- 1State Key Laboratory for Biology of Plant Diseases and Insect Pests, Institute of Plant Protection, Chinese Academy of Agricultural Sciences, Beijing, China
- 2Key Laboratory of Biosafety, Ministry of Environmental Protection, Nanjing, China
- 3Nanjing Institute of Environmental Sciences, Ministry of Environmental Protection, Nanjing, China
- 4Guangxi Key Laboratory of Biology for Crop Diseases and Insect Pests, Institute of Plant Protection, Guangxi Academy of Agricultural Sciences, Nanning, China
Chill tolerance plays a crucial role that allows insect species to adapt to cold environments. Two Chinese geographical populations (Laibin and Yangzhou populations) were selected to understand the chill resistance and evolutionary potential in the Ophraella communa, a biological control agent of the invasive common ragweed, Ambrosia artemisiifolia. Super-cooling point assays, knockdown tests under static low-temperature conditions and determination of glycerol content were studied. ANOVAs indicated significant differences regarding chill coma recovery time, super-cooling point, and glycerol content across populations and sexes. The narrow-sense heritability (h2) estimates of cold resistance based on a parental half-sibling breeding design ranged from 0.39 to 0.53, and the h2 value was significantly higher in the Yangzhou population than in the Laibin population. Additive genetic variances were significantly different from zero for cold tolerance. The Yangzhou population of O. communa has a strong capability to quickly gain resistance to cold. We conclude that the O. communa beetle has a plasticity that can provide cold resistance in the changing climate conditions.
Introduction
Poikilothermic character is a crucial and widely recognized factor affecting insect development. Fluctuations in environmental temperature can affect the survival, development, and establishment of an insect in the field. Many insect species are currently experiencing habitat destruction due to climate change (Hoffmann et al., 2003), and some are facing previously unencountered low temperatures during the winter months. Cold climates often impede the establishment and persistence of insect populations in temperate regions. Across the long process of evolution, many types of insects have undergone several physiological changes that improved their cold-hardiness (Danks, 1996; Bale, 2002; Chen and Kang, 2002; Wang and Kang, 2005). Cold-hardiness may be a coping strategy that would allow insects to successfully migrate and establish themselves under new environmental conditions (Hoffmann, 2003; Chen et al., 2011; Kellermann et al., 2012a; Findsen et al., 2013). Due to a plastic cold-hardiness, insects can often survive at subzero temperatures during cold winter months (Salt, 1961; Kostal et al., 1998; Overgaard et al., 2007). Many previous studies have suggested that cold tolerance differs significantly across various geographical populations of an insect species mainly due to acclimation to different climatic conditions (Chen and Kang, 2002; Worland et al., 2004; Regniere and Bentz, 2007; Zhou et al., 2011b).
Adaptive phenotypic plasticity and genetic adaptation are the main ways in which natural populations respond to strong environmental stresses. Generally, natural selection can lead to micro-evolutionary changes in some particular traits of wild populations of insects (Merilä et al., 2001; Roff, 2003; Kellermann et al., 2009; Ma et al., 2014). Species distribution is often closely related to the appropriate genetic variation in the key traits which is necessary for adaptation to the changing environmental conditions (Merilä and Crnokrak, 2001). In general, evolutionary change requires that the traits subject to selection will be heritable (Roff, 2003), and thus the key traits for adapting a new environment are often coupled with genetic variation. A rapid evolutionary change in natural populations has provided the evidence for the abundance of genetic variation (Reznick et al., 1997; Hendry and Kinnison, 1999; Kellermann et al., 2009; Ma et al., 2014). Pliability of cold tolerances in insects is also closely associated with the genetic variation to overcome the cold spell in winter months (Gibert et al., 2001; Kimura, 2004; Kellermann et al., 2009; Hoffmann, 2010; Arboleda-Bustos and Segarra, 2011). Nevertheless, the evolution of plasticity, such as cold tolerances for example, under extreme conditions has become increasingly important for natural predators of specific pests increasingly exposed to colder envirenment, and there is an urgent need to excavate empirical information for evolved adaptive phenotypic plasticity.
Ophraella communa LeSage (Coleoptera: Chrysomelidae), is native to North America (Futuyma, 1990; Palmer and Goeden, 1991), and it has been considered as an important biological control agent of invasive common ragweed, Ambrosia artemisiifolia L. (Asterales: Asteraceae) in China (Chen et al., 2009; Guo et al., 2011; Zhou et al., 2011c, 2014). A. artemisiifolia has caused serious economic, ecological, and health problems (Zhou et al., 2011a), and it has extensively invaded and established itself in over 21 provinces in China (Zhou et al., 2011a). A. artemisiifolia ages and dies after the end of October, and its seeds in soil germinate and grow new seedlings from late April to early June next year. O. communa adults often hibernate in soil from the end of October to early May or June of the following year. In general, air temperatures differ significantly with latitudes. The hibernating O. communa adults from different latitudes often need to overcome different low temperatures in the cold winter months. Our previous studies have demonstrated that hibernating O. communa use freeze avoidance to survive winter cold and geographically separated populations of O. communa are diverging with respect to baseline cold hardiness because of the differing severity of low temperatures experienced during the coldest winter season in each locality (Zhou et al., 2011a). The Yangzhou population may be one of the most northern populations of this species which ranges from Laibin, Guangxi Province, north to the border of Yangzhou City, Jiangsu Province. Moreover, significant differences were observed in cold hardiness across different seasonal populations of O. communa have been reported (Zhou et al., 2011b). This indicates the cold-hardiness plasticity of O. communa plays an important role in adapting to seasonal and geographic changes in air temperatures.
As A. artemisiifolia gradually expands northward, O. communa encounters a colder environment, and new selection pressures may result in genetic variation within the species and may even result in the rapid adaptive microevolution of chill tolerance traits. Short-term evolutionary potential depends on the intra-specific additive genetic variance. The additive variance is often measured as heritability, the fraction of the total phenotypic variance that is additive. In this way, heritability is a common measure of evolutionary potential. In general, the proportion of intra-population phenotypic variance (VP) due to additive genetic variation, termed narrow-sense heritability (h2), may be the most fundamental aspect of a trait’s genetic architecture, and has evolutionary significance (Lynch and Walsh, 1998; Visscher et al., 2008; Hoffmann and Sgro, 2011). In this study, coefficient of additive genetic variance (CVA) served as a measure of evolvability, because its numerical value has a more direct interpretation as the expected relative change in a trait under a unit strength of selection (Houle, 1992; Sgro and Hoffmann, 1998; Hansen et al., 2003). Few empirical studies have clearly demonstrated how the transgenerational effects contribute to insects’ adaptability to cold conditions. Previous studies in Drosophila melanogaster and Bemisia tabaci have produced evidence of adaptive cross-generation plasticity, which has suggested that variance in evaluability is greater when insects are exposed to a changing temperature stress (Cui et al., 2008; Mitchell and Hoffmann, 2010; Ma et al., 2014). Species with adequate genetic variability are often able to make rapid evolutionary adjustments in nature. In this way, temperature resistance plasticity of insects may be heritable (Ma et al., 2014). Therefore, understanding the evolutionary potential in cold resistance plasticity can help predict the future responses of O. communa to climate change.
In the present study, we hypothesize that the cold-hardiness plasticity of overwintering O. communa adults should be heritable in geographically separated cold climates in the winter months. Two geographical populations (Laibin and Yangzhou) were selected to estimate specific parameters crucial to cold tolerance, including super cooling point (Lee, 1989; Chen and Kang, 2002; Andreadis and Athanassiou, 2017), glycerol content (Krunic and Salt, 1971; Minder et al., 1984), and chill coma recovery time (Findsen et al., 2013) of the adults. The purpose of this study was to compare the evolutionary potential of the baseline cold hardiness of hibernating O. communa populations from two different geographical regions, and so provide insight into the potential of this beetle to range further into China. To this end, we used laboratory measurements to compare the heritability of cold hardiness in overwintering O. communa adults from the two geographical populations. Based on estimation of the heritability of cold hardiness, we will explain how O. communa establishes its populations successfully to survive cool climates during winter months in different latitude localities of China.
Materials and Methods
Host Plants and Insects
The beetle Ophraella communa LeSage can survive throughout the year on the common ragweed (Ambrosia artemisiifolia L.) from May to November in the open fields and hibernate in the soil during the winter months.
The overwintering O. communa adults were collected from naturally grown ragweed, in the fields of Laibin (Guangxi Zhuang Autonomous Region, South China) and Yangzhou (Jiangsu Province, East China) when they emerged from the soils in the spring 2014. The adults were maintained (two geographical populations were reared separately) in the laboratory at a temperature of 27 ± 1°C, a relative humidity of 70 ± 5% RH, and a light dark photoperiod of 14:10 h. The beetles were bred gregariously in insect rearing cages (40 cm × 60 cm). The cages contained ragweed plants for eating, climbing, molting, and hiding. The moisture levels (15.5–18.5%) were maintained along with sterilized soil to facilitate ragweed growth.
Laibin belongs to subtropical monsoon climate and its location is 23°43′7.47″N, 109°24′54.10″E, where the average lowest temperatures in November, December, January, and February were 6.7°C, 3.2°C, 2.6°C, and 4.7°C, respectively, and the average low temperatures in November, December, January, and February were 14.1°C, 10.3°C, 8.2°C, and 12.1°C, respectively. Yangzhou also belongs to subtropical monsoon climate and its location is 32°16′12.53″N, 119°10′31.99″E, where the average lowest temperatures in November, December, January, and February were -0.4°C, -5.4°C, -6.7°C, and -4.6°C, respectively, and the average low temperatures in November, December, January, and February were 7.7°C, 1.7°C, -0.5°C, and 2.1°C, respectively. Although Laibin and Yangzhou both belong to subtropical monsoon climate, Laibin have higher temperature all year-round, especially in winter (Figure 1). The data were provided by the China meteorological data service center (CMDC)1.
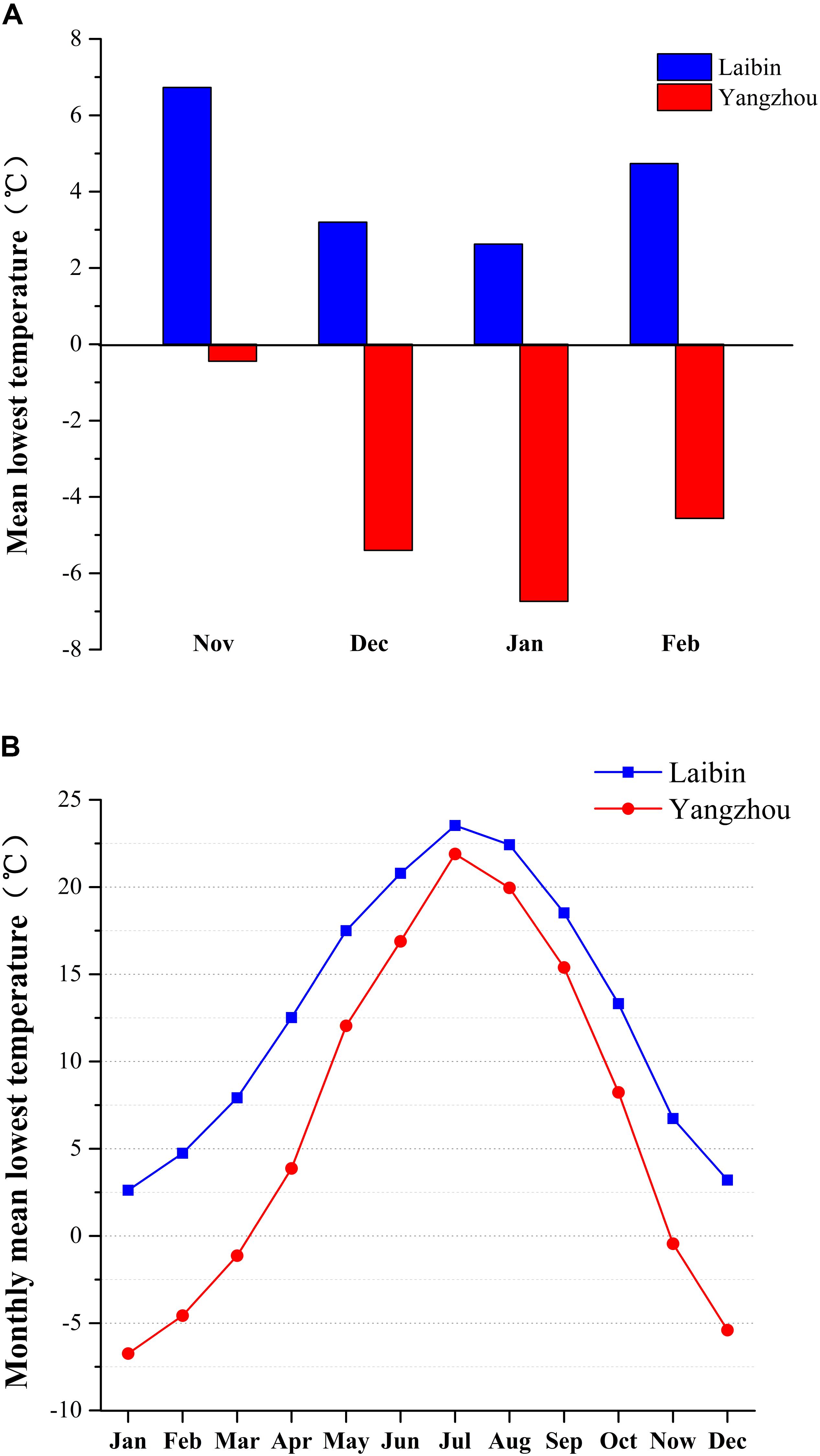
FIGURE 1. Mean lowest temperature, monthly mean low temperature, and monthly mean temperatures in Laibin and Yangzhou, China from 2007 to 2014. Data were obtained from the official website of China Meteorological Data Sharing Service System at http://data.cma.cn. The weather station providing these data is located in Laibin, Guangxi (23°43′7.47″N, 109°24′54.10″E) and Yangzhou, Jiangsu (32°16′12.53″N, 119°10′31.99″E). (A) The average monthly minimum temperatures in the winter months from 2007 to 2014. (B) The averages from daily minimum temperature in each month from 2007 to 2014.
Experimental Design and Data Collection
After a generation, male and female beetles’ generation 1 (G1) were transferred to separate plastic boxes (24 cm × 16 cm × 9 cm) covered with organdy mesh fabric. These were placed in a temperature-regulated laboratory within 24 h, and then maintained for 2 days on common ragweed plants in insect rearing cages. The two geographical populations were reared for one generation to ensure a large, effective population size.
Paternal Half-Sibling Breeding Design
When the adult beetles of generation 2 (G2) were 3 days old (sexual maturity), we randomly assigned one male (sire) to mate with five virgin females (dams) in turn, in a transparent plastic box containing a glass vial (3 cm × 8 cm) with a ragweed branch from each geographical population (N = 30 for one population). The ragweed branch was for mating, oviposition, climbing, molting, and hiding. After mating was complete, we gently removed each female to a new “house,” numbered them by mating pair, then placed another female in the old “house.” After all pairs had finished mating, we checked females’ oviposition daily. When the female laid her eggs, was removed from the vial by aspiration immediately, left the eggs behind. Then we observed the eggs until they hatched.
When their offspring, generation 3 (G3) emerged, 10 females of each combination were collected, reared on ragweed plants, mated with males, and scored for cold tolerance traits (two females for cold stress, two females for testing of super-cooling point and glycerol content separately) within 3 h of eclosion. The breeding experiment was performed in the laboratory at 27°C, at humidity of 65–75% RH under a photoperiod of 14L: 10D cycle with light coming on at 08:00.
Chill Coma Recovery Time-TRC
To compare the cold resistance between the populations and sexes, 400 individuals were randomly selected from each mass bred population and scored for knockdown resistance (100 females and 100 males per cold stress experiment) on the third day after eclosion.
Five adults were randomly selected from every replication, the centrifuge tubes were paced (one adult/tube) in temperature controller (-10 ± 0.1°C). The centrifuge tubes were removed, immediately rubbed dry, and placed on a piece of paper, where they were allowed to recover at room temperature (25°C) without any mechanical stimuli. Then we started a timer observed their activity, noted the recovery time, measured as period from placement on the paper to the beetle reaching an upright position.
Super-Cooling Point
We randomly selected 150 males and 150 females from both geographical populations, a thermocouple was used to test the super-cooling point, we cut one 2 mm aperture of the pipet tips, then placed the probe of thermo detector in said pipet tips. Connect the probe with the insect body, then put it in a low temperature refrigerator (-20°C and -30°C). Insects obtain marked cooling at an average rate of about 0.5°C/min, when the body fluids starts turning to ice, latent heat is released within the insect, producing a large bending curve in the recorder, distinguished the super-cooling point (when the curve rose to a certain value, and began to decline; we recorded the freezing point) numbered and recorded the data.
Determination of Glycerol Content
Glycerol contents were estimated from 100 randomly selected males and 100 randomly selected females from both geographical populations (400 specimens in total). Experimental procedures were conducted according to the kit instructions (provided by Nanjing Jiancheng Bioengineering Institute) and data were recorded.
Statistical Analysis
Data were analyzed using mixed modeling within a restricted maximum likelihood framework implemented in Proc Mixed in SAS (version 9.2. SAS Institute Inc., Cary, NC, United States) with following the model:
Here the constant (α) was ensemble average of each protocol. A previous study suggested that observer error may affect the estimation of variance components for thermal tolerance traits (Castaneda et al., 2012). All three protocols, (knockdown test, super-cooling point assays, and determination of glycerol content), were performed by a single person. We here considered protocol run (B) as a fixed effect, and three random effects were fit: sire (ds), dam nested within sire (dd), and the residual (e.).
The total phenotypic variance () for the breeding design for estimating genetic parameters was represented as follows:
Here , , and , are the sire, dam, and intra-group level variance components, respectively. Because we used a half-sib breeding design, the sire variance, , is one-fourth of the additive genetic variance (VA) (McGuigan and Blows, 2010). Thus, to estimate VA, we multiplied the sire variance by four.
The additive genetic variance for each trait was first estimated using a univariate model. Log likelihood ratio tests were performed, where the final model for each trait was compared to a model in which was set to zero to determine whether the levels of additive genetic variance for each trait were significantly different from zero (de Assis et al., 2010; McGuigan and Blows, 2010; Simonsen and Stinchcombe, 2010). The phenotypic variance (VP) in knockdown resistance was computed using all known relationships among individuals. We then estimated the narrow-sense heritability for both traits. Narrow-sense heritability for each trait was estimated as the additive genetic variance (VA) divided by the total phenotypic variance (VP) (Frankham, 1996). We conducted Student’s t-tests to determine whether the variance components and heritability estimates differed significantly. Estimates of evolvability, IA, and the additive genetic coefficient of variance,
where is the trait mean (Hansen et al., 2011), were also computed for both traits.
For the population and sexual comparisons (G3), two-way ANOVA was performed to evaluate the differences in the indexes, followed by the Tukey multiple comparison test. The population (Laibin and Yangzhou) and sex (female and male) were used as the two factors. P < 0.05 was considered statistically significant.
Results
Differences in Cold Resistance Between the Populations and Sexes
Figure 2 showed the mean value of Trc, SCP, and GC in two populations of O. communa beetles (G3). An ANOVA indicated significant differences between the two populations (F3,178 = 74.99, P < 0.0001) and between males and females (F3,178 = 19.55, P < 0.0001) in Trc (Figure 2A). Similar results were observed for SCP of population (F3,199 = 20.77, P < 0.0001) and sex (F3,199 = 23.13, P < 0.0001), and GC of population (F3,397 = 10.41, P = 0.0014) and sex (F3,397 = 9.01, P = 0.0029). Generally females showed markedly more cold resistance than males.
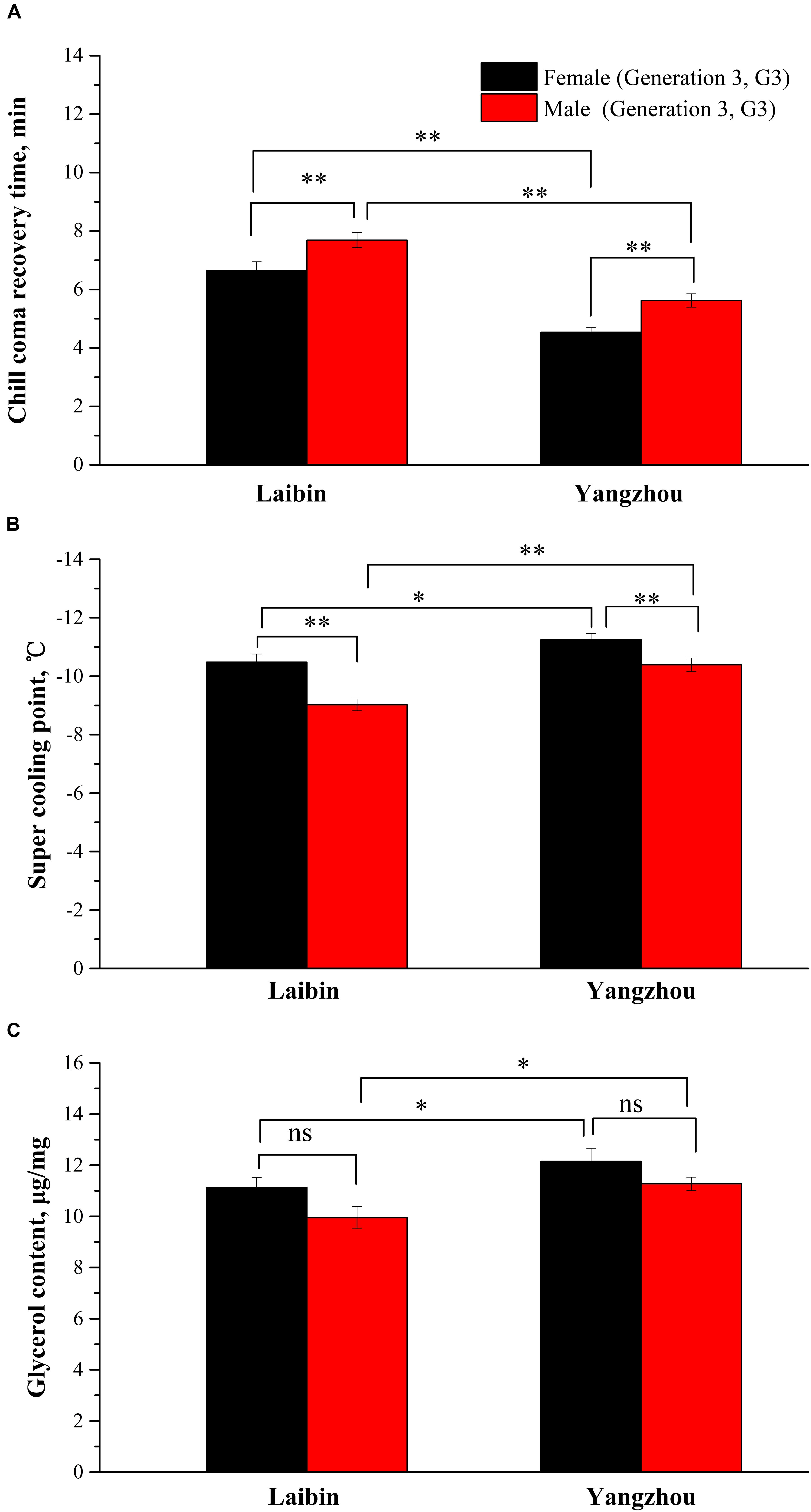
FIGURE 2. Index of cold resistance between sexes. (A) Chill coma recovery time (min). (B) Super-cooling point (°C). (C) Glycerol content (μg/mg) for the Laibin (G3) and Yangzhou population (G3) of Ophraella communa beetles (mean ± SE). ∗P < 0.05, ∗∗P < 0.01.
Heritability and Genetic Variance Components
As shown in Table 1, the estimates of genetic variance differed statistically significantly between the two populations, Narrow-sense heritability estimates were significantly different for the chill coma recovery times of both the Laibin and Yangzhou populations, at values of 0.39 ± 0.01∗ and 0.53 ± 0.01∗, respectively. Significant levels of additive genetic variance were detected in chill coma recovery time (2.15 ± 0.01) of the Laibin population and in chill coma recovery time (5.44 ± 0.02) and super-cooling point (1.67 ± 0.22) of the Yangzhou population. For chill coma recovery time, the narrow-sense heritability estimates were significant for both the Laibin (0.39 ± 0.01∗) and Yangzhou populations (0.53 ± 0.01∗). The coefficient of environmental variation was slightly larger (CVE of glycerol content, 30.34 > 27.37) for the Laibin population and that of and additive genetic variation was slightly smaller (CVA of chill coma recovery time, 0.539 < 1.111; CVA of super-cooling point, 0.181 < 0.393; CVA of glycerol content, 0.231 < 0.250).
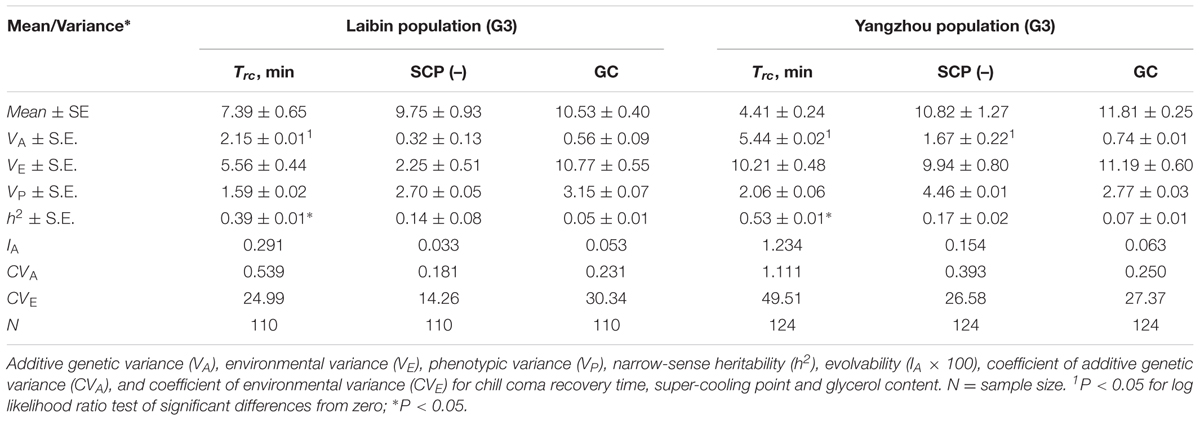
TABLE 1. Narrow-sense heritability (h2), variance, and coefficient of variation components for chill coma recovery time (Trc), super-cooling point (SCP), and glycerol content (GC) (Laibin and Yangzhou populations) of Ophraella communa beetles.
Discussion
Different populations react differently to cold stress. What one population finds very stressful another may not (Overgaard et al., 2011; Hoffmann, 2013). Temperature has an important influence on insect population distribution, life history, behavior, and species abundance (Dahlgaard et al., 2001; Kimura, 2004; Hoffmann, 2010). For many insects, chill tolerance is crucial to the ability to persist in cold environments (Findsen et al., 2013). Cold resistance is associated with the distributions of many insect species, particularly in temperate regions. Insects have low levels of resistance. A. artemisiifolia has become distributed across over 21 provinces from Southern to Northern China, covering a range of different climatic temperatures (Guo et al., 2011), its seeds grow new seedlings from late April to early June next year (Teshler et al., 2001). O. communa can still survive the cold winter months in the field (Zhou et al., 2011b). In the research studies conducted, we found that the hibernation survival range of beetle population in Langfang City, Hebei Province (39°30′38″N, 116°36′2″E) (Zhou, unpublished data), is still smaller than the range of ragweed. Previous studies have suggested there may be significantly differences in cold hardiness among different seasonal and geographic populations of O. communa (Zhou et al., 2011a,b), and it is important to determine whether cold tolerance can be inherited by different geographic populations.
In our study, we have further documented genetic differences in cold tolerance between populations of O. communa. The Yangzhou population was found to be more cold-tolerant than in the Laibin population. This indicates a significant difference in the adaptive strategies to cold climates between the Laibin and Yangzhou populations of O. communa. As shown, the Yangzhou population can survive in a colder climatic environment than the Laibin population. In general, adapting and shifting their geographical distributions rendered some species better adapted to their environment (Kirkpatrick and Peischl, 2012). Previous studies have suggested that conspecific populations of the fruit fly Drosophila melanogaster from different environments may vary substantially in stress resistance because of acclimation to local conditions (Hoffmann et al., 2005). This is particularly evident when exploring the relationship between cold tolerance and minimal environmental temperature, such that strong correlations exist between cold tolerance and environmental distribution (Chen et al., 2011; Kellermann et al., 2012b).
Given adequate genetic variability, insect species are often able to make rapid evolutionary adjustments. Under pressure of the global climate warming, the majority of studies on insect adaptation have focused on the heat resistance of poikilotherms. Estimates of heritability in D. melanogaster based on knockdown time experiments at 39°C suggest values ranging from 0.03 to 0.28 (Huey et al., 1992; McColl et al., 1996), whereas experiments at 38°C suggest values ranging from 0.14 to 0.22 (Mitchell and Hoffmann, 2010). These estimates might not be directly comparable to those obtained here, which ranged from 0.47 to 0.51 at 45°C, due to the difference in the temperature used to assess the trait and to the different species examined. Nevertheless, the values obtained here showed that a substantial proportion of the total phenotypic variation in heat resistance was caused by additive genetic variation. Few studies have examined the genetic variance of cold resistance (Huey et al., 1992; Charo-Karisa et al., 2005; Kellermann et al., 2009). Estimates of heritability on recovery from a chill coma at 0°C suggest values ranging from 0.01 to 0.38 (Charo-Karisa et al., 2005). Our estimates of heritability for O. communa based on chill coma recovery time at -10°C (0.39–0.53) also indicated that a substantial proportion of the total phenotypic variation was caused by additive genetic variation.
Insects typically need to overcome cold conditions in order to become established in a given habitat (Bale, 1996). Cold acclimation, heritability and evolutionary potential could all drive cold hardiness (Sinclair et al., 2003). Cold acclimation was found to have enormous benefits at low temperatures, such that only cold-acclimated flies were able to find resource when they were released into cold environments (Kristensen et al., 2008). Because the insects that prey on pest species tend to follow insect pests into new areas, cold resistance is an important part of their arsenal. A. artemisiifolia has spread from south to north in China, so O. communa needs to adapt more colder winter climates if it is to suppress the population of A. artemisiifolia in colder areas in the northern subtropics or even in temperate regions. Based on the results of our study, we found O. communa populations to have considerable evolutionary potential with respect to cold tolerance in colder areas, and its heritability and evolutionary potential drive cold hardiness. This indicates that the beetle has a good plasticity of cold tolerance in nature, so it may be able to overcome the cold climate in Northern China whether the beetle experiences further artificial or natural cold acclimation.
Author Contributions
ZZ conceived and designed the work, and also edited the manuscript. CZ and FM performed the experiments and wrote the manuscript. HC and JG helped with the theoretical analysis. FW helped to revise the manuscript.
Funding
This work was supported by the National Natural Science Foundation of China for Excellent Young Scholars (No. 31322046) and National Natural Science Foundation of China (No. 31672089).
Conflict of Interest Statement
The authors declare that the research was conducted in the absence of any commercial or financial relationships that could be construed as a potential conflict of interest.
Acknowledgments
We would like to thank Yao Wang (Institute of Plant Protection, Chinese Academy of Agricultural Sciences) for help during the experiment. We also thank LetPub for editing the English.
Footnotes
References
Andreadis, S. S., and Athanassiou, C. G. (2017). A review of insect cold hardiness and its potential in stored product insect control. Crop Prot. 91, 93–99. doi: 10.1016/j.cropro.2016.08.013
Arboleda-Bustos, C. E., and Segarra, C. (2011). The Dca gene involved in cold adaptation in Drosophila melanogaster arose by duplication of the ancestral regucalcin gene. Mol. Biol. Evol. 28, 2185–2195. doi: 10.1093/molbev/msr040
Bale, J. S. (1996). Insect cold hardiness: a matter of life and death. Eur. J. Entomol. 93, 369–382.
Bale, J. S. (2002). Insects and low temperatures: from molecular biology to distributions and abundance. Philos. Trans. R. Soc. B 357, 849–862. doi: 10.1098/rstb.2002.1074
Castaneda, L. E., Calabria, G., Betancourt, L. A., Rezende, E. L., and Santos, M. (2012). Measurement error in heat tolerance assays. J. Therm. Biol. 37, 432–437. doi: 10.1016/j.jtherbio.2012.03.005
Charo-Karisa, H., Rezk, M. A., Bovenhuis, H., and Komen, H. (2005). Heritability of cold tolerance in Nile tilapia, Oreochromis niloticus, juveniles. Aquaculture 249, 115–123. doi: 10.1016/j.aquaculture.2005.04.029
Chen, B., and Kang, L. (2002). Cold hardiness and supercooling capacity in the pea leafminer Liriomyza huidobrensis. Cryo Lett. 23, 173–182.
Chen, H., Zhou, Z., Guo, J., Wang, M., and Peng, Z. (2009). A review on population control of the ragweed, Ambrosia artemisiifolia. Plant Protect. 35, 20–24.
Chen, I. C., Hill, J. K., Ohlemuller, R., Roy, D. B., and Thomas, C. D. (2011). Rapid range shifts of species associated with high levels of climate warming. Science 333, 1024–1026. doi: 10.1126/science.1206432
Cui, X., Wan, F., Xie, M., and Liu, T. (2008). Effects of heat shock on survival and reproduction of two whitefly species, Trialeurodes vaporariorum and Bemisia tabaci biotype B. J. Insect Sci. 8:24. doi: 10.1673/031.008.2401
Dahlgaard, J., Hasson, E., and Loeschcke, V. (2001). Behavioral differentiation in oviposition activity in Drosophila buzzatii from highland and lowland populations in Argentina: plasticity or thermal adaptation? Evolution 55, 738–747.
Danks, H. V. (1996). The wider integration of studies on insect cold-hardiness. Eur. J. Entomol. 93, 383–403.
de Assis, G. M. L., Ruggieri, A. C., Mercadante, M. E. Z., de Camargo, G. M. F., and Carneiro Júnior, J. M. (2010). Selection of alfalfa cultivars adapted for tropical environments with repeated measures using PROC MIXED of SAS® System. Plant Genet. Resour. 8, 55–62. doi: 10.1017/S1479262109990153
Findsen, A., Andersen, J. L., Calderon, S., and Overgaard, J. (2013). Rapid cold hardening improves recovery of ion homeostasis and chill coma recovery time in the migratory locust. Locusta migratoria. J. Exp. Biol. 216, 1630–1637. doi: 10.1242/jeb.081141
Futuyma, D. J. (1990). Observations on the taxonomy and natural history of Ophraella Wilcox (Coleoptera: Chrysomelidae), with a description of a new species. J. N. Y. Entomol. Soc. 98, 163–186.
Gibert, P., Moreteau, B., Pétavy, G., Karan, D., and David, J. R. (2001). Chill-coma tolerance, a major climatic adaptation among Drosophila species. Evolution 55, 1063–1068. doi: 10.1111/j.0014-3820.2001.tb00623.x
Guo, J., Zhou, Z., Zheng, X., Chen, H., Wan, F., and Luo, Y. (2011). Control efficiency of leaf beetle, Ophraella communa, on the invasive common ragweed, Ambrosia artemisiifolia, at different growing stages. Biocontrol Sci. Technol. 21, 1049–1063. doi: 10.1080/09583157.2011.603823
Hansen, T. F., Pélabon, C., and Houle, D. (2011). Heritability is not evolvability. Evol. Biol. 38:258. doi: 10.1007/s11692-011-9127-6
Hansen, T. F., Pélabon, C., Armbruster, W. S., and Carlson, M. L. (2003). Evolvability and genetic constraint in Dalechampia blossoms: components of variance and measures of evolvability. J. Evol. Biol. 16, 754–765. doi: 10.1046/j.1420-9101.2003.00556.x
Hendry, A. P., and Kinnison, M. T. (1999). Perspective: the pace of modern life: measuring rates of contemporary microevolution. Evolution 53:1637. doi: 10.2307/2640428
Hoffmann, A. A. (2003). Low potential for climatic stress adaptation in a rainforest Drosophila species. Science 301, 100–102. doi: 10.1126/science.1084296
Hoffmann, A. A. (2010). Physiological climatic limits in Drosophila: patterns and implications. J. Exp. Biol. 213, 870–880. doi: 10.1242/jeb.037630
Hoffmann, A. A. (2013). Upper thermal limits in terrestrial ectotherms: how constrained are they? Funct. Ecol. 27, 934–949. doi: 10.1111/j.1365-2435.2012.02036.x
Hoffmann, A. A., and Sgro, C. M. (2011). Climate change and evolutionary adaptation. Nature 470, 479–485. doi: 10.1038/nature09670
Hoffmann, A. A., Shirriffs, J., and Scott, M. (2005). Relative importance of plastic vs genetic factors in adaptive differentiation: geographical variation for stress resistance in Drosophila melanogaster from eastern Australia. Funct. Ecol. 19, 222–227. doi: 10.1111/j.1365-2435.2005.00959.x
Hoffmann, A. A., Sørensen, J. G., and Loeschcke, V. (2003). Adaptation of Drosophila to temperature extremes: bringing together quantitative and molecular approaches. J. Therm. Biol. 28, 175–216. doi: 10.1016/S0306-4565(02)00057-8
Houle, D. (1992). Comparing evolvability and variability of quantitative traits. Genetics 130, 195–204.
Huey, R. B., Crill, W. D., Kingsolver, J. G., and Weber, K. E. (1992). A method for rapid measurement of heat or cold resistance of small insects. Funct. Ecol. 6, 489–494. doi: 10.2307/2389288
Kellermann, V., Loeschcke, V., Hoffmann, A. A., Kristensen, T. N., Fløjgaard, C., David, J. R., et al. (2012a). Phylogenetic constraints in key functional traits behind species climate niches: patterns of desiccation and cold resistance across 95 Drosophila species. Evolution 66, 3377–3389. doi: 10.1111/j.1558-5646.2012.01685.x
Kellermann, V., Overgaard, J., Hoffmann, A. A., Flojgaard, C., Svenning, J. C., and Loeschcke, V. (2012b). Upper thermal limits of Drosophila are linked to species distributions and strongly constrained phylogenetically. Proc. Natl. Acad. Sci. U.S.A. 109, 16228–16233. doi: 10.1073/pnas.1207553109
Kellermann, V., van Heerwaarden, B., Sgro, C. M., and Hoffmann, A. A. (2009). Fundamental evolutionary limits in ecological traits drive Drosophila species distributions. Science 325, 1244–1246. doi: 10.1126/science.1175443
Kimura, M. T. (2004). Cold and heat tolerance of drosophilid flies with reference to their latitudinal distributions. Oecologia 140, 442–449. doi: 10.1007/s00442-004-1605-4
Kirkpatrick, M., and Peischl, S. (2012). Evolutionary rescue by beneficial mutations in environments that change in space and time. Philos. Trans. R. Soc. B 368:20120082. doi: 10.1098/rstb.2012.0082
Kostal, V., Sula, J., and Simek, P. (1998). Physiology of drought tolerance and cold hardiness of the Mediterranean tiger moth Cymbalophora pudica during summer diapause. J. Insect Physiol. 44, 165–173. doi: 10.1016/S0022-1910(97)00047-4
Kristensen, T. N., Hoffmann, A. A., Overgaard, J., Sorensen, J. G., Hallas, R., and Loeschcke, V. (2008). Costs and benefits of cold acclimation in field-released Drosophila. Proc. Natl. Acad. Sci. U.S.A. 105, 216–221. doi: 10.1073/pnas.0708074105
Krunic, M. D., and Salt, R. W. (1971). Seasonal changes of glycerol content and supercooling points of Megachile rotundata L. and M. relativa Crass. Can. J. Zool. 49, 663–666. doi: 10.1139/z71-104
Lee, R. E. Jr. (1989). Insect cold-hardiness: to freeze or not to freeze. Bioscience 39, 308–313. doi: 10.2307/1311113
Lynch, M., and Walsh, B. (1998). Genetics and the Analysis of Quantitative Traits. Sunderland, MA: Sinauer Associates,Inc.
Ma, F., Lü, Z., Wang, R., and Wan, F. (2014). Heritability and evolutionary potential in thermal tolerance traits in the invasive Mediterranean Cryptic species of Bemisia tabaci (Hemiptera: Aleyrodidae). PLoS One 9:e103279. doi: 10.1371/journal.pone.0103279
McColl, G., Hoffmann, A. A., and McKechnie, S. W. (1996). Response of two heat shock genes to selection for knockdown heat resistance in Drosophila melanogaster. Genetics 143, 1615–1627.
McGuigan, K., and Blows, M. W. (2010). Evolvability of individual traits in a multivariate context: partitioning the additive genetic variance into common and specific components. Evolution 64, 1899–1911. doi: 10.1111/j.1558-5646.2010.00968.x
Merilä, J., and Crnokrak, P. (2001). Comparison of genetic differentiation at marker loci and quantitative traits. J. Evol. Biol. 14, 892–903. doi: 10.1046/j.1420-9101.2001.00348.x
Merilä, J., Sheldon, B. C., and Kruuk, L. E. (2001). Explaining stasis: microevolutionary studies in natural populations. Genetica 11, 199–222. doi: 10.1023/A:1013391806317
Minder, I. F., Dudash, A. V., and Chesnek, S. I. (1984). Seasonal changes in the resistance to cold and the content of glycogen, glycerine and reducing sugars in the body of hibernating larvae of the codling moth (Laspeyresia pomonella). Zoologicheskii Zhurnal 63, 1355–1362.
Mitchell, K. A., and Hoffmann, A. A. (2010). Thermal ramping rate influences evolutionary potential and species differences for upper thermal limits in Drosophila. Funct. Ecol. 24, 694–700. doi: 10.1111/j.1365-2435.2009.01666.x
Overgaard, J., Hoffmann, A. A., and Kristensen, T. N. (2011). Assessing population and environmental effects on thermal resistance in Drosophila melanogaster using ecologically relevant assays. J. Therm. Biol. 36, 409–416. doi: 10.1016/j.jtherbio.2011.07.005
Overgaard, J., Malmendal, A., Sørensen, J. G., Bundy, J. G., Loeschcke, V., Nielsen, N. C., et al. (2007). Metabolomic profiling of rapid cold hardening and cold shock in Drosophila melanogaster. J. Insect Physiol. 53, 1218–1232. doi: 10.1016/j.jinsphys.2007.06.012
Palmer, W. A., and Goeden, R. D. (1991). The host range of Ophraella communa Lesage (Coleoptera: Chrysomelidae). Coleopts. Bull. 45, 115–120.
Regniere, J., and Bentz, B. (2007). Modeling cold tolerance in the mountain pine beetle, Dendroctonus ponderosae. J. Insect Physiol. 53, 559–572. doi: 10.1016/j.jinphys.2007.02.007
Reznick, R., Regehr, G., MacRae, H., Martin, J., and McCulloch, W. (1997). Testing technical skill via an innovative “bench station” examination. Am. J. Surg. 173, 226–230. doi: 10.1016/S0002-9610(97)89597-9
Roff, D. (2003). Evolution: evolutionary danger for rainforest species. Science 301, 58–59. doi: 10.1126/science.1087384
Salt, R. W. (1961). A comparison of injury and survival of larvae of Cephus cinctus Nort, after intracellular and extracellular freezing. Can. J. Zool. 39, 349–357. doi: 10.1139/z61-038
Sgro, C. M., and Hoffmann, A. A. (1998). Effects of temperature extremes on genetic variances for life history traits in Drosophila melanogaster as determined from parent-offspring comparisons. J. Evol. Biol. 11, 1–20. doi: 10.1007/s000360050063
Simonsen, A. K., and Stinchcombe, J. R. (2010). Quantifying evolutionary genetic constraints in the ivyleaf morning glory. Ipomoea hederacea. Int. J. Plant Sci. 171, 972–986. doi: 10.1086/656512
Sinclair, B. J., Addo-Bediako, A., and Chown, S. L. (2003). Climatic variability and the evolution of insect freeze tolerance. Biol. Rev. Camb. Philos. Soc. 78, 181–195. doi: 10.1017/S1464793102006024
Teshler, M. P., DiTommaso, A., Gagnon, J. A., and Watson, A. K. (2001). “Ambrosia artemisiifolia L., Common Ragweed (Asteraceae),” in Biological Control Programmes in Canada, eds P. G. Mason and J. T. Huber (Wallingford: The Centre for Agriculture and Bioscience International), 290–294.
Visscher, P. M., Hill, W. G., and Wray, N. R. (2008). Heritability in the genomics era - concepts and misconceptions. Nat. Rev. Genet. 9, 255–266. doi: 10.1038/nrg2322
Wang, H. S., and Kang, L. (2005). Effect of cooling rates on the cold hardiness and cryoprotectant profiles of locust eggs. Cryobiology 51, 220–229. doi: 10.1016/j.cryobiol.2005.07.003
Worland, M. R., Wharton, D. A., and Byars, S. G. (2004). Intracellular freezing and survival in the freeze tolerant alpine cockroach Celatoblatta quinquemaculata. J. Insect Physiol. 50, 225–232. doi: 10.1016/j.jinsphys.2003.12.001
Zhou, Z., Chen, H., Zheng, X., Guo, J., Guo, W., Li, M., et al. (2014). Control of the invasive weed Ambrosia artemisiifolia with Ophraella communa and Epiblema strenuana. Biocontrol Sci. Technol. 24, 950–964. doi: 10.1080/09583157.2014.897305
Zhou, Z., Guo, J., Li, M., Ai, H., and Wan, F. (2011a). Seasonal changes in cold hardiness of Ophraella communa. Entomol. Exp. Appl. 140, 85–90. doi: 10.1111/j.1570-7458.2011.01128.x
Zhou, Z., Guo, J., Michaud, J. P., Li, M., and Wan, F. (2011b). Variation in cold hardiness among geographic populations of the ragweed beetle, Ophraella communa LeSage (Coleoptera: Chrysomelidae), a biological control agent of Ambrosia artemisiifolia L. (Asterales: Asteraceae), in China. Biol. Invasions 13, 659–667. doi: 10.1007/s10530-010-9857-x
Keywords: Ophraella communa, heritability, cold hardiness, super cooling point, glycerol content, chill coma recovery time
Citation: Zhao C, Ma F, Chen H, Wan F, Guo J and Zhou Z (2018) Heritability and Evolutionary Potential Drive Cold Hardiness in the Overwintering Ophraella communa Beetles. Front. Physiol. 9:666. doi: 10.3389/fphys.2018.00666
Received: 07 March 2018; Accepted: 14 May 2018;
Published: 05 June 2018.
Edited by:
Su Wang, Beijing Academy of Agriculture and Forestry Sciences, ChinaReviewed by:
Dandan Wei, Southwest University, ChinaShannon Bryn Olsson, National Centre for Biological Sciences, India
Copyright © 2018 Zhao, Ma, Chen, Wan, Guo and Zhou. This is an open-access article distributed under the terms of the Creative Commons Attribution License (CC BY). The use, distribution or reproduction in other forums is permitted, provided the original author(s) and the copyright owner are credited and that the original publication in this journal is cited, in accordance with accepted academic practice. No use, distribution or reproduction is permitted which does not comply with these terms.
*Correspondence: Zhongshi Zhou, zhongshizhou@yahoo.com
†These authors have contributed equally to this work as joint first authors.