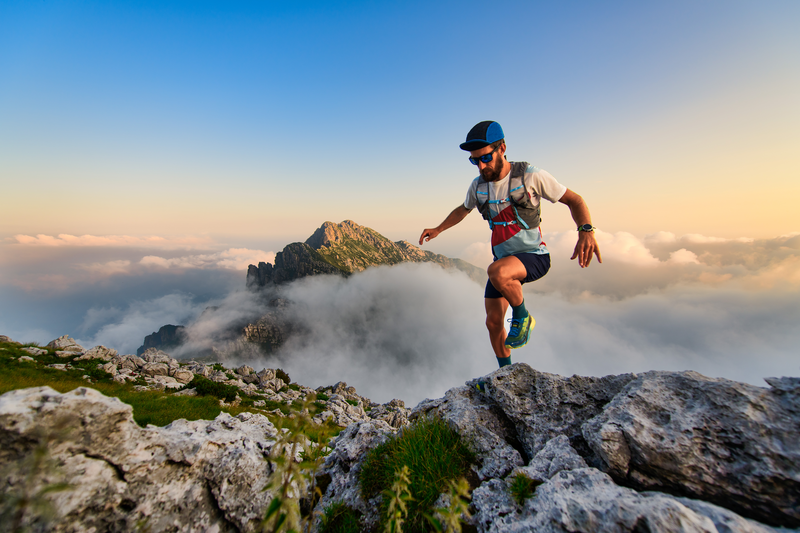
95% of researchers rate our articles as excellent or good
Learn more about the work of our research integrity team to safeguard the quality of each article we publish.
Find out more
ORIGINAL RESEARCH article
Front. Physiol. , 17 July 2018
Sec. Aquatic Physiology
Volume 9 - 2018 | https://doi.org/10.3389/fphys.2018.00924
Recently, the crustacean female sex hormone (CFSH), which is considered a female-specific hormone, has been shown to play a crucial role in female phenotypes in crustaceans. In this study, two transcripts (Sp-CFSH1 and Sp-CFSH2) encoding the same CFSH precursor were cloned from the mud crab Scylla paramamosain. Homology and phylogenetic analysis showed that CFSHs were homologous to interleukin-17 and highly conserved among brachyuran crabs. PCR analysis revealed that Sp-CFSH was expressed exclusively in the eyestalk ganglion of both prepubertal males and females, and surprisingly, the abundance of Sp-CFSH transcripts detected in the males were not significantly different from that of the females (P > 0.05). In addition, mRNA in situ hybridization showed that Sp-CFSH was localized in the X-organ of the male eyestalk ganglion. During the development of the androgenic gland (AG), the level of Sp-IAG mRNA in AG remained at low levels from stages I to II (early stage) but had a significant increase at stage III (mature stage). In contrast, the level of Sp-CFSH transcripts in the eyestalk ganglion was high in the early stage but extremely low in the mature stage. To investigate the potential function of CFSH in male S. paramamosain, the recombinant protein (∼20 kDa) was expressed in Escherichia coli and was subsequently added to AG explants in vitro. It was demonstrated that recombinant Sp-CFSH protein significantly reduced the expression of Sp-IAG in the AG explants at a concentration of 10−6 M (P < 0.05). In conclusion, our study provides the first piece of evidence that shows CFSH from the eyestalk ganglion acts as a negative regulator inhibiting the development of AG in crustaceans.
In the animal kingdom, most species have separate genders, which often can be distinguished by different sexual characteristics relating to male and female in gonads, the genital tract, or the external genitalia (Nagaraju, 2011). Under the control of chromosome composition and/or other factors, the primitive gonad in the embryo differentiates into testis or ovary, and the secondary male or female characteristics gradually appear with ontogenetic development (Hughes, 2001; Campos-Ramos et al., 2006). For brachyuran crabs, gender could be identified based on the secondary sexual features at the juvenile crab stage, such as the differentiations in the gonopore, pleopods, and abdominal shape (Lee et al., 1994; Nagaraju, 2011). For example, in the crab Eriocheir japonicus, the first pair of pleopods in males starts to appear at the third juvenile crab stage, but the third to fifth pleopods degenerate in males, while the second to fifth pleopods continue to develop and branch into two podites in females at the fourth juvenile crab stage (Lee et al., 1994).
In crustaceans, male sex differentiation is largely controlled by the androgenic gland (AG), an organ unique to males (Charniaux-Cotton, 1992). The function of this gland was first reported in the amphipod Orchestia gammarellus (Charniaux-Cotton, 1954). Thereafter, the androgenic gland hormone (AGH), also termed AG-specific insulin-like peptide (IAG), and the cDNA of IAG were isolated and characterized from the terrestrial isopod Armadillidium vulgare (Okuno et al., 1997, 1999). By removing AG or by the silencing of IAG gene from males, as well as by AG implantation or injection of AG extracts into females, the influence of AG/IAG on primary and secondary sex characteristics has been verified in various crustacean species (reviewed by Khalaila et al., 2001; Sagi and Khalaila, 2001; Barki et al., 2003; Manor et al., 2004; Rosen et al., 2010; Ventura et al., 2011b). For instance, in the giant freshwater prawn Macrobrachium rosenbergii, removing AG from juvenile males resulted in sex reversal (Sagi et al., 1997). Furthermore, silencing of the Mr-IAG led to the hypertrophy of AG and the arrest of testicular spermatogenesis (Ventura et al., 2009). It has also been shown that the expression of Mr-IAG could be detected at the postlarval stage; during the subsequent development, the pubertal males still required IAG to develop into reproductive-competent adult males (Ventura et al., 2011a).
In crustacean males, embryonic gonads develop into testes under the control of AG; on the other hand, embryonic gonads develop into ovaries in females without the influence of a well-defined equivalent gland (Katakura, 1989). However, the mechanisms underlying the regulation of female sex differentiation and sexual characteristics maintenance remain unclear. Recently, Zmora and Chung (2013) isolated a female specific hormone, named crustacean female sex hormone (CFSH), from the eyestalk of the female blue crab (Callinectes sapidus) and demonstrated that it played a crucial role in the development of female reproductive phenotypes. It was shown that the silencing of CFSH or bilateral eyestalk ablation impaired the mating and maternal care structures of females, such as absent or misplaced gonopores, sharper abdomens, as well as shorter and fewer setae on pleopods (Zmora and Chung, 2013). However, the absence of CFSH neither resulted in ovarian degeneration nor sex reversal; therefore, CFSH appears to be responsible for sex differentiation rather than sex determination (Zmora and Chung, 2013; Adkins-Regan, 2014). The discovery of CFSH suggested that AGH was not the sole sex differentiation hormone in crustaceans, and like males, female crustaceans may also require a feminizing hormone to reach sexual maturity (Adkins-Regan, 2014).
The endocrine axis of the eyestalk – AG – testis has been established in male crustaceans, in which AG has been shown to be regulated by the negative control of the eyestalk (Khalaila et al., 2002). For example, in the spot shrimp Pandalus platyceros and the swimming crab Portunus pelagicus, the hypertrophic AG and the increased numbers of IAG-producing cells were observed in males that had bilateral eyestalks removed (Hoffman, 1968; Sroyraya et al., 2010). Likewise, in the mud crab Scylla paramamosain and the blue crab C. sapidus, eyestalk ablation resulted in the up-regulation of IAG expression in AG (Chung et al., 2011; Zhang et al., 2014). These previous studies all demonstrated that the activity of AG was inhibited by the specific substance(s) derived from the eyestalk; however, the exact mechanism(s) responsible for this inhibition is still unknown (Khalaila et al., 2002).
To date, only a few CFSHs have been isolated from crustacean species, and little is known about the functions of this newly discovered hormone. In C. sapidus, CFSH was considered as a female-specific hormone, although CFSH was also detected in the eyestalk of the mature male C. sapidus at extremely low levels (Zmora and Chung, 2013). The presence of CSFH in the male eyestalk may indicate that CFSH also has a role to play in males. It is therefore interesting to explore whether CFSH is also present in males of other crab species. If yes, could it also be involved in regulating male sex differentiation, governed principally by AG/IAG.
In this study, we investigated a putative function of CFSH in male mud crab S. paramamosain. We firstly cloned two transcripts of Sp-CFSH from the eyestalk ganglion of S. paramamosain and found that Sp-CFSH had high expression in prepubertal males. Based on the expression profiles of Sp-CFSH and Sp-IAG during the development of AG, we hypothesized that CFSH might act as an inhibitory factor suppressing the development of AG. To verify this hypothesis, we cloned and purified the recombinant Sp-CFSH protein (rCFSH) from the prokaryotic expression system and subsequently added it into AG explants to vindicate the role of CFSH in inhibiting Sp-IAG expression.
The mad crabs (S. paramamosain) used for the experiments were obtained from a local fish market in Xiamen, Fujian, China. They were maintained in rectangular tanks with seawater at a temperature of 27 ± 2°C and a salinity of 28 ± 0.5 ppt for a week, during which they were fed with the clam Ruditapes philippinarum. Before tissue dissection, prepubertal crabs (females: carapace width 6.8 ± 0.5 cm, body weight 103.2 ± 4.3 g; males: carapace width 7.4 ± 0.5 cm, body weight 110.3 ± 3.2 g) at intermolt stage were placed on ice for anesthetization (n = 6). Various tissues including eyestalk ganglion, thoracic ganglion, cerebral ganglion, hepatopancreas, hemocytes, muscle, heart, stomach, gill, epidermis, Y-organ, mandibular organ, and gonad (ovary or testis) were then collected for tissue distribution analysis.
The development of AG in S. paramamosain can be classified into four stages (Ye et al., 2003; Liu et al., 2008): At stage I, the gland is short and the glandular cells are of small amounts. At stage II, the gland distinctively takes on a cord-figure. At stage III, the volume of the gland reaches its peak, with hyperplasia in some portion. At stage IV, the gland no longer grows and degenerate rapidly. Eyestalk ganglia and AGs from male crabs (n = 6) at stage I (carapace width 6.1 ± 0.5 cm, body weight 45.2 ± 4.3 g), stage II (carapace width 9.3 ± 0.5 cm, body weight 145.6 ± 10.5 g), and stage III (carapace width 14.3 ± 0.5 cm, body weight 245.8 ± 12.5 g) were collected for Sp-CFSH and Sp-IAG expression (GenBank accession number: KJ870255) profiles analysis. Tissues were immediately frozen in liquid nitrogen, and stored at −80°C until total RNA extraction.
Total RNA was extracted from the eyestalk ganglion of a mature female via TRIzol® reagent (Invitrogen). The first-strand cDNA was generated with 1 μg total RNA using RevertAid First Strand cDNA Synthesis Kit (Fermentas). Degenerate primers CFSH-DF1 and CFSH-DR1 were designed based on the CFSH sequences of C. sapidus (GenBank accession number: GU016328.1) and Carcinus maenas (GenBank accession number: HM594946.1). The PCR reaction was performed in the ABI 2720 Thermal Cycler (Applied Biosystems) with Ex-Taq polymerase (Takara) under the following conditions: 95°C for 3 min; 35 cycles of 95°C for 30 s, 58°C for 30 s and 72°C for 50 s, followed by 72°C for 10 min final extension. After the amplification, the products were visually examined by 1.0% agarose gel. Subsequently, the fragment of Sp-CFSH was purified and then inserted into PMD19-T vector (Takara) for sequencing.
To obtain the full length cDNA of Sp-CFSH, 5′ and 3′ rapid amplification cDNA ends (RACE) were performed with SMARTTM RACE cDNA Amplification Kit (Clontech) according to the manufacturer’s instructions. The 5′-RACE cDNA template was first amplified with primers CFSH5R1 and the universal primer, and then a nested PCR was performed with the dilute amplicon by primer CFSH5R2 and the short universal primer in the same conditions. The 3′-ends of the sequences were obtained by using 3′-RACE cDNA with universal primer and CFSH3F1, and nested amplification with nested universal primer and CFSH3F2. The two rounds of 3′-RACE-PCR were performed following the conditions of 5′-RACE-PCR. All PCR products were extracted using a 1.0% agarose gel and then subjected to sequence as described above. Finally, the sequences were assembled and its homology was examined using the Blast N. The primers were all listed in Supplementary Table 1.
The open reading frame (ORF) was predicted by the ORF finder1. The signal peptide was predicted by Signal P4.02. The deduced CFSH amino acid sequences of crustacean species were arranged using Clustal X software. The phylogenetic tree was constructed by the maximum likelihood method with MEGA6 software.
Total RNA was extracted from eyestalk ganglion and other tissues (including thoracic ganglion, cerebral ganglion, hepatopancreas, hemocytes, muscle, heart, epidermis, stomach, Y-organ, mandibular organ, gill, and gonad). After being treated with DNase I (Fermentas) at 37°C for 30 min, 1 μg total RNA was reverse-transcribed with primer oligo-dT using a RevertAid First Strand cDNA Synthesis Kit (Fermentas). Tissues expression profiles were examined with a semi-quantitative PCR assay. Arginine kinase (AK, GenBank accession number: JQ031765), a housekeeping gene, was used as an internal control. The PCR was carried out with primer pairs CFSH-QF/-QR and AK-QF/-QR (Supplementary Table 1), respectively, as the following conditions: 94°C for 3 min, 35 cycles of 94°C for 30 s, 58°C for 30 s, and 72°C for 30 s, final extension 72°C for 10 min. PCR products were examined by 1.0% agarose gel and visualized by using ethidium bromide (EB).
For mRNA in situ hybridization, a pair of gene-specific primer, CFSH-IF and CFSH-IR (Supplementary Table 1), was used for the generation of antisense and sense RNA probes. Digoxigenin (DIG) labeled RNA probes were synthesized via the T7 RNA polymerase (Roche). Based on the tissue expression profiles of Sp-CFSH, eyestalk ganglia from male S. paramamosain were dissected, and then fixed in 4% paraformaldehyde. After serial dehydration and other treatments, fixed tissues were embedded in paraffin and then sectioned with a thickness of 7 μm, which were prepared for hybridization using the protocol as stated by Shechter et al. (2005). Hybridization was carried out according to the manufacturer’s protocol of DIG RNA Labeling Kit (SP6/T7, Roche, Switzerland).
The fragment encoding the mature hormone of Sp-CFSH was obtained by PCR with primers CFSH-EF and CFSH-ER (Supplementary Table 1) containing two restriction enzyme sites, BamH I and Spe I, and then sub-cloned into the prokaryotic expression vector pET-His. Subsequently, the recombinant plasmid generated was introduced into the host BL21 (DE3) E. coli by transformation for protein expression. Transformed cells were cultured at 37°C in LB medium with 50 μg/ml ampicillin. When OD600 reached to 0.6, isopropyl-β-D-thiogalactoside (IPTG) was added to a final concentration of 0.8 mM and cells were cultured for another 8 h at 18°C for protein expression. After harvest by centrifugation, cell pellets were resuspended in binding buffer (20 mM sodium phosphate, 500 mM NaCl, and 20 mM imidazole; pH 8.0) and dissociated by ultrasonic wave. The soluble fraction and insoluble fraction were separated by centrifugation at 12,000 × g for 15 min at 4°C and analyzed by 15% SDS–PAGE. The precipitate was harvested and resuspended in binding buffer containing 8 M urea, loaded on an Ni-NTA HisTrapTM FF crude column (GE Healthcare) according to the manufacturer’s instructions, and then washed with 10 ml of the elution buffer (20 mM sodium phosphate, 500 mM NaCl, and 8 M urea) containing 50 mM imidazole, 10 ml of the elution buffer containing 100 mM imidazole, and 5 ml of the elution buffer containing 200 mM imidazole in order. The rCFSH was eluted with 6 ml of the elution buffer containing 300 mM imidazole. Eluting solution were collected and examined by 15% SDS–PAGE. After that, purified rCFSH was renatured using the measure of graded urea dialysis and confirmed by Western blotting. For immunoblotting analysis, the electrophoresed proteins were transferred to a PVDF membrane and analyzed as previously described by Imjongjirak et al. (2005). Finally, the renatured protein was stored at -20°C until use.
The biological activity of rCFSH for inhibiting AG was determined by an in vitro experiment. The male crabs (body weight: 168.5 ± 3.5 g; carapace width: 10.3 ± 0.5 cm) were anesthetized on ice for 10 min and sterilized in 75% ethanol for 10 min. AG which is attached to spermaduct were immediately dissected and washed gently by crab saline with antibiotics penicillin G (300 IU/ml) and streptomycin (300 mg/ml) for five times. The AGs were placed in a 24-well cultured plate with 400 μl L15 medium containing antibiotics right after being separated from spermaduct and pre-cultured for an hour. The experiment was divided into two groups: with rCFSH at a final concentration of 10−6 M as experimental group and without rCFSH as control group, each group has four repeats (n = 4). Finally, AG samples were incubated for another 12 h and RNA was isolated for qRT-PCR assay.
The qRT-PCR was used to determine the relative expression of Sp-CFSH in the eyestalk ganglion and Sp-IAG in the AG, respectively. The cDNA samples were generated using TransScript® II One-Step gDNA Removal and cDNA Synthesis SuperMix (TransGen Biotech) according to the manufacturer’s protocol. 1 μg total RNA was reverse-transcribed with random primer and the cDNAs generated were diluted four times for qRT-PCR analysis. The reaction system of the qRT-PCR in this study was 20 μl in volume containing 10 μl 2× PCR Master Mix with SYBR Green, 2 μl dilute cDNA, 0.8 μl each primer (1 mM), and 6.5 μl water. The reaction was performed with 7500 Fast Real-Time PCR (Applied Biosystems) under the following conditions: 94°C for 10 min followed by 40 cycles of 94°C for 30 s, 58°C for 30 s, 72°C for 30 s, and a final extension 72°C for 10 min. The reactions were completed in triplicate and normalized to the internal control gene AK. Primers used for qRT-PCR were all listed in Supplementary Table 1.
We calculated the qRT-PCR data using the 2−ΔΔCt method, and then performed statistical analysis. Levene’s test was used for homoscedasticity and statistical significance of the data (P < 0.05) was determined using one-way analysis of variance (ANOVA) with post Duncan multiple comparison test (SPSS 20.0). The results were presented as mean ± SEM.
Two transcripts of CFSH, named Sp-CFSH1 (GenBank accession number: MF489232) and Sp-CFSH2 (GenBank accession number: MF489233), were cloned from the eyestalk ganglia of S. paramamosain by using PCR with degenerate primers, followed by 5′- and 3′-RACE. It was found that the nucleotide sequences of Sp-CFSH1 (1032 bp) and Sp-CFSH2 (916 bp) were identical in 5′-UTR and ORF but different in 3′-UTR (Figure 1A), which could be translated into a same hormone (223-aa). The predicted CFSH contained a 23-aa signal peptide, a 33-aa CFSH precursor-related peptide, a dibasic cleavage site (KR), and a 167-aa mature CFSH (Figure 2) in order, suggesting that it was synthesized as a preprohormone. There were eight cysteine residues that formed four putative intramolecular disulfide bridges in the mature CFSH: C46-150, C80-112, C105-119, and C107-148 (Figure 1B).
FIGURE 1. (A) The nucleotide and deduced amino acid sequence of Sp-CFSH1 and Sp-CFSH2 from the mud crab S. paramamosain. The ORF is shown in a single letter code below the nucleotide sequence. The CFSH precursor-related peptide is boxed, the dibasic cleavage site (KR) and eight cysteine residues are indicated in red. The mature peptide and the microsatellites in the 3′-UTR are underlined. (B) A schematic diagram of prepro-CFSH. Signal peptide (blue bar), CFSH precursor-related peptide (green bar), a dibasic cleavage site KR (red bar), and the mature hormone (black bar). The eight cysteine residues are predicted to form four putative disulfide bridges connected with lines (C46-150, C80-112, C105-119, and C107-148).
The amino acid sequences of Sp-CFSH share 95 and 90% identity with that of CFSH in C. sapidus and C. maenas, respectively. Phylogenetic analysis revealed that CFSH is a highly conserved hormone among brachyuran crabs (Figures 2, 3). The mature hormones of CFSH, as well as CFSH-like, were determined to contain an interleukin-17 (IL-17) domain, indicating that they belonged to a new protein family homologous to the IL-17 family in vertebrates (Figure 2). In decapod crustaceans, CFSH can be classified into two types (Figure 3).
FIGURE 2. Sequence alignment of deduced amino acid sequences of CFSH cDNAs of brachyuran crabs. Note the conservation of CFSH in brachyuran crabs. Conserved residues are indicated by the black highlights. The signal peptide is highlighted by a black box. The cleavage site (KR) and the domain IL-17 are boxed in red.
FIGURE 3. Phylogenetic tree of amino acid sequences of the CFSH in decapod crustaceans. The tree was built by the maximum likelihood (ML) method. Callinectes sapidus (GenBank accession number: GU016328.1), Carcinus maenas (GenBank accession number: HM594946.1), and the other sequences of CFSH were obtained from (Veenstra, 2016).
Tissue distribution was determined by PCR with sequence specific primer pairs. The cDNAs of various tissues used for Sp-CFSH expression analysis were from prepubertal females and males. This revealed that Sp-CFSH was exclusively expressed in the eyestalk ganglia of both sexes of S. paramamosain (Figure 4A). Unexpectedly, Sp-CFSH was found to be highly expressed in the eyestalk ganglia of males, which was not significantly different from that of females (P > 0.05) (Figure 4B).
FIGURE 4. (A) Tissue distribution of Sp-CFSH in both sexes of prepubertal mud crab S. paramamosain. The analysis was generated by PCR assays with cDNAs from various tissues of both sexes and compared with arginine kinase (AK) gene. 1, eyestalk ganglion; 2, thoracic ganglion; 3, cerebral ganglion; 4, stomach; 5, ovary or testis; 6, hepatopancreas; 7, heart; 8, hemocytes; 9, muscle; 10, Y-organ; 11, mandibular organ; 12, gill; 13, epidermis; and 14, negative control was shown with the amplification of water. (B) The relative expression of Sp-CFSH between male and female S. paramamosain. Data are shown as means ± SEM of six separate individuals (n = 6).
The eyestalk ganglia can be divided into lamina ganglionaris (LG), medulla externa (ME), medulla interna (MI), and medulla terminalis (MT) (Figure 5E). The X-organ is located at the MT (Figure 5E). The antisense Sp-CFSH probers gave a clear signal in cytoplasm of neuroendocrine cells in the X-organ of male (Figures 5A–C). No signal was observed when a sense probe was used as a negative control (Figure 5D).
FIGURE 5. Localization of Sp-CFSH-expressing cells in the eyestalk ganglia of male S. paramamosain. (A–C) Localization of Sp-CFSH mRNA by the antisense probe. Arrows indicate the positive signals of Sp-CFSH. (D) Negative control result with the sense probe. (E) Hematoxylin and eosin staining. LG, lamina ganglionaris; ME, medulla externa; MI, medulla interna; MT, medulla terminalis.
The expression profiles of Sp-CFSH and Sp-IAG were demonstrated by qRT-PCR. Based on the definition of AG development (Ye et al., 2003; Liu et al., 2008), Sp-CFSH had a high expression at stage I, but decreased gradually from stage II onward, and its expression level was extremely low at stage III (mature stage) of AG development (Figure 6A). On the contrary, the level of Sp-IAG transcript in the AG increased during the development of AG and reached the peak at stage III, almost 40-fold higher than the level detected at stage I (P < 0.001) (Figure 6B). This result was in accordance with the histological results that showed the volume of AG, as well as the number of endocrine cells, increased substantially at stage III compared with stage I and stage II (Figures 6C–E).
FIGURE 6. Expression patterns of Sp-CFSH (A) and Sp-IAG (B), and histological characterization of the androgenic gland (C–E) at three different development stages of male S. paramamosain. Data are shown as means ± SEM of six separate individuals (n = 6). Asterisks ∗∗∗ (P < 0.001) on the top of the error bars indicate highly significant differences from stage I. (C) Stage I; (D) stage II; (E) stage III. SD, sperm duct; AG, androgenic gland.
The predicted molecular mass of mature CFSH was 18.61 kDa. The rCFSH was expressed with pET-His plasmid as an N-terminal His-tagged fusion protein in the prokaryotic expression system, which increased the molecular weight of the fusion protein to approximately 20 kDa. The whole cell lysate of E. coli BL21 with recombinant plasmid, induced with IPTG, in addition to the soluble and insoluble fractions were analyzed by 15% SDS–PAGE (Supplementary Figure 1A). It showed that the recombinant protein of Sp-CFSH (∼20 kDa) was expressed after IPTG induction for 8 h. In contrast to the supernatant, the fusion protein was mostly expressed in the precipitate of ultrasonically broken E. coli BL21 (DE3). High-purity protein products were obtained after using Ni-NTA column affinity chromatography at 300 mM imidazole concentration (Supplementary Figure 1B). By detecting with prepared anti-His-tag rabbit antibody, the purified recombinant protein, after renaturation, was confirmed by Western blotting; it showed that the antibody could specifically bind to the purified protein (Supplementary Figure 1C). Finally, the renatured protein was obtained by graded urea dialysis.
Using the level of Sp-IAG transcript as an indicator of AG development, the inhibitory effect of rCFSH on the development of AG was vindicated. The result showed that the Sp-IAG transcripts were 60% inhibited when rCFSH was added into the culture medium at a final concentration of 10−6 M, which was significantly different from the control (P < 0.05) (Figure 7).
FIGURE 7. Sp-IAG mRNA level in the AG explants after treatment with recombinant Sp-CFSH protein (rCFSH). Data are shown as means ± SEM of four separate repeats (n = 4). Asterisks (P < 0.05) on the top of the error bar indicates significant difference from the control group.
In crustaceans, studies of sexual differentiation were mainly focused on AG in males (Ventura et al., 2011b). The recent discovery that CFSH was responsible for regulating the development of female phenotypes in the blue crab C. sapidus is a major breakthrough (Zmora and Chung, 2013). To date, although the full length of CFSH was merely characterized from two crab species, C. sapidus and C. maenas, CFSH sequences were identified in several other brachyuran crabs, such as Portunus trituberculatus (Veenstra, 2015, 2016) and Eriocheir sinensis (Liu et al., 2015; Veenstra, 2016). Moreover, CFSH homologies were also found in shrimps and freshwater crayfish, including Fenneropenaeus merguiensis (Powell et al., 2015), Sagmariasus verreauxi (Ventura et al., 2014), Procambarus clarkii (Veenstra, 2015), M. rosenbergii (Suwansa-ard et al., 2015; Veenstra, 2016), and Litopenaeus vannamei (Veenstra, 2016). In the present study, we cloned two transcripts of CFSH, Sp-CFSH1 and Sp-CFSH2, from the eyestalk ganglion of S. paramamosain. The nucleotide sequence of these two transcripts were the same in 5′-UTR and ORF, but different in 3′-UTR (Figure 1A). Sp-CFSH1 (1032 bp) was longer than Sp-CFSH2 (916 bp) and the identified CFSH in C. sapidus (927 bp) (Zmora and Chung, 2013). The difference in the length of 3′-UTR implied potential regulation at the translational level in crustaceans (Manor et al., 2007; Ventura et al., 2009). Alternative gene splicing might be responsible for the presence of two transcripts of CFSH in S. paramamosain. This phenomenon is similar to the findings of IAGs in C. sapidus and F. chinensis (Li et al., 2012; Chung, 2014). Microsatellite repeat sequences were found in the 3′-UTR of both Sp-CFSH1 and Sp-CFSH2 (Figure 1A). It has been reported that microsatellites in the 3′-UTRs can cause transcription slippage and influence the stability of mRNAs (Ruggiero et al., 2003; Li et al., 2004). However, the functional importance of these repeats is unknown and varies depending on the species (Li et al., 2004). It would be interesting to understand the precise regulation of these microsatellites in 3′-UTR of Sp-CFSH. Clearly it warrants further research.
Crustacean female sex hormone homologies were firstly named by Veenstra (2016), which were classified into two types. Here we reanalyzed these sequences and subsequently renamed the homologous proteins as CFSH-like, based on the IL-17 domain. Interestingly, another CFSH-like protein was found in E. sinensis and three CFSH-like proteins were found in coexistence in P. clarkii, M. rosenbergii, and L. vannamei, respectively (Figure 3). It appears common that this gene have several paralogs in crustacean species (Veenstra, 2016). The primary structure of CFSH and CFSH-like is conserved, which all contain a precursor-related peptide and an IL-17 domain in the mature hormone. The identification of the IL-17 domain in the mature hormone suggests that a new protein family homology to the IL-17 family may exist in crustaceans. Up to now, the function of this new protein family in crustaceans is unknown except CFSH. In vertebrates, the IL-17 family is a group of potent proinflammatory cytokines produced by the activated memory T cells (Shabgah et al., 2014; Kumar et al., 2016). It has been reported that the IL-17 signaling pathway is involved in the innate and adaptive immunity of vertebrates. Recently, the involvement of IL-17 in immunity has also been documented in some invertebrates such as pearl oyster Pinctada fucata (Wu et al., 2013). Furthermore, cytokines, also known as immunoregulatory proteins, have been shown to affect the neuroendocrine events of reproduction, e.g., ovarian/testicular function (Ben-Rafael and Orvieto, 1992; Rutanen, 1993). It has been reported that IL-1 can stimulate testosterone secretion in adult male rat Leydig cells in vitro (Warren et al., 1990). Although structural similarity does not necessarily imply functional similarity, the IL-17 signaling pathway provides a clue to understand the CFSH signaling system.
In crustaceans, the X-organ appeared to be the only site of CFSH synthesis. In S. paramamosain, our result showed that Sp-CFSH was exclusively expressed in the eyestalk ganglion. This is the same as the previous study of C. sapidus, in which CFSH was specifically present in the X-organ-sinus gland of the eyestalk ganglion (Zmora and Chung, 2013). Similarly in S. verreauxi and F. merguiensis, CFSH-like was uniquely expressed in the eyestalk ganglion (Ventura et al., 2014; Powell et al., 2015). CFSH eyestalk specificity was further confirmed by the result of in situ hybridization of the present study, which showed that Sp-CFSH mRNA was localized in the neuroendocrine cells of X-organ (Figure 5), a result in agreement with the findings of an immunohistology study in C. sapidus by Zmora and Chung (2013). The eyestalk specificity of CFSH might explain observation in several crustaceans, such as the spider crab Libinia emarginata (Saigusa et al., 2002) and the blue crab C. sapidus (Zmora and Chung, 2013). Female eyestalk ablation at the prepuberty stage led to impaired mating and brooding systems.
Our result showed that Sp-CFSH was highly expressed in prepubertal male S. paramamosain, which was not significantly different from the levels found in females (Figure 4B). In S. verreauxi, CFSH-like was also found to be expressed in both male and female eyestalk ganglia at roughly same levels by comparative transcriptome analysis (Ventura et al., 2014). These results contradicted an earlier study that suggested CFSH was female specific: CFSH was reported to have a high expression in the eyestalk ganglia of adult females, but was extremely low in adult males in C. sapidus. Moreover, CFSH proteins were only isolated from female sinus gland extracts (Zmora and Chung, 2013). The difference probably was related to different development stages of crabs used in tissue distribution analyses in the two studies. As observed in this study, the level of Sp-CFSH was high in the prepubertal males but also extremely low in mature males (Figure 6A). In fact, the low expression in mature males, in our experiment, was similar to that of the adult male C. sapidus (Zmora and Chung, 2013). Unfortunately, the expression of CFSH in prepubertal C. sapidus males was not studied, so no comparison could be made. Nevertheless, our result clearly demonstrated that CFSH is not a female specific hormone.
AG and/or IAG have been shown to play a crucial role in regulating male sex differentiation. For example, in M. rosenbergii, the male morphotypic differentiation and gonad activity was positively related to the level of Mr-IAG (Ventura et al., 2011a), and the change of Mr-IAG transcript level was closely correlated with the development of AG (Phoungpetchara et al., 2011). In addition, AG ablation could turn males into neo-females (Aflalo et al., 2006), and the silencing of Mr-IAG in inmature males impeded spermatogenesis and the development of male secondary sexual characteristics (Ventura et al., 2009). In the present study, it was also revealed that both the volume of AG and the amount of endocrine cells increased substantially at stage III when compared to stages I and II (Figures 6C–E); correspondingly, the level of Sp-IAG transcripts rose sharply at stage III (P < 0.001) (Figure 6B).
In crustacean males, it is well known that AG is under the inhibitory control of the eyestalk ganglion, because eyestalk ablation can result in hypertrophy (Sroyraya et al., 2010). The AG inhibiting factor(s) produced by the eyestalk ganglion might be diverse. In the freshwater prawn Macrobrachium nipponense, it was reported that both gonad-inhibiting hormone (GIH) and molt-inhibiting hormone (MIH) had the inhibitory effects on IAG gene expression (Li et al., 2015). In S. paramamosain, eyestalk ablation of males (body weight, 150.5 ± 20.3 g) led to the up-regulation of Sp-IAG expression (Zhang et al., 2014), suggesting that Sp-IAG was also negatively regulated by the eyestalk-derived factor(s). In this study, Sp-CFSH was found to have the opposite expression tendency compared to Sp-IAG during the development of AG. That is, at the early stage, high expression of Sp-CFSH transcripts was detected in eyestalk ganglion (Figure 6A) whereas the expression of Sp-IAG transcripts was low in AG (Figure 6B). Therefore, we hypothesized that CFSH might suppress the development of AG at this early stage. To verify this, the recombinant CFSH (rCFSH) was obtained to assay its bioactivity in vitro and the result showed that the Sp-IAG expression transcripts in AG were reduced by about 60% by rCFSH, at a concentration of 10-6 M (Figure 7). Such a result demonstrates that CFSH generates an inhibitory effect on AG activity. Hence, our results suggest that CFSH might regulate male sex differentiation via AG.
Our result also showed that at the mature stage (stage III) of male S. paramamosain, the expression of Sp-CFSH was extremely low (Figure 6A), while the level of Sp-IAG transcript was high (Figure 6B). A possible explanation for this phenomenon could be that AG/IAG affected the expression of Sp-CFSH in eyestalk ganglion in reverse. In the red claw crayfish Cherax quadricarinatus, implantation of AG into immature females caused the inhibition of female secondary sex characteristics (Khalaila et al., 2001; Manor et al., 2004), especially the maternal brooding-related characteristics, namely the relative abdomen widths and the number of simple setae were significantly reduced (Barki et al., 2003); it is now known that the maternal brooding-related characteristics were largely controlled by CFSH (Zmora and Chung, 2013). Clearly, such an explanation is largely speculative, further proposed designed experiments, such as in vivo bioassay that can confirm whether AG/IAG impacts upon CFSH expression, are needed to test this hypothesis.
In summary, our study characterized two transcripts of Sp-CFSH from the eyestalk ganglion of the mud crab S. paramamosain. It appears for the first time that CFSH has been reported to be highly expressed in male crustaceans; and the inhibitory effect of CFSH on AG has been demonstrated. Furthermore, our research suggests the existence of putative antagonism between CFSH and IAG in male S. paramamosain, which warrants future investigation.
AL carried out the majority of the experiments and prepared the manuscript. JL and FL were involved in cDNA cloning, hybridization in situ, and statistical analysis. YH was involved in histological analysis, qRT-PCR analysis, data analysis, and GW was involved in the data analysis and preparation of the manuscript. HY designed the experiments, analyzed the results, and prepared the manuscript.
This study was supported by the National Natural Science Foundation of China (grant numbers 41476119 and 31772827) and the Xiamen Southern Oceanographic Center (grant number 14GZY56HJ26).
The authors declare that the research was conducted in the absence of any commercial or financial relationships that could be construed as a potential conflict of interest.
The authors would like to thank Dr. Chaoshu Zeng (James Cook University) for his assistance with English and the reviewers for their helpful suggestions on the manuscript.
The Supplementary Material for this article can be found online at: https://www.frontiersin.org/articles/10.3389/fphys.2018.00924/full#supplementary-material
Adkins-Regan, E. A. (2014). New hormone negates a default principle. Endocrinology 155, 10–11. doi: 10.1210/en.2013-2078
Aflalo, E. D., Hoang, T. T. T., Nguyen, V. H., Lam, Q., Nguyen, D. M., Trinh, Q. S., et al. (2006). A novel two-step procedure for mass production of all-male populations of the giant freshwater prawn Macrobrachium rosenbergii. Aquaculture 256, 468–478. doi: 10.1016/j.aquaculture.2006.01.035
Barki, A., Karplus, I., Khalaila, I., Manor, R., and Sagi, A. (2003). Male-like behavioral patterns and physiological alterations induced by androgenic gland implantation in female crayfish. J. Exp. Biol. 206, 1791–1797. doi: 10.1242/jeb.00335
Ben-Rafael, Z., and Orvieto, R. (1992). Cytokines—involvement in reproduction. Fertil. Steril. 58, 1093–1099. doi: 10.1016/S0015-0282(16)55548-4
Campos-Ramos, R., Garza-Torres, R., Guerrero-Tortolero, D. A., Maeda-Martínez, A. M., and Obregón-Barboza, H. (2006). Environmental sex determination, external sex differentiation and structure of the androgenic gland in the Pacific white shrimp Litopenaeus vannamei (Boone). Aquacult. Res. 37, 1583–1593. doi: 10.1111/j.1365-2109.2006.01604.x
Charniaux-Cotton, H. (1954). Discovery in, an amphipod crustacean (Orchestia gammarella) of an endocrine gland responsible for the differentiation of primary and secondary male sex characteristics. C. R. Hebd. Seances Acad. Sci. 239, 780–782.
Charniaux-Cotton, H. (1992). Arthropoda-Crustacea: sexual differentiation. Reprod. Biol. Invertebr. 5, 281-323.
Chung, J. S. (2014). An insulin-like growth factor found in hepatopancreas implicates carbohydrate metabolism of the blue crab Callinectes sapidus. Gen. Comp. Endocrinol. 199, 56–64. doi: 10.1016/j.ygcen.2014.01.012
Chung, J. S., Manor, R., and Sagi, A. (2011). Cloning of an insulin-like androgenic gland factor (IAG) from the blue crab, Callinectes sapidus: implications for eyestalk regulation of IAG expression. Gen. Comp. Endocrinol. 173, 4–10. doi: 10.1016/j.ygcen.2011.04.017
Hoffman, D. L. (1968). Seasonal eyestalk inhibition on the androgenic glands of a protandric shrimp. Nature 218, 170–172. doi: 10.1038/218170a0
Hughes, I. A. (2001). Minireview: sex differentiation. Endocrinology 142, 3281–3287. doi: 10.1210/endo.142.8.8406
Imjongjirak, C., Klinbunga, S., and Sittipraneed, S. (2005). Cloning, expression and genomic organization of genes encoding major royal jelly protein 1 and 2 of the honey bee (Apis cerana). J. Biochem. Mol. Biol. 38, 49–57. doi: 10.5483/bmbrep.2005.38.1.049
Katakura, Y. (1989). Endocrine and genetic control of sex differentiation in the malacostracan crustacea. Invertebr. Reprod. Dev. 16, 177–181. doi: 10.1080/07924259.1989.9672075
Khalaila, I., Katz, T., Abdu, U., Yehezkel, G., and Sagi, A. (2001). Effects of implantation of hypertrophied androgenic glands on sexual characters and physiology of the reproductive system in the female red claw crayfish, Cherax quadricarinatus. Gen. Comp. Endocrinol. 121, 242–249. doi: 10.1006/gcen.2001.7607
Khalaila, I., Manor, R., Weil, S., Granot, Y., Keller, R., and Sagi, A. (2002). The eyestalk –androgenic gland– testis endocrine axis in the crayfish Cherax quadricarinatus. Gen. Comp. Endocrinol. 127, 147–156. doi: 10.1016/S0016-6480(02)00031-X
Kumar, P., Monin, L., Castillo, P., Elsegeiny, W., Horne, W., Eddens, T., et al. (2016). Intestinal interleukin-17 receptor signaling mediates reciprocal control of the gut microbiota and autoimmune inflammation. Immunity 44, 659–671. doi: 10.1016/j.immuni.2016.02.007
Lee, T., Yamauchi, M., and Yamazaki, F. (1994). Sex differentiation in the crab Eriocheir japonicus (Decapoda, Grapsidae). Invertebr. Reprod. Dev. 25, 123–137. doi: 10.1080/07924259.1994.9672377
Li, F., Bai, H., Zhang, W., Fu, H., Jiang, F., Liang, G., et al. (2015). Cloning of genomic sequences of three crustacean hyperglycemic hormone superfamily genes and elucidation of their roles of regulating insulin-like androgenic gland hormone gene. Gene 561, 68–75. doi: 10.1016/j.gene.2015.02.012
Li, S., Li, F., Sun, Z., and Xiang, J. (2012). Two spliced variants of insulin-like androgenic gland hormone gene in the Chinese shrimp, Fenneropenaeus chinensis. Gen. Comp. Endocrinol. 177, 246–255. doi: 10.1016/j.ygcen.2012.04.010
Li, Y., Korol, A. B., Fahima, T., and Nevo, E. (2004). Microsatellites within genes: structure, function, and evolution. Mol. Biol. Evol. 21, 991–1007. doi: 10.1093/molbev/msh073
Liu, H., Cheung, K. C., and Chu, K. H. (2008). Cell structure and seasonal changes of the androgenic gland of the mud crab Scylla paramamosain (Decapoda: Portunidae). Zool. Stud. 47, 720–732.
Liu, Y., Hui, M., Cui, Z., Luo, D., Song, C., Li, Y., et al. (2015). Comparative transcriptome analysis reveals sex-biased gene expression in juvenile Chinese mitten crab Eriocheir sinensis. PLoS One 10:e0133068. doi: 10.1371/journal.pone.0133068
Manor, R., Aflalo, E. D., Segall, C., Well, S., Azulay, D., Ventura, T., et al. (2004). Androgenic gland implantation promotes growth and inhibits vitellogenesis in Cherax quadricarinatus females held in individual compartments. Invertebr. Reprod. Dev. 45, 151–159. doi: 10.1080/07924259.2004.9652584
Manor, R., Weil, S., Oren, S., Glazer, L., Aflalo, E. D., Ventura, T., et al. (2007). Insulin and gender: an insulin-like gene expressed exclusively in the androgenic gland of the male crayfish. Gen. Comp. Endocrinol. 150, 326–336. doi: 10.1016/j.ygcen.2006.09.006
Nagaraju, G. P. (2011). Reproductive regulators in decapod crustaceans: an overview. J. Exp. Biol. 214, 3–16. doi: 10.1242/jeb.047183
Okuno, A., Hasegawa, Y., and Nagasawa, H. (1997). Purification and properties of androgenic gland hormone from the terrestrial isopod Armadillidium vulgare. Zool. Sci. 14, 837–842. doi: 10.2108/zsj.14.837
Okuno, A., Hasegawa, Y., Ohira, T., Katakura, Y., and Nagasawa, H. (1999). Characterization and cDNA cloning of androgenic gland hormone of the terrestrial isopod Armadillidium vulgare. Biochem. Biophys. Res. Commun. 264, 419–423. doi: 10.1006/bbrc.1999.1522
Phoungpetchara, I., Tinikul, Y., Poljaroen, J., Chotwiwatthanakun, C., Vanichviriyakit, R., Sroyraya, M., et al. (2011). Cells producing insulin-like androgenic gland hormone of the giant freshwater prawn, Macrobrachium rosenbergii, proliferate following bilateral eyestalk-ablation. Tissue Cell 43, 165–177. doi: 10.1016/j.tice.2011.02.001
Powell, D., Knibb, W., Remilton, C., and Elizur, A. (2015). De-novo transcriptome analysis of the banana shrimp (Fenneropenaeus merguiensis) and identification of genes associated with reproduction and development. Mar. Genomics 22, 71–78. doi: 10.1016/j.margen.2015.04.006
Rosen, O., Manor, R., Weil, S., Gafni, O., Linial, A., Aflalo, E. D., et al. (2010). A sexual shift induced by silencing of a single insulin-like gene in crayfish: ovarian upregulation and testicular degeneration. PLoS One 5:e15281. doi: 10.1371/journal.pone.0015281
Ruggiero, T., Olivero, M., Follenzi, A., Naldini, L., Calogero, R., and Di-Renzo, M. F. (2003). Deletion in a (T) 8 microsatellite abrogates expression regulation by 3′-UTR. Nucleic Acids Res. 31, 6561–6569. doi: 10.1093/nar/gkg858
Rutanen, E. M. (1993). Cytokines in reproduction. Ann. Med. 25, 343–347. doi: 10.3109/07853899309147295
Sagi, A., and Khalaila, I. (2001). The crustacean androgen: a hormone in an isopod and androgenic activity in decapods. Am. Zool. 41, 477–484.
Sagi, A., Snir, E., Khalaila, I. (1997). Sexual differentiation in decapod crustaceans: role of the androgenic gland. Invertebr. Reprod. Dev. 31, 55–61. doi: 10.1080/07924259.1997.9672563
Saigusa, M., Terajima, M., and Yamamoto, M. (2002). Structure, formation, mechanical properties, and disposal of the embryo attachment system of an estuarine crab, Sesarma haematocheir. Biol. Bull. 203, 289–306. doi: 10.2307/1543572
Shabgah, A. G., Fattahi, E., and Shahneh, F. Z. (2014). Interleukin-17 in human inflammatory diseases. Postepy Dermatol. Alergol. 31, 256–261. doi: 10.5114/pdia.2014.40954
Shechter, A., Aflalo, E. D., Davis, C., and Sagi, A. (2005). Expression of the reproductive female-specific vitellogenin gene in endocrinologically induced male and intersex Cherax quadricarinatus crayfish. Biol. Reprod. 73, 72–79. doi: 10.1095/biolreprod.104.038554
Sroyraya, M., Chotwiwatthanakun, C., Stewart, M. J., Soonklang, N., Kornthong, N., Phoungpetchara, I., et al. (2010). Bilateral eyestalk ablation of the blue swimmer crab, Portunus pelagicus, produces hypertrophy of the androgenic gland and an increase of cells producing insulin-like androgenic gland hormone. Tissue Cell 42, 293–300. doi: 10.1016/j.tice.2010.07.003
Suwansa-ard, S., Thongbuakaew, T., Wang, T., Zhao, M., Elizur, A., Hanna, P. J., et al. (2015). In silico neuropeptidome of female Macrobrachium rosenbergii based on transcriptome and peptide mining of eyestalk, central nervous system and ovary. PLoS One 10:e0123848. doi: 10.1371/journal.pone.0123848
Veenstra, J. A. (2015). The power of next-generation sequencing as illustrated by the neuropeptidome of the crayfish Procambarus clarkii. Gen. Comp. Endocrinol. 224, 84–95. doi: 10.1016/j.ygcen.2015.06.013
Veenstra, J. A. (2016). Similarities between decapod and insect neuropeptidomes. Peer J. 4:e2043. doi: 10.7717/peerj.2043
Ventura, T., Cummins, S. F., Fitzgibbon, Q., Battaglene, S., and Elizur, A. (2014). Analysis of the central nervous system transcriptome of the eastern rock lobster Sagmariasus verreauxi reveals its putative neuropeptidome. PLoS One 9:e97323. doi: 10.1371/journal.pone.0097323
Ventura, T., Manor, R., Aflalo, E. D., Weil, S., Raviv, S., Glazer, L., et al. (2009). Temporal silencing of an androgenic gland-specific insulin-like gene affecting phenotypical gender differences and spermatogenesis. Endocrinology 150, 1278–1286. doi: 10.1210/en.2008-0906
Ventura, T., Manor, R., Aflalo, E. D., Weil, S., Khalaila, I., Rosen, O., et al. (2011a). Expression of an androgenic gland-specific insulin-like peptide during the course of prawn sexual and morphotypic differentiation. ISRN Endocrinol. 2011:476283. doi: 10.5402/2011/476283
Ventura, T., Rosen, O., and Sagi, A. (2011b). From the discovery of the crustacean androgenic gland to the insulin-like hormone in six decades. Gen. Comp. Endocrinol. 173, 381–388. doi: 10.1016/j.ygcen.2011.05.018
Warren, D. W., Pasupuleti, V., Lu, Y., Platler, B. W., and Horton, R. (1990). Tumor necrosis factor and interleukin-1 stimulate testosterone secretion in adult male rat Leydig cells in vitro. J. Androl. 11, 353–360.
Wu, S., Huang, X., Li, Q., and He, M. (2013). Interleukin-17 in pearl oyster (Pinctada fucata): molecular cloning and functional characterization. Fish Shellfish Immunol. 34, 1050–1056. doi: 10.1016/j.fsi.2013.01.005
Ye, H., Li, S., Huang, H., Wang, G., and Lin Q. (2003). Histological studies on the development of androgenic gland in mud crab, Scylla paramamosain. J. Fish. Sci. Chin. 10, 376–381. doi: 10.3321/j.issn:1005-8737.2003.05.005
Zmora, N., and Chung, J. S. (2013). A novel hormone is required for the development of reproductive phenotypes in adult female crabs. Endocrinology 155, 230–239. doi: 10.1210/en.2013-1603
Keywords: crustacean female sex hormone, eyestalk ganglion, androgenic gland, sex differentiation, mud crab
Citation: Liu A, Liu J, Liu F, Huang Y, Wang G and Ye H (2018) Crustacean Female Sex Hormone From the Mud Crab Scylla paramamosain Is Highly Expressed in Prepubertal Males and Inhibits the Development of Androgenic Gland. Front. Physiol. 9:924. doi: 10.3389/fphys.2018.00924
Received: 20 October 2017; Accepted: 25 June 2018;
Published: 17 July 2018.
Edited by:
Anna Di Cosmo, Università degli Studi di Napoli Federico II, ItalyReviewed by:
Zhigang Shen, Huazhong Agricultural University, ChinaCopyright © 2018 Liu, Liu, Liu, Huang, Wang and Ye. This is an open-access article distributed under the terms of the Creative Commons Attribution License (CC BY). The use, distribution or reproduction in other forums is permitted, provided the original author(s) and the copyright owner(s) are credited and that the original publication in this journal is cited, in accordance with accepted academic practice. No use, distribution or reproduction is permitted which does not comply with these terms.
*Correspondence: Haihui Ye, aGFpaHVpeWVAeG11LmVkdS5jbg==
Disclaimer: All claims expressed in this article are solely those of the authors and do not necessarily represent those of their affiliated organizations, or those of the publisher, the editors and the reviewers. Any product that may be evaluated in this article or claim that may be made by its manufacturer is not guaranteed or endorsed by the publisher.
Research integrity at Frontiers
Learn more about the work of our research integrity team to safeguard the quality of each article we publish.