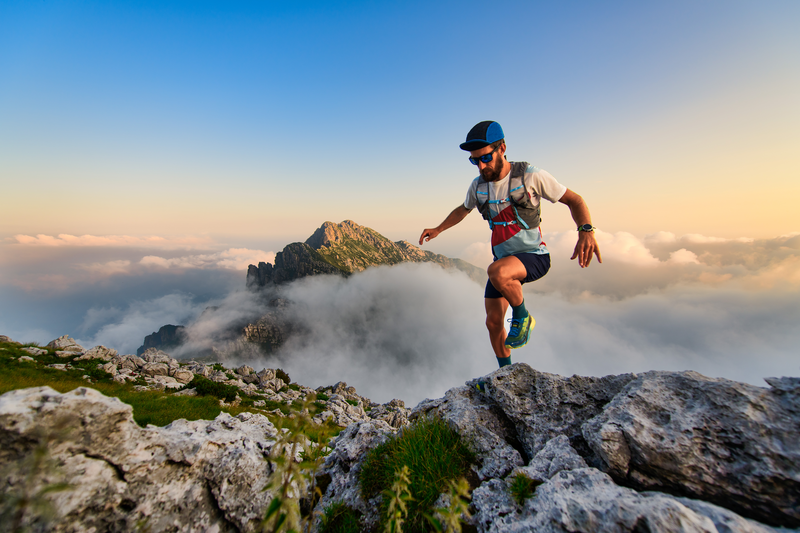
94% of researchers rate our articles as excellent or good
Learn more about the work of our research integrity team to safeguard the quality of each article we publish.
Find out more
ORIGINAL RESEARCH article
Front. Pharmacol. , 18 August 2020
Sec. Inflammation Pharmacology
Volume 11 - 2020 | https://doi.org/10.3389/fphar.2020.01266
This article is part of the Research Topic Novel Anti-inflammatory Approaches for Cystic Fibrosis Lung Disease: Identification of Molecular Targets and Design of Innovative Therapies View all 21 articles
Chronic inflammation is a deleterious process occurring in several pulmonary diseases; it is a driving force promoting tumorigenesis. By regulating local cyclic nucleotide concentration, cyclic nucleotide phosphodiesterases (PDE) govern important biological processes, including inflammation and proliferation. The aim of this study was to investigate the anti-inflammatory and anti-proliferative effects of NCS 613, a specific PDE4 inhibitor, on TNFα-treated human lung adenocarcinoma cell line (A549) and on human lung adenocarcinoma explants. PDE4 isoforms and inflammatory pathways mediated by p38 MAPK, ERK1/2, and IκBα were analyzed by Western blot and immunostainings. Proliferation were performed using [3H]-thymidine incorporation under different experimental conditions. TNFα-stimulation increased p38 MAPK phosphorylation and NF-κB translocation into the nucleus, which was abolished by NCS 613 treatment. Concomitantly, NCS 613 restores IκBα detection level in human adenocarcinoma. An IC50 value of 8.5 μM was determined for NCS 613 on anti-proliferative properties while ERK1/2 signaling was down-regulated in A549 cells and lung adenocarcinoma explants. These findings shed light on PDE4 signaling as a key regulator of chronic inflammation and cancer epithelial cell proliferation. It suggests that PDE4 inhibition by NCS 613 represent potential and interesting strategy for therapeutic intervention in tackling chronic inflammation and cell proliferation.
Chronic inflammation, which is a deleterious process occurring in inflammatory respiratory diseases, is believed to be a tumor promoter in cancer induction (Lee et al., 2009; Balkwill and Mantovani, 2010; Phillips, 2020). Evidence suggests that recurrent injury and inflammation result in genetic alterations that predispose to lung cancer (Pikarsky and Ben-Neriah, 2006). NF-кB and p38 MAPK pathways play important role in human inflammatory diseases (COPD, rheumatoid arthritis…) and cancer (Koutras et al., 2009; Wong, 2009; Luangdilok et al., 2011). NF-кB dimers binding to target promoters lead to pro-inflammatory genes transcriptions and regulation of apoptosis and proliferation (Jeon et al., 2010). NF-κB activation is prevented by IκBα, an inhibitory protein which maintains NF-кB in an inactive state in the cytoplasm (Kamata et al., 2010). Downstream of surface membrane receptor activation, cyclic nucleotide phosphodiesterases (PDE) which encompass 11 families play a pivotal role in cAMP and cGMP signaling (Lugnier, 2006; Conti and Beavo, 2007). These enzymes hydrolyze cyclic nucleotides as a feedback mechanism for rapidly returning local nucleotide concentration to basal levels (Keravis et al., 2012; Maurice et al., 2014; Baillie et al., 2019; Lugnier et al., 2020). By regulating local cyclic nucleotides levels, PDEs contribute to their compartmentalization and govern number of biological processes, including inflammation and proliferation (Growcott et al., 2006; Sosroseno and Sugiatno, 2008, Abusnina et al., 2011a; Abusnina et al., 2011b; Alhosin, et al., 2011; Keravis, et al., 2011, Yougbare et al., 2013; Li et al., 2018; Baillie et al., 2019). Specific cAMP hydrolyzing phosphodiesterases (PDE4) have been shown to be involved in the control of inflammatory responses (Houslay, 2010). Inflammation is strongly correlated to lower intracellular cAMP levels. PDE4 implication has been reported in lung diseases related to chronic inflammation such as smoking-induced lung injury, fibrosis, asthma and COPD (Dunkern et al., 2007; Udalov et al., 2010; Diamant and Spina, 2011; Yougbare et al., 2014; Phillips, 2020). Inhibition of PDE4 activity causes elevation of intracellular cAMP levels and subsequent downregulation of a variety of inflammatory cell signaling (Yougbare et al., 2011). NCS 613 is a potent PDE4 inhibitor, which is more selective for the PDE4C subtype (IC50 = 1.4 nM) (Figure 1E). Compared other PDE4 inhibitors such as pentoxifylline and denbufylline, NCS 613 was the most potent and effective molecule in inhibiting both basal and LPS-induced TNFα secretion from leucocytes of lupus patients (Keravis et al., 2012). We previously showed that in vivo administration of NCS 613, a new and potent PDE4 inhibitor, reduced neutrophil recruitment in LPS-treated mouse bronchi and exhibits anti-inflammatory effects by decreasing TNFα secretion in guinea pig (Boichot et al., 2000). Interestingly, NCS 613 did not stimulate gastric acid secretion suggesting that this compound may produce gastrointestinal side effects (Boichot et al., 2000). Thus, PDE4 isozymes represent attractive targets for inflammation and cancer (Keravis et al., 2012; Li et al., 2018; Baillie et al., 2019; Hsien Lai et al., 2020). The above observations prompted us to investigate whether or not cellular signaling involved in inflammation and proliferation processes may be prevented by PDE4 inhibition and local increase in cAMP concentration. This study aimed to investigate the anti-inflammatory and antiproliferative effects of NCS 613 on human lung adenocarcinoma cell line (A549) and cultured human lung adenocarcinoma explants.
The study was approved by our institutional Ethics Committee (Protocol number CRC 05-088 S1R2). Human lung explants were obtained from 4 patients aged between 50-60 years undergoing surgery for lung carcinoma resection. Tissues samples were collected from fresh lobectomy and transported to the laboratory in physiological Krebs’ solution (Sosroseno and Sugiatno, 2008). Human lung adenocarcinoma cell line (A549 cells) was from Abcam (# ab7910). NCS 613 (patent FR0601958) was given by J.J. Bourguignon and C. Lugnier (Faculty of pharmacy, Strasbourg). PDE antibodies used for Western blot analysis and immuno-staining were from FabGennix Inc. Anti-Phospho and total ERK1/2, anti-IκBα, anti-p65-NF-κB, Anti-phospho, and total p38-MAPK were from Cell Signaling Technology. [3H]-thymidine (250 μCi) was obtained from New England Nuclear.
The adenocarcinoma human alveolar basal epithelial cells, A549 cells (1x 105), were allowed growth for 24 h in T-75 culture flask, as previously described (Giard et al., 1973), in RPMI culture medium supplemented with 0.3% penicillin (100 IU/ml) and streptomycin (0.1 mg/ml) in presence of 1% fetal bovine serum at 37°C under 5% CO2. After 6 h starving period (without fetal bovine serum), cells were stimulated with TNFα to induce inflammation and treated with NCS 613 for 48 h under the following experimental conditions: control, +10 μM NCS 613, + 10 ng/ml TNFα, and +TNFα + NCS 613.
A549 cells were also cultured in 24-well plate in the presence of increasing NCS 613 concentrations of 0, 1, 2.5, 5, 7.5, 10, and 30 μM for 48 h. [3H]-thymidine incorporation was performed in triplicate for a 24 h period with 1 μCi tritiated thymidine. Harvested cells were lysed with cold 20% Trichloroacetic Acid (TCA) followed by DNA precipitation with 96% ethanol on Whatman paper. Radioactivity was quantified with β-counter using 5 ml scintillation liquid. IC50 value for NCS 613 was determined on cell proliferation as assessed by [3H]-thymidine incorporation (Denton, 1998).
After removal of connective tissues, lung adenocarcinoma explants were cultured in 6-wells culture plates containing RPMI as previously described for pulmonary tissue culture (Guibert et al., 2005). Explants were cultured in either control condition or treated with 1, 3, or 10 μM NCS 613. Tissues and harvested cells were homogenized with a Polytron in Radio-Immuno-Precipitation Assay buffer (RIPA) and supernatant aliquots were stored at -80°C until use.
Proteins (25 μg) from lung adenocarcinoma and A549 cells lysates were subjected to Western blot analysis. Briefly, protein samples were electrophoresed on 10% SDS polyacrylamide gels and electro-transferred onto Polyvinylidene Difluoride (PVDF) membranes. Immunodetection was carried out with the following antibodies: anti-PDE4A, anti-PDE4B, anti-PDE4C, anti-PDE4D, anti-IκBα, anti-p38 MAPK, anti-ERK1/2 and anti-GAPDH. Immobilized antigens were detected by chemiluminescence using horseradish peroxidase-conjugated secondary antibodies, an ECL kit and autoradiography films.
Smears were made from harvested A549 cells, delineated with Dako Pen and fixed in 4%.
Paraformaldehyde (PFA) overnight before immuno-staining. After rinsing in TBS buffered (50 mM Tris, 150 mM NaCl, pH 7.6) and permeabilization with 0.1% Triton in PBS, smears were saturated with PBS + 4% BSA for 1 h at room temperature. Immuno-stainings were performed using specific antibodies directed against PDE4B, PDE4C, and NF-κB, and subsequently revealed by secondary antibodies coupled to either Alexa-488 or Alexa-654 fluorescent probe. Cell nuclei were labeled with DAPI (1 μM). Slides were mounted using Vectashield mounting medium and observed with a Leica microscope at 40X and 60X magnifications. Images were captured at the same magnification and exposure time allowing fluorescence comparison between the various experimental conditions.
Results are expressed as means ± S.E.M. with n indicating the number of experiments.
Statistical analyses were performed using a one-way ANOVA followed by a Bonferroni post-test. Differences were considered statistically significant when p<0.05.
Quantitative analysis of Western blot results showed that NCS 613 prevents PDE4A 70 kDa expression in A549 cells while TNFα significantly increases it. In these cells, the specific PDE4 inhibitor overcomes the overexpression of PDE4A (Figure 1A). Furthermore, long term inhibition of PDE4 by NCS 613 leads to induction of PDE4B 96 kDa in adenocarcinoma A549 cell line (Figure 1B). PDE4B 96 kDa expression was undetectable following TNFα stimulation and its expression increased upon NCS 613 treatment (Figure 1B). Conversely, the PDE4B 66 kDa isoform was increased upon TNFα treatment (Figure 1C). The PDE4C 66 kDa isoform on the other hand was increased in presence of 10 μM NCS 613 (Figures 1D, E). However, we did not detect PDE4D isoforms by Western blot in A549 cell line. These results suggest that cAMP accumulation subsequent to PDE4 inhibition induces a shift in isoform transcription and biosynthesis. The data also revealed that TNFα stimulation significantly abolished PDE4B 96 kDa while it increased PDE4A 70 kDa and PDE4B 66 kDa detection in A549 cells, suggesting that these later isoforms could be involved in TNFα signaling (Figures 1A, C). As expected, NCS 613 treatment overcame the TNFα-induced PDE4A 70 kDa and PDE4B 66 kDa increases which return to control level, hence indicating that this compound can interfere with inflammation signaling pathways.
Figure 1 Detection of PDE4A, PDE4B, and PDE4C expressions in control and TNFα-stimulated A549 cells following NCS 613 treatment. A549 cells were stimulated with TNFα to induce inflammation and treated with NCS 613 for 48 h under the following experimental conditions: control, +10 μM NCS 613, + 10 ng/ml TNFα, and +TNFα + NCS 613. Relative protein expression levels were calculated following digitalization and image analysis from 3 independent experiments. (A) NCS 613 prevents and TNFα increases PDE4A 70 kDa overexpression; (B) NCS 613 induces PDE4B 96 kDa expression in A549 cell line; (C) TNFα stimulation induces PDE4B 66 kDa upregulation which is decreased with NCS 613 treatment; (D) PDE4C 66 kDa increases upon NCS 613 treatment; (E) representative immuno-reactive bands revealed by Western blot analysis (n=3). Unpaired Student’s t-test. Mean ± SEM. **p < 0.01 and ***p < 0.001.
Immuno-cytofluorescent staining confirms that NCS 613 treatment increased basal nuclear PDE4B and PDE4C expression (Figure 2). PDE4B 96 kDa could correspond to the nuclear isoform since this protein was only upregulated with NCS 613 treatment (Figure 2C). Immunostaining consistently showed that TNFα stimulation significantly increased PDE4B expression in A549 cells (Figure 2D). PDE4B 96 kDa was not expressed in A549 TNFα-stimulated cells (see Figure 1B), the 66 kDa isoform could correspond to the up-regulated cytoplasmic isoform. Interestingly, 10 μM NCS 613 treatment decreased PDE4B protein expression in TNFα-stimulated cells (Figure 2E). Complementary experiments were performed to delineate cellular location of PDE4C. Concomitant PDE4C detection using a specific monoclonal antibody and DAPI counter-staining demonstrates the nuclear localization of this isoform (Figures 2F, G). Lower panels show that NCS 613 treatments (5 and 10 μM) increased the detection of PDE4C isoform in A549 cells (Figures 2 G–I). Together these results indicate that NCS 613 treatment modulates the compartmentalized expression of PDE4 isoforms.
Figure 2 PDE4B and PDE4C immuno-cyto-staining. (A–E) Detection of PDE4B isoforms in A549 cells under control and TNFα treated conditions, in the absence or presence of NCS 613. (A) Negative control showing anti-PDE4B specificity; (B) PDE4B basal level in A549 cells; (C) NCS 613 increases PDE4B expression; (D) TNFα stimulation greatly increases PDE4B expression which is reduce by NCS 613 (E). (F–I) Detection of PDE4C in A549 cells. (F) Negative control and (G) Basal PDE4C location; (H) and (I) NCS 613 treatments increase nuclear PDE4C in A549 cells. Data are representative of 3 independent experiments. Shown are merged images [x60 (A–E) and x40 (F–I) magnification] of DAPI staining (red) and anti-rabbit-Alexa Fluor 488 (green) recognizing primary anti-PDE4B or anti-PDE4C antibody (green).
After 72 h culture, phospho-p38 MAPK was detected in both control and TNFα-treated A549 cells (Figure 3A), whereas NCS 613 treatment abolished p38 MAPK phosphorylation demonstrating a net effect of this compound. TNFα stimulation significantly increases p38 MAPK phosphorylation facilitating down-stream effectors activation. NCS 613 treatment abolishes the TNFα-induced p38 MAPK phosphorylation, while the total p38 MAPK expression level remains unchanged (Figure 3A). Since it has been reported that p38 MAPK phosphorylation induces NF-κB activation (Morin et al., 2008; Smith et al., 2010; Sigala et al., 2011), antibodies against the NF-κB p65-subunit were used to detect NF-κB translocation into the nucleus. NCS 613 treatment reduces NF-κB basal detection in these cells (Figure 3B). In contrast, TNFα stimulation enhances of NF-κB translocation into the nucleus, while NCS 613 treatment counteracted the effects of TNFα on p65-NF-κB translocation. Together, these results indicate that intracellular cAMP level, which is increased during NCS 613 treatment, modulates p38 MAPK phosphorylation and downstream nuclear translocation of p65-subunit of NF-κB.
Figure 3 Effect of NCS 613 on p38 MAPK phosphorylation and NF-κB nuclear translocation upon TNFα stimulation. (A) TNFα increases and NCS 613 reduces p38 MAPK phosphorylation in A549 cells; (B) NCS 613 prevents NF-κB translocation into nucleus while TNFα stimulation greatly increases NF-κB p65-subunit translocation into nucleus. Shown are merged images (60x magnification) of DAPI staining and anti-mouse Alexa 654 recognizing primary p65-NF-κB antibody (n=3). Unpaired Student’s t-test. Mean ± SEM. **p < 0.01 and ***p < 0.001.
Increasing evidences have revealed that tissue inflammation is a recurrent component in cancer (Balkwill and Mantovani, 2010; Smith et al., 2010). To assess the putative anti-inflammatory effects of NCS 613, IκBα expression was used as a lung inflammation marker (lower detection indicates larger inflammation). IκBα was detected in fresh human lung parenchyma (positive control, Figure 4) whereas IκBα was undetectable in untreated (control) lung adenocarcinoma explants cultured for 72, which is correlated with an increased inflammation process. In contrast, NCS 613 (at 3 and 10 μM) rescued IκBα detection in a concentration-dependent manner, which could correlate with a lower inflammation status of the tissues.
Figure 4 Concentration dependent effect of NCS 613 treatment on IκBα detection in adenocarcinoma explants. IκBα was detected in fresh human lung parenchyma. After a 72 h culture period, IκBα was undetectable in control adenocarcinoma explants suggesting a high inflammation level. Three and 10 µM NCS 613 treatment reestablished IκBα detection level (n= 4). Unpaired Student’s t-test. Mean ± SEM. **p < 0.01 and ***p < 0.001.
In the absence of treatment, A549 cells proliferate and become highly confluent (Figure 5A). Interestingly, NCS 613 treatment opposed this effect on cells proliferation and induced cells apoptosis (Figures 5B, C). DMSO up to 0.3 ‰, used as vehicle did not exhibit any toxicity toward A549 cells (Figures 4D, E). Of note, NCS 613 (2.5–30 μM) significantly decreased [3H]-thymidine incorporation, hence correlating with a lower cell proliferation rate. Moreover, inhibition curve displays an anti-proliferative effect of NCS 613 with an IC50 value of 8.5 μM. NCS 613 treatment reduces ERK 1/2 phosphorylation as well as total ERK 1/2 in A549 cells (Figures 6A, B) as well as in human lung adenocarcinoma explants (Figures 6C, D) attesting that this pathway is likely involved in the anti-proliferative effect of this compound. Several putative pathways involved in inflammatory responses and cell proliferation are likely modulated by NCS 613. As shown in the present results, TNFα induces PDE4B up-regulation in order to hydrolyze cAMP and activates p38 MAPK and NF-κB pathways, which in turn leads to IκBα degradation. NCS 613 on the other hand increases cAMP levels and thus prevents p38 MAPK phosphorylation. NCS 613 can also inhibit cell proliferation by a down-regulation of ERK1/2 signaling. The sustained inhibition of PDE4 by NCS 613 induces the synthesis of novel isoforms as a feedback mechanism to modulate cAMP levels.
Figure 5 Anti-proliferative effect of NCS 613 on A549 cells. (A) Control: A549 cells display rapid proliferation rate toward confluency. (B, C) A549 cells treated with either 5 µM or 10 µM NCS 613 for 48 h results in lower density and higher apoptosis. Plates are representative of three independent experiments; (D) Bar histogram showing [3H]-thymidine incorporation into DNA. Gradual (2.5–30 µM) NCS 613 treatment significantly decreases A549 cells proliferation; (E) Concentration dependent inhibition curve displaying the antiproliferative effects of NCS 613 from which an IC50 value of 8.5 µM was calculated. Unpaired Student’s t-test. Mean ± SEM. ***p < 0.001.
Figure 6 Effect of NCS 613 treatment on ERK1/2 phosphorylation. (A, B) Concentration dependent effect of NCS 613 on the phosphorylation level of ERK1/2 as a function of experimental conditions (n=3); (C, D) Concentration dependent effect of NCS 613 on phospho-ERK1/2 detection in cultured lung adenocarcinoma explants (n=4). Unpaired Student’s t-test. Mean ± SEM. *p < 0.05, **p < 0.01 and ***p < 0.001.
Chronic inflammation is a hallmark of pulmonary diseases, which can lead to emphysema and airways hyperresponsiveness. In the present study, we were able to delineate the role of PDE4 in TNFα-induced inflammation in A549 cells in which PDE4A 70 kDa and PDE4B 66 kDa were significantly up-regulated but not PDE4C and PDE4D. This upregulation of PDE4 isoforms, downstream of TNFα-receptor activation, facilitates cAMP breakdown and activation of inflammatory effectors. Under these conditions, A549 cells consistently expressed higher cytoplasmic levels of PDE4B as revealed by immuno-staining. NCS 613 treatment, which inhibits PDE4 activity, restored intracellular cAMP levels, resulting in an opposite effect on inflammatory markers. In a previous report, LPS-induced TNFα secretion was shown to be significantly reduced in macrophages from bronchi-alveolar fluid of PDE4B-deficient mice (Jin et al., 2005). Comparing the effect rolipram, NCS 613 and RP 73401, or the cell permeable analogue N6 -2’-O-dibutyryladenosine 3’,5’-cyclic monophosphate (db- cAMP) on histamine release by mast cells from diabetic rats, we observed that these molecules led to a decrease of histamine secretion in vitro (Barreto et al., 2004). Importantly, the effectiveness of either NCS 613 or db cAMP in inhibiting antigen-induced degranulation is comparable in both normal and diabetic mast cells. Our current data suggest that the PDE4A 70 kDa and PDE4B 66 kDa isoforms are likely involved in TNFα signaling, which is consistent with the conclusion of a recent study showing that the induction of PDE4B is required for Toll-like receptor signaling in monocytes and macrophages (Jin et al., 2010).
Herein, the PDE4B 96 kDa increase in A549 cells by NCS 613 treatment, suggests that this isoform is likely involved in the regulation of cAMP metabolism. This is also supported by the upregulation of nuclear PDE4C in A549 cells. Moreover, other reports revealed that sustained increased in intracellular cAMP enhanced PDE4A/4B gene expressions in U937 cells as well as l PDE4B/4D in human myometrial cells (Dunkern et al., 2007). Interestingly, it was previously reported that PDE4B2 was upregulated by IL-1β at mRNA and protein levels from human myometrial cells through a prostaglandin E2- and cyclic adenosine 3’, 5’-monophosphate-dependent pathway (Oger et al., 2002). PDE4 activity and expression in cultured pulmonary microvascular endothelial cells from rat are activated by a PKA in situ phosphorylation in short-term and induced in long-term via increases of intracellular cAMP concentrations (Zhu et al., 2004). Our work showed the specificity and functions of PDE4B and PDE4C isoforms in which 96 kDa and 66 kDa proteins respectively regulate cAMP level in A549 cells. PDE4 compartmentalization in cells tightly regulates cAMP level underpinning a complex cAMP signaling network (Houslay, 2010; Yougbare et al., 2013). Two pathways involved in the typical inflammatory response were assessed in the present study. The stress kinase p38 MAPK is thought to regulate NF-κB translocation to nuclei (Moriyuki et al., 2009; Morin et al., 2010) and TNFα stimulation increases p38 MAPK phosphorylation. In addition increasing evidence suggested that PDE4 inhibitor are negative modulator of p38 phosphorylation (Kwak et al., 2005; Yougbare et al., 2011). NCS 613 totally abolishes p38 MAPK phosphorylation and reduces NF-κB translocation to the nucleus. Consequently, the use of NCS 613 opposes to p38 MAPK activation by stress stimuli (TNFα, LPS) and its phosphorylation leading to rapid activation of NF-κB (Morin et al., 2010). Consistent with these observations, NCS 613 treatment stabilizes IκBα binding to NF-κB and prevents nuclear translocation of the latter. Under pro-inflammatory conditions, IκBα is usually phosphorylated by IκK and then ubiquitinated, leading to its degradation into proteasomes, and lower cytoplasmic detection and NF-κB translocation to the nuclei (Houslay and Adams, 2010; Kamata et al., 2010). In cultured human lung adenocarcinoma explants IκBα was undetectable in control (untreated) conditions whereas NCS 613 was able to restore IκBα detection in a concentration-dependent manner. Thus, IκBα protection down regulates NF-κB activation which have important implications in lung cancer prevention and immunotherapy (Lin et al., 2010). Our current data suggest that NCS 613 may have potent anti-inflammatory properties in A549 cells and lung carcinoma explants.
Microscopic observation of A549 cells under specific PDE4 inhibitor treatment provides the first evidence that NCS 613 can also markedly affect cell proliferation. A549 cells death was observed with Trypan blue staining during cells count. Trypan blue, a vital stain used to selectively color dead cells in blue reveals A549 cells death mediated by NCS 613 treatment. Other compounds like CC-8075 and CC-8062 are PDE4 inhibitors that have been shown an anti-proliferative effect on various cell lines by increasing cAMP (Koutras et al., 2009; Mouratidis et al., 2009; Hsien Lai et al., 2020). The [3H]-thymidine incorporation proliferation assay confirms that NCS 613 decreases cell proliferation concomitantly with lower detection of inflammatory markers suggesting the contribution of the latter in cancer progression. Several studies have used mouse cancer models and have provided direct genetic evidence for the critical role of NF-κB in carcinogenesis (Pikarsky and Ben-Neriah, 2006). With an IC50 value of 8.5 μM on cell proliferation, NCS 613 reduces cell division and likely induces apoptosis in malignant cells, which is corroborated by the downregulation of phospho- ERK1/2 in A549 cells. This observation could be correlated to a recent finding suggesting a predominant role of PDE4B in controlling molecular pathophysiological processes involved in the proliferation of human lung fibroblasts (Selige et al., 2010). The mechanism by which cAMP induced apoptosis in A549 cells remains to be established.
The present data highlight the role of PDE4B in controlling inflammatory responses and cell proliferation pathways. NCS 613 targets PDE4 and, indirectly, p38 MAPK as well as NF-κB and ERK1/2 signaling, leading to a down-regulation of inflammation in both the human A549 cell line and lung adenocarcinoma explants. NCS 613 thus represents a potent anti-inflammatory compound, which did not induce gastrointestinal side effects at 10 mg/kg i.v. (Boichot et al., 2000), which may be useful in the treatment of chronic inflammatory diseases and cancer prevention in pre-clinical studies. Further studies are needed to assess whether NCS 613 induces apoptosis in A549 and lung adenocarcinoma.
The raw data supporting the conclusions of this article will be made available by the authors, without undue reservation.
The studies involving human participants were reviewed and approved by Protocol number CRC 05-088 S1R2. The patients/participants provided their written informed consent to participate in this study.
IY designed and performed all the experiments, analyzed and interpreted data, and prepared and revised the manuscript. LB, AA, and YS conducted some experiments and prepared the manuscript. CM and TK provided guidance for study design and data interpretation. CL contributed to study design and data interpretation, as well as manuscript preparation. As the principle investigator, ER supervised study design, data interpretation, and manuscript preparation.
This work was supported by transition grants from the FMSS and CRCHUS. IY and CM were recipients of PhD-studentships from the European Doctorate College EDC (Strasbourg) and NSERC of Canada, respectively.
The authors declare that the research was conducted in the absence of any commercial or financial relationships that could be construed as a potential conflict of interest.
The authors thank Drs Marco Sirois and Chantal Sirois for their help in patient recruitment.
The authors thank Dr. Edmond Rizcallah and the members of the pathology laboratory for their technical support.
Abusnina, A., Alhosin, M., Keravis, T., Muller, C. D., Fuhrmann, G., Bronner, C., et al. (2011a). Down-regulation of cyclic nucleotide phosphodiesterase PDE1A is the key event of p73 and UHRF1 deregulation in thymoquinone-induced acute lymphoblastic leukemia cell apoptosis. Cell Signal 23 (1), 152–160. doi: 10.1016/j.cellsig.2010.08.015
Abusnina, A., Keravis, T., Yougbare, I., Bronner, C., Lugnier, C. (2011b). Anti-proliferative effect of curcumin on melanoma cells is mediated by PDE1A inhibition that regulates the epigenetic integrator UHRF1. Mol. Nutr. Food Res. 55 (11), 1677–1689. doi: 10.1002/mnfr.201100307
Alhosin, M., Sharif, T., Mousli, M., Etienne-Selloum, N., Fuhrmann, G., Schini-Kerth, V. B., et al. (2011). Down-regulation of UHRF1, associated with re-expression of tumor suppressor genes, is a common feature of natural compounds exhibiting anti-cancer pprperties. J. Exp. Clin. Cancer. Res. 30 (1), 41. doi: 10.1186/1756-9966-30-41
Baillie, G. S., Tejeda, G. S., Kelly, M. P. (2019). Therapeutic targeting of 3’,5’-cyclic nucleotide phosphodiesterases: inhibition and beyond. Nat. Rev. Drug Discovery 18 (10), 770–796. doi: 10.1038/s41573-019-0033-4
Balkwill, F., Mantovani, A. (2010). Cancer and inflammation: implications for pharmacology and therapeutics. Clin. Pharmacol. Ther. 87 (4), 401–406. doi: 10.1038/clpt.2009.312
Barreto, E. O., Carvalho, V. F., Lagente, V., Lugnier, C., Cordeiro, R. S., Martins, M. A., et al. (2004). Increased levels of cyclic adenosine monophosphate contribute to the hyporesponsiveness of mast cells in alloxan diabetes. Int. Immunopharmacol. 4 (6), 755–762. doi: 10.1016/j.intimp.2004.03.001
Boichot, E., Wallace, J. L., Germain, N., Corbel, M., Lugnier, C., Lagente, V., et al. (2000). Anti-inflammatory activities of a new series of selective phosphodiesterase 4 inhibitors derived from 9-benzyladenine. J. Pharmacol. Exp. Ther. 292 (2), 647–653.
Conti, M, Beavo, J. (2007). Biochemistry and physiology of cyclic nucleotide phosphodiesterases: essential components in cyclic nucleotide signaling. Annu. Rev. Biochem. 76, 481–511. doi: 10.1146/annurev.biochem.76.060305.150444
Denton, C. P. (1998). Leucine incorporation and thymidine incorporation. Methods Mol. Biol. 79, 169–177. doi: 10.1385/0-89603-448-8:169
Diamant, Z., Spina, D. (2011). PDE4-inhibitors: a novel, targeted therapy for obstructive airways disease. Pulm. Pharmacol. Ther. 24 (4), 353–360. doi: 10.1016/j.pupt.2010.12.011
Dunkern, T. R., Feurstein, D., Rossi, G. A., Sabatini, F., Hatzelmann, A. (2007). Inhibition of TGF-beta induced lung fibroblast to myofibroblast conversion by phosphodiesterase inhibiting drugs and activators of soluble guanylyl cyclase. Eur. J. Pharmacol. 572 (1), 12–22. doi: 10.1016/j.ejphar.2007.06.036
Giard, D. J., Aaronson, S. A., Todaro, G. J., Arnstein, P., Kersey, J. H., Dosik, H., et al. (1973). In vitro cultivation of human tumors: establishment of cell lines derived from a series of solid tumors. J. Natl. Cancer Inst. 51 (5), 1417–1423. doi: 10.1093/jnci/51.5.1417
Growcott, E. J., Spink, K. G., Ren, X., Afzal, S., Banner, K. H., Wharton, J. (2006). Phosphodiesterase type 4 expression and anti-proliferative effects in human pulmonary artery smooth muscle cells. Respir. Res. 7, 9. doi: 10.1186/1465-9921-7-9
Guibert, C., Savineau, J. P., Crevel, H., Marthan, R., Rousseau, E. (2005). Effect of short-term organoid culture on the pharmaco-mechanical properties of rat extra- and intrapulmonary arteries. Br. J. Pharmacol. 146 (5), 692–701. doi: 10.1038/sj.bjp.0706379
Houslay, M. D., Adams, D. R. (2010). Putting the lid on phosphodiesterase 4. Nat. Biotechnol. 28 (1), 38–40. doi: 10.1038/nbt0110-38
Houslay, M. D. (2010). Underpinning compartmentalised cAMP signalling through targeted cAMP breakdown. Trends Biochem. Sci. 35 (2), 91–100. doi: 10.1016/j.tibs.2009.09.007
Hsien Lai, S., Zervoudakis, G., Chou, J., Gurney, M. E., Quesnelle, K. M. (2020). PDE4 subtypes in cancer. Oncogene 39 (19), 3791–3802. doi: 10.1038/s41388-020-1258-8
Jeon, K., II, Xu, X., Aizawa, T., Lim, J. H., Jono, H., Kwon, D. S., et al. (2010). Vinpocetine inhibits NF-kappaB-dependent inflammation via an IKK-dependent but PDE-independent mechanism. Proc. Natl. Acad. Sci. U.S.A. 107 (21), 9795–9800. doi: 10.1073/pnas.0914414107
Jin, S. L., Lan, L., Zoudilova, M., Conti, M. (2005). Specific role of phosphodiesterase 4B in lipopolysaccharide-induced signaling in mouse macrophages. J Immunol. 175 (3), 1523–1531. doi: 10.4049/jimmunol.175.3.1523
Jin, S. L., Goya, S., Nakae, S., Wang, D., Bruss, M., Hou, C., et al. (2010). Phosphodiesterase 4B is essential for T(H)2-cell function and development of airway hyperresponsiveness in allergic asthma. J. Allergy Clin. Immunol. 126 (6), 1252–9 e12. doi: 10.1016/j.jaci.2010.08.014
Kamata, H., Tsuchiya, Y., Asano, T. (2010). IkappaBbeta is a positive and negative regulator of NF-kappaB activity during inflammation. Cell Res. 20 (11), 1178–1180. doi: 10.1038/cr.2010.147
Keravis, T., Monneaux, F., Yougbare, I., Gazi, L., Bourguignon, J. J., Muller, S., et al. (2012). Disease progression in MRL/lpr lupus-prone mice is reduced by NCS 613, a specific cyclic nucleotide phosphodiesterase type 4 (PDE4) inhibitor. PloS One 7 (1), e28899. doi: 10.1371/journal.pone.0028899
Koutras, A., Giannopoulou, E., Kritikou, I., Antonacopoulou, A., Evans, T. R., Papavassiliou, A. G., et al. (2009). Antiproliferative effect of exemestane in lung cancer cells. Mol. Cancer 8, 109. doi: 10.1186/1476-4598-8-109
Kwak, H. J., Song, J. S., Heo, J. Y., Yang, S. D., Nam, J. Y., Cheon, H. G. (2005). Roflumilast inhibits lipopolysaccharide-induced inflammatory mediators via suppression of nuclear factor-kappaB, p38 mitogen-activated protein kinase, and c-Jun NH2-terminal kinase activation. J. Pharmacol. Exp. Ther. 315 (3), 1188–1195. doi: 10.1124/jpet.105.092056
Lee, G., Walser, T. C., Dubinett, S. M. (2009). Chronic inflammation, chronic obstructive pulmonary disease, and lung cancer. Curr. Opin. Pulm. Med. 15 (4), 303–307. doi: 10.1097/MCP.0b013e32832c975a
Li, H., Zuo, J., Tang, W. (2018). Phosphodiesterase-4 Inhibitors for the Treatment of Inflammatory Diseases. Front. Pharmacol. 9, 1048. doi: 10.3389/fphar.2018.01048
Lin, Y., Bai, L., Chen, W., Xu, S. (2010). The NF-kappaB activation pathways, emerging molecular targets for cancer prevention and therapy. Expert Opin. Ther. Targets 14 (1), 45–55. doi: 10.1517/14728220903431069
Luangdilok, S., Box, C., Harrington, K., Rhys-Evans, P., Eccles, S. (2011). MAPK and PI3K signalling differentially regulate angiogenic and lymphangiogenic cytokine secretion in squamous cell carcinoma of the head and neck. Eur. J. Cancer 47 (4), 520–529. doi: 10.1016/j.ejca.2010.10.009
Lugnier, C. (2006). Cyclic nucleotide phosphodiesterase (PDE) superfamily: a new target for the development of specific therapeutic agents. Pharmacol. Ther. 109 (3), 366–398. doi: 10.1016/j.pharmthera.2005.07.003
Lugnier, C., Meyer, A., Tahla, S., Geny, B. (2020). Cyclic nucleotide phosphodiesterases: new targets in the metabolic syndrome? Pharmacol. Ther. 208, 107475. doi: 10.1016/j.pharmthera.2020.107475
Maurice, D. A, Ke, H., Ahmad, F., Wang, Y., Chung, J., Manganiello, V. (2014). Advances in targeting cyclic nucleotide phosphodiesterases. Nat. Rev. Drug. Discov. 13 (4), 290–314. doi: 10.1038/nrd4228
Morin, C., Sirois, M., Echave, V., Gomes, M. M., Rousseau, E. (2008). EET displays anti-inflammatory effects in TNF-alpha stimulated human bronchi: putative role of CPI-17. Am. J. Respir. Cell Mol. Biol. 38 (2), 192–201. doi: 10.1165/rcmb.2007-0232OC
Morin, C., Sirois, M., Echave, V., Albadine, R., Rousseau, E. (2010). 17,18-epoxyeicosatetraenoic acid targets PPARgamma and p38 mitogen-activated protein kinase to mediate its anti-inflammatory effects in the lung: role of soluble epoxide hydrolase. Am. J. Respir. Cell Mol. Biol. 43 (5), 564–575. doi: 10.1165/rcmb.2009-0155OC
Moriyuki, K., Sekiguchi, F., Matsubara, K., Nishikawa, H., Kawabata, A. (2009). Proteinase-activated receptor-2-triggered prostaglandin E(2) release, but not cyclooxygenase-2 upregulation, requires activation of the phosphatidylinositol 3-kinase/Akt/nuclear factor-kappaB pathway in human alveolar epithelial cells. J. Pharmacol. Sci. 111 (3), 269–275. doi: 10.1254/jphs.09155FP
Mouratidis, P. X., Colston, K. W., Bartlett, J. B., Muller, G. W., Man, H. W., Stirling, D., et al. (2009). Antiproliferative effects of CC-8062 and CC-8075 in pancreatic cancer cells. Pancreas 38 (1), 78–84. doi: 10.1097/MPA.0b013e318183d72f
Oger, S., Méhats, C., Dallot, E., Cabrol, D., Leroy, M. J. (2002). Evidence for a role of phosphodiesterase 4 in lipopolysaccharide-stimulated prostaglandin E2 production and matrix metalloproteinase-9 activity in human amniochorionic membranes. J. Immunol. 174 (12), 8082–8089. doi: 10.4049/jimmunol.174.12.8082
Phillips, J. E. (2020). Inhaled Phosphodiesterase 4 (PDE4) Inhibitors for Inflammatory Respiratory Diseases. Front. Pharmacol. 11, 259. doi: 10.3389/fphar.2020.00259
Pikarsky, E., Ben-Neriah, Y. (2006). NF-kappaB inhibition: a double-edged sword in cancer. Eur. J. Cancer 42 (6), 779–784. doi: 10.1016/j.ejca.2006.01.011
Selige, J., Tenor, H., Hatzelmann, A., Dunkern, T. (2010). Cytokine-dependent balance of mitogenic effects in primary human lung fibroblasts related to cyclic AMP signaling and phosphodiesterase 4 inhibition. J. Cell Physiol. 223 (2), 317–326. doi: 10.1002/jcp.22037
Sigala, I., Zacharatos, P., Toumpanakis, D., Michailidou, T., Noussia, O., Theocharis, S., et al. (2011). MAPKs and NF-kappaB differentially regulate cytokine expression in the diaphragm in response to resistive breathing: the role of oxidative stress. Am. J. Physiol. Regul. Integr. Comp. Physiol. 300 (5), R1152–R1162. doi: 10.1152/ajpregu.00376.2010
Smith, L. A., Paszkiewicz, G. M., Hutson, A. D., Pauly, J. L. (2010). Inflammatory response of lung macrophages and epithelial cells to tobacco smoke: a literature review of ex vivo investigations. Immunol. Res. 46 (1–3), 94–126. doi: 10.1007/s12026-009-8133-6
Sosroseno, W., Sugiatno, E. (2008). Cyclic-AMP-dependent proliferation of a human osteoblast cell line (HOS cells) induced by hydroxyapatite: effect of exogenous nitric oxide. Acta BioMed. 79 (2), 110–116.
Udalov, S., Dumitrascu, R., Pullamsetti, S. S., Al-tamari, H. M., Weissmann, N., Ghofrani, H. A., et al. (2010). Effects of phosphodiesterase 4 inhibition on bleomycin-induced pulmonary fibrosis in mice. BMC Pulm. Med. 10, 26. doi: 10.1186/1471-2466-10-26
Wong, K. K. (2009). Recent developments in anti-cancer agents targeting the Ras/Raf/ MEK/ERK pathway. Recent Pat. Anticancer Drug Discovery 4 (1), 28–35. doi: 10.2174/157489209787002461
Yougbare, I., Morin, C., Senouvo, F. Y., Sirois, C., Albadine, R., Lugnier, C., et al. (2011). NCS 613, a potent and specific PDE4 inhibitor, displays anti-inflammatory effects on human lung tissues. Am. J. Physiol. Lung Cell Mol. Physiol. 301 (4), L441–L450. doi: 10.1152/ajplung.00407.2010
Yougbare, I., Boire, G., Roy, M., Lugnier, C., Rouseau, E. (2013). NCS 613 exhibits anti-inflammatory effects on PBMCs from lupus patients by inhibiting p38 MAPK and NF-kappaB signalling pathways while reducing proinflammatory cytokine production. Can. J. Physiol. Pharmacol. 91 (5), 353–361. doi: 10.1139/cjpp-2012-0233
Yougbare, I., Keravis, T., Abusnina, A., Decossas, M., Schall, N., Muller, S., et al. (2014). Cyclic GMP catabolism up-regulation in MRL/lpr lupus-prone mice is associated with organ remodeling. Biochim. Biophys. Acta 1842 (7), 916–926. doi: 10.1016/j.bbadis.2014.03.001
Keywords: PDE4 inhibitor, cAMP signaling, inflammation, proliferation, human lung adenocarcinoma
Citation: Yougbare I, Belemnaba L, Morin C, Abusnina A, Senouvo YF, Keravis T, Lugnier C and Rousseau E (2020) NCS 613, a Potent PDE4 Inhibitor, Displays Anti-Inflammatory and Anti-Proliferative Properties on A549 Lung Epithelial Cells and Human Lung Adenocarcinoma Explants. Front. Pharmacol. 11:1266. doi: 10.3389/fphar.2020.01266
Received: 24 May 2020; Accepted: 31 July 2020;
Published: 18 August 2020.
Edited by:
Carla Maria Pedrosa Ribeiro, University of North Carolina at Chapel Hill, United StatesReviewed by:
Federica Finetti, University of Siena, ItalyCopyright © 2020 Yougbare, Belemnaba, Morin, Abusnina, Senouvo, Keravis, Lugnier and Rousseau. This is an open-access article distributed under the terms of the Creative Commons Attribution License (CC BY). The use, distribution or reproduction in other forums is permitted, provided the original author(s) and the copyright owner(s) are credited and that the original publication in this journal is cited, in accordance with accepted academic practice. No use, distribution or reproduction is permitted which does not comply with these terms.
*Correspondence: Eric Rousseau, RXJpYy5Sb3Vzc2VhdUBVU2hlcmJyb29rZS5jYQ==
†Present address: Lazare Belemnaba, Research Institute of Health Sciences, Ouagadougou, Burkina Faso
Disclaimer: All claims expressed in this article are solely those of the authors and do not necessarily represent those of their affiliated organizations, or those of the publisher, the editors and the reviewers. Any product that may be evaluated in this article or claim that may be made by its manufacturer is not guaranteed or endorsed by the publisher.
Research integrity at Frontiers
Learn more about the work of our research integrity team to safeguard the quality of each article we publish.