- 1Colegio de Ciencias de la Salud, Universidad San Francisco de Quito, Quito, Ecuador
- 2Facultad de Enfermería, Pontificia Universidad Católica del Ecuador, Quito, Ecuador
- 3Instituto Politécnico Nacional, Durango, Mexico
- 4Facultad de Ciencias Médicas, Universidad Central del Ecuador, Quito, Ecuador
Hypertensive disorders during pregnancy constitute one of the main causes of maternal and perinatal morbidity and mortality across the world and particularly in developing countries such as Ecuador. However, despite its impact on public health, the primary pathophysiological processes involved are yet to be elucidated. It has been proposed, among other theories, that an abnormal placentation may induce an endothelial dysfunction, which is ultimately responsible for the final clinical manifestations. Mitochondria, particularly from trophoblastic cells, are responsible for the production of energy, which is extremely important for normal placentation. The malfunction in this supply of energy may produce higher levels of free radicals. In both production of energy and free radicals, coenzyme Q10 (CoQ10) plays a crucial role in electron transport. As such, the role of CoQ10 in the genesis and prevention of preeclampsia has become the focus of a number of research groups, including that of the authors. Developing an in-depth understanding of these mechanisms might allow us to design new and feasible strategies with which we can reduce preeclampsia, particularly in the Latin-American countries.
Introduction
Maternal mortality remains a significant public health problem in Ecuador (The Maternal Health Study [MNPI], 1999; Centro de Estudios de Población y Desarrollo Social [CEPAR], 2004; Instituto Nacional Ecuatoriano de Estadísticas y Censos [INEC], 2017). The main cause for maternal mortality is preeclampsia – a disease characterized by hypertension, increased vascular resistance, endothelial dysfunction, proteinuria, and coagulopathy – that usually manifests in the second trimester of pregnancy (National High Blood Pressure Education Program Working Group Report on High Blood Pressure in Pregnancy, 1990). Preeclampsia is defined as an increase in blood pressure that is equal to or greater than 140/90 mmHg and 24 h of proteinuria equal to or greater than 300 mg/dl (Solomon and Seely, 2001). The presence of preeclampsia during pregnancy also causes complications to the newborn and is associated with low birth weight, preterm delivery, and neonatal mortality (López-Jaramillo, 1993). However, despite several years of basic research, the genesis and the pathophysiology of preeclampsia still remain unknown (Armaly et al., 2018).
For several years, we have dedicated our research efforts toward acquiring a better understanding of preeclampsia and designing new strategies, concepts, and hypotheses that might allow us to unlock the enigma of the etiology of preeclampsia (Calle and Teran, 2018). In this paper, we describe the evidence that highlights the putative role of abnormalities in placental implantation and, more specifically, in terms of mitochondrial function at the placental level on the genesis of preeclampsia. The effects of these alterations upon the maternal endothelium are discussed elsewhere (Roberts and Redman, 1993; Evora, 2000; Granger et al., 2001; Saito and Nakashima, 2014).
Development of the Placenta During Normal Pregnancy and in Preeclampsia
The placenta is a temporary organ that forms a physical and functional connection between the mother and the embryo/fetus during development. However, in contrast to other organs, the environment in which the placenta develops during the first 8–10 weeks is poor in oxygen; it has been demonstrated that this is essential for blastocyst implantation and further embryo and placental development (Genbacev et al., 1997; Goldman-Wohl and Yagel, 2002).
The trophoblast cells differentiate into the embryo and are derived from the trophectoderm and develop into the blastocyst during development; these cells are essential in maintaining the further development of a normal pregnancy (Genbacev et al., 1997). The trophoblast adheres to the uterus and begins to penetrate into the endometrial stroma. Previous research has shown that proteolytic enzymes such as metalloproteinase act as facilitators in the penetration process by degrading the extracellular matrix (Goldman-Wohl and Yagel, 2002).
The implantation of the blastocyst and primitive placental formation are associated with active penetration of the trophoblast into the endometrial epithelium and adjacent stroma in a low-pressure oxygen environment, which facilitates this process (Genbacev et al., 1997). Subsequently, when the trophoblast is in an environment of high oxygen pressure (Genbacev et al., 1997), it begins the process of differentiation from an early proliferative to an invasive phenotype that will continue to penetrate up to the first third of the myometrium (Zhou et al., 1997b). Interestingly, these cells can also invade the maternal spiraled arteries, which helps to refurbish and replace endothelial and muscular cells (Zhou et al., 1997b). This procedure, known as the conversion, is the replacement of the fetal by maternal endothelium and a loss of elasticity in the arterial vessels. Arteries, then by losing their elasticity, become more compliant, like veins, to increase its capability, according to the demand during pregnancy. In this way, blood flow increases in order to supply nutrients and oxygen to the embryo/fetus during development.
The syncytiotrophoblast is responsible for nutrient exchange, and it is derived from the fusion of the trophoblast cells, which creates an impermeable cellular wallpaper (Goldman-Wohl and Yagel, 2002). In addition to the maintenance of the placental nutrients, the syncytiotrophoblast is also responsible for gaseous exchange as well as the production of hormones and growth factors.
Dependence on oxygen is necessary for normal placental development (De Marco and Caniggia, 2002). The processes underlying the differentiation and the invasion of the trophoblast into the endometrium consume significant amounts of energy (Widschwendter et al., 1998). Based on this requirement, placental development requires an organ acting as an oxygen sensor to modulate energy production. Experimental studies have demonstrated that mitochondria and, more specifically, respiratory chain complexes appear to be responsible for such regulation (De Marco and Caniggia, 2002).
To determine the mechanisms responsible for how and why the placenta and trophoblastic cells can detect oxygen levels may have significant clinical relevance because preeclampsia or the retardation of intrauterine growth involves changes in placentation.
Abnormal Placentation During Preeclampsia
Several studies have shown that an alteration in placental function is linked to the development of preeclampsia. This pathology can occur in pregnancies without a fetus being present, for example, in the condition referred to as mole hydatidiform (Page, 1939) or via poor placentation (Goldman-Wohl and Yagel, 2002) and thus cause an increase in the placental mass, which is the characteristic of gestational diabetes (Solomon and Seely, 2001) or multiple pregnancies (Thornton and Macdonald, 1991). Notably, preeclampsia resolves after delivery (Redman, 1991). Furthermore, histopathological studies have demonstrated morphological changes in vessels from preeclamptic placentas in a process that has come to be known as “endotheliosis” (Gerretsen et al., 1981). A number of experimental studies have analyzed these observations (Gerretsen et al., 1981; Redman, 1991; Colburn et al., 1994; Graham and McCrae, 1996; Lim et al., 1997; Zhou et al., 1997a) and have noted that during preeclampsia, there are two functional abnormalities: first, invasion of the trophoblast to the uterine parenchyma is not deeper and invasion to the vasculature does not reach up to the decidua portion and into the spiral arteries. As a consequence, maternal vessels do not complete the normal physiological changes known as conversion. Consequently, the diameter of the myometrial vessels in preeclampsia is less than half of those in normal pregnancy (Brosens et al., 1972); furthermore, these vessels maintain their responsiveness to vasoconstrictors such as angiotensin or epinephrine (van der Graaf et al., 2013). Second, the number of vessels showing evidence of trophoblastic invasion is reduced in comparison with normal pregnancy (Khong et al., 1986).
However, there has been significant debate over the potential causes of such alterations. An immunological hypothesis targets a potential maternal sensitization process against fetal tissue. It has been shown that longer sexual cohabitation (Dekker and Baha, 2001) and sexual practices that expose the maternal environment to the partner’s semen, for example, oral sex or intercourse without a condom (Koelman et al., 2000), can reduce a women’s risk for the further development of preeclampsia (Saftlas et al., 2014).
In the maternal decidua, there is a significant concentration of leucocytes; of these, the most important are the natural killers (NK cells). During preeclampsia, the trophoblast experiences an increased level of cellular lysis by maternal NK cells (Colburn et al., 1994), which is responsible for an abnormal sensitization process. Therefore, during preeclampsia, there is a reduction in the expression of human leucocyte antigen G (HLA-G) in the invasive trophoblast (Colburn et al., 1994), making these cells more susceptible to attack by NK cells present in the maternal decidua. This process seems to be maintained throughout pregnancy, meaning that trophoblastic cells do not have the resources to support appropriate implantation. In these cases, the production of lysis enzymes in the extracellular matrix from the maternal decidua is abnormal (Lim et al., 1997) and the enzymes produced have structural problems and, therefore, are not able to function appropriately (Graham and McCrae, 1996). Consequently, the trophoblast cannot break down the maternal extracellular matrix and, hence, invasion is not possible.
In addition, during the process of placentation, several factors, such as growth factors and intercellular adhesion molecules, control trophoblast differentiation and also the process of conversion. During preeclampsia, these factors and their functions are often abnormal; thus, trophoblast invasion and conversion are also abnormal (Zhou et al., 1997a; Goldman-Wohl and Yagel, 2002).
Finally, for all these cellular processes, it is known that the trophoblast requires oxygen to produce energy at the mitochondrial level (Genbacev et al., 1997; De Marco and Caniggia, 2002). Several reports have been now published showing an abnormal mitochondrial function in the trophoblast cells during preeclampsia (Furui et al., 1994; Vuorinen et al., 1998; Wang and Walsh, 1998; Roberts and Lían, 2002).
Mitochondria and Preeclampsia
Mitochondria are intracellular organelles responsible for energy production through the respiratory chain in a process that uses oxygen to form adenosine triphosphate (ATP), which is known as oxidative phosphorylation (Davidson, 1994). This process is characterized by the transport of electrons from NADH and FADH2 to the five separate enzymatic complexes in the mitochondrial membrane. Oxygen is the final receptor of electrons at the end of the respiratory chain, where it is ultimately reduced to water. However, during this process, normally 2–3% of oxygen is not completely reduced and leads to the formation of reactive oxygen species (ROS), particularly, superoxide (, 16). Superoxide is mainly generated by coenzyme Q10 (CoQ10) partially reduced – and by complex III in the respiratory chain (Lenaz, 2001). Consequently, superoxide can be converted into hydrogen peroxide by superoxide dismutase in the mitochondria (Wang and Walsh, 1996).
Considering the demand for oxygen and energy required for normal placental development, it is logical to assume that any alterations at this level might compromise the overall process of placentation. Indeed, observational studies have demonstrated a higher incidence of preeclampsia in a family with mitochondrial dysfunction (Torbergsen et al., 1989). In addition, in women with preeclampsia (Folgerø et al., 1996) and their first-degree relatives, there is an abnormality in the expression of mitochondrial genes responsible for energy production, such as cytochrome C oxidase (Matsubara et al., 1997), or electron exchange processes preferentially favor oxidation (Wang and Walsh, 1998). On the contrary, it is well known that hypoxic conditions, for example, living at an altitude, lead to major susceptibility to the development of preeclampsia (Zamudio, 2003). In this sense, there is significant evidence showing that increased generation of ROS plays a key role in the development of preeclampsia (Davidge et al., 1992; Branch et al., 1994; Mikhail et al., 1994; López-Jaramillo et al., 1998; Little and Gladen, 1999; Staff et al., 1999; Sikkema et al., 2001). Reports have also confirmed that the origin of ROS is most likely from the placenta and, in particular, mitochondria (Wang and Walsh, 1998). A previous study reported that the number of mitochondria in placental tissue is far greater in women with preeclampsia and that these mitochondria have greater susceptibility to lipid peroxidation (Wang and Walsh, 1998); however, these observations were made in only a small number of patients (Table 1).
In this sense, CoQ10, the only non-polar electron transporter into the mitochondrial respiratory chain, plays a key role in both production of energy and formation of ROS (Davidson, 1994; Lenaz, 2001). Circulating CoQ10 is known to act as a potent antioxidant, either directly (Kaikkonen et al., 1999) or via the regeneration of vitamin E (Hodson and Watts, 2003). Therefore, it seems logical to suggest that CoQ10 could be involved in the pathogenesis of preeclampsia.
Coenzyme Q10 During Normal Pregnancy and Preeclampsia
The CoQ10 or ubiquinone, is a fat-soluble molecule synthesized endogenously from phenylalanine (benzoquinone ring) and mevalonic acid (responsible for isoprenoid units) and with a small contribution derived from the diet (Davidson, 1994). The CoQ10 participates in energy generation and plays a key role in mitochondrial respiration, as it is responsible for electron transport from complex I and II to complex III (Davidson, 1994). Thus, any alteration in the mitochondrial CoQ10 might result in reduced formation of energy and an increase in generation of ROS (Lenaz, 2001). On the contrary, CoQ10 acts as an antioxidant for lipoproteins, both membrane-bound and circulating (Hodson and Watts, 2003). In the later environment, the function of CoQ10 is related to its capability to increase the bioavailability of vasoactive substances such as nitric oxide (NO; Hodson and Watts, 2003; Teran, 2003).
Only a few studies have investigated CoQ10 during human pregnancy (Noia et al., 1996, 1998). These studies showed that the levels of CoQ10 increase progressively, starting in the first trimester and continue to rise until delivery (Table 2). In 2003, the authors’ group reported that Ecuadorian normal pregnant women, although with recognized nutritional deficiencies, had CoQ10 levels within the normal range as reported for other populations, but higher than non-pregnant women (Teran et al., 2003). However, the authors reported for the first time that women with preeclampsia show a significant reduction in CoQ10 levels (Teran et al., 2003), a result that was later confirmed by other authors (Palan et al., 2004). At that time, our working hypothesis was that during preeclampsia, there may be a “mechanism” that consumes CoQ10 (not related to diet), which might arise because of increased production of ROS. To investigate further this possibility, the author’s group investigated CoQ10 levels in the placenta and the umbilical cord and interestingly found that the levels were significantly higher in women with preeclampsia (Teran et al., 2005), suggesting a compensatory accumulation. However, all those studies were done in Quito, a high-altitude city (2800 m above the sea level). So, in a subsequent study, plasma CoQ10 was measured in Ecuadorian women living at sea level; results showed that normal pregnant women had significantly lower levels of CoQ10, but in those with preeclampsia, the difference was not as evident as in those patients living at high altitude; these observations may be related to the small sample size of this study (Teran et al., 2008). Interestingly, placental content of CoQ10 was also significantly higher in preeclamptic women at sea level (Teran et al., 2008). It was also consistent with a later study showing that CoQ10 levels in mitochondria from placentas of women with preeclampsia were significantly higher compared with mitochondria from normal placentas (Teran et al., 2007; Table 2).
According to our present data, it is likely that women with preeclampsia have already-established alterations in their levels of CoQ10 compared with normal pregnant women; these changes occur both in the plasma and the placenta. However, they occur to a greater extent in the mitochondria. However, all of these previous studies were conducted in women, who had already developed the disease; consequently, it was not possible to determine if a reduction in CoQ10 was a cause or a consequence. With this information, we decided to set up a randomized, double-blinded, and placebo-controlled clinical trial providing 200 mg of CoQ10 daily to 235 pregnant women starting from week 20 up to delivery; we then determined the rate of preeclampsia in this cohort of patients. At the end of the study, there were more patients in the preeclampsia placebo group (25.6% vs. 14.4%), demonstrating, also for the first time, that supplementation of CoQ10 represents an effective intervention to reduce the risk of developing preeclampsia (Teran et al., 2009).
Post hoc analysis showed that before supplementation, at week 20 of pregnancy, plasma CoQ10 levels showed no difference between controls and the supplemented groups. Interestingly, at delivery, placental tissue showed no differences in the placebo group when compared between women with normal pregnancy and those with preeclampsia, while in the CoQ10 group, women with preeclampsia showed significantly higher placental levels compared with normal pregnant women. However, mitochondrial levels of CoQ10 in the placenta from pregnant women with preeclampsia receiving placebo did not show significant differences when compared with those receiving supplementation of CoQ10. These results suggest that in women with preeclampsia, although CoQ10 reduced preeclampsia and was present in high levels in placental tissue, the mitochondrial levels of CoQ10 did not change significantly (Hernandez et al., 2017).
Conclusion
In conclusion, preeclampsia is associated with abnormal placental development as a result of several factors; of these, the dysfunction of the mitochondria appears to be the most important. In that case, the lack of energy and the associated increase of free radicals might be due to the deficiency or the consumption of CoQ10, as supplementation of CoQ10 was shown to be an effective intervention for the reduction of the rate of preeclampsia (Figure 1).
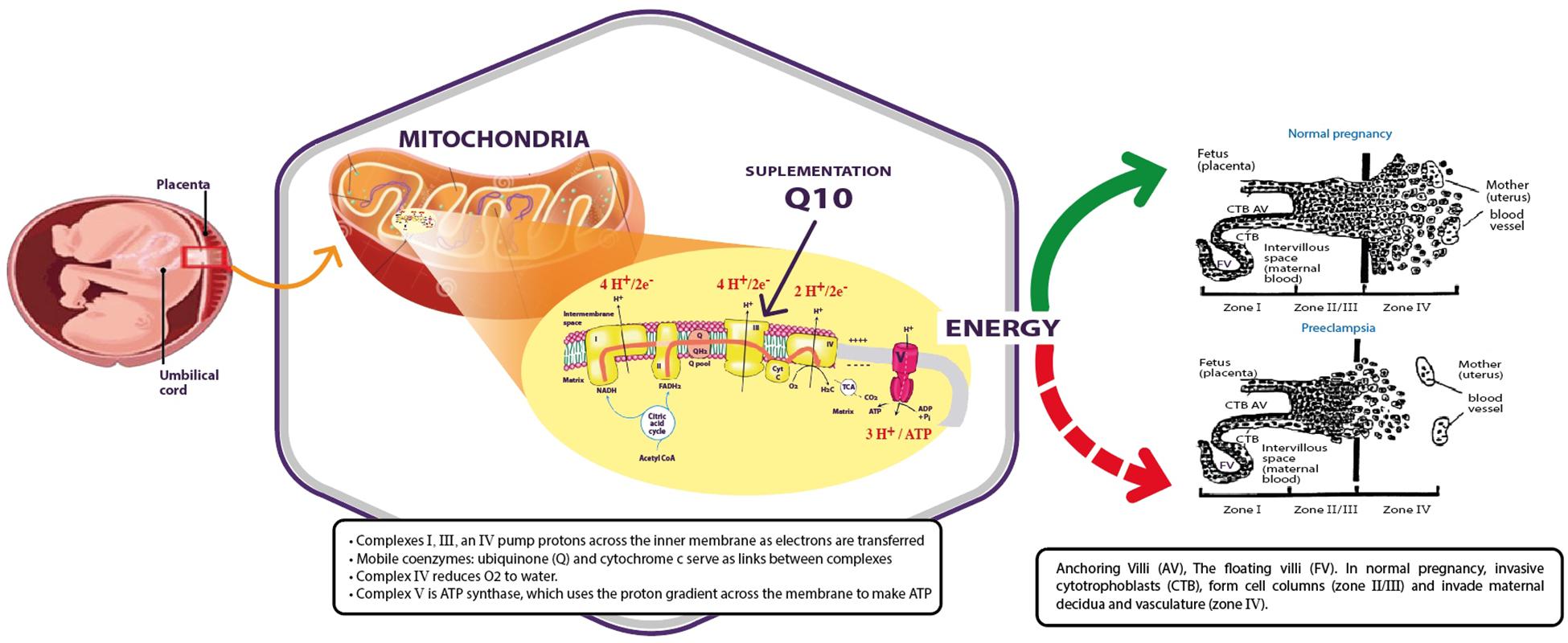
FIGURE 1. Mitochondria produce energy for placental development during normal pregnancy (green arrow) that is abnormal in preeclamptic women (red dashed arrow). Supplementation of coenzyme Q10 might restore the activity of the mitochondria.
Author Contributions
ET, IH, and AC contributed to the conception and design of the studies. LT and ST organized the databases. ET, ST, and AC performed the statistical analysis. ET, IH, and ST wrote the first draft of the manuscript. C-GH, MS-M, and GM wrote sections of the manuscript. All authors contributed to manuscript revision, read, and approved of the submitted version.
Conflict of Interest Statement
The authors declare that the research was conducted in the absence of any commercial or financial relationships that could be construed as a potential conflict of interest.
Acknowledgments
Thanks to Mrs. Sandra Vivero and Ms. Marcia Racines-Orbe for their technical support. Studies were possible by several grants sanctioned to ET from SENESCYT Ecuador, Jarrow Formulas, and Universidad San Francisco de Quito. Last, but not the least, to all those women, who kindly participated in our research.
References
Armaly, Z., Jadaon, J. E., Jabbour, A., and Abassi, Z. A. (2018). Preeclampsia: novel mechanisms and potential therapeutic approaches. Front. Physiol. 25:973. doi: 10.3389/fphys.2018.00973
Branch, D. W., Mitchell, M., Miller, E., Palinski, W., and Witztum, J. (1994). Preeclampsia and serum antibodies to oxidized low-density lipoprotein. Lancet 343, 645–646. doi: 10.1016/S0140-6736(94)92639-5
Brosens, I. A., Robertson, W. B., and Dixon, H. G. (1972). The role of the spiral arteries in the pathogenesis of preeclampsia. Obstet. Gynecol. Annu. 1, 177–191.
Calle, A., and Teran, E. (2018). “Estudio de la preeclampsia en Ecuador: del calcio a la coenzima Q10,” in La Medicina Ecuatoriana en el Siglo XXI. Academia Ecuatoriana de Medicina, Tomo I, eds J. Breilh, F. Hidalgo, and F. Bonilla (Quito: Editorial Corporación Editora Nacional), 421–437.
Centro de Estudios de Población y Desarrollo Social [CEPAR] (2004). Encuesta Demográfica y de Salud Materna e Infantil - ENDEMAIN. Quito: CEPAR.
Colburn, G. T., Chiag, M. H., and Main, E. K. (1994). Expression of the nonclassic histocompatibility antigen HLA-G by preeclamptic placenta. Am. J. Obstet. Gynecol. 170, 1244–1250. doi: 10.1016/S0002-9378(94)70134-2
Davidge, S. T., Hubel, C. A., Brayden, R. D., Capeless, E. C., and McLaughlin, M. K. (1992). Sera antioxidant activity in uncomplicated and preeclamptic pregnancies. Obstet. Gynecol. 79, 897–901.
Davidson, V. (1994). “Chapter 22: Mitochondrial electron transport and oxidative phosphorylation,” in Biochemistry, 3rd Edn, eds V. Davidson and D. Sittman (Pennsylvania, PA: Harwal Publishing), 379–386.
De Marco, C., and Caniggia, I. (2002). Mechanisms of oxygen sensing in human trophoblast cells. Placenta 23, S58–68. doi: 10.1053/plac.2002.0809
Dekker, G., and Baha, S. (2001). Primary, secondary and tertiary prevention of preeclampsia. Lancet 357, 209–215. doi: 10.1016/S0140-6736(00)03599-6
Evora, P. (2000). An open discussion about endothelial dysfunction: is it timely of propose a classification? Int. J. Cardiol. 73, 289–292. doi: 10.1016/S0167-5273(00)00223-0
Folgerø, T., Storbakk, N., Torbergsen, T., and Oian, P. (1996). Mutations in mitochondrial transfer ribonucleic acid genes in pre-eclampsia. Am. J. Obstet. Gynecol. 174, 1626–1630. doi: 10.1016/S0002-9378(96)70619-1
Furui, T., Karauchi, O., Tanaka, M., Mizutani, S., Ozawa, T., and Tomoda, Y. (1994). Decrese in cytochrome c oxidase and cytochrome oxidase subunit I messenger RNA levels in preeclamptic pregnancies. Obstet. Gynecol. 84, 283–288.
Genbacev, O., Zhou, Y., Ludlow, J., and Fisher, S. (1997). Regulation of human placental development by oxygen tension. Science 277, 1669–1672. doi: 10.1126/science.277.5332.1669
Gerretsen, G., Huisjes, H., and Elema, J. D. (1981). Morphological changes of the spiral arteries in the placenta bed in relation to preeclampsia an fetal growth retardation. Br. J. Obstet. Gynaecol. 88, 876–881. doi: 10.1111/j.1471-0528.1981.tb02222.x
Goldman-Wohl, D., and Yagel, S. (2002). Regulation of trophoblastocyst invasion: from normal implantation to preeclampsia. Mol. Cell. Endocrinol. 187, 233–238. doi: 10.1016/S0303-7207(01)00687-6
Graham, C., and McCrae, K. (1996). Altered expression of gelatinase and surface-associated plasminogen activator activity by trophoblast cells isolated from placentas of preeclamptic patient. Am. J. Obstet. Gynecol. 175, 555–562. doi: 10.1053/ob.1996.v175.a74404
Granger, J., Alexander, B., Bennett, W., and Khalil, R. (2001). Pathophysiology of pregnancy-induced hypertension. AJH 14, S178–S185. doi: 10.1016/S0895-7061(01)02086-6
Hernandez, I., Vivero, S., Racines-Orbe, M., Calle, A., Molina, G., and Teran, E. (2017). Placental and mitochondrial Q10 content after CoQ10 supplementation during pregnancy. Free Radic. Biol. Med. 108:S77. doi: 10.1016/j.freeradbiomed.2017.04.259
Hodson, J., and Watts, G. (2003). Can coenzyme Q10 improve vascular function and blood pressure? Potential for effective therapeutic reduction in vascular oxidative stress. BioFactors 18, 129–136. doi: 10.1002/biof.5520180215
Instituto Nacional Ecuatoriano de Estadísticas y Censos [INEC] (2017). Principales Causas de Morbi-Mortalidad Materna en el Ecuador. Quito: INEC.
Kaikkonen, J., Nyyssonen, K., Toumainen, T., Ristonmaa, U., and Salonen, J. (1999). Determinants of plasma coenzymen Q10 in humans. FEBS Lett. 443, 163–166. doi: 10.1016/S0014-5793(98)01712-8
Khong, T. Y., De Wolf, F., Robertson, W. B., and Brosens, I. (1986). Inadequate maternal vascular response to placentation in pregnancies complicated by preeclampsia and by small for gestational age infants. Br. J. Obstet. Gynecol. 93, 1049–1059. doi: 10.1111/j.1471-0528.1986.tb07830.x
Koelman, C., Coumans, A., Nijman, H., Doxiadias, I., Dekker, G., and Glaas, F. (2000). Correlation between oral sex and a low incidence of preeclampsia: a role for soluble HLA in seminal fluid? J. Reprod. Immunol. 46, 155–166. doi: 10.1016/S0165-0378(99)00062-5
Lenaz, G. (2001). A critical appraisal of the mitochondiral coenzyme Q pool. FEBS Lett. 509, 151–155. doi: 10.1016/S0014-5793(01)03172-6
Lim, K., Zhou, Y., Janatpour, M., Mcmaster, M., Bass, K., Chun, S., et al. (1997). Human cytotrophoblast differentiation/invasion is abnormal in preeclampsia. Am. J. Pathol. 151, 1809–1818.
Little, R., and Gladen, B. (1999). Levels of lipid peroxides in uncomplicated pregnancy: a review of the literature. Reprod. Toxicol. 13, 347–352. doi: 10.1016/S0890-6238(99)00033-7
López-Jaramillo, P. (1993). “Epidemiología de la hipertensión inducido por el embarazo: situación nacional,” in Hipertensión Inducida por el Embarazo. Fisiopatología y Prevención, ed. P. En Lopez-Jaramillo (Quito: UNICEF), 1–13.
López-Jaramillo, P., Terán, E., Ringqvist, A., Moya, W., Rivera, J., and Berrazueta, J. R. (1998). “Oxidised low-density lipoproteins and nitric oxide during normal pregnancy and preeclampsia,” in Biology of Nitric Oxide, Part 6, eds S. Moncada, N. Toda, H. Maeda, and E. A. Higgs (London: Portland Press), 322.
Matsubara, S., Minakami, H., Sato, I., and Saito, T. (1997). Decrease in cytochrome C oxidase activity detected cytochemically in the placental trophoblast of patients with pre-eclampsia. Placenta 18, 255–259. doi: 10.1016/S0143-4004(97)80059-8
Mikhail, M., Anyaegbunam, A., Garfinkel, D., Palan, R., Basu, J., and Rommey, S. (1994). Preeclampsia and antioxidants nutrients: decreased plasma levels of reduced ascorbic acid, ( tocopherol, and beta-carotene in women with preeclampsia. Am. J. Obstet. Gynecol. 171, 150–157. doi: 10.1016/0002-9378(94)90462-6
National High Blood Pressure Education Program Working Group Report on High Blood Pressure in Pregnancy (1990). Am. J. Obstet. Gynecol. 163, 1691–1712.
Noia, G., Littarru, G. P., De Santis, M., Oradei, A., Mactromarino, C., Trivellini, C., et al. (1996). Coenzyme Q10 in pregnancy. Fetal Diagn. Ther. 11, 264–270. doi: 10.1159/000264313
Noia, G., Romano, D., De Santis, M., Mariorenzi, S., Caruso, A., and Mancuso, S. (1998). Coenzyme Q10 fetal plasma levels. Fetal Diagn. Ther. 13, 127–130. doi: 10.1159/000020820
Page, W. (1939). The relation between hydatid moles, relative ischemia of the gravid uterus, and the placental origin of eclampsia. Am. J. Obstet. Gynecol. 37, 291–293.
Palan, P. R., Shaban, D. W., Martino, T., and Mikhail, M. S. (2004). Lipid-Soluble antioxidants and pregnancy: maternal serum levels of coenzyme Q(10), alpha-tocopherol and gamma-tocopherol in preeclampsia and normal pregnancy. Gynecol. Obstet. Invest. 58, 8–13.
Redman, C. (1991). Current topic: pre-eclampsia and the placenta. Placenta 12, 301–308. doi: 10.1016/0143-4004(91)90339-H
Roberts, D., and Redman, C. (1993). Pre-eclampsia: more then pregnancy induced hypertension. Lancet 341, 1447–1451. doi: 10.1016/0140-6736(93)90889-O
Roberts, J., and Lían, K. (2002). Recents insights into the pathogenesis of preeclampsia. Placenta 23, 359–372. doi: 10.1053/plac.2002.0819
Saftlas, A. F., Rubenstein, L., Prater, K., Harland, K. K., Field, E., and Triche, E. W. (2014). Cumulative exposure to paternal seminal fluid prior to conception and subsequent risk of preeclampsia. J. Reprod. Immunol. 10, 104–110. doi: 10.1016/j.jri.2013.07.006
Saito, S., and Nakashima, A. (2014). A review of the mechanism for poor placentation in early-onset preeclampsia: the role of autophagy in trophoblast invasion and vascular remodeling. J. Reprod. Immunol. 10, 80–88. doi: 10.1016/j.jri.2013.06.002
Sikkema, J., van Rijn, B., Frnak, A., Bruinse, H., de Roos, R., Stroes, E., et al. (2001). Placental superoxide is increased in preeclampsia. Placenta 22, 304–308. doi: 10.1053/plac.2001.0629
Solomon, C., and Seely, A. (2001). Hypertension in pregnancy a manifestation of the insulin resistance syndrome? Hypertension 37, 232–239.
Staff, C., Halvorsen, B., Ranheim, T., and Henriksen, T. (1999). Elevated level of free 8-iso-prostaglandin F2 alpha in decidua basalis of women with preeclampsia. Am. J. Obstet. Gynecol. 181, 1211–1215. doi: 10.1016/S0002-9378(99)70110-9
Teran, E. (2003). Ubiquinone Reacts with Nitric Oxide to Prevent the Activity of Purified Complex I. Docotoral thesis, University of London, London.
Teran, E., Chedraui, P., Racines-Orbe, M., Vivero, S., Villena, F., Duchicela, F., et al. (2008). Coenzyme Q10 levels in women with preeclampsia living at different altitudes. Biofactors 32, 185–190. doi: 10.1002/biof.5520320122
Teran, E., Hernandez, I., Nieto, B., Tavara, R., Ocampo, J. E., and Calle, A. (2009). Coenzyme Q10 supplementation during pregnancy reduces the risk of pre-eclampsia. Int. J. Gynaecol. Obstet. 105, 43–45. doi: 10.1016/j.ijgo.2008.11.033
Teran, E., Mora, L., Hernández, I., Racines-Orbe, M., Vivero, S., Vela, P., et al. (2007). Niveles intracelulares de coenzima Q10 durante el embarazo normal y en el complicado con preeclampsia. Rev. Ecuatoriana Ginecol. Obstet 14, 86–89.
Teran, E., Racines, M., Vivero, S., Escudero, C., Molina, G., and Calle, A. (2003). Preeclampsia is associated with a decrease in plasma coenzyme Q10 levels. Free Radic. Biol. Med. 35, 1453–1456. doi: 10.1016/j.freeradbiomed.2003.08.004
Teran, E., Vivero, S., Racines-Orbe, M., Castellanos, A., Chuncha, G., Enriquez, G., et al. (2005). Coenzyme Q10 is increased in placenta and cord blood during preeclampsia. BioFactors 25, 153–158. doi: 10.1002/biof.5520250117
The Maternal Health Study [MNPI] (1999). Futures Group International. Indice de Esfuerzo de Programa Materno y Neonatal: Ecuador. Available at: http://www.policyproject.com/policycircle/documents/MNPI/Ecuador_MNPI.pdf
Thornton, J., and Macdonald, A. (1991). Twin mothers, pregnancy hypertension and preeclampsia. Br. J. Obstet. Gynaecol. 106, 570–575. doi: 10.1111/j.1471-0528.1999.tb08326.x
Torbergsen, T., Oian, P., Mathiesen, E., and Borud, O. (1989). Preeclampsia a mitochondrial disease? Acta Obstet. Gynecol. Scand. 68, 145–148. doi: 10.3109/00016348909009902
van der Graaf, A. M., Wiegman, M. J., Plösch, T., Zeeman, G. G., van Buiten, A., Henning, R. H., et al. (2013). Endothelium-dependent relaxation and angiotensin II sensitivity in experimental preeclampsia. PLoS One 8:e79884. doi: 10.1371/journal.pone.0079884
Vuorinen, K., Remes, A., Sormune, R., Tapanainen, J., and Hasseinen, I. (1998). Placental mitochondrial DNA and respiratory chain enzymes in the etiology of preeclampsia. Obstet. Gynecol. 91, 950–955.
Wang, Y., and Walsh, S. (1998). Placental mitochondria as a source of oxidative stress in preeclampsia. Placenta 19, 581–586. doi: 10.1016/S0143-4004(98)90018-2
Wang, Y., and Walsh, S. W. (1996). Antioxidant activities and mRNA expression of SOD, catalase and glutathione peroxidase in normal and preeclamptic placentas. J. Soc. Gynecol. Investig. 3, 179–184. doi: 10.1177/107155769600300404
Widschwendter, M., Schrocksnadel, H., and Mortl, M. G. (1998). Pre-eclampsia: a disorder of placental mitochondria? Mol. Med. Today 4, 286–291. doi: 10.1016/S1357-4310(98)01293-3
Zamudio, S. (2003). The placenta at high altitude. High Alt. Med. Biol. 4, 171–191. doi: 10.1089/152702903322022785
Zhou, Y., Damsky, C., and Fisher, S. (1997a). Preeclampsia is associated with failure of human cytothrophoblast to mimic a vascular adhesion phenotype. One cause of defective endovascular invasion in this syndrome? J. Clin. Invest. 9, 2152–2164.
Keywords: preeclampsia, placenta, mitochondria, coenzyme Q10, pregnancy
Citation: Teran E, Hernández I, Tana L, Teran S, Galaviz-Hernandez C, Sosa-Macías M, Molina G and Calle A (2018) Mitochondria and Coenzyme Q10 in the Pathogenesis of Preeclampsia. Front. Physiol. 9:1561. doi: 10.3389/fphys.2018.01561
Received: 23 August 2018; Accepted: 18 October 2018;
Published: 15 November 2018.
Edited by:
Antonio Colantuoni, Università degli Studi di Napoli Federico II, ItalyReviewed by:
Andrea Moriondo, Università degli Studi dell’Insubria, ItalyDominga Lapi, Università degli Studi di Pisa, Italy
Copyright © 2018 Teran, Hernández, Tana, Teran, Galaviz-Hernandez, Sosa-Macías, Molina and Calle. This is an open-access article distributed under the terms of the Creative Commons Attribution License (CC BY). The use, distribution or reproduction in other forums is permitted, provided the original author(s) and the copyright owner(s) are credited and that the original publication in this journal is cited, in accordance with accepted academic practice. No use, distribution or reproduction is permitted which does not comply with these terms.
*Correspondence: Enrique Teran, eteran@usfq.edu.ec