- 1National Key Laboratory of Photoelectric Technology and Functional Materials (Culture Base), Institute of Photonics and Photon-Technology, Northwest University, Xi'an, China
- 2Shaanxi Engineering Technology Research Center for Solid State Lasers and Application, Xi'an, China
- 3Provincial Key Laboratory of Photo-Electronic Technology, Institute of Photonics and Photon-Technology, Northwest University, Xi'an, China
- 4Key Laboratory of Time and Frequency Primary Standards, National Time Service Center, Chinese Academy of Sciences, Xi'an, China
This study details the implementation of a wavelength-tunable ultra-stable optical frequency comb (OFC), which is generated by a passively mode-locked all-polarization-maintaining Erbium (Er)-doped fiber laser based on a non-linear amplifying loop mirror. The center wavelength can be tuned from 950 to 1,080 nm and from 1,650 to 2,080 nm by adjusting the pump's power. Standard deviations of 100 μHz (τ = 1 s) for repetition rate and 330 μHz (τ = 1 s) for carrier-envelop offset frequency were measured in 15 h. The corresponding Allan deviations were 2.4 × 10−17 and 8.2 × 10−17 at 1 s. The repetition rate and carrier-envelop offset frequency of OFC were counted by a ∏-type counter from K+K Messtechnik.
Introduction
Optical frequency combs (OFCs) have garnered interest due to their versatility in applications, such as ultra-low noise microwave generation [1–3], high resolution spectroscopy [4, 5], astronomical spectrograph [6, 7], time and frequency transfer and optical clocks [8–10]. For the aforementioned applications, the frequency instability of OFCs is the most important parameters. Beyond that, many other characteristics of OFCs, such as robustness, turn-key operation ability, high power output, high repetition rate and wide wavelength range, must be considerate.
OFCs are dependent on the repetition rate and carrier-envelop offset frequency of femtosecond pulses in microwave clocks. The optical frequency of a comb tooth given by νn = fceo + nfrep, where fceo is the carrier-envelope offset frequency, frep is the repetition rate, and n is an integer. Typically, fceo and frep refer to radiofrequency (RF). To achieve superior short-term stability systems, OFCs require narrow linewidth lasers phase-locked to a high fineness optical cavity as reference. OFCs may obtain short-term stability from sub-hertz linewidth lasers. Consequently, narrow-linewidth lasers are crucial to superior short-term stability OFCs. There have been significant performance improvements in narrow linewidth lasers in recent years. Cole et al. presented a new optical coating technology based on direct-bonded monocrystalline multilayers to improve mechanical loss and optical quality [11]. Kessler et al. constructed a silicon single-crystal optical cavity, and obtained a sub-40 mHz linewidth laser at 1.5 μm [12]. Matei et al. reported a narrow linewidth laser, stabilized with silicon Fabry-Perot cavity, operated at linewidth as small as 5 mHz at 194 THz [13]. Zhang et al. reported a laser locked to a silicon cavity operating at 4 K with a median linewidth of 17 mHz [14].
The measurements of optical atomic clock transitions often require the comparison of different species of atomic clocks [15, 16]. To operate several kinds of clocks with the same OFC, multi-wavelength systems are required. OFCs based on Erbium (Er)-doped fiber lasers have been proven capable of long-term operation. However, the wavelength-tunable ultra-stable fiber-based combs operating beyond dozens of nanometers have not been proven.
OFCs based on Er-doped fiber lasers are better choice because they are turnkey, robust, and support compact design. Er fiber-based OFCs based on non-linear amplifying loop mirror (NALM) [17–19], semiconductor saturable absorber mirror (SESAM) [20–23], and non-linear polarization rotation (NPR) [24–26] have been proven to be efficient alternatives. However, NPR with a single-mode (SM) fiber design is undesirable because its mode-locking state is easily disrupted by environmental perturbations. All of polarization-maintaining (PM) design is a solution to this problem. Sinclair el al. employed SESAM and NALM-based designs, using PM fibers, to demonstrate a self-referenced OFC based on mode locked Er-doped fiber laser that can operate outside the metrology lab [23]. Feng built a SESAM-based PM fiber OFC, which provided better environmental stability [22]. Kuse et al. reported a fully stabilized all-PM Er fiber-based OFC with a NALM optimized with an additional phase bias [18]. Compared with OFCs based on SESAM mode-locked fiber lasers, OFCs based on NALM have lower intrinsic phase noise and timing jitter. Therefore, tunable low-noise OFC with NALM represents a promising means to realizing both superior short-term stability and PM fiber configuration.
This study demonstrates a wavelength-tunable ultra-stable OFC based on PM Er-doped fiber laser, which is phase-locked to a 1,550-nm sub-hertz linewidth fiber laser. The widely tunable range is due to instantaneous Kerr non-linearity effect of highly non-linear fiber pumped with an Er-doped comb. The center wavelength could be tuned from 950 to 1,080 nm and from 1,650 to 2,080 nm by adjusting the input power of the pump comb. The 1550-nm narrow linewidth fiber laser, with a most probable linewidth of 185 mHz and a frequency instability of 7 × 10−17 at 1–10 s averaging time, was realized by frequency-stabilization with high fineness Fabry-Perot cavity using a standard Pound–Drever–Hall technique [27]. The femtosecond fiber laser oscillator was a 200 MHz Er-doped mode-locked PM fiber laser, based on the NALM mechanism. A stage fiber amplifier, pulse compressor and octave-spanning spectrum in highly non-linear fiber (HNLF) were used in obtaining the fceo signal. Fully phase stabilization of the comb was obtained by locking frep and fceo via standard phase locked loop technique. A long-term stability of 100 μHz (τ = 1 s) for frep, and 330 μHz (τ = 1 s) for fceo were measured in 15 h, and the corresponding Allan deviations were 2.4 × 10−17 and 8.2 × 10−17 at 1 s gate-time.
Experimental Results and Discussion
The complete fiber system shown in Figure 1 consists of an all-PM Er fiber oscillator (IMRA GmbH) with phase biased NALM as an artificial saturable absorber. This oscillator is set to provide pulses of ~1 ps at a 200 MHz repetition rate, and an average power of 1.5 mW. The output spectra, with a full-width-half-maximum (FWHM), is about 50 nm from the laser cavity.
Referring to Figure 1, the black lines represent PM fiber, red lines represent free space ray, and the blue lines represent electrical signal path. OFCs consist of are an electro-optic modulator (EOM), a piezo actuator (PZT), an optical isolator (ISO), a wavelength-division multiplexer (WDM), a laser diode (LD), an erbium doped fiber (EDF), a highly non-linear fiber (HNLF), a periodically-poled lithium niobate crystal (PPLN), a polarizer (Pol.), two photo detectors (APD), and a 1,550-nm sub-hertz linewidth continuous wave laser.
To achieve an octave-spanning spectrum, part of the Er-doped fiber oscillator (~1.5 mW) was launched into a short pulse Er-doped fiber amplifier (EDFA). The EDFA consisted of 1.5 m of normal dispersion PM Er-doped fiber. EDFA was bidirectionally pumped by 976-nm laser diodes at maximum output power of 750 mW. After amplification, the pulses were compressed using PM1550 fiber, which is anomalously dispersion at the laser wavelength. The compressed pulses, measured by an intensity auto-correlator (Femtochrome, FR-103XL), showed a pulse width of 60 fs (an average output power of ~285 mW). The 285-mW pulses were then coupled into 30 cm length of HNLF. After non-linear spectral broadening in the HNLF, the optical spectrum spanned more than one octave, from 980 to 2,000 nm, as seen in Figure 2A. The standard collinear self-referencing approach was used for detecting the fceo by doubling the ~2,000 nm portion of the light and heterodyning it against the ~1,000 nm end of the comb to generate an RF beat signal. The periodically poled lithium niobate (PPLN) crystal was utilized for doubling light from 2,000 to 1,000 nm. The octave-spanning light and the fundamental light were both filtered at 1,000 nm by an optical band-pass filter with 10 nm bandwidth. An amplified photodetector was then used to obtain fceo signal. The signal to noise ratio (SNR) of fceo at a resolution bandwidth (RBW) of 300 kHz was more than 30 dB, as shown in Figure 2B. The fceo signal was then sent to a homemade phase-locked loop to stabilize at an RF reference. This was achieved by controlling a fast electro-optic modulator (EOM) in the laser cavity [28]. To transfer the short-term stability of narrow-linewidth laser to OFC, the OFC was phase-locked to a cavity-stabilized sub-hertz linewidth fiber laser. The 1,550-nm narrow linewidth fiber laser (with a most probable linewidth of 185 mHz and frequency instability of 7.0 × 10−17 at 1–10 s averaging time) was realized by frequency-stabilization to high-finesse Fabry-Perot cavity using the Pound-Drever-Hall technique. More information on 1,550-nm sub-hertz linewidth fiber laser can be found in [27].
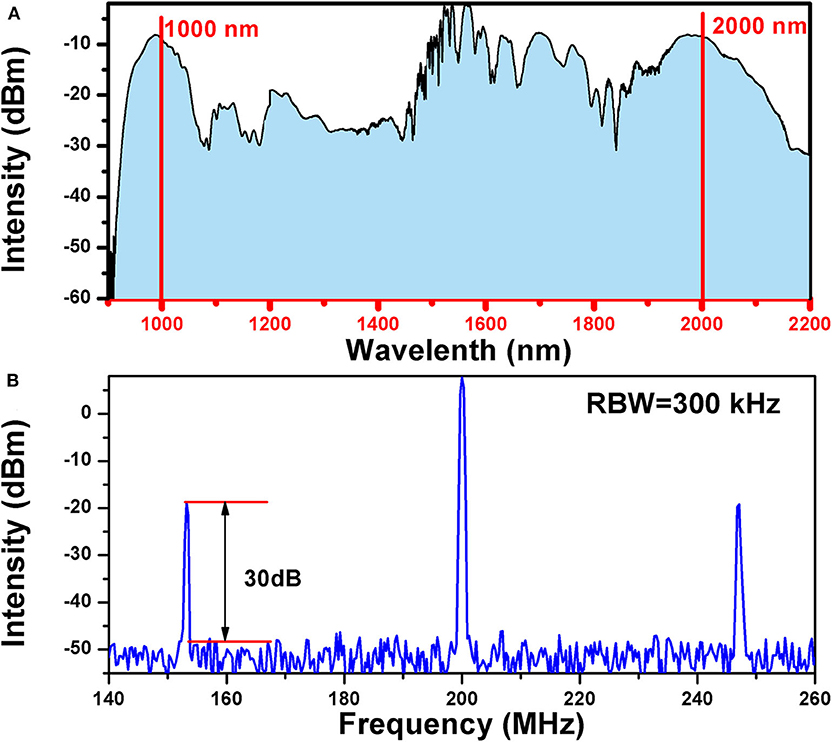
Figure 2. (A) Octave-spanning super-continuum spectrum after the HNLF. (B) fceo heterodyne signal at a 300 kHz resolution bandwidth (RBW).
To make the phase-locked loop robust, a 40 × frequency divider was employed. The long-term stability of fceo frequency was also demonstrated. Figure 3A shows the frequency fluctuation of fceo recorded over more than 15 h by the frequency counter at 1 s gate-time (FXQE80, K+K Messtechnik). The standard deviation of fceo was estimated to be about 330 μHz and the frequency fluctuation about 2 mHz. In-loop relative frequency instability of fceo (normalized with the optical frequency of ~195 THz) was about 8.2 × 10−17 at 1 s, as shown in Figure 3B. The value is below those of the best optical clocks.
The repetition rate of OFC can be stabilized to an RF or optical standard. In order to transfer the stability of the optical reference to the comb and reduce the noise on the comb lines, the fiber oscillator was stabilized relative to a 1,550 nm, sub-hertz linewidth continuous wave laser. Two servo actuators were used to increase the bandwidth of the beat lock; one controlling a slow PZT, and the other a fast EOM inside the fiber oscillator. The PZT had a low bandwidth for removing low-frequency noise and the EOM had a high bandwidth for removing high-frequency noise. Figure 4A shows the frequency fluctuation of the beat signal, which was counted with a commercial counter in 1 s gate-time. The beat frequency offset from a 25-MHz reference was recorded over 15 h with an RF frequency counter. The standard deviation of the beat signal was calculated to be 100 μHz. As seen in Figure 4B, the corresponding Allan deviation of the in-loop optical beat note was 2.4 × 10−17 at 1 s, and scales down with a slope of 1/τ, reaching around 1 × 10−20 at 1 h.
To generate the maximum output power at desired wavelength, a short section of HNLF with a larger non-linear coefficient (24 W−1·km−1), and shorter zero dispersion wavelength (1,350 nm) were employed. The compact Er fiber amplifier provided a maximum average optical power of 350 mW, with pulse width as short as 60 fs, and a repetition rate of 200 MHz. The output power of the amplifier was varied from 145 to 350 mW in order to change the wavelength. After femtosecond pulse propagating a 10-cm HNLF, the spectrum with blue shifter part and red shifted part were shown in Figure 5. This was primarily due to the instantaneous Kerr non-linearity with an intense femtosecond pump. The HNLF output contains a blue-shifted dispersive wave from 950 to 1,080 nm and a red-shifted soliton ranging from 1,650 to 2,080 nm for different average powers in the pump beam.
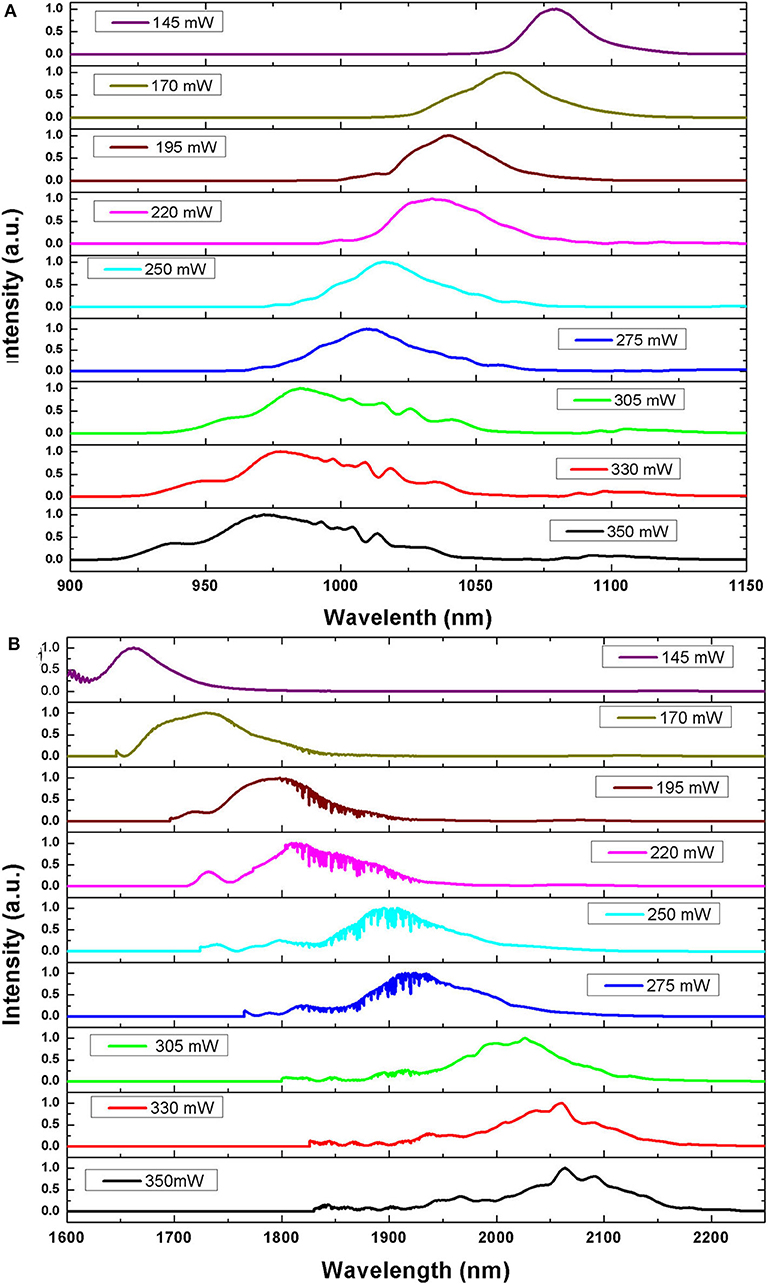
Figure 5. Typical tunable OFC spectrum at different pump power. (A) Short wavelength part; (B) Long wavelength part.
Conclusions
In conclusion, a wavelength tunable ultra-stable OFC based on all-polarization-maintaining Er-doped fiber laser for laser frequency stabilization and measurement, was developed. The fiber-based frequency comb with a wavelength-tunable configuration can transfer both linewidth and short-term stability. The widely tunable range is attributed to the instantaneous Kerr non-linearity effect of highly non-linear fiber pumped with an Er-doped comb. The center wavelength could be tuned from 950 to 1,080 nm and from 1,650 to 2,080 nm by adjusting the pump's input power. A full phase stabilization of the comb was obtained by locking frep and fceo via standard phase-locked loop technique. A long-term stability of 100 μHz (τ = 1 s) for frep, and 330 μHz (τ = 1 s) for fceo were measured in 15 h, and the corresponding Allan deviations were 2.4 × 10−17 and 8.2 × 10−17 at 1 s. Tunable ultra-stable combs are applicable in optical atomic clocks, comparison of different atomic clock species, measuring the frequency of quantum transitions, low-phase noise frequency synthesis, among others.
Data Availability Statement
All datasets generated for this study are included in the article/supplementary material.
Author Contributions
LH and KW conceived the study. XH and PZ are responsible for experiment setting and paper writing. YZ, QL, and HG are mainly engaged in picture editing and related data processing. All authors have made positive contributions to the work.
Funding
This work is supported by National Natural Science Foundation of China (No. 11804276), Natural Science Foundation of Shaanxi, China (Nos. 2019JQ-524, 2020JQ-434) and Scientific Research Program Funded by Shaanxi Provincial Education Department (No. 17JS122).
Conflict of Interest
The authors declare that the research was conducted in the absence of any commercial or financial relationships that could be construed as a potential conflict of interest.
References
1. Fortier TM, Kirchner MS, Quinlan F, Taylor J, Bergquist JC, Rosenband T, et al. Generation of ultrastable microwaves via optical frequency division. Nature Photonics. (2011) 5:425. doi: 10.1038/nphoton.2011.121
2. Millo J, Abgrall M, Lours M, English EML, Jiang H, Guéna J, et al. Ultralow noise microwave generation with fiber-based optical frequency comb and application to atomic fountain clock. App. Phys. Lett. (2009) 94:141105. doi: 10.1063/1.3112574
3. Portuondo-Campa E, Buchs G, Kundermann S, Balet L, Lecomte S. Ultra-low phase-noise microwave generation using a diode-pumped solid-state laser based frequency comb and a polarization-maintaining pulse interleaver. Opt Express. (2015) 23:32441–51. doi: 10.1364/OE.23.032441
4. Diddams SA. The evolving optical frequency comb [Invited]. J Opt Soc Am B. (2010) 27:B51–62. doi: 10.1364/JOSAB.27.000B51
5. Newbury NR. Searching for applications with a fine-tooth comb. Nat Photon. (2011) 5:186. doi: 10.1038/nphoton.2011.38
6. Li C-H, Benedick AJ, Fendel P, Glenday AG, Kärtner FX, Phillips DF, et al. A laser frequency comb that enables radial velocity measurements with a precision of 1 cm s-1. Nature. (2008) 452:610. doi: 10.1038/nature06854
7. Steinmetz T, Wilken T, Araujo-Hauck C, Holzwarth R, Hänsch TW, Pasquini L, et al. Laser Frequency combs for astronomical observations. Science. (2008) 321:1335–7. doi: 10.1126/science.1161030
8. Diddams SA, Udem T, Bergquist JC, Curtis EA, Drullinger RE, Hollberg L, et al. An optical clock based on a single trapped 199Hg+ Ion. Science. (2001) 293:825–8. doi: 10.1126/science.1061171
9. Jiang YY, Ludlow AD, Lemke ND, Fox RW, Sherman JA, Ma LS, et al. Making optical atomic clocks more stable with 10–16-level laser stabilization. Nat Photon. (2011) 5:158. doi: 10.1038/nphoton.2010.313
10. Ludlow AD, Boyd MM, Ye J, Peik E, Schmidt PO. Optical atomic clocks. Rev Modern Phys. (2015) 87:637–701. doi: 10.1103/RevModPhys.87.637
11. Cole GD, Zhang W, Martin MJ, Ye J, Aspelmeyer M. Tenfold reduction of Brownian noise in high-reflectivity optical coatings. Nat Photon. (2013) 7:644. doi: 10.1038/nphoton.2013.174
12. Kessler T, Hagemann C, Grebing C, Legero T, Sterr U, Riehle F, et al. A sub-40-mHz-linewidth laser based on a silicon single-crystal optical cavity. Nat Photon. (2012) 6:687. doi: 10.1038/nphoton.2012.217
13. Matei DG, Legero T, Häfner S, Grebing C, Weyrich R, Zhang W, et al. 1.5 µ m Lasers with Sub-10 mHz Linewidth. Phys Rev Lett. (2017) 118:263202. doi: 10.1103/PhysRevLett.118.263202
14. Zhang W, Robinson JM, Sonderhouse L, Oelker E, Benko C, Hall JL, et al. Ultrastable silicon cavity in a continuously operating closed-cycle cryostat at 4 K. Phys Rev Lett. (2017) 119:243601. doi: 10.1103/PhysRevLett.119.243601
15. Nakajima Y, Inaba H, Hosaka K, Minoshima K, Onae A, Yasuda M, et al. A multi-branch, fiber-based frequency comb with millihertz-level relative linewidths using an intra-cavity electro-optic modulator. Opt Express. (2010) 18:1667–76. doi: 10.1364/OE.18.001667
16. Hisai Y, Akamatsu D, Kobayashi T, Okubo S, Inaba H, Hosaka K, et al. Development of 8-branch Er:fiber frequency comb for Sr and Yb optical lattice clocks. Opt Express. (2019) 27:6404–14. doi: 10.1364/OE.27.006404
17. Hänsel W, Hoogland H, Giunta M, Schmid S, Steinmetz T, Doubek R, et al. All polarization-maintaining fiber laser architecture for robust femtosecond pulse generation. Appl Phys B. (2017) 123:41. doi: 10.1007/s00340-016-6598-2
18. Kuse N, Jiang J, Lee C-C, Schibli T, Fermann M. All polarization-maintaining Er fiber-based optical frequency combs with nonlinear amplifying loop mirror. Opt Express. (2016) 24:3095–102. doi: 10.1364/OE.24.00309
19. Li Y, Kuse N, Rolland A, Stepanenko Y, Radzewicz C, Fermann ME. Low noise, self-referenced all polarization maintaining Ytterbium fiber laser frequency comb. Opt Express. (2017) 25:18017-23. doi: 10.1364/OE.25.018017
20. Kuse N, Lee CC, Jiang J, Mohr C, Schibli TR, Fermann ME. Ultra-low noise all polarization-maintaining Er fiber-based optical frequency combs facilitated with a graphene modulator. Opt Express. (2015) 23:24342–50. doi: 10.1364/OE.23.024342
21. Fehrenbacher D, Sulzer P, Liehl A, Kälberer T, Riek C, Seletskiy DV, et al. Free-running performance and full control of a passively phase-stable Er:fiber frequency comb. Optica. (2015) 2:917–23. doi: 10.1364/OPTICA.2.000917
22. Feng Y, Xu X, Hu X, Liu Y, Wang Y, Zhang W, et al. Environmental-adaptability analysis of an all polarization-maintaining fiber-based optical frequency comb. Opt Express. (2015) 23:17549–59. doi: 10.1364/OE.23.017549
23. Sinclair LC, Coddington I, Swann WC, Rieker GB, Hati A, Iwakuni K, et al. Operation of an optically coherent frequency comb outside the metrology lab. Opt Express. (2014) 22:6996–7006. doi: 10.1364/OE.22.006996
24. Pang L, Han H, Zhao Z, Liu W, Wei Z. Ultra-stability Yb-doped fiber optical frequency comb with 2 × 10−18/s stability in-loop. Opt Express. (2016) 24:28993–9000. doi: 10.1364/OE.24.028993
25. Yan M, Li W, Yang K, Zhou H, Shen X, Zhou Q, et al. High-power Yb-fiber comb with feed-forward control of nonlinear-polarization-rotation mode-locking and large-mode-area fiber amplification. Opt Lett. (2012) 37:1511–3. doi: 10.1364/OL.37.001511
26. Ycas G, Osterman S, Diddams SA. Generation of a 660–2100 nm laser frequency comb based on an erbium fiber laser. Opt Lett. (2012) 37:2199–201. doi: 10.1364/OL.37.002199
27. Tai Z-Y, Zhang Y-Y, Zhang X-F, Guo W-G, Zhang S-G, Jiang H-F. Transportable 1555-nm ultra-stable laser with sub-0.185-Hz linewidth. Chin Phys Lett. (2017) 34:090602. doi: 10.1088/0256-307x/34/9/090602
Keywords: optical frequency comb, mode-locked Er-doped fiber laser, all-polarization-maintaining fiber, tunable comb, ulta-stable comb
Citation: He X, Zhang P, Zhang Y, Lin Q, Guo H, Hou L and Wang K (2020) Wavelength-Tunable Ultra-Stable Optical Frequency Comb Based on All-Polarization-Maintaining Fiber Laser. Front. Phys. 8:226. doi: 10.3389/fphy.2020.00226
Received: 01 May 2020; Accepted: 25 May 2020;
Published: 30 June 2020.
Edited by:
Xiaohui Li, Shaanxi Normal University, ChinaReviewed by:
Youjian Song, Tianjin University, ChinaWenjun Liu, Beijing University of Posts and Telecommunications (BUPT), China
Copyright © 2020 He, Zhang, Zhang, Lin, Guo, Hou and Wang. This is an open-access article distributed under the terms of the Creative Commons Attribution License (CC BY). The use, distribution or reproduction in other forums is permitted, provided the original author(s) and the copyright owner(s) are credited and that the original publication in this journal is cited, in accordance with accepted academic practice. No use, distribution or reproduction is permitted which does not comply with these terms.
*Correspondence: Lei Hou, bGhvdUBud3UuZWR1LmNu; Kaige Wang, d2FuZ2tnQG53dS5lZHUuY24=