- The State Key Laboratory of Power Transmission Equipment & System Security and New Technology, Chongqing University, Chongqing, China
Plasma-activated water (PAW) is produced by microhollow cathode discharge (MHCD), where air is the working gas used. The treatment effect of PAW against Penicillium is expressed by the germination inhibition rate and the sporoderm-breakage rate. The maximum values of the germination inhibition rate and the sporoderm-breakage rate are 82.31 and 66.91%, respectively. In this paper, the importance of the reaction of HNO2 and H2O2 in Penicillium inactivation is illustrated, which is most likely due to its product—ONOOH. The effective impact of ONOOH on inactivation beyond its theoretical lifetime is indirectly reflected by the treatment efficiency, and the complexity of peroxynitrite chemistry may be responsible for prolonging this effect. Moreover, according to the difference of concentration magnitude between HNO2 and H2O2, and the reaction of them under acidic conditions, a method to increase the H2O2 production is demonstrated, which can contribute to improving the yield of ONOOH.
Introduction
Recently, the demand for food with low preservatives and low processing levels has increased, and the requirements for food quality have also improved. How to quickly and effectively kill pathogens on food surfaces without affecting its quality is one a key issue in the field of food safety and preservation. In order to overcome these problems, plasma-activated water (PAW) is being rapidly developed. It has an outstanding inactivation ability and can efficiently inactivate a wide variety of micro-organisms [1–5]. Compared with using the plasma directly, the main advantage of PAW is its harmlessness. The dangers of the electric field, charged particles, and thermal damage to tissue can be avoided by the PAW treatment with only reactive species [6]. PAW that is generated by treating water with plasma differs from the conventional disinfection method, which uses chemicals, and represents an environmentally friendly and cost-effective bactericide. Based on the above advantages, PAW has been widely used and has made satisfactory progress in many fields, especially in the food industry [7, 8].
Air plasma that is generated by dielectric barrier discharge (DBD), gliding arc, or corona discharge adjacent to water creates an acidic solution that contains hydrogen peroxide (H2O2), nitrate (), and nitrite () anions, along with other long-lived secondary products [9–14]. These species may be partly responsible for the extended biological effects of PAW. Additional effects are exerted by short-lived reactive species, especially the post-discharge evolution of these species.·OH, O, HO2, ONOOH, and O2NOOH have been reported to be present in PAW [15–18]. ONOOH is considered by many researchers to be of substantial significance in PAW inactivation. For example, Brisset and Hnatiuc [15] and Brisset and Pawlat [17] presumed that ONOOH is likely to be an intermediate in acidic solutions and analyzed its formation pathway. Lukes et al. [19] provided evidence of the formation of ONOOH in PAW through a pseudo-second-order post-discharge reaction of H2O2 and HNO2. However, these studies are all analytical and do not explain the importance of ONOOH from an experimental perspective. Theoretically, the lifetime of ONOOH is very short [20]. In addition, it requires a period of time after being obtained to be adopted for PAW, which is at least on the order of seconds. It is unknown whether PAW remains effective in practical applications beyond the lifetime of ONOOH, namely, whether the inactivation efficiency is affected by ONOOH. Very few experiments have been conducted to investigate this. To resolve this uncertainty, in this study, the behaviors of ONOOH in the process are analyzed, namely its biological effects. Furthermore, it was proven in a previous study that adding water into the working gas can increase the content of OH radicals in the plasma [21–23] and, subsequently, increase the yield of H2O2. Is there a way to improve the production of ONOOH if it does have an important role in the application? To address the issue, in this paper, air-water microhollow cathode discharge (MHCD) plasma-jet-activated water is generated for the study of the treatment efficiency.
Fungi are ubiquitous in nature and closely related to human life. Most fungi are saprophytic and not pathogenic, and even play significant roles in various fields. However, a few fungal species present a threat to agricultural production by causing diseases such as rice blast, [24] wheat rust, [25], and citrus rot [26]. Hence, the research on fungi is of substantial importance. However, in previous studies, attention was rarely paid to the effects of PAW against fungi. Furthermore, the treatment effect of fungi is reflected by the germination inhibition rate, which is a typical parameter for an evaluation method that is used in biology [27]. Hence, the fungi do not germinate for a period of time. This does not mean that they are actually inactivated and can no longer germinate, but just that they are inhibited, and these spores may continue to germinate during the extended incubation time. Thus, the germination inhibition rate is not equal to the inactivation rate. Then, how many fungi have actually been inactivated? And is it possible to conduct a more in-depth investigation? In this study, the treatment effect of PAW on Penicillium, which is used to represent typical fungi, is investigated more thoroughly, thereby resulting in sufficient analysis of the relevant mechanisms.
Materials and Methods
Penicillium Preparation
The Italicum Sacc, which is a species of Penicillium, was obtained from the Chinese Academy of Agricultural Sciences Citrus Research Institute,. The P. digitatum was inoculated using potato dextrose agar medium and incubated for 5–7 days at 25°C. Then, the most viable spores were selected for the treatment, diluted with sterilized water to 2.0 × 107 per milliliter, and kept in the refrigerator at 4°C. For plasma treatment, 3 mL of sterilized water was injected into petri dishes 35 mm in diameter, and the distance from the equipment nozzle to the suspension surface was ~4 mm. Then, 150 μL of PAW was added to 60 μL of the spore suspension. It was necessary to vibrate the spore suspension for 1 min to mix it homogeneously prior to treatment. After a period of time, 600 μL of the medium was added and placed in a constant-temperature incubator shaker for 16 h to germinate the spores of P. digitatum. The temperature was set to 25°C and the rotating speed was set to 220 r min−1.
Experimental Setup
The experimental setup is schematically illustrated in Figure 1. Plasma activated water used in this study is generated by treating water with a microhollow cathode discharge (MHCD) jet device at atmospheric pressure. The MHCD jet is set above the surface of the water, which is a common method to produce PAW [4, 8]. The reactor consisted of two metal molybdenum electrodes and a sapphire insulator, where the cathode and anode were made of metal molybdenum and had diameters of 15 and 30.5 mm, respectively. The sapphire between two electrodes acted as the dielectric layer, which was 30.5 mm in diameter and 0.6 mm thick. These dimensions were designed to effectively avoid surface discharge between the two electrodes. All three layers had a microhole with a diameter of 0.5 mm in the center. By adjusting the coaxial alignment of the three microholes during assembly, the gas flowed through the micropore and formed the discharge channel. To ensure the safety of the operator, a clamping device that was made of Teflon was used to prevent high-voltage shock. As illustrated in Figure 1, the power supply to the microhollow cathode discharge device was provided by a negative HVDC power supply (Xi'an Zhaofu Electronic Company: 0–30 and 2 kW), and its output voltage range was 0 to −30 kV and power was 2 kW. A 400 kΩ resistor was connected in series between the negative high-voltage output of the power supply and the cathode of the MHCD device, with the objectives of limiting the current and ensuring the stable operation of the plasma jet. The gas flow consisted of two parts: the bottle air flow QA, which was almost dry air, and the humid air flow QW, which was obtained by passing through the homemade Monteggia bottle that contained the secondary distilled water. The water content in the working gas was precisely adjusted by two mass flow controllers (MFCs) of model Sevenstar D07-7 and could be varied in a well-defined way (from 2.53 to 9.57 mg L−1) by changing the settings of the gas flowmeters of the two parts. The relationship between water ratio and water content was evaluated by the Karl Fischer titration method. The main advantage of the gas-liquid mixing method that is used in this experiment was that the particles of water vapor in the obtained working gas were sufficiently small for distributing equably in the gas flow to realize a stable condition. The pure bottle air that was used in this study was provided by Wangmiao Industrial Trading Company in Chongqing, and its water content was <1 ppm.
Measurement Methods
To identify the gas-phase active components that were produced by the MHCD jet, FTIR spectroscopy in the spectral range from 400 to 4,000 cm−1 was used, which was conducted in a poly tetra fluoro ethylene (PTFE) cell using a Nicolet iS50 with a spectral resolution of 2 cm−1. The cell consisted of two KBr windows, each with a diameter of 4 cm, which were positioned at each end of the tube, while the gas inlet and outlet were fixed sideways along its length of 10 cm. A vacuum pump was used to evacuate the gas-phase product from the cell to obtain a more accurate result. Furthermore, the ultraviolet-visible spectrophotometer (Beijing BeiFen RayLeigh Company, UV 2200) was adopted to measure the active species in PAW, such as and . The measurement was done by placing the PAW in a cuvette and then placing the cuvette in the spectrophotometer. And the content of H2O2 that was generated by the air–water MHCD in the phosphate buffer saline (PBS) buffer was detected via electrochemical measurement.
Results and Discussion
Germination Inhibition by Plasma-Activated Water
In this study, to explore the ability of plasma-activated water (PAW) to inactivate Penicillium, a series of experiments are conducted under various parameter conditions and the treatment efficiency of the air-water MHCD plasma jet is evaluated in terms of the germination inhibition rate of the spores, which is calculated via Equation 1. In this formula, Sc and St represent the rates at which spores are germinated in the control group and the treatment group, respectively, where the germination rate is obtained by dividing the number of germinated spores by the total number in each group. The main advantage of this approach is that is excludes some of the spores that are not originally germinated. During the experiment, we are surprised to find that the total number of spores in the treated group is significantly reduced compared with the control group under various conditions. For example, as shown in Figure 2, which presents the spore germination figure of Penicillium at the applied voltage of −6 kV and treatment time of 9 min, the total number of spores decreases to 106 in the treatment group (Figure 2b) compared with 350 in the control group (Figure 2a). When preparing the solution, its concentration in the experimental group is the same as that in the control group; hence, the numbers of spores should be approximately equal. However, now there is a large difference in the total number of spores, which could be related to sporoderm breakage caused by PAW. Then, the commonly used method for calculating the germination inhibition rate (Equation 1) is no longer applicable. Since the number of broken spores is not known, we use the total number of spores in the control group as that of the experimental group to reduce the error. Therefore, Equation 2 is adopted. It expresses that, based on Equation 1, the total number of spores in the experimental group is equal to that in the control group, in which the inhibition effect of PAW on the spores can be identified.
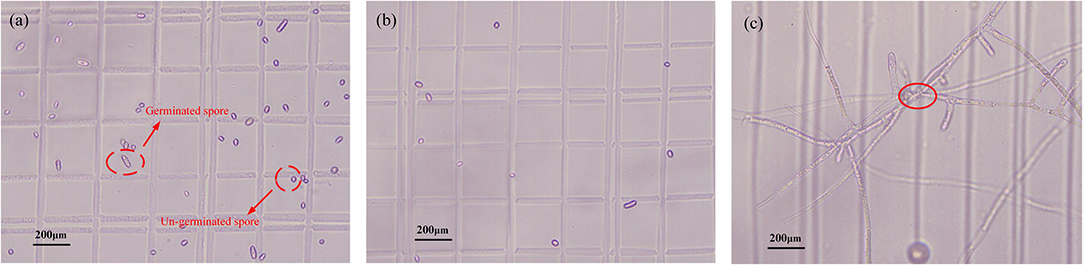
Figure 2. Spore germination of Penicillium (at a magnification of 400 times). (a) The control group, (b) after PAW treatment, and (c) spores that grew into hyphae after prolonged cultivation.
Equation 1:
where Sc and St are the rates of spore germination in the control group and the treatment group, respectively.
Equation 2:
where Nc and Nt are the numbers of spores that were germinated in the control group and the treatment group, respectively.
In this experiment, the cultivation time is set to 16 h. Within a limited time, it is easy to distinguish the germinated and the ungerminated spores; however, it is difficult to distinguish the spores after long-term cultivation. Since some spores grew into hyphae and intertwined with each other after prolonged cultivation, those fungi are impossible to recognize under a microscopic view. In the red circle in Figure 2c, we cannot determine whether there is one bifurcated spore or two intertwined spores. Hence, via experiments, we selected 16 h as the cultivation time.
In the experiment, it is demonstrated that the inhibition efficiency is strongly affected by the applied voltage, processing time, and storage time. Treatment time, response time, and storage time, respectively, refers to the treatment of water by the jet, the treatment of fungi spores solution by PAW, and the period of time after being obtained to be adopted for PAW. First, the change laws of the germination inhibition rate of PAW with the applied voltage and the treatment time are investigated when the response time is set at 10 min, the water content is 9.57 mg L−1, and the storage time is 5 min, as plotted in Figure 3. From the result, we find that the inhibition efficiency increases with the increase of the applied voltage, namely, the plasma-activated water at a high voltage is favorable for inhibiting Penicillium. When the treatment time is 3 min and the applied voltage is −3 kV, the corresponding germination inhibition rate is 41.40%. Then, the inhibition rate increases to 72.91% as the voltage is increased to −6 kV. This behavior is also suitable for other treatment times. Figure 3 shows the change in the inhibition rate with the treatment time. According to Figure 3, the inhibition efficiency increases with the treatment time when the other conditions remain unchanged. For example, under the −6 kV condition, when the treatment time is set at 3 min, the inhibition efficiency of the plasma-activated water against Penicillium is 72.91%. When the treatment time increases to 9 min, the corresponding germination inhibition rate increases to 82.31%. This behavior is similar to that under other voltage conditions. Thus, increasing the applied voltage or treatment time improves the inhibition efficiency of PAW.
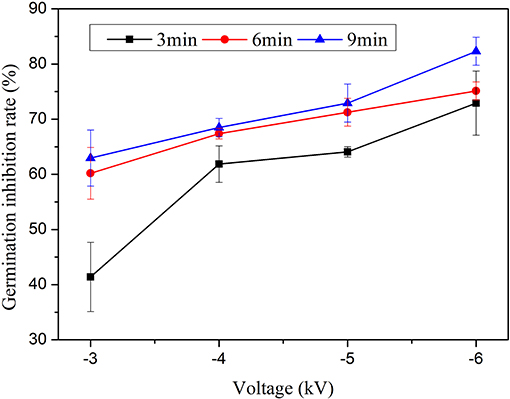
Figure 3. Changes in the inhibition efficiency with the treatment time at various voltages when the water content is 9.57 mg L−1, the response time is 10 min, and the storage time is 5 min.
Figure 4 plots the change curves of the inhibition efficiency under various response times when the treatment time is 6 min, storage time is 5 min, and the water content is 9.57 mg L−1. The reaction time is measured from the addition of PAW into the Penicillium suspension to the addition of a large amount of the medium to the mixture (to prevent PAW from continuing to act on Penicillium) [14]. The results prove that the germination inhibition rate against Penicillium increases substantially when the response time increases from 5 to 15 min. Figure 5 plots the variation of the germination inhibition efficiency with the storage time. The germination inhibition rate significantly decreases with the increase in the delay time. The PAW that has been stored for 24 h remains effective for the treatment of Penicillium; however, its efficiency is reduced ~2 times. For example, in the case of −3 kV voltage and 9.57 mg L−1 water content, when the storage time is set to 0 min, the germination inhibition rate is 63.52%, and it decreases to 33.11% as the storage time increases to 24 h. The behavior at the −6 kV condition is similar. Among all the experimental conditions, the germination inhibition rate attains its highest value of 82.31% at −6 kV applied voltage when the treatment time and water content are 9 min and 9.57 mg L−1, respectively. Moreover, according to Figure 5, there was no significant difference in inhibition efficiency within the first 15 min. The fastest relative reduction occurs in the time range from 15 to 30 min. Hence, the active species that are generated in the liquid are sufficiently stable to continue acting within a limited time, while the inhibition efficiency of the liquids decreases substantially upon a longer delay.
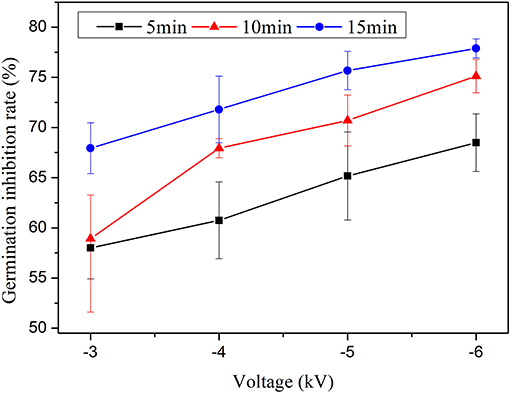
Figure 4. Changes in the germination inhibition efficiency with the response time when the treatment time is 6 min, the storage time is 5 min, and the water content is 9.57 mg L−1.
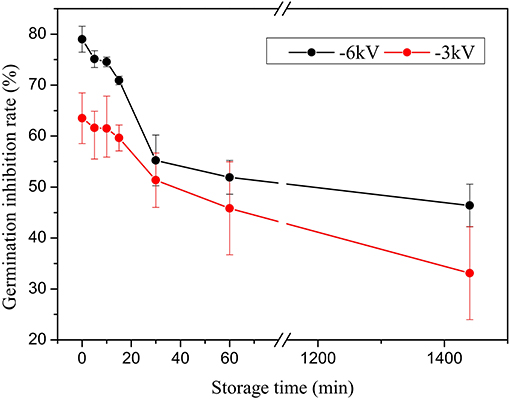
Figure 5. Changes in the germination inhibition efficiency with the storage time in the case of 6 min treatment time, 9.57 mg L−1 water content, and 10 min response time.
Measurements of the Sporoderm-Breakage Rate
The total number of spores rapidly decreases, as shown in Figure 2. Hence, the sporoderms of some spores are directly broken with the application of PAW. The strong inactivation effect of PAW is also observed here. The spores that are broken are inactivated and no longer germinate. If we can quantify the proportion of spores that are broken, it will be useful for studying the properties of PAW. Hence, the sporoderm-breakage rate is measured. DNA is present in cells, and each spore contains DNA. If the sporoderm is broken, the DNA spills out of the cell and is destroyed; hence, the number of spores that remain in the solution is reflected by the DNA content. DNA, namely, deoxyribonucleic acid, is similar to other substances, such as proteins and nitric acid, that have high absorbance at distinct wavelengths. Therefore, it can be measured by a spectrometer. The method is described as follows: Destruction of the cytoderm is a prerequisite for DNA extraction [28]. Therefore, we apply the method of grinding with liquid nitrogen to destroy the cytoderm. To prevent insufficient milling, lywallzyme (Beijing TianGen Company, RT410) is added to further degrade the sample. After that, a mixture of various substances is obtained, which mainly include sugars, proteins, and DNA. Then, the Plant Genomic DNA Kit (Beijing TianGen Company, DP305) is used to perform targeted extraction of the DNA from the mixture, and the content of DNA is measured via spectrophotometry. In Figure 6, the variation of the sporoderm-breakage rate with the treatment time is plotted. As the treatment time increases, the sporoderm-breakage rate increases. For instance, when the voltage and water content are fixed at −6 kV and 9.57 mg L−1, respectively, the sporoderm-breakage rate is 50.9% at 3 min treatment time, and when the treatment time is 9 min, the corresponding sporoderm-breakage rate is 66.9%. The results demonstrate that the spores are completely inactivated due to the sporoderm being broken.
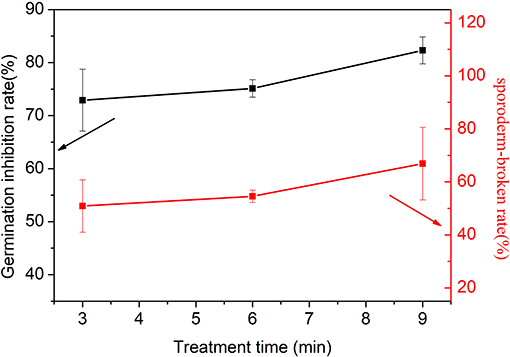
Figure 6. Variations of the germination inhibition rate and sporoderm-breakage rate with the treatment time under 9.57 mg L−1 water content, −6 kV voltage, 10 min response time, and 5 min storage time.
The inactivated Penicillium spores are divided into two parts: those with a broken sporoderm and those that have been inactivated but not broken. The latter are still present in PAW and do not germinate under longer culture. Since other spores grew into hyphae and entangle with each other after prolonged cultivation, as shown in Figure 2, we cannot judge those spores that are inactivated but not broken. Therefore, the consideration of both the germination inhibition rate and the sporoderm-breakage rate will be the most comprehensive method for evaluating the treatment effect of PAW on the fungi.
Detection and Analysis of Products in PAW
To obtain additional insight into the process, liquid analytics are necessary. Therefore, we use an ultraviolet spectrophotometer to detect active species in PAW. The existence of both and is obtained from the UV-VIS spectra, as shown in Figure 7. A group of 5 peaks in the range of 330–395 nm is a characteristic profile of [29]. The peak at 302 nm is mainly attributed to [30]. The measured absorbance values are converted to absolute concentrations through a standard curve. The experimental results demonstrate that the concentration of increases whereas that of decreases upon longer exposure to plasma, where a transformation between them is observed. As expressed in reactions (1) - (2), the disproportionation of nitrites in acidic medium and the reaction with OH may be partly responsible for this result. In addition, the yields of and both increase with the applied voltage. For example, when the applied voltage and treatment time are set at −4 kV and 9 min, respectively, the concentration of is 7.45 mmol/L and that of is 1.92 mmol/L. As the voltage increases to −6 kV, the corresponding concentrations are 10.83 and 2.5 mmol/L. The behaviors of and are consistent with our results of germination inhibition and indicate that those in the solution may play an important role in the inactivation properties of PAW.
According to previous studies, products in PAW may include O3, , , H2O2. However, neither O3 nor H2O2 is detected in this study.
To assess these processes comprehensively, liquid analytics must be combined with plasma diagnostic investigations. Thus, the gas products of a plasma jet are observed via an FTIR absorption measurement. The variations of two infrared spectral regions with the voltage and water content are plotted in Figure 8, in which the main products are nitrogen oxides such as NO2, NO, and N2O, without the absorption of O3. The experimental results demonstrate that the products in the plasma jet are strongly affected by the applied voltage. For instance, when the flow rate and water content are set at 2.5 L min−1 and 2.53 mg L−1, respectively, the peak value of NO increases with the applied voltage. The main reason for this is related to reactions (3)-(5). With respect to NO2, when the water content is fixed at 2.53 mg L−1, as the applied voltage increases from 3 to 6 kV, its peak value increases, which is mainly due to reactions (6)-(8). Therefore, combined with reactions (9)-(10), this indicates that these products, as precursors of the liquid products, contribute to the changes in HNO2 and HNO3 in PAW. In addition, the behaviors of the gas products as functions of the voltage are consistent with our results from the obtained UV spectra. Since the gaseous products in this system do not include O3, almost no O3 is detected in the PAW within the detection limit; instead, products of further reaction of nitrogen oxides are detected. Most likely, due to the small-hole discharge, inside of which is a small arc, the local temperature is high, thereby resulting in O3 not being detected. However, since the gas in the small holes blows the arc, the arc is cooled; hence, the blowing temperature is not high. For H2O2, the reaction (11) is widely recognized [31–33]. In the case of excess HNO2, H2O2 is completely reacted, thereby resulting in its absence from PAW.
Significance and Lifetime of ONOOH in PAW
To determine whether the treatment effect of PAW is realized only by the mixture of HNO2 and HNO3, we mixed HNO2 and HNO3 solutions in which the two species are in the same concentration as PAW. We found that the inhibition effect is non-significant and the germination inhibition rate is only 8.48%. Furthermore, at concentrations of nearly 102 times the initial concentrations, an inhibition rate of 60.71% was realized, which is similar to the treatment effect of PAW. In the case of such a low germination inhibition rate, the sporoderm-breakage rate must be much lower. Hence, the combination of HNO2 and HNO3 does not play a central role in the process, and it should not be the main factor for sporoderm-breakage of Penicillium. Therefore, the additional species or products that are generated from the plasma treatment play an important or synergistic role in the inhibition properties of PAW.
Considering the reaction of nitrites (HNO2) with hydrogen peroxide (H2O2) to form peroxynitrous acid (ONOOH) under acidic conditions, it is speculated that ONOOH plays an important role in the application of PAW. Due to the overlapping spectra of HNO3 and ONOOH as isomers, ONOOH cannot be detected in PAW. However, this does not mean that it is not present. Thus, we adopt the method of mixing H2O2 and HNO2 to obtain a solution with the same concentrations as in PAW, and we further investigate the treatment effect of ONOOH. To conduct the experiment, we determine the amount of H2O2 that is produced in PAW. In this experiment, the content of H2O2 that is generated by treating phosphate buffer saline (PBS) buffer using the MHCD is detected via electrochemical measurement. Under this condition, the reaction between HNO2 and H2O2 is prevented. The method was described in detail in previous papers [21, 34, 35].
Figure 9 plots the variations of the concentration of H2O2 with the voltage and treatment time when the water content is 9.57 mg L−1 and the flow rate is 2.5 L min−1. The experiment results demonstrate that the concentration of H2O2 that is produced increases with the applied voltage. For example, when the treatment time is set at 9 min, the concentration of H2O2 ranges from 1.26 μmol L−1 at −3 kV up to 3.08 μmol L−1 at −6 kV. In addition, the H2O2 content that is produced in the PBS buffer increases with the treatment time. For example, in the case of an applied voltage of −6 kV and a water content of 9.57 mg L−1, when the jet processing time is 3 min, the generated H2O2 concentration is 1.02 μmol L−1. If the processing time increases to 9 min, the corresponding H2O2 concentration is 3.08 μmol L−1. The behaviors at other applied voltages (−3, −4, and −5 kV) is similar. The variation of H2O2 accords with that of the germination inhibition rate in the presence of sufficient HNO2, thereby suggesting that the intermediate products of ONOOH that are generated by the reaction between H2O2 and HNO2 may play a synergistic role in this process.
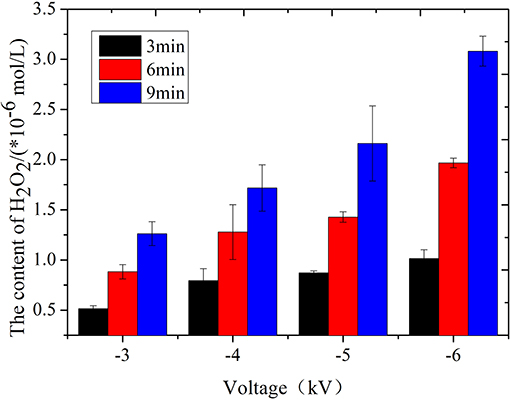
Figure 9. Effect of the treatment time of the air-water jet on the content of H2O2 at four applied voltages.
Combined with the results for H2O2 that are presented above, the relationship between the contents of ·OH radicals and H2O2 is analyzed. H2O2 may be generated via reactions (12-16) when the voltage is <-4.5 kV, where some of the H2O2 is detected. As the parameters are increased, more ·OH radicals are produced, which increases the number of pathways for generating H2O2, as expressed in reactions (17) and (18).
To further explore whether or not ONOOH plays an important role in the process, H2O2 is added into the mixture of HNO3 and HNO2, namely, mixture solutions with the same concentrations of HNO3, HNO2, and H2O2 as in the PAW that is produced under −6 kV voltage, 6 min treatment time, 10 min response time, and 9.57 mg L−1 water content are prepared. Then, these mixtures are treated with Penicillium. According to Figure 10, the inhibition effect of the solution is significantly improved and is close to that of PAW at the corresponding concentrations. The inhibition effect is ~8 times as strong compared with the solution to which only HNO3 and HNO2 have been added. This indicates that in addition to HNO2 and HNO3, the product ONOOH generated by the reaction of H2O2 and HNO2 is involved, and it enhances the treatment effect. Due to the strong increase in the treatment efficiency, the sporoderm-breakage rate is measured to determine whether it is related to ONOOH. According to the results, the maximum sporoderm-breakage rate is attained at 47.49%, which is even higher than the germination inhibition rate under the condition that H2O2 is not added. It is demonstrated that ONOOH is generated by the reaction of HNO2 and H2O2 under acidic conditions and plays an important role in improving the germination inhibition rate and the sporoderm-breakage rate.
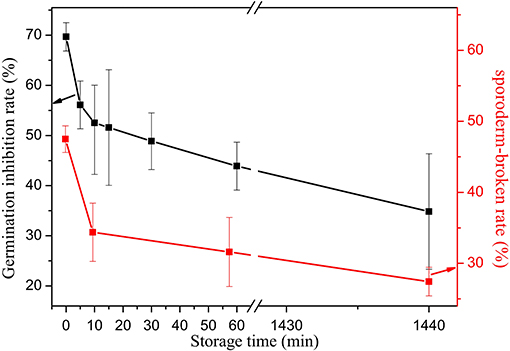
Figure 10. Variations of the germination inhibition rate and the sporoderm-breakage rate with the storage time for a mixed solution.
ONOOH is a transient substance, and its lifetime is very short. The germination inhibition rate of PAW that has been aged for 24 h is still almost 6 times that of the HNO2 and HNO3 mixture. Is this caused by ONOOH? And is the high efficiency of PAW attributable to the important role that is played by ONOOH? To answer these questions, the variation of the treatment efficiency with the storage time for the HNO3, HNO2, and H2O2 mixture is studied. According to Figure 10, although both the sporoderm-breakage rate and the germination inhibition rate for the mixture decrease with the increase of the storage time, we find that the mixture is also effective after being stored for 1,440 min and the magnitude of the reduction with the storage time is ~30%, which is similar to that of PAW. In addition, the treatment effect of in an acidic environment is largely intensified by H2O2, namely, it is proven that ONOOH has a substantial impact on inactivation beyond its theoretical lifetime. Since ONOOH and HNO3 are isomers, it is not possible to detect ONOOH via UV spectroscopy, Raman spectroscopy, or mass spectroscopy. However, in this study, we detect the presence of ONOOH. Moreover, the reason why a transient substance can age for 24 h is analyzed as follows: Approximately 30% of the ONOOH, which is a short-lived substance, is decomposed into ·OH and radicals [reaction (19)], while the remainder transforms to nitric acid, which is immediately followed by dissociation into and H+ [reaction (20)] [36, 37]. However, due to Le Chatelier's principle, in the case of a high HNO3 concentration, the latter reaction is expected to be significantly weakened. Hence, ONOOH may be present when the HNO3 concentration is high. In addition, ONOOH may be present in the cyclic reaction to prolong its effect in a short period of time, as indicated in blue in Figure 11. Nevertheless, as time goes on, complex chemical kinetics are not sufficient to maintain it, and ONOOH is gradually dissipated, thereby resulting in reduced inactivation efficiency. Overall, the complexity of the peroxynitrite chemistry is related to the prolonged treatment phenomena of PAW.
Moreover, although the addition of H2O2 into the mixture of HNO3 and HNO2 significantly improves the treatment efficiency against Penicillium, it is not as effective as PAW with the same concentration, even if it is used immediately after mixing. Therefore, in addition to ONOOH, other substances are involved in the cyclic kinetic process and enhance the treatment effect. We posit that and O− are also useful. A species such as cannot be obtained by mixing HNO3, HNO2, and H2O2; however, it could be generated by the collision of electrons with O2 or O3 in the plasma discharge region. The strong performance of in medical inactivation has been proven in previous research. O− can be acquired by or ·OH in the discharge area. As transient substances, their lifetimes are very short. However, combined with the cyclic reactions of O−- O3- HO2 – and OH – O− – – O3, which are marked in red in Figure 11, it is reasonably speculated that and O− remain effective beyond their longevity, thereby contributing to the stronger effect of PAW than the mixture. Their behaviors and the roles that are played by these species in the process merit further exploration in the future.
Possible Ways to Increase the Yield of ONOOH
According to the analysis above, an indirect indication of the important role of ONOOH is proposed; hence, increasing the yield of ONOOH will have a positive effect on the treatment of Penicillium. According to the data that are presented above, the productions of HNO2 and H2O2 differ by an order of magnitude, namely, the yield of HNO2 is much larger than that of H2O2. Therefore, according to the reaction of H2O2 and HNO2 under acidic conditions, more H2O2 can contribute to more ONOOH being produced. Thus, we studied a method for improving H2O2 production. Figure 12 plots the variation curve of the concentration of H2O2 with the water content when the treatment time is 9 min and the flow rate is 2.5 L min−1. The experimental results demonstrate that, with increasing water content, the concentration of H2O2 increases substantially. For instance, when the applied voltage and the treatment time are fixed at −6 kV and 9 min, respectively, the H2O2 content increases to 3.08 μmol L−1 at 9.57 mg L−1 water content from 1.03 μmol L−1 at 2.53 mg L−1 water content. Therefore, increasing the water content in the working gas contributes to the formation of H2O2.
To verify our assumption, the variations of the germination inhibition rate and the sporoderm-breakage rate of PAW with the water content are investigated when the response time is set at 10 min, the voltage is −6 kV, and the storage time is 5 min, as shown in Figure 13. It is confirmed that the inhibition efficiency is strongly affected by the water content, namely, when the water content is increased, the germination inhibition rate increases substantially. For example, under the treatment time of 6 min, when the water content is 2.5 L min−1, the germination inhibition rate is 63.52%, which increases to 75.12% when the water content reaches 9.57 mg L−1. At the same time, according to Figure 13, the sporoderm-breakage rate increases when increasing the water content of the working gas. For example, under the condition of 9 min treatment time, when the water content is fixed at 9.57 mg L−1, the corresponding sporoderm-breakage rate is 43.96%, which increases to 66.91% as the water content increases to 9.57 mg L−1. This behavior is also observed for other treatment times.
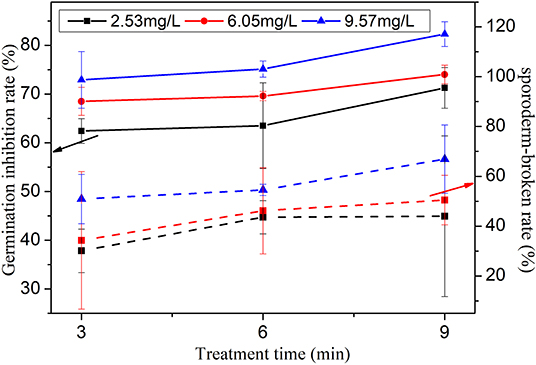
Figure 13. Variations of the germination inhibition rate and the sporoderm-breakage rate with the water content and the treatment time under −6 kV voltage, 10 min response time, and 5 min storage time.
According to the experimental results, the germination inhibition rate and the sporoderm-breakage rate effectively increase as the water content in the working gas increases, which is most likely attributable to the increase in ONOOH production through the reaction between HNO2 and H2O2. Thus, adding water into the working gas of the MHCD jet may be an effective approach for increasing the concentration of ONOOH in PAW. It also indirectly indicates the important role of ONOOH in the application of PAW.
Conclusion
In this paper, for the application of PAW to Penicillium inactivation, the general formula for calculating the germination inhibition rate is modified to minimize the experimental errors as much as possible. In addition, the sporoderm-breakage rate is utilized to assess the treatment effect of PAW on fungi, which is the most comprehensive method. We find that the maximum values of the germination inhibition rate and the sporoderm-breakage rate are 82.31 and 66.91%, respectively. The high treatment efficiency of PAW is demonstrated not only for bacteria but also for fungi. This result is meaningful because the topic of plasma agriculture is very hot, and fungi are abundant in agriculture. Moreover, we artificially prepare a solution with the corresponding concentrations of H2O2, HNO3, and HNO2 in PAW. It is demonstrated from the results that the reaction of H2O2 and HNO2 is involved in inactivating Penicillium, and its product ONOOH may play an important role in the process. Furthermore, the effective impact of ONOOH on inactivation beyond the theoretical lifetime is indirectly reflected by the treatment efficiency, and the complexity of peroxynitrite chemistry is responsible for prolonging its effect. Moreover, according to the difference of concentration magnitude between HNO2 and H2O2, and the reaction of them under acidic conditions, a method to increase the H2O2 production is demonstrated, which can contribute to improving the yield of ONOOH. It can reflect on the germination inhibition rate and the sporoderm-breakage rate.
Data Availability Statement
The raw data supporting the conclusions of this article will be made available by the authors, without undue reservation.
Author Contributions
KL and SL: designed experiments and wrote the manuscript. SL and CR: carried out the experiments. KL, SL, and CR: analyzed experimental results. All authors: contributed to the article and approved the submitted version.
Funding
This work was supported by National Natural Science Foundation of China (NSFC) under Grants No. 51877021, and sponsored by Natural Science Foundation of Chongqing under Grants No. cstc2018jcyjAX0449, Fundamental Research Funds for the Central Universities under Grants No. 2018CDQYDQ0004, and Key Research and Development Plan of Science & Technology Department of Sichuan Province under Grants No. 2017GZ0094.
Conflict of Interest
The authors declare that the research was conducted in the absence of any commercial or financial relationships that could be construed as a potential conflict of interest.
References
1. Kamgang-Youbi G, Herry JM, Brisset JL, Bellon-Fontaine MN, Doubla A, Naïtali M. Impact on disinfection efficiency of cell load and of planktonic/adherent/detached state: case of Hafnia alvei inactivation by plasma activated water. Appl Microbiol Biot. (2008) 81:449–57. doi: 10.1007/s00253-008-1641-9
2. Naïtali M, Kamgang-Youbi G, Herry JM, Bellon-Fontaine MN, Brisset JL. Combined Effects of long-living chemical species during microbial inactivation using atmospheric plasma-treated water. Appl Environ Microb. (2010) 76:7662–4. doi: 10.1128/AEM.01615-10
3. Kamgang-Youbi G, Herry JM, Meylheuc T, Brisset JL, Bellon-Fontaine MN, Doubla A, et al. Microbial inactivation using plasma-activated water obtained by gliding electric discharges. Appl Microbiol. (2009) 48:13–18. doi: 10.1111/j.1472-765X.2008.02476.x
4. Zhang Q, Liang YD, Feng HQ, Ma RN, Tian Y, Zhang J, et al. A study of oxidative stress induced by non-thermal plasma-activated water for bacterial damage. Appl Phys Lett. (2013) 102:203701. doi: 10.1063/1.4807133
5. Oehmigen K, Hähnel M, Brandenburg R, Wilke CH, Weltmann KD, Woedtke TV. the role of acidification for antimicrobial activity of atmospheric pressure plasma in liquids. Plasma Process Polym. 7:250–7. doi: 10.1002/ppap.200900077
6. Ercan UK, Wang H, Ji H, Fridman G, Brooks AD, Joshi SG. Nonequilibrium plasma-activated antimicrobial solutions are broad-spectrum and retain their efficacies for extended period of time. Plasma Process Polym. (2013) 10:544–55. doi: 10.1002/ppap.201200104
7. Patange A, Lu P, Boehm D, Cullen PJ, Bourke P. Efficacy of cold plasma functionalised water for improving microbiological safety of fresh produce and wash water recycling. Food Microbiol. (2019) 84:103226. doi: 10.1016/j.fm.2019.05.010
8. Ma RN, Yu S, Tian Y, Wang K, Sun CD, Li X, et al. Effect of non-thermal plasma-activated water on fruit decay and quality in postharvest Chinese bayberries. Food Bioprocess Tech. (2016) 9:1825–34. doi: 10.1007/s11947-016-1761-7
9. Liu ZC, Liu DX, Chen C, Li D, Yang AJ, Rong MZ, et al. Physicochemical processes in the indirect interaction between surface air plasma and deionized water. J Phys D Appl Phys. (2015) 48:495201. doi: 10.1088/0022-3727/48/49/495201
10. Moussa D, Abdelmalek F, Benstaali B, Addou A, Hnatiuc E, Brisset JL. Acidity control of the gliding arc treatments of aqueous solutions: application to pollutant abatement and biodecontamination. Eur Phys J Appl Phys. (2005) 29:189–99. doi: 10.1051/epjap:2004211
11. Kozakova Z, Klimova EJ, Obradovic BM, Dojcinovic BP, Krcma F, Kuraica MM, et al. Comparison of liquid and liquid-gas phase plasma reactors for discoloration of azo dyes: Analysis of degradation products. Plasma Process Polym. (2018) 15:e1700178. doi: 10.1002/ppap.201700178
12. Joshi AA, Locke BR, Arce P, Finney WC. Formation of hydroxyl radicals, hydrogen peroxide and aqueous electrons by pulsed streamer corona discharge in aqueous solution. J Hazard Mater. (1995) 41:3–30. doi: 10.1016/0304-3894(94)00099-3
13. Oehmigen K, Winter J, Hähnel M, Wilke C, Brandenburg R, Weltmann KD, et al. Estimation of possible mechanisms of escherichia coli inactivation by plasma treated sodium chloride solution. Plasma Process Polym. (2011) 8:904–13. doi: 10.1002/ppap.201000099
14. Traylor MJ, Pavlovich MJ, Karim S, Hait P, Sakiyama Y, Clark DS, et al. Long-term antibacterial efficacy of air plasma-activated water. J Phys D Appl Phys. (2011) 44:472001. doi: 10.1088/0022-3727/44/47/472001
15. Brisset JL, Hnatiuc E. Peroxynitrite: a re-examination of the chemical properties of non-thermal discharges burning in air over aqueous solutions. Plasma Chem Plasma. (2012) 32:655–74. doi: 10.1007/s11090-012-9384-x
16. Tresp H, Hammer MU, Winter J, Weltmann KD, Reuter S. Quantitative detection of plasma-generated radicals in liquids by electron paramagnetic resonance spectroscopy. J Phys D Appl Phys. (2013) 46:435401. doi: 10.1088/0022-3727/46/43/435401
17. Brisset JL, Pawlat J. Chemical effects of air plasma species on aqueous solutes in direct and delayed exposure modes: discharge, post-discharge and plasma activated water. Plasma Chem Plasma. (2016) 36:355–81. doi: 10.1007/s11090-015-9653-6
18. Machala Z, Tarabova B, Hensel K, Spetlikova E, Sikurova L, Lukes P. Formation of ROS and RNS in water electro-sprayed through transient spark discharge in air and their bactericidal effects. Plasma Process Polym. (2013) 10:649–59. doi: 10.1002/ppap.201200113
19. Lukes P, Dolezalova E, Sisrova I, Clupek M. Aqueous-phase chemistry and bactericidal effects from an air discharge plasma in contact with water: evidence for the formation of peroxynitrite through a pseudo-second-order post-discharge reaction of H2O2 and HNO2. Plasma Sources Sci Technol. (2014) 23:015019. doi: 10.1088/0963-0252/23/1/015019
20. Juliane LF, Sergey AN, Mitchio O, Coleen MR, Joseph SF, Paul OW. Cis-cis and trans-perp HOONO: action spectroscopy and isomerization kinetics. J Chem Phys. (2004) 121:1432–48. doi: 10.1063/1.1760714
21. Liu K, Wang CY, Hu HM, Lei JZ, Han L. Indirect treatment effects of water–air MHCD jet on the inactivation of penicillium digitatum suspension. IEEE T Plasma Sci. (2016) 44:2729. doi: 10.1109/TPS.2016.2608926
22. Srivastava N, Wang CJ. Effects of water addition on OH radical generation and plasma properties in an atmospheric argon microwave plasma jet. J Appl Phys. (2011) 110:053304. doi: 10.1063/1.3632970
23. Li L, Nikiforov A, Xiong Q, Britun N, Snyders R, Lu X, et al. OH radicals distribution in an Ar-H2O atmospheric plasma jet. Phys Plasmas. (2013) 20:093502. doi: 10.1063/1.4820945
24. Dean RA, Talbot NJ, Ebbole DJ. The genome sequence of the rice blast fungus Magnaporthe grisea. Nature. (2005) 434:980–6. doi: 10.1038/nature03449
25. Hovmoller MS, Yahyaoui AH, Milus EA, Justesen FA. Rapid global spread of two aggressive strains of a wheat rust fungus. Mol Ecol. (2008) 17:3818–26. doi: 10.1111/j.1365-294X.2008.03886.x
26. Timmer LW. Preventive and curative trunk treatments for control of phytophthora foot rot of citrus. Phytopathology. 67:1149. doi: 10.1094/Phyto-67-1149
27. He JL, Wu DT, Zhang Q, Chen H, Li HY, Han QH, et al. Efficacy and mechanism of cinnamon essential oil on inhibition of colletotrichum acutatum isolated from ‘Hongyang’ Kiwifruit. Front Microbiol. (2018) 9:1288. doi: 10.3389/fmicb.2018.01288
28. Dauphin LA, Moser BD, Bowen MD. Evaluation of five commercial nucleic acid extraction kits for their ability to inactivate Bacillus anthracis spores and comparison of DNA yields from spores and spiked environmental samples. J Microbiol Methods. (2009) 76:30–7. doi: 10.1016/j.mimet.2008.09.004
29. Piskarev IM. Effect of spark discharge plasma on water, physiological saline, Hanks' solution. High Energy Chem. (2018) 52:348–54. doi: 10.1134/S0018143918040124
30. Friedman HA, Toth LM, Bell JT. Method for Selectively Reducing Plutonium Values by a Photochemical Process. United States Patent. 4131527 (1978).
31. Anbar M, Taube H. Interaction of nitrous acid with hydrogen peroxide and with water. J Am Chem Soc. (1954) 76:6243–47. doi: 10.1021/ja01653a007
32. Tarabová B, Luke P, Hammer MU, Jablonowski H, von Woedtke T, Reuter S, et al. Fluorescent measurement of peroxynitrite /peroxynitrous acid in cold air plasma treated aqueous solutions. Phys Chem Chem Phys. (2019) 21:8883–96. doi: 10.1039/C9CP00871C
33. Takahama U, Tanaka M, Oniki T, Hirota S. Reactions of thiocyanate in the mixture of nitrite and hydrogen peroxide under acidic conditions: Investigation of the reactions simulating the mixture of saliva and gastric juice. Free Radic Res. (2007) 41:627–37. doi: 10.1080/10715760701218566
34. Yu L, Tian Y, Gao A, Shi Z, Liu Y, Li C. Bi-Module sensing device to in situ quantitatively detect hydrogen peroxide released from migrating tumor cells. PLoS ONE. (2015) 10:0127610-1. doi: 10.1371/journal.pone.0127610
35. Shi ZZ, Wu XS, Gao L, Tian Y, Yu L. Electrodes/paper sandwich devices for in situ sensing of hydrogen peroxide secretion from cells growing in gels-in-paper 3-dimensional matrix. Anal Methods. (2014) 6:4446. doi: 10.1039/C4AY00695J
36. Goldstein S, Lind J, Merenyi G. Chemistry of peroxynitrites as compared to peroxynitrates. Chem Rev. (2005) 105:2457–70. doi: 10.1021/cr0307087
Keywords: ONOOH, plasma activated-water, Penicillium, sporoderm-breakage rate, water containing
Citation: Liu K, Liu S and Ran C (2020) The Effect of Air-Water-Plasma-Jet-Activated Water on Penicillium: The Reaction of HNO2 and H2O2 Under Acidic Condition. Front. Phys. 8:242. doi: 10.3389/fphy.2020.00242
Received: 01 April 2020; Accepted: 03 June 2020;
Published: 30 July 2020.
Edited by:
Mounir Laroussi, Old Dominion University, United StatesReviewed by:
Jayr Amorim, Aeronautics Institute of Technology (ITA), BrazilEugen Stamate, Technical University of Denmark, Denmark
Copyright © 2020 Liu, Liu and Ran. This is an open-access article distributed under the terms of the Creative Commons Attribution License (CC BY). The use, distribution or reproduction in other forums is permitted, provided the original author(s) and the copyright owner(s) are credited and that the original publication in this journal is cited, in accordance with accepted academic practice. No use, distribution or reproduction is permitted which does not comply with these terms.
*Correspondence: Kun Liu, bGl1a3VuJiN4MDAwNDA7Y3F1LmVkdS5jbg==