- 1School of Pharmacy, University College London, London, United Kingdom
- 2Department of Pharmacognosy, Faculty of Pharmacy, Umm Al-Qura University, Makkah, Saudi Arabia
- 3Department of Natural Products and Alternative Medicine, Faculty of Pharmacy, King Abdulaziz University, Jeddah, Saudi Arabia
- 4Department of Translational Research and New Technologies in Medicine and Surgery, University of Pisa, Pisa, Italy
Medicinal plants indicated for chronic diseases usually have good safety margins as they are intended for lifelong treatments. We hypothesized that they may provide patients with baseline protection to cancers and multidrug resistance-reversing phytochemicals resulting in successful prevention and/or adjuvant treatment of chemotherapy-resistant cancers. We selected 27 popular herbal infusions widely used in Nigeria for diabetes and studied their effects on a panel of liver (HepG2), colon (Caco2), and skin (B16-F10) cancer cells. Cytotoxicity was measured using the SRB staining assay. The 2D antimigratory effect was evaluated using an Oris™ platform. The P-glycoprotein (P-gp) efflux activity was evaluated using Rh-123 as a fluorescent probe. The inhibition of tyrosinase-mediated melanogenesis was evaluated by colorimetric enzymatic assays. Our results show that melanoma cell proliferation was strongly inhibited by Anogeissus leiocarpus (Combretaceae), Bridelia ferruginea (Phyllanthaceae), D. ogea (Leguminosae), and Syzygium guineense (Myrtaceae) extracts (GI50 = 50 µg/ml). Alstonia boonei (Apocynaceae), Gongronema latifolium (Asclepiadaceae), and Strophanthus hispidus (Apocynaceae) were preferentially toxic against Caco2 (GI50 = 50, 5 and 35 µg/ml, respectively). The most active extracts against different drug resistance mechanisms were B. ferruginea (inhibition of P-gp efflux, and impairing tyrosinase activity) and X. americana (inhibition of P-gp efflux). A. leiocarpus, Kaya senegalensis (Meliaceae), S. guineense, and Terminalia avicennioides (Combretaceae) significantly inhibited B16-F10 cell migration. Lupeol, ursolic acid, quercitrin, epicatechin, gallic acid, and ellagic acid were dereplicated by HPLC and HPTLC as their bioactive phytochemicals. In conclusion, the above in-vitro activities of herbal infusions regularly consumed by Nigerian diabetic patients may either act as a baseline chemoprotection or as sensitizing agents.
Introduction
Natural products are both the base of traditional medicine and important sources of lead molecules in drug discovery (Thomford et al., 2018). One of the main global challenges to current cancer treatment is the emergence of multidrug resistance (MDR) to chemotherapeutic drugs, defined as acquired resistance to structurally and/or functionally unrelated drugs (Viale and Draetta, 2016). It is known that many herbal extracts (traditional medicines) act as chemosensitizers or resistance modulators (Tinoush et al., 2020). A key aspect of their activity is their ability to modulate multiple survival pathways with very low toxicity (Aung et al., 2017). Despite intense research, the choice of synthetic drugs against MDR is very limited (Dallavalle et al., 2020). The search for new natural product addressing this gap offers potential new avenues. Hence, our research groups is actively screening and testing for candidates from natural sources that can modulate the function, or expression, of disease-linked ABC transporters (Syed and Coumar, 2016).
The selected 27 plants in this work are widely used for diabetes and metabolic disorders in Nigeria, the most populated African country. Most of them are easily found in herbal markets all over the country for other primary indications including both common infective and non-communicable diseases Some of them have anecdotical records of use for the treatment of cancer without in-depth studies (Ezuruike and Prieto, 2014). These traditional medicines are consumed for years with apparently little toxicity and therefore may provide with a baseline defense against cancer, by killing or preventing tumor cells to acquire new hallmarks (Pan and Ho, 2008; Hodek et al., 2009). The real impact of cancer in Nigeria was largely unknown due to either a lack of statistics or under-reporting until the creation of the Nigerian National System of Cancer Registries in 2009 (The African Cancer Registry Network; Jedy-Agba et al., 2012). Emerging figures point out that more than 100,000 patients diagnosed with cancer and 70,000 deaths occur every year. These figures mean that about 1 in 10 Nigerians develop cancer before age 75 (International Agency for Research on Cancer).
In line with the topic of this special issue, we studied the cytotoxicity of the aqueous plant extracts (infusions) toward a panel of liver (HepG2), colon (Caco2) and skin (B16-F10) cancer cells as well as their effects upon selected mechanisms of drug resistance in cancer cells, namely, the efflux of xenobiotics by P-gp (Ozben, 2006), and the ability to inhibit tyrosinase-mediated melanogenesis and melanoma migration capacity (Pinon et al., 2011). Finally, we have proceeded to the identification of potential bioactive metabolites contributing to the effects of these plant extracts.
Materials and Methods
Plant Material
Plant materials were either bought in markets or collected from different regions in Nigeria in November 2012 by one of the authors (U.F. Ezuruike). Table 1 summarizes their botanical details and precedence. Identification of collected plant samples collected in the north was done by Mr. Ibrahim Muazzam, an ethnobotanist and staff of the Department of Medicinal Plant Research & Traditional Medicine, National Institute for Pharmaceutical Research & Development (NIPRD). Plant samples collected in the south were verified by staff of the Forestry Research Institute of Nigeria (FRIN) in Ibadan. Vouchers were deposited in the Department of Pharmaceutical and Biological Chemistry, University College London School of Pharmacy, United Kingdom. Voucher numbers are provided in Supplementary materials.
Plant Sample Preparation and Extraction
All plant samples were air-dried for about two weeks (average temperature 30°C) to remove as much moisture as possible to prevent deterioration. Samples were then packed in freezer bags and shipped to the School of Pharmacy, University College London. Upon arrival, they were further dried in a Samas Vickers® laboratory drying chamber electrically heated to 30°C with continuous ventilation. Samples were powdered using a laboratory scale mill (MF 10 Basic IKA WERKE blender) or an industrial size mill (Fritsch, Germany), depending on the part of the plant collected and the quantity of plant material.
The procedure for extraction of the plant samples (infusion or decoction) was based on the traditional use of the plants as described by the respondents (Table 1). For infusion, 300 ml of boiled distilled water was added to 10 g of plant material with continuous stirring for 15 min on a laboratory hot plate magnetic stirrer (IKA WERKE labortechnik electric stirrer). For decoction, 20 g of plant material was heated continuously under reflux in a round bottom flask containing 400 ml of distilled water for 1 h using an Electrothermal® 3-in-1 laboratory heating mantle. All extracted plant samples were then allowed to stand until cold before filtering with a Buchner flask. All the filtered extracts were frozen in round bottom flasks and then freeze dried (Edwards Pirani 50 L Savant super modulo freeze drier) to obtain the dried extract. All dried plant extracts were then stored in 10 ml sample bottles at −20°C until needed.
For analyses and cell assays, plant stock concentrations (50 mg/ml) were prepared by dissolving crude extracts in bidistilled, microfiltered, aseptic water (MiliQ). The solutions were cold sterilized by microfiltration (Millipore disk filters, 0.22 microns) in a laminar flow cabinet into sterile microcentrifuge tubes. These aliquots were at −80°C until further testing.
HPTLC Analyses
Plant extracts and phytochemical standards were diluted in methanol to a concentration of 50 and 1 mg/ml, respectively. A Linomat 5 (CAMAG, Switzerland) was used to apply 5 μl of the samples to HPTLC silica gel (Merck, Germany). The plates were developed using a CAMAG ADC2 automatic developing chamber. The method included 30-s pre-drying, 10 min humidity control using magnesium chloride to 48.3% relative humidity and 20 min saturation time, using saturation pads all done at 25.2°C. The mobile phase used was Chloroform-Methanol (8:2). During development, the solvent front was allowed to migrate 80 mm before a drying time of 5 min. For derivatization, we used Anisaldehyde/H2SO4. All visualization and analysis were done using CAMAG TLC visualizer both before and after derivatization. Phytochemical standards and solvents were from Sigma-Aldrich (UK).
HPLC-DAD Analyses
Active plant samples were fingerprinted by HPLC-UV. Chromatograms were obtained in an Agilent 1100 Series (Gradient Quaternary Pump, Online degasser, Photodiode array detector) Software ChemStation. Elution conditions for phytomarkers were as previously described (Giner et al., 1993) on a Phenomenex® C18 column (250 × 4.6 mm id, 5 μm). Solvent A (H2O + Acetic Acid 0.2%) and B (methanol + Acetic Acid 0.2% were mixed in gradient mode as follows: 0 min 90% A, 0 to 5 min 80% A, 5 to 45 min 50% A, 45 to 55 min 20% A; flow rate 0.8 ml/min. The injection volume, column temperature, and UV wavelength were set at 80 μl, 30°C and 254 nm, respectively. Phytochemical standards and solvents were from Sigma-Aldrich (UK).
Cell Lines and Cell Culture
Caco-2 wild type cells (Sigma-Aldrich) were cultured in DMEM medium (GIBCO) supplemented with 10% FBS, penicillin (100 U/ml), streptomycin (100 μg/ml), and 1% non-essential amino acid (NEEA). The HepG2 and Caco2 cells were purchased from Sigma (UK). B16-F10 murine melanoma cell lines were from Professor Kostas Kostarelos (Centre for Drug Delivery Research, School of Pharmacy, UCL, UK). HepG2 cells (Sigma-Aldrich) were cultured in MEM medium (GIBCO) supplemented with 10% FBS, penicillin (100 U/ml), streptomycin (100 μg/ml). B16-F10 cells were cultured in DMEM medium (GIBCO) supplemented with 10% FBS, penicillin (100 U/ml), streptomycin (100 μg/ml). B16-F10 Cells were grown at 37°C and maintained in DMEM Glutamex (Dulbecco’s Modified Eagle Media) containing 4,500 mg/L D-glucose, L-glutamine (3.97 mM) and sodium pyruvate. The media was supplemented with 10% of heat-inactivated fetal bovine serum (FBS) (Gibco) and 1% penicillin-streptomycin antibiotic which consists of 10,000 units of penicillin and 10,000 µl of streptomycin per ml to inhibit bacterial growth. All cells were kept at 37°C in an incubator with a humidified atmosphere of 5% CO2.
Antiproliferation Assays
The NCI protocol for SRB staining was followed as previously described (Skehan et al., 1990; National Cancer Institute, 2020). Briefly, HepG2, Caco-2, and B16-F10 cells were cultured in 96-well tissue culture plates by adding 200 μl/well of a suspension of 2 × 104, 1 × 104, and 2 × 104 cells/well respectively. After cells reached 80% confluence the culture medium was replaced with fresh medium containing 100 μg/ml of plant extracts. After 24-h incubation with the plant extracts, media containing the samples was removed, and cells were fixed with 100 µl of cold 40% w/v trichloroacetic acid (TCA) solution in deionized water. The plates were incubated at 4°C for 1 h and then rinsed five times with slow running-tap water. The TCA-fixed cells were stained by adding 100 µl of SRB solution (0.4% SRB in 0.1% acetic acid) and left at room temperature for 1 h. Afterward, the plates were quickly rinsed four times with 1% acetic acid and flicked to remove unbound dye and then left to air-dry overnight. After drying completely, the protein bound SRB was solubilized by adding 100 µl of 10 mM Tris base buffer solution to each well. The plates were agitated in an orbital shaker for 30 min. The optical density was measured at 492 nm by using a microtiter plate reader (Tecan, Switzerland). Both Growth Inhibition 50% (GI50) and Maximum Non-Toxic Concentrations (MNTC) were calculated for each cell line.
Rhodamine 123 Uptake Assay
Rhodamine uptake/efflux assays were conducted as previously described (Chieli et al., 2009) with minor modifications. Cells were seeded into 96-well plates for 24 h to allow for attachment. Then, the growth media was changed to serum free media. Rhodamine (5 µg/ml) was added to the wells containing 100 µg/ml of all the extracts to be tested. 20 µM verapamil was used as positive control. After 2 h incubation, cells were washed with 20 µM verapamil in PBS. Cells were lysed with 100 µl of 0.1% Triton X-100 in PBS and the plates were placed in the incubator for 15 min. 80 µl of each of the cell lysates in each well was transferred to a 96-well black plate and the fluorescence intensity of each well was measured in a Tecan® plate reader (Exc-485 nm, Em-525 nm). The cellular accumulation of Rh-123 for each of the extracts was expressed as the percentage of the accumulation measured for rhodamine only, that is under control conditions.
Tyrosinase Activity Assay
Tyrosinase inhibitory activity was assessed as previously described (Masuda et al., 2007). Briefly, L-tyrosine 2.5 mM -in PBS- and the sample- in DMSO- are mixed in a total volume of 160 µl to which 40 µl of Mushroom tyrosinase (46 units/ml) is added. After 20 min, the absorbance was measured for each well at 475 nm, then the % of Tyrosinase inhibition calculated against the control and corrected with matched blanks. All reagents were from Sigma-Aldrich (UK).
2D Migration Assay
The cell migration was measured using OrisTM Universal Cell Migration Assembly Kit. In this assay, inserts were used to create an exclusion zone in a cell monolayer. For the migration assay, the sterile stoppers were introduced in a flat-bottom 96-well plate before starting cell seeding. B16-F10 cells were plated at a density of 7.5 × 104 cells per well. After 24 h of incubation, a monolayer had been formed with an exclusion zone in the center. Afterward, the stoppers were removed manually and then the cells were incubated with the MNTC of each extract, vehicle control and hydroxyurea at (75 µM), which used as a positive control in this assay. At the end of the incubation period, the cells were fixed with 100 µl of cold 40% TCA. The plates were kept at 4°C for 1 h and rinsed with water. The cells were stained by the addition of 50 µl of trypan blue and incubated at room temperature for 1 h. Another washing step was performed, and the plate was then left to air-dry completely overnight. Measurement of zone closure was performed by digital images obtained by optical scanning (transmittance mode) by a Snapscan e50 (Agfa-Gevaert, Germany). Image J (National Health Institute, USA) was used to measure an average gap distance value and compare to the vehicle control in order to monitor the migratory capacity of the treated cells. The migration rate was calculated as follows: % Migration rate = [gap distance (day 0) − gap distance of the extract (24 h)]/[gap distance (day 0) − gap distance of vehicle control (24 h)].
Statistics
Curve fittings, GI50s and IC50s were performed with Excel® (Microsoft, Redmon) and GraphPad® Instat version 3 (GraphPad Software, La Jolla, US). Significance of the results was assessed with Instat® (GraphPad Software, La Jolla, US).
Results and Discussion
Cytotoxicity of the Extracts
Rather than calculating exact values, we classified the GI50 between standard doses and applied the threshold proposed by Suffness and Pezzuto (1999), which establishes that crude extracts showing an GI50 ≤ 100 µg/ml can be considered to be cytotoxic and selected for further studies, whereas the most promising ones are those with an GI50 lower than 30 µg/ml. None of the plants shown cytotoxicity toward all the cells in the panel, thus providing certain degree of specificity. The most promising cytotoxic activity was shown by G. latifolium against Caco2 colorectal cancer cells (GI50 < 10 µg/ml). Three species (D. ogea, S. hispidus, and A. senegalensis) were able to exert toxic effects on two different cell lines. Rat melanoma B16-F10 cells proliferation was strongly inhibited by A. leiocarpus, B. ferruginea, and S. guineense extracts, while A. boonei G. latifolium, S. dulcis, and S. hispidus were preferentially toxic against the human colon carcinoma Caco2 cell line. Finally, extracts from K. ivorensis and S. hispidus affected HepG2 human liver carcinoma cells only (Table 2).
Effect on Putative Drug Resistance Mechanisms
The MDR-1 gene and its product, P-glycoprotein, are considered to be among the most important drug-resistance mechanisms in cancer cells (Ozben, 2006). Nine extracts obtained from A. senegalensis, B. ferruginea, C. filiformis, D. ogea, K. ivorensis, S. guineense, T. avicennioides, and X. americana produced a change in intracellular accumulation of Rh-123 which was significantly different from the control cells in Caco-2 cells (Figure 1) which suggests that they are acting as P-gp inhibitors.
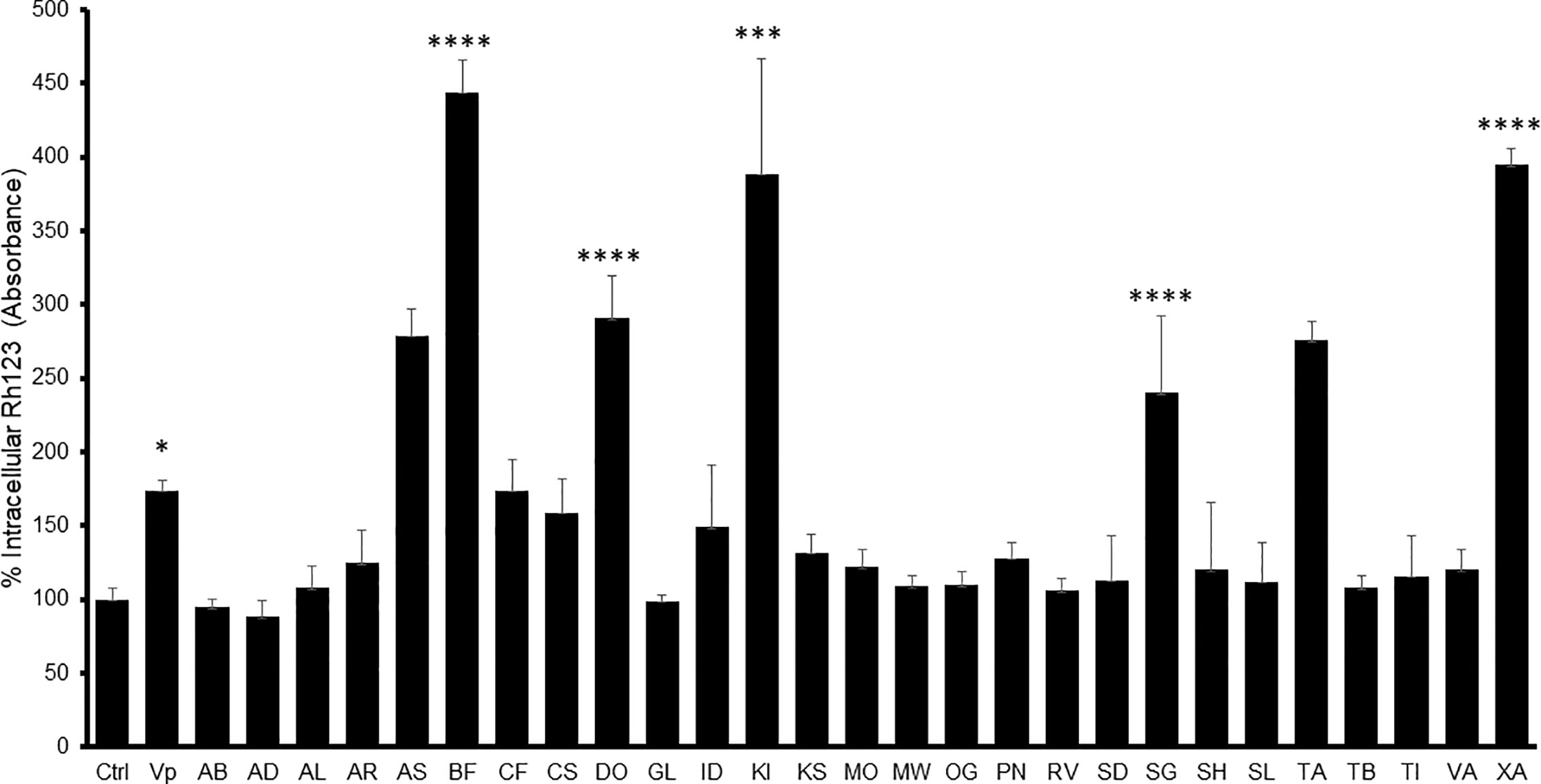
Figure 1 Intracellular Rh-123 concentration in Caco-2 cells after treatment with either extract (final concentration 100 µg/ml) or reference drug (Verapamil, VP). One-way ANOVA followed by Bonferroni’s post-test; Data are presented as the mean ± SD (n = 3), (****) p <0.0001 (***) p < 0.001; (*) p < 0.05, respectively.
In Caco-2 cells, the highest observed inhibitory effect was produced by B. ferruginea with more than 500% increase in Rh-123 accumulation compared to control cells followed by XA1 with more than 400% increase. The inhibitory effects of B. ferruginea and X. americana were thrice and twice higher than the effect of the positive control verapamil, respectively. Such high changes in intracellular Rh-123 accumulation by plant extracts are not uncommon. A similar study carried out with the stem bark extract of Mangifera indica produced a 1,000% increase in intracellular Rh-123 accumulation (Chieli et al., 2009).
Another emerging multidrug resistance mechanism in melanoma is melanin synthesis. Chen et al. demonstrated that melanosomes contribute to the refractory properties of melanoma cells by sequestering cytotoxic drugs and increasing melanosome-mediated drug export. Thus, preventing melanosomal sequestration of cytotoxic drugs by inhibiting the functions of melanosomes may have great potential as an approach to improving the chemosensitivity of melanoma cells (Chen et al., 2006). Furthermore, the same authors later established that the intrinsic MDR of melanoma cells is related to the ABC transporter systems -which includes P-gp- that may also play a critical role in reducing toxic melanin intermediates and metabolites (Chieli et al., 2009). The extracts that inhibited DOPA production under 100 µg/ml were A. senegalensis (22% ± 12), B. ferruginea (48% ± 10), D. ogea (32%± 14) and S. guineense (11%± 1) with Kojic acid showing an IC50 of 12.65 ± 3 µM in our conditions.
Antimigratory Activity
Figure 2 shows the effect of the active extracts on the cellular migration of the B16-F10. In vitro antimigratory activity against the highly metastatic B16-F10 cell line at 200 µg/ml was shown by A. boonei, A. leiocarpus, K. senegalensis, and T. avicennioides, while pro-migratory activity was detected for C. filiformis. The decoction of S. guineense was the most active at inhibiting migration but the cell population shown signs of toxicity. Of note, D. ogea, A. senegalensis, B. ferruginea, M. whiteii, and T. bangwensis did not exhibit a significant inhibitory effect of the 2D cell migration assay even when assayed at cytotoxic concentrations. When tested at MNTC only A. boonei, K. senegalensis, and T. avicennioides kept their activity.
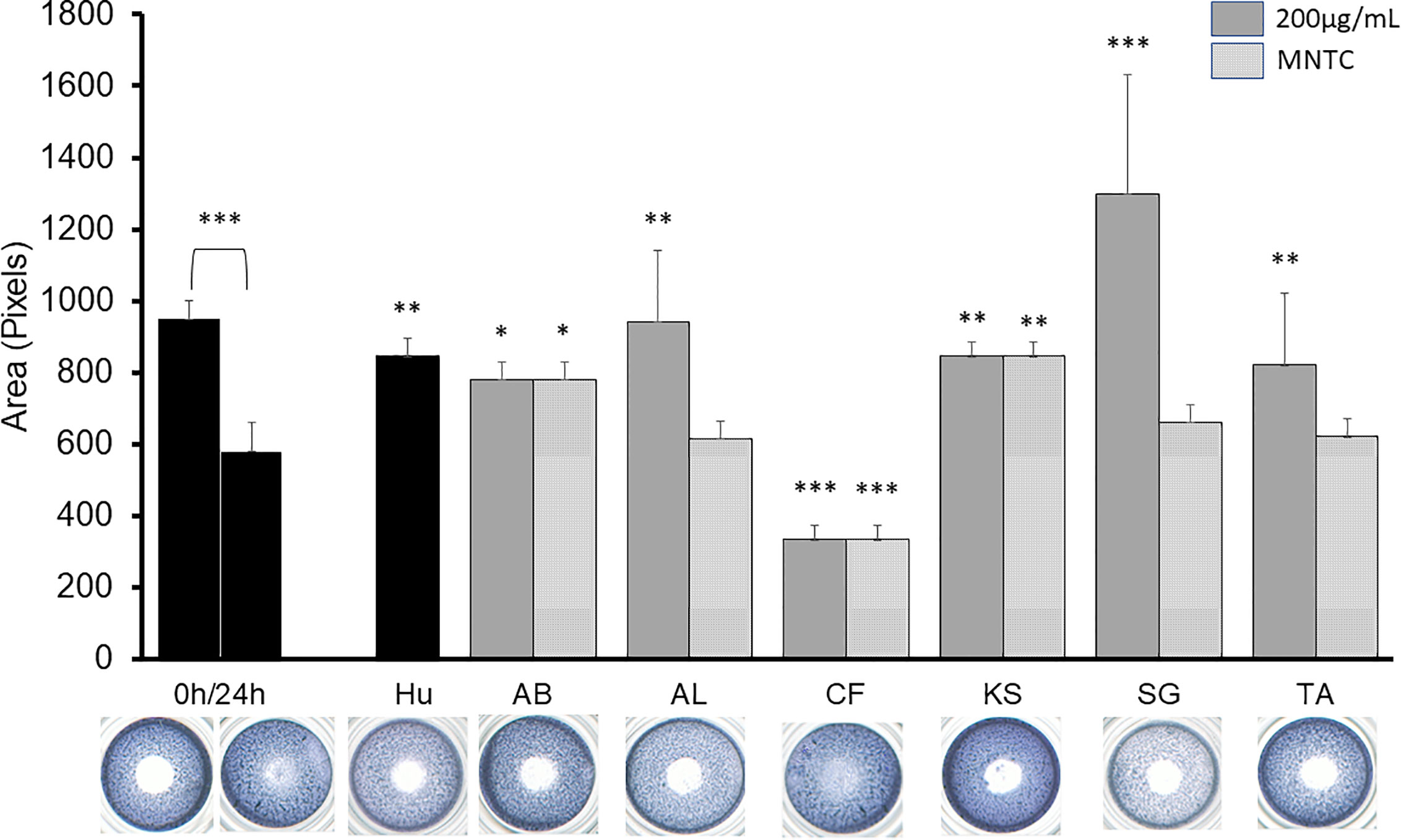
Figure 2 2D anti-migratory effects of the selected extracts at both GI50, MNTC and reference compound (HU 75 µM) on B16-F10 murine melanoma cells for 24 h. Area of the gap (measured in pixels) in a confluent cell monolayer immediately after gap generation (0 h) and at 24 h post-wounding (n = 3) with representative microscopy images for the treated gaps. The significance of inhibitory effect was determined by one-way ANOVA followed by Bonferroni’s post-test with respect to t24 control; Data are presented as the mean ± SD (n = 3), (***) p < 0.001; (**) p < 0.01; (*) p < 0.05, respectively.
A sequential liquid/liquid extraction of the extracts was performed to obtain chloroform (C), butanol (B) and water (W) fractions with significant antimigratory effects at 100 µg/ml as shown in Figure 3: AB-C, AL-B, KS-B, KS-W, SG-B, SG-W, and TA-W. When tested at MNTC only AB-C, KS-B, and KS-W kept their activity intact.
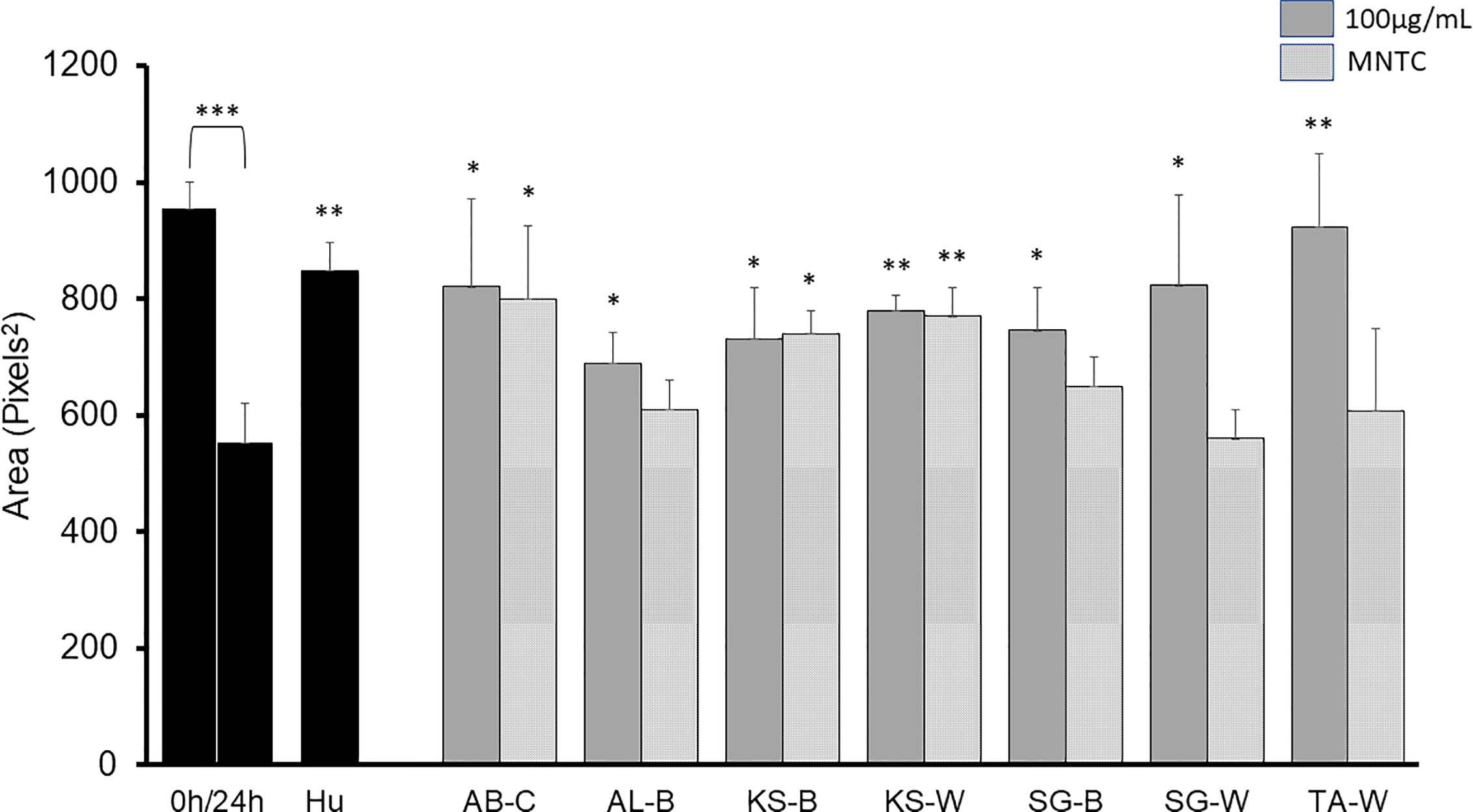
Figure 3 2D anti-migratory effects of fractions of active plant extracts with inhibitory effect at both GI50 and MNTC on B16-F10 murine melanoma cells after 24 h. Area of the gap (measured in pixels) in a confluent cell monolayer immediately after gap generation (0 h) and at 24 h post-wounding. HU: Hydroxyurea (reference compound, 75 µM); C: chloroform fraction; B: butanol fraction; W: water fraction. Data are presented as the mean ± SD (n = 3), (***) p < 0.001; (**) p < 0.01; (*) p < 0.05, respectively.
Dereplication of Known Bioactive Compounds
Screening of natural products for potential anticancer activities must always be accompanied by dereplication strategies so to avoid needless isolation of known bioactive compounds (Corley and Durley, 1994).
All extracts were chromatographed and dereplicated for Gallic acid, caffeic acid, epicatechin, epigallocatechin, catechin, vitexin rhamnoside, vitexin, rutin, ellagic acid, quercitrin, hesperidin, quercetin, luteolin, kaempferol, ursolic acid, betulinic acid, and lupeol. The resulting chromatograms are provided as supplementary materials. Supplementary Table 1 summarizes the phenolic compounds detected in our plant samples versus those already reported in literature for the plant species.
The activities of A. boonei may be explained by the presence of lupeol [1], ursolic acid [2] (TLC) and quercitrin [6] (HPLC); A. leiocarpus contains Epicatechin [5], Gallic acid [3], and ellagic acid [4] (HPLC) and S. guineense Gallic acid [3] (HPLC). Their chemical structures are presented in Figure 4.
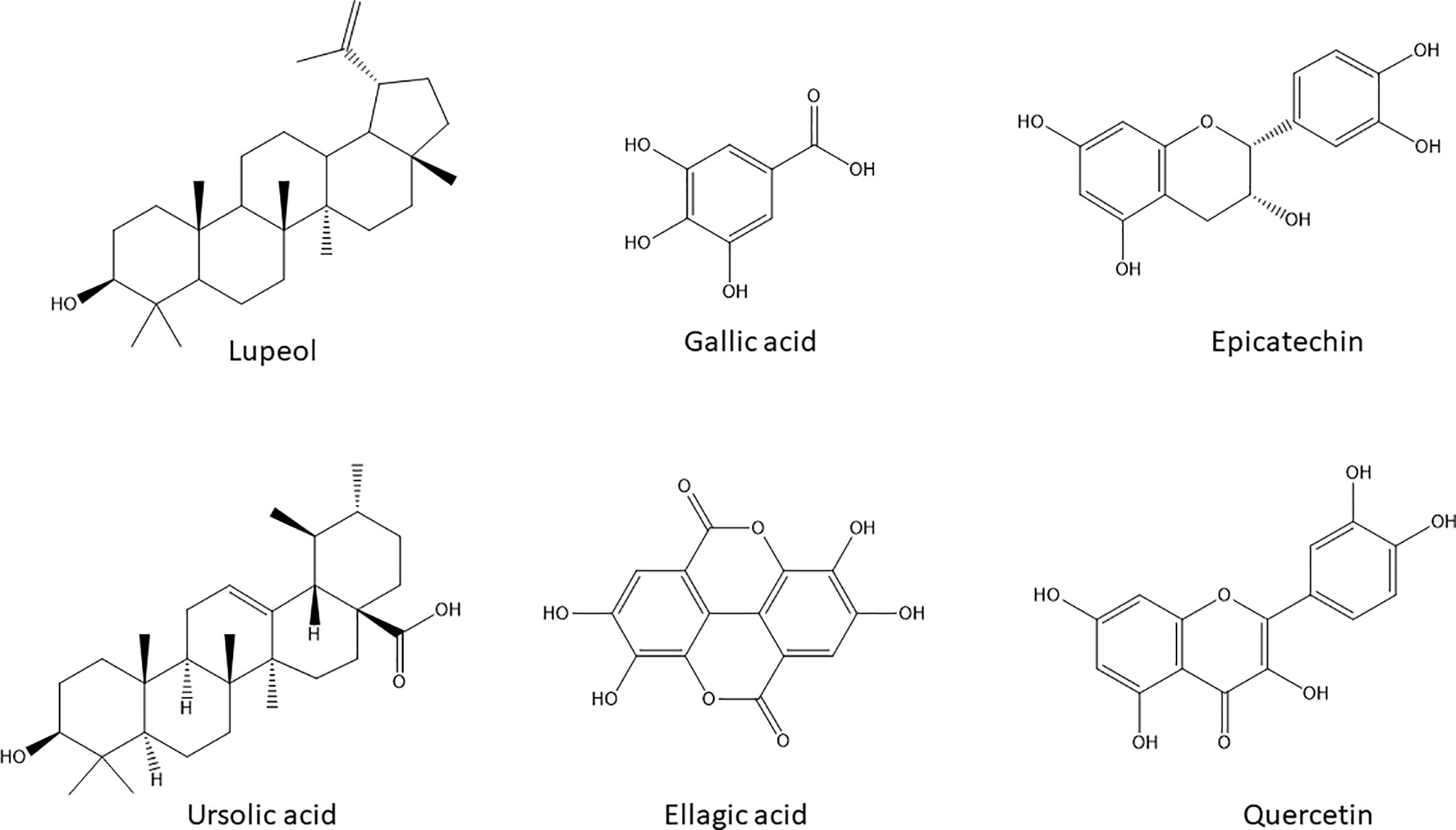
Figure 4 Chemical structures of the natural products with potential anticancer and antimigratory activities dereplicated by HPLC-DAD and HPTLC in the selected active extracts.
Lupeol [1] and ursolic acid [2] are regarded as powerful inducers of apoptosis in cancer cells with added modulatory effects upon drug resistance mechanisms in cancer cells including P-gp activity (Yan et al., 2014). Gallic acid [3] besides its well- known antioxidant activity can decrease P-gp expression in HK-2 cells (Chieli et al., 2010) and directly modify its function on KB-C2 cells (Kitagawa et al., 2004). Ellagic acid [4], Epicatechin [5], and quercitrin [6] are also endowed with anticarcinogenic effects by inhibiting tumor cell proliferation, inducing apoptosis and inhibiting drug-resistance processes—specially efflux pumps and transporters—required for tumor growth (Tan et al., 2013; Zhang et al., 2014). Moreover, all these chemicals are inhibitors of migration of melanoma cell lines (Alqathama and Prieto, 2015).
Conclusions
This study results demonstrated that some of these herbal medicines are endowed with significant in vitro cytotoxicity and inhibit MDR mechanisms. More refined studies would be necessary to ascertain the in vivo and/or clinical significance of such properties. In terms of direct cytotoxic effects, the aqueous extracts of G. latifolium and S. hispidus emerge as promising leads according the strictest standards (GI50 < 30 µg/ml, Caco2 cells). It is conceivable that traditional infusions can reach pharmacologically active levels in the gastrointestinal system. Therefore, their chronic intake by diabetic patients may provide a baseline protection against colon cancer. This warrants further in vivo studies to confirm if their active principles are not degraded in the stomach and can reach the colon.
On the other hand, B. ferruginea and X. americana shown the largest effects on P-gp efflux, a clinically relevant target. These herbs have additional inhibitory effects on other putative MDR mechanisms namely depletion of glutathione as per our previous work (Ezuruike et al., 2019) and impairing tyrosinase activity (as per this work), respectively. Thus, they are clear candidates for adjuvant or combination cancer therapy.
Our study also shows for first time that the following herbal extracts exert in vitro antimigratory activity against the highly metastatic B16-F10 cell line: A. leiocarpus, K. senegalensis, S. guineense, and T. avicennioides. Some of their bioactive phytochemicals (lupeol, ursolic acid gallic acid, ellagic acid, epicatechin, and quercitrin) were dereplicated by HPLC and HPTLC.
Although further studies are needed to attest the clinical relevance of our findings, we hope that this work will stimulate similar research toward establishing the clinical relevance of these herbal medicines in Nigeria.
Data Availability Statement
The raw data supporting the conclusions of this article will be made available by the authors, without undue reservation, to any qualified researcher.
Author Contributions
AA performed cytotoxicity assays and 2D migratory assays (B16-F10 cells). UE collected plant materials in the field, performed cytotoxicity assays (Caco2, HepG2) and P-gp assay. AY performed tyrosinase activity assays. AM and EC designed, supervised, and advised on P-gp assay. JP designed the overall work, supervised all assays, performed HPLC analyses, and wrote the manuscript.
Funding
This research project was conducted with support from the Saudi Arabian Ministry of Education, Umm Al-Qura University (SACB Ref. UMU397) and The Commonwealth (Scholarship NGCS-2010-306).
Conflict of Interest
The authors declare that the research was conducted in the absence of any commercial or financial relationships that could be construed as a potential conflict of interest.
Acknowledgments
AA was a holder of a full-PhD scholarship from the Saudi Arabian Ministry of Education. AM was a holder of a full-PhD scholarship from the CNPq—Grant number: 201327/2012-0Ms. AE was a holder of a full-PhD scholarship from The Commonwealth. AY was a holder of a full-MSc scholarship from the Saudi Arabian Ministry of Education.
Supplementary Material
The Supplementary Material for this article can be found online at: https://www.frontiersin.org/articles/10.3389/fphar.2020.546439/full#supplementary-material
References
Alqathama, A., Prieto, J. M. (2015). Natural products with therapeutic potential in melanoma metastasis. Nat. Prod. Rep. 32, 1170–1182. doi: 10.1039/C4NP00130C
Aung, T. N., Qu, Z., Kortschak, R. D., Adelson, D. L. (2017). Understanding the Effectiveness of Natural Compound Mixtures in Cancer through Their Molecular Mode of Action. Int. J. Mol. Sci. 18, 656. doi: 10.3390/ijms18030656
Chen, K. G., Valencia, J. C., Lai, B., Zhang, G., Paterson, J. K., Rouzaud, F., et al. (2006). Melanosomal sequestration of cytotoxic drugs contributes to the intractability of malignant melanomas. Proc. Natl. Acad. Sci. U. States America 103, 9903–9907. doi: 10.1073/pnas.0600213103
Chieli, E., Romiti, N., Rodeiro, I., Garrido, G. (2009). In vitro effects of Mangifera indica and polyphenols derived on ABCB1/P-glycoprotein activity. Food Chem. Toxicol. 47, 2703–2710. doi: 10.1016/j.fct.2009.07.017
Chieli, E., Romiti, N., Rodeiro, I., Garrido, G. (2010). In vitro modulation of ABCB1/P-glycoprotein expression by polyphenols from Mangifera indica. Chem. Biol. Interact. 186, 287–294. doi: 10.1016/j.cbi.2010.05.012
Corley, D. G., Durley, R. C. (1994). Strategies for database dereplication of natural products. J. Natural Prod. 57, 1484–1490. doi: 10.1021/np50113a002
Dallavalle, S., Dobričić, V., Lazzarato, L., Gazzano, E., Machuqueiro, M., Pajeva, I., et al. (2020). Improvement of conventional anti-cancer drugs as new tools against multidrug resistant tumors. Drug Resist. Update 50, 100682. doi: 10.1016/j.drup.2020.100682
Ezuruike, U. F., Prieto, J. M. (2014). The use of plants in the traditional management of diabetes in Nigeria: pharmacological and toxicological considerations. J. Ethnopharmacol. 155, 857–924. doi: 10.1016/j.jep.2014.05.055
Ezuruike, U. F., Chieli, E., Prieto, J. M. (2019). In Vitro Modulation of Glibenclamide Transport by P-glycoprotein Inhibitory Antidiabetic African Plant Extracts. Planta Med. 85, 987–996. doi: 10.1055/a-0948-9072
Giner, R. M., Recio, M. C., Cuellar, M. J., Máñez, S., Peris, J. B., Stübing, G., et al. (1993). A Taxonomical Study of the Subtribe Leontodontinae Based on the Distribution of Phenolic Compounds. Biochem. Syst. Ecol. 21, 613–616. doi: 10.1016/0305-1978(93)90061-U
Hodek, P., Krízková, J., Burdová, K., Sulc, M., Kizek, R., Hudecek, J., et al. (2009). Chemopreventive compounds–view from the other side. Chem. Biol. Interact. 180, 1–9. doi: 10.1016/j.cbi.2009.01.003
International Agency for Research on Cancer I. Global Cancer Observatory [Online]. Available at: https://gco.iarc.fr/ (Accessed 2020 2020).
Jedy-Agba, E., Curado, M. P., Ogunbiyi, O., Oga, E., Fabowale, T., Igbinoba, F., et al. (2012). Cancer incidence in Nigeria: A report from population-based cancer registries. Cancer Epidemiol. 36, e271–e278. doi: 10.1016/j.canep.2012.04.007
Kitagawa, S., Nabekura, T., Kamiyama, S., Kitagawa, S. (2004). Inhibition of P-glycoprotein function by tea catechins in KB-C2 cells. JPP 56, 1001–1005. doi: 10.1211/0022357044003
Masuda, T., Fujita, N., Odaka, Y., Takeda, Y., Yonemori, S., Nakamoto, K., et al. (2007). Tyrosinase inhibitory activity of ethanol extracts from medicinal and edible plants cultivated in okinawa and identification of a water-soluble inhibitor from the leaves of Nandina domestica. Biosci. Biotechnol. Biochem. 71, 2316–2320. doi: 10.1271/bbb.70249
National Cancer Institute (2020). NCI-60 Screening Methodology. [Online]. Available at: https://dtp.cancer.gov/discovery_development/nci-60/methodology.htm (Accessed 2018 2018).
Ozben, T. (2006). Mechanisms and strategies to overcome multiple drug resistance in cancer. FEBS Lett. 580, 2903–2909. doi: 10.1016/j.febslet.2006.02.020
Pan, M. H., Ho, C. T. (2008). Chemopreventive effects of natural dietary compounds on cancer development. Chem. Soc. Rev. 37, 2558–2574. doi: 10.1039/b801558a
Pinon, A., Limami, Y., Micallef, L., Cook-Moreau, J., Liagre, B., Delage, C., et al. (2011). A novel form of melanoma apoptosis resistance: melanogenesis up-regulation in apoptotic B16-F0 cells delays ursolic acid-triggered cell death. Exp. Cell Res. 317, 1669–1676. doi: 10.1016/j.yexcr.2011.04.014
Skehan, P., Storeng, R., Scudiero, D., Monks, A., Mcmahon, J., Vistica, D., et al. (1990). New colorimetric cytotoxicity assay for anticancer-drug screening. J. Natl. Cancer Inst. 82, 1107–1112. doi: 10.1093/jnci/82.13.1107
Syed, S. B., Coumar, M. S. (2016). P-Glycoprotein Mediated Multidrug Resistance Reversal by Phytochemicals: A Review of SAR & Future Perspective for Drug Design. Curr. Top. Med. Chem. 16, 2484–2508. doi: 10.2174/1568026616666160212123814
Tan, K. W., Li, Y., Paxton, J. W., Birch, N. P., Scheepens, A. (2013). Identification of novel dietary phytochemicals inhibiting the efflux transporter breast cancer resistance protein (BCRP/ABCG2). Food Chem. 138, 2267–2274. doi: 10.1016/j.foodchem.2012.12.021
Thomford, N. E., Senthebane, D. A., Rowe, A., Munro, D., Seele, P., Maroyi, A., et al. (2018). Natural Products for Drug Discovery in the 21st Century: Innovations for Novel Drug Discovery. Int. J. Mol. Sci. 19, 1578. doi: 10.3390/ijms19061578
Tinoush, B., Shirdel, I., Wink, M. (2020). Phytochemicals: Potential Lead Molecules for MDR Reversal. Front. Pharmacol. 11, 832. doi: 10.3389/fphar.2020.00832
Viale, A., Draetta, G. F. (2016). Metabolic Features of Cancer Treatment Resistance. Recent Results Cancer Res. 207, 135–156. doi: 10.1007/978-3-319-42118-6_6
Yan, X. -J., Gong, L. -H., Zheng, F. -Y., Cheng, K. -J., Chen, Z. -S., Shi, Z., et al. (2014). Triterpenoids as reversal agents for anticancer drug resistance treatment. Drug Discov. Today 19, 482–488. doi: 10.1016/J.DRUDIS.2013.07.018
Keywords: cell viability, cell migration, multi drug resistance, tyrosinase, medicinal plants
Citation: AlQathama A, Ezuruike UF, Mazzari ALDA, Yonbawi A, Chieli E and Prieto JM (2020) Effects of Selected Nigerian Medicinal Plants on the Viability, Mobility, and Multidrug-Resistant Mechanisms in Liver, Colon, and Skin Cancer Cell Lines. Front. Pharmacol. 11:546439. doi: 10.3389/fphar.2020.546439
Received: 28 March 2020; Accepted: 26 August 2020;
Published: 15 September 2020.
Edited by:
Patrícia Mendonça Rijo, CBIOS, Universidade Lusófona Research Center for Biosciences & Health Technologies, PortugalReviewed by:
Sayeed Ahmad, Jamia Hamdard University, IndiaFrancis-Alfred Unuagbe Attah, University of Ilorin, Nigeria
Copyright © 2020 AlQathama, Ezuruike, Mazzari, Yonbawi, Chieli and Prieto. This is an open-access article distributed under the terms of the Creative Commons Attribution License (CC BY). The use, distribution or reproduction in other forums is permitted, provided the original author(s) and the copyright owner(s) are credited and that the original publication in this journal is cited, in accordance with accepted academic practice. No use, distribution or reproduction is permitted which does not comply with these terms.
*Correspondence: Aljawharah AlQathama, YWFxYXRoYW1hQHVxdS5lZHUuc2E=