- 1Department of Medicine, VA Palo Alto Health Care System, Palo Alto, CA, United States
- 2Department of Medicine, Stanford University School of Medicine, Stanford, CA, United States
Leukotrienes are biologically active eicosanoid lipid mediators that originate from oxidative metabolism of arachidonic acid. Biosynthesis of leukotrienes involves a set of soluble and membrane-bound enzymes that constitute a machinery complex primarily expressed by cells of myeloid origin. Leukotrienes and their synthetic enzymes are critical immune modulators for leukocyte migration. Increased concentrations of leukotrienes are implicated in a number of inflammatory disorders. More recent work indicates that leukotrienes may also interact with a variety of tissue cells, contributing to the low-grade inflammation of cardiovascular, neurodegenerative, and metabolic conditions, as well as that of cancer. Leukotriene signaling contributes to the active tumor microenvironment, promoting tumor growth and resistance to immunotherapy. This review summarizes recent insights into the intricate roles of leukotrienes in promoting tumor growth and metastasis through shaping the tumor microenvironment. The emerging possibilities for pharmacological targeting of leukotriene signaling in tumor metastasis are considered.
Introduction
Low-grade inflammation and dysregulated immune responses are components of the tumor microenvironment (TME), pivotal for tumor growth and response to immunotherapies (Binnewies et al., 2018). While therapies that target the immune system, such as checkpoint inhibition, have significantly improved cancer prognosis, not all cancer patients respond to immunomodulatory treatments. Additionally, some who respond initially may develop treatment resistance and autoimmunity (Wei et al., 2018). A better understanding of the biology of TME may improve the efficacy of immunotherapies and reduce the potential adverse side-effects.
Leukotrienes are proinflammatory lipid mediators that initiate inflammation and mount adaptive immune responses for host defense (Peters-Golden et al., 2005; Peters-Golden and Henderson, 2007). Activated leukotriene signaling is implicated in inflammatory manifestations of a variety of pathologies. Recent studies have demonstrated that leukotrienes also play crucial roles in shaping the tumor microenvironment. This review summarizes recent efforts in elucidating how leukotrienes modulate tumor pathophysiology and discuss possible means to harness leukotriene signaling pathways in cancer therapeutics.
Leukotriene Synthesis
There are two types of leukotrienes (LTs): the dihydroxy fatty acid leukotriene B4 (LTB4) and cysteinyl-leukotrienes (CysLTs), including the fatty acid-peptide conjugate LTC4 and its metabolites, LTD4 and LTE4. Leukotrienes are mainly produced by myeloid cells, including, macrophages/monocytes, neutrophils, eosinophils, and mast cells (Peters-Golden et al., 2005; Peters-Golden and Henderson, 2007).
Leukotrienes biosynthesis starts from the production of polyunsaturated arachidonic acid (AA) from membrane phospholipids by phospholipase A2 (PLA2)s, especially the cytosolic form, PLA2α (cPLA2α) (Haeggstrom and Funk, 2011). 5-lipoxygenase (5-LO) is the most critical enzyme for leukotrienes production, which requires a set of stimulatory factors for its full activation, including 5-LO–activating protein (FLAP) and coactosin-like protein (Wan et al., 2017). Arachidonic acid is subsequently converted into leukotrienes in a concerted three-step reaction: first, AA is dioxygenated into 5-hydroperoxy-6-trans-8,11,14-cis-eicosatetraenoic acid [5(S)-HpETE]; second, 5(S)-HpETE is dehydrated to yield the transient epoxide intermediate, LTA4; and lastly, depending on the presence and functional coupling of 5-LO to its downstream enzymes, LTA4 is further converted to LTB4 by LTA4 hydrolase (LTA4H), or LTC4 by LTC4 synthase (LTC4S), which conjugates LTA4 with glutathione (Peters-Golden and Henderson, 2007; Haeggstrom and Funk, 2011; Wan et al., 2017) (Figure 1). Unlike many other enzymes, the catalytic activity of 5-LO and its contribution to inflammatory responses depend on its subcellular compartmentalization, phosphorylation state, and proximity to other eicosanoid-forming enzymes (Peters-Golden and Brock, 2001; Luo et al., 2003; Radmark and Samuelsson, 2009). Nuclear localized 5-LO translocates to the inner nuclear envelope and endoplasmic reticulum (ER)/Golgi membrane to facilitate the biosynthesis of LTB4. 5-LO from the cytoplasm favors the production of CysLT and the anti-inflammatory eicosanoid lipid, such as lipoxin A4 (LXA4) (Peters-Golden and Brock, 2001; Luo et al., 2003; Mandal et al., 2008; Radmark and Samuelsson, 2009; Haeggstrom and Funk, 2011). Non-leukocyte cells generally do not contain the full spectrum of the synthetic enzymes of leukotrienes. However, these cells, including vascular endothelial cells, express LTA4H and may convert LTA4, generated by neighboring immune cells, to LTB4, a mechanism known as transcellular LT synthesis (Gijon et al., 2007; Sala et al., 2010).
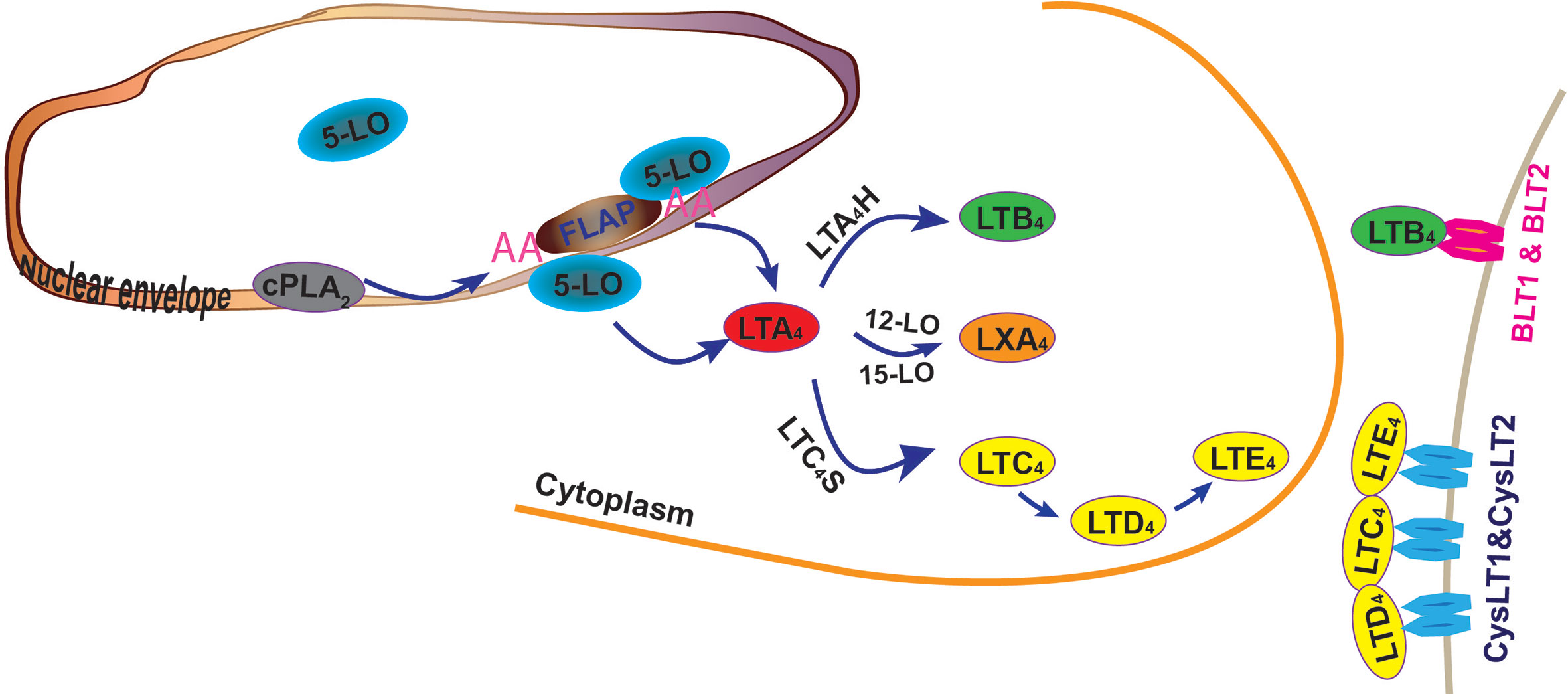
Figure 1 Biosynthesis of leukotrienes. Arachidonic acid (AA) is first generated by cytoplasmic phospholipase A2 (cPLA2) and converted into leukotriene A4 (LTA4) through the cooperative actions of 5-lipoxygenase (5-LO) and 5-LO–activating protein (FLAP). From the inner nuclear membrane, AA is metabolized into LTA4 by inner nuclear membrane-localized 5-LO and is further converted into LTB4 by LTA4 hydrolase (LTA4H). AA is also metabolized to LTA4 by 5-LO located on the outer nuclear membrane and converted to LTC4. LTC4S (LTC4 synthase) conjugates LTA4 with glutathione to form LTC4 and its metabolites, LTD4 and LTE4. (LTA4 may also be metabolized into lipoxin A4 [LXA4] by 12-LO or 15-LO.) Leukotrienes signal through two sets of G-protein coupled receptors, which are receptors for LTB4 (BLT1 and BLT2) and receptors for LTC4, LTD4, and LTE4 (CysLT1 and CysLT2).
Leukotrienes signal through two sets of G-protein coupled receptors (GPCRs), with BLT1 (high affinity) and BLT2 (low affinity) serving as receptors for LTB4, and CysLT1, CysLT2, and CysLTE (also known as gpr99) as receptors for CysLTs (Peters-Golden and Henderson, 2007; Nakamura and Shimizu, 2011). Leukotrienes act in a paracrine and cell type-dependent manner, exerting their functions at nanomolar concentrations (Peters-Golden and Henderson, 2007).
Leukotrienes and Inflammatory Disorders
LTB4 is noted to play essential roles in a variety of acute and chronic inflammatory diseases, including diabetes, obesity, Alzheimer’s disease, myocardial infarction, asthma, idiopathic lung fibrosis, chronic obstructive pulmonary disease (COPD), pulmonary arterial hypertension (PAH), lymphedema, and cancer (Back, 2009; Drakatos et al., 2009; Izumo et al., 2009; Wang and Dubois, 2010; Price et al., 2011; Tian et al., 2013; Li et al., 2015; Qian et al., 2015; Wculek and Malanchi, 2015; Tian et al., 2017; Michael et al., 2019; Tian et al., 2019). Mechanisms of actions of LTB4 in these conditions include chemotaxis for immune cell populations, facilitating endothelial adherence, and induction of blood and lymphatic vascular endothelial injury (Back, 2009; Drakatos et al., 2009; Izumo et al., 2009; Wang and Dubois, 2010; Price et al., 2011; Tian et al., 2013; Li et al., 2015; Qian et al., 2015; Wculek and Malanchi, 2015; Tian et al., 2017; Michael et al., 2019; Tian et al., 2019). LTB4 is one of the most powerful identified chemotactic molecules (Subramanian et al., 2017). LTB4 not only summons neutrophils, macrophages, mast cells, eosinophils, dendritic cells (DCs), T cells, and B cells to the site of tissue injury but also promotes the survival of these immune cells in lymphoid organs and peripheral tissues (Del Prete et al., 2007; Peters-Golden and Henderson, 2007; Subramanian et al., 2017). CysLTs is endowed with overlapping but distinct function in promoting inflammation responses when compared with LTB4. The classical properties of CysLTs in the vasculature, LTD4 in particular, are the regulation of smooth muscle contraction in the microcirculation and respiratory tract (Peters-Golden and Henderson, 2007; Araujo et al., 2018). CysLTs also induce pathological angiogenesis, maladaptive proliferative responses, expression of adhesion molecules (I-CAM-1 and VCAM-1), and loss of endothelial barrier function (Sjostrom et al., 2003; Uzonyi et al., 2006; Moos et al., 2008; Duah et al., 2013).
In the lung, 5-LO and LTB4 are elevated in the airways of asthma and COPD patients, as well as in the pulmonary arterioles of PAH patients, where the concentrations of LTB4 correlate with the severity of the disease. The upregulation of LTB4 and subsequent interaction with its receptors stimulate the migration of immune cells into the lungs (Drakatos et al., 2009; Tian et al., 2013). Specifically, LTB4 promotes the migration of immature and mature DCs along the gradient of CCL19 and CCL21 by increasing the membrane expression of CCR7 (Del Prete et al., 2007). LTB4 induces the rapid integrin-mediated arrest of rolling of effector and memory CD8+ cells and thereby mediates cytotoxic T cell trafficking (Goodarzi et al., 2003). Mast cells may secrete LTB4 and recruit T cells in response to tissue inflammation and infection, including allergy, asthma, and rheumatoid arthritis (Goodarzi et al., 2003). Mast cells and macrophages promote the migration of neutrophils in the presence of LTB4. Notably, 5-LO and LTB4 also interact with a number of pulmonary proinflammatory signaling molecules, including NF-κB, TNF-α, MAPK, and IL-6/STAT3, suggesting that 5-LO/LTB4 may further amplify the proinflammatory circuits. As an example, mice lacking 5-LO or BLT1 are impaired in TLR (toll-like receptor)-mediated NF-κB activation in pulmonary macrophages (Serezani et al., 2011).
In addition to being immunomodulatory in the lung, recent efforts also suggest that 5-LO and LTB4 exert key roles in the pulmonary vasculature: LTB4 promotes the growth and activation of pulmonary arterial smooth muscle cell (SMC) and adventitial fibroblasts. 5-LO and LTB4 also induce early death of pulmonary arterial endothelial cells (ECs), cause the survival cells to become apoptosis-resistant and proliferative, change the properties of these ECs to acquire endogenous 5-LO expression, and transform them into proinflammatory phenotypes; responses are implicated in the PAH pathogenesis (Tian et al., 2013; Qian et al., 2015; Tian et al., 2019). Prominent expression of LTB4 in the brain, adrenals, heart, adipose tissues, skin, as well as, in lymph fluid suggests an additional, less well-recognized function of this eicosanoid lipid. Emerging evidence indicates a pivotal role of LTB4 in insulin resistance and hepatic steatosis, with LTB4 directly enhancing macrophage chemotaxis, reducing insulin-stimulated glucose uptake in myocytes, and inhibiting insulin-mediated suppression of hepatic glucose output (Li et al., 2015). LTB4 induces the migration and proliferation of coronary artery SMCs, and BLT1 is highly expressed in the human carotid artery atherosclerotic plaques (Back, 2009). Expression of 5-LO is significantly elevated in post-mortem brain tissues of neurodegenerative diseases; also, blocking 5-LO is beneficial to aged transgenic mice with pre-existing behavioral abnormality and tau neuropathology (Tian et al., 2017; Giannopoulos et al., 2019). 5-LO activation and increased LTB4 concentrations are found in animal model and human lymphedema (Tian et al., 2017). LTB4, at low physiological concentrations, is required for lymphangiogenesis and wound healing (Tian et al., 2017; Ramalho et al., 2018). Conversely, LTB4, in high pathological concentrations, interferes with the protective VEGFR3 and Notch signaling in lymphatic endothelial cells, causing lymphatic vascular damage, abnormal lymphatic drainage, and lymphedema (Tian et al., 2017). The pivotal roles of LTB4 signaling in lymphedema are further supported in two Phase II clinical studies, where pharmacological blockage of LTB4 signaling is beneficial in restoring a failing lymphatic circulation (Rockson et al., 2018).
Cysteinyl leukotriene production has been directly linked to the pathogenesis of asthma (Peters-Golden and Henderson, 2007; Price et al., 2011). Extensive evidence shows that CysLTs regulate most of the key features of asthma, including airway SMC constriction, increased microvascular permeability, compromised respiratory cilia activities, airway remodeling, bronchial hyperresponsiveness, and maladaptive survival of immune cells (Price et al., 2011). Importantly, three selective antagonists of CysLT receptors (i.e., pranlukast, montelukast, zafirlukast) and the inhibitor of 5-LO (zileuton, zyfloR) have been used in the clinical management of asthma for nearly 20 years (Price et al., 2011). These drugs are well-tolerated and efficient in restricting bronchoconstrictor challenge mediated by allergen and exercise. CysLTs are also key mediators in allergic rhinitis, acting on nasal vascular ECs, enhancing DC-stimulated antigen presentation, activating interstitial cells (eosinophils, mast cells, macrophages, and neutrophils), and modulating nasal allergic inflammation and clinical symptoms (Sousa et al., 2002).
Inflammation in Cancer
Chronic inflammation is a significant risk factor for the development of cancer (Coussens and Werb, 2002). Immune cells, orchestrating with cancer cells and surrounding stromal cells, form an inflammatory TME (Wang et al., 2017). Cells within the TME are highly plastic. Inflammation may shape the TME towards a more malignant state and direct tumor-promoting signals (Figure 2). Recent transcriptomic and metabolic studies of the TME indicate enhanced expression of inflammatory cytokines and chemokines in primary tumors and metastatic lesions, which is correlated with an increased number of inflammatory infiltrates and poor clinical prognosis (Galon et al., 2006; Zhang et al., 2020). Neutralization or genetic silencing of inflammatory signaling in preclinical models diminishes tumor growth and progression. As an example, colitis-associated cancer is associated with IL-23 producing myeloid cells and IL-23-dependent TME (McGovern and Powrie, 2007). This review focuses on discussing the tumor-promoting functionality of inflammation.
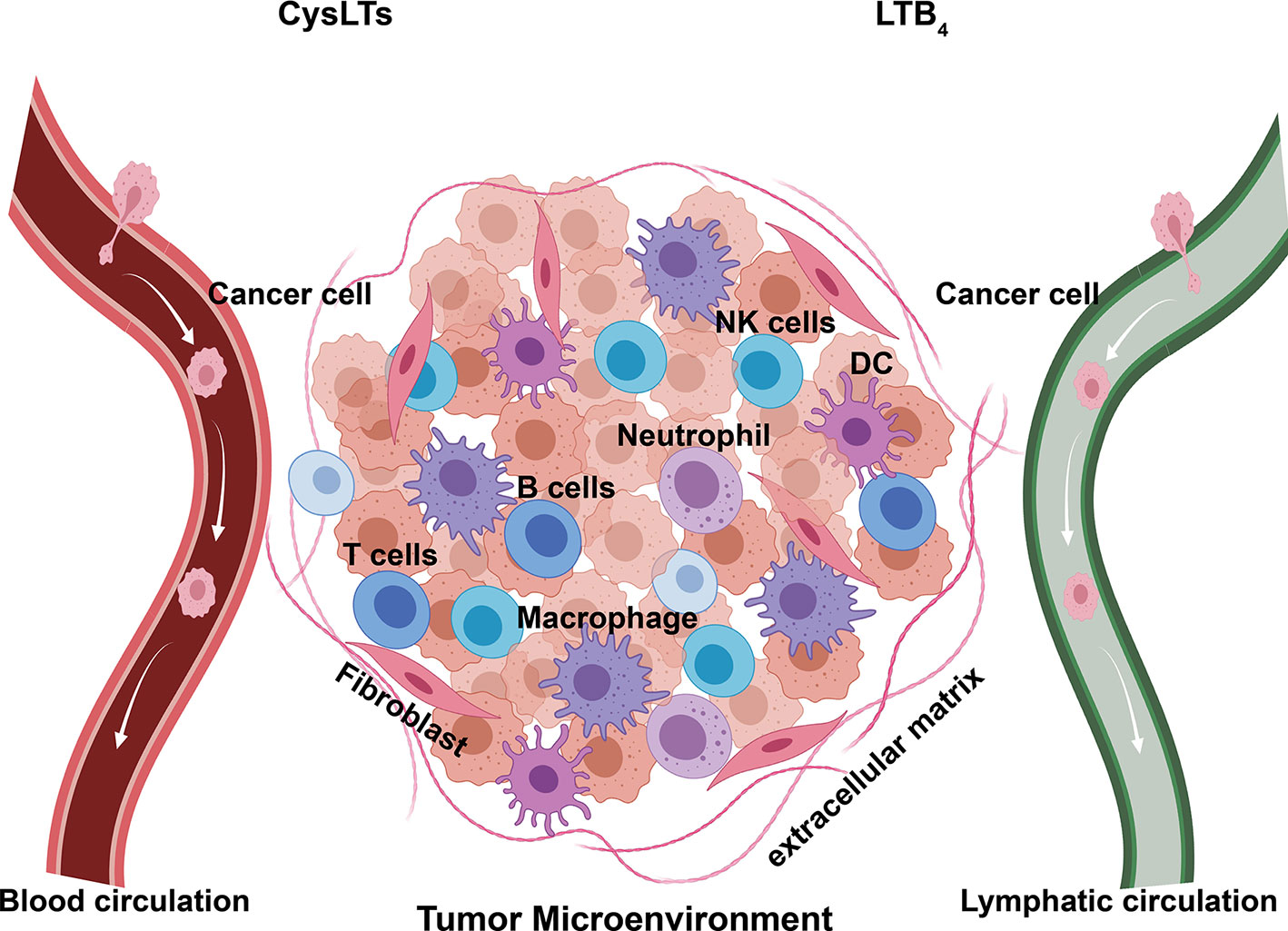
Figure 2 Process of cancer metastasis. 1) Genetic risks and environmental factors (e.g., inflammation) cause epithelial cell transform into cancer cell phenotype. 2) Cancer cells and cancer stem cells proliferate to generate primary tumor. 3) The carcinoma cells recruit a variety of stromal cells and immune cells to form tumor microenvironment (TME). 4) Cancer cells, under the influence of TME, acquire an invasive phenotype through epithelial-to-mesenchymal-transition (EMT) and intravasate into blood vascular or lymphatic circulation. 5) The malignant cancer cells exit the circulation and develop into secondary tumor at the distant organs.
Inflammation and Tumor Initiation
Over 15% of cancers are predisposed by infection, chronic inflammation, and autoimmunity (Abu-Shakra et al., 2001; Greten and Grivennikov, 2019; Valencia et al., 2019). The most prominent examples include inflammatory bowel disease that may risk patients for colon cancer, and chronic hepatitis increases the likelihood of developing liver cancer (Uko et al., 2012). A variety of proinflammatory environmental cues may prompt cancer development, including inhalation of fine particles and tobacco smoke. Obesity, hyperglycemia, and excessive lipid accumulation promote low-grade inflammation and increase the risk of liver, pancreatic, colon, breast cancer, and other malignancies (Abu-Shakra et al., 2001; Pietrzyk et al., 2015; Greten and Grivennikov, 2019; Valencia et al., 2019). Type II diabetes is increasingly recognized as a risk factor for cancer, promoting tumorigenesis through obesity-induced inflammation and obesity-related tissue injury (Giovannucci et al., 2010).
Two interdependent events are postulated to be required for tumor initiation: 1) genetic and epigenetic alterations of tumor-suppressive pathways and oncogenic signaling, and 2) generation and growth of transformed cell clones. Inflammation, driven by macrophages and neutrophils, potently produces reactive oxygen species, which may induce gene variants (Mittal et al., 2014). Inflammation may cause germline and somatic mutations in Tp53 and other cancer-related genes and increase the tumor mutational burden (Werner et al., 2020). IL-22, IL-6, TNF-α, and IL-1β not only cause DNA damage but also activate epigenetic machinery in epithelial cells (Grivennikov et al., 2010). Inflammatory responses may trigger the de-differentiation of epithelial cells into tumor-initiating stem cell-like cells (Sainz et al., 2016; Ayob and Ramasamy, 2018). Notably, NF-κB and IL-6/STAT3 signaling increase the survival and proliferation of the transformed cells, so-called inflammation-driven cell survival (Grivennikov et al., 2010; Chaturvedi et al., 2011; Kumari et al., 2016). Evident in liver and skin cancers, inflammation-induced cell death is required for the growth of neighboring transformed tumor cells (i.e., autophagy) (Yun and Lee, 2018). While inflammation in early-stage tumors is localized, systemic inflammation prevails during the late stage of tumor invasion and magnify the cancer sequela, as exemplified by tobacco-smoke and obesity activating neutrophils to promote breast cancer metastasis into the lungs (Walser et al., 2008; Yu et al., 2018).
Inflammation and Tumor Progression
In a fashion similar to tumor initiation, inflammation provides direct growth signaling for tumor proliferation (Coussens and Werb, 2002). Additionally, inflammatory mediators may induce tumor cell plasticity within the TME by antagonizing potential anti-tumor immunity, stimulating angiogenesis, and recruiting fibroblasts and other stromal cells to support tumor metastasis. Furthermore, inflammatory molecules modify stromal and tumor cells metabolism and tissue stiffness by regulating the formation of extracellular matrix (Chaturvedi et al., 2011). For instance, IL-6, IL-17, and IL-11 may increase the proliferation of tumor cells, under conditions, such as chronic hypoxia, lack of nutrients, or insufficiency of anti-tumor immunity (Muz et al., 2015; Kumari et al., 2016; Zhong et al., 2016; McGeachy et al., 2019). IL-8 has been shown to recruit macrophages and neutrophils to the TME and to stimulate the angiogenic responses of vascular ECs in a paracrine manner. IL-8 may also induce carcinoma cells to acquire a mesenchymal-like phenotype (i.e., epithelial-to-mesenchymal transition, EMT) (Waugh and Wilson, 2008).
Inflammation and Tumor Metastasis
Majority of tumor-related death is due to cancer metastasis. Growing number of studies support the role of inflammation in cancer mortality (Coussens and Werb, 2002). The migration of primary cancer cells away from the epithelium into the neighboring tissues depends on EMT (Brabletz et al., 2018; Pastushenko and Blanpain, 2019). EMT enhances the mobility of cancer cells and allows them to break from the basal membrane and enter into lymphatic and blood circulation for further dissemination (Brabletz et al., 2018; Pastushenko and Blanpain, 2019). Cancer stem cells adapt transcriptional and functional similarities to mesenchymal cells in motility (Brabletz et al., 2018; Pastushenko and Blanpain, 2019). TNF-α and IL-1β directly induce the expression of key transcription factors for EMT, SLUG, SNAIL, and Twist (Brabletz et al., 2018; Pastushenko and Blanpain, 2019). IL-11 is associated with the recruitment of fibroblasts, supporting tumor invasion, immune escape, and selection of malignant cancerous cells; increased IL-11 expression is correlated with worse clinical prognosis (Zhong et al., 2016). IL-17 activates neutrophils and drives breast cancer metastasis by facilitating the formation of a pre-metastatic niche (Gu et al., 2015). Inflammatory signals also prompt the expression of tissue-specific adhesion molecules and integrins, thereby aid the generation of tropism of metastasis (Bendas and Borsig, 2012). In malignant tissue, tumor-associated macrophages (TAMs) are the most abundant cell types of TME; these polarized macrophages mediate tumor growth and angiogenesis, secrete pro-tumor signaling molecules, and suppressing anti-tumor adaptive immune responses (Noy and Pollard, 2014; Che et al., 2017).
Therapy-Induced Inflammation in Cancer
Chemotherapy, radiotherapy, and surgical interventions may generate inflammatory responses as well. Damage-associated molecular patterns (DAMPs) released from dying tumor cells may regulate the synthesis of IL-1β and other cytokines; DAMPs also induce and sustain de novo anti-tumor T cell responses (Hernandez et al., 2016). In contrast, dying tumor cells can stimulate the production of TNF-α, epidermal growth factor (EGF), IL-6, and Wnt ligands, which in turn recruit myeloid cells and fibroblasts to the local TME, serving as anti-cell death signals and decreasing the efficacy of anti-tumor therapies (Labi and Erlacher, 2015). For example, paracrine EGFs, which are secreted from macrophages or fibroblasts, are major factors causing cancer therapy resistance (Srivatsa et al., 2017).
Leukotrienes in Cancer
Elevated 5-LO and leukotriene signaling is reported in various forms of cancers, including cancer of the pancreas, colon, stomach, prostate, ovaries, and lungs (Wang and Dubois, 2010; Jala et al., 2017). These proinflammatory mediators may modulate the initiation, progression, and metastasis of tumors through regulating the proliferation, apoptosis, migration, and invasion of cancer cells. 5-LO and leukotriene signaling may also be capable of shaping the TME through inducing the migration and activation of immune cells, production of growth factors, secretion of proinflammatory mediators and angiogenic factors; they may also directly interact with blood and lymphatic endothelium and influence the migration of cancer cells (Figure 3). The remainder of this review discusses the specific links between 5-LO and leukotriene-mediated signaling in cancer by summarizing and speculating the roles of 5-LO and leukotriene in promoting tumorigenesis and tumor microenvironment.
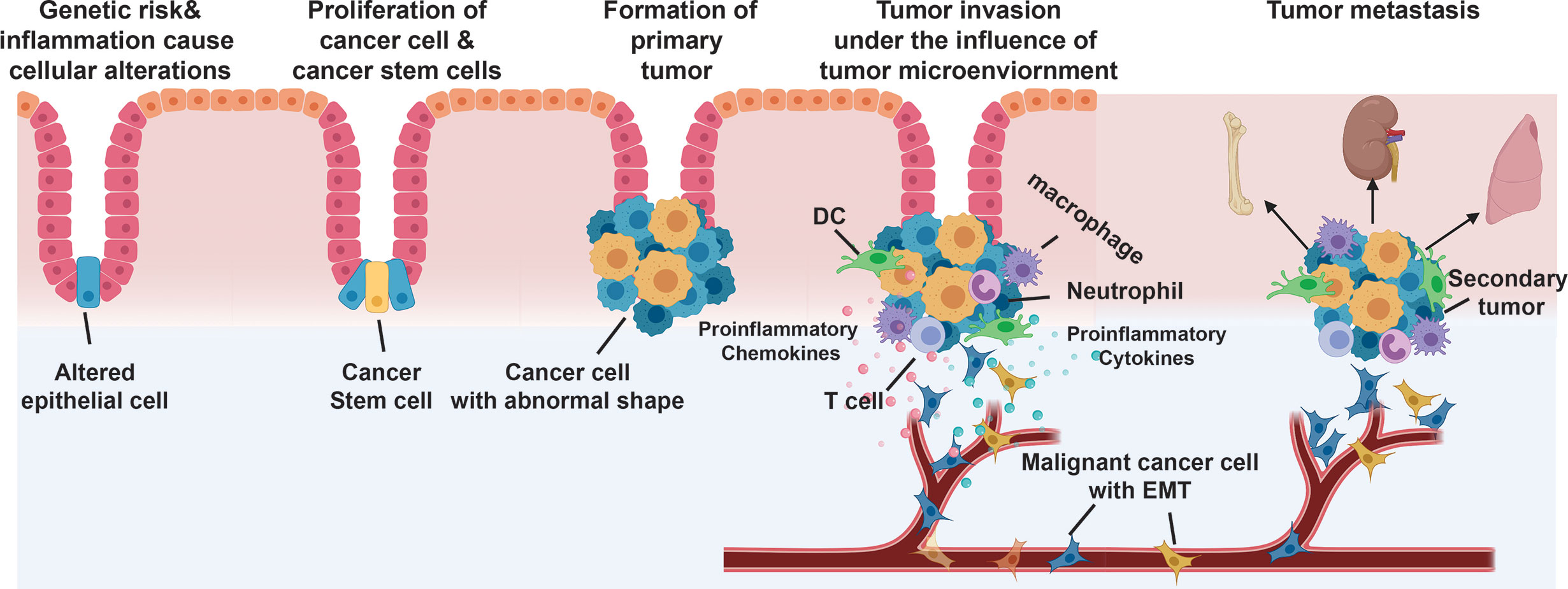
Figure 3 Components of tumor microenvironment (TME). Established primary tumor consists of a wide array of cellular infiltrates, including immune cells of both innate and adaptive immunity. These cells secrete proinflammatory cytokines, chemokines, and leukotrienes, as well as form a complex regulatory network that fosters tumor metastasis by creating an environment enabling cancer to evade immune surveillance and destruction. DC, dendritic cells; NK cells, natural killing cells; CysLTs, LTC4, LTD4, and LTE4.
5-LO and Leukotriene Signaling and Cell Growth
Western/Northern blotting and histology are not able to detect 5-LO expression in healthy pulmonary ECs (Walker et al., 2002; Zhang et al., 2002; Porter et al., 2014). However, antagonizing 5-LO signaling arrests ECs from dividing in culture and chronic hypoxia induces abnormal EC proliferation in a 5-LO-dependent fashion, implicating that 5-LO expression is required for EC mitosis (Walker et al., 2002; Porter et al., 2014). Additionally, 5-LO is expressed in the nuclei of a number of cancer stem cells; 5-LO causes ECs lack of BMPR2 signaling to transform into a cancer stem cell-like phenotype, and targeting 5-LO suppresses the adverse growth responses (Romano et al., 2001; Chen et al., 2009a; Chen et al., 2009b; Roos et al., 2014; Tian et al., 2019). The mechanisms by which 5-LO signaling regulate cell proliferation is not understood but is postulated to be related to the nuclear localization of this eicosanoid enzyme. Consistently, inhibition of LTA4H impedes the growth of colon cancer cells, suggesting that LTB4 is essential for cancer cell growth (Jeong et al., 2009; Lin et al., 2016; Zhao et al., 2019). LTB4 directly stimulates colon cancer cell growth and survival through a BLT1/ERK-dependent pathway in vitro; it induces the proliferation of human pancreatic cancer cells through MAPK/ERK and PI3K/Akt-dependent pathways; both LTB4 and CysLTs prompt the expansion of CD24+CD90+ metastasis-initiating cells (Tong et al., 2005; Ihara et al., 2007; Wculek and Malanchi, 2015). Activation of LTD4 promotes the growth and survival of human intestinal epithelial cells through multiple parallel pathways, including GSK3β/β-catenin, PKC/Raf/ERK1, and ERK2 signaling (Paruchuri et al., 2002; Mezhybovska et al., 2006). By contrast, inhibition of LTD4 signaling by a CysLT1 antagonist causes apoptosis of prostate carcinoma cells (Matsuyama et al., 2007; Larre et al., 2008). Increased CysLT1 and CysLT2 expression are associated with LTD4-induced apoptosis-resistance, hyper-proliferation of colorectal cancer cells, and poor clinical prognosis (Magnusson et al., 2007; Jeong et al., 2009). A prominent role of leukotrienes in the regulation of stem cell homeostasis is established, with LTB4 and LTD4 known to stimulate the proliferation and maturation of several types of stem cells, progenitor cells, and cancer stem cells (Chung et al., 2005; Paruchuri et al., 2006; Wada et al., 2006).
Leukotrienes and Epithelial (Endothelial)-to-Mesenchymal Transition
Alterations in cell phenotypes, EMT in particular, have been shown to play an important role in tumorigenic processes (Brabletz et al., 2018; Pastushenko and Blanpain, 2019). Complete and partial EMT in cancer is executed by EMT-activating transcription factors, including SNAIL, TWIST, and ZEB families, which regulate all stages of cancer progression from initiation, primary tumor growth, invasion, metastasis, to colonization, as well as resistance to therapy (Brabletz et al., 2018; Pastushenko and Blanpain, 2019). In addition, EMT-activating transcription factors have been shown to be critical for the maintenance of cancer stemness (Pastushenko and Blanpain, 2019). Specifically, studies focusing on IL-6, IL-8, and TNF-α demonstrate that expression of these inflammatory mediators is associated with clinical occurrences of EMT and resistance to EGFR inhibition (Singh et al., 2013; Thompson et al., 2015).
In culture, LTB4, via BLT2, promotes EMT and the expression of vimentin in some human cancer cell lines through activation of reactive oxygen species, NF-κB, TGF-β, and ERK (Kim et al., 2014). LTB4 also upregulates the production of IL-8 in breast cancer cells, while blocking BLT2 suppresses the formation of metastatic lung nodules in animal models of breast cancer metastasis (Kim et al., 2012). In coordination with NF-κB signaling, LTB4 mediates the synthesis of both IL-6 and IL-8 to increase the invasiveness of cancer cells (Thompson et al., 2015). Exogenous LTB4 or 5-LO causes pulmonary arterial EC, derived from patients with BMPR2 mutations, to undergo endothelial-to-mesenchymal-transition (EndMT) through activated TGF-β signaling; these cells lose the typical endothelial cobblestone appearance and, instead, acquires an elongated mesenchymal cell shape with increased expression of mesenchymal markers (vimentin, SM-actin, and SLUG) and inflammatory molecules (IL-6, IL-1β, and TNF-α), a process resembling EMT (Tian et al., 2019).
Leukotrienes and Tumor Microenvironment
A growing body of literature indicates that 5-LO and leukotrienes are critical components TME, mediating the crosstalk between epithelial cells, stromal cells, and immune cells. In lung cancer, a complex molecular interaction initiated by mast cell-derived LTB4 has been described, in which mast cells orchestrate with tumor-promoting neutrophils through production of LTB4 (Malaviya and Abraham, 2000; Satpathy et al., 2015). Neutrophils support the metastatic transformation and colonization of breast cancer cells through leukotriene-mediated ERK activation (Wculek and Malanchi, 2015). Treatment with zileuton reduces systemic inflammation, blocks macrophage infiltrates, and decreases polyp burden of the small intestine and colon in a murine model of polyposis (Gounaris et al., 2015). Genetic deletion or pharmacological ablation of 5-LO or LTA4H significantly reduces the tumor burden in K-ras–driven pancreatic ductal adenocarcinoma and in xenograft mouse model of human pancreatic cancer, through reduction of TNF-α secretion (Oi et al., 2010; Knab et al., 2015). TNF-α, one of the main cytokines in the TME, has a context-dependent role in tumor growth (Balkwill, 2009). In a cooperative manner with TNF-α, LTB4 may influence the growth, survival, invasion, and metastasis of tumor cells.
Leukotrienes and Tumor Suppression
Human and animal studies suggest a positive correlation of prolonged survival with the presence of tumor-infiltrating CD8+ T cells (Galon et al., 2006; Kmiecik et al., 2013). LTB4/BLT1 signaling is required for cytotoxic T cells accumulation during allergic inflammation (Miyahara et al., 2005). A recent study demonstrates a pivotal function of LTB4/BLT1 signaling for the tumor immune suppression of CD8+ T cells: CD8+ T cells depletion enhances tumor growth in wild-type but not in BLT1−/− mice, implicating the importance of BLT1 in CD8+ T cells cancer immunity (Sharma et al., 2013). The presence of tumor-infiltrating lymphocytes correlates with the responsiveness to PD-1 (programmed cell death protein 1)-targeting cancer therapies (Yao et al., 2018). Notably, PD-1 blockade fails to reduce melanoma growth in BLT1−/− mice due to deficiency in T cell infiltrations (Chheda et al., 2016). Collectively, these findings suggest an important role of LTB4 signaling to facilitate the migration of tumor-infiltrating lymphocytes in anti-tumor immunity in cancer.
Leukotrienes in Angiogenesis and Lymphangiogenesis
To initiate metastasis, cancer cells disseminate from the primary tumor either through blood (hematogenous spread after angiogenesis) or through the lymphatic (lymphogenous spread after lymphangiogenesis) circulation (Christiansen and Detmar, 2011; Ziyad and Iruela-Arispe, 2011). It is generally believed that alterations in the primary TME and EMT of tumor cells facilitate the migration towards blood or lymphatic vessels (Christiansen and Detmar, 2011; Ziyad and Iruela-Arispe, 2011). Although not fully understood how the route of intravasation is determined (i.e., hematogenous or lymphogenous), inflammatory signaling appears to be the key regulator attracting tumor cells towards circulation (Noonan et al., 2008; Zuazo-Gaztelu and Casanovas, 2018). Blocking 5-LO or LTB4/BLT2 signaling significantly inhibits the VEGF-A-mediated angiogenic responses (Tsopanoglou et al., 1994). LTC4 and LTD4, working through CysLT2, enhance angiogenesis and the permeability of blood vessels, independent of VEGF-A signaling, and thereby, contribute to tumor metastasis (Kim et al., 2009). LTB4 poses bimodal effects on lymphatic vessel health: at low concentrations, LTB4 promotes lymphatic EC growth and sprouting, while high concentrations of LTB4 inhibit lymphangiogenesis and induce apoptosis of lymphatic ECs (Tian et al., 2017). Recent studies have suggested that a viable lymphatic vasculature promotes the efficacy of immunotherapy (Fankhauser et al., 2017; Jiang, 2020). Therefore, LTB4 may also influence immunotherapeutics by affecting the growth and survival of lymphatic vessels.
Therapeutic Interventions Targeting Leukotrienes and 5-LO
Many anti-inflammatory agents, including NSAIDs (nonsteroidal anti-inflammatory drugs) which inhibits cyclooxygenase (COX-1, COX-2, or both) or both COX and 5-LO, as well as, specific inhibitors of 5-LO and leukotriene signaling, have demonstrated promising results in interfering with the tumor microenvironment (Cruz-Correa et al., 2002; Baron, 2003; Rayburn et al., 2009; Wong, 2019). In particular, a meta-analysis of 16 independent studies, including 202,780 patients with a diagnosis of prostate, lung, colorectal, and breast cancers, suggests the potential of NSAID in reducing tumor incidence and mortality (Cruz-Correa et al., 2002). Specifically, familial adenomatous polyposis (FAP) patients receiving NSAID (celecoxib or sulindac), dual blockers of COX-2 and 5-LO, display a decreased recurrence, lower polyp number, and a regression of existing adenomas (Steinbach et al., 2000; Cruz-Correa et al., 2002; Maier et al., 2008; Steinbrink et al., 2010; Lynch et al., 2016). Celecoxib was approved by FDA as adjuvant therapy for FAP in 2011. The potential usage of rofecoxib or valdecoxib, two other dual inhibitors of COX-2 and 5-LO, as adjuvant therapy for tumor metastasis, is still under investigation. Nevertheless, the application of NSAIDs as anticancer agents remains controversial because of their possible gastrointestinal and cardiovascular toxicity.
Antagonists specific for 5-LO and leukotrienes are well-tolerated and confer no adverse effect in the gastrointestinal and cardiovascular systems. Despite numerous reports of the anti-tumor properties of these agents in preclinical and cell culture studies, only a few clinical trials have been conducted to evaluate their potential in cancer treatment. The 5-LO inhibitor, zileuton, has shown positive results in treating experimental models of colon, lung, and pancreatic cancers (Rioux and Castonguay, 1998; Wenger et al., 2002; Chen et al., 2004; Wculek and Malanchi, 2015). Blocking FLAP also indicates therapeutic benefit in the hamster model of pancreatic cancer. A few clinical trials have been conducted using LY293111, a well-tolerated inhibitor of BLT1, in patients with pancreatic cancer and non-small cell lung cancer; no significant difference in short-term survival was noted from these human studies (Ding et al., 2005; Janne et al., 2014). Notably, LTA4H inhibitor, ubenimex, has been marketed in Japan for over 30 years as an adjunct therapy for adult acute leukemia and lung cancers.
Various natural compounds, found in food and plants, may inhibit COX-2 and 5-LO pathways. Resveratrol (enriched in grapes and red wine), ginsenosides (found in ginseng), s-allylmercaptocysteine (purified from garlic), and turmeric curcumin (present in curry) are among these categories (Das and Das, 2007; Park et al., 2007; Li et al., 2014; Jeong et al., 2016). A number of recent clinical studies demonstrate encouraging results in the usage of these natural compounds in combination with conventional cancer therapies, possibly through arresting cancer cell growth, potentiating cellular apoptosis, inhibiting NF-κB, MAPK, JNK, and VEGF pathways and sequestering reactive oxygen species (Hassan, 2004; Park et al., 2007; Hofseth and Wargovich, 2007; Howard et al., 2008).
Concluding Remarks
Promising preclinical results have motivated the pharmaceutical industry to develop anti-5-LO and anti-leukotriene drugs to treat a wide range of cancers (Orafaie et al., 2020). To date, most of the small molecule inhibitors in these classes failed to merit clinical application. Leukotrienes are typically generated by activated leukocytes; therefore, the impact of these mediators depends on the temporal accumulation and composition of immune cells at various stages of cancer. Leukotrienes fall into a big category of eicosanoid metabolites which are interconnected in a complex manner. Modulating one pathway may likely cause shunting the eicosanoid synthesis to lipids with opposing bioaction [e.g., blocking macrophage production of LTB4 causes increased expression of PGE2, which is implicated in multiple pro-tumor responses (Nakanishi and Rosenberg, 2013)]. Inflammation is the primary risk factor for the development of certain types of cancers. The proinflammatory tumor microenvironment is pivotal to the spread of cancer. However, immunotherapies, such as checkpoint inhibition and adoptive cell transfer, may benefit from a fine-tuned and tumor-specific T cell response. Understanding the communications between cancer and inflammation will facilitate the discovery of the next breakthrough in cancer management. 5-LO and leukotriene signalling is a critical component of the inflammatory tumor microenvironment: leukotrienes are strong chemoattractants for leukocytes; 5-LO and leukotrienes may elicit robust immune reactions by promoting the growth and survival of immune cells; 5-LO and leukotrienes participate in the maladaptive growth response of cancer cells and may modulate the expansion of blood and lymphatic vessels around primary tumor (i.e., angiogenesis and lymphangiogenesis); and last but not least, interactions between 5-LO and leukotriene signaling and a number of inflammatory pathways (e.g., COX, IL-1, IL-6, IL-17, NF-κB, and TNF-α) are identified in various inflammatory conditions, including cancers. Elucidating the biology of eicosanoids in tumorigenesis, profiling these biologically active lipids and their associated enzymes and receptors in cancer, and gaining structural insight into the eicosanoid proteins are crucial steps to inform future efforts in developing biomarkers and designing drug targets.
Author Contributions
Conceived and participated in the writing of this manuscript (WT, XJ SR). Assisted in the preparation and editing of the manuscript (DK, TG, MN).
Funding
This work was supported by Stanford Endowed Chair funds to (MN and SR), the NIH K12 HL120001-05 (to XJ) and the NIH HL095686, HL141105, HL138473 to (MN).
Conflict of Interest
The authors declare that the research was conducted in the absence of any commercial or financial relationships that could be construed as a potential conflict of interest.
References
Abu-Shakra, M., Buskila, D., Ehrenfeld, M., Conrad, K., Shoenfeld, Y. (2001). Cancer and autoimmunity: autoimmune and rheumatic features in patients with malignancies. Ann. Rheum. Dis. 60 (5), 433–441. doi: 10.1136/ard.60.5.433
Araujo, A. C., Wheelock, C. E., Haeggstrom, J. Z. (2018). The Eicosanoids, Redox-Regulated Lipid Mediators in Immunometabolic Disorders. Antioxid. Redox Signal 29 (3), 275–296. doi: 10.1089/ars.2017.7332
Ayob, A. Z., Ramasamy, T. S. (2018). Cancer stem cells as key drivers of tumour progression. J. BioMed. Sci. 25 (1), 20. doi: 10.1186/s12929-018-0426-4
Back, M. (2009). Leukotriene signaling in atherosclerosis and ischemia. Cardiovasc. Drugs Ther. 23 (1), 41–48. doi: 10.1007/s10557-008-6140-9
Balkwill, F. (2009). Tumour necrosis factor and cancer. Nat. Rev. Cancer 9 (5), 361–371. doi: 10.1038/nrc2628
Baron, J. A. (2003). Epidemiology of nonsteroidal anti-inflammatory drugs and cancer. Prog. Exp. Tumor Res. 37, 1–24. doi: 10.1159/000071364
Bendas, G., Borsig, L. (2012). Cancer cell adhesion and metastasis: selectins, integrins, and the inhibitory potential of heparins. Int. J. Cell Biol. 2012, 676731. doi: 10.1155/2012/676731
Binnewies, M., Roberts, E. W., Kersten, K., Chan, V., Fearon, D. F., Merad, M., et al. (2018). Understanding the tumor immune microenvironment (TIME) for effective therapy. Nat. Med. 24 (5), 541–550. doi: 10.1038/s41591-018-0014-x
Brabletz, T., Kalluri, R., Nieto, M. A., Weinberg, R. A. (2018). EMT in cancer. Nat. Rev. Cancer 18 (2), 128–134. doi: 10.1038/nrc.2017.118
Chaturvedi, M. M., Sung, B., Yadav, V. R., Kannappan, R., Aggarwal, B. B. (2011). NF-kappaB addiction and its role in cancer: ‘one size does not fit all’. Oncogene 30 (14), 1615–1630. doi: 10.1038/onc.2010.566
Che, D., Zhang, S., Jing, Z., Shang, L., Jin, S., Liu, F., et al. (2017). Macrophages induce EMT to promote invasion of lung cancer cells through the IL-6-mediated COX-2/PGE2/beta-catenin signalling pathway. Mol. Immunol. 90, 197–210. doi: 10.1016/j.molimm.2017.06.018
Chen, X., Wang, S., Wu, N., Sood, S., Wang, P., Jin, Z., et al. (2004). Overexpression of 5-lipoxygenase in rat and human esophageal adenocarcinoma and inhibitory effects of zileuton and celecoxib on carcinogenesis. Clin. Cancer Res. 10 (19), 6703–6709. doi: 10.1158/1078-0432.CCR-04-0838
Chen, Y., Hu, Y., Zhang, H., Peng, C., Li, S. (2009a). Loss of the Alox5 gene impairs leukemia stem cells and prevents chronic myeloid leukemia. Nat. Genet. 41 (7), 783–792. doi: 10.1038/ng.389
Chen, Y., Li, D., Li, S. (2009b). The Alox5 gene is a novel therapeutic target in cancer stem cells of chronic myeloid leukemia. Cell Cycle 8 (21), 3488–3492. doi: 10.4161/cc.8.21.9852
Chheda, Z. S., Sharma, R. K., Jala, V. R., Luster, A. D., Haribabu, B. (2016). Chemoattractant Receptors BLT1 and CXCR3 Regulate Antitumor Immunity by Facilitating CD8+ T Cell Migration into Tumors. J. Immunol. 197 (5), 2016–2026. doi: 10.4049/jimmunol.1502376
Christiansen, A., Detmar, M. (2011). Lymphangiogenesis and cancer. Genes Cancer 2 (12), 1146–1158. doi: 10.1177/1947601911423028
Chung, J. W., Kim, G. Y., Mun, Y. C., Ahn, J. Y., Seong, C. M., Kim, J. H. (2005). Leukotriene B4 pathway regulates the fate of the hematopoietic stem cells. Exp. Mol. Med. 37 (1), 45–50. doi: 10.1038/emm.2005.6
Coussens, L. M., Werb, Z. (2002). Inflammation and cancer. Nature 420 (6917), 860–867. 10.1038/nature01322
Cruz-Correa, M., Hylind, L. M., Romans, K. E., Booker, S. V., Giardiello, F. M. (2002). Long-term treatment with sulindac in familial adenomatous polyposis: a prospective cohort study. Gastroenterology 122 (3), 641–645. doi: 10.1053/gast.2002.31890
Das, S., Das, D. K. (2007). Anti-inflammatory responses of resveratrol. Inflammation Allergy Drug Targets 6 (3), 168–173. doi: 10.2174/187152807781696464
Del Prete, A., Shao, W. H., Mitola, S., Santoro, G., Sozzani, S., Haribabu, B. (2007). Regulation of dendritic cell migration and adaptive immune response by leukotriene B4 receptors: a role for LTB4 in up-regulation of CCR7 expression and function. Blood 109 (2), 626–631. doi: 10.1182/blood-2006-02-003665
Ding, X. Z., Talamonti, M. S., Bell, R. H., Jr., Adrian, T. E. (2005). A novel anti-pancreatic cancer agent, LY293111. Anticancer Drugs 16 (5), 467–473. doi: 10.1097/00001813-200506000-00001
Drakatos, P., Lykouras, D., Sampsonas, F., Karkoulias, K., Spiropoulos, K. (2009). Targeting leukotrienes for the treatment of COPD? Inflammation Allergy Drug Targets 8 (4), 297–306. doi: 10.2174/187152809789352177
Duah, E., Adapala, R. K., Al-Azzam, N., Kondeti, V., Gombedza, F., Thodeti, C. K., et al. (2013). Cysteinyl leukotrienes regulate endothelial cell inflammatory and proliferative signals through CysLT(2) and CysLT(1) receptors. Sci. Rep. 3, 3274. doi: 10.1038/srep03274
Fankhauser, M., Broggi, M. A. S., Potin, L., Bordry, N., Jeanbart, L., Lund, A. W., et al. (2017). Tumor lymphangiogenesis promotes T cell infiltration and potentiates immunotherapy in melanoma. Sci. Transl. Med. 9 (407). doi: 10.1126/scitranslmed.aal4712
Galon, J., Costes, A., Sanchez-Cabo, F., Kirilovsky, A., Mlecnik, B., Lagorce-Pages, C., et al. (2006). Type, density, and location of immune cells within human colorectal tumors predict clinical outcome. Science 313 (5795), 1960–1964. doi: 10.1126/science.1129139
Giannopoulos, P. F., Chiu, J., Pratico, D. (2019). Learning Impairments, Memory Deficits, and Neuropathology in Aged Tau Transgenic Mice Are Dependent on Leukotrienes Biosynthesis: Role of the cdk5 Kinase Pathway. Mol. Neurobiol. 56 (2), 1211–1220. doi: 10.1007/s12035-018-1124-7
Gijon, M. A., Zarini, S., Murphy, R. C. (2007). Biosynthesis of eicosanoids and transcellular metabolism of leukotrienes in murine bone marrow cells. J. Lipid Res. 48 (3), 716–725. doi: 10.1194/jlr.M600508-JLR200
Giovannucci, E., Harlan, D. M., Archer, M. C., Bergenstal, R. M., Gapstur, S. M., Habel, L. A., et al. (2010). Diabetes and cancer: a consensus report. Diabetes Care 33 (7), 1674–1685. doi: 10.2337/dc10-0666
Goodarzi, K., Goodarzi, M., Tager, A. M., Luster, A. D., von Andrian, U. H. (2003). Leukotriene B4 and BLT1 control cytotoxic effector T cell recruitment to inflamed tissues. Nat. Immunol. 4 (10), 965–973. doi: 10.1038/ni972
Gounaris, E., Heiferman, M. J., Heiferman, J. R., Shrivastav, M., Vitello, D., Blatner, N. R., et al. (2015). Zileuton, 5-lipoxygenase inhibitor, acts as a chemopreventive agent in intestinal polyposis, by modulating polyp and systemic inflammation. PLoS One 10 (3), e0121402. doi: 10.1371/journal.pone.0121402
Greten, F. R., Grivennikov, S. I. (2019). Inflammation and Cancer: Triggers, Mechanisms, and Consequences. Immunity 51 (1), 27–41. doi: 10.1016/j.immuni.2019.06.025
Grivennikov, S. I., Greten, F. R., Karin, M. (2010). Immunity, inflammation, and cancer. Cell 140 (6), 883–899. doi: 10.1016/j.cell.2010.01.025
Gu, K., Li, M. M., Shen, J., Liu, F., Cao, J. Y., Jin, S., et al. (2015). Interleukin-17-induced EMT promotes lung cancer cell migration and invasion via NF-kappaB/ZEB1 signal pathway. Am. J. Cancer Res. 5 (3), 1169–1179. doi: 2156-6976/ajcr0005648
Haeggstrom, J. Z., Funk, C. D. (2011). Lipoxygenase and leukotriene pathways: biochemistry, biology, and roles in disease. Chem. Rev. 111 (10), 5866–5898. doi: 10.1021/cr200246d
Hassan, H. T. (2004). Ajoene (natural garlic compound): a new anti-leukaemia agent for AML therapy. Leuk. Res. 28 (7), 667–671. doi: 10.1016/j.leukres.2003.10.008
Hernandez, C., Huebener, P., Schwabe, R. F. (2016). Damage-associated molecular patterns in cancer: a double-edged sword. Oncogene 35 (46), 5931–5941. doi: 10.1038/onc.2016.104
Hofseth, L. J., Wargovich, M. J. (2007). Inflammation, cancer, and targets of ginseng. J. Nutr. 137 (1 Suppl), 183S–185S. doi: 10.1093/jn/137.1.183S
Howard, E. W., Lee, D. T., Chiu, Y. T., Chua, C. W., Wang, X., Wong, Y. C., et al. (2008). Evidence of a novel docetaxel sensitizer, garlic-derived S-allylmercaptocysteine, as a treatment option for hormone refractory prostate cancer. Int. J. Cancer 122 (9), 1941–1948. doi: 10.1002/ijc.23355
Ihara, A., Wada, K., Yoneda, M., Fujisawa, N., Takahashi, H., Nakajima, A. (2007). Blockade of leukotriene B4 signaling pathway induces apoptosis and suppresses cell proliferation in colon cancer. J. Pharmacol. Sci. 103 (1), 24–32. doi: 10.1254/jphs.FP0060651
Izumo, T., Kondo, M., Nagai, A. (2009). Effects of a leukotriene B4 receptor antagonist on bleomycin-induced pulmonary fibrosis. Eur. Respir. J. 34 (6), 1444–1451. doi: 10.1183/09031936.00143708
Jala, V. R., Bodduluri, S. R., Satpathy, S. R., Chheda, Z., Sharma, R. K., Haribabu, B. (2017). The yin and yang of leukotriene B4 mediated inflammation in cancer. Semin. Immunol. 33, 58–64. doi: 10.1016/j.smim.2017.09.005
Janne, P. A., Paz-Ares, L., Oh, Y., Eschbach, C., Hirsh, V., Enas, N., et al. (2014). Randomized, double-blind, phase II trial comparing gemcitabine-cisplatin plus the LTB4 antagonist LY293111 versus gemcitabine-cisplatin plus placebo in first-line non-small-cell lung cancer. J. Thorac. Oncol. 9 (1), 126–131. doi: 10.1097/JTO.0000000000000037
Jeong, C. H., Bode, A. M., Pugliese, A., Cho, Y. Y., Kim, H. G., Shim, J. H., et al. (2009). [6]-Gingerol suppresses colon cancer growth by targeting leukotriene A4 hydrolase. Cancer Res. 69 (13), 5584–5591. doi: 10.1158/0008-5472.CAN-09-0491
Jeong, Y. Y., Ryu, J. H., Shin, J. H., Kang, M. J., Kang, J. R., Han, J., et al. (2016). Comparison of Anti-Oxidant and Anti-inflammatory Effects between Fresh and Aged Black Garlic Extracts. Molecules 21 (4), 430. doi: 10.3390/molecules21040430
Jiang, X. (2020). Lymphatic vasculature in tumor metastasis and immunobiology. J. Zhejiang Univ. Sci. B. 21 (1), 3–11. 10.1631/jzus.B1800633
Kim, G. Y., Lee, J. W., Cho, S. H., Seo, J. M., Kim, J. H. (2009). Role of the low-affinity leukotriene B4 receptor BLT2 in VEGF-induced angiogenesis. Arterioscler. Thromb. Vasc. Biol. 29 (6), 915–920. doi: 10.1161/ATVBAHA.109.185793
Kim, H., Choi, J. A., Park, G. S., Kim, J. H. (2012). BLT2 upregulates interleukin-8 production and promotes the invasiveness of breast cancer cells. PLoS One 7 (11), e49186. doi: 10.1371/journal.pone.0049186
Kim, H., Choi, J. A., Kim, J. H. (2014). Ras promotes transforming growth factor-beta (TGF-beta)-induced epithelial-mesenchymal transition via a leukotriene B4 receptor-2-linked cascade in mammary epithelial cells. J. Biol. Chem. 289 (32), 22151–22160. doi: 10.1074/jbc.M114.556126
Kmiecik, J., Poli, A., Brons, N. H., Waha, A., Eide, G. E., Enger, P. O., et al. (2013). Elevated CD3+ and CD8+ tumor-infiltrating immune cells correlate with prolonged survival in glioblastoma patients despite integrated immunosuppressive mechanisms in the tumor microenvironment and at the systemic level. J. Neuroimmunol. 264 (1-2), 71–83. doi: 10.1016/j.jneuroim.2013.08.013
Knab, L. M., Schultz, M., Principe, D. R., Mascarinas, W. E., Gounaris, E., Munshi, H. G., et al. (2015). Ablation of 5-lipoxygenase mitigates pancreatic lesion development. J. Surg. Res. 194 (2), 481–487. doi: 10.1016/j.jss.2014.10.021
Kumari, N., Dwarakanath, B. S., Das, A., Bhatt, A. N. (2016). Role of interleukin-6 in cancer progression and therapeutic resistance. Tumour Biol. 37 (9), 11553–11572. doi: 10.1007/s13277-016-5098-7
Labi, V., Erlacher, M. (2015). How cell death shapes cancer. Cell Death Dis. 6, e1675. doi: 10.1038/cddis.2015.20
Larre, S., Tran, N., Fan, C., Hamadeh, H., Champigneulles, J., Azzouzi, R., et al. (2008). PGE2 and LTB4 tissue levels in benign and cancerous prostates. Prostaglandins Other Lipid Mediat. 87 (1-4), 14–19. doi: 10.1016/j.prostaglandins.2008.05.001
Li, X., Lu, Y., Jin, Y., Son, J. K., Lee, S. H., Chang, H. W. (2014). Curcumin inhibits the activation of immunoglobulin e-mediated mast cells and passive systemic anaphylaxis in mice by reducing serum eicosanoid and histamine levels. Biomol. Ther. (Seoul) 22 (1), 27–34. doi: 10.4062/biomolther.2013.092
Li, P., Oh, D. Y., Bandyopadhyay, G., Lagakos, W. S., Talukdar, S., Osborn, O., et al. (2015). LTB4 promotes insulin resistance in obese mice by acting on macrophages, hepatocytes and myocytes. Nat. Med. 21 (3), 239–247. doi: 10.1038/nm.3800
Lin, Y. L., Tsai, N. M., Hsieh, C. H., Ho, S. Y., Chang, J., Wu, H. Y., et al. (2016). In vivo amelioration of endogenous anti-tumor autoantibodies via low-dose P4N through the LTA4H/activin A/BAFF pathway. Proc. Natl. Acad. Sci. U. S. A. 113 (48), E7798–E7807. doi: 10.1073/pnas.1604752113
Luo, M., Jones, S. M., Peters-Golden, M., Brock, T. G. (2003). Nuclear localization of 5-lipoxygenase as a determinant of leukotriene B4 synthetic capacity. Proc. Natl. Acad. Sci. U. S. A. 100 (21), 12165–12170. doi: 10.1073/pnas.2133253100
Lynch, P. M., Burke, C. A., Phillips, R., Morris, J. S., Slack, R., Wang, X., et al. (2016). An international randomised trial of celecoxib versus celecoxib plus difluoromethylornithine in patients with familial adenomatous polyposis. Gut 65 (2), 286–295. doi: 10.1136/gutjnl-2014-307235
Magnusson, C., Ehrnstrom, R., Olsen, J., Sjolander, A. (2007). An increased expression of cysteinyl leukotriene 2 receptor in colorectal adenocarcinomas correlates with high differentiation. Cancer Res. 67 (19), 9190–9198. doi: 10.1158/0008-5472.CAN-07-0771
Maier, T. J., Tausch, L., Hoernig, M., Coste, O., Schmidt, R., Angioni, C., et al. (2008). Celecoxib inhibits 5-lipoxygenase. Biochem. Pharmacol. 76 (7), 862–872. doi: 10.1016/j.bcp.2008.07.009
Malaviya, R., Abraham, S. N. (2000). Role of mast cell leukotrienes in neutrophil recruitment and bacterial clearance in infectious peritonitis. J. Leukoc. Biol. 67 (6), 841–846. doi: 10.1002/jlb.67.6.841
Mandal, A. K., Jones, P. B., Bair, A. M., Christmas, P., Miller, D., Yamin, T. T., et al. (2008). The nuclear membrane organization of leukotriene synthesis. Proc. Natl. Acad. Sci. U. S. A. 105 (51), 20434–20439. doi: 10.1073/pnas.0808211106
Matsuyama, M., Hayama, T., Funao, K., Kawahito, Y., Sano, H., Takemoto, Y., et al. (2007). Overexpression of cysteinyl LT1 receptor in prostate cancer and CysLT1R antagonist inhibits prostate cancer cell growth through apoptosis. Oncol. Rep. 18 (1), 99–104. doi: 10.3892/or.18.1.99
McGeachy, M. J., Cua, D. J., Gaffen, S. L. (2019). The IL-17 Family of Cytokines in Health and Disease. Immunity 50 (4), 892–906. doi: 10.1016/j.immuni.2019.03.021
McGovern, D., Powrie, F. (2007). The IL23 axis plays a key role in the pathogenesis of IBD. Gut 56 (10), 1333–1336. doi: 10.1136/gut.2006.115402
Mezhybovska, M., Wikstrom, K., Ohd, J. F., Sjolander, A. (2006). The inflammatory mediator leukotriene D4 induces beta-catenin signaling and its association with antiapoptotic Bcl-2 in intestinal epithelial cells. J. Biol. Chem. 281 (10), 6776–6784. doi: 10.1074/jbc.M509999200
Michael, J., Marschallinger, J., Aigner, L. (2019). The leukotriene signaling pathway: a druggable target in Alzheimer’s disease. Drug Discovery Today 24 (2), 505–516. doi: 10.1016/j.drudis.2018.09.008
Mittal, M., Siddiqui, M. R., Tran, K., Reddy, S. P., Malik, A. B. (2014). Reactive oxygen species in inflammation and tissue injury. Antioxid. Redox Signal 20 (7), 1126–1167. doi: 10.1089/ars.2012.5149
Miyahara, N., Takeda, K., Miyahara, S., Taube, C., Joetham, A., Koya, T., et al. (2005). Leukotriene B4 receptor-1 is essential for allergen-mediated recruitment of CD8+ T cells and airway hyperresponsiveness. J. Immunol. 174 (8), 4979–4984. doi: 10.4049/jimmunol.174.8.4979
Moos, M. P., Mewburn, J. D., Kan, F. W., Ish, S., Abe, M., Sakimura, K., et al. (2008). Cysteinyl leukotriene 2 receptor-mediated vascular permeability via transendothelial vesicle transport. FASEB J. 22 (12), 4352–4362. doi: 10.1096/fj.08-113274
Muz, B., de la Puente, P., Azab, F., Azab, A. K. (2015). The role of hypoxia in cancer progression, angiogenesis, metastasis, and resistance to therapy. Hypoxia (Auckl) 3, 83–92. doi: 10.2147/HP.S93413
Nakamura, M., Shimizu, T. (2011). Leukotriene receptors. Chem. Rev. 111 (10), 6231–6298. doi: 10.1021/cr100392s
Nakanishi, M., Rosenberg, D. W. (2013). Multifaceted roles of PGE2 in inflammation and cancer. Semin. Immunopathol. 35 (2), 123–137. doi: 10.1007/s00281-012-0342-8
Noonan, D. M., De Lerma Barbaro, A., Vannini, N., Mortara, L., Albini, A. (2008). Inflammation, inflammatory cells and angiogenesis: decisions and indecisions. Cancer Metastasis Rev. 27 (1), 31–40. doi: 10.1007/s10555-007-9108-5
Noy, R., Pollard, J. W. (2014). Tumor-associated macrophages: from mechanisms to therapy. Immunity 41 (1), 49–61. doi: 10.1016/j.immuni.2014.06.010
Oi, N., Jeong, C. H., Nadas, J., Cho, Y. Y., Pugliese, A., Bode, A. M., et al. (2010). Resveratrol, a red wine polyphenol, suppresses pancreatic cancer by inhibiting leukotriene A(4)hydrolase. Cancer Res. 70 (23), 9755–9764. doi: 10.1158/0008-5472.CAN-10-2858
Orafaie, A., Mousavian, M., Orafaie, H., Sadeghian, H. (2020). An overview of lipoxygenase inhibitors with approach of in vivo studies. Prostaglandins Other Lipid Mediat. 148, 106411. doi: 10.1016/j.prostaglandins.2020.106411
Park, S., Yeo, M., Jin, J. H., Lee, K. M., Kim, S. S., Choi, S. Y., et al. (2007). Inhibitory activities and attenuated expressions of 5-LOX with red ginseng in Helicobacter pylori-infected gastric epithelial cells. Dig. Dis. Sci. 52 (4), 973–982. doi: 10.1007/s10620-006-9440-6
Paruchuri, S., Hallberg, B., Juhas, M., Larsson, C., Sjolander, A. (2002). Leukotriene D(4) activates MAPK through a Ras-independent but PKCepsilon-dependent pathway in intestinal epithelial cells. J. Cell Sci. 115 (Pt 9), 1883–1893.
Paruchuri, S., Mezhybovska, M., Juhas, M., Sjolander, A. (2006). Endogenous production of leukotriene D4 mediates autocrine survival and proliferation via CysLT1 receptor signalling in intestinal epithelial cells. Oncogene 25 (50), 6660–6665. doi: 10.1038/sj.onc.1209666
Pastushenko, I., Blanpain, C. (2019). EMT Transition States during Tumor Progression and Metastasis. Trends Cell Biol. 29 (3), 212–226. doi: 10.1016/j.tcb.2018.12.001
Peters-Golden, M., Brock, T. G. (2001). Intracellular compartmentalization of leukotriene synthesis: unexpected nuclear secrets. FEBS Lett. 487 (3), 323–326. doi: 10.1016/S0014-5793(00)02374-7
Peters-Golden, M., Henderson, W. R., Jr. (2007). Leukotrienes. N Engl. J. Med. 357 (18), 1841–1854. doi: 10.1056/NEJMra071371
Peters-Golden, M., Canetti, C., Mancuso, P., Coffey, M. J. (2005). Leukotrienes: underappreciated mediators of innate immune responses. J. Immunol. 174 (2), 589–594. doi: 10.4049/jimmunol.174.2.589
Pietrzyk, L., Torres, A., Maciejewski, R., Torres, K. (2015). Obesity and Obese-related Chronic Low-grade Inflammation in Promotion of Colorectal Cancer Development. Asian Pac. J. Cancer Prev. 16 (10), 4161–4168. doi: 10.7314/APJCP.2015.16.10.4161
Porter, K. M., Kang, B. Y., Adesina, S. E., Murphy, T. C., Hart, C. M., Sutliff, R. L., et al. (2014). Chronic hypoxia promotes pulmonary artery endothelial cell proliferation through H2O2-induced 5-lipoxygenase. PLoS One 9 (6), e98532. doi: 10.1371/journal.pone.0098532
Price, D., Musgrave, S. D., Shepstone, L., Hillyer, E. V., Sims, E. J., Gilbert, R. F., et al. (2011). Leukotriene antagonists as first-line or add-on asthma-controller therapy. N Engl. J. Med. 364 (18), 1695–1707. doi: 10.1056/NEJMoa1010846
Qian, J., Tian, W., Jiang, X., Tamosiuniene, R., Sung, Y. K., Shuffle, E. M., et al. (2015). Leukotriene B4 Activates Pulmonary Artery Adventitial Fibroblasts in Pulmonary Hypertension. Hypertension 66 (6), 1227–1239. doi: 10.1161/HYPERTENSIONAHA.115.06370
Radmark, O., Samuelsson, B. (2009). 5-Lipoxygenase: mechanisms of regulation. J. Lipid Res. 50 (Suppl), S40–S45. doi: 10.1194/jlr.R800062-JLR200
Ramalho, T., Filgueiras, L., Silva-Jr, I. A., Pessoa, A. F. M., Jancar, S. (2018). Impaired wound healing in type 1 diabetes is dependent on 5-lipoxygenase products. Sci. Rep. 8 (1), 14164. doi: 10.1038/s41598-018-32589-7
Rayburn, E. R., Ezell, S. J., Zhang, R. (2009). Anti-Inflammatory Agents for Cancer Therapy. Mol. Cell Pharmacol. 1 (1), 29–43. doi: 10.4255/mcpharmacol.09.05
Rioux, N., Castonguay, A. (1998). Inhibitors of lipoxygenase: a new class of cancer chemopreventive agents. Carcinogenesis 19 (8), 1393–1400. doi: 10.1093/carcin/19.8.1393
Rockson, S. G., Tian, W., Jiang, X., Kuznetsova, T., Haddad, F., Zampell, J., et al. (2018). Pilot studies demonstrate the potential benefits of anti-inflammatory therapy in human lymphedema. JCI Insight 3 (20). doi: 10.1172/jci.insight.123775
Romano, M., Catalano, A., Nutini, M., D'Urbano, E., Crescenzi, C., Claria, J., et al. (2001). 5-lipoxygenase regulates malignant mesothelial cell survival: involvement of vascular endothelial growth factor. FASEB J. 15 (13), 2326–2336. doi: 10.1096/fj.01-0150com
Roos, J., Oancea, C., Heinssmann, M., Khan, D., Held, H., Kahnt, A. S., et al. (2014). 5-Lipoxygenase is a candidate target for therapeutic management of stem cell-like cells in acute myeloid leukemia. Cancer Res. 74 (18), 5244–5255. doi: 10.1158/0008-5472.CAN-13-3012
Sainz, B., Jr., Carron, E., Vallespinos, M., Machado, H. L. (2016). Cancer Stem Cells and Macrophages: Implications in Tumor Biology and Therapeutic Strategies. Mediators Inflammation 2016, 9012369. doi: 10.1155/2016/9012369
Sala, A., Folco, G., Murphy, R. C. (2010). Transcellular biosynthesis of eicosanoids. Pharmacol. Rep. 62 (3), 503–510. doi: 10.1016/S1734-1140(10)70306-6
Satpathy, S. R., Jala, V. R., Bodduluri, S. R., Krishnan, E., Hegde, B., Hoyle, G. W., et al. (2015). Crystalline silica-induced leukotriene B4-dependent inflammation promotes lung tumour growth. Nat. Commun. 6, 7064. doi: 10.1038/ncomms8064
Serezani, C. H., Lewis, C., Jancar, S., Peters-Golden, M. (2011). Leukotriene B4 amplifies NF-kappaB activation in mouse macrophages by reducing SOCS1 inhibition of MyD88 expression. J. Clin. Invest. 121 (2), 671–682. doi: 10.1172/JCI43302
Sharma, R. K., Chheda, Z., Jala, V. R., Haribabu, B. (2013). Expression of leukotriene B(4) receptor-1 on CD8(+) T cells is required for their migration into tumors to elicit effective anti-tumor immunity. J. Immunol. 191 (6), 3462–3470. doi: 10.4049/jimmunol.1300967
Singh, J. K., Simoes, B. M., Howell, S. J., Farnie, G., Clarke, R. B. (2013). Recent advances reveal IL-8 signaling as a potential key to targeting breast cancer stem cells. Breast Cancer Res. 15 (4), 210. doi: 10.1186/bcr3436
Sjostrom, M., Johansson, A. S., Schroder, O., Qiu, H., Palmblad, J., Haeggstrom, J. Z. (2003). Dominant expression of the CysLT2 receptor accounts for calcium signaling by cysteinyl leukotrienes in human umbilical vein endothelial cells. Arterioscler. Thromb. Vasc. Biol. 23 (8), e37–e41. doi: 10.1161/01.ATV.0000082689.46538.DF
Sousa, A. R., Parikh, A., Scadding, G., Corrigan, C. J., Lee, T. H. (2002). Leukotriene-receptor expression on nasal mucosal inflammatory cells in aspirin-sensitive rhinosinusitis. N Engl. J. Med. 347 (19), 1493–1499. doi: 10.1056/NEJMoa013508
Srivatsa, S., Paul, M. C., Cardone, C., Holcmann, M., Amberg, N., Pathria, P., et al. (2017). EGFR in Tumor-Associated Myeloid Cells Promotes Development of Colorectal Cancer in Mice and Associates With Outcomes of Patients. Gastroenterology 153 (1), 178–190 e10. doi: 10.1053/j.gastro.2017.03.053
Steinbach, G., Lynch, P. M., Phillips, R. K., Wallace, M. H., Hawk, E., Gordon, G. B., et al. (2000). The effect of celecoxib, a cyclooxygenase-2 inhibitor, in familial adenomatous polyposis. N Engl. J. Med. 342 (26), 1946–1952. doi: 10.1056/NEJM200006293422603
Steinbrink, S. D., Pergola, C., Buhring, U., George, S., Metzner, J., Fischer, A. S., et al. (2010). Sulindac sulfide suppresses 5-lipoxygenase at clinically relevant concentrations. Cell Mol. Life Sci. 67 (5), 797–806. doi: 10.1007/s00018-009-0206-0
Subramanian, B. C., Majumdar, R., Parent, C. A. (2017). The role of the LTB4-BLT1 axis in chemotactic gradient sensing and directed leukocyte migration. Semin. Immunol. 33, 16–29. doi: 10.1016/j.smim.2017.07.002
Thompson, P. A., Khatami, M., Baglole, C. J., Sun, J., Harris, S. A., Moon, E. Y., et al. (2015). Environmental immune disruptors, inflammation and cancer risk. Carcinogenesis 36 (Suppl 1), S232–S253. doi: 10.1093/carcin/bgv038
Tian, W., Jiang, X., Sung, Y. K., Shuffle, E., Wu, T. H., Kao, P. N., et al. (2013). Blocking macrophage leukotriene b4 prevents endothelial injury and reverses pulmonary hypertension. Sci. Transl. Med. 5 (200), 200ra117. doi: 10.1126/scitranslmed.3006674
Tian, W., Rockson, S. G., Jiang, X., Kim, J., Begaye, A., Shuffle, E. M., et al. (2017). Leukotriene B4 antagonism ameliorates experimental lymphedema. Sci. Transl. Med. 9 (389). doi: 10.1126/scitranslmed.aal3920
Tian, W., Jiang, X., Tamosiuniene, R., Sung, Y. K., Qian, J., Dhillon, G., et al. (2019). Phenotypically Silent Bone Morphogenetic Protein Receptor 2 Mutations Predispose Rats to Inflammation-Induced Pulmonary Arterial Hypertension by Enhancing the Risk for Neointimal Transformation. Circulation 140 (17), 1409–1425. doi: 10.1161/CIRCULATIONAHA.119.040629
Tong, W. G., Ding, X. Z., Talamonti, M. S., Bell, R. H., Adrian, T. E. (2005). LTB4 stimulates growth of human pancreatic cancer cells via MAPK and PI-3 kinase pathways. Biochem. Biophys. Res. Commun. 335 (3), 949–956. doi: 10.1016/j.bbrc.2005.07.166
Tsopanoglou, N. E., Pipili-Synetos, E., Maragoudakis, M. E. (1994). Leukotrienes C4 and D4 promote angiogenesis via a receptor-mediated interaction. Eur. J. Pharmacol. 258 (1-2), 151–154. doi: 10.1016/0014-2999(94)90068-X
Uko, V., Thangada, S., Radhakrishnan, K. (2012). Liver disorders in inflammatory bowel disease. Gastroenterol. Res. Pract. 2012, 642923. doi: 10.1155/2012/642923
Uzonyi, B., Lotzer, K., Jahn, S., Kramer, C., Hildner, M., Bretschneider, E., et al. (2006). Cysteinyl leukotriene 2 receptor and protease-activated receptor 1 activate strongly correlated early genes in human endothelial cells. Proc. Natl. Acad. Sci. U. S. A. 103 (16), 6326–6331. doi: 10.1073/pnas.0601223103
Valencia, J. C., Egbukichi, N., Erwin-Cohen, R. A. (2019). Autoimmunity and Cancer, the Paradox Comorbidities Challenging Therapy in the Context of Preexisting Autoimmunity. J. Interferon Cytokine Res. 39 (1), 72–84. doi: 10.1089/jir.2018.0060
Wada, K., Arita, M., Nakajima, A., Katayama, K., Kudo, C., Kamisaki, Y., et al. (2006). Leukotriene B4 and lipoxin A4 are regulatory signals for neural stem cell proliferation and differentiation. FASEB J. 20 (11), 1785–1792. doi: 10.1096/fj.06-5809com
Walker, J. L., Loscalzo, J., Zhang, Y. Y. (2002). 5-Lipoxygenase and human pulmonary artery endothelial cell proliferation. Am. J. Physiol. Heart Circ. Physiol. 282 (2), H585–H593. doi: 10.1152/ajpheart.00003.2001
Walser, T., Cui, X., Yanagawa, J., Lee, J. M., Heinrich, E., Lee, G., et al. (2008). Smoking and lung cancer: the role of inflammation. Proc. Am. Thorac. Soc. 5 (8), 811–815. doi: 10.1513/pats.200809-100TH
Wan, M., Tang, X., Stsiapanava, A., Haeggstrom, J. Z. (2017). Biosynthesis of leukotriene B4. Semin. Immunol. 33, 3–15. doi: 10.1016/j.smim.2017.07.012
Wang, D., Dubois, R. N. (2010). Eicosanoids and cancer. Nat. Rev. Cancer 10 (3), 181–193. doi: 10.1038/nrc2809
Wang, M., Zhao, J., Zhang, L., Wei, F., Lian, Y., Wu, Y., et al. (2017). Role of tumor microenvironment in tumorigenesis. J. Cancer 8 (5), 761–773. doi: 10.7150/jca.17648
Waugh, D. J., Wilson, C. (2008). The interleukin-8 pathway in cancer. Clin. Cancer Res. 14 (21), 6735–6741. doi: 10.1158/1078-0432.CCR-07-4843
Wculek, S. K., Malanchi, I. (2015). Neutrophils support lung colonization of metastasis-initiating breast cancer cells. Nature 528 (7582), 413–417. doi: 10.1038/nature16140
Wei, S. C., Duffy, C. R., Allison, J. P. (2018). Fundamental Mechanisms of Immune Checkpoint Blockade Therapy. Cancer Discovery 8 (9), 1069–1086. doi: 10.1158/2159-8290.CD-18-0367
Wenger, F. A., Kilian, M., Bisevac, M., Khodadayan, C., von Seebach, M., Schimke, I., et al. (2002). Effects of Celebrex and Zyflo on liver metastasis and lipidperoxidation in pancreatic cancer in Syrian hamsters. Clin. Exp. Metastasis 19 (8), 681–687. doi: 10.1023/A:1021387826867
Werner, M., Pace, S., Czapka, A., Jordan, P. M., Gerstmeier, J., Koeberle, A., et al. (2020). Communication between human macrophages and epithelial cancer cell lines dictates lipid mediator biosynthesis. Cell Mol. Life Sci. doi: 10.1007/s00018-019-03413-w
Wong, R. S. Y. (2019). Role of Nonsteroidal Anti-inflammatory Drugs (NSAIDs) in Cancer Prevention and Cancer Promotion. Adv. Pharmacol. Sci. 2019, 3418975. doi: 10.1155/2019/3418975
Yao, H., Wang, H., Li, C., Fang, J. Y., Xu, J. (2018). Cancer Cell-Intrinsic PD-1 and Implications in Combinatorial Immunotherapy. Front. Immunol. 9, 1774. doi: 10.3389/fimmu.2018.01774
Yu, D., Zheng, W., Johansson, M., Lan, Q., Park, Y., White, E., et al. (2018). Overall and Central Obesity and Risk of Lung Cancer: A Pooled Analysis. J. Natl. Cancer Inst. 110 (8), 831–842. doi: 10.1093/jnci/djx286
Yun, C. W., Lee, S. H. (2018). The Roles of Autophagy in Cancer. Int. J. Mol. Sci. 19 (11), 3466. doi: 10.3390/ijms19113466
Zhang, Y. Y., Song, J., Zhao, Z., Yang, M., Chen, M., Liu, C., et al. (2002). Expression of 5-lipoxygenase in pulmonary artery endothelial cells. Biochem. J. 361 (Pt 2), 267–276. doi: 10.1042/0264-6021:3610267
Zhang, Y., Walker, J.L., Huang, A., Keaney, J.F., Clish, C.B., Serhan, C.N, et al. (2020). Single-cell transcriptome analysis reveals tumor immune microenvironment heterogenicity and granulocytes enrichment in colorectal cancer liver metastases. Cancer Lett. 470, 84–94. doi: 10.1016/j.canlet.2019.10.016
Zhao, S., Yao, K., Li, D., Liu, K., Jin, G., Yan, M., et al. (2019). Inhibition of LTA4H by bestatin in human and mouse colorectal cancer. EBioMedicine 44, 361–374. doi: 10.1016/j.ebiom.2019.05.008
Zhong, Z., Hu, Z., Jiang, Y., Sun, R., Chen, X., Chu, H., et al. (2016). Interleukin-11 promotes epithelial-mesenchymal transition in anaplastic thyroid carcinoma cells through PI3K/Akt/GSK3beta signaling pathway activation. Oncotarget 7 (37), 59652–59663. doi: 10.18632/oncotarget.10831
Ziyad, S., Iruela-Arispe, M. L. (2011). Molecular mechanisms of tumor angiogenesis. Genes Cancer 2 (12), 1085–1096. doi: 10.1177/1947601911432334
Keywords: cancer, leukotrienes, inflammation, tumor microenvironment, LTB4
Citation: Tian W, Jiang X, Kim D, Guan T, Nicolls MR and Rockson SG (2020) Leukotrienes in Tumor-Associated Inflammation. Front. Pharmacol. 11:1289. doi: 10.3389/fphar.2020.01289
Received: 18 May 2020; Accepted: 04 August 2020;
Published: 19 August 2020.
Edited by:
Emanuela Ricciotti, University of Pennsylvania, United StatesReviewed by:
Dipak Panigrahy, Harvard Medical School, United StatesOliver Werz, Friedrich Schiller University Jena, Germany
Copyright © 2020 Tian, Jiang, Kim, Guan, Nicolls and Rockson. This is an open-access article distributed under the terms of the Creative Commons Attribution License (CC BY). The use, distribution or reproduction in other forums is permitted, provided the original author(s) and the copyright owner(s) are credited and that the original publication in this journal is cited, in accordance with accepted academic practice. No use, distribution or reproduction is permitted which does not comply with these terms.
*Correspondence: Mark R. Nicolls, bW5pY29sbHNAc3RhbmZvcmQuZWR1; Stanley G. Rockson, cm9ja3NvbkBzdGFuZm9yZC5lZHU=
†These authors have contributed equally to this work