- 1Department of Medical Physiology, School of Medicine, University of Granada, Granada, Spain
- 2PROmoting FITness and Health Through Physical Activity Research Group, Department of Physical Education and Sports, Faculty of Sport Sciences, University of Granada, Granada, Spain
The popularity of whole-body electromyostimulation is growing during the last years, but there is a shortage of studies that evaluate its effects on physical fitness and sport performance. In this study, we compared the effects of a periodized and functional whole-body-electromyostimulation training on maximum oxygen uptake (VO2max), ventilatory thresholds (VT1 and VT2), running economy (RE), and lower-body muscle strength in runners, vs. a traditional whole-body-electromyostimulation training. A total of 12 male recreational runners, who had been running 2–3 times per week (90–180 min/week) for at least the previous year and had no previous experience on WB-EMS training, were enrolled in the current study. They were randomly assigned to a periodized and functional whole-body-electromyostimulation training group (PFG) (n = 6; 27.0 ± 7.5 years; 70.1 ± 11.1 kg; 1.75 ± 0.05 m) whose training program involved several specific exercises for runners, or a traditional whole-body-electromyostimulation training group (TG) (n = 6; 25.8 ± 7.4 years; 73.8 ± 9.8 kg; 1.73 ± 0.07 m), whose sessions were characterized by circuit training with 10 dynamic and general exercises without external load. The training programs consisted of one whole-body electromyostimulation session and one 20-min running session per week, during 6 weeks. The PFG followed an undulating periodization model and a selection of functional exercises, whereas the TG followed a traditional session structure used in previous studies. Both groups were instructed to stop their habitual running training program. VO2max, VT1, VT2, RE, and lower body muscle strength (vertical jump) were measured before and after the intervention. The PFG obtained significantly higher improvements when compared with the TG in terms of VO2max (2.75 ± 0.89 vs. 1.03 ± 1.01 ml/kg/min, P = 0.011), VT2 (2.95 ± 1.45 vs. 0.35 ± 0.85 ml/kg/min, P = 0.005), VO2max percentage at VT2 (5.13 ± 2.41 vs. 0.63 ± 1.61%), RE at VT1 (−7.70 ± 2.86 vs. −3.50 ± 2.16 ml/kg/km, P = 0.048), RE at 90% of VT2 (−15.38 ± 4.73 vs. −3.38 ± 4.11 ml/kg/km, P = 0.005), and vertical jump in Abalakov modality (2.95 ± 0.94 vs. 0.52 ± 1.49 cm, P = 0.008). Therefore, we conclude that running performance improvements were better after a 6-week program following an undulating periodization and consisting on functional exercises when compared with a 6-week traditional WB-EMS program.
Introduction
Running has become increasingly popular in recent decades, and numerous novice runners start to practice this activity each year all over the world (Lee et al., 2017). Aerobic running performance is determined by different physiological parameters such as (i) maximum oxygen consumption (VO2max), (ii) ventilatory threshold 1 (VT1), (iii) ventilatory threshold 2 (VT2), (iv) running economy (RE) (Jones and Carter, 2000; Barnes and Kilding, 2015), and (v) muscular strength, among others (Tucker et al., 2013). Strength training is able to increase all of them (Paavolainen et al., 1999; Jones and Carter, 2000; Beattie et al., 2014). Local electromyostimulation training seems to be an alternative to traditional strength training (Filipovic et al., 2012), yet there is a lack of studies evaluating its effects on running performance-related parameters.
Whole-body electromyostimulation (WB-EMS) has recently emerged as an innovative training modality, and enables the simultaneous exogenous muscle activation of up to 18 regions with a total area of 2,800 cm2 covered by electrodes. It allows the configuration of different intensities in each region. To note, WB-EMS improves muscle strength in elite soccer players (Filipovic et al., 2016), in postmenopausal women (Kemmler et al., 2010), and in healthy untrained middle-aged men (Kemmler et al., 2016). WB-EMS training has some advantages: (i) training sessions are relatively short (<20 min), (ii) training frequency is low (3 sessions in 2 weeks) (Kemmler et al., 2016), (iii) sessions are dynamic and entertaining, (iv) it is a novel training stimulus, and (v) it could be used in training cessation situations such as injuries. However, to our knowledge, there are no intervention trials studying the effects of WB-EMS on aerobic performance parameters in runners.
WB-EMS intervention studies commonly use a pre-determined configuration of the electrical parameters, which is not modified during the training sessions. Moreover, the selection of exercises applied only includes motor patterns with a short range of motion and isometric actions (Kemmler et al., 2010, 2012, 2014, 2016; Kemmler and von Stengel, 2013). It is well-known that the application of a periodized training is more effective than non-periodized programs in resistance training programs (Harries et al., 2015). In addition, the application of exercise tasks involving specific running action (functional and transference task) enhance aerobic performance in endurance athletes (Balsalobre-Fernández et al., 2016). Thus, although it seems plausible that a WB-EMS training program including periodization (stimuli variation applied to achieve a specific training goal) and transference (specificity in exercise selection) as principles of sport training would result in additional benefits to those exerted by traditional WB-EMS training, empirical evidence is needed to confirm this hypothesis.
The purpose of the current study was to compare the effects of a 6-week periodized and functional WB-EMS training on VO2max, VT1, VT2, RE, and lower-body muscle strength in runners, vs. a traditional whole-body-electromyostimulation training. Our hypothesis is that the periodized and functional WB-EMS training will improve running performance when compared with traditional WB-EMS.
Methods
Participants
We recruited 15 healthy male recreational runners, who had been running 2–3 times per week (132.7 ± 28.6 min/week) for at least the previous year and had no previous experience on WB-EMS training. Three participants did not complete the study (due to job and family reasons) and were excluded from further analysis. The participants signed an informed consent. The study was approved by the Human Research Ethics Committee of the University of Granada (200/CEIH/2016) and complied with the revised ethical guidelines of the Declaration of Helsinki.
Design
A randomized controlled trial design (ClinicalTrials.gov ID: NCT03425981) was applied following CONSORT statements. The participants were randomized into two groups: (i) the PFG (n = 6) and (ii) the TG (n = 6). Participants were instructed to do one supervised WB-EMS training session and one RE test per week, and to stop their training routines while the WB-EMS program was performed. Both WB-EMS programs sessions had the same total duration.
WB-EMS Training Program
The WB-EMS training programs were designed considering different electrical parameters: (i) frequency; number of electrical pulses per time unit; it has been shown that there is not a selectively activation of muscle fibers using low or high frequencies, but fast fibers are predominantly recruited independently of the frequency applied (Gregory and Bickel, 2005), (ii) impulse width; which could influence the intensity of muscle contraction and is specific for each muscle group (Filipovic et al., 2011), (iii) impulse intensity; percentage of maximum voluntary contraction; in spite no data about it was provided in WB-EMS studies, several studies that applied local EMS showed a stimulation intensity of >50% maximum voluntary contraction is required to produce physiological improvements (Filipovic et al., 2011), and (iv) duty cycle; ratio between time receiving electrical stimuli and the total cycle time (Filipovic et al., 2011).
The WB-EMS training program consisted on six training sessions (1 per week). Before starting the program, the participants went through a familiarization session to learn movement patterns and to be adapted to the electrical stimuli. An experienced National Strength and Conditioning Association Certified Personal Trainer (NSCA-CPT) supervised all training sessions. Specific exercises of each training program modality are collected in Supplementary Material.
WB-EMS Periodized and Functional Running Training Group (PFG)
The PFG followed an undulating periodization model (understanding periodization as a systematic planning of athletic or physical training). The training sessions were divided into four parts (the participants only did movements when receiving electrical impulse in all cases): warm up (A), strength training (B), high intensity interval power training (C), and high intensity interval training (D). The electrical parameters, except impulse intensity, were modified across different parts of the session (see Table 1) in order to follow the recommendations for each exercise modality to improve strength and aerobic performance improvements (Kemmler et al., 2014; Amaro-Gahete et al., 2017). The impulse intensity was individually adjusted by RPE every 2 min. We applied a circuit training methodology (no rest between exercises) in all phases and we did not use any external load. In phase A, the participants performed 7–10 repetitions (1 set) of 3 exercises; both concentric and eccentric phases took 2 s each in every repetition. In phase B, the participants did 1–2 sets of 5–10 repetitions of 6 exercises; the concentric phase took 1 s and the eccentric phase duration was 3 s. In phase C, the participants performed 1 set of 8 exercises; they were instructed to do as many repetitions as possible in 10 s with a 10-s rest between exercises. In phase D, the participants did 1–2 interval sets with two different intensities: moderate intensity (65% VO2max speed) and high intensity (>85% VO2max speed) running on a treadmill (30 s each set at both intensities).
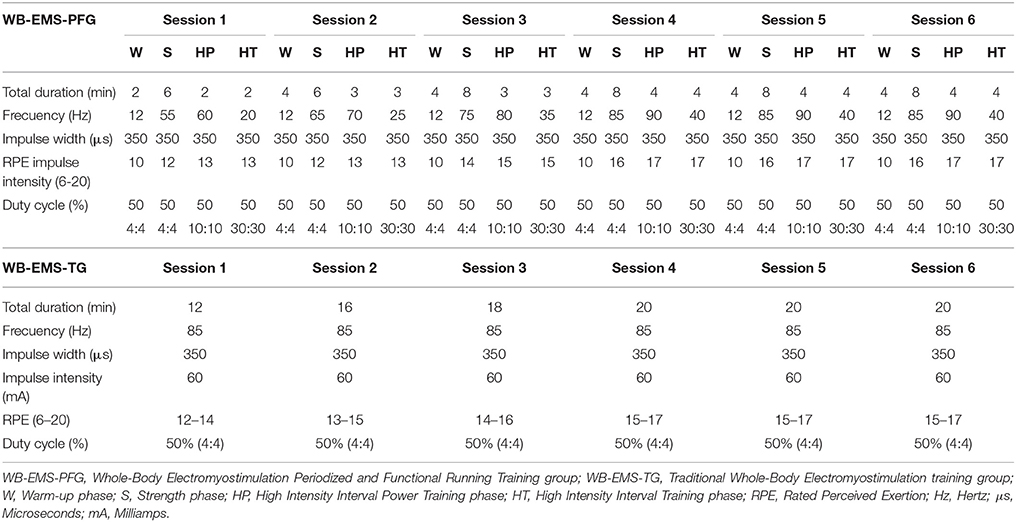
Table 1. Electric parameters description in WB-EMS-PFG and WB-EMS-TG sessions (proposed progression).
The exercises selected were functional and specific for the running discipline in order to comply with the principle of specificity. The exercises used in phase A (see Figure S1) were: (i) ½ squat and arm curl, (ii) ½ squat and bench flies, and (iii) ½ squat and horizontal push. The exercises used in phase B (see Figures S2.1, S2.2) were (i) lunge and knee-hip flexion, (ii) American swing, (iii) push-up, (iv) Bulgarian squat and vertical press, (v) sumo squat and lateral raises, and (vi) dead lift and horizontal pull. We used explosive and plyometric exercises in phase C (see Figures S3.1, S3.2, e.g. step jump, arms and leg frequency and cadence, climber, side step jump, skipping etc.). Finally, in phase D, the participants performed running intervals at different intensities.
The rationale of our periodization was based on the principle of progression, because it is not well-determined the best combination of electrical parameter in WB-EMS training for improve running performance.
Traditional WB-EMS Training Group (TG)
The TG program was based on training interventions applied in previous WB-EMS studies (Kemmler et al., 2010, 2012, 2014, 2016; Kemmler and von Stengel, 2013). The electrical impulse and training load were increased along the program (see Table 1). The impulse intensity was individually adjusted by RPE (Borg, 1982) every 2 min during all sessions.
The sessions were structured in a circuit format and consisted on 10 dynamic and general exercises without external load (see Figures S4.1, S4.2): (i) ½ squat and arm curl, (ii) dead lift and horizontal pull, (iii) ½ squat and trunk flexion, (v) ½ squat and vertical push, (v) ½ squat and lateral raises, (vi) ½ squat and horizontal push, (vii) squat and frontal raises, (viii) dead lift and triceps kick, (ix) squat and lateral flies, and (x) trunk rotation. The participants performed six to 10 sets (1 exercise repetition per set) depending on the total session duration, and both concentric and eccentric phases took 2 s in every repetition (a 4-s rest between exercises and 30 s between sets).
Performance-Related Variables
Two assessment days took place before and after the intervention. An anthropometric evaluation and a maximal treadmill exercise test were performed on day 1, and a vertical jump test and a RE test were performed on day 2. The participants were instructed not to consume alcohol, caffeine, and not to do vigorous-intensity exercise on the 48 h prior to the assessment days. Both assessment days were separated by 48 h.
Anthropometry
Body mass was determined by a scale (SECA, Hamburg, Germany) and height was determined by stadiometer (SECA, Hamburg, Germany); body mass index was also calculated (kg/m2).
VO2max
VO2max was assessed in a maximum treadmill (H/P/Cosmos Pulsar treadmill, H/P/Cosmos Sport & Medical GMBH, Germany) exercise test with a progressive incremental protocol (Noakes et al., 1990; Machado et al., 2013). In brief, after a warm-up consisting on walking at 5 km/h for 3 min, the incremental protocol started with an initial speed of 8 km/h (1% grade), which was increased 1 km/h every minute until the participants reached their volitional exhaustion. Thereafter, the participants performed a cooling-down period (4 km/h and 0% grade during 5 min). O2 consumption and CO2 production were measured with a gas analyzer (Oxycon Pro; Jaeger, Höchberg, Germany). The gas analyzer was calibrated with a known gas mixture (0% O2 and 5.5 CO2) and environmental air (20.9% O2 and 0.03% CO2) immediately before each test. Consistently across assessments, the participants were strongly encouraged to invest maximum effort. The participants were previously familiarized with the 6–20 Borg scale (Borg, 1982), which was used to measure the RPE during the last 15 s of each stage and at exhaustion. Heart rate was recorded every 5 s (Polar RS300, Kempele, Finland). We also registered respiratory, RPE, and heart rate parameters during the cooling-down period. The gas exchange data was averaged every 10 s and was downloaded for later analysis.
Ventilatory Thresholds
In the maximum treadmill exercise test, VT1 and VT2 were estimated from gas exchange data through different respiratory variables: minute ventilation (VE) and equivalents for oxygen (VE/VO2) and carbon dioxide (VE/VCO2) by two independent researchers (FA and AG), and a third researcher opinion was sought when they disagreed (AO). VT1 and VT2 were determined following a validated methodology applied in previous studies (Lucía et al., 2000).
Running Economy
RE was determined during a treadmill protocol following a specific protocol used in previous studies (Guglielmo et al., 2009; Shaw et al., 2014; Holmes et al., 2015). The treadmill test consisted of two 10-min stages at two different intensities; (i) speed at VT1 and (ii) speed at 90% of VT2. The first 2-min record of each stage were discarded in order to ensure that the participants reached the steady-state criteria. RE (oxygen cost of running a kilometer at a specific velocity) was calculated using the following equation: RE = VO2 (ml/kg/min)/[speed (km/h)/60] (Guglielmo et al., 2009; Shaw et al., 2014; Holmes et al., 2015). RE was assessed before, during (once per week), and after the training program considering pre-intervention VT1 and 90% VT2 speed.
Lower Body Muscular Strength
Vertical jump performance was assessed using the countermovement (CMJ) and Abalakov jump (ABJ) tests. In standing position, with legs straight and both hands on hips, the participant performs a vertical jump with an earlier fast counter movement. The Abalakov jump is similar to the CMJ, but now the participants is allowed to freely coordinate the arms and trunk movements to reach the maximum height (Bosco et al., 1983). The jumping height was calculated from the flight time using kinematic equations (Lehance et al., 2005) estimated by Ergo Jump Bosco System® (Globus, Treviso, Italy). Before carrying out the tests, a standardized warm-up was performed which included a 5-min run at 50% of heart rate reserve, and mobility and muscle activation exercises.
Statistical Analyses
All outcome variables were checked for normality with a graphical test (QQ-Plots) and results are expressed as mean and SD or median and ranges. The baseline and post intervention data were compared using Student's paired t-test. One-way analysis of covariance (ANCOVA) was used to examine the effect of group (fixed factor) on VO2max change, i.e., post-VO2max minus pre-VO2max (dependent variable), adjusting for baseline value. The same analyses were used for maximal aerobic speed, oxygen uptake at VT1 and VT2, VO2max percentage in VT1 and VT2, VT1 and VT2 speed, RE at VT1 speed and 90% of VT2 speed, and vertical jump (countermovement jump and Abalakov jump). Multiple comparisons were adjusted by Bonferroni. Two-way ANOVA (Time x Group) was used to determine changes in RE during the intervention study; we applied an adjustment by Greenhouse-Geisser when the Mauchly test of sphericity was significant (p < 0.05). We conducted all statistical analyses using SPSS Statistics (version 20, IBM, Ehningen, Germany) software, setting level of significance at p < 0.05.
Results
There were no WB-EMS-related adverse effects. The baseline characteristics by training group are shown in Table 2. We observed no statistically significant differences at baseline (Table 3). The participants reported similar values of the impulse intensity (measured by RPE) than the pre-established ranges (mean difference ± SD, 0.71 ± 0.24 and 0.64 ± 0.34). This fact confirmed that participants of WB-EMS group adhered to the protocol designed in term of impulse intensity.
Anthropometry
BMI significantly decreased in a similar fashion in the PFG and the TG (from 22.6 ± 2.8 to 22.1 ± 2.6 kg/m2, and from 24.5 ± 3.3 to 24.1 ± 3.2 kg/m2, P = 0.022 and P = 0.021, respectively).
VO2max
There were no changes in absolute VO2max (ml/min−1) levels in the TG, whereas changes were border on significance (P = 0.082) in the PFG (Figures 1A,B). VO2max in relative terms (ml/kg/min) increased in PGF group and a tendency were observed in TG after training (P < 0.001 and P = 0.053 for the PFG and the TG respectively, Figure 1C), being the pre-post changes higher in the PFG group than in the TG (mean difference: 1.72 ml/kg/min, P = 0.011, Figure 1D). Similarly, maximal aerobic speed increased in the PFG (P = 0.041, Figure 1E), whereas no changes were observed in the TG (Figure 1E). Between group changes comparisons were border of significance for maximal aerobic speed (mean difference: 1.01 vs. −0.17 km/h for the PFG and the TG, respectively, P = 0.065, Figure 1F).
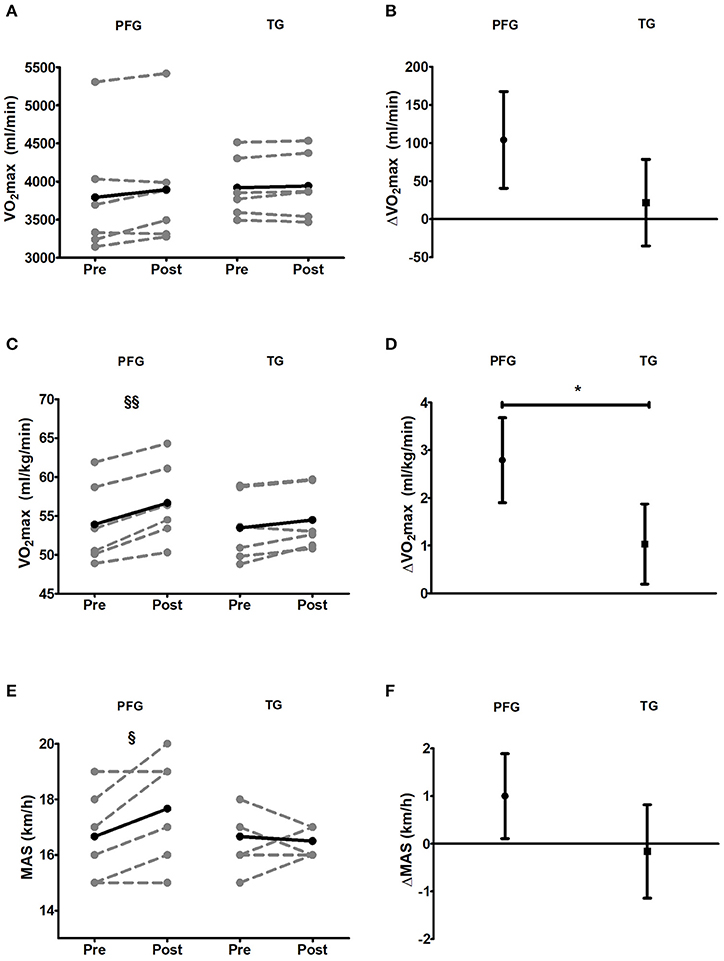
Figure 1. Pre and post 6-week intervention values and mean change (95% CI) in maximal oxygen uptake (absolute and relative values), and maximal aerobic speed after the intervention program. (A,B) Maximal oxygen uptake in absolute values [VO2max (ml/min)]; (C,D) Maximal oxygen uptake in relative values [VO2max (ml/kg/min)]; (E,F) Maximal Aerobic Speed [MAS (km/h)]. §P < 0.05, §§P < 0.01, §§§P < 0.001 (analysis pre-post; Student's paired t-test). *P < 0.05, **P < 0.01, ***P < 0.001 (analysis between groups; analysis of covariance). PFG, Periodized, and Functional group; TG, Traditional Whole-Body-Electromyostimulation training.
Ventilatory Thresholds
There were no significant pre-post changes in VO2 at VT1, VO2max percentage in VT1, and VT1 speed in the TG (all P > 0.076), yet, significant pre-post differences in VO2 at VT1, and VT1 speed were observed in the PFG. There were no significant between groups differences when comparing pre-post changes (all P > 0.094, Table 3).
Regarding VT2, there were significant pre-post differences in VO2 at VT2, VO2max percentage in VT2, and VT2 speed in the PFG (all P < 0.004), but not in the TG (all P > 0.360). Changes in VO2 at VT2, VO2max percentage in VT2, and VT2 speed were significantly different in the PFG and the TG (all P < 0.008) (see Figure 2).
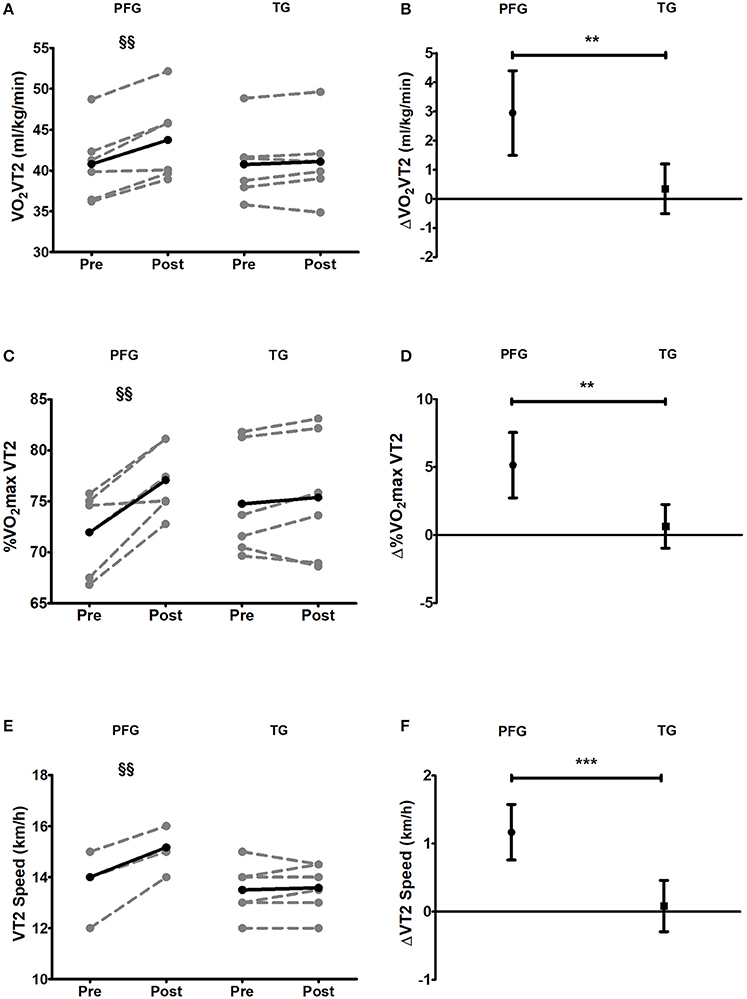
Figure 2. Pre and post 6-week intervention values and mean change (95% CI) in oxygen uptake at ventilatory threshold 2, maximal oxygen uptake percentage in ventilatory threshold 2, and ventilatory threshold 2 speed. (A,B) Oxygen uptake at ventilatory threshold 2 [VO2VT2s (ml/kg/min)]; (C,D) Maximal oxygen uptake percentage in ventilatory threshold 2 [%VO2max VT2]; (E,F) Ventilatory Threshold 2 Speed [VT2 speed (km/h)]. §P < 0.05, §§P < 0.01, §§§P < 0.001 (analysis pre-post; Student's paired t-test). *P < 0.05, **P < 0.01, ***P < 0.001 (analysis between groups; ANCOVA). PFG, Periodized and Functional Whole-Body- Electromyostimulation training; TG, Traditional Whole-Body-Electromyostimulation training.
Running Economy
RE at VT1 speed and 90% VT2 speed improved in the PFG (P < 0.001) (Figures 3A,C, respectively), but it did not in the TG (P > 0.111), and significant differences were found when comparing pre-post changes between groups (P < 0.05 and P < 0.01 for VT1 speed and 90% VT2, respectively). Repeated measures analysis showed that between-group differences in RE at VT1 speed appeared after 6 weeks of intervention (P < 0.05), whereas differences in RE at 90% of VT2 speed appeared in the fourth week (P < 0.05) and continued until the post-intervention assessment (P < 0.01) (Figures 3B,D).
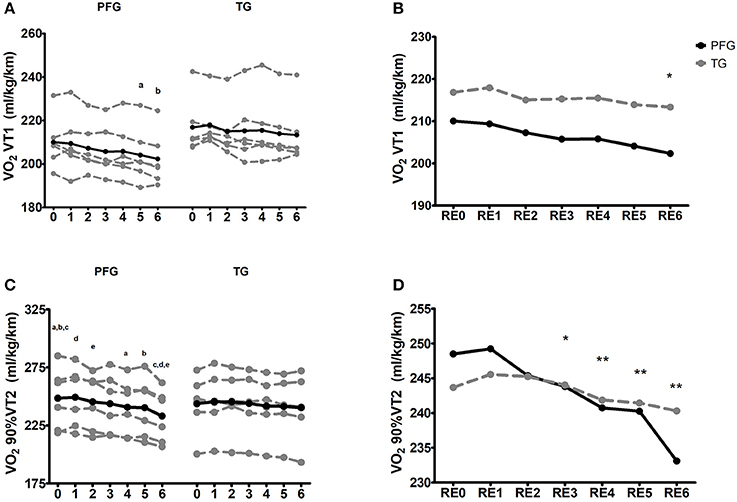
Figure 3. Running economy (RE) kinetics at ventilatory threshold 1 speed, and 90% of ventilatory threshold 2 speed after the intervention program. (A,B) Running economy at ventilatory threshold 1 speed [VO2 VT1 (ml/kg/km)], (C,D) running economy at 90% of ventilatory threshold 2 speed [VO2 90%VT2 (ml/kg/km)]. RE0 corresponded to the first running economy test (pre-test); RE1 corresponded to the running economy test performed in week 1; RE2 corresponded to the running economy test performed in week 2; RE3 corresponded to the running economy test performed in week 3. RE4 corresponded to the running economy test performed in week 4; RE5 corresponded to the running economy test performed in week 5; RE6 corresponded to the running economy test performed in week 6 (post-test). Repeated letters (a–a; b–b, etc.) indicate P < 0.05 in different weeks (analysis throughout time; Repeated ANOVA measures). *P < 0.05, **P < 0.01, ***P < 0.001 (analysis between groups of the pre-test differences; ANCOVA). PFG, Periodized and Functional Whole-Body-Electromyostimulation training; TG, Traditional Whole-Body-Electromyostimulation training.
Lower Body Muscle Strength
The levels of CMJ increased after the intervention in both the PFG and the TG (P < 0.05 in both cases), being pre-post changes similar between groups (P = 0.203) (Figures 4A,B). The pre-post changes in ABJ were, however, different between the PFG vs. the TG (P < 0.01, Figures 4C,D), and we observed no pre-post differences in the TG (P = 0.724).
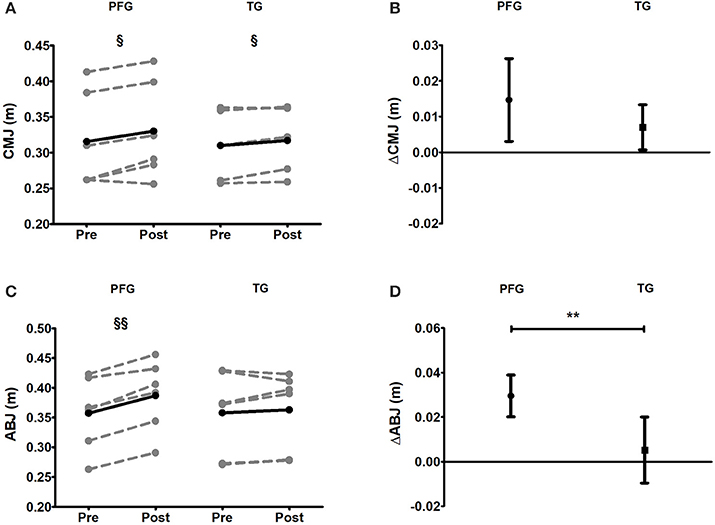
Figure 4. Pre and post 6-week intervention values and mean change (95% CI) countermovement jump and Abalakov jump after the intervention program. (A,B) Countermovement jump [CMJ (m)], (C,D) Abalakov jump [ABJ (m)]. §P < 0.05, §§P < 0.01, §§§P < 0.001 (analysis pre-post; Student's paired t-test). *P < 0.05, **P < 0.01, ***P < 0.001 (analysis between groups; ANCOVA). PFG, Periodized and Functional Whole-Body-Electromyostimulation training; TG, Traditional Whole-Body-Electromyostimulation training.
Discussion
This study shows that the effects of a 6-week periodized and functional WB-EMS training program on running performance-related parameters including VO2max, VT2, RE, and ABJ in recreational runners are better than those observed after following a traditional WB-EMS training program. These findings reinforce the fact that the effects of WB-EMS training on running performance can be greatly improved if it is correctly designed. To our knowledge, this is the first study analyzing the effect of WB-EMS on running performance-related parameters in recreational runners.
VO2max
VO2max is considered one of the best predictor of running performance (Tucker et al., 2013). We observed an increment of 2.75 ml/kg/min in the PFG. These findings do not concur with an intervention program consisting on two combined strength sessions per week which lasted for 11 weeks while maintaining its endurance training volume in trained athletes, which increased several strength parameters and muscle fiber cross sectional area of both fiber type I and fiber type II, but no changes in VO2max were found (Vikmoen et al., 2016). However, a meta-analysis (Sloth et al., 2013) evaluated the effects of high intensity interval training interventions (lasting 2–8 weeks) on VO2max in healthy sedentary or recreationally active adults, and showed a similar VO2max increase (ranged between 4.2 and 13.4%). These improvements are similar to those observed in the PFG.
Traditionally, WB-EMS training programs do not vary electrical frequency, electrical intensity, width impulse, and duty cycle, within the training sessions. Moreover, the selection of exercises usually consists on a set of non-functional movements (i.e., not motor related with the competition movement) (Filipovic et al., 2011; Amaro-Gahete et al., 2017). We designed a training intervention that followed (i) an undulating periodization training model, and (ii) a functional selection of exercises to improve running performance. We observed that absolute VO2max increased by 2.78% after the 6-week intervention program in the PFG compared with the TG. When expressed in relation to body mass (ml/kg/min), an improvement on VO2max could be explained either by a reduction on body mass and/or an increase in VO2max itself (ml/min). Our data suggest that improvements obtained in VO2max relative to body mass were determined only by body mass loss in the TG; however, VO2max in relative terms were strongly influenced by VO2max in absolute terms (P = 0.08) in the PFG.
Periodized resistance training programs result on higher benefits over muscle strength compared to non-periodized training programs, which also seems to be the case in WB-EMS training programs (Williams et al., 2017). On the other hand, Nuhr et al. reported that a local electromyostimulation training program (10 weeks, 4 h per day, 7 days per week) at low frequency (15 Hz) applied in quadriceps and hamstring muscles of both legs in healthy men improve maximal aerobic-oxidative capacity (Nuhr et al., 2003). Interpreting our results, despite the fact that both WB-EMS training programs had the same time exposure (same number of sessions and duration), we cannot discard that differences in overall training load (e.g., different muscular tension) explain the observed differences.
Ventilatory Thresholds
It is well-known that VT1 and VT2, especially the latter, have an important role in long-distance running performance (Jones and Carter, 2000; Barnes and Kilding, 2015). The effect of concurrent training on VT1 and VT2 is controversial. Two studies reported little changes on VT2 in runners (Mikkola et al., 2011; Støren et al., 2013), which concur with our findings in the TG. In contrast, others observed substantial improvements in VT2 (Guglielmo et al., 2009; Taipale et al., 2013) after following resistance training program in trained athletes. In our study, the PFG experienced an increase of 7% in VO2 at VT2. Of note is that an improvement in VT1 and VT2 speed, and VO2 at VT1 and VT2, could be an indirect effect of an improvement on VO2max and/or RE (see below). However, the increase of VO2max percentage at which VT2 is achieved suggest that a direct improvement of VT2 was observed in the PFG.
Running Economy
RE, defined as the oxygen consumption required at a given absolute submaximal exercise intensity, is considered critical for running performance in trained individuals with homogenous VO2max (Saunders et al., 2004). Our findings concur with those of Taipale et al. who reported substantial improvements in RE after 8 weeks of combined explosive strength and endurance training in runners (Taipale et al., 2013).
Although we cannot know whether the periodization or the use of functional exercises are the main cause of the improvement in RE, the performance of functional exercises with running actions at maximal speed with superimposed electromyostimulation could be an important factor to improve RE. The PFG included a period of high intensity short run intervals with superimposed electromyostimulation; this fact could determine some advantages over the TG inasmuch this training modality did not include running actions. Therefore, this fact could contribute to maximize performance at high speed intensity. On the other hand, we cannot discard that following a periodization training model could increase the improvements obtained (Harries et al., 2015).
Lower Body Muscle Strength
Several studies have shown the importance of lower body muscle strength in running performance, especially in RE (Dumke et al., 2010). Our findings concur with those observed by other studies using WB-EMS and local electromyostimulation training (12–28 sessions) on the lower body muscles in trained athletes (Babault et al., 2007; Filipovic et al., 2011, 2016). Filipovic et al. (2016) applied a WB-EMS intervention twice a week, which consisted on 3 × 10 maximal squat jumps with a set pause of 60 s. They found significant increases in squat jump, CMJ, and drop jump (all P < 0.05), which concur with our findings. Our study showed an increase in CMJ in both training groups, whereas significant improvement in ABJ was only observed in the PFG. This fact could be explained by differences in the exercises used and the electrical parameters applied in both intervention groups (e.g., the inclusion of several plyometric exercises in the PFG). Since the main difference between CMJ and ABJ is the contribution of interlimb coordination, the improvement obtained in ABJ in the PFG when compared to the TG, suggest that a periodized and functional WB-EMS training program could have a considerable impact on inter-limb coordination (Dal Pupo et al., 2013). However, more studies are needed to elucidate which physiological or biomechanical mechanism may explain the differences reported between groups.
Possible reasons which could explain the physiological improvements observed are: (i) a better efficiency of mitochondrial metabolism, what is related with an increase in the rate of lipid utilization [it is well-known that this fact produces an important descent in rate of glycogen depletion (Esfarjani and Laursen, 2007)], (ii) an increased capillary density after an specific training program, yet we applied low frequencies in different parts of PFG sessions and it is well-known that low frequencies produce a development of capillarization process (Miyamoto et al., 2016), and (iii) positive changes in neurological process related to lower limb coordination, recruitment and synchronization of motor units, and co-activation of muscles, which could results in a greater leg stiffness and muscular strength (Barnes and Kilding, 2015); only PFT include an wide values spectrum of the different electrical parameters manipulated, and specific functional task (plyometric or explosive strength exercises) oriented to improve these facts. All of the previous items could produce a delay in local and peripheral fatigue resulting in a decrease of oxygen uptake for a specific speed and, consequently, getting a better running performance.
Limitations
The results of this study should be considered with caution as there are some limitations. The main limitation of this study (as all WB-EMS previous studies) is the lack of information about the total electrical impulse magnitude applied. Indeed, the best methodology to control WB-EMS training load is currently unknown. Moreover, the low sample size and the inexperienced participants in WB-EMS training may have influenced the results, since improvements produced by the application of a novel stimulus are expected. In addition, we cannot know whether these results extend to longer training programs, and or to not professional athletes or patients. We did not confirm VO2peak by checking VO2max plateau with a constant work rate test (110% of the maximal intensity reached after the ramp protocol test) because our participants were recreational runners and this validation is not necessary in this population (Poole and Jones, 2017). One of the strengths is that we included a positive control group which allowed to avoid the existence of false positive cases.
Conclusion
A 6-week periodized and functional WB-EMS training program produces considerable higher on VO2max, VT2, RE, and ABJ in recreational runners compared with a traditional WB-EMS training program. Our findings indicate that WB-EMS training, as a novel stimulus, could complement or even modify the common training methods in runners. In addition, our results strongly support that WB-EMS training must be carefully designed and supervised, not only for safety reasons, but also for efficiency issues. This concept is particularly relevant in the commercial context where this type of technology could be understood as a completely autonomous training methodology. More studies are needed to better understand the practical applications of this new training methodology in other populations, to elucidate whether the improvements obtained are due to the exercises selected, or to the electrical parameters, or to its combination, and also to elucidate whether a traditional strength program get higher improvements in running performance than WB-EMS training.
Data Availability Statement
The raw data supporting the conclusions of this manuscript will be made available by the authors, without undue reservation, to any qualified researcher.
Author Contributions
FA-G, AD-l-O, LR-G, and AG conceived and designed research. FA-G, AD-l-O, LR-G, and LJ-F conducted experiments. FA-G and JR analyzed data. FA-G wrote the manuscript. AG and JR revised the manuscript. All authors read and approved the manuscript.
Funding
The study is supported by the Spanish Ministry of Education (FPU 13/04365, FPU14/04172, and FPU15/03960). The study was partially supported by the University of Granada, Plan Propio de Investigación 2016, Excellence actions: Units of Excellence; Unit of Excellence on Exercise and Health (UCEES). This study is part of a Ph.D. Thesis conducted in the Biomedicine Doctoral Studies of the University of Granada, Spain.
Conflict of Interest Statement
The authors declare that the research was conducted in the absence of any commercial or financial relationships that could be construed as a potential conflict of interest.
Acknowledgments
The authors would like to thank all the runners who participated in this study for their time and effort. We are grateful to Ms. Carmen Sainz Quinn for assistance with the English language, and to Manuel Castillo for constructive scientific discussions.
Supplementary Material
The Supplementary Material for this article can be found online at: https://www.frontiersin.org/articles/10.3389/fphys.2018.00720/full#supplementary-material
References
Amaro-Gahete, F. J., de la, O. A., Jurado-Fasoli, L., Ruiz, J. R., and Gutiérrez, Á. (2017). Could superimposed electromyostimulation be an effective training to improve aerobic and anaerobic capacity? methodological considerations for its development. Eur. J. Appl. Physiol. 117, 1513–1515. doi: 10.1007/s00421-017-3625-x
Babault, N., Cometti, G., Bernardin, M., Pousson, M., and Chatard, J. (2007). Effects of electromyostimulation training on muscle strength and power of elite rugby players. J. Strength Cond. Res. 21, 431–437. doi: 10.1519/R-19365.1
Balsalobre-Fernández, C., Santos-Concejero, J., and Grivas, G. V. (2016). Effects of strength training on running economy in highly trained runners: a systematic review with meta-analysis of controlled trials. J. Strength Cond. Res. 30, 2361–2368. doi: 10.1519/JSC.0000000000001316
Barnes, K. R., and Kilding, A. E. (2015). Strategies to improve running economy. Sport Med. 45, 37–56. doi: 10.1007/s40279-014-0246-y
Beattie, K., Kenny, I. C., Lyons, M., and Carson, B. P. (2014). The effect of strength training on performance in endurance athletes. Sports Med. 44, 845–865. doi: 10.1007/s40279-014-0157-y
Borg, G. A. (1982). Psychophysical bases of perceived exertion. Med. Sci. Sports Exerc. 14, 377–381.
Bosco, C., Luhtanen, P., and Komi, P. V. (1983). A simple method for measurement of mechanical power in jumping. Eur. J. Appl. Physiol. Occup. Physiol. 50, 273–282.
Dal Pupo, J., Dias, J. A., Gheller, R. G., Detanico, D., and dos Santos, S. G. (2013). Stiffness, intralimb coordination, and joint modulation during a continuous vertical jump test. Sport Biomech. 12, 259–271. doi: 10.1080/14763141.2013.769619
Dumke, C. L., Pfaffenroth, C. M., McBride, J. M., and McCauley, G. O. (2010). Relationship between muscle strength, power and stiffness and running economy in trained male runners. Int. J. Sports Physiol. Perform. 5, 249–261.
Esfarjani, F., and Laursen, P. B. (2007). Manipulating high-intensity interval training: effects on VO2max, the lactate threshold and 3000 m running performance in moderately trained males. J. Sci. Med. Sport 10, 27–35. doi: 10.1016/j.jsams.2006.05.014
Filipovic, A., Grau, M., Kleinöder, H., Zimmer, P., Hollmann, W., and Bloch, W. (2016). Effects of a whole-body electrostimulation program on strength, sprinting, jumping, and kicking capacity in elite soccer players. J. Sports Sci. Med. 15, 639–648.
Filipovic, A., Kleinöder, H., Dörmann, U., and Mester, J. (2011). Electromyostimulation-A systematic review of the influence of training regimens and stimulation parameters on effectiveness in electromyostimulation training of selected strength parameters. J. Strength Cond. Res. 25, 3218–3230. doi: 10.1519/JSC.0b013e318212e3ce
Filipovic, A., Kleinöder, H., Dörmann, U., and Mester, J. (2012). Electromyostimulation-a systematic review of the effects of different electromyostimulation methods on selected strength parameters in trained and elite athletes. J. Strength Cond. Res. 26, 2600–2614. doi: 10.1519/JSC.0b013e31823f2cd1
Gregory, C. M., and Bickel, C. S. (2005). Recruitment patterns in human skeletal muscle during electrical stimulation. Phys. Ther. 85, 358–364. doi: 10.1093/ptj/85.4.358
Guglielmo, L. G. A., Greco, C. C., and Denadai, B. S. (2009). Effects of strength training on running economy. Int. J. Sports Med. 30, 27–32. doi: 10.1055/s-2008-1038792
Harries, S. K., Lubans, D. R., and Callister, R. (2015). Systematic review and meta-analysis of linear and undulating periodized resistance training programs on muscular strength. J. Strength Cond. Res. 29, 1113–1125. doi: 10.1519/JSC.0000000000000712
Holmes, K., Cannon, A., Wingerd, E., Stepler, K., Fish, A., Peterson, M., et al. (2015). Effects of 3-weeks of high-intensity interval training on running economy and endurance. Int. J. Exerc. Sci. 41, 1–10.
Jones, A. M., and Carter, H. (2000). The effect of endurance training on parameters of aerobic fitness. Sports Med. 29, 373–386. doi: 10.2165/00007256-200029060-00001
Kemmler, W., Bebenek, M., Engelke, K., and von Stengel, S. (2014). Impact of whole-body electromyostimulation on body composition in elderly women at risk for sarcopenia: the training and electrostimulation trial (TEST-III). Age 36, 395–406. doi: 10.1007/s11357-013-9575-2
Kemmler, W., Schliffka, R., Mayhew, J. L., von Stengel, S., et al. (2010). Effects of whole-body electromyostimulation on resting metabolic rate, body composition, and maximum strength in postmenopausal women: the training and electrostimulation trial. J. Strength Cond. Res. 24, 1880–1887. doi: 10.1519/JSC.0b013e3181ddaeee
Kemmler, W., Teschler, M., Weißenfels, A., Bebenek, M., Fröhlich, M., Kohl, M., et al. (2016). Effects of whole-body electromyostimulation versus high-intensity resistance exercise on body composition and strength: a randomized controlled study. Evid. Based Complement. Altern. Med. 3, 44–55. doi: 10.1155/2016/9236809
Kemmler, W., and von Stengel, S. (2013). Whole-body electromyostimulation as a means to impact muscle mass and abdominal body fat in lean, sedentary, older female adults: subanalysis of the TEST-III trial. Clin. Interv. Aging 8, 1353–1364. doi: 10.2147/CIA.S52337
Kemmler, W., Von Stengel, S., Schwarz, J., and Mayhew, J. L. (2012). Effect of whole-body electromyostimulation on energy expenditure during exercise. J. Strength Cond. Res. 26, 240–245. doi: 10.1519/JSC.0b013e31821a3a11
Lee, D. C., Brellenthin, A. G., Thompson, P. D., Sui, X., Lee, I. M., and Lavie, C. J. (2017). Running as a key lifestyle medicine for longevity. Prog. Cardiovasc. Dis. 60, 45–55. doi: 10.1016/j.pcad.2017.03.005
Lehance, C., Croisier, J., and Bury, T. (2005). Optojump system efficiency in the assessment of lower limbs explosive strength. Sci. Sport 20, 131–135.
Lucía, A., Hoyos, J., and Chicharro, J. L. (2000). The slow component of VO2 in professional cyclists. Br. J. Sports Med. 34, 367–374. doi: 10.1136/bjsm.34.5.367
Machado, F. A., Kravchychyn, A. C., Peserico, C. S., da Silva, D. F., and Mezzaroba, P. V. (2013). Incremental test design, peak “aerobic” running speed and endurance performance in runners. J. Sci. Med. Sport 16, 577–582. doi: 10.1016/j.jsams.2012.12.009
Mikkola, J., Vesterinen, V., Taipale, R., Capostagno, B., Häkkinen, K., and Nummela, A. (2011). Effect of resistance training regimens on treadmill running and neuromuscular performance in recreational endurance runners. J. Sports Sci. 29, 1359–1371. doi: 10.1080/02640414.2011.589467
Miyamoto, T., Kamada, H., Tamaki, A., and Moritani, T. (2016). Low-intensity electrical muscle stimulation induces significant increases in muscle strength and cardiorespiratory fitness. Eur. J. Sport Sci. 16, 1104–1110. doi: 10.1080/17461391.2016.1151944
Noakes, T. D., Myburgh, K. H., and Schall, R. (1990). Peak treadmill running velocity during the VO2 max test predicts running performance. J. Sports Sci. 8, 35–45. doi: 10.1080/02640419008732129
Nuhr, M., Crevenna, R., Gohlsch, B., Bittner, C., Pleiner, J., Wiesinger, G., et al. (2003). Functional and biochemical properties of chronically stimulated human skeletal muscle. Eur. J. Appl. Physiol. 89, 202–208. doi: 10.1007/s00421-003-0792-8
Paavolainen, L., Häkkinen, K., Hämäläinen, I., Nummela, A., and Rusko, H. (1999). Explosive-strength training improves 5-km running time by improving running economy and muscle power. J. Appl. Physiol. 86, 1527–1533.
Poole, D. C., and Jones, A. M. (2017). Measurement of the maximum oxygen uptake VO2max: VO2peak is no longer acceptable. J. Appl. Physiol. 122, 997–1002. doi: 10.1152/japplphysiol.01063.2016
Saunders, P. U., Pyne, D. B., Telford, R. D., and Hawley, J. A. (2004). Factors affecting running economy in trained distance runners. Sports Med. 34, 465–485. doi: 10.2165/00007256-200434070-00005
Shaw, A. J., Ingham, S. A., and Folland, J. P. (2014). The valid measurement of running economy in runners. Med. Sci. Sports Exerc. 46, 1968–1973. doi: 10.1249/MSS.0000000000000311
Sloth, M., Sloth, D., Overgaard, K., and Dalgas, U. (2013). Effects of sprint interval training on VO2max and aerobic exercise performance: a systematic review and meta-analysis. Scand. J. Med. Sci. Sports 23, 1–12. doi: 10.1111/sms.12092
Støren, Ø., Ulevåg, K., Larsen, M. H., Støa, E. M., and Helgerud, J. (2013). Physiological determinants of the cycling time trial. J. Strength Cond. Res. 27, 2366–2373. doi: 10.1519/JSC.0b013e31827f5427
Taipale, R. S., Mikkola, J., Vesterinen, V., Nummela, A., and Häkkinen, K. (2013). Neuromuscular adaptations during combined strength and endurance training in endurance runners: maximal versus explosive strength training or a mix of both. Eur. J. Appl. Physiol. 113, 325–335. doi: 10.1007/s00421-012-2440-7
Tucker, R., Santos-Concejero, J., and Collins, M. (2013). The genetic basis for elite running performance. Br. J. Sports Med. 47, 545–549. doi: 10.1136/bjsports-2013-092408
Vikmoen, O., Raastad, T., Seynnes, O., Bergstrøm, K., Ellefsen, S., and Rønnestad, B. R. (2016). Effects of heavy strength training on running performance and determinants of running performance in female endurance athletes. PLoS ONE 11:e0150799. doi: 10.1371/journal.pone.0150799
Keywords: WB-EMS, VO2max, running economy, VT2, training periodization
Citation: Amaro-Gahete FJ, De-la-O A, Sanchez-Delgado G, Robles-Gonzalez L, Jurado-Fasoli L, Ruiz JR and Gutiérrez A (2018) Functional Exercise Training and Undulating Periodization Enhances the Effect of Whole-Body Electromyostimulation Training on Running Performance. Front. Physiol. 9:720. doi: 10.3389/fphys.2018.00720
Received: 19 April 2018; Accepted: 24 May 2018;
Published: 13 June 2018.
Edited by:
Wolfgang Kemmler, Institut für Medizinische Physik, Friedrich-Alexander-Universität Erlangen-Nürnberg, GermanyReviewed by:
Michael Fröhlich, Technische Universität Kaiserslautern, GermanyNicolas Wirtz, German Sport University Cologne, Germany
Jürgen Giessing, Universität Koblenz Landau, Germany
Copyright © 2018 Amaro-Gahete, De-la-O, Sanchez-Delgado, Robles-Gonzalez, Jurado-Fasoli, Ruiz and Gutiérrez. This is an open-access article distributed under the terms of the Creative Commons Attribution License (CC BY). The use, distribution or reproduction in other forums is permitted, provided the original author(s) and the copyright owner are credited and that the original publication in this journal is cited, in accordance with accepted academic practice. No use, distribution or reproduction is permitted which does not comply with these terms.
*Correspondence: Francisco J. Amaro-Gahete, amarof@ugr.es
Angel Gutiérrez, gutierre@ugr.es