- 1Department of Nephrology, Radboud University Medical Center, Nijmegen, Netherlands
- 2Department of Nephrology, Jeroen Bosch Hospital, ‘s-Hertogenbosch, Netherlands
- 3Department of Geriatric Medicine Jeroen Bosch Hospital, ‘s-Hertogenbosch, Netherlands
- 4Department of Clinical Pharmacology, Jeroen Bosch Hospital, ‘s-Hertogenbosch, Netherlands
- 5Department of Medicine for Older People, VU University Medical Center, Amsterdam, Netherlands
Background: Coronavirus disease 2019 (COVID-19) is caused by severe acute respiratory syndrome coronavirus 2 (SARS-CoV-2). It is currently unknown whether immunosuppressive drugs are advantageous or detrimental in patients with COVID-19. Immunosuppressive drugs could be harmful in the initial phase of COVID-19. In this phase, the host immune response is necessary to inhibit viral replication. However, immunosuppressive drugs might have a beneficial effect in the later, more severe phase of COVID-19. In this phase, an overshoot of the host immune response (the “cytokine storm”) can cause ARDS, multiorgan failure and mortality.
Aim: To summarize the available evidence on the effect of immunosuppressive drugs on infection with SARS-CoV-2. The effects of immunosuppressive drugs on similar pandemic coronaviruses may resemble the effects on SARS-CoV-2. Thus, we also included studies on the severe acute respiratory syndrome coronavirus (SARS-CoV) and Middle East respiratory syndrome coronavirus (MERS-CoV)
Methods: The study protocol was registered in PROSPERO (registration number CRD42020181137). We included randomized controlled trials (RCTs), cohort studies with a control group and case-control studies concerning humans ≥ 18 years old. We also included in-vitro studies and animal studies with a control group.
Results and Conclusion: Sixty-nine studies were included. Interestingly, MPA inhibits SARS-CoV-2 replication in-vitro. Clinical studies are needed to confirm the inhibitory effect of MPA on SARS-CoV-2 replication in-vivo. There are indications that corticosteroids and IL-6 inhibitors, like tocilizumab, can reduce mortality and prevent mechanical ventilation in patients with COVID-19. However, observational studies have contradictory results and the risk of bias is high. Thus, these results have to be confirmed in high-quality clinical trials before these drugs can be implemented as standard care. Based on the positive results of CNIs, mTOR inhibitors and thiopurine analogues in in-vitro studies with SARS-CoV and MERS-CoV, it would be interesting to investigate their effects on SARS-CoV-2 replication.
Introduction
Coronavirus disease 2019 (COVID-19), caused by severe acute respiratory syndrome coronavirus 2 (SARS-CoV-2), has a mild course in the majority of patients. However, approximately 14% of the patients require hospitalization for oxygen support and 5% is admitted to the intensive care unit (ICU) (World Health Organization (WHO), 2020).
COVID-19 has a triphasic course (Siddiqi and Mehra, 2020). In the first phase, patients have mild respiratory and systemic symptoms such as dry cough, malaise and fever. An adequate response of the innate and adaptive immune system can eliminate the virus and preclude disease progression to the next, more severe stages (Shi et al., 2020). In the second phase, viral multiplication and localized inflammation of the lung tissue occurs, causing viral pneumonia. A minority of patients with COVID-19 will undergo a transition into the third and most severe phase of illness: a syndrome of systemic hyperinflammation, also referred to as secondary hemophagocytic lymphohistiocytosis or the cytokine storm syndrome. These patients have high levels of pro-inflammatory cytokines, such as interleukin (IL)-2, IL-6, IL-7 and tumor-necrosis factor-α (TNF-α) (Huang et al., 2020; Zhang et al., 2020). In this phase, patients can develop acute respiratory distress syndrome (ARDS) and multiorgan failure, which are the main causes of mortality of COVID-19 (Cao et al., 2020; Du et al., 2020; Mehta et al., 2020; Ruan et al., 2020; Wu et al., 2020).
It is hypothesized that immunosuppressive drugs could be used to prevent and treat the hyperinflammatory phase of COVID-19. However, these drugs inhibit the host immune response against the virus as well. Thus, they could be harmful in earlier stages of COVID-19. The net effect of immunosuppressive drugs in patients with COVID-19 is currently subject of debate.
The primary aim of this review is to summarize the available evidence on the effect of immunosuppressive drugs on infection with SARS-CoV-2. Given the short time period since the start of the COVID 19 pandemic, relatively few studies on COVID-19 are completed. Therefore, we also searched for papers reporting the effect of immunosuppressive drugs on infection with severe acute respiratory syndrome coronavirus (SARS-CoV) and Middle East respiratory syndrome coronavirus (MERS-CoV), two other pandemic coronaviruses belonging to the Nidovirales order (de Wit et al., 2016; Huang et al., 2020). These viruses are the causative agents of severe acute respiratory syndrome (SARS) and Middle East respiratory syndrome (MERS), which share many features with COVID-19 (Wang et al., 2004; Yin and Wunderink, 2018; Wang D. et al., 2020). Thus, the effects of immunosuppressive drugs on these viruses may resemble the effects on SARS-CoV-2. The results of this review can be used in the development of evidence-based guidelines for the management of patients with COVID-19 and of patients using immunosuppressive drugs during the COVID-19 pandemic.
Methods
We conducted a PubMed search, without limitations for the publication date or publication type. In the search, the terms “COVID-19”, “SARS”, “MERS” (and multiple synonyms) were combined with search terms for the different classes of immunosuppressive drugs (see: Supplementary File 1). The last search was performed on 8 July 2020. All papers were screened by title and abstract, and the full text of potentially eligible papers was read. In addition, the reference list of all identified papers was screened manually. To be included in our review, studies had to meet the following inclusion criteria:
1. Studies concerning humans ≥ 18 years old, animals or cells infected with SARS-CoV-2, SARS-CoV or MERS-CoV.
2. In-vitro or in-vivo treatment with any of the following immunosuppressive drugs: calcineurin inhibitors (CNIs; cyclosporine (CsA), tacrolimus(TAC)), antimetabolites (like mycophenolic acid (MPA), azathioprine (AZA), methotrexate), mammalian target of rapamycin (mTOR) inhibitors (sirolimus, everolimus), corticosteroids (like methylprednisolone, hydrocortisone, prednisone, dexamethasone), cyclophosphamide, rituximab, alemtuzumab, IL-6 inhibitors (like tocilizumab), basiliximab, anakinra, dupilumab, brodalumab, secukinumab, ixekizumab, anti-TNF-α inhibitors (like infliximab), abatacept, belatacept, or eculizumab.
3. Data on one or more of the following outcome measures: viral load, viral replication, clinical outcome (e.g. mortality rate, ICU admission rate, length of hospital stay).
4. Study type: in-vitro study, animal study with control group, randomized controlled trial (RCT), cohort study with control group, case-control study.
5. Language: English.
We categorized the obtained information per immunosuppressive drug class. In addition, we sorted the data according to the type of outcome parameter, i.e. viral load or clinical outcome. The study protocol was registered in PROSPERO (registration number CRD42020181137).
Results
The database search yielded 1939 search hits, with 69 studies matching the inclusion criteria. A summary of the results is presented in Table 1.
Corticosteroids
Corticosteroids have a wide range of anti-inflammatory and immunomodulatory effects, including inhibition of the synthesis of pro-inflammatory cytokines, reduction of leucocyte trafficking, and induction of apoptosis of T-lymphocytes (Lansbury et al., 2019). In addition, corticosteroids might increase the sensitivity to vasopressors (Lansbury et al., 2019) and can be used to treat adrenal insufficiency, which is present in 20% of the patients with critical illness, and in up to 60% of those with sepsis and septic shock (Hui et al., 2018).
In Table 2, the 38 clinical studies matching the inclusion criteria are summarized.
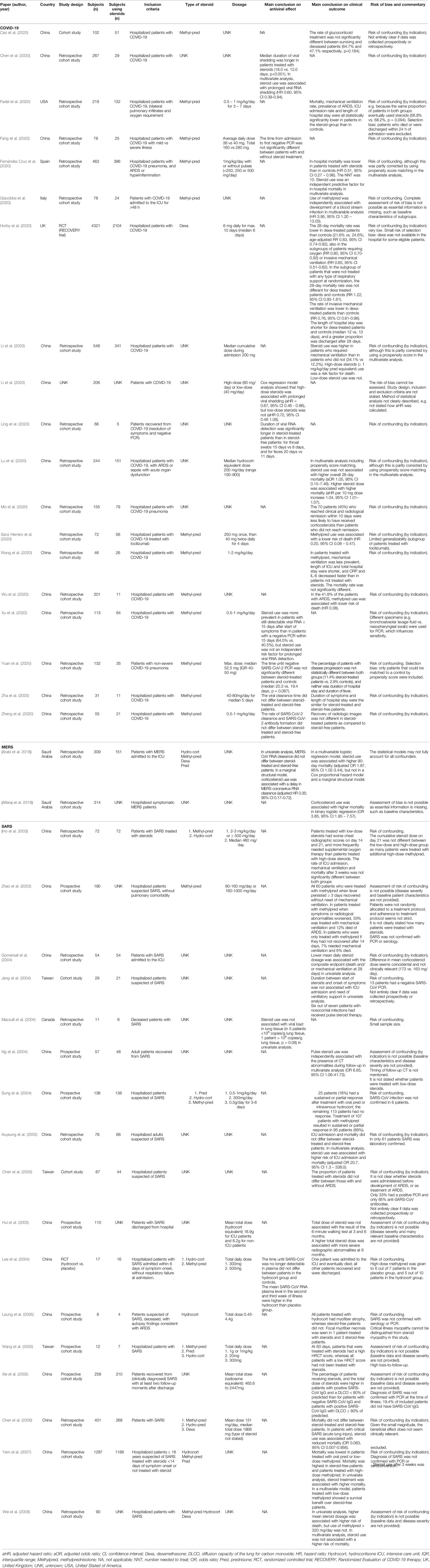
Table 2 Overview of clinical studies of corticosteroids in the treatment of patients with COVID-19, SARS and MERS.
Viral Load
1. SARS-CoV-2: Five observational studies found that SARS-CoV-2 clearance time did not differ between steroid-treated patients and those not treated with steroids (Fang et al., 2020; Xu et al., 2020; Yuan et al., 2020; Zha et al., 2020; Zheng et al., 2020). Contrarily, three other observational studies report that steroid treatment was associated with a longer time until SARS-CoV-2 clearance (Chen et al., 2020; Ling et al., 2020; Xu et al., 2020). The risk of confounding was high in all these studies.
2. SARS-CoV: In a double-blinded RCT, patients with SARS were randomized to treatment with intravenous hydrocortisone (300 mg/day for 12 days) or placebo to study the effect of hydrocortisone on SARS-CoV clearance time (Lee et al., 2004). Patients with comorbidity, respiratory failure or symptoms for ≥ 5 days were excluded. In the second and third week of illness, the mean SARS-CoV RNA plasma levels were higher in the hydrocortisone group than in the placebo group, but the SARS-CoV clearance time did not differ between both groups. However, the risk of confounding is high, because high-dose methylprednisolone (500 mg/day) was given to 5 out of 10 patients in the hydrocortisone group and to 6 out of 7 patients in the placebo group. In a retrospective cohort study, steroid use was not associated with the viral load in lung tissue obtained at autopsy in 11 SARS patients (Mazzulli et al., 2004). However, the study may have been underpowered and the risk of confounding is high.
3. MERS-CoV: In an observational study in patients with MERS admitted to the ICU, steroid use was associated with a delay in MERS-CoV RNA clearance (Arabi et al., 2018). This study has a high risk of confounding.
Clinical Outcome
1. SARS-CoV-2: In an open-label RCT, patients with COVID-19 were randomized in a ratio of 1:2 to treatment with dexamethasone (6 mg daily, oral or intravenous, for a maximum of 10 days) at hospital admission or standard care (controls) (Recovery Collaborative Group et al., 2020). Age, sex, comorbidities and the requirement for respiratory support were similar in both groups. The median number of days since onset of symptoms was 8 days (IQR 5-13 days) for patients in the dexamethasone group and 9 days (IQR 5-13 days) for controls. Overall, the 28-day mortality rate was lower in dexamethasone-treated patients compared to controls (21.6 vs. 24.6%; age-adjusted RR 0.83, 95% CI 0.75–0.93). Similarly, dexamethasone use was associated with a lower 28-day mortality rate in the subgroups of patients requiring oxygen (RR 0.82, 95% CI 0.72–0.94) or invasive mechanical ventilation (RR 0.64, 95% CI 0.51–0.81) at randomization. Only in the subgroup of patients who were not treated with any type of respiratory support at randomization, there was no significant difference in the 28-day mortality rate between dexamethasone-treated patients and controls (RR 1.19, 95% CI 0.91–1.55). Dexamethasone use was also associated with a lower rate of invasive mechanical ventilation (RR 0.76, 95% CI 0.61–0.96) and shorter length of hospital stay (median 12 vs. 13 days).
Six retrospective cohort studies also found that the use of steroids was associated with beneficial outcomes in patients with COVID-19 (Fadel et al., 2020; Fernandez Cruz et al., 2020; Lu et al., 2020; Sanz Herrero et al., 2020; Wang Y. et al., 2020; Wu et al., 2020). Two of these studies found that patients treated with steroids had a lower prevalence of mechanical ventilation and shorter length of ICU and hospital stay than controls (Fadel et al., 2020; Wang et al., 2020). In three other studies that only included COVID-19 patients with ARDS, the mortality rate was lower in patients treated with steroids than in controls (Fernandez Cruz et al., 2020; Lu et al., 2020; Wu et al., 2020). In COVID-19 patients treated with tocilizumab, additional administration of steroids was also associated with a lower mortality rate (Sanz Herrero et al., 2020).
In contrast, two retrospective cohort studies found that steroid use was associated with detrimental outcome (Giacobbe et al., 2020; Mo et al., 2020). The first study included COVID-19 patients who were admitted to the ICU. They found that methylprednisolone use was independently associated with development of a blood stream infection (Giacobbe et al., 2020). Another retrospective cohort study found that patients treated with steroids were less likely to reach clinical and radiological remission within 10 days than patients who did not use steroids (Mo et al., 2020). However, this study has a high risk of confounding by indication.
Lastly, one cohort study in hospitalized COVID-19 patients found that the rate of steroid treatment was not significantly different between surviving and deceased patients (Cao et al., 2020). Again, there was significant risk of confounding.
2. SARS-CoV: Similar to the results for SARS-CoV-2, observational studies in patients with SARS have different results. In one cohort study that included patients with severe SARS, steroid use was associated with reduced mortality (Chen et al., 2006). Three other cohort studies found that treatment with steroids had no effect on the mortality rate (Chen et al., 2006; Wei et al., 2009) or development of ARDS (Chen et al., 2005) in patients with SARS.
Several other cohort studies report that steroid use was associated with detrimental outcome (Auyeung et al., 2005; Leung et al., 2005; Ng et al., 2004; Wang et al., 2005; Xie et al., 2005; Yam et al., 2007). Two retrospective cohort studies report that steroid use was associated with a higher risk of ICU admission and mortality (Auyeung et al., 2005; Yam et al., 2007). In two studies that included patients who recovered from SARS, steroid use was associated with the presence of CT abnormalities and a lower diffusion capacity of the lungs during follow-up (Ng et al., 2004; Wang et al., 2005; Xie et al., 2005). However, the risk of confounding by indication is high. Another cohort study investigated autopsy findings of 8 SARS patients with ARDS. They found that all patients with hydrocortisone had myofiber atrophy, whereas this was absent in patients who were not treated with steroids (Leung et al., 2005). However, critical illness neuropathy could not be distinguished from steroid myopathy in this study.
3. MERS-CoV: One retrospective cohort study included MERS patients who were admitted to the ICU. Steroid use was associated with a higher 90-day mortality in a multivariable logistic regression model. However, in a Cox proportional hazard model, steroid use was not associated with a higher mortality rate (Arabi et al., 2018). This suggests that their statistical models did not adjust for all confounders. Another retrospective cohort study found that steroid use was associated with a higher mortality in hospitalized MERS patients (Alfaraj et al., 2019). It was not possible to assess the risk of bias in this study, because essential information is missing, such as baseline characteristics.
Effect of Steroid Dose
1. SARS-CoV-2: Two retrospective cohort studies found that a higher steroid dose was associated with death and prolonged time until viral clearance (Li S. et al., 2020), while use of low-dose steroids was not (Li S. et al., 2020; Li X. et al., 2020). Another retrospective cohort study found that each 10 mg increase in steroid dose was associated with a 4% increase in the risk of mortality after 28 days in COVID-19 patients with ARDS or sepsis (Lu et al., 2020). However, the steroid dose tended to be the highest in the patients who were most severely ill. Thus, there is significant risk of confounding by indication in these studies.
2. SARS-CoV: In one retrospective cohort study, a lower mean steroid dose was associated with a higher 28-day mortality and mechanical ventilation rate (Gomersall et al., 2004). Contrarily, another retrospective cohort study found that a higher mean steroid dose was associated with a higher mortality rate (Wei et al., 2009). Two other retrospective cohort studies found no association between steroid dose and clinical outcome (Ho et al., 2003; Hui et al., 2005). The first study found that the rate of ICU admission, mechanical ventilation or mortality after 3 weeks were not significantly different for patients treated with high-dose or low-dose steroids (Ho et al., 2003). The other study reports that steroid dose was not associated with the results of the 6-minute walking test or the severity of radiographic abnormalities at 6 months after SARS (Hui et al., 2005). In all of these studies, the risk of confounding (by indication) is high.
3. MERS-CoV: No studies available.
Timing of Steroid Administration
1. SARS-CoV-2: No studies available.
2. SARS-CoV: Two observational studies investigated whether the timing of steroid administration was associated with clinical outcomes in patients with SARS (Zhao et al., 2003; Jang et al., 2004). The first study reports that the time between onset of symptoms and start of steroids was not associated with the risk of ICU admission or mechanical ventilation (Jang et al., 2004). The second study found that early treatment with high-dose methylprednisolone might be beneficial for SARS patients (Zhao et al., 2003). In this study, treatment with methylprednisolone was started at different moments in the course of the disease: (A) if patients had not recovered after 14 days, (B) if symptoms or radiological abnormalities worsened, or (C) if fever persisted for ≥ 3 days after admission. The rate of mechanical ventilation was 7, 33, and 0%, respectively, and the mortality rate was 6, 12, and 0%, respectively. However, patients were not randomized to different treatment protocols, and consequently, baseline characteristics and disease severity were different. In both studies, the risk of confounding is high.
3. MERS-CoV: No studies available.
Calcineurin Inhibitors (CNI): Cyclosporin A (CsA) and Tacrolimus (TAC)
CNIs (cyclosporine A (CsA) and tacrolimus (TAC)) are used to prevent rejection after organ transplantation and to treat autoimmune diseases, like inflammatory bowel disease. CsA and TAC inhibit T-cell activation. In-vivo, CsA and TAC form complexes with cyclophilins and FK506-binding proteins, respectively. These complexes prevent the phosphatase activity of calcineurin. As a result, the dephosphorylation of the nuclear factor of activated T cells is decreased (Carbajo-Lozoya et al., 2012; Ma et al., 2016).
In addition, cyclophilins, the binding proteins of CsA, catalyze the cis/trans isomerization of propyl peptide bonds. This is an essential step in correct folding of proteins, such as cellular and viral proteins (Ma-Lauer et al., 2020). This function of cyclophilin A is found to be essential for the replication of SARS-CoV-2 and other viruses belonging to the Nidovirales order (Carbajo-Lozoya et al., 2014).
Viral Load
1. SARS-CoV-2: No studies available.
2. SARS-CoV and MERS-CoV: Several in-vitro studies showed that CsA significantly inhibits the viral replication and the cytopathic effect (CPE: the virus-induced changes in host cells that cause cell death) of SARS-CoV and MERS-CoV in infected cells (Vero, Huh7, Calu-3, and human lung tissue) in a dose-dependent manner (de Wilde et al., 2011; Pfefferle et al., 2011; Carbajo-Lozoya et al., 2012; de Wilde et al., 2013; Li et al., 2018; Sauerhering et al., 2020). One of these studies found that a high concentration of CsA (15 µM) completely inhibited the CPE, without affecting the viability of the cells (de Wilde et al., 2013). Next to these in-vitro effects, CsA also inhibited MERS-CoV viral replication and reduced cellular apoptosis in ex-vivo cultures of bronchial and lung tissue (Li et al., 2018).
Similar to CsA, TAC inhibited the viral replication of SARS-CoV in Vero cells in a dose-dependent manner (Carbajo-Lozoya et al., 2012). In this study, high-dose TAC reduced SARS-CoV titers 11.112-fold after only 24 h (Carbajo-Lozoya et al., 2012).
Clinical Outcome
No studies matching the inclusion criteria.
Antimetabolites
Mycophenolic Acid (MPA)
Mycophenolic acid (MPA) and its prodrugs, mycophenolate mofetil (MMF) and mycophenolate sodium, are used in the treatment of autoimmune diseases and to prevent rejection in organ transplant recipients. MPA inhibits inosine-5’-monophosphate dehydrogenase, which leads to depletion of intracellular guanosine and deoxyguanosine nucleotides. This suppresses DNA synthesis and thus proliferation of T and B lymphocytes (Villarroel et al., 2009).
Viral Load
1. SARS-CoV-2: One in-vitro study found that MPA inhibits SARS-CoV-2 replication in VeroE6/TMPRSS2 cells (Kato et al., 2020). In another study (Han et al., 2020), human pluripotent stem cells (hPSC) were differentiated into lung organoids and then infected with SARS-CoV-2. In these lung organoids, MPA inhibited viral replication while the CPE of SARS-CoV-2 was still observed, even with high concentrations of MPA.
2. SARS-CoV: MPA does not inhibit the proteolytic activity of SARS-CoV PLpro (Cheng et al., 2015) or SARS-CoV replication in Vero cells (Barnard et al., 2006).
3. MERS-CoV: Two studies showed that MPA effectively inhibits the proteolytic activity of the papain-like protease (PLpro) of MERS-CoV (Cheng et al., 2015; Lin et al., 2018). PLpro is responsible for the cleavage of nonstructural proteins, which are essential for viral maturation. Three other in-vitro studies showed that MPA significantly inhibited the replication and CPE of MERS-CoV in Vero cells (Chan et al., 2013; Hart et al., 2014; Shen et al., 2019). This effect was dose-dependent.
In contrast, an in-vivo study in marmosets infected with MERS-CoV found that the mean viral load in the lungs was higher in MMF-treated animals than in controls (Chan et al., 2015). However, since MERS-CoV does not cause lethal disease in marmosets, this animal model does not adequately resemble human MERS (Johnson et al., 2015).
Clinical Outcome
1. SARS-CoV-2: No studies available.
2. SARS-CoV: No studies available.
3. MERS-CoV: In a retrospective cohort study of 51 hospitalized patients with MERS, eight patients (16%) had received MMF as experimental treatment for MERS. Overall, 19 (37%) patients were admitted to the ICU and eventually died. All other patients survived. In univariate analysis, MMF treatment was associated with survival. However, MMF was given to the less severely ill patients and, seven of the eight MMF-treated patients (87.5%) were also treated with interferon beta (Al Ghamdi et al., 2016). Thus, there is significant risk of confounding.
Thiopurine Analogues
Thiopurine analogues (azathioprine (AZA) and 6-mercaptopurine (6MP)) are used as anticancer treatment, to prevent rejection in organ transplant recipients and as treatment of several chronic autoimmune diseases. AZA is a prodrug of 6MP. In-vivo, 6MP is converted into 6-thioguanine (6TG) which is incorporated into cellular DNA. This prevents further DNA replication (Chen et al., 2009).
Viral Load
1. SARS-CoV-2: No studies available.
2. SARS-CoV and MERS-CoV: 6MP and 6TG effectively inhibit the proteolytic activity of PLpro of MERS-CoV and SARS-CoV in a dose-dependent manner (IC50 of 12 to 27 µM) in inhibition assays with peptide and fluorogenic substrates (Chou et al., 2008; Cheng et al., 2015; Lin et al., 2018). These results suggest that 6MP and 6TG can inhibit the replication of MERS-CoV and SARS-CoV in-vitro.
Clinical Outcome
No studies matching the inclusion criteria.
Mammalian Target of Rapamycin (mTOR) Inhibitors
Sirolimus (rapamycin) and everolimus are mTOR inhibitors. These drugs are used to prevent acute rejection in organ transplantation (Pfefferle et al., 2011). In higher doses, everolimus is also used as anticancer drug (Pfefferle et al., 2011). In-vivo, sirolimus and everolimus bind to the FK-binding protein 12 (FKBP-12). This sirolimus/everolimus-FKBP-12 complex binds to mTOR, a phosphatidylinositol kinase-related kinase. This inhibits protein synthesis, cell cycle progression and cell growth (Wullschleger et al., 2006).
Viral Load
1. SARS-CoV-2: No studies available.
2. SARS-CoV: No studies available.
3. MERS-CoV: One in-vitro study showed that sirolimus and everolimus dose-dependently reduce MERS-CoV infection in a hepatocyte derived cell line. For both drugs, the cytotoxicity was < 10% (Kindrachuk et al., 2015).
Clinical Outcome
No studies matching the inclusion criteria.
Anti-Cytokine Agents
Anti-Tumor-Necrosis-Factor-α (TNF- α) Agents
There are several types of anti-tumor-necrosis-factor-α (TNF-α) agents: infliximab, adalimumab and golimumab (monoclonal antibodies against TNF-α), certolizumab (TNF-α binding fragment of a monoclonal antibody) and etanercept (fusion protein composed of the extracellular portion of the TNF-receptor-2 and the Fc portion of immunoglobulin G1). Anti-TNF- α agents are used in the treatment of autoimmune diseases, like rheumatoid arthritis and inflammatory bowel disease. TNF-α is a pro-inflammatory cytokine that recruits neutrophils and monocytes to the area of inflammation and activates intracellular signaling in various cells of the immune system (Mitoma et al., 2018).
Viral Replication
No studies matching the inclusion criteria.
Clinical Outcome
1. SARS-CoV-2: No studies available.
2. SARS-CoV: In an animal study, mice infected with SARS-CoV were treated with an anti-TNF-α monoclonal antibody or an isotype-matched control antibody. In mice treated with the anti-TNF-α monoclonal antibody, the onset of weight loss and respiratory illness was delayed compared to controls. However, the mortality rate after 10 days was similar in both groups (Nagata et al., 2008).
3. MERS-CoV: No studies available.
Anakinra
Anakinra is an IL-1 receptor antagonist that is registered for the treatment of several autoinflammatory diseases, such as adult-onset Stills disease and familial Mediterranean fever (Cavalli et al., 2020).
Viral Replication
No studies matching the inclusion criteria.
Clinical Outcome
1. SARS-CoV-2: A retrospective cohort study (Cavalli et al., 2020) included patients with COVID-19 with moderate-to-severe ARDS and hyperinflammation (CRP ≥ 100 mg/L and/or ferritin ≥ 900 ng/mL). Patients treated with mechanical ventilation or other anti-inflammatory agents were excluded. Patients in the intervention group (n = 29) received high-dose anakinra (5mg/kg twice daily intravenously). The control group (n = 16) consisted of patients who retrospectively met the eligibility criteria for anakinra, but who presented to the hospital before the availability of the drug. The 3-week mortality rate was lower in anakinra-treated patients compared to controls (HR = 0.20, 95% CI 0.04–0.63, p = 0.009), but more anakinra-treated patients than controls still required mechanical ventilation after 3 weeks (17 vs. 6%). The percentage of patients that was discharged and had resumed normal activities after 3 weeks was not significantly different (45 vs. 44%). A limitation of this study is the significant risk of confounding. For example, there were significant differences between patients treated with anakinra and controls.
2. SARS-CoV: No studies available.
3. MERS-CoV: No studies available.
Tocilizumab and other IL-6 Inhibitors
IL-6 is an important pro-inflammatory cytokine that is involved in the acute phase response and differentiation and function of B and T cells (Mosharmovahed et al., 2020). Of the three commercially available IL-6 inhibitors (tocilizumab, sarilumab and siltuximab), tocilizumab is the most well-known (Khiali et al., 2020). Tocilizumab is a recombinant humanized anti-IL-6 receptor monoclonal antibody. Tocilizumab is used to treat several autoinflammatory diseases, like rheumatoid arthritis, giant cell arteritis and the chimeric antigen receptor T-cell induced cytokine storm syndrome (Khiali et al., 2020). Notably, Il-6 inhibitors were not available at the time of the SARS and MERS pandemics. The nine retrospective cohort studies matching the inclusion criteria are summarized in Table 3.
Viral Replication
No studies matching the inclusion criteria.
Clinical Outcome
1. SARS-Cov-2: The nine studies matching the inclusion criteria report conflicting results. One retrospective cohort study found that treatment of COVID-19 patients with tocilizumab was associated with a higher mortality rate and higher prevalence of bacterial infection (Quartuccio et al., 2020). However, tocilizumab-treated patients were more severely ill than controls. They were also significantly older and more frequently received antivirals and glucocorticoids than controls.
In four other studies, the mortality and ICU admission rate were not significantly different between patients treated with an IL-6-inhibitor (tocilizumab or sarilumab) and controls (Campochiaro et al., 2020; Colaneri et al., 2020; Della-Torre et al., 2020; Rojas-Marte et al., 2020). Similarly, the discharge rate (Campochiaro et al., 2020), duration of hospitalization (Campochiaro et al., 2020), blood stream infections (Giacobbe et al., 2020), serious adverse event rate (including infections and bacteremia) (Campochiaro et al., 2020) and rate of clinical improvement after 28 days (Della-Torre et al., 2020) were not significantly different. In three of these studies (Colaneri et al., 2020; Della-Torre et al., 2020; Rojas-Marte et al., 2020), the controls were matched to the tocilizumab-treated patients on several important patient characteristics in order to reduce the risk of confounding by indication. In the fourth study (Campochiaro et al., 2020), the patient characteristics and disease severity at admission were not significantly different between both groups and the eligibility criteria for tocilizumab were clearly defined. Moreover, controls were recruited among patients who fulfilled these eligibility criteria before and after the time period of tocilizumab availability.
Lastly, three other studies report a higher 3-week survival rate (Capra et al., 2020), a lower rate of mechanical ventilation (Klopfenstein et al., 2020) and a lower rate of ICU admission (Moreno-García et al., 2020) in patients treated with tocilizumab. Remarkably, in two of these studies, the tocilizumab-treated patients were more critically ill at admission than controls, but their outcome was better.
In summary, one study found that tocilizumab was associated with a higher mortality rate, but this study has a high risk of confounding. Four observational studies found no effect of tocilizumab or sarilumab and four other studies showed a beneficial effect of tocilizumab.
2. SARS-CoV: No studies available.
3. MERS-CoV: No studies available.
Immunosuppressive Drugs Without Studies Matching Eligibility Criteria
We could not identify any studies matching our inclusion criteria for the following drugs: abatacept, alemtuzumab, basiliximab, belatacept, brodalumab, cyclophosphamide, dupilumab, eculizumab, ixekizumab, methotrexate, rituximab, and secukinumab.
Discussion
There are 30 studies meeting the inclusion criteria for our review that studied the effect of immunosuppressive drugs on infection with SARS-CoV-2. These studies investigated the effects of corticosteroids, mycophenolic acid (MPA), anakinra or tocilizumab.
Interestingly, MPA inhibits SARS-CoV-2 replication in-vitro (Han et al., 2020; Kato et al., 2020). This is in contrast with COVID-19 guidelines aimed at patients using immunosuppressive drugs for a pre-existing disease (Lopez et al., 2020; British Transplantation Society, 2020; Société Francophone de Transplantation (SFT) SFdN et al., 2020). These expert-opinion-based guidelines recommend to discontinue MPA or reduce the MPA dose in patients with COVID-19. Based on the results of our review, this approach can be questioned. Clinical studies are needed to confirm the inhibitory effect of MPA on SARS-CoV-2 replication in-vivo.
The results of some clinical studies suggest that corticosteroids are beneficial in patients with COVID-19, especially in mitigating the effects of the cytokine storm. An RCT found that dexamethasone use was associated with a lower 28-day mortality rate, a shorter length of hospital stay and a lower prevalence of mechanical ventilation (Recovery Collaborative Group et al., 2020). The beneficial effect of dexamethasone was greatest in the most severely ill patients. For example, in patients treated with mechanical ventilation, dexamethasone reduced the risk of mortality with 36% (95% CI 19–49%), whereas dexamethasone had no effect on the mortality rate of patients who did not require oxygen support. The results of this RCT support our hypothesis that immunosuppressive drugs can be used to prevent and treat the hyperinflammatory phase of COVID-19.
Six cohort studies confirmed that steroid use was associated with a better clinical outcome in patients with COVID-19 (Fadel et al., 2020; Fernandez Cruz et al., 2020; Lu et al., 2020; Sanz Herrero et al., 2020; Wang et al., 2020; Wu et al., 2020). However, two cohort studies have opposite results (Giacobbe et al., 2020; Mo et al., 2020). They found that steroid use was associated with a higher risk of blood stream infections (Giacobbe et al., 2020) and a lower 10-day remission rate (Mo et al., 2020). Unfortunately, all cohort studies have a high risk of confounding (by indication), which limits their validity and generalizability. Thus, more high-quality studies are needed to confirm the effect of steroids on the clinical outcome of COVID-19.
Moreover, it has to be established whether corticosteroids have an effect on the host immune response against the virus. An adequate response of the immune system in the first phase of COVID-19 can prevent progression to viral pneumonia (Shi et al., 2020). As expected, there were no studies that found that corticosteroid use was associated with accelerated SARS-CoV-2 clearance. However, some cohort studies found that steroid use was associated with a delay in SARS-CoV-2 clearance (Chen et al., 2020; Ling et al., 2020; Xu et al., 2020), while other studies report that steroid use was not associated with SARS-CoV-2 clearance time (Fang et al., 2020; Xu et al., 2020; Yuan et al., 2020; Zha et al., 2020; Zheng et al., 2020). These studies have a high risk of confounding (by indication). Thus, we could not draw firm conclusions on the effect of corticosteroids on viral replication.
There is growing interest in IL-6 inhibitors, like tocilizumab. Patients with severe COVID-19 have high levels of IL-6 (Mosharmovahed et al., 2020) and in three cohort studies, tocilizumab use was associated with lower mortality and ICU admission rate (Capra et al., 2020; Klopfenstein et al., 2020; Moreno-García et al., 2020). However, other cohort studies report contradictory results (Campochiaro et al., 2020; Colaneri et al., 2020; Della-Torre et al., 2020; Giacobbe et al., 2020; Quartuccio et al., 2020; Rojas-Marte et al., 2020). Furthermore, the risk of confounding in these observational studies is high. As a result, it is not clear yet whether IL-6 inhibitors can be used to prevent and treat the cytokine storm. Fortunately, several research groups have published a protocol for a RCT to assess the efficacy and safety of tocilizumab as treatment of COVID-19 (Cellina et al., 2020; Fu et al., 2020; Rilinger et al., 2020).
The observational studies for corticosteroids and IL-6 inhibitors report contradictory results, which is probably related to heterogeneity in study populations. In addition, there could have been differences in local treatment protocols. These factors limit the possibility of conducting a meta-analysis. Furthermore, in many studies there are differences in patient characteristics (such as age, gender and comorbidity) and treatment regimens between patients in the intervention group and control group. Moreover, in many cases, the most severely ill patients were treated with immunosuppressive drugs, whereas less severely ill patients were not. This might have resulted in confounding by indication.
Currently, the number of studies on the effect of immunosuppressive drugs on COVID-19 is still limited. SARS and MERS are also caused by a coronavirus and share many features with COVID-19 (Wang D. et al., 2004; Yin and Wunderink, 2018; Wang D. et al., 2020). Thus, the effects of immunosuppressive drugs on these viruses may resemble the effects on SARS-CoV-2. We were able to include 39 additional studies that studied the effect of immunosuppressive drugs on infection with SARS-CoV and MERS-CoV. CNIs and mTOR inhibitors inhibit the viral replication of SARS-CoV and MERS-CoV in-vitro. There is also indirect evidence that thiopurine analogues inhibit the viral replication of SARS-CoV. It would therefore be interesting to investigate the effect of CNIs, mTOR inhibitors and thiopurine analogues on the viral replication of COVID-19.
Notably, there are some important differences between SARS-CoV-2, SARS-CoV and MERS-CoV. First, the nucleotide sequence of the genome of SARS-CoV-2 is only 79.7% similar to SARS-CoV (Zhou et al., 2020). However, the similarity of the nucleotide sequence of envelope, nucleocapsid and spike proteins is as high as 96, 90, and 77%, respectively (Zhou et al., 2020). The similarity of the genome of SARS-CoV-2 and MERS-CoV is only 54%, and for the spike protein, a transmembrane protein involved in binding of the virus to the host receptor, 31.9% (Zhou et al., 2020). Second, MERS-CoV uses dipeptidyl peptidase 4 (DPP4) as host cell receptor (Raj et al., 2013), while SARS-CoV-2 and SARS-CoV use ACE2 (Li et al., 2003; Hoffmann et al., 2020). Lastly, the case fatality rate of MERS-CoV is much higher than of COVID-19 and SARS. While interpreting the results of this review, these differences should be kept in mind.
Our study has some limitations. First, we did not fully meet all the requirements of a systematic review, since we have only searched one database and there was no second reviewer. Second, we only included “genuine” immunosuppressive drugs in our review. As a consequence, we excluded antiviral/antiparasitic drugs, like (hydroxy)chloroquine, despite the fact that some would argue that this drug has immunosuppressive properties.
Conclusions
Some immunosuppressive drugs may be beneficial in the treatment of COVID-19. MPA inhibits SARS-CoV-2 replication in-vitro. There are indications that corticosteroids and IL-6 inhibitors, like tocilizumab, can reduce mortality and prevent mechanical ventilation in patients with COVID-19. These results have to be confirmed in high-quality clinical trials before these drugs can be implemented as standard care. Based on the positive results of CNIs, mTOR inhibitors and thiopurine analogues in in-vitro studies with SARS-CoV and MERS-CoV, it would be interesting to investigate their effects on SARS-CoV-2 replication.
Author Contributions
RM: idea for the review. All authors: research design. TS: PubMed search, data extraction, writing first draft of the manuscript. All authors contributed to the article and approved the submitted version.
Conflict of Interest
The authors declare that the research was conducted in the absence of any commercial or financial relationships that could be construed as a potential conflict of interest.
Supplementary Material
The Supplementary Material for this article can be found online at: https://www.frontiersin.org/articles/10.3389/fphar.2020.01333/full#supplementary-material
References
Al Ghamdi, M., Alghamdi, K. M., Ghandoora, Y., Alzahrani, A., Salah, F., Alsulami, A., et al. (2016). Treatment outcomes for patients with Middle Eastern Respiratory Syndrome Coronavirus (MERS CoV) infection at a coronavirus referral center in the Kingdom of Saudi Arabia. BMC Infect. Dis. 16, 174. doi: 10.1186/s12879-016-1492-4
Alfaraj, S. H., Al-Tawfiq, J. A., Assiri, A. Y., Alzahrani, N. A., Alanazi, A. A., Memish, Z. A. (2019). Clinical predictors of mortality of Middle East Respiratory Syndrome Coronavirus (MERS-CoV) infection: A cohort study. Travel Med. Infect. Dis. 29, 48–50. doi: 10.1016/j.tmaid.2019.03.004
Arabi, Y. M., Mandourah, Y., Al-Hameed, F., Sindi, A. A., Almekhlafi, G. A., Hussein, M. A., et al. (2018). Corticosteroid Therapy for Critically Ill Patients with Middle East Respiratory Syndrome. Am. J. Respir. Crit. Care Med. 197 (6), 757–767. doi: 10.1164/rccm.201706-1172OC
Auyeung, T. W., Lee, J. S., Lai, W. K., Choi, C. H., Lee, H. K., Lee, J. S., et al. (2005). The use of corticosteroid as treatment in SARS was associated with adverse outcomes: a retrospective cohort study. J. Infect. 51 (2), 98–102. doi: 10.1016/j.jinf.2004.09.008
Barnard, D. L., Day, C. W., Bailey, K., Heiner, M., Montgomery, R., Lauridsen, L., et al. (2006). Enhancement of the infectivity of SARS-CoV in BALB/c mice by IMP dehydrogenase inhibitors, including ribavirin. Antiviral Res. 71 (1), 53–63. doi: 10.1016/j.antiviral.2006.03.001
British Transplantation Society (2020). Guidance on the management of transplant recipients diagnosed with or suspected of having COVID19. Version 25 March, Available at: https://bts.org.uk/wp-content/uploads/2020/03/Clinical_management_transplant_recipients.pdf.
Campochiaro, C., Della-Torre, E., Cavalli, G., De Luca, G., Ripa, M., Boffini, N., et al. (2020). Efficacy and safety of tocilizumab in severe COVID-19 patients: a single-centre retrospective cohort study. Eur. J. Int. Med. 76, 43–49. doi: 10.1016/j.ejim.2020.05.021
Cao, J., Tu, W. J., Cheng, W., Yu, L., Liu, Y. K., Hu, X., et al. (2020). Clinical Features and Short-term Outcomes of 102 Patients with Corona Virus Disease 2019 in Wuhan, China. Clin. Infect. Dis. 71 (15), 748–755. doi: 10.1093/cid/ciaa243
Capra, R., De Rossi, N., Mattioli, F., Romanelli, G., Scarpazza, C., Sormani, M. P., et al. (2020). Impact of low dose tocilizumab on mortality rate in patients with COVID-19 related pneumonia. Eur. J. Int. Med. 76, 31–35. doi: 10.1016/j.ejim.2020.05.009
Carbajo-Lozoya, J., Muller, M. A., Kallies, S., Thiel, V., Drosten, C., von Brunn, A. (2012). Replication of human coronaviruses SARS-CoV, HCoV-NL63 and HCoV-229E is inhibited by the drug FK506. Virus Res. 165 (1), 112–117. doi: 10.1016/j.virusres.2012.02.002
Carbajo-Lozoya, J., Ma-Lauer, Y., Malesevic, M., Theuerkorn, M., Kahlert, V., Prell, E., et al. (2014). Human coronavirus NL63 replication is cyclophilin A-dependent and inhibited by non-immunosuppressive cyclosporine A-derivatives including Alisporivir. Virus Res. 184, 44–53. doi: 10.1016/j.virusres.2014.02.010
Cavalli, G., De Luca, G., Campochiaro, C., Della-Torre, E., Ripa, M., Canetti, D., et al. (2020). Interleukin-1 blockade with high-dose anakinra in patients with COVID-19, acute respiratory distress syndrome, and hyperinflammation: a retrospective cohort study. Lancet Rheumatol. 2 (6), e325–e331. doi: 10.1016/S2665-9913(20)30127-2
Cellina, M., Orsi, M., Bombaci, F., Sala, M., Marino, P., Oliva, G. (2020). Favorable changes of CT findings in a patient with COVID-19 pneumonia after treatment with tocilizumab. Diagn. Interv. Imaging 101 (5), 323–324. doi: 10.1016/j.diii.2020.03.010
Chan, J. F., Chan, K. H., Kao, R. Y., To, K. K., Zheng, B. J., Li, C. P., et al. (2013). Broad-spectrum antivirals for the emerging Middle East respiratory syndrome coronavirus. J. Infect. 67 (6), 606–616. doi: 10.1016/j.jinf.2013.09.029
Chan, J. F., Yao, Y., Yeung, M. L., Deng, W., Bao, L., Jia, L., et al. (2015). Treatment With Lopinavir/Ritonavir or Interferon-beta1b Improves Outcome of MERS-CoV Infection in a Nonhuman Primate Model of Common Marmoset. J. Infect. Dis. 212 (12), 1904–1913. doi: 10.1093/infdis/jiv392
Chen, C. Y., Lee, C. H., Liu, C. Y., Wang, J. H., Wang, L. M., Perng, R. P. (2005). Clinical features and outcomes of severe acute respiratory syndrome and predictive factors for acute respiratory distress syndrome. J. Chin. Med. Assoc. 68 (1), 4–10. doi: 10.1016/S1726-4901(09)70124-8
Chen, R. C., Tang, X. P., Tan, S. Y., Liang, B. L., Wan, Z. Y., Fang, J. Q., et al. (2006). Treatment of severe acute respiratory syndrome with glucosteroids: the Guangzhou experience. Chest 129 (6), 1441–1452. doi: 10.1378/chest.129.6.1441
Chen, X., Chou, C. Y., Chang, G. G. (2009). Thiopurine analogue inhibitors of severe acute respiratory syndrome-coronavirus papain-like protease, a deubiquitinating and deISGylating enzyme. Antivir. Chem. Chemother. 19 (4), 151–156. doi: 10.1177/095632020901900402
Chen, X., Zhu, B., Hong, W., Zeng, J., He, X., Chen, J., et al. (2020). Associations of Clinical Characteristics and Treatment Regimens with Viral RNA Shedding Duration in Patients with COVID-19. Int. J. Infect. Dis. 98, 252–260. doi: 10.1016/j.ijid.2020.06.091
Cheng, K. W., Cheng, S. C., Chen, W. Y., Lin, M. H., Chuang, S. J., Cheng, I. H., et al. (2015). Thiopurine analogs and mycophenolic acid synergistically inhibit the papain-like protease of Middle East respiratory syndrome coronavirus. Antiviral Res. 115, 9–16. doi: 10.1016/j.antiviral.2014.12.011
Chou, C. Y., Chien, C. H., Han, Y. S., Prebanda, M. T., Hsieh, H. P., Turk, B., et al. (2008). Thiopurine analogues inhibit papain-like protease of severe acute respiratory syndrome coronavirus. Biochem. Pharmacol. 75 (8), 1601–1609. doi: 10.1016/j.bcp.2008.01.005
Colaneri, M., Bogliolo, L., Valsecchi, P., Sacchi, P., Zuccaro, V., Brandolino, F., et al. (2020). Tocilizumab for Treatment of Severe COVID-19 Patients: Preliminary Results from SMAtteo COvid19 REgistry (SMACORE). Microorganisms 8 (5), 695. doi: 10.3390/microorganisms8050695
de Wilde, A. H., Zevenhoven-Dobbe, J. C., van der Meer, Y., Thiel, V., Narayanan, K., Makino, S., et al. (2011). Cyclosporin A inhibits the replication of diverse coronaviruses. J. Gen. Virol. 92 (Pt 11), 2542–2548. doi: 10.1099/vir.0.034983-0
de Wilde, A. H., Raj, V. S., Oudshoorn, D., Bestebroer, T. M., van Nieuwkoop, S., Limpens, R., et al. (2013). MERS-coronavirus replication induces severe in vitro cytopathology and is strongly inhibited by cyclosporin A or interferon-alpha treatment. J. Gen. Virol. 94 (Pt 8), 1749–1760. doi: 10.1099/vir.0.052910-0
de Wit, E., van Doremalen, N., Falzarano, D., Munster, V. J. (2016). SARS and MERS: recent insights into emerging coronaviruses. Nat. Rev. Microbiol. 14 (8), 523–534. doi: 10.1038/nrmicro.2016.81
Della-Torre, E., Campochiaro, C., Cavalli, G., De Luca, G., Napolitano, A., La Marca, S., et al. (2020). Interleukin-6 blockade with sarilumab in severe COVID-19 pneumonia with systemic hyperinflammation: an open-label cohort study. Ann. Rheum. Dis. doi: 10.1136/annrheumdis-2020-218122
Du, Y., Tu, L., Zhu, P., Mu, M., Wang, R., Yang, P., et al. (2020). Clinical Features of 85 Fatal Cases of COVID-19 from Wuhan: A Retrospective Observational Study. Am. J. Respir. Crit. Care Med. 201 (11), 1372–1379. doi: 10.2139/ssrn.3546088
Fadel, R., Morrison, A. R., Vahia, A., Smith, Z. R., Chaudhry, Z., Bhargava, P., et al. (2020). Early Short Course Corticosteroids in Hospitalized Patients with COVID-19. Clin. Infect. Dis. ciaa601. doi: 10.1093/cid/ciaa601
Fang, X., Mei, Q., Yang, T., et al. (2020). Low-dose corticosteroid therapy does not delay viral clearance in patients with COVID-19. J. Infect. doi: 10.1016/j.jinf.2020.03.039
Fernandez Cruz, A., Ruiz-Antoran, B., Munoz Gomez, A., Sancho Lopez, A., Mills Sanchez, P., Centeno Soto, G. A., et al. (2020). Impact of Glucocorticoid Treatment in Sars-Cov-2 Infection Mortality: A Retrospective Controlled Cohort Study. Antimicrob. Agents Chemother. AAC.01168-20. doi: 10.1128/AAC.01168-20
Fu, B., Xu, X., Wei, H. (2020). Why tocilizumab could be an effective treatment for severe COVID-19? J. Transl. Med. 18 (1), 164. doi: 10.1186/s12967-020-02339-3
Giacobbe, D. R., Battaglini, D., Ball, L., Brunetti, I., Bruzzone, B., Codda, G., et al. (2020). Bloodstream infections in critically ill patients with COVID-19. Eur. J. Clin. Invest. e13319. doi: 10.1111/eci.13319
Gomersall, C. D., Joynt, G. M., Lam, P., Li, T., Yap, F., Lam, D., et al. (2004). Short-term outcome of critically ill patients with severe acute respiratory syndrome. Intens. Care Med. 30 (3), 381–387. doi: 10.1007/s00134-003-2143-y
Han, Y., Yang, L., Duan, X., Duan, F., Nilsson-Payant, B. E., Yaron, T. M., et al. (2020). Identification of Candidate COVID-19 Therapeutics using hPSC-derived Lung Organoids. bioRxiv. 2020.05.05.079095. doi: 10.1101/2020.05.05.079095
Hart, B. J., Dyall, J., Postnikova, E., Zhou, H., Kindrachuk, J., Johnson, R. F., et al. (2014). Interferon-beta and mycophenolic acid are potent inhibitors of Middle East respiratory syndrome coronavirus in cell-based assays. J. Gen. Virol. 95 (Pt 3), 571–577. doi: 10.1099/vir.0.061911-0
Ho, J. C., Ooi, G. C., Mok, T. Y., Chan, J. W., Hung, I., Lam, B., et al. (2003). High-dose pulse versus nonpulse corticosteroid regimens in severe acute respiratory syndrome. Am. J. Respir. Crit. Care Med. 168 (12), 1449–1456. doi: 10.1164/rccm.200306-766OC
Hoffmann, M., Kleine-Weber, H., Schroeder, S., Kruger, N., Herrler, T., Erichsen, S., et al. (2020). SARS-CoV-2 Cell Entry Depends on ACE2 and TMPRSS2 and Is Blocked by a Clinically Proven Protease Inhibitor. Cell 181 (2), 271–280. doi: 10.1016/j.cell.2020.02.052
Huang, C., Wang, Y., Li, X. (2020). Clinical features of patients infected with 2019 novel coronavirus in Wuhan, China (vol 395, pg 497, 2020). Lancet 395 (10223), 496–496. doi: 10.1016/S0140-6736(20)30183-5
Hui, D. S., Joynt, G. M., Wong, K. T., Gomersall, C. D., Li, T. S., Antonio, G., et al. (2005). Impact of severe acute respiratory syndrome (SARS) on pulmonary function, functional capacity and quality of life in a cohort of survivors. Thorax 60 (5), 401–409. doi: 10.1136/thx.2004.030205
Hui, D. S., Lee, N., Chan, P. K., Beigel, J. H. (2018). The role of adjuvant immunomodulatory agents for treatment of severe influenza. Antiviral Res. 150, 202–216. doi: 10.1016/j.antiviral.2018.01.002
Jang, T. N., Yeh, D. Y., Shen, S. H., Huang, C. H., Jiang, J. S., Kao, S. J. (2004). Severe acute respiratory syndrome in Taiwan: analysis of epidemiological characteristics in 29 cases. J. Infect. 48 (1), 23–31. doi: 10.1016/j.jinf.2003.09.004
Johnson, R. F., Via, L. E., Kumar, M. R., Cornish, J. P., Yellayi, S., Huzella, L., et al. (2015). Intratracheal exposure of common marmosets to MERS-CoV Jordan-n3/2012 or MERS-CoV EMC/2012 isolates does not result in lethal disease. Virology 485, 422–430. doi: 10.1016/j.virol.2015.07.013
Kato, F., Matsuyama, S., Kawase, M., Hishiki, T., Katoh, H., Takeda, M. (2020). Antiviral activities of mycophenolic acid and IMD-0354 against SARS-CoV-2. Microbiol. Immunol. doi: 10.1111/1348-0421.12828
Khiali, S., Khani, E., Entezari-Maleki, T. (2020). A Comprehensive Review on Tocilizumab in COVID-19 Acute Respiratory Distress Syndrome. J. Clin. Pharmacol. 10.1002/jcph.1693. doi: 10.1002/jcph.1693
Kindrachuk, J., Ork, B., Hart, B. J., Mazur, S., Holbrook, M. R., Frieman, M. B., et al. (2015). Antiviral potential of ERK/MAPK and PI3K/AKT/mTOR signaling modulation for Middle East respiratory syndrome coronavirus infection as identified by temporal kinome analysis. Antimicrob. Agents Chemother. 59 (2), 1088–1099. doi: 10.1128/AAC.03659-14
Klopfenstein, T., Zayet, S., Lohse, A., Balblanc, J. C., Badie, J., Royer, P. Y., et al. (2020). Tocilizumab therapy reduced intensive care unit admissions and/or mortality in COVID-19 patients. Med. Mal. Infect. 50 (5), 397–400. doi: 10.1016/j.medmal.2020.05.001
Lansbury, L., Rodrigo, C., Leonardi-Bee, J., Nguyen-Van-Tam, J., Lim, W. S. (2019). Corticosteroids as adjunctive therapy in the treatment of influenza. Cochrane Database Syst. Rev. 2, CD010406. doi: 10.1002/14651858.CD010406.pub3
Lee, N., Allen Chan, K. C., Hui, D. S., Ng, E. K., Wu, A., Chiu, R. W., et al. (2004). Effects of early corticosteroid treatment on plasma SARS-associated Coronavirus RNA concentrations in adult patients. J. Clin. Virol. 31 (4), 304–309. doi: 10.1016/j.jcv.2004.07.006
Leung, T. W., Wong, K. S., Hui, A. C., To, K. F., Lai, S. T., Ng, W. F., et al. (2005). Myopathic changes associated with severe acute respiratory syndrome: a postmortem case series. Arch. Neurol. 62 (7), 1113–1117. doi: 10.1001/archneur.62.7.1113
Li, W., Moore, M. J., Vasilieva, N., Sui, J., Wong, S. K., Berne, M. A., et al. (2003). Angiotensin-converting enzyme 2 is a functional receptor for the SARS coronavirus. Nature 426 (6965), 450–454. doi: 10.1038/nature02145
Li, H. S., Kuok, D. I. T., Cheung, M. C., Ng, M. M. T., Ng, K. C., Hui, K. P. Y., et al. (2018). Effect of interferon alpha and cyclosporine treatment separately and in combination on Middle East Respiratory Syndrome Coronavirus (MERS-CoV) replication in a human in-vitro and ex-vivo culture model. Antiviral Res. 155, 89–96. doi: 10.1016/j.antiviral.2018.05.007
Li, S., Hu, Z., Song, X. (2020). High-dose but not low-dose corticosteroids potentially delay viral shedding of patients with COVID-19. Clin. Infect. Dis. doi: 10.1093/cid/ciaa829
Li, X., Xu, S., Yu, M., Wang, K., Tao, Y., Zhou, Y., et al. (2020). Risk factors for severity and mortality in adult COVID-19 inpatients in Wuhan. J. Allergy Clin. Immunol. 146 (1), 110–118. doi: 10.1016/j.jaci.2020.04.006
Lin, M. H., Moses, D. C., Hsieh, C. H., Cheng, S. C., Chen, Y. H., Sun, C. Y., et al. (2018). Disulfiram can inhibit MERS and SARS coronavirus papain-like proteases via different modes. Antiviral Res. 150, 155–163. doi: 10.1016/j.antiviral.2017.12.015
Ling, Y., Xu, S. B., Lin, Y. X., Tian, D., Zhu, Z. Q., Dai, F. H., et al. (2020). Persistence and clearance of viral RNA in 2019 novel coronavirus disease rehabilitation patients. Chin. Med. J. (Engl.). 133 (9), 1039–1043. doi: 10.1097/CM9.0000000000000774
Lopez, V., Vazquez, T., Alonso-Titos, J., Cabello, M., Alonso, A., Beneyto, I., et al. (2020). Recommendations on management of the SARS-CoV-2 coronavirus pandemic (Covid-19) in kidney transplant patients. Nefrologia. 40 (3), 265–271. doi: 10.1016/j.nefro.2020.03.002
Lu, X., Chen, T., Wang, Y., Wang, J., Yan, F. (2020). Adjuvant corticosteroid therapy for critically ill patients with COVID-19. Crit. Care 24 (1), 241. doi: 10.1186/s13054-020-02964-w
Ma, C., Li, F., Musharrafieh, R. G., Wang, J. (2016). Discovery of cyclosporine A and its analogs as broad-spectrum anti-influenza drugs with a high in vitro genetic barrier of drug resistance. Antiviral Res. 133, 62–72. doi: 10.1016/j.antiviral.2016.07.019
Ma-Lauer, Y., Zheng, Y., Malesevic, M., von Brunn, B., Fischer, G., von Brunn, A. (2020). Influences of cyclosporin A and non-immunosuppressive derivatives on cellular cyclophilins and viral nucleocapsid protein during human coronavirus 229E replication. Antiviral Res. 173, 104620. doi: 10.1016/j.antiviral.2019.104620
Mazzulli, T., Farcas, G. A., Poutanen, S. M., Willey, B. M., Low, D. E., Butany, J., et al. (2004). Severe acute respiratory syndrome-associated coronavirus in lung tissue. Emerg. Infect. Dis. 10 (1), 20–24. doi: 10.3201/eid1001.030404
Mehta, P., McAuley, D. F., Brown, M., Sanchez, E., Tattersall, R. S., Manson, J. J., et al. (2020). COVID-19: consider cytokine storm syndromes and immunosuppression. Lancet 395 (10229), 1033–1034. doi: 10.1016/S0140-6736(20)30628-0
Mitoma, H., Horiuchi, T., Tsukamoto, H., Ueda, N. (2018). Molecular mechanisms of action of anti-TNF-alpha agents - Comparison among therapeutic TNF-alpha antagonists. Cytokine 101, 56–63. doi: 10.1016/j.cyto.2016.08.014
Mo, P., Xing, Y., Xiao, Y., Deng, L., Zhao, Q., Wang, H., et al. (2020). Clinical characteristics of refractory COVID-19 pneumonia in Wuhan, China. Clin. Infect. Dis. ciaa270. doi: 10.1093/cid/ciaa270
Moreno-García, E., Rico, V., Albiach, L., Aguero, D., Ambrosioni, J., Bodro, M., et al. (2020). Tocilizumab is associated with reduced risk of ICU admission and mortality in patients with SARS-CoV-2 infection. MedRxiv Preprint. doi: 10.1101/2020.06.05.20113738
Mosharmovahed, B., Fatahi, Y., Mohebbi, B., Ghorbanian, S. A., Assadiasl, S. (2020). Tocilizumab in transplantation. Eur. J. Clin. Pharmacol. 76 (6), 765–773. doi: 10.1007/s00228-020-02864-6
Nagata, N., Iwata, N., Hasegawa, H., Fukushi, S., Harashima, A., Sato, Y., et al. (2008). Mouse-passaged severe acute respiratory syndrome-associated coronavirus leads to lethal pulmonary edema and diffuse alveolar damage in adult but not young mice. Am. J. Pathol. 172 (6), 1625–1637. doi: 10.2353/ajpath.2008.071060
Ng, C. K., Chan, J. W., Kwan, T. L., To, T. S., Chan, Y. H., Ng, F. Y., et al. (2004). Six month radiological and physiological outcomes in severe acute respiratory syndrome (SARS) survivors. Thorax 59 (10), 889–891. doi: 10.1136/thx.2004.023762
Pfefferle, S., Schopf, J., Kogl, M., Friedel, C. C., Muller, M. A., Carbajo-Lozoya, J., et al. (2011). The SARS-coronavirus-host interactome: identification of cyclophilins as target for pan-coronavirus inhibitors. PloS Pathog. 7 (10), e1002331. doi: 10.1371/journal.ppat.1002331
Quartuccio, L., Sonaglia, A., McGonagle, D., Fabris, M., Peghin, M., Pecori, D., et al. (2020). Profiling COVID-19 pneumonia progressing into the cytokine storm syndrome: Results from a single Italian Centre study on tocilizumab versus standard of care. J. Clin. Virol. 129, 104444. doi: 10.1016/j.jcv.2020.104444
Raj, V. S., Mou, H., Smits, S. L., Dekkers, D. H., Muller, M. A., Dijkman, R., et al. (2013). Dipeptidyl peptidase 4 is a functional receptor for the emerging human coronavirus-EMC. Nature 495 (7440), 251–254. doi: 10.1038/nature12005
Recovery Collaborative Group, Horby, P., Lim, W. S., Emberson, J. R., Mafham, M., Bell, J. L., et al. (2020). Dexamethasone in Hospitalized Patients with Covid-19 - Preliminary Report. N. Engl. J. Med. doi: 10.1056/NEJMoa2021436
Rilinger, J., Kern, W. V., Duerschmied, D., Supady, A., Bode, C., Staudacher, D. L., et al. (2020). A prospective, randomised, double blind placebo-controlled trial to evaluate the efficacy and safety of tocilizumab in patients with severe COVID-19 pneumonia (TOC-COVID): A structured summary of a study protocol for a randomised controlled trial. Trials 21 (1), 470. doi: 10.1186/s13063-020-04447-3
Rojas-Marte, G. R., Khalid, M., Mukhtar, O., Hashmi, A. T., Waheed, M. A, Ehrlich, S., et al. (2020). Outcomes in Patients with Severe COVID-19 Disease Treated with Tocilizumab - A Case- Controlled Study. QJM 113 (8), 546–550. doi: 10.1093/qjmed/hcaa206
Ruan, Q., Yang, K., Wang, W., Jiang, L., Song, J. (2020). Clinical predictors of mortality due to COVID-19 based on an analysis of data of 150 patients from Wuhan, China. Intens. Care Med. 46 (5), 846–848. doi: 10.1007/s00134-020-05991-x
Sanz Herrero, F., Puchades Gimeno, F., Ortega Garcia, P., Ferrer Gomez, C., Ocete Mochon, M. D., Garcia Deltoro, M. (2020). Methylprednisolone added to tocilizumab reduces mortality in SARS-CoV-2 pneumonia: An observational study. J. Int. Med. doi: 10.1111/joim.13145
Sauerhering, L., Kupke, A., Meier, L., Dietzel, E., Hoppe, J., Gruber, A. D., et al. (2020). Cyclophilin Inhibitors Restrict Middle East Respiratory Syndrome Coronavirus Via Interferon lambda In Vitro And In Mice. Eur. Respir. J. 1901826. doi: 10.1183/13993003.01826-2019
Shen, L., Niu, J., Wang, C., Huang, B., Wang, W., Zhu, N., et al. (2019). High-Throughput Screening and Identification of Potent Broad-Spectrum Inhibitors of Coronaviruses. J. Virol. 93 (12). doi: 10.1128/JVI.00023-19
Shi, Y., Wang, Y., Shao, C., Huang, J., Gan, J., Huang, X., et al. (2020). COVID-19 infection: the perspectives on immune responses. Cell Death Differ. 27 (5), 1451–1454. doi: 10.1038/s41418-020-0530-3
Siddiqi, H. K., Mehra, M. R. (2020). COVID-19 Illness in Native and Immunosuppressed States: A Clinical-Therapeutic Staging Proposal. J. Heart Lung Transplant. 39 (5), 405–407. doi: 10.1016/j.healun.2020.03.012
Société Francophone de Transplantation (SFT) SFdN, Dialyse et Transplantation (SFNDT) and Groupe Infection et Immunodépression, Société de pathologie infectieuse de langue française (SPILF) (2020). Prise en charge de l’infection due au SARS-CoV-2 chez les patients adultes transplantés d’organe solide. Version 2 april, URL: https://www.sfndt.org/sites/www.sfndt.org/files/medias/documents/Recos%20COVID19%20guide%20pratique%20SFT%20SFNDT%20SPILF%2003%2004%2020.pdf.
Sung, J. J., Wu, A., Joynt, G. M., Yuen, K. Y., Lee, N., Chan, P. K., et al. (2004). Severe acute respiratory syndrome: report of treatment and outcome after a major outbreak. Thorax 59 (5), 414–420. doi: 10.1136/thx.2003.014076
Villarroel, M. C., Hidalgo, M., Jimeno, A. (2009). Mycophenolate mofetil: An update. Drugs Today (Barc) 45 (7), 521–532. doi: 10.1358/dot.2009.45.7.1384878
Wang, J. T., Sheng, W. H., Fang, C. T., Chen, Y. C., Wang, J. L., Yu, C. J., et al. (2004). Clinical manifestations, laboratory findings, and treatment outcomes of SARS patients. Emerg. Infect. Dis. 10 (5), 818–824. doi: 10.3201/eid1005.030640
Wang, C. H., Liu, C. Y., Wan, Y. L., Chou, C. L., Huang, K. H., Lin, H. C., et al. (2005). Persistence of lung inflammation and lung cytokines with high-resolution CT abnormalities during recovery from SARS. Respir. Res. 6, 42. doi: 10.1186/1465-9921-6-42
Wang, D., Hu, B., Hu, C., Zhu, F., Liu, X., Zhang, J., et al. (2020). Clinical Characteristics of 138 Hospitalized Patients With 2019 Novel Coronavirus-Infected Pneumonia in Wuhan, China. JAMA 323 (11), 1061–1069. doi: 10.1001/jama.2020.1585
Wang, Y., Jiang, W., He, Q., Wang, J. T., Sheng, W. H., Fang, Y. C, Chen, Y. C., Wang, J. L., Yu, C. J, et al. (2020). A retrospective cohort study of methylprednisolone therapy in severe patients with COVID-19 pneumonia. Signal Transduct. Target Ther. 5 (1), 57. doi: 10.1038/s41392-020-0158-2
Wei, M. T., de Vlas, S. J., Yang, Z., Borsboom, G. J., Wang, L., Li, H., et al. (2009). The SARS outbreak in a general hospital in Tianjin, China: clinical aspects and risk factors for disease outcome. Trop. Med. Int. Health 14 (Suppl 1), 60–70. doi: 10.1111/j.1365-3156.2009.02347.x
World Health Organization (WHO) (2020). Clinical management of severe acute respiratory infection (SARI) when COVID-19 disease is suspected: Interim guidance V 1.2, URL: https://www.who.int/publications-detail/clinical-management-of-severe-acute-respiratory-infection-when-novel-coronavirus-(ncov)-infection-is-suspected.
Wu, C., Chen, X., Cai, Y., Xia, J., Zhou, X., Xu, C., et al. (2020). Risk Factors Associated With Acute Respiratory Distress Syndrome and Death in Patients With Coronavirus Disease 2019 Pneumonia in Wuhan, China. JAMA Int. Med. 180 (7), 1–11. doi: 10.1001/jamainternmed.2020.0994
Wullschleger, S., Loewith, R., Hall, M. N. (2006). TOR signaling in growth and metabolism. Cell 124 (3), 471–484. doi: 10.1016/j.cell.2006.01.016
Xie, L., Liu, Y., Xiao, Y., Tian, Q., Fan, B., Zhao, H., et al. (2005). Follow-up study on pulmonary function and lung radiographic changes in rehabilitating severe acute respiratory syndrome patients after discharge. Chest 127 (6), 2119–2124. doi: 10.1378/chest.127.6.2119
Xu, K., Chen, Y., Yuan, J., Yi, P., Ding, C., Wu, W., et al. (2020). Factors associated with prolonged viral RNA shedding in patients with COVID-19. Clin. Infect. Dis. 71 (15), 799–806. doi: 10.1093/cid/ciaa351
Yam, L. Y., Lau, A. C., Lai, F. Y., Shung, E., Chan, J., Wong, V., et al. (2007). Corticosteroid treatment of severe acute respiratory syndrome in Hong Kong. J. Infect. 54 (1), 28–39. doi: 10.1016/j.jinf.2006.01.005
Yin, Y. D., Wunderink, R. G. (2018). MERS, SARS and other coronaviruses as causes of pneumonia. Respirology 23 (2), 130–137. doi: 10.1111/resp.13196
Yuan, M., Xu, X., Xia, D., Tao, Z., Yin, W., Tan, W., et al. (2020). Effects of Corticosteroid Treatment for Non-Severe COVID-19 Pneumonia: A Propensity Score-Based Analysis. Shock. doi: 10.1097/SHK.0000000000001574
Zha, L., Li, S., Pan, L., Tefsen, B., Li, Y., French, N., et al. (2020). Corticosteroid treatment of patients with coronavirus disease 2019 (COVID-19). Med. J. Aust. 212 (9), 416–420. doi: 10.5694/mja2.50577
Zhang, W., Zhao, Y., Zhang, F., Wang, Q., Li, T., Liu, Z., et al. (2020). The use of anti-inflammatory drugs in the treatment of people with severe coronavirus disease 2019 (COVID-19): The Perspectives of clinical immunologists from China. Clin. Immunol. 214, 108393. doi: 10.1016/j.clim.2020.108393
Zhao, Z., Zhang, F., Xu, M., Huang, K., Zhong, W., Cai, W., et al. (2003). Description and clinical treatment of an early outbreak of severe acute respiratory syndrome (SARS) in Guangzhou, PR China. J. Med. Microbiol. 52 (Pt 8), 715–720. doi: 10.1099/jmm.0.05320-0
Zheng, C., Wang, J., Guo, H., Lu, Z., Ma, Y., Zhu, Y., et al. (2020). Risk-adapted Treatment Strategy For COVID-19 Patients. Int. J. Infect. Dis. 94, 74–77. doi: 10.1016/j.ijid.2020.03.047
Keywords: COVID-19, SARS-CoV-2, coronavirus, immunosuppressive drugs, corticosteroids, mycophenolic acid, calcineurin inhibitors, mTOR inhibitors
Citation: Schoot TS, Kerckhoffs APM, Hilbrands LB and van Marum RJ (2020) Immunosuppressive Drugs and COVID-19: A Review. Front. Pharmacol. 11:1333. doi: 10.3389/fphar.2020.01333
Received: 28 May 2020; Accepted: 11 August 2020;
Published: 28 August 2020.
Edited by:
Dieter Steinhilber, Goethe University Frankfurt, GermanyReviewed by:
Arpan Acharya, University of Nebraska Medical Center, United StatesPrasanta Kumar Dash, University of Nebraska Medical Center, United States
Copyright © 2020 Schoot, Kerckhoffs, Hilbrands and van Marum. This is an open-access article distributed under the terms of the Creative Commons Attribution License (CC BY). The use, distribution or reproduction in other forums is permitted, provided the original author(s) and the copyright owner(s) are credited and that the original publication in this journal is cited, in accordance with accepted academic practice. No use, distribution or reproduction is permitted which does not comply with these terms.
*Correspondence: Tessa S. Schoot, dC5zY2hvb3RAamJ6Lm5s