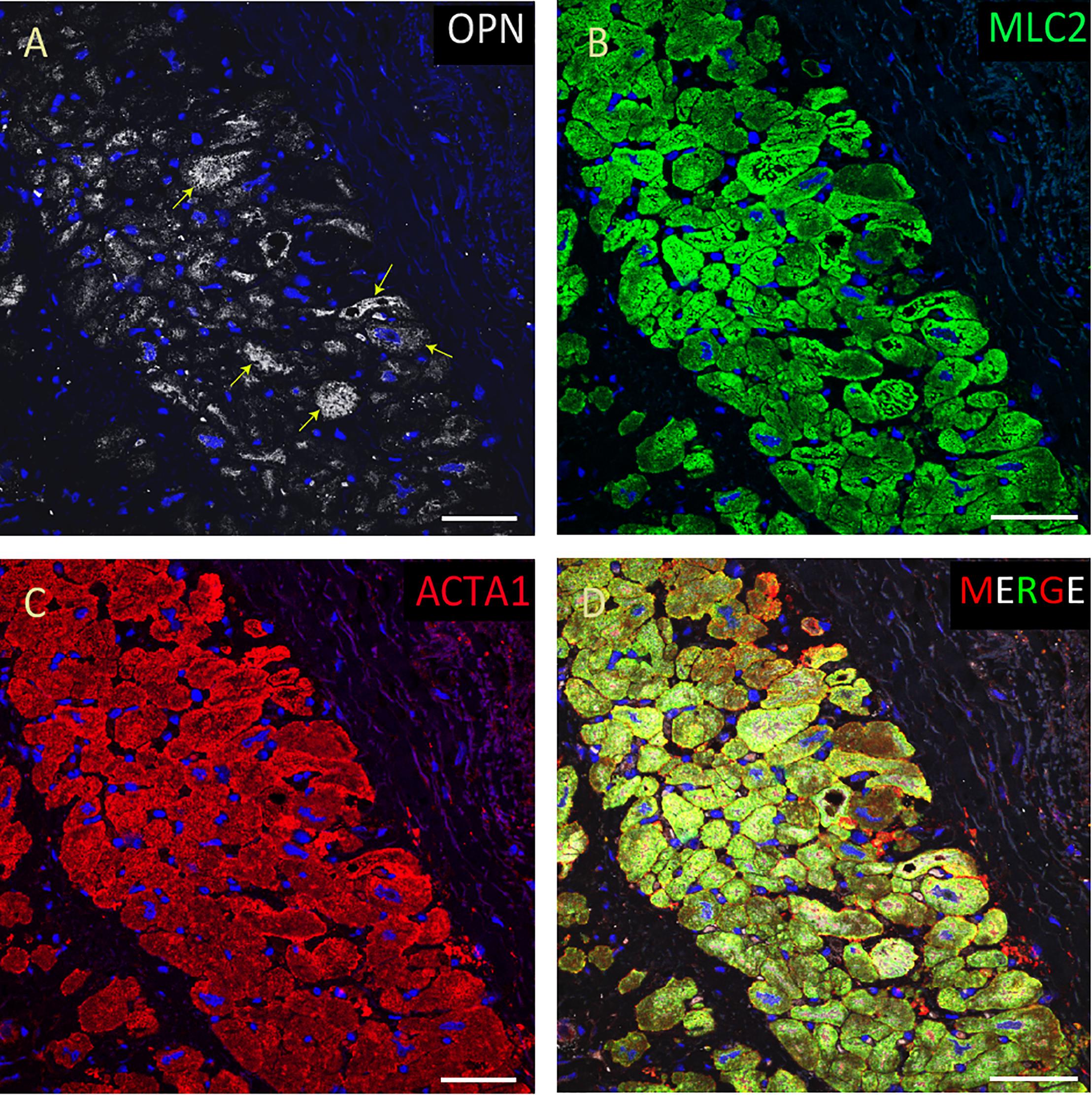
Figure 6. OPN expression is minimal in the cardiomyocytes from an elderly patient with left ventricular hypertrophy and papillary muscle infarct. In the elderly patient with left ventricular hypertrophy and papillary muscle infarct, OPN (white in A) is expressed in only a few number of MLC (green in C), and ACTA1 (red in C) positive cardiomyocytes (arrows). (D) is the merged panel. Scale bar = 50 μm.
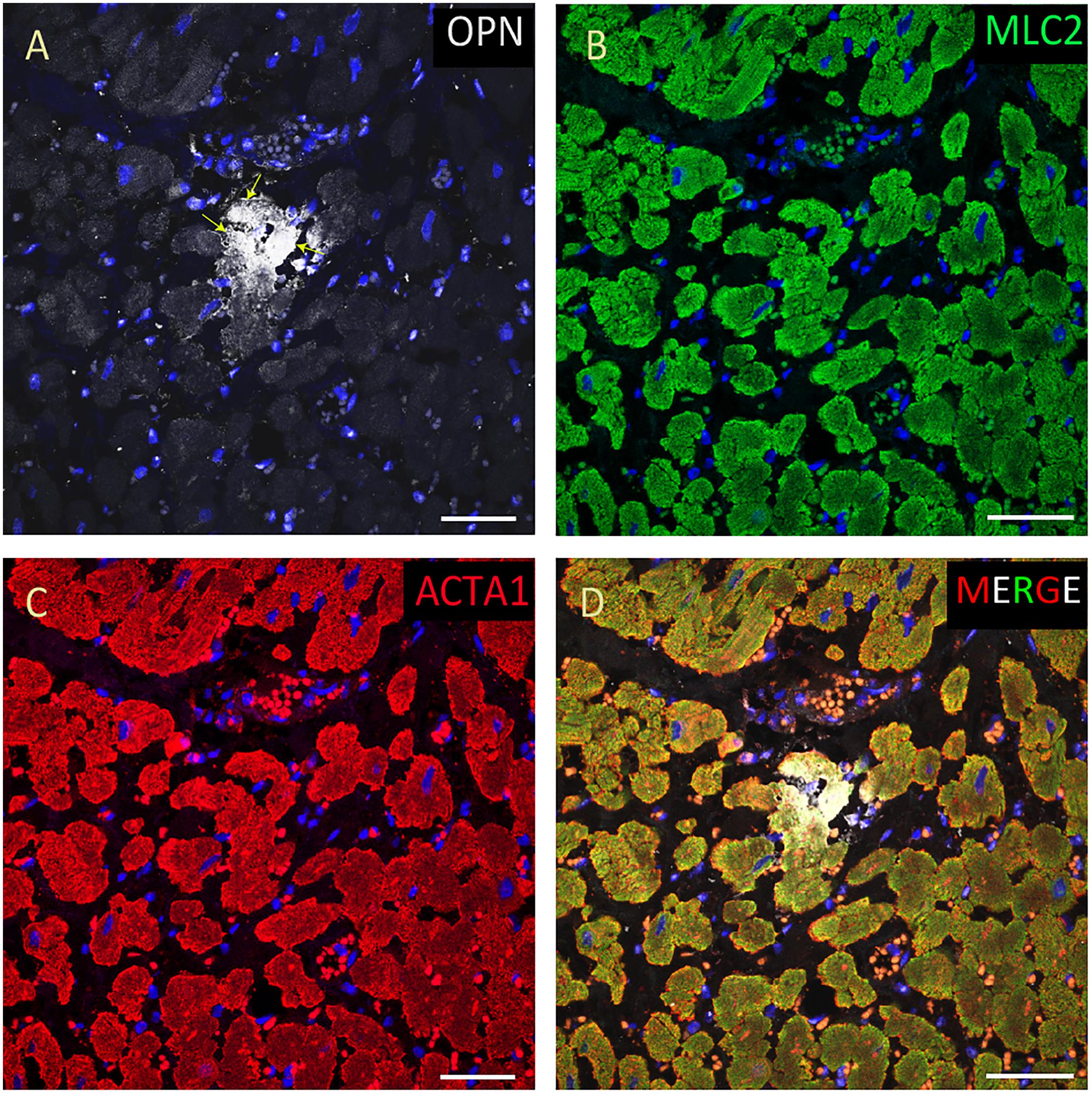
Figure 7. OPN expression is minimal in the cardiomyocytes from an elderly patient. In the elderly patient with sepsis and pneumonia, OPN (white in A) is expressed in only a few number of MLC (green in B), and ACTA1 (red in C) positive cardiomyocytes (arrows). (D) is the merged panel. Scale bar = 50 μm.
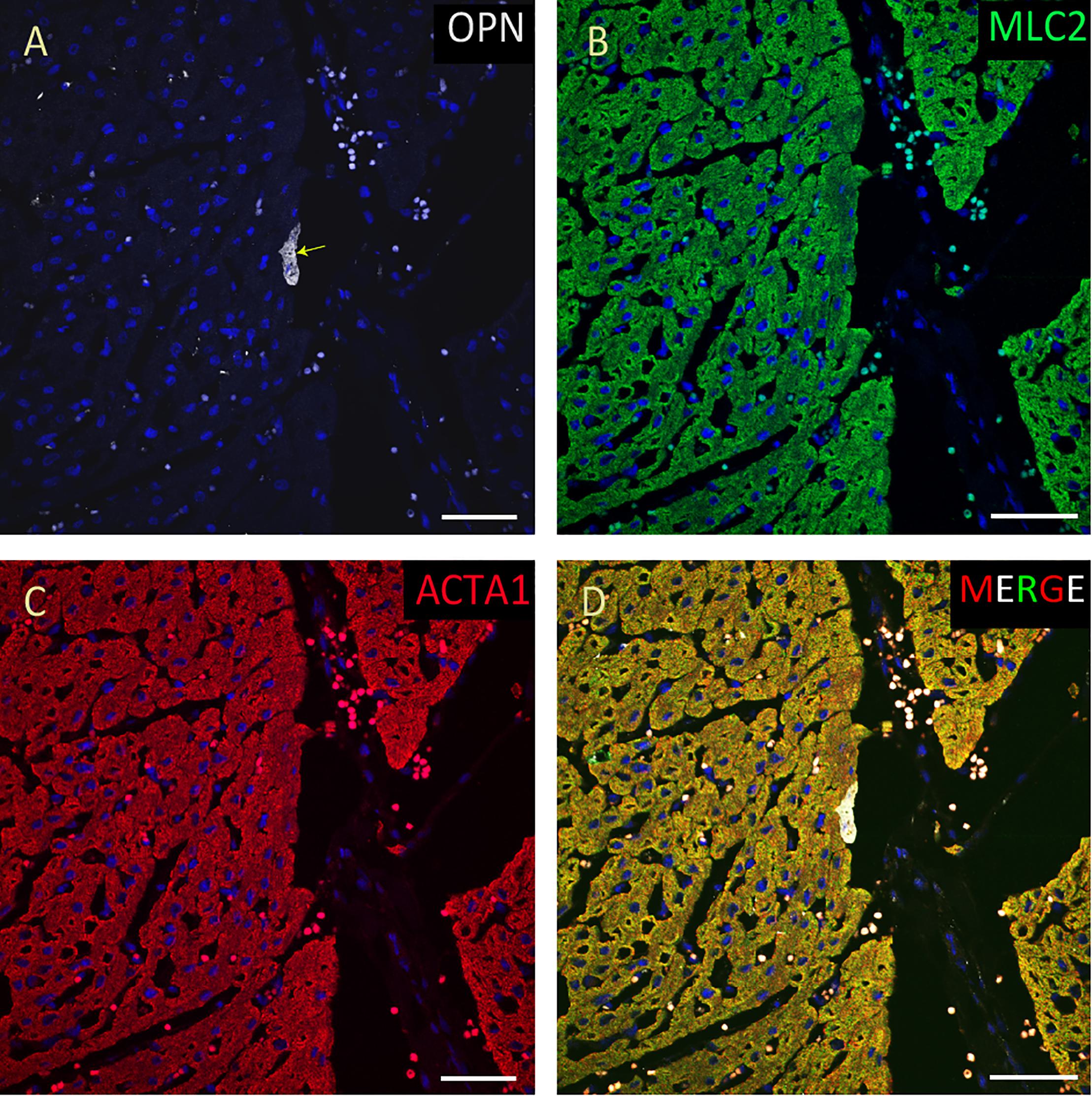
Figure 8. OPN expression is minimal in the cardiomyocytes from a pediatric patient without any infection. In the pediatric patient with ompholocele and without any infection, OPN (white in A) is barely expressed in a few number of MLC (green in B), and ACTA1 (red in C) positive cardiomyocytes (arrow). (D) is the merged panel. Scale bar = 50 μm.
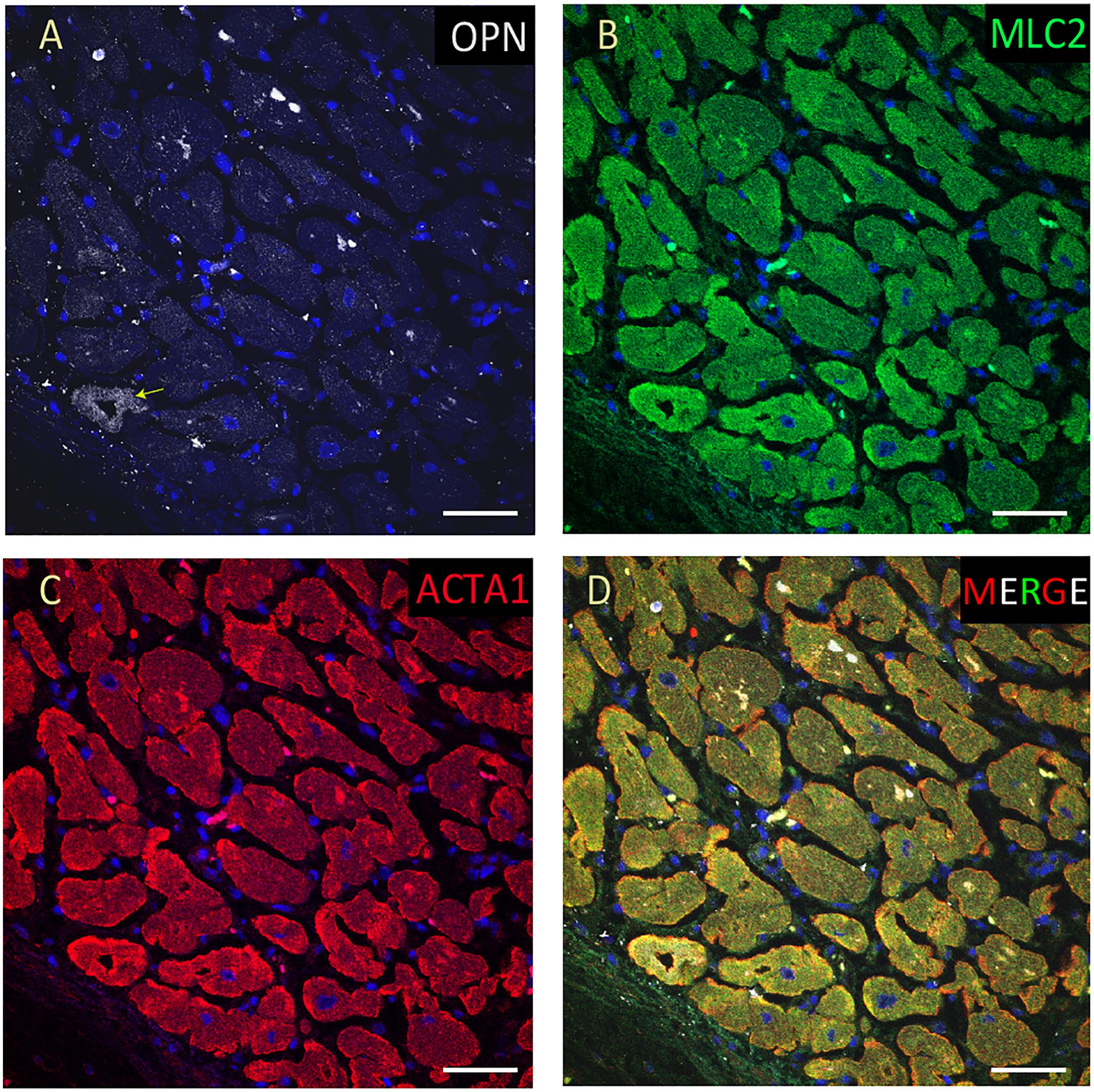
Figure 9. OPN expression is barely detected in the cardiomyocytes from an elderly patient with hypertension and sepsis. In the adult patient with sepsis and hypertension, OPN (white in A) is barely expressed in a few number of MLC (green in B), and ACTA1 (red in C) positive cardiomyocytes (arrow). (D) is the merged panel. Scale bar = 50 μm.
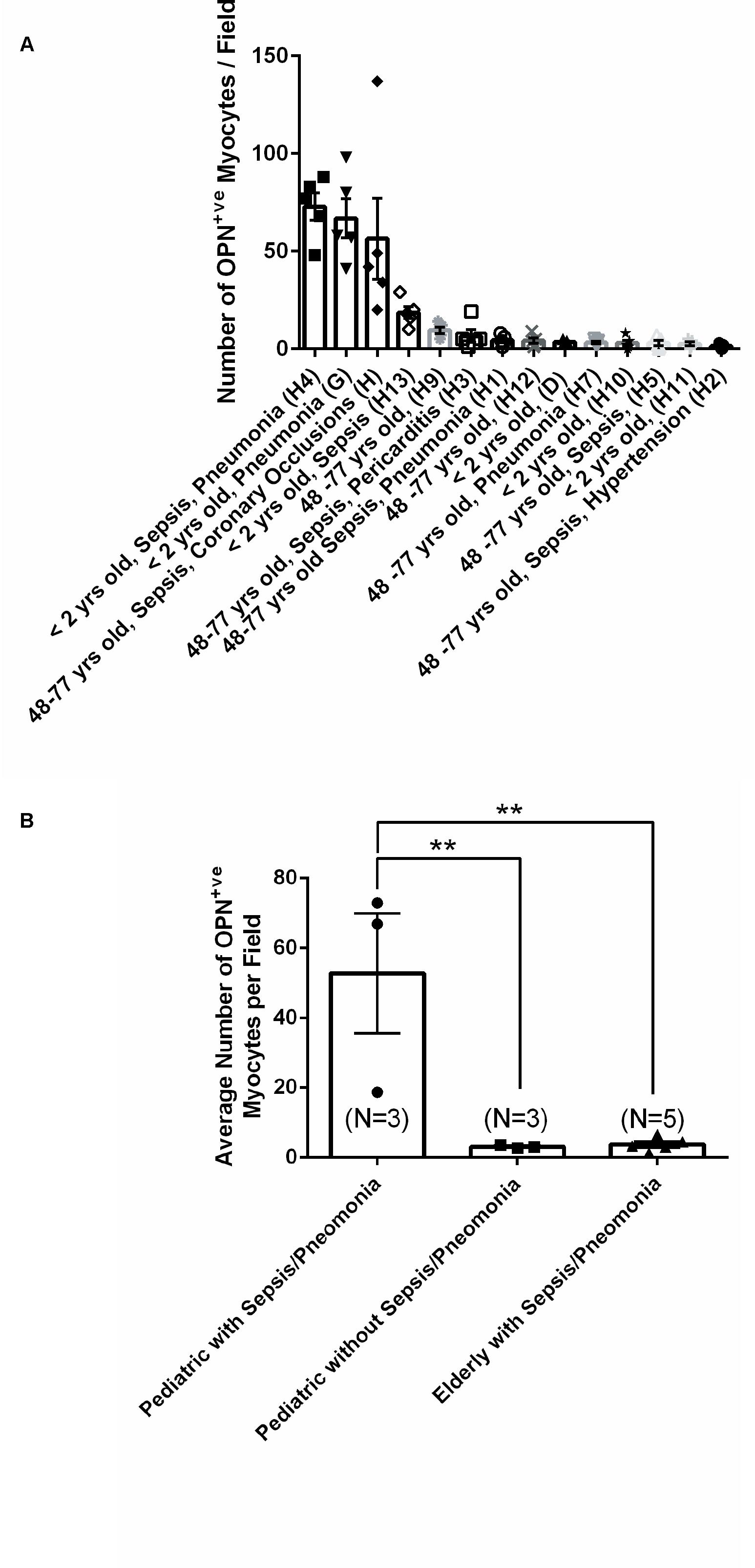
Figure 10. Quantification of the number of OPN positive cardiomyocytes in each of the fourteen myocardial specimens. (A) A quantitative analysis was performed to manually count the number of ACTA1 and MLC2 positive cardiomyocytes that express OPN in representative fields. Five images per sample were counted and averaged. (B) Statistical analysis was performed on the three main groups. N = 3–5 patients per group. ∗∗P < 0.001 using ANOVA with Tukey multiple correction.
Discussion
Our study shows that OPN expression was increased in cardiomyocytes of pediatric patients with sepsis or pneumonia but not in those of elderly patients without evidence of comorbid Coronary Artery Disease. According to the Center for Disease Control and Prevention, in the United States 270,000 people die from sepsis each year (Rhee et al., 2017). For newborns sepsis was the seventh leading cause of infant death in 2016 (Kochanek et al., 2017), followed by influenza and pneumonia (Kochanek et al., 2017). In sepsis, respiratory tract infections, particularly pneumonia, are the most common and associated with the highest mortality (Mayr et al., 2014). Both sepsis and pneumonia have been reported to induce heart dysfunction (Merx and Weber, 2007; Rudiger and Singer, 2007, 2013; Corrales-Medina et al., 2011; Brown et al., 2014).
Myocardial dysfunction during sepsis and pneumonia has been variously associated with increased circulating cytokines and prostanoids, endothelin upregulation, impaired oxygen utilization, and increased levels of C-reactive protein (Kumar et al., 1996; Merx and Weber, 2007; Musher et al., 2007; Hunter and Doddi, 2010; Singanayagam et al., 2012). Increased OPN levels in plasma have also been reported (Kadota et al., 2005; Vaschetto et al., 2008), but the corresponding levels in cardiac tissues have not been investigated. OPN is overexpressed in the heart coincident with myocardial infarction (Trueblood et al., 2001), hypertrophy (Graf et al., 1997; Li et al., 2017), and myocarditis (Szalay et al., 2009) in a cell type- and stimulant-dependent manner (Singh et al., 2010). Mechanistically, interleukin-1β combined with interferon–γ (IFN-γ) increase OPN expression in cardiac microvascular endothelial cells (Singh et al., 1995), while angiotensin II alone or combined with TNF-α or interleukin-1β increase OPN gene expression in cardiac fibroblast (Xie et al., 2004), and glucocorticoid hormone can increase the expression of OPN in cardiomyocytes and cardiac microvascular endothelial cells (Singh et al., 1995).
Graf et al. (1997) showed that endothelin-1 and norepinephrine induce OPN expression in cultured rat neonatal cardiac myocytes. Interestingly high levels of circulating endothelin-1 have also been linked with sepsis (Bocking et al., 1979; Shindo et al., 1998; Rudiger and Singer, 2007), providing a possible explanation for our results in the pediatric specimens. In our studies the highest cardiomyocyte OPN levels were found in patient (H4), a baby born prematurely who died due to necrotizing enterocolitis. The subject had edematous heart muscle due sepsis and multi-organ failure, and patient (G), a child with fungal pneumonia. In both subjects the increased cardiomyocyte OPN expression may be linked with coincident inflammation. Our results are consistent with a central role for infection/inflammation in the activation of OPN expression in the pediatric heart. Notably, the children with full term ompholocele, holoprosencephaly, or premature birth, but no infection, showed only low-level cardiomyocyte OPN.
Interestingly, in the hearts of the older patients with sepsis, few cardiomyocytes expressed OPN. Among these, only the heart with severe coronary arterial occlusions showed high levels of cardiomyocyte OPN, consistent with the known link between Coronary Artery Disease, myocardial infarction, and OPN (Trueblood et al., 2001). It seems likely that age is a determining factor in the possible association between sepsis and cardiac OPN. Consistent with this, Luce et al. (2007) reported that cytokine responses differ widely between neonates and adults. Paliwal et al. (2012) reported higher OPN expression in macrophages of aged mice with skeletal muscle injury. Conversely, Schultz et al. (2002, 2004) reported exaggerated inflammatory responses in term and preterm infants compared to adults with attenuation of the pro-inflammatory reaction during infection that can lead to severe organ dysfunction in infants. Developing neonatal cardiomyocyte also differ from adults and there may be different responses to sepsis and inflammation (Luce et al., 2007).
Patients with pneumococcal pneumonia are at increased risk for concurrent acute cardiac events (Musher et al., 2007). Increased levels of inflammatory cytokines, C-reactive protein, and fibrinogen, along with increased myocardial demand for oxygen and lowered blood oxygen levels, are among the factors that have been reported to lead to cardiac dysfunction in patients with bacterial pneumonia. Based on our findings, increased OPN level may contribute to these pneumonia-caused cardiac events. However, further studies with larger sample sizes are needed to confirm relationships between cardiomyocyte OPN, inflammation, and age.
Conclusion
In this study, we report markedly increased levels of OPN in cardiomyocytes of pediatric patients with sepsis or sepsis and pneumonia. Both sepsis and pneumonia involve activation of the immune system, inflammatory responses followed by cardiac dysfunction. This is the first study to report such increased cardiomyocyte OPN expression in pediatric sepsis or pneumonia. Our findings highlight a potentially important role for OPN in sepsis- or pneumonia-mediated cardiac dysfunction in pediatric patients.
Ethics Statement
This study was carried out in accordance with the recommendations of the University of Miami Institutional Review Board (IRB protocol # 20180439). The study was exempt because the samples used were all autopsy samples.
Author Contributions
CI, KP, and KJ-W performed the experiments. CI and KP analyzed the results. SG provided the pathology samples, clinical information, and data interpretation. CI and LS wrote the manuscript.
Funding
This work was supported by the following grants to LS: National Institute of Health (R56HL132209 and 1R01HL140468) and the Miami Heart Research Institute.
Conflict of Interest Statement
The authors declare that the research was conducted in the absence of any commercial or financial relationships that could be construed as a potential conflict of interest.
References
Ashizawa, N., Graf, K., Do, Y. S., Nunohiro, T., Giachelli, C. M., Meehan, W. P., et al. (1996). Osteopontin is produced by rat cardiac fibroblasts and mediates A(II)-induced DNA synthesis and collagen gel contraction. J. Clin. Invest. 98, 2218–2227. doi: 10.1172/Jci119031
Bocking, J. K., Sibbald, W. J., Holliday, R. L., Scott, S., and Viidik, T. (1979). Plasma catecholamine levels and pulmonary dysfunction in sepsis. Surg. Gynecol. Obstet. 148, 715–719.
Brown, A. O., Mann, B., Gao, G., Hankins, J. S., Humann, J., Giardina, J., et al. (2014). Streptococcus pneumoniae translocates into the myocardium and forms unique microlesions that disrupt cardiac function. PLoS Pathog. 10:e1004383. doi: 10.1371/journal.ppat.1004383
Chang, J. H., Hung, W. Y., Bai, K. J., Yang, S. F., and Chien, M. H. (2016). Utility of plasma osteopontin levels in management of community-acquired pneumonia. Int. J. Med. Sci. 13, 673–679. doi: 10.7150/ijms.16175
Cobbs, A., Ballou, K., Chen, X., George, J., and Zhao, X. (2018). Saturated fatty acids bound to albumin enhance osteopontin expression and cleavage in renal proximal tubular cells. Int. J. Physiol. Pathophysiol. Pharmacol. 10, 29–38.
Corrales-Medina, V. F., Musher, D. M., Wells, G. A., Chirinos, J. A., Chen, L., and Fine, M. J. (2012). Cardiac complications in patients with community-acquired pneumonia: incidence, timing, risk factors, and association with short-term mortality. Circulation 125, 773–781. doi: 10.1161/CIRCULATIONAHA.111.040766
Corrales-Medina, V. F., Suh, K. N., Rose, G., Chirinos, J. A., Doucette, S., Cameron, D. W., et al. (2011). Cardiac complications in patients with community-acquired pneumonia: a systematic review and meta-analysis of observational studies. PLoS Med. 8:e1001048. doi: 10.1371/journal.pmed.1001048
Dal-Secco, D., DalBó, S., Lautherbach, N. E. S., Gava, F. N., Celes, M. R. N., Benedet, P. O., et al. (2017). Cardiac hyporesponsiveness in severe sepsis is associated with nitric oxide-dependent activation of G protein receptor kinase. Am. J. Physiol. Heart Circ. Physiol. 313, H149–H163. doi: 10.1152/ajpheart.00052.2016
Dhainaut, J. F., Lanore, J. J., de Gournay, J. M., Huyghebaert, M. F., Brunet, F., Villemant, D., et al. (1988). Right ventricular dysfunction in patients with septic shock. Intensive Care Med. 14(Suppl. 2), 488–491. doi: 10.1007/BF00256967
Ding, W., Yousefi, K., Goncalves, S., Goldstein, B. J., Sabater, A. L., Kloosterboer, A., et al. (2018). Osteopontin deficiency ameliorates Alport pathology by preventing tubular metabolic deficits. JCI Insight doi: 10.1172/jci.insight.94818 [Epub ahead of print].
Fortis, S., Khadaroo, R. G., Haitsma, J. J., and Zhang, H. (2015). Osteopontin is associated with inflammation and mortality in a mouse model of polymicrobial sepsis. Acta Anaesthesiol. Scand. 59, 170–175. doi: 10.1111/aas.12422
Graf, K., Do, Y. S., Ashizawa, N., Meehan, W. P., Giachelli, C. M., Marboe, C. C., et al. (1997). Myocardial osteopontin expression is associated with left ventricular hypertrophy. Circulation 96, 3063–3071. doi: 10.1161/01.CIR.96.9.3063
Hunter, J. D., and Doddi, M. (2010). Sepsis and the heart. Br. J. Anaesth. 104, 3–11. doi: 10.1093/bja/aep339
Icer, M. A., and Gezmen-Karadag, M. (2018). The multiple functions and mechanisms of osteopontin. Clin Biochem. 59, 17–24. doi: 10.1016/j.clinbiochem.2018.07.003
Jardin, F., Fourme, T., Page, B., Loubieres, Y., Vieillard-Baron, A., Beauchet, A., et al. (1999). Persistent preload defect in severe sepsis despite fluid loading: a longitudinal echocardiographic study in patients with septic shock. Chest 116, 1354–1359. doi: 10.1378/chest.116.5.1354
Jin, X., Fu, G.-X., Li, X.-D., Zhu, D.-L., and Gao, P.-J. (2011). Expression and Function of Osteopontin in Vascular Adventitial Fibroblasts and Pathological Vascular Remodeling. PLoS One 6:e23558. doi: 10.1371/journal.pone.0023558
Kadota, J., Mizunoe, S., Mito, K., Mukae, H., Yoshioka, S., Kawakami, K., et al. (2005). High plasma concentrations of osteopontin in patients with interstitial pneumonia. Respir Med. 99, 111–117. doi: 10.1016/j.rmed.2004.04.018
Kato, A., Okura, T., Hamada, C., Miyoshi, S., Katayama, H., Higaki, J., et al. (2014). Cell Stress Induces Upregulation of Osteopontin via the ERK Pathway in Type II Alveolar Epithelial Cells. PLoS One 9:e100106. doi: 10.1371/journal.pone.0100106
Khadour, F. H., Panas, D., Ferdinandy, P., Schulze, C., Csont, T., Lalu, M. M., et al. (2002). Enhanced NO and superoxide generation in dysfunctional hearts from endotoxemic rats. Am. J. Physiol. Heart Circ. Physiol. 283, H1108–H1115. doi: 10.1152/ajpheart.00549.2001
Kochanek, K. D., Murphy, S., Xu, J., and Arias, E. (2017). Mortality in the United States, 2016. NCHS Data Brief 293, 1–8.
Kumar, A., Thota, V., Dee, L., Olson, J., Uretz, E., and Parrillo, J. E. (1996). Tumor necrosis factor alpha and interleukin 1beta are responsible for in vitro myocardial cell depression induced by human septic shock serum. J. Exp. Med. 183, 949–958. doi: 10.1084/jem.183.3.949
Landesberg, G., Gilon, D., Meroz, Y., Georgieva, M., Levin, P. D., Goodman, S., et al. (2012). Diastolic dysfunction and mortality in severe sepsis and septic shock. Eur. Heart J. 33, 895–903. doi: 10.1093/eurheartj/ehr351
le Roux, D. M., and Zar, H. J. (2017). Community-acquired pneumonia in children - a changing spectrum of disease. Pediatr. Radiol. 47, 1392–1398. doi: 10.1007/s00247-017-3827-8
Leibovici, L., Gafter-Gvili, A., Paul, M., Almanasreh, N., Tacconelli, E., Andreassen, S., et al. (2007). Relative tachycardia in patients with sepsis: an independent risk factor for mortality. QJM 100, 629–634. doi: 10.1093/qjmed/hcm074
Li, J., Yousefi, K., Ding, W., Singh, J., and Shehadeh, L. A. (2017). Osteopontin RNA aptamer can prevent and reverse pressure overload-induced heart failure. Cardiovasc. Res. 113, 633–643. doi: 10.1093/cvr/cvx016
Liu, Y. C., Yu, M. M., Shou, S. T., and Chai, Y. F. (2017). Sepsis-induced cardiomyopathy: mechanisms and treatments. Front. Immunol. 8:1021. doi: 10.3389/fimmu.2017.01021
Lopez, B., Gonzalez, A., Lindner, D., Westermann, D., Ravassa, S., Beaumont, J., et al. (2013). Osteopontin-mediated myocardial fibrosis in heart failure: a role for lysyl oxidase? Cardiovasc. Res. 99, 111–120. doi: 10.1093/cvr/cvt100
Lorenzen, J. M., Schauerte, C., Hubner, A., Kolling, M., Martino, F., Scherf, K., et al. (2015). Osteopontin is indispensible for AP1-mediated angiotensin II-related miR-21 transcription during cardiac fibrosis. Eur. Heart J. 36, 2184–2196. doi: 10.1093/eurheartj/ehv109
Luce, W. A., Hoffman, T. M., and Bauer, J. A. (2007). Bench-to-bedside review: developmental influences on the mechanisms, treatment and outcomes of cardiovascular dysfunction in neonatal versus adult sepsis. Crit. Care 11:228. doi: 10.1186/cc6091
Lund, S. A., Giachelli, C. M., and Scatena, M. (2009). The role of osteopontin in inflammatory processes. J. Cell Commun. Signal. 3, 311–322. doi: 10.1007/s12079-009-0068-0
Matkovich, S. J., Al Khiami, B., Efimov, I. R., Evans, S., Vader, J., Jain, A., et al. (2017). Widespread down-regulation of cardiac mitochondrial and sarcomeric genes in patients with sepsis. Crit. Care Med. 45, 407–414. doi: 10.1097/CCM.0000000000002207
Mattila, J. T., Fine, M. J., Limper, A. H., Murray, P. R., Chen, B. B., and Lin, P. L. (2014). Pneumonia. Treatment and diagnosis. Ann. Am. Thorac. Soc. 11(Suppl. 4), S189–S192. doi: 10.1513/AnnalsATS.201401-027PL
Mayr, F. B., Yende, S., and Angus, D. C. (2014). Epidemiology of severe sepsis. Virulence 5, 4–11. doi: 10.4161/viru.27372
Merx, M. W., and Weber, C. (2007). Sepsis and the heart. Circulation 116, 793–802. doi: 10.1161/CIRCULATIONAHA.106.678359
Musher, D. M., Rueda, A. M., Kaka, A. S., and Mapara, S. M. (2007). The association between pneumococcal pneumonia and acute cardiac events. Clin. Infect. Dis. 45, 158–165. doi: 10.1086/518849
Paliwal, P., Pishesha, N., Wijaya, D., and Conboy, I. M. (2012). Age dependent increase in the levels of osteopontin inhibits skeletal muscle regeneration. Aging 4, 553–566. doi: 10.18632/aging.100477
Parker, M. M., McCarthy, K. E., Ognibene, F. P., and Parrillo, J. E. (1990). Right ventricular dysfunction and dilatation, similar to left ventricular changes, characterize the cardiac depression of septic shock in humans. Chest 97, 126–131. doi: 10.1378/chest.97.1.126
Poelaert, J., Declerck, C., Vogelaers, D., Colardyn, F., and Visser, C. A. (1997). Left ventricular systolic and diastolic function in septic shock. Intensive Care Med. 23, 553–560. doi: 10.1007/s001340050372
Qing, C., Peishun, S., Liying, Z., Chunliang, X., Chunxing, Z., Yanyan, H., et al. (2014). An osteopontin-integrin interaction plays a critical role in directing adipogenesis and osteogenesis by mesenchymal stem cells. Stem Cells 32, 327–337. doi: 10.1002/stem.1567
Rhee, C., Dantes, R., Epstein, L., Murphy, D. J., Seymour, C. W., Iwashyna, T. J., et al. (2017). Incidence and Trends of Sepsis in US Hospitals Using Clinical vs Claims Data, 2009-2014. JAMA 318, 1241–1249. doi: 10.1001/jama.2017.13836
Rudiger, A., and Singer, M. (2007). Mechanisms of sepsis-induced cardiac dysfunction. Crit. Care Med. 35, 1599–1608. doi: 10.1097/01.CCM.0000266683.64081.02
Rudiger, A., and Singer, M. (2013). The heart in sepsis: from basic mechanisms to clinical management. Curr. Vasc. Pharmacol. 11, 187–195.
Schultz, C., Rott, C., Temming, P., Schlenke, P., Möller, J. C., and Bucsky, P. (2002). Enhanced interleukin-6 and interleukin-8 synthesis in term and preterm infants. Pediatr. Res. 51:317. doi: 10.1203/00006450-200203000-00009
Schultz, C., Temming, P., Bucsky, P., GÖPel, W., Strunk, T., and HÄRtel, C. (2004). Immature anti-inflammatory response in neonates. Clin. Exp. Immunol. 135, 130–136. doi: 10.1111/j.1365-2249.2004.02313.x
Shindo, T., Kurihara, H., Kurihara, Y., Morita, H., and Yazaki, Y. (1998). Upregulation of endothelin-1 and adrenomedullin gene expression in the mouse endotoxin shock model. J. Cardiovasc. Pharmacol. 31(Suppl. 1), S541–S544. doi: 10.1097/00005344-199800001-00156
Singanayagam, A., Singanayagam, A., Elder, D. H., and Chalmers, J. D. (2012). Is community-acquired pneumonia an independent risk factor for cardiovascular disease? Eur. Respir. J. 39, 187–196. doi: 10.1183/09031936.00049111
Singh, K., Balligand, J. L., Fischer, T. A., Smith, T. W., and Kelly, R. A. (1995). Glucocorticoids increase osteopontin expression in cardiac myocytes and microvascular endothelial cells. Role in regulation of inducible nitric oxide synthase. J. Biol. Chem. 270, 28471–28478. doi: 10.1074/jbc.270.47.28471
Singh, M., Dalal, S., and Singh, K. (2014). Osteopontin: at the cross-roads of myocyte survival and myocardial function. Life Sci. 118, 1–6. doi: 10.1016/j.lfs.2014.09.014
Singh, M., Foster, C. R., Dalal, S., and Singh, K. (2010). Osteopontin: role in extracellular matrix deposition and myocardial remodeling post-MI. J. Mol. Cell Cardiol. 48, 538–543. doi: 10.1016/j.yjmcc.2009.06.015
Sodhi, C. P., Phadke, S. A., Batlle, D., and Sahai, A. (2001). Hypoxia stimulates osteopontin expression and proliferation of cultured vascular smooth muscle cells. Potentiation by high glucose. Diabetes 50, 1482–1490. doi: 10.2337/diabetes.50.6.1482
Stawowy, P., Blaschke, F., Pfautsch, P., Goetze, S., Lippek, F., Wollert-Wulf, B., et al. (2002). Increased myocardial expression of osteopontin in patients with advanced heart failure. Eur. J. Heart Fail. 4, 139–146. doi: 10.1016/S1388-9842(01)00237-9
Suffredini, A. F., Fromm, R. E., Parker, M. M., Brenner, M., Kovacs, J. A., Wesley, R. A., et al. (1989). The cardiovascular response of normal humans to the administration of endotoxin. N. Engl. J. Med. 321, 280–287. doi: 10.1056/NEJM198908033210503
Szalay, G., Sauter, M., Haberland, M., Zuegel, U., Steinmeyer, A., Kandolf, R., et al. (2009). Osteopontin: a fibrosis-related marker molecule in cardiac remodeling of enterovirus myocarditis in the susceptible host. Circ. Res. 104, 851–859. doi: 10.1161/CIRCRESAHA.109.193805
Tavener, S. A., Long, E. M., Robbins, S. M., McRae, K. M., Van Remmen, H., and Kubes, P. (2004). Immune cell Toll-like receptor 4 is required for cardiac myocyte impairment during endotoxemia. Circ. Res. 95, 700–707. doi: 10.1161/01.RES.0000144175.70140.8c
Trueblood, N. A., Xie, Z., Communal, C., Sam, F., Ngoy, S., Liaw, L., et al. (2001). Exaggerated left ventricular dilation and reduced collagen deposition after myocardial infarction in mice lacking osteopontin. Circ. Res. 88, 1080–1087. doi: 10.1161/hh1001.090842
van der Windt, G. J., Hoogendijk, A. J., Schouten, M., Hommes, T. J., de Vos, A. F., Florquin, S., et al. (2011). Osteopontin impairs host defense during pneumococcal pneumonia. J. Infect. Dis. 203, 1850–1858. doi: 10.1093/infdis/jir185
Vaschetto, R., Nicola, S., Olivieri, C., Boggio, E., Piccolella, F., Mesturini, R., et al. (2008). Serum levels of osteopontin are increased in SIRS and sepsis. Intensive Care Med. 34, 2176–2184. doi: 10.1007/s00134-008-1268-4
Vincent, J. L., Bakker, J., Marecaux, G., Schandene, L., Kahn, R. J., and Dupont, E. (1992). Administration of anti-TNF antibody improves left ventricular function in septic shock patients. Results of a pilot study. Chest 101, 810–815. doi: 10.1378/chest.101.3.810
Watts, J. A., Kline, J. A., Thornton, L. R., Grattan, R. M., and Brar, S. S. (2004). Metabolic dysfunction and depletion of mitochondria in hearts of septic rats. J. Mol. Cell Cardiol. 36, 141–150. doi: 10.1016/j.yjmcc.2003.10.015
Xie, Z., Singh, M., and Singh, K. (2004). ERK1/2 and JNKs, but not p38 kinase, are involved in reactive oxygen species-mediated induction of osteopontin gene expression by angiotensin II and interleukin-1beta in adult rat cardiac fibroblasts. J. Cell. Physiol. 198, 399–407. doi: 10.1002/jcp.10419
Zhang, Z.-X., Shek, K., Wang, S., Huang, X., Lau, A., Yin, Z., et al. (2010). Osteopontin expressed in tubular epithelial cells regulates NK cell-mediated kidney ischemia reperfusion injury. J. Immunol. 185, 967–973. doi: 10.4049/jimmunol.0903245
Keywords: osteopontin, sepsis, pneumonia, pediatric, heart, cardiomyocyte
Citation: Irion CI, Parrish K, John-Williams K, Gultekin SH and Shehadeh LA (2018) Osteopontin Expression in Cardiomyocytes Is Increased in Pediatric Patients With Sepsis or Pneumonia. Front. Physiol. 9:1779. doi: 10.3389/fphys.2018.01779
Received: 27 September 2018; Accepted: 23 November 2018;
Published: 10 December 2018.
Edited by:
Paras Kumar Mishra, University of Nebraska Medical Center, United StatesReviewed by:
Fadi N. Salloum, Virginia Commonwealth University, United StatesSakthivel Sadayappan, University of Cincinnati, United States
Copyright © 2018 Irion, Parrish, John-Williams, Gultekin and Shehadeh. This is an open-access article distributed under the terms of the Creative Commons Attribution License (CC BY). The use, distribution or reproduction in other forums is permitted, provided the original author(s) and the copyright owner(s) are credited and that the original publication in this journal is cited, in accordance with accepted academic practice. No use, distribution or reproduction is permitted which does not comply with these terms.
*Correspondence: Lina A. Shehadeh, lshehadeh@med.miami.edu