- 1Flow Pharma Inc., Pleasant Hill, CA, United States
- 2Department of Anesthesia, Critical Care and Pain Medicine, Massachusetts General Hospital, Boston, MA, United States
- 3Department of Microbiology and Immunology, University of Texas Medical Branch, Galveston, TX, United States
- 4Department of Medicine, Columbia University, New York, NY, United States
Cytokine release syndrome (CRS) is known to be a factor in morbidity and mortality associated with acute viral infections including those caused by filoviruses and coronaviruses. IL-6 has been implicated as a cytokine negatively associated with survival after filovirus and coronavirus infection. However, IL-6 has also been shown to be an important mediator of innate immunity and important for the host response to an acute viral infection. Clinical studies are now being conducted by various researchers to evaluate the possible role of IL-6 blockers to improve outcomes in critically ill patients with CRS. Most of these studies involve the use of anti-IL-6R monoclonal antibodies (α-IL-6R mAbs). We present data showing that direct neutralization of IL-6 with an α-IL-6 mAb in a BALB/c Ebolavirus (EBOV) challenge model produced a statistically significant improvement in outcome compared with controls when administered within the first 24 h of challenge and repeated every 72 h. A similar effect was seen in mice treated with the same dose of α-IL-6R mAb when the treatment was delayed 48 h post-challenge. These data suggest that direct neutralization of IL-6, early during the course of infection, may provide additional clinical benefits to IL-6 receptor blockade alone during treatment of patients with virus-induced CRS.
Introduction
Under normal circumstances, interleukin-6 (IL-6) is secreted transiently by myeloid cells as part of the innate immune response to injury or infections. However, unregulated synthesis and secretion of IL-6 has contributed to a host of pathological effects such as rheumatoid arthritis. (Swaak et al., 1988) Furthermore, IL-6 induces differentiation of B cells and promotes CD4+ T cell survival during antigen activation and inhibits TGF-beta differentiation, providing a crucial link between innate and acquired immune responses (Korn et al., 2008; Dienz and Rincon, 2009). These actions place IL-6 in a central role in mediating and amplifying cytokine release syndrome (CRS), commonly associated with Ebola virus disease (EVD) infections. (Wauquier et al., 2010). CRS is known to be a factor in morbidity and mortality associated with acute viral infections including those caused by filoviruses and coronaviruses. For example, non-survivors of the West African EBOV epidemics exhibited significantly elevated levels of the overall inflammatory response cytokines and monokines compared to survivors (Ruibal et al., 2016). It is thought that prolonged exposure to elevated inflammatory cytokine levels is toxic to T cells and results in their apoptotic and necrotic cell death (Younan et al., 2018). Both lymphopenia and elevated serum Il-6 levels are found in Ebola virus infection and are known to be inversely correlated with survival in patients post-infection (Wauquier et al., 2010)and in mouse models of Ebola infection (Herst et al., 2020). However, IL-6 has also been shown to be an important mediator of innate immunity and important for the host recovery from acute viral infection (Yang et al., 2017). Elevated IL-6 levels are also observed in SARS-CoV-2 infections, severe influenza, rhinovirus, RSV infection, as well as in similar respiratory infections (Hayden et al., 1998; Tang et al., 2016; Kerrin et al., 2017; Conti et al., 2020). Originally developed for the treatment of arthritis, α-IL-6R mAbs have been used to treat CRS as a complication of cancer therapy using adaptive T-cell therapies. (Lee et al., 2014; Tanaka et al., 2016; Ascierto et al., 2020). Warnings admonishing the use of IL-6 blockers in the context of acute infection are present in the package inserts for tocilizumab (Genentech, 2014), sarilumab (Sanofi, 2017) and siltuximab (EUSA, 2015). Early mixed results of CRS treatment with IL-6 blockers (Herper, 2020; ClinicalTrialsGenetech, 2020; ClinicalTrialsEUSA, 2020; Taylor, 2020; Saha et al., 2020), and our own observations of the role of IL-6 in morbidity and mortality associated with Ebola virus infection (Herst et al., 2020), led us to evaluate the clinical effects of treatment with not only antibody directed against the IL-6 receptor, but also with mAb directed to IL-6 itself. We report here on the observed differences between treatments with α-IL-6R mAbs and α-IL-6 mAbs in a mouse model of EBOV infection and comment on how IL-6 blockade may be relevant to the management and therapy for patients with Ebola infection as well as patients infected with SARS-CoV-2.
Methods
Virus Strain
For in-vivo experiments, a well-characterized mouse-adapted Ebola virus (maEBOV) stock (Bray et al., 1998; Lane et al., 2019) (Ebola virus M. musculus/COD/1976/Mayinga-CDC-808012), derived from the 1976 Zaire ebolavirus isolate Yambuku-Mayinga (Genebank accession NC002549), was used for all studies. All work involving infectious maEBOV was performed in a biosafety level (BSL) 4 laboratory, registered with the Centers for Disease Control and the Prevention Select Agent Program for the possession and use of biological select agents.
Animal Studies
Animal studies were conducted at the University of Texas Medical Branch (UTMB), Galveston, TX in compliance with the Animal Welfare Act and other federal statutes and regulations relating to animal research. UTMB is fully accredited by the Association for the Assessment and Accreditation of Laboratory Animal Care International and has an approved OLAW Assurance. BALB/c mice (Envigo; n = 146) were challenged with 100 plaque forming units (PFU) of maEBOV via intraperitoneal (i.p.) injection as described previously (Hodge et al., 2016; Comer et al., 2019). Experimental groups of 10 mice each were administered rat anti-mouse-IL-6 IgG1 monoclonal antibody (BioXCell, BE0046, Lebanon, NH, RRID AB1107709) or rat anti-mouse-IL-6R IgG2 monoclonal antibody (BioXCell, BE0047, RRID AB1107588) at a dose of 100 μg in sterile saline via intravenous (i.v.) administration via an indwelling central venous catheter, or 400 μg via i.p. injection at 24, 48, or 72 h post-challenge. Antibody dosing was based on amounts previously reported to neutralize IL-6 and IL-6R in mice (Barber et al., 2014; Liang et al., 2015). Antibody dosing was performed once for the i.v. group or continued at 72-h intervals for the i.p. groups resulting in a total of four doses over the 14-day study period as summarized in Figure 1 and Tables S2–S5 (Supplemental Materials). Control mice (n=36) were challenge with maEBOV in parallel, but were treated with antibody vehicle alone. Serum IL-6 measurements were performed in control rodents at necropsy as previously described (Herst et al., 2020).
In Vivo Clinical Observations and Scoring
Following maEBOV challenge, mice were examined daily and scored for alterations in clinical appearance and health as previously described(Lane et al., 2019). Briefly, mice were assigned a score of 1 = Healthy; score 2 = Lethargic and/or ruffled fur (triggers a second observation); score 3 = Ruffled fur, lethargic and hunched posture, orbital tightening (triggers a third observation); score 4 = Ruffled fur, lethargic, hunched posture, orbital tightening, reluctance to move when stimulated, paralysis or greater than 20% weight loss (requires immediate euthanasia) and no score = deceased (Table S1, Supplemental Materials).
Statistical Methods
Descriptive and comparative statistics including arithmetic means, standard errors of the mean (SEM), Survival Kaplan-Meier plots and Log-rank (Mantel-Cox) testing, D’Agostino & Pearson test for normality, Area-Under-The-Curve and Z Statistics were calculated using R with data from GraphPad Prism files. The clinical composite score data used to calculate the AUC measures were normally distributed. The significance of comparisons (p values) of AUC data was calculated using the Z statistic. p values <.05 were considered statistically significant.
Results
Following maEBOV challenge, mice were dosed i.v. at 24, 48, or 72 h post-challenge with a single dose of α-IL-6R mAb, a single i.p. dose of α-IL-6R mAb 24 h after maEBOV challenge, or an initial i.p. dose of α-IL-6 or xtalpha-IL-6R mAb, followed by additional i.p. doses at 72-h intervals for a total of four doses. Mice were observed for up to 14 days as summarized in Figure 1. The average serum IL-6 concentration at necropsy for mice (n=5) challenged with maEBOV was 1092 ± 505 pg/ml, a concentration similar to that reported in a previous publication for mice challenged with 10 PFU of maEBOV (Chan et al., 2019). In mice not challenged with maEBOV the average serum IL-6 was 31 ± 11 pg/ml. The survival and average clinical score for mice receiving a single i.v. dose of α-IL-6R mAb is shown in Figure S1A, B (Supplemental Materials). Little to no effects on survival or clinical score were observed following maEBOV challenge and a single i.v. dose of α-IL-6R mAb.
The survival patterns for i.v. mAb treated and untreated groups following maEBOV challenge were statistically different and most untreated mice succumbed to maEBOV infection by day seven (Figure S1, Supplementary Materials). Because neither survival score alone or average clinical score represented the overall possible clinical benefits of mAb treatment, a secondary composite outcome measure was calculated from the quotient of mouse survival and the average clinical score for each day, similar to that previously reported (Kaempf et al., 2019). We then summed these scores across the last 12 days of observation to create an AUC Survival/Clinical Score (see Figure S1C, Supplemental Materials). The Z statistic and significance level for this metric was calculated for each experimental condition. We found a minor clinical benefit (p < 0.01) when mice were given one 100 μg dose of α-IL-6R mAb via central venous catheter at 72 h after maEBOV challenge, relative to vehicle alone, using the experimental design described in Table S2 (Supplementary Materials).
Since the maEBOV challenge was administered intraperitoneally and murine peritoneal macrophages represent a significant depot of cells (Cassado et al., 2015) able to produce IL-6 (Vanoni et al., 2017) following toll-like receptor activation, we next compared the activities of α-IL-6 and α-IL-6R mAbs administered intraperitoneally following maEBOV challenge (Figures 2–5). We observed significant differences in the AUC Survival/Clinical Score when α-IL-6R mAb was administered 48 h post-maEBOV challenge and then repeated three times at 72-h intervals. The most significant beneficial effect on the AUC Survival/Clinical Score (Figure 5) was seen when α-IL-6 mAb was administered beginning at 24 h post-maEBOV challenge, and then repeated three times at 72-h intervals.
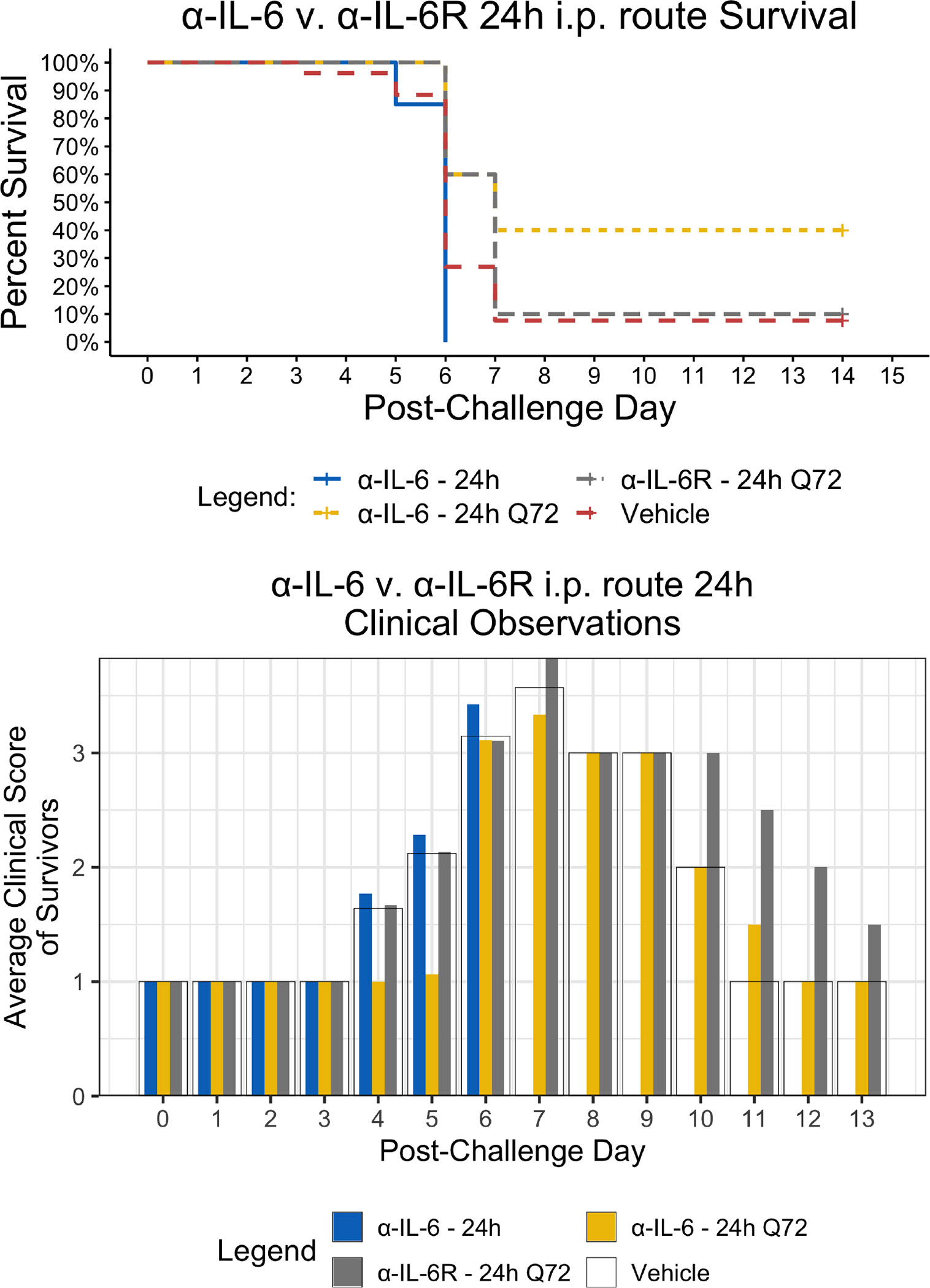
Figure 2 Kaplan-Meier Survival Plots and Average clinical scores for a single or multiple i.p. doses of α-IL-6 or α-IL-6R administered 24 h after maEBOV challenge and followed by repeat dosing every 72 h for a total of four doses. The survival curves were significantly different by Log-rank (Mantel-Cox) testing (P < 0.05). SEM of the average clinicals scores were < 10% of the mean.
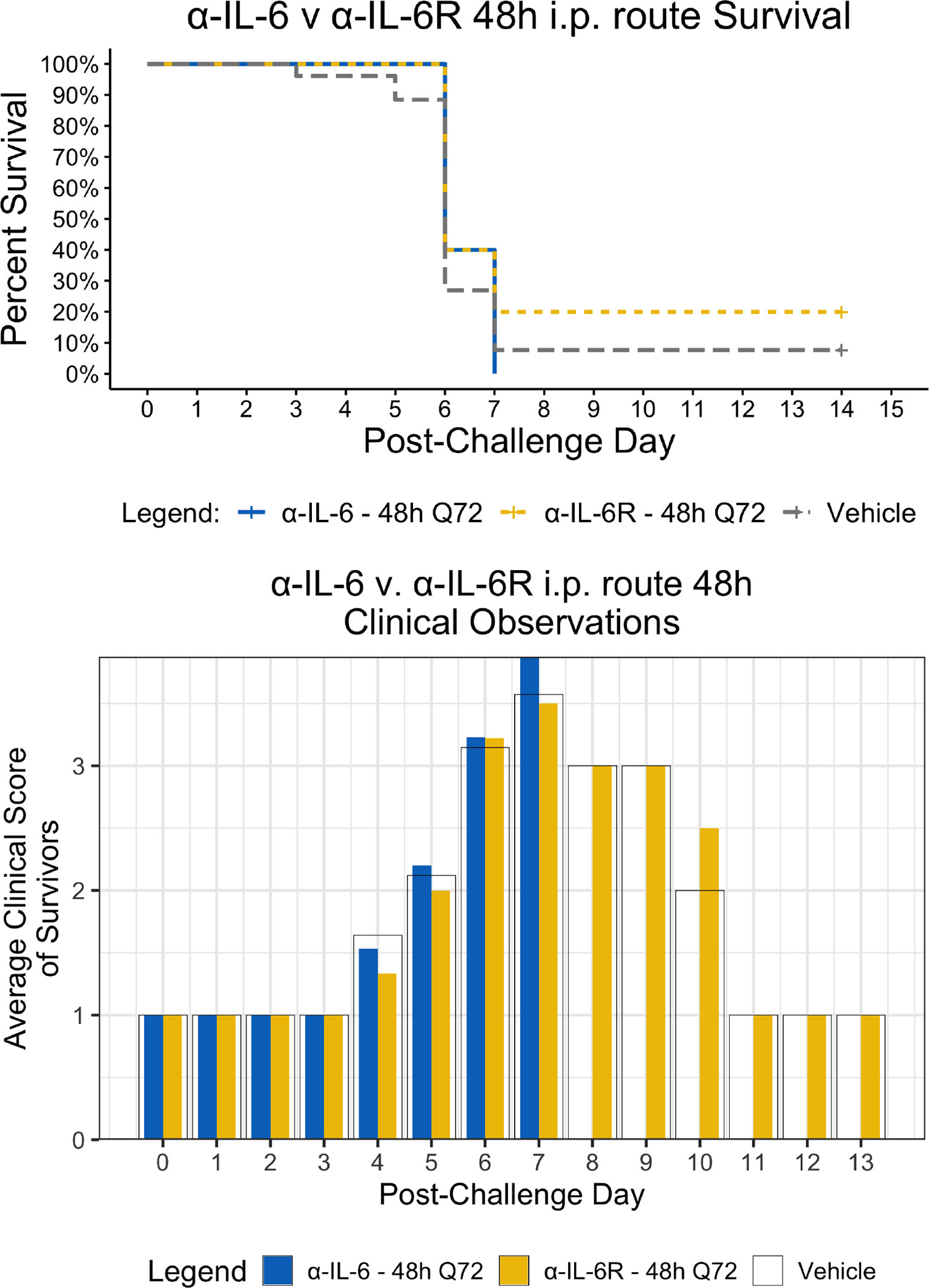
Figure 3 Kaplan-Meier Survival Plots and Average clinical scores for multiple i.p. doses of α-IL-6 or α-IL-6R administered 48 h after maEBOV challenge and followed by repeat dosing every 72 h for a total of four doses. The survival curves were significantly different by Log-rank (Mantel-Cox) testing (P < 0.05). SEM of the average clinical scores were < 10% of the mean.
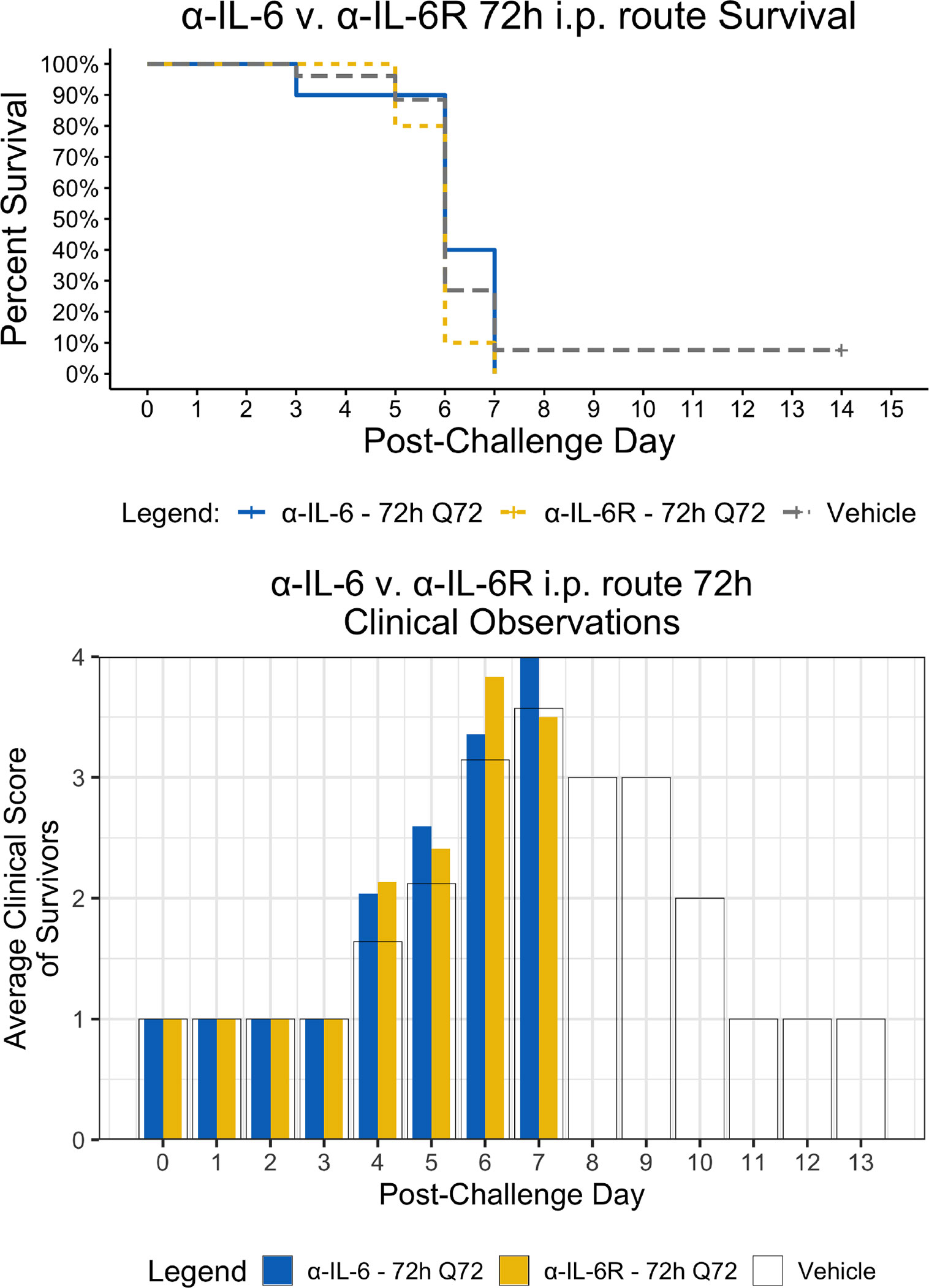
Figure 4 Kaplan-Meier Survival Plots and Average clinical scores for multiple i.p. doses of α-IL-6 or α-IL-6R administered 72 h after maEBOV challenge and followed by repeat dosing every 72 h for a total of four doses. The survival curves were significantly different by Log-rank (Mantel-Cox) testing (P < 0.05). SEM of the average clinical scores were < 10% of the mean.
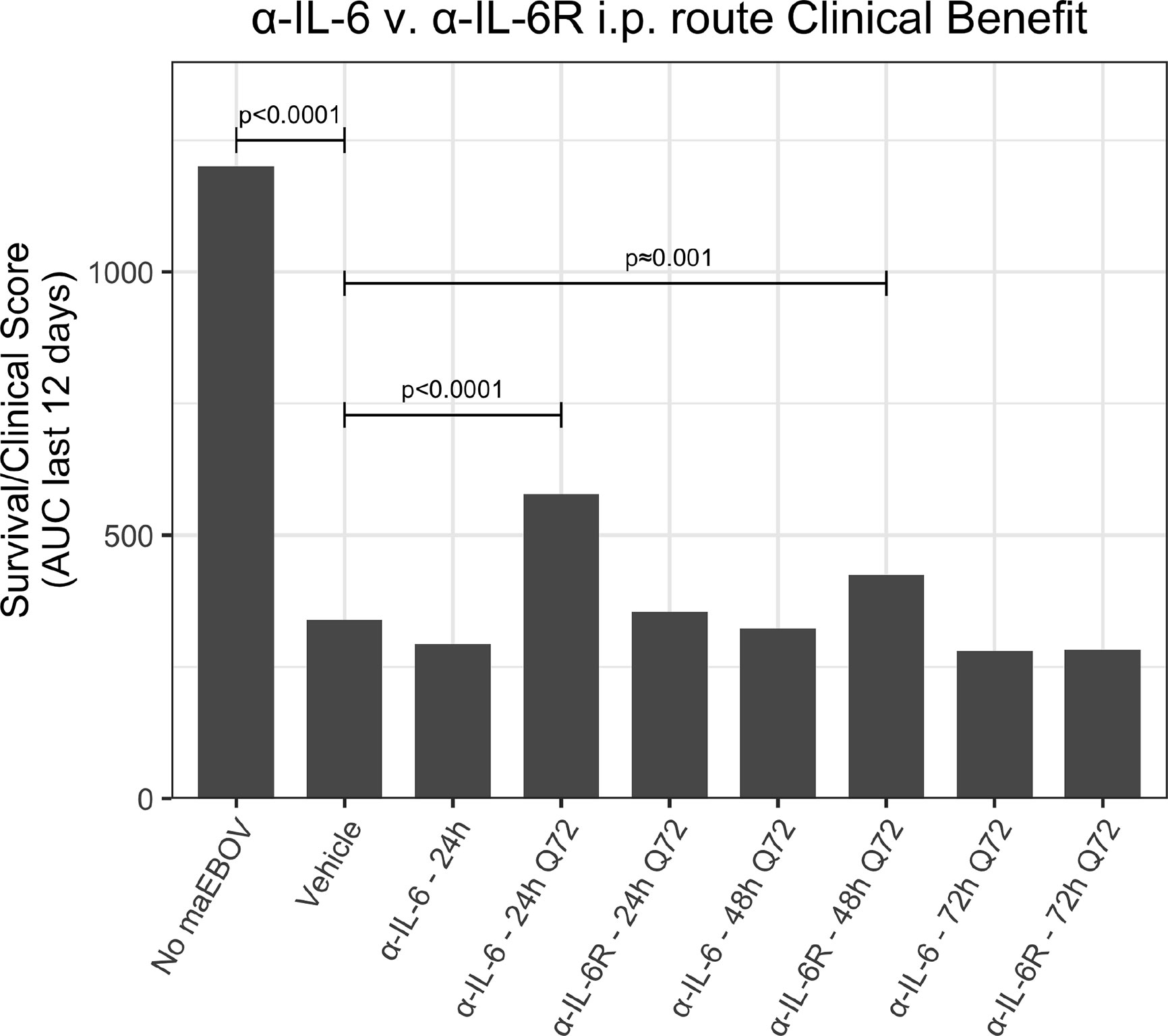
Figure 5 A clinical benefit metric was calculated as an area under curve for survival/clinical scores for 120 mice receiving a single or multiple i.p. doses of α-IL-6 or α-IL-6R mAb following maEBOV challenge on day 0. The given p values are determined from the Z statistic calculated for each experimental condition.
Discussion
While EVD is classified as a viral haemorrhagic fever, there are many similarities between EVD and COVID-19, the disease caused by infection with SARS-CoV-2 that can present as an acute respiratory distress syndrome (ARDS) (Zhou et al., 2020; Chen et al., 2020; Huang et al., 2020a; Lescure et al., 2020). Like EVD, elevated IL-6 was found to be significantly correlated with death in COVID-19 patients (Ruan et al., 2020), suggesting that patients with clinically severe SARS-CoV-2 infection might also have a CRS syndrome (Huang et al., 2020b). Both EVD and COVID-19 (Younan et al., 2019; Tan et al., 2020) are associated with lymphopenia. Since the severity of SARS-CoV-1 infection has been shown to be associated with increased serum concentrations of IL-6, clinical scientists have proposed non-corticosteroid based immunosuppression by using IL-6 blockade as a means to treat hyper inflammation observed in certain patients with SARS-CoV-2 infections (Wong et al., 2004; Mehta et al., 2020a). The potential value of using IL-6 blockade to treat COVID-19 patients was discussed early during the 2020 SARS-CoV-2 outbreak (Liu et al., 2020; Mehta et al., 2020b). Indeed, a recent (5/24/2020) search of ClinicalTrials.gov revealed at least 62 clinical trials examining the efficacy and safety of α-IL-6R mAbs and α-IL-6 mAbs for management of patients with COVID-19; 45 studies for tocilizumab (α-IL-6R mAbs), 14 for sarilumab (α-IL-6R mAbs) and 3 for siltuximab (α-IL-6 mAbs). Most of the studies involve the use of α-IL-6R mAbs and have shown promising results (summarized in Tables 1, 2), but there is clear need for improvement.
Using a mouse model of Ebola infection, we found clinical benefit when mice were administered multiple i.p. doses of α-IL-6R mAb 48 h after maEBOV challenge. At both earlier (24 h) and later (72 h) time points of initiation of administration of α-IL-6R mAb, we observed little to no effects on the clinical benefit score. Similarly, we found clinical benefit when α-IL-6 mAb was administered beginning at 24 h post-maEBOV challenge, and then repeated three times at 72-h intervals, but no benefit was observed if α-IL-6 mAb was initiated at 48 or 72 h post challenge. These data suggest that α-IL-6 mAb therapy may also have clinical benefits similar to α-IL-6R mAb particularly when given early during the course of maEBOV infection.
Previous experiments in the murine EBOV system (Herst et al., 2020) suggest that some degree of activation of innate immunity and IL 6 release benefits survival post maEBOV challenge. It may be the case that the observed clinical benefits of α-IL-6 mAbs are associated with incomplete blockade of the Il-6 response particularly later than 24 post challenge. Overall our data suggest that human clinical trials evaluating the benefits of α-IL-6 mAbs versus α-IL-6R mAbs titversus combined early α-IL-6 mAb and later α-IL-6R mAb is warranted to evaluate the potential of IL-6 pathway blockade in the during Ebola or SARS-CoV-2 infection.
Although antibody blood levels were not obtained during the mouse studies described here, we present a pharmacokinetic model based on literature values (Medesan et al., 1998; EUSA, 2015; Sanofi, 2017) shown in Table S5 in Supplemental Materials. Simulated PK curves for each of the three experiments described is shown in Figure 6. Dosing α-IL-6 mAb at 24 h after challenge produced a clinical benefit, whereas dosing α-IL-6R beginning at the same time point did not. The shorter terminal half-life of α-IL-6 mAb (T1/2 = 57h) versus α-IL-6R mAb (T1/2 = 223h), possibly due to isotype specific differences in glycosylation (Cobb, 2019) may help explain why giving α-IL-6 mAb early after infection provided the most observed clinical benefit. As can be seen from the simulated PK profile in Figure 6C, repeated dosing every 72 h, beginning 24 h after challenge, is predicted to maintain blood levels peaking at about 200 μg/ml. This is in contrast to blood levels predicted after similar dosing of α-IL-6R where the blood levels continue to increase over the study period. These differences seen in the simulated PK profiles may have allowed α-IL-6 mAb to partially block IL-6, allowing innate immunity to develop, while still providing sufficient blockade to reduce the deleterious clinical effects of IL-6 as the study progressed. In addition, it may be that the stoichiometry of α-IL-6 blockade versus α-IL-6R may favor achieving partial blockade early during the evolution of CRS given that the amount of IL-6 present may exceed the number of IL-6 receptors. It is also possible that IL-6 may act on other sites not blocked by α-IL-6R mAb, and that this may yield a potential advantage of using α-IL-6 mAb to treat CRS brought about by a viral infection.
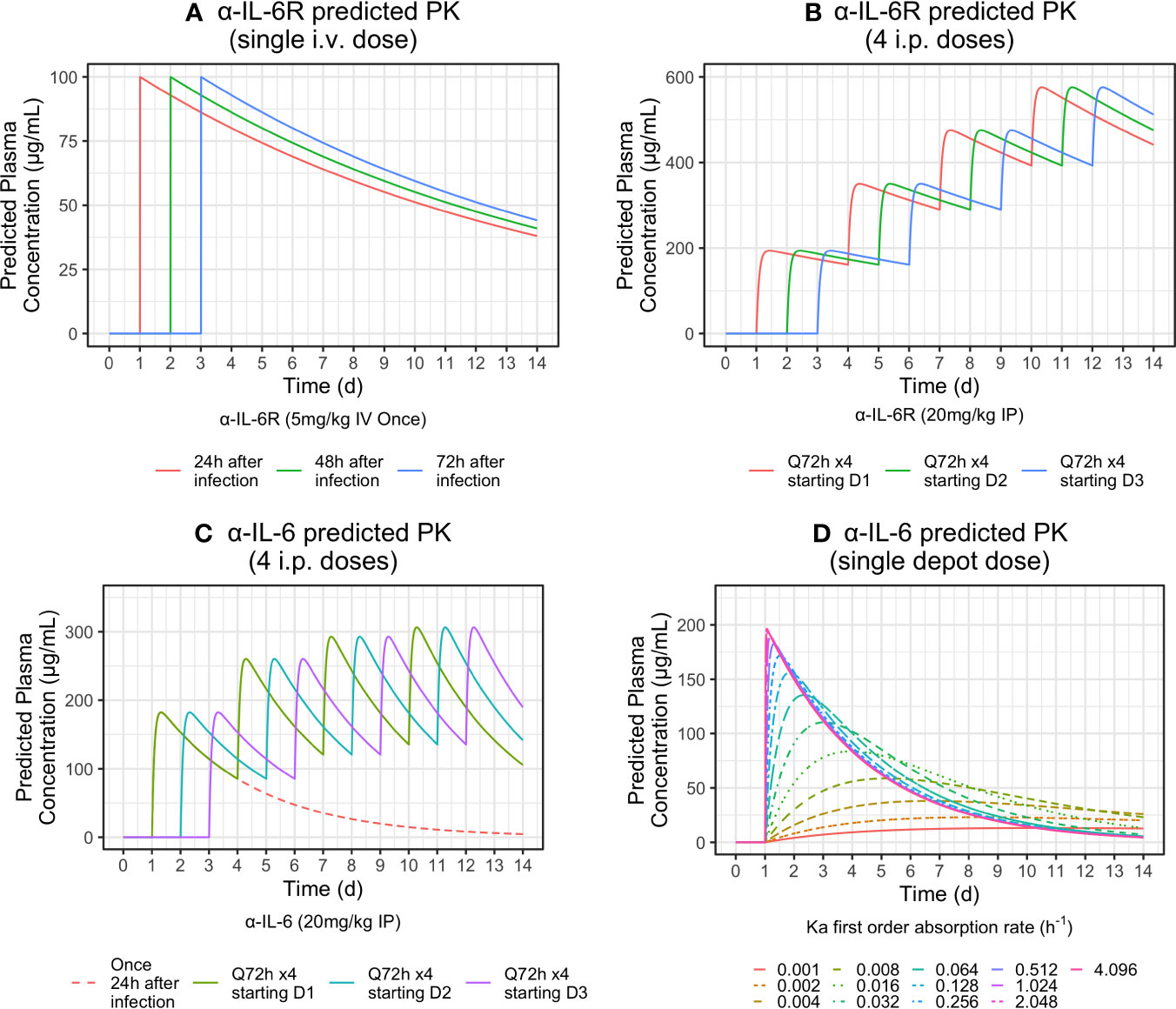
Figure 6 Simulated PK profiles for i.v. and i.p. routes of administration based on literature PK parameters shown in Table S5 in Supplementary Materials were determined. The top-left panel (A) models the i.v. delivery experiment. The top-right panel (B) and bottom-left panel (C) model i.p. delivery experiments one and two. For each of these simulations, mice were dosed a total of four times at 72-h intervals, beginning 24 h after challenge. The bottom-right panel (D) models release profiles for simulated controlled release scenarios with different absorption rates as indicated by the listed Ka parameters after a single depot injection of 20 mg/kg.
It may be possible to develop a controlled release formulation of α-IL-6 mAb to obtain a clinically beneficial effect from the administration of α-IL-6 mAb, α-IL-6R mAb, or a combination of both, after a single injection early during the course of SARS-CoV-2 infection. For example, Figure 6, bottom-right panel, shows various predicted controlled release PK profiles of α-IL-6 mAb that could be achieved by using delivery systems producing different first order rates of delivery from an injection depot of 20 mg/kg. Correlation of these release profiles with the AUC Survival/Clinical score described here in pre-clinical models could lead to the development of a single dose treatment mitigating the effects of CRS on the host.
Concluding Remarks
Although the previous reports of use of IL-6 blockers to treat CRS have shown mixed results, recent clinical data for α-IL-6 and α-IL-6R mAbs have shown early promise in human trials for treatment of severe influenza and corona virus infections (Gritti et al., 2020; Xu et al., 2020). Pre-clinical studies and various ongoing clinical trials evaluating the potential benefit of IL-6 blockers, for example, early α-IL-6 mAb and later α-IL-6R mAb, for the treatment of patients with CRS may provide clinical correlation with the results presented here.
Data Availability Statement
The raw data supporting the conclusions of this article will be made available by the authors, without undue reservation, to any qualified researcher.
Ethics Statement
The animal study was reviewed and approved by UTMB which operates under OLAW assurance number D16-00202(A3314-01).
Author Contributions
All authors contributed to the article and approved the submitted version. CM and TB performed the study under BSL-4 conditions and generated the data presented here.
Funding
This study was funded by Flow Pharma, Inc. which had no influence over the content of this manuscript or the decision to publish.
Conflict of Interest
RR, SB, RC, TH, LW, and CH are employees of Flow Pharma, Inc. compensated in cash and stock, and are named inventors on various issued and pending patents assigned to Flow Pharma. Some of these patents pending are directly related to the study presented here. PH is a member of Flow Pharma’s Scientific Advisory Board.
The remaining authors declare that the research was conducted in the absence of any commercial or financial relationships that could be construed as a potential conflict of interest.
Supplementary Material
The Supplementary Material for this article can be found online at: https://www.frontiersin.org/articles/10.3389/fphar.2020.574703/full#supplementary-material
References
Ascierto, P. A., Fox, B. A., Urba, W. J., Anderson, A. C., Atkins, M. B., Borden, E. C., et al. (2020). Insights from immuno-oncology: the Society for Immunotherapy of Cancer Statement on access to IL-6-targeting therapies for COVID-19. J. ImmunoTher. Cancer 8, e000878. doi: 10.1136/jitc-2020-000878
Barber, D. L., Andrade, B. B., Mcberry, C., Sereti, I., Sher, A. (2014). Role of IL-6 in Mycobacterium avium-associated immune reconstitution inflammatory syndrome. J. Immunol. 192, 676–682. doi: 10.4049/jimmunol.1301004
Biran, N., Ip, A., Ahn, J., Go, R. C., Wang, S., Mathura, S., et al. (2020). Tocilizumab among patients with covid-19 in the intensive care unit: a multicentre observational study. Lancet Rheumatol. 2665–9913. doi: 10.1016/S2665-9913(20)30277-0
Bray, M., Davis, K., Geisbert, T., Schmaljohn, C., Huggins, J. (1998). A Mouse Model for Evaluation of Prophylaxis and Therapy of Ebola Hemorrhagic Fever. J. Infect. Dis. 178, 651–661. doi: 10.1086/515386
Cassado, A., d., A., D’Império Lima, M. R., Bortoluci, K. R. (2015). Revisiting Mouse Peritoneal Macrophages: Heterogeneity, Development, and Function. Front. Immunol. 6:225:225. doi: 10.3389/fimmu.2015.00225
Chan, M., Leung, A., Griffin, B. D., Vendramelli, R., Tailor, N., Tierney, K., et al. (2019). Generation and Characterization of a Mouse-Adapted Makona Variant of Ebola Virus. Viruses 11, 987–995. doi: 10.3390/v11110987
Chen, N., Zhou, M., Dong, X., Qu, J., Gong, F., Han, Y., et al. (2020). Epidemiological and clinical characteristics of 99 cases of 2019 novel coronavirus pneumonia in Wuhan, China: a descriptive study. Lancet 395, 507–513. doi: 10.1016/s0140-6736(20)30211-7
ClinicalTrialsEUSA (2020). EUSA Pharma initiates study of siltuximab to treat Covid-19 patients. Clin. Trials Arena.
ClinicalTrialsGenetech (2020). Genentech’s arthritis drug tocilizumab shows promise in Covid-19 trial. Clin. Trials Arena.
Cobb, B. A. (2019). The history of IgG glycosylation and where we are now. Glycobiology 30, 202–213. doi: 10.1093/glycob/cwz065
Comer, J., Escaffre, O., Neef, N., Brasel, T., Juelich, T., Smith, J., et al. (2019). Filovirus Virulence in Interferon α/βand γDouble Knockout Mice, and Treatment with Favipiravir. Viruses 11:137. doi: 10.3390/v11020137
Conti, P., Ronconi, G., Caraffa, A., Gallenga, C., Ross, R., Frydas, I., et al. (2020). Induction of pro-inflammatory cytokines (IL-1 and IL-6) and lung inflammation by Coronavirus-19 (COVI-19 or SARS-CoV-2): anti-inflammatory strategies. J. Biol. Regul. Homeost. Agents 34 (2), 327–331. doi: 10.23812/conti-e
Della-Torre, E., Campochiaro, C., Cavalli, G., De Luca, G., Napolitano, A., La Marca, S., et al. (2020). Interleukin-6 blockade with sarilumab in severe covid-19 pneumonia with systemic hyperinflammation: an open-label cohort study. Ann. Rheum. Dis. 79 (10), 1277–1285. doi: 10.1136/annrheumdis-2020-218122
Dienz, O., Rincon, M. (2009). The effects of IL-6 on CD4 T cell responses. Clin. Immunol. 130, 27–33. doi: 10.1016/j.clim.2008.08.018
EUSA (2015). Drug Approval Package: SYLVANT (siltuximab) (Washington, DC: U.S. Food & Drug Administration).
Genentech (2014). Drug Approval Package: Actemra (tocilizumab) Solution for Subcutaneous Injection (Washington, DC: U.S. Food & Drug Administration).
Gritti, G., Raimondi, F., Ripamonti, D., Riva, I., Landi, F., Alborghetti, L., et al. (2020). Use of siltuximab in patients with COVID-19 pneumonia requiring ventilatory support. medRxiv. doi: 10.1101/2020.04.01.20048561
Guaraldi, G., Meschiari, M., Cozzi-Lepri, A., Milic, J., Tonelli, R., Menozzi, M., et al. (2020). Tocilizumab in patients with severe covid-19: a retrospective cohort study. Lancet Rheumatol. 2, e474 – e484. doi: 10.1016/S2665-9913(20)30173-9
Hayden, F., Fritz, R., Lobo, M., Alvord, W., Strober, W., Straus, S. (1998). Local and systemic cytokine responses during experimental human influenza A virus infection. Relation to symptom formation and host defense. J. Clin. Invest. 101, 643–649. doi: 10.1172/JCI1355
Herper, M. (2020). Closely watched arthritis drug disappoints as a Covid-19 treatment, studies show. STAT.
Herst, C., Burkholz, S., Sidney, J., Sette, A., Harris, P., Massey, S., et al. (2020). An effective CTL peptide vaccine for Ebola Zaire Based on Survivors’ CD8+ targeting of a particular nucleocapsid protein epitope with potential implications for COVID-19 vaccine design. Vaccine. 38 (28), 4464–4475. doi: 10.1016/j.vaccine.2020.04.034
Hodge, T., Draper, K., Brasel, T., Freiberg, A., Squiquera, L., Sidransky, D., et al. (2016). Antiviral effect of ranpirnase against Ebola virus. Antiviral Res. 132, 210–218. doi: 10.1016/j.antiviral.2016.06.009
Huang, C., Wang, Y., Li, X., Ren, L., Zhao, J., Hu, Y., et al. (2020a). Clinical features of patients infected with 2019 novel coronavirus in Wuhan, China. Lancet 395, 497–506. doi: 10.1016/s0140-6736(20)30183-5
Huang, C., Wang, Y., Li, X., Ren, L., Zhao, J., Hu, Y., et al. (2020b). Clinical features of patients infected with 2019 novel coronavirus in Wuhan, China. Lancet 395, 497–506. doi: 10.1016/S0140-6736(20)30183-5
Kaempf, J. W., Wang, L., Dunn, M. (2019). Using a composite morbidity score and cultural survey to explore characteristics of high proficiency neonatal intensive care units. Arch. Dis. Childhood - Fetal Neonatal Ed. 104, F13–F17. doi: 10.1136/archdischild-2017-313715
Kerrin, A., Fitch, P., Errington, C., Kerr, D., Waxman, L., Riding, K., et al. (2017). Differential lower airway dendritic cell patterns may reveal distinct endotypes of rsv bronchiolitis. Thorax 72, 620–627. doi: 10.1136/thoraxjnl-2015-207358
Klopfenstein, T., Zayet, S., Lohse, A., Balblanc, J.-C., Badie, J., Royer, P.-Y., et al. (2020). Tocilizumab therapy reduced intensive care unit admissions and/or mortality in covid-19 patients. Med. Maladies Infect. 50, 397–400. doi: 10.1016/j.medmal.2020.05.001
Korn, T., Mitsdoerffer, M., Croxford, A. L., Awasthi, A., Dardalhon, V. A., Galileos, G., et al. (2008). IL-6 controls Th17 immunity in vivo by inhibiting the conversion of conventional T cells into Foxp3+ regulatory T cells. PNAS; Proc. Natl. Acad. Sci. 105, 18460–18465. doi: 10.1073/pnas.0809850105
Lane, T. R., Massey, C., Comer, J. E., Anantpadma, M., Freundlich, J. S., Davey, R. A., et al. (2019). Repurposing the antimalarial pyronaridine tetraphosphate to protect against Ebola virus infection. PloS Neglect. Trop. Dis. 13, 1–26. doi: 10.1371/journal.pntd.0007890
Lee, D. W., Gardner, R., Porter, D. L., Louis, C. U., Ahmed, N., Jensen, M., et al. (2014). Current concepts in the diagnosis and management of cytokine release syndrome. Blood 124, 188–195. doi: 10.1182/blood-2014-05-552729
Lescure, F.-X., Bouadma, L., Nguyen, D., Parisey, M., Wicky, P.-H., Behillil, S., et al. (2020). Clinical and virological data of the first cases of COVID-19 in Europe: a case series. Lancet Infect. Dis. 20 (6), 697–705. doi: 10.1016/s1473-3099(20)30200-0
Liang, Y., Yang, K., Guo, J., Wroblewska, J., Fu, Y. X., Peng, H. (2015). Innate lymphotoxin receptor mediated signaling promotes HSV-1 associated neuroinflammation and viral replication. Sci. Rep. 5, 10406. doi: 10.1038/srep10406
Liu, B., Li, M., Zhou, Z., Guan, X., Xiang, Y. (2020). Can we use interleukin-6 (IL-6) blockade for coronavirus disease 2019 (COVID-19)-induced cytokine release syndrome (CRS)? J. Autoimmun. 111 (1), 102452. doi: 10.1016/j.jaut.2020.102452
Luo, P., Liu, Y., Qiu, L., Liu, X., Liu, D., Li, J. (2020). Tocilizumab treatment in covid-19: A single center experience. J. Med. Virol. 92, 814–818. doi: 10.1002/jmv.25801
Medesan, C., Cianga, P., Mummert, M., Stanescu, D., Ghetie, V., Ward, E. S. (1998). Comparative studies of rat IgG to further delineate the Fc:FcRn interaction site. European. J. Immunol. 28, 2092–2100. doi: 10.1002/(sici)1521-4141(199807)28:07<2092::aid-immu2092>3.0.co;2-e
Mehta, P., McAuley, D. F., Brown, M., Sanchez, E., Tattersall, R. S., Manson, J. J. (2020a). COVID-19: consider cytokine storm syndromes and immunosuppression. Lancet 395, 1033–1034. doi: 10.1016/S0140-6736(20)30628-0
Mehta, P., McAuley, D. F., Brown, M., Sanchez, E., Tattersall, R. S., Manson, J. J. (2020b). COVID-19: consider cytokine storm syndromes and immunosuppression. Lancet 395, 1033–1034. doi: 10.1016/s0140-6736(20)30628-0
Montesarchio, V., Parella, R., Iommelli, C., Bianco, A., Manzillo, E., Fraganza, F., et al. (2020). Outcomes and biomarker analyses among patients with covid-19 treated with interleukin 6 (il-6) receptor antagonist sarilumab at a single institution in italy. J. ImmunoTher. Cancer 8 (2), e001089. doi: 10.1136/jitc-2020-001089
Price, C. C., Altice, F. L., Shyr, Y., Koff, A., Pischel, L., Goshua, G., et al. (2020). Tocilizumab treatment for cytokine release syndrome in hospitalized covid-19 patients: Survival and clinical outcomes. Chest S0012–3692 (20), 31670–31676. doi: 10.1016/j.chest.2020.06.006
Ruan, Q., Yang, K., Wang, W., Jiang, L., Song, J. (2020). Clinical predictors of mortality due to COVID-19 based on an analysis of data of 150 patients from Wuhan, China. Intens. Care Med. 46, 846–848. doi: 10.1007/s00134-020-05991-x
Ruibal, P., Oestereich, L., Lüdtke, A., Becker-Ziaja, B., Wozniak, D. M., Kerber, R., et al. (2016). Unique human immune signature of Ebola virus disease in Guinea. Nature 533, 100–104. doi: 10.1038/nature17949
Saha, A., Sharma, A. R., Bhattacharya, M., Sharma, G., Lee, S.-S., Chakraborty, C. (2020). Tocilizumab: A Therapeutic Option for the Treatment of Cytokine Storm Syndrome in COVID-19. Arch. Med. Res. 51 (6), 595–597. doi: 10.1016/j.arcmed.2020.05.009
Sanofi (2017). Drug Approval Package: Kevzara (sarilumab) Injection (U.S. Food & Drug Administration).
Sciascia, S., Aprà, F., Baffa, A., Baldovino, S., Boaro, D., Boero, R., et al. (2020). Pilot prospective open, single-arm multicentre study on off-label use of tocilizumab in patients with severe COVID-19. Clin. Exp. Rheumatol. 38 (3), 529–532.
Sinha, P., Mostaghim, A., Bielick, C. G., McLaughlin, A., Hamer, D. H., Wetzler, L. M., et al. (2020). Early administration of interleukin-6 inhibitors for patients with severe covid-19 disease is associated with decreased intubation, reduced mortality, and increased discharge. Int. J. Infect. Dis. 99, 28–33. doi: 10.1016/j.ijid.2020.07.023
Somers, E. C., Eschenauer, G. A., Troost, J. P., Golob, J. L., Gandhi, T. N., Wang, L., et al. (2020). Tocilizumab for treatment of mechanically ventilated patients with COVID-19. Clin. Infect. Dis. Ciaa954. doi: 10.1093/cid/ciaa954
Swaak, A. J., Rooyen, A. V., Nieuwenhuis, E., Aarden, L. A. (1988). lnterleukin-6 (IL-6) in Synovial Fluid and Serum of Patients with Rheumatic Diseases. Scand. J. Rheumatol. 17, 469–474. doi: 10.3109/03009748809098809
Tan, L., Wang, Q., Zhang, D., Ding, J., Huang, Q., Tang, Y., et al. (2020). Lymphopenia predicts disease severity of COVID-19: a descriptive and predictive study. Signal Transduct. Target Ther. 5, 33. doi: 10.1038/s41392-020-0148-4
Tanaka, T., Narazaki, M., Kishimoto, T. (2016). Immunotherapeutic implications of IL-6 blockade for cytokine storm. Immunotherapy 8, 959–970. doi: 10.2217/imt-2016-0020
Tang, F., Hansbro, P., Burgess, J., Ammit, A., Baines, K., Oliver, B. (2016). A novel immunomodulatory function of neutrophils on rhinovirus-activated monocytes in vitro. Thorax 71, 1039–1049. doi: 10.1136/thoraxjnl-2015-207781
Toniati, P., Piva, S., Cattalini, M., Garrafa, E., Regola, F., Castelli, F., et al. (2020). Tocilizumab for the treatment of severe covid-19 pneumonia with hyperinflammatory syndrome and acute respiratory failure: A single center study of 100 patients in brescia, italy. Autoimmun. Rev. 19:102568. doi: 10.1016/j.autrev.2020.102568 Special issue COVID19 and Autoimmunity.
Vanoni, S., Tsai, Y.-T., Waddell, A., Waggoner, L., Klarquist, J., Divanovic, S., et al. (2017). Myeloid-derived NF-κβnegative regulation of PU.1 and c/EBP-β-driven pro-inflammatory cytokine production restrains LPS-induced shock. Innate Immun. 23, 175–187. doi: 10.1177/1753425916681444
Wauquier, N., Becquart, P., Padilla, C., Baize, S., Leroy, E. M. (2010). Human fatal zaire ebola virus infection is associated with an aberrant innate immunity and with massive lymphocyte apoptosis. PloS Negl. Trop. Dis. 4 (10), e837. doi: 10.1371/journal.pntd.0000837
Wong, C. K., Lam, C. W. K., Wu, A. K. L., Ip, W. K., Lee, N. L. S., Chan, I. H. S., et al. (2004). Plasma inflammatory cytokines and chemokines in severe acute respiratory syndrome. Clin. Exp. Immunol. 136, 95–103. doi: 10.1111/j.1365-2249.2004.02415.x
Xu, X., Han, M., Li, T., Sun, W., Wang, D., Fu, B., et al. (2020). Effective treatment of severe COVID-19 patients with tocilizumab. PNAS; Proc. Natl. Acad. Sci. 117, 10970–10975. doi: 10.1073/pnas.2005615117
Yang, M., Wang, C., Yang, S., Leu, C., Chen, S., Wu, C., et al. (2017). IL-6 ameliorates acute lung injury in influenza virus infection. Sci. Rep. 7, 43829. doi: 10.1038/srep43829
Younan, P., Iampietro, M., Bukreyev, A. (2018). Disabling of lymphocyte immune response by Ebola virus. PloS Pathog. 14, e1006932. doi: 10.1371/journal.ppat.1006932
Younan, P., Santos, R., Ramanathan, P., Iampietro, M., Nishida, A., Dutta, M., et al. (2019). Ebola virus-mediated T-lymphocyte depletion is the result of an abortive infection. PloS Pathog. 15, e1008068. doi: 10.1371/journal.ppat.1008068
Keywords: Ebola (EBOV), COVID-19, SARS-CoV-2, Anti-IL-6, Anti-IL-6R, cytokine release syndrome
Citation: Rubsamen R, Burkholz S, Massey C, Brasel T, Hodge T, Wang L, Herst C, Carback R and Harris P (2020) Anti-IL-6 Versus Anti-IL-6R Blocking Antibodies to Treat Acute Ebola Infection in BALB/c Mice: Potential Implications for Treating Cytokine Release Syndrome. Front. Pharmacol. 11:574703. doi: 10.3389/fphar.2020.574703
Received: 21 June 2020; Accepted: 31 August 2020;
Published: 23 September 2020.
Edited by:
Filippo Drago, University of Catania, ItalyReviewed by:
Charles Daniel Murin, The Scripps Research Institute, United StatesGene Garrard Olinger, MRI Global Inc, United States
Copyright © 2020 Rubsamen, Burkholz, Massey, Brasel, Hodge, Wang, Herst, Carback and Harris. This is an open-access article distributed under the terms of the Creative Commons Attribution License (CC BY). The use, distribution or reproduction in other forums is permitted, provided the original author(s) and the copyright owner(s) are credited and that the original publication in this journal is cited, in accordance with accepted academic practice. No use, distribution or reproduction is permitted which does not comply with these terms.
*Correspondence: Paul Harris, UGVoMUBjdW1jLmNvbHVtYmlhLmVkdQ==