- 1Department of Integrative Physiology, University of Colorado Boulder, Boulder, CO, United States
- 2Department of Psychology and Neuroscience, University of Colorado Boulder, Boulder, CO, United States
- 3Department of Neurology, Anschutz Medical Campus, University of Colorado Denver, Boulder, CO, United States
Fatigue is one of the most debilitating symptoms of multiple sclerosis (MS), and the underlying mechanisms are poorly understood. When exposed to a physical or cognitive challenge, individuals with MS tend to exhibit greater declines in task performance (performance fatigability) and increased levels of self-reported fatigue (perceived fatigability), but these effects may be attenuated by greater intellectual capacity. The purpose of our study was to examine the influence of intelligence on fatigability in persons with MS. We hypothesized that greater intellectual capacity confers some protection against heightened levels of fatigue and fatigability associated with MS. Twelve adults with relapsing-remitting MS were compared with 12 control (CO) subjects who were matched for age, sex, and premorbid intellectual capacity. Performance fatigability was measured as the decline in maximal voluntary contraction (MVC) torque after 60 isometric contractions (10 s contraction at 25% MVC, 5 s rest) performed with the knee extensor muscles. Perceived fatigability was assessed with the modified fatigue impact scale (MFIS) questionnaire (trait fatigability) and the Borg rating of perceived exertion (RPE, state fatigability). Persons with MS reported greater MFIS scores (MS: 43 ± 14; CO: 11 ± 8, P ≤ 0.001). Initial MVC torque for the knee extensors did not differ between the groups (MS: 112 ± 38 N⋅m; CO: 107 ± 44 N⋅m) and the decline (performance fatigability) was similar for both groups (MS: -16 ± 19 N⋅m; CO: -13 ± 16 N⋅m). RPE increased during the fatiguing contraction for both groups (P < 0.001) but was significantly greater in magnitude (main effect for group, P = 0.03) and increased more for the MS group (group × time interaction, P = 0.05). Torque steadiness declined during the fatiguing contractions (main effect for time, P = 0.05) and was less steady for the MS group (main effect for group, P = 0.02). Performance and full-4 IQ was correlated with the decline in torque steadiness for the MS group (r = -0.63, P < 0.05; r = -0.64, P < 0.05). Intellectual capacity was not associated with fatigability in persons with MS but was associated with adjustments in muscle activation during the fatiguing contractions.
Introduction
Multiple sclerosis (MS) is a neurological disorder that compromises the integrity of signaling pathways in the central nervous system through chronic inflammatory responses and neurodegeneration (Trapp and Nave, 2008). Although the clinical progression of MS varies widely between individuals (Lublin and Reingold, 1996; Confavreux and Vukusic, 2006), one of the most common symptoms of the disease is a heightened level of fatigue (Fisk et al., 1994; Alvarenga-Filbo et al., 2015; Loy et al., 2017; Penner and Paul, 2017), the underlying mechanisms of which are poorly understood.
The word fatigue is commonly used throughout the literature to describe a wide variety of conditions, which leads to uncertainty in its intended meaning when it is not clearly defined. In an effort to remain consistent with more recent suggestions to adopt a unified taxonomy (Kluger et al., 2013; Enoka and Duchateau, 2016; Zijdewind et al., 2016), we define fatigue as a symptom that emerges in an individual due to adaptations in both perceived fatigability and performance fatigability. Perceived fatigability is assessed by self-report and reflects challenges to homeostasis and the psychological state of the individual. Trait levels of perceived fatigability refer to sensations reported by an individual over longer durations of time (days to weeks), whereas state levels indicate a momentary value. Performance fatigability is quantified as a decline in the ability to perform a given task due to adjustments in muscle activation and contractile capacity.
Symptoms of fatigue can severely limit activities of daily life and are often reported to have a more negative impact on quality of life than physical disability (Fisk et al., 1994; Amato et al., 2001; Janardhan and Bakshi, 2002; Alvarenga-Filbo et al., 2015). In addition to the debilitating effects of fatigue, MS often leads to various other cognitive and motor impairments. Cognitive impairments may include declines in processing speed, memory and executive function (Chiaravalloti and DeLuca, 2008), and motor impairments commonly include declines in mobility, strength, force steadiness, and performance fatigability (Koch et al., 2007; Dalgas et al., 2008; Hadjimichael et al., 2008). Some studies, however, have shown that possessing a higher premorbid intellectual capacity may confer protection against declines in cognitive function with progression of a neurological disease, an idea referred to as cognitive reserve. The concept of cognitive reserve posits that cognitive impairments develop more gradually as a neurological disease progresses in people with greater intellectual capacity (Stern, 2009). Persons with MS who have greater intellectual capacity, for example, can achieve comparable levels of performance on behavioral tasks with less modulation of cortical activity compared with those who have fewer years of education or lower vocabulary scores (Benedict et al., 2010; Sumowski et al., 2010).
Although current evidence on the relation between perceived fatigability and cognitive function appears equivocal (Morrow et al., 2009), persons with MS often report that fatigue interferes with cognitive functioning (Krupp et al., 1988; Monks, 1989) and that increases in fatigue occur after experiencing a cognitive challenge (Bailey et al., 2007; Tartaglia et al., 2008). Furthermore, a cognitive challenge worsens motor performance in individuals with MS more so than control subjects, and the increased cognitive demand is associated with elevated levels of perceived fatigability (Wolkorte et al., 2015). These findings suggest that intellectual capacity may be related to perceived and performance fatigability.
The purpose of our study was to examine the influence of intellectual capacity on perceived and performance fatigability in persons with MS. Intellectual capacity was measured by the Wechsler Abbreviated Scale of Intelligence. Given that intellectual capacity protects against MS-related declines in cognitive function (Sumowski et al., 2009a,b) and cerebral efficiency (Sumowski et al., 2010) and that fatigue is associated with greater levels of cortical activity (Enoka and Duchateau, 2016; Zijdewind et al., 2016; Loy et al., 2017; Severijns et al., 2017), we hypothesized that individuals with MS who had higher levels of intellectual capacity would experience lower levels of fatigue and fatigability.
Materials and Methods
Twelve adults (39 ± 15 years, 1 man) with a diagnosis of relapsing-remitting MS and 12 control subjects (41 ± 14 years, 1 man) who were matched for age, sex, and intellectual capacity participated in the study after written informed consent was obtained. Participants with MS were included if they did not have a change in prescription medications (for ≥90 days), were able to walk ≥100 m unassisted, complained that fatigue interfered with their quality of life, and did not experience a relapsing episode within 90 days of participating in the study. None of the participants had a history of cardiovascular incidents, seizures, or traumatic brain injuries, and all participants were right-handed.
The experimental protocol was approved by the Institutional Review Board at the University of Colorado Boulder (protocol#: 12-0421) and conformed to the Declaration of Helsinki. The study involved one visit to the laboratory, which lasted ∼2 h. Intellectual capacity, executive function, walking performance, perceived fatigability, and performance fatigability were assessed during this visit.
Intellectual Capacity
Intellectual capacity was assessed with the Wechsler Abbreviated Scale of Intelligence (WASI) (Wechsler, 1999). The test provides a performance intelligence quotient (IQ) score derived from the following: the block design and matrix reasoning subtests, a verbal IQ score based on the vocabulary and similarities subtests, and a Full-4 scale that is a composite of the performance and verbal IQ scores. Verbal performance is resistant to declines caused by the progression of MS and was therefore used to estimate premorbid intellectual capacity (Lezak, 2004; Chiaravalloti and DeLuca, 2008; Sumowski et al., 2009a) and to match control participants with those in the MS group. The Behavior Rating Inventory of Executive Function (BRIEF) served as an additional measure of cognitive function by assessing difficulties in goal-directed behavior during activities of daily living (Roth et al., 2005).
Walking Performance
The 25-foot walk test was used to measure functional capacity of the lower extremities and was performed without the use of orthotic or other assistive devices. Participants were directed to one end of a 25-foot walkway, which was clearly marked with white lines at both ends. The instructions were to walk as quickly as possible, but safely, from one end to the other, and without slowing down until well past the end line. Measurements were made by one of the investigators using a digital stopwatch. The walk was performed a second time in the opposite direction, and the faster of the two times is reported.
Perceived Fatigability
Trait levels of fatigue experienced by participants were quantified using the Modified Fatigue Impact Scale (MFIS) questionnaire, which considers physical, cognitive, and social factors influencing perceived fatigability (Kos et al., 2006). Participants were asked to indicate how often fatigue has had an influence on 21 different situations over the 4 weeks immediately preceding the study. Additionally, participants were asked to report the state level of fatigue at seven instances during a series of fatiguing contractions using the modified Borg scale (6–20) (Borg et al., 1987).
Performance Fatigability
Participants performed 60 intermittent isometric contractions with the knee extensor muscles. After determining maximal voluntary contraction (MVC) torque, participants were asked to increase knee extensor torque to the target (25% MVC torque) displayed on the monitor (gain: 3% MVC force/cm) in front of them and to hold it as steady as possible for 10 s. The investigator verbally prompted the participant to begin each contraction and then to relax for 5 s. This procedure was repeated for 60 contractions. The protocol was performed with both the left and right legs in a randomized order with ∼ 20 min of rest between the two trials. MVC torque was measured before and after the 60 contractions. Performance fatigability was quantified as the change in MVC torque from before to after the intermittent isometric contractions. Rating of perceived exertion (RPE) was measured with the modified Borg scale (6–20) after every 10th contraction. The scale was anchored with six representing rest or no exertion, and 20 corresponding to the strongest possible effort.
Experimental Setup
Participants were seated in an upright position with hips and knees flexed to ∼1.57 rad. A strain gauge force transducer (JR3, Woodland, CA, United States) was positioned to contact the anterior aspect of the lower leg (∼8 cm above the ankle). The applied force was displayed on a monitor located at eye level ∼60 cm in front of the subject. The distance between the axis of rotation for the knee joint and the horizontal plane of the lower leg that contacted the center of the force transducer was measured and used to calculate torque about the knee joint. The upper body was secured to the chair using 5 cm wide nylon straps across the shoulders and lap in order to minimize movement during contractions.
Electromyographic (EMG) signals were recorded from the vastus lateralis muscle using Ag-AgCl surface electrodes (Covidien, Mansfield, MA, United States) arranged in a bipolar configuration. Three pairs of electrodes were placed over the vastus lateralis along a line between the anterior superior iliac spine and the lateral border of the patella in the presumed direction of the muscle fibers. Three reference electrodes were placed over the medial surface of the tibia. EMG signals were amplified (1000×), band-pass filtered (13–1000 Hz) (Coulbourn Instruments, Allentown, PA, United States), sampled at 2 kHz (Cambridge Electronic Design, Cambridge, England, United Kingdom), and stored on a computer (Dell, Plano, TX, United States).
Maximal Voluntary Contractions
Maximal voluntary contraction torque was measured before the 60 contractions to determine maximal knee extensor strength, to provide a reference value for calculating a target torque of 25% MVC, and to record peak EMG activity. MVC torque was measured again immediately after the 60 contractions to assess performance fatigability. MVCs were performed with the knee extensor muscles by gradually increasing torque from rest to maximum over ∼3 s and maintaining this torque for ∼3 s. The investigators provided strong verbal encouragement during each MVC, and the gain of the visual feedback was adjusted between trials. At least two trials were performed with ≥90 s of rest between consecutive trials. If peak torques were not within 5% of each other for the two trials, or if a participant indicated that one of the efforts was not maximal, additional MVCs were performed until these criteria were met. The greatest peak torque during these trials was used as the maximal value.
Data Analysis
Force and EMG data were digitized (Power 1401; Cambridge Electronic Design, Cambridge, England, United Kingdom) and stored on a computer for offline analysis using Spike2 data acquisition/analysis software (Cambridge Electronic Design, Cambridge, England, United Kingdom). Force signals were sampled at 1 kHz.
The maximal EMG amplitude during the MVC trial with the maximal torque was quantified as the root-mean-square (RMS) value during a 0.5 s interval that spanned the peak of the EMG. This value was used to normalize subsequent EMG recordings. EMG data recorded during the 60 contractions were quantified as the normalized RMS value during 8 s intervals for the first, 10th, 20th, 30th, 40th, 50th, and 60th contraction. Torque steadiness was quantified during the same 8-s intervals as the coefficient of variation for torque [% = (SD/mean) × 100; Galganski et al. (1993)].
Statistical Analysis
Dependent t-tests were used to compare dependent variables before and after the 60 contractions and independent t-tests were used to compare group differences of dependent variables and subject characteristics. Two-factor analysis of variance was used to examine changes in dependent variables measured across the 60 contractions (first contraction, and every tenth contraction up to the 60th contraction) and differences between the two groups (MS and Control). Dependent variables measured during the fatigue protocol included EMG amplitude for the vastus lateralis muscle, knee extensor torque steadiness (the coefficient of variation for torque), and ratings of perceived exertion. One of the subjects in the MS group was unable to complete all 60 contractions, so data obtained during the last performed contraction were also used for the 50th and 60th contractions. Data collected on both legs were averaged for each participant as there were no significant differences between the left and right legs. Spearman’s correlation coefficients were determined between all measures of physical and cognitive function. Correlations were performed separately for the two groups. A significance level for all statistical tests was set at P ≤ 0.05. Data are presented as mean ± standard deviation (SD) in the text and as mean ± standard error (SE) in the figures.
Results
By design, individuals with MS reported significantly greater levels of trait fatigue than the control (CO) group, as measured with the MFIS questionnaire (Figure 1A; MS: 43 ± 14; CO: 11 ± 8, P ≤ 0.001). In contrast, there were no statistically significant differences between MS and CO groups for verbal IQ (Table 1; MS: 112 ± 13; CO: 114 ± 10), as the two groups were matched for this attribute. Performance IQ (MS: 119 ± 14; CO: 122 ± 11) and Full-4 IQ (MS: 118 ± 14; CO: 120 ± 10) also did not differ significantly between the two groups. The overall intellectual capacity of the MS group, therefore, was similar to that for the CO group, despite the MS participants having been diagnosed with the disease for 9.4 ± 6.4 years (range: 0.2–19 years) prior to participating in the study. However, a measure of executive function (BRIEF) was different between groups (MS: 122 ± 19; CO: 90 ± 17, P ≤ 0.001), indicating lower executive function in the MS participants. Greater BRIEF scores were correlated with greater levels of trait fatigue (MFIS), but this relation was only significant (P < 0.05) for the CO group (Table 2).
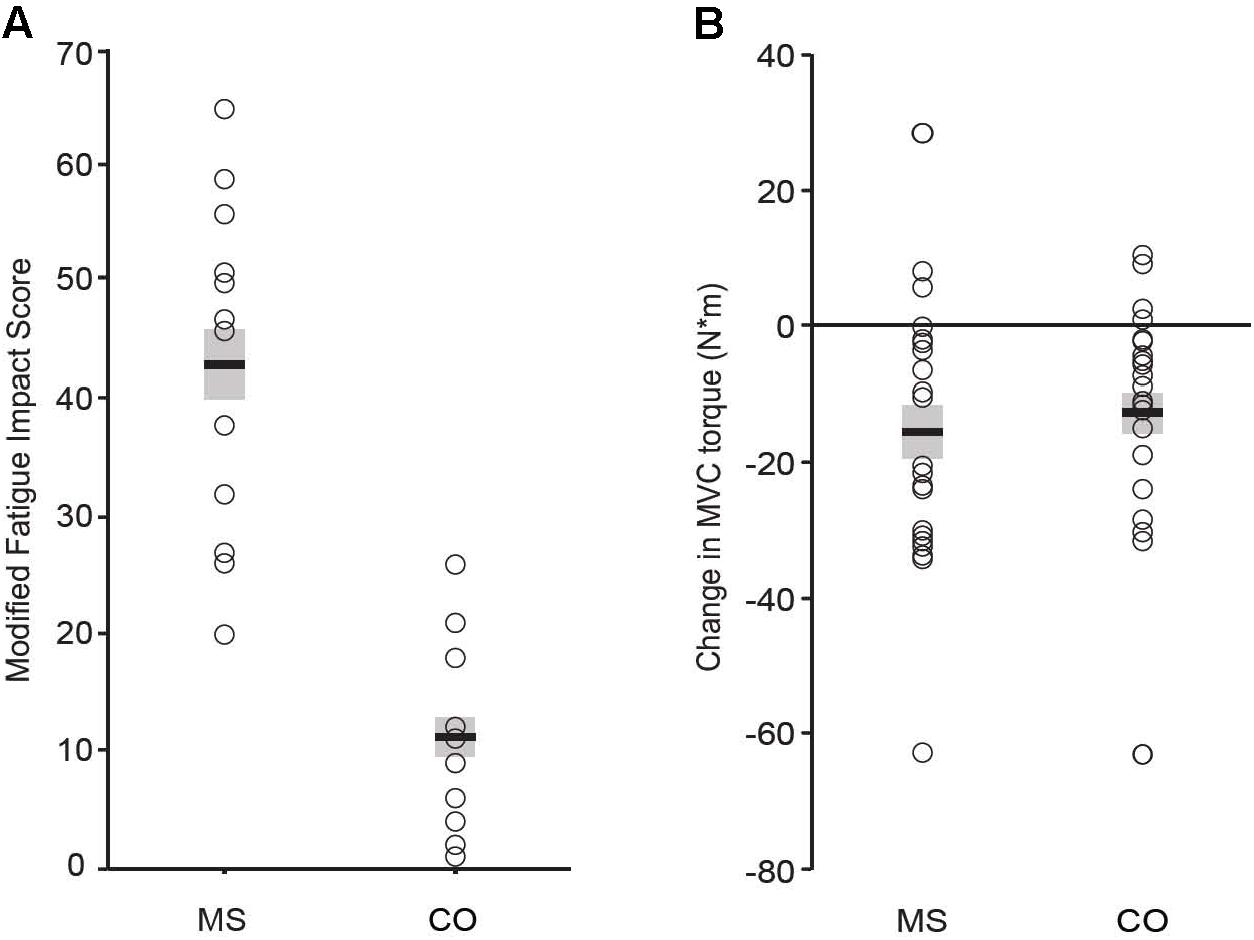
FIGURE 1. Perceived trait fatigue (A). Reported levels of fatigue (MFIS score) were greater for the MS group (P = 0.001). Performance fatigability (B). Declines in MVC torque were significant (P = 0.001 for both groups) and did not differ between groups (P = 0.74).
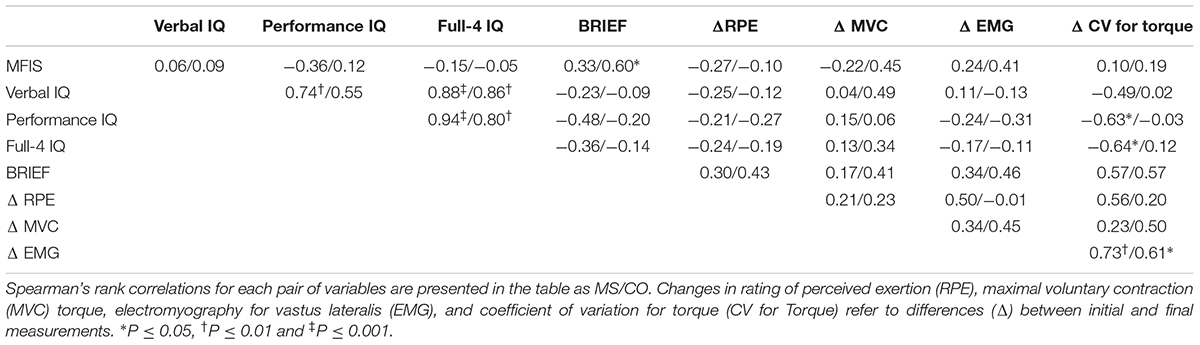
TABLE 2. Correlation coefficients for pairs of variables are shown for both multiple sclerosis (MS) subjects and control (CO) subjects.
There were no statistically significant group differences in either the 25-foot walk time (MS: 3.6 ± 0.4 s; CO: 3.3 ± 0.4 s) or knee extensor MVC torque prior to the 60 contractions (MS: 112 ± 38 N⋅m; 107 ± 44 N⋅m). Participants performed the 60 contractions with both legs separately due to the possibility of disease progression having an unequal effect on both sides of the body (Chung et al., 2008). However, changes in EMG, RPE, coefficient of variation for torque, and declines in MVC torque did not differ between left and right legs for either group of participants, so data for both legs were averaged for each participant. The two groups exhibited similar significant declines in MVC torque after the 60 contractions (Figure 1B; MS: -16 ± 19 N⋅m; CO: -13 ± 16 N⋅m).
Normalized EMG amplitude for vastus lateralis (MS: 38 ± 37% increase; CO: 31 ± 24% increase) increased, similarly, between groups (P = 0.49) during the 60 contractions (Figure 2; P < 0.001). Although the coefficient of variation for torque increased for both groups (MS: 66 ± 187% increase; CO: 40 ± 49% increase) during the 60 contractions (Figure 3; main effect for time, P = 0.05), the MS group was less steady (main effect for group, P = 0.02). Increases in coefficient of variation for torque were strongly correlated with increases in EMG amplitude for both MS (r = 0.73, P ≤ 0.001) and CO (r = 0.61, P ≤ 0.05) groups (Table 2). In addition, the increase in the coefficient of variation for torque during the 60 contractions observed for the MS group (start: 2.5 ± 1.6%; end: 3.9 ± 4.3%) was inversely correlated (Table 2) with two measures of intellectual capacity (Performance IQ: r = -0.63, P ≤ 0.05; Full-4 IQ: r = -0.64, P ≤ 0.05), but not verbal IQ.
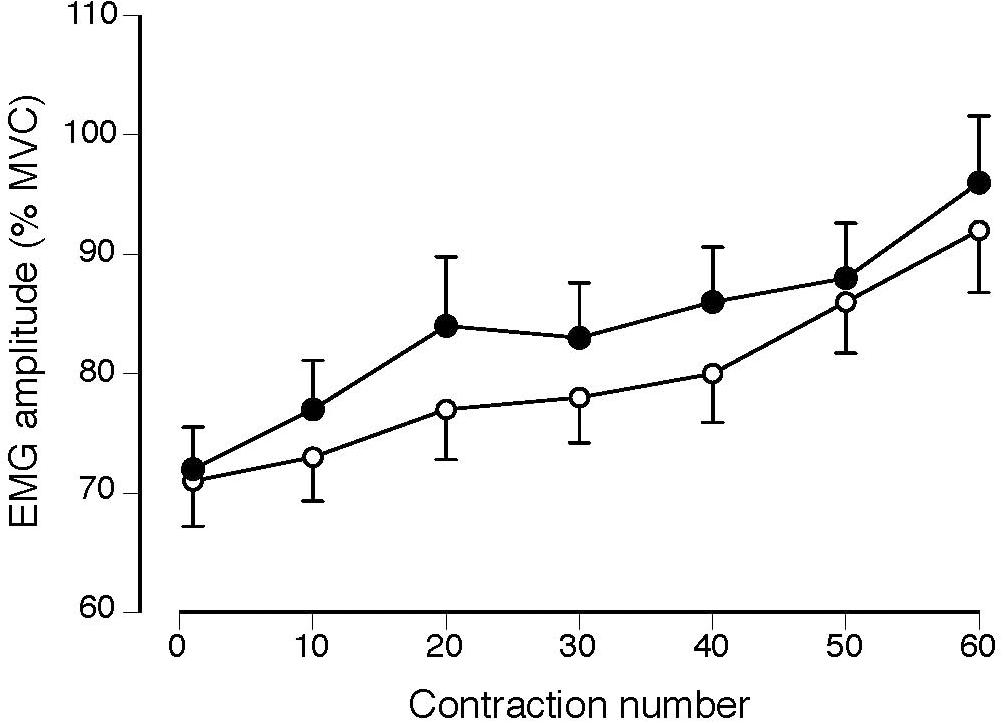
FIGURE 2. Normalized EMG amplitude for the vastus lateralis muscle in MS (filled circles) and CO subjects (open circles) increased during the 60 contractions (P < 0.001) with no difference between groups (P = 0.49).
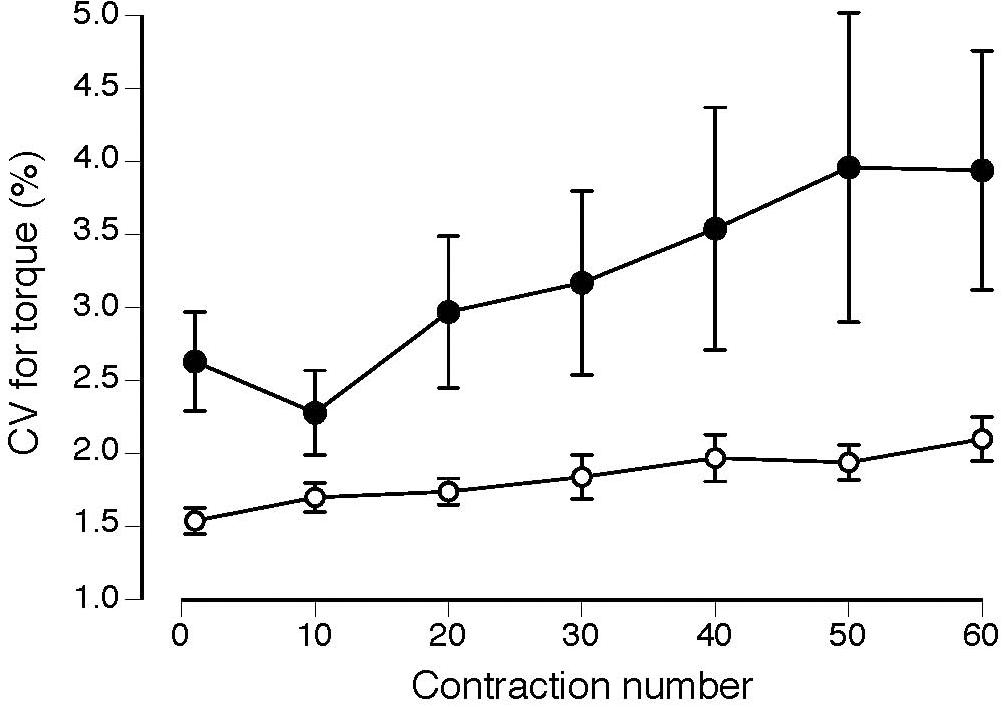
FIGURE 3. Coefficient of variation (CV) for torque increased during the 60 contractions (main effect for time, P = 0.05) for the MS (filled circles) and CO subjects (open circles) and the MS group was less steady (greater CV for torque) throughout the task (main effect for group, P = 0.02).
Rating of perceived exertion increased for both groups during the 60 contractions (Figure 4; P < 0.001), but was significantly greater in magnitude (group effect, P = 0.03) and increased more for the MS group than the CO group (group × time interaction, P = 0.05). The MS group tended to exhibit a greater incidence of reaching maximal effort with 5 participants reaching a final RPE of 20 at the end of the 60 contractions and only one participant for the CO group (χ2 = 3.6, P = 0.06).
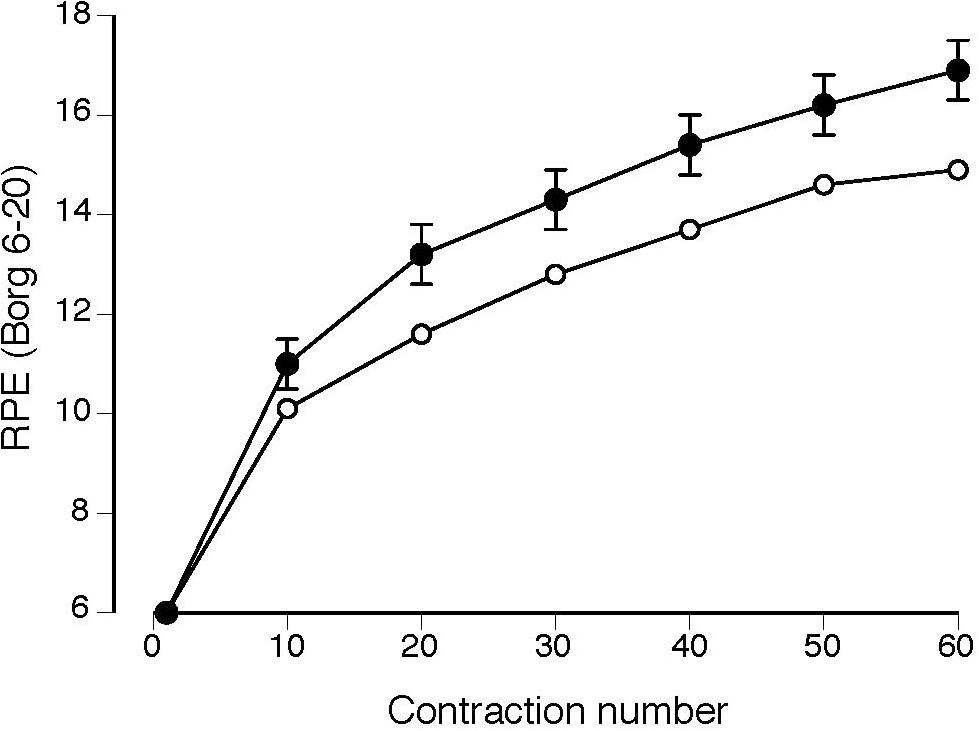
FIGURE 4. Rating of perceived exertion (RPE) increased more for the MS group (filled circles) during the 60 isometric contractions (group × time interaction, P = 0.05) than for the control group (open circles). Values reported as mean ± SE.
Discussion
MS participants had greater trait levels of perceived fatigability (MFIS) and greater state levels of perceived fatigability (RPE) during the intermittent contractions, but similar levels of performance fatigability (decline in MVC force) compared with the control group. Contrary to our hypothesis, there was no association between intellectual capacity and either perceived or performance fatigability. Our main finding was that torque steadiness worsened at a greater rate in the MS group during the fatiguing contractions with the knee extensors and that the change in torque steadiness for the MS group was inversely related to intellectual capacity as measured by performance IQ and full-4 IQ, but not verbal IQ. There were no group differences for Performance IQ suggesting that cognitive function, as measured by performing the block design and matrix reasoning tests, was spared in this sample of MS participants. However, differences in performance IQ within the MS group were associated with a reduced ability to maintain a steady muscle contraction with the knee extensors. Further, MS participants had greater impairments in executive function as measured by the BRIEF score. These findings indicate that intellectual capacity as measured by verbal and performance IQ appear to be retained despite 9.4 ± 6.4 years (range: 0.2–19 years) of disease progression, however, executive function may be impaired earlier by MS.
Muscle Activation and Intelligence
Due to the association between the increase in perceived fatigability (RPE) and EMG amplitude during submaximal fatiguing contractions (Hunter et al., 2002; Rudroff et al., 2007), the more rapid increase in RPE for the MS group in the current study should have been accompanied by a quicker increase in EMG amplitude during the fatigue protocol. In contrast, the increase in EMG amplitude for the vastus lateralis muscle was similar for the two groups (Figure 2). However, normalized measures of EMG amplitude as recorded with bipolar electrodes are relatively insensitive to modest changes in the underlying motor unit activity (Mottram et al., 2005; Rudroff et al., 2013; Farina et al., 2014).
Nonetheless, the more rapid increase in the coefficient of variation for torque – a measure of torque steadiness – for the MS group suggests that the neural drive to the muscles differed between the two groups during the 60 isometric contractions. The synaptic inputs received by motor neurons arise from three sources: a control signal received by all motor neurons, independent noise, and common noise (Farina and Negro, 2015; Farina et al., 2016). Although the timing of the action potentials discharged by each motor neuron is relatively independent, the control signal to produce the required muscle force (neural drive to muscle) depends on the common synaptic input received by the motor neurons. Critically, there is a strong association between the estimated variance in the common synaptic input to motor neurons and the amplitude of the force fluctuations (force steadiness) during a steady submaximal contraction (Farina et al., 2016; Feeney et al., 2018; Thompson et al., 2018). Thus, the more rapid increase in the coefficient of variation for torque during the fatigue protocol for the MS participants in the current study suggests quantitative differences between the two groups in the variance in the control signal generated by the nervous system during this task.
Consistent with the interpretation of a significant role for motor unit activity in modulating motor function in persons with MS, Almuklass et al. (2018) found that some of the variance in the performance of walking tests by individuals with MS could be explained by the discharge characteristics of motor units during steady submaximal contractions. They found that 40% of the variance in a test of walking endurance (6-min test) and maximal gait speed (25-ft test) could be explained by predictor variables that included the mean interval between consecutive action potentials (interspike interval) for soleus and medial gastrocnemius during submaximal isometric contractions, the strength of the dorsiflexor muscles, and force steadiness of the plantar flexor muscles.
Our findings indicated a strong correlation between the worsening of torque steadiness during the 60 isometric contractions and intellectual capacity for the MS group, but not the control group. Studies that have examined the interactions between cognitive and motor function have shown that the ability to exert a steady force during a voluntary contraction is compromised when the individual is exposed to a cognitive challenge (Zijdewind et al., 2006), and that this effect is greater during fatiguing contractions (Lorist et al., 2002) and in older adults (Vanden Noven et al., 2014; Pereira et al., 2015). Similarly, D’Orio et al. (2012) found that measures of processing speed, executive function, memory and general intelligence were among the cognitive variables that were significantly associated with walking speed and fall frequency in persons with MS. General intelligence as measured by the WASI test was associated with walking speed (r = -0.272, P = 0.017) as well as frequency of falls (r = -0.229, P = 0.043). Although measures of intellectual capacity were not correlated with walking performance in our study and time to complete the 25-foot walk did not differ between the two groups, intellectual capacity was inversely correlated with a worsening of torque steadiness during the 60 isometric contractions for the MS group, which may indicate that changes in the neural control of leg muscles may precede declines in walking performance.
Fatigue and Fatigability
Despite greater trait and state levels of perceived fatigability, performance fatigability for the MS participants was not different from control participants. The groups had similar declines in knee extensor torque after the fatigue protocol and similar increases in EMG amplitude. Similarities between groups could not be explained by baseline differences in MVC torque or mobility as measured by the 25-foot walk test. Steens et al. (2012a) reported similar observations when they compared the decrease in MVC force after a 2-min sustained maximal contraction with a hand muscle exhibited by 20 persons with relapsing-remitting MS and 20 age- and sex-matched control subjects. Based on a multiple-regression analysis to explain the variance in the trait level of fatigue (Fatigue Severity Scale, FSS) for the MS participants, they found that 45% of the variance in the FSS score could be explained by the measure of performance fatigability (decline in MVC force) and normalized muscle strength (MVC force). When a measure of depression (Hospital Anxiety and Depression Scale Questionnaire) was included in the analysis, the regression model explained 77% of variance in the trait level of fatigue (FSS score).
In another study, Steens et al. (2012b) compared the adjustments exhibited by persons with relapsing-remitting MS and control subjects during the 2 min sustained maximal contraction with a hand muscle. As in their other study (Steens et al., 2012a), performance fatigability (decline in MVC force) was similar for the two groups of participants. Nonetheless, there were significant differences between the two groups in the changes in the level of voluntary activation (twitch interpolation technique) and cortical activation as determined with functional MRI. Compared with the control subjects, the MS participants exhibited a more substantial and variable reduction in voluntary activation and less of an increase in cortical activation during the fatiguing contraction. A multiple-regression analysis indicated that control subjects who were stronger experienced greater performance fatigability, as reported in other studies (Hunter and Enoka, 2001; Keller-Ross et al., 2014), and that performance fatigability for the individuals with MS was less for those who could sustain greater levels of voluntary activation (Wolkorte et al., 2016).
Based on the results of Steens et al. (2012b), a greater increase in perceived fatigability (RPE) during the fatigue protocol in our study might be explained by a tendency for persons with MS to experience a greater reduction in voluntary activation during fatiguing contractions. Thus, it is necessary for these individuals to increase the levels of cortical activation to achieve the submaximal target torque during the fatigue protocol.
Limitations
Although our study enrolled relatively few participants with a heterogeneous disease status, one of its strengths was that the participants in the MS group reported greater levels of trait fatigue despite no significant group differences in intellectual capacity, strength, or walking speed, as such differences may have influenced measured levels of trait fatigue. Consequently, our findings may not generalize to a wider population of individuals with MS who have varying levels of disability. Also, we did not control the medications being taken by our participants to treat symptoms other than to ensure that they were on stable doses of medications.
Conclusion
Although our study found no statistically significant associations between the trait level of fatigue and measures of performance and perceived fatigability for the MS participants during a series of intermittent isometric contractions, intellectual capacity was associated with adjustments in torque steadiness during the fatiguing contraction. This finding substantiates further inquiry into the utility of the performance IQ score to detect changes in neuromuscular function that precede physical disability due to disease progression in persons with MS.
Author Contributions
JG contributed to the conception of the study, participant recruitment and testing, data analysis and interpretation, and writing and approval of the manuscript. AR and BC contributed to participant recruitment and testing, data analysis, and writing and approval of the manuscript. KK and GC contributed to testing, data analysis, and approval of the manuscript. MB and JC contributed to conception of the study, participant recruitment, data interpretation, and approval of the manuscript. RE contributed to conception of the study, participant recruitment, data interpretation, and writing and approval of the manuscript.
Funding
The research was supported by an award (Seed Grant) from the University of Colorado Boulder.
Conflict of Interest Statement
The authors declare that the research was conducted in the absence of any commercial or financial relationships that could be construed as a potential conflict of interest.
References
Almuklass, A. M., Davis, L., Hamilton, L. D., Vieira, T., Botter, A., and Enoka, R. M. (2018). Motor unit discharge characteristics and walking performance of individuals with multiple sclerosis. J. Neurophysiol. 119, 1273–1282. doi: 10.1152/jn.00598.2017
Alvarenga-Filbo, H., Ponziani, G., Rossi, F., Liedl, C. L., Stefanile, C., and Rossi, L. (2015). Does fatigue occur in MS patients without disability? Int. J. Neurosci. 125, 107–115. doi: 10.3109/00207454.2014.909415
Amato, M. P., Ponziani, G., Rossi, F., Liedl, C. L., Stefanile, C., and Rossi, L. (2001). Quality of life in multiple sclerosis: the impact of depression, fatigue and disability. Mult. Scler. 7, 340–344. doi: 10.1177/135245850100700511
Bailey, A., Channon, S., and Beaumont, J. G. (2007). The relationship between subjective fatigue and cognitive fatigue in advanced multiple sclerosis. Mult. Scler. 13, 73–80. doi: 10.1177/1352458506071162
Benedict, R. H., Morrow, S. A., Weinstock Guttman, B., Cookfair, D., and Schretlen, D. J. (2010). Cognitive reserve moderates decline in information processing speed in multiple sclerosis patients. J. Int. Neuropsychol. Soc. 16, 829–835. doi: 10.1017/S1355617710000688
Borg, G., Hassmén, P., and Lagerström, M. (1987). Perceived exertion related to heart rate and blood lactate during arm and leg exercise. Eur. J. Appl. Physiol. 65, 679–685. doi: 10.1007/BF00424810
Chiaravalloti, N. D., and DeLuca, J. (2008). Cognitive impairment in multiple sclerosis. Lancet Neurol. 7, 1139–1151. doi: 10.1016/S1474-4422(08)70259-X
Chung, L. H., Remelius, J. G., Van Emmerik, R. E. A., and Kent-Braun, J. A. (2008). Leg power asymmetry and postural control in women with multiple sclerosis. Med. Sci. Sports Exerc. 40, 1717–1724. doi: 10.1249/MSS.0b013e31817e32a3
Confavreux, C., and Vukusic, S. (2006). Natural history of multiple sclerosis: a unifying concept. Brain 129, 606–616. doi: 10.1093/brain/awl007
Dalgas, U., Stenager, E., and Ingemann-Hansen, T. (2008). Review: multiple sclerosis and physical exercise: recommendations for the a lication of resistance-, endurance- and combined training. Mult. Scler. 14, 35–53. doi: 10.1177/1352458507079445
D’Orio, V. L., Foley, F. E., Armentano, F., Picone, A. M., Kim, S., and Holtzer, R. (2012). Cognitive and motor functioning in patients with multiple sclerosis: neuropsychological predictors of walking speed and falls. J. Neurol. Sci. 316, 42–46. doi: 10.1016/j.jns.2012.02.003
Enoka, R. M., and Duchateau, J. (2016). Translating fatigue to human performance. Med. Sci. Sports Exerc. 48, 2228–2238. doi: 10.1249/MSS.0000000000000929
Farina, D., Merletti, R., and Enoka, R. M. (2014). The extraction of neural strategies from the surface EMG: an update. J. Appl. Physiol. 117, 1215–1230. doi: 10.1152/japplphysiol.00162.2014
Farina, D., and Negro, F. (2015). Common synaptic input to motor neurons, motor unit synchronization, and force control. Exerc. Sport Sci. Rev. 43, 23–33. doi: 10.1249/JES.0000000000000032
Farina, D., Negro, F., Muceli, S., and Enoka, R. M. (2016). Principles of motor unit physiology evolve with advances in technology. Physiology 31, 83–94. doi: 10.1152/physiol.00040.2015
Feeney, D. F., Mani, D., and Enoka, R. M. (2018). Variability in common synaptic input to motor neurons modulates both force steadiness and pegboard time in young and older adults. J. Physiol. 596, 3793–3806. doi: 10.1113/JP275658
Fisk, J. D., Pontefract, A., Ritvo, P. G., Archibold, C. J., and Murray, T. J. (1994). The impact of fatigue on patients with multiple sclerosis. Can. J. Neurol. Sci. 21, 9–14. doi: 10.1017/S0317167100048691
Galganski, M. E., Fuglevand, A. J., and Enoka, R. M. (1993). Reduced control of motor output in a human hand muscle of elderly subjects during submaximal contractions. J. Neurophysiol. 69, 2108–2115. doi: 10.1152/jn.1993.69.6.2108
Hadjimichael, O., Vollmer, J., and Oleen-Burkey, M. (2008). Fatigue characteristics in multiple sclerosis: the North American Research Committee on Multiple Sclerosis (NARCOMS) survey. Health Qual. Life Outcomes 6:100. doi: 10.1186/1477-7525-6-100
Hunter, S. K., and Enoka, R. M. (2001). Sex differences in the fatigability of arm muscles depends on the absolute force during isometric contractions. J. Appl. Physiol. 91, 2686–2694. doi: 10.1152/jappl.2001.91.6.2686
Hunter, S. K., Ryan, D. L., Ortega, J. D., and Enoka, R. M. (2002). Task differences with the same load torque alter the endurance time of submaximal fatiguing contractions in humans. J. Neurophysiol. 88, 3087–3096. doi: 10.1152/jn.00232.2002
Janardhan, V., and Bakshi, R. (2002). Quality of life in patients with multiple sclerosis: the impact of fatigue and depression. J. Neurol. Sci. 205, 51–58. doi: 10.1016/S0022-510X(02)00312-X
Keller-Ross, M. L., Pereira, H. M., Pruse, J., Yoon, T., Schindler-Delap, B., Nielson, K. A., et al. (2014). Stressor-induced increase in muscle fatigability of young men and women is predicted by strength but not voluntary activation. J. Appl. Physiol. 116, 767–778. doi: 10.1152/japplphysiol.01129.2013
Kluger, B. M., Krupp, L. B., and Enoka, R. M. (2013). Fatigue and fatigability in neurologic illnesses: proposal for a unified taxonomy. Neurology 80, 409–416. doi: 10.1212/WNL.0b013e31827f07be
Koch, M., Mostert, J., and Heersema, D. (2007). Tremor in multiple sclerosis. J. Neurol. 254, 133–145. doi: 10.1007/s00415-006-0296-7
Kos, D., Nagels, G., D’Hooghe, M. B., Duportail, M., and Kerckhofs, E. (2006). A rapid screening tool for fatigue impact in multiple sclerosis. BMC Neurol. 6:27. doi: 10.1186/1471-2377-6-27
Krupp, L. B., Alvarez, L. A., LaRocca, N., and Scheinberg, L. (1988). Fatigue in multiple sclerosis. Arch. Neurol. 45, 435–437. doi: 10.1001/archneur.1988.00520280085020
Lorist, M. M., Kernell, D., Meijman, T. F., and Zijdewind, I. (2002). Motor fatigue and cognitive task performance in humans. J. Physiol. 545, 313–319. doi: 10.1113/jphysiol.2002.027938
Loy, B. D., Taylor, R. L., Fling, B. W., and Horak, F. B. (2017). Relationship between perceived fatigue and performance fatigability in people with multiple sclerosis: a systematic review and meta-analysis. J. Psychosom. Res. 100, 1–7. doi: 10.1016/j.jpsychores.2017.06.017
Lublin, F. D., and Reingold, S. C. (1996). Defining the clinical course of multiple sclerosis: results of an international survey. Neurology 46, 907–911. doi: 10.1212/WNL.46.4.907
Monks, J. (1989). Experiencing symptoms in chronic illness: fatigue in multiple sclerosis. Int. Disabil. Study 11, 78–83. doi: 10.3109/03790798909166394
Morrow, S. A., Weinstock-Guttman, B., Munschauer, F. E., Hojnacki, D., and Benedict, R. H. (2009). Subjective fatigue is not associated with cognitive impairment in multiple sclerosis: cross-sectional and longitudinal analysis. Mult. Scler. 15, 998–1005. doi: 10.1177/1352458509106213
Mottram, C. J., Jakobi, J. M., Semmler, J. G., and Enoka, R. M. (2005). Motor-unit activity differs with load type during a fatiguing contraction. J. Neurophysiol. 93, 1381–1392. doi: 10.1152/jn.00837.2004
Penner, I. K., and Paul, F. (2017). Fatigue as a symptom or comorbidity of neurological diseases. Nat. Rev. Neurosci. 13, 662–675.
Pereira, H. M., Spears, V. C., Schlinder-Delap, B., Yoon, T., Nielson, K. A., and Hunter, S. K. (2015). Age and sex differences in steadiness of elbow flexor muscles with imposed cognitive demand. Eur. J. Appl. Physiol. 115, 1367–1379. doi: 10.1007/s00421-015-3113-0
Roth, R. M., Isquith, P. K., and Gioia, G. A. (2005). Behavioral Rating Inventory of Executive Function—Adult Version. Lutz, FL: Psychological Assessment Resources, Inc.
Rudroff, T., Barry, B. K., Stone, A. L., Barry, C. J., and Enoka, R. M. (2007). Accessory muscle activity contributes to the variation in time to task failure for different arm postures and loads. J. Appl. Physiol. 102, 1000–1006. doi: 10.1152/japplphysiol.00564.2006
Rudroff, T., Kalliokoski, K. K., Block, D. E., Gould, J. R., Klingensmith, W. C., and Enoka, R. M. (2013). PET/CT imaging of age- and task-associated differences in muscle activity during fatiguing contractions. J. Appl. Physiol. 114, 1211–1219. doi: 10.1152/japplphysiol.01439.2012
Severijns, D., Zijdewind, I., Dalgas, U., Lamers, I., Lismont, C., and Feys, P. (2017). The assessment of motor fatigability in persons with multiple sclerosis: a systematic review. Neurorehabil. Neural Repair 31, 413–431. doi: 10.1177/1545968317690831
Steens, A., de Vries, A., Hemmen, J., Heersema, D. J., Mauritz, N., and Zijdewind, I. (2012a). Fatigue perceived by multiple sclerosis patients is associated with muscle fatigue. Neurorehabil. Neural Repair 26, 48–57. doi: 10.1177/1545968311416991
Steens, A., Heersema, D. J., Maurits, N. M., Renken, R. J., and Zijdewind, I. (2012b). Mechanisms underlying muscle fatigue differ between multiple sclerosis patients and controls: a combined electrophysiological and neuroimaging study. Neuroimage 59, 3110–3118. doi: 10.1016/j.neuroimage.2011.11.038
Stern, Y. (2009). Cognitive reserve. Neuropsychologica 47, 2015–2028. doi: 10.1016/j.neuropsychologia.2009.03.004
Sumowski, J. F., Chiaravalloti, N., and DeLuca, J. (2009a). Cognitive reserve protects against cognitive dysfunction in multiple sclerosis. J. Clin. Exp. Neuropsyc. 31, 913–926. doi: 10.1080/13803390902740643
Sumowski, J. F., Chiaravalloti, N. D., Wylie, G. R., and DeLuca, J. (2009b). Cognitive reserve moderates the negative effect of brain atrophy on cognitive efficiency in multiple sclerosis. J. Int. Neuropsych. Soc. 15, 606–612. doi: 10.1017/S1355617709090912
Sumowski, J. F., Wylie, G. R., DeLuca, J., and Chiaravalloti, N. (2010). Intellectual enrichment is linked to cerebral efficiency in multiple sclerosis: functional magnetic resonance imaging evidence for cognitive reserve. Brain 133, 362–374. doi: 10.1093/brain/awp307
Tartaglia, M. C., Narayanan, S., and Arnold, D. L. (2008). Mental fatigue alters the pattern and increases the volume of cerebral activation required for a motor task in multiple sclerosis patients with fatigue. Eur. J. Neurol. 15, 413–419. doi: 10.1111/j.1468-1331.2008.02090.x
Thompson, C. K., Negro, F., Johnson, M. D., Holmes, M. R., McPherson, L. M., Powers, R. K., et al. (2018). Robust and accurate decoding of motoneuron behavior and prediction of the resulting force output. J. Physiol. 596, 2643–2659. doi: 10.1113/JP276153
Trapp, B. D., and Nave, K. A. (2008). Multiple sclerosis: an immune or neurodegenerative disorder? Annu. Rev. Neurosci. 31, 247–269. doi: 10.1146/annurev.neuro.30.051606.094313
Vanden Noven, M. L., Pereira, H. M., Yoon, T., Stevens, A. A., Nielson, K. A., and Hunter, S. K. (2014). Motor variability during sustained contractions increases with cognitive demand in older adults. Front. Aging Neurosci. 6:97. doi: 10.3389/fnagi.2014.00097
Wechsler, D. (1999). Wechsler Abbreviated Scale of Intelligence. San Antonio, TX: Psychological Corporation.
Wolkorte, R., Heersema, D. J., and Zijdewind, I. (2015). Reduced dual-task performance in MS patients is further decreased by muscle fatigue. Neurorehabil. Neural Repair 29, 424–435. doi: 10.1177/1545968314552529
Wolkorte, R., Heersema, D. J., and Zijdewind, I. (2016). Reduced voluntary activation during brief and sustained contractions of a hand muscle in secondary-progressive multiple sclerosis patients. Neurorehabil. Neural Repair 30, 307–316. doi: 10.1177/1545968315593809
Zijdewind, I., Prak, R. F., and Wolkorte, R. (2016). Fatigue and fatigability in persons with multiple sclerosis. Exerc. Sport Sci. Rev. 44, 123–128. doi: 10.1249/JES.0000000000000088
Keywords: multiple sclerosis, fatigue, performance fatigability, perceived exertion, force steadiness, EMG, cognitive reserve
Citation: Gould JR, Reineberg AE, Cleland BT, Knoblauch KE, Clinton GK, Banich MT, Corboy JR and Enoka RM (2018) Adjustments in Torque Steadiness During Fatiguing Contractions Are Inversely Correlated With IQ in Persons With Multiple Sclerosis. Front. Physiol. 9:1404. doi: 10.3389/fphys.2018.01404
Received: 04 May 2018; Accepted: 14 September 2018;
Published: 17 October 2018.
Edited by:
Inge Zijdewind, University Medical Center Groningen, NetherlandsReviewed by:
Christopher Thompson, Temple University, United StatesStuart Goodall, Northumbria University, United Kingdom
Manda Linea Keller-Ross, University of Minnesota Twin Cities, United States
Copyright © 2018 Gould, Reineberg, Cleland, Knoblauch, Clinton, Banich, Corboy and Enoka. This is an open-access article distributed under the terms of the Creative Commons Attribution License (CC BY). The use, distribution or reproduction in other forums is permitted, provided the original author(s) and the copyright owner(s) are credited and that the original publication in this journal is cited, in accordance with accepted academic practice. No use, distribution or reproduction is permitted which does not comply with these terms.
*Correspondence: Jeffrey R. Gould, Z291bGRqQGNvbG9yYWRvLmVkdQ== Roger M. Enoka, ZW5va2FAY29sb3JhZG8uZWR1