- 1Parasite Chemotherapy Unit, Swiss Tropical and Public Health Institute, Basel, Switzerland
- 2Faculty of Science, University of Basel, Basel, Switzerland
- 3Faculty of Pharmacy, University of Khartoum, Khartoum, Sudan
- 4Faculty of Pharmacy, University of Science and Technology, Omdurman, Sudan
In a screening of Sudanese medicinal plants for antiprotozoal activity, the chloroform fractions obtained by liquid-liquid partitioning from ethanolic extracts of fruits of Croton gratissimus var. gratissimus and stems of Cuscuta hyalina Roth ex Schult. exhibited in vitro activity against axenically grown Leishmania donovani amastigotes. This antileishmanial activity was localized by HPLC-based activity profiling. Targeted preparative isolation afforded flavonoids 1–6, 3-methoxy-4-hydroxybenzoic acid (7), and benzyltetrahydroisoquinoline alkaloids laudanine (8) and laudanosine (9) from C. gratissimus, and pinoresinol (10), isorhamnetin (11), (-)-pseudosemiglabrin (12), and kaempferol (13) from C. hyalina. The antiprotozoal activity of 1–13 against L. donovani (axenic and intracellular amastigotes), Trypanosoma brucei rhodesiense (bloodstream forms), and Plasmodium falciparum (erythrocytic stages), and the cytotoxicity in L6 murine myoblast cells were determined in vitro. Quercetin-3,7-dimethylether (6) showed the highest activity against axenic L. donovani (IC50, 4.5 µM; selectivity index [SI], 12.3), P. falciparum (IC50, 7.3 µM; SI, 7.6), and T. b. rhodesiense (IC50, 2.4 µM; SI, 23.2). The congener ayanin (2) exhibited moderate antileishmanial (IC50, 8.2 µM; SI, 12.2), antiplasmodial (IC50, 7.8 µM; SI, 12.9), and antitrypanosomal activity (IC50, 11.2 µM; SI, 8.9). None of the compounds showed notable activity against the intramacrophage form of L. donovani.
Introduction
Parasitic protozoa are the causative agents of devastating, yet often neglected diseases. The kinetoplastids, a group of flagellated protozoa, cause neglected tropical diseases that put more than one billion people around the globe at risk (WHO|World Health Organization, n.d.; Khalid, 2012). These diseases are human African trypanosomiasis (HAT) caused by Trypanosoma brucei spp., Chagas’ disease caused by Trypanosoma cruzi, and Leishmaniasis caused by Leishmania spp. (Stuart et al., 2008). The apicomplexan parasite Plasmodium falciparum is the causative agent of malaria tropica which claims more than 400,000 lives every year (Organization, W.H. 2019).
These infections are of high public health relevance and socio-economic impact. Most of the currently available drugs have drawbacks in terms of toxicity, limited availability of oral therapeutic dosage forms, development of resistance, or non-affordability.
Natural products have in many instances provided new leads to combat neglected tropical diseases (Schmidt et al., 2012). As part of an ongoing screening project of Sudanese medicinal plants for antiprotozoal activity (Mahmoud et al., 2020a; Mahmoud et al., 2020b), the chloroform extract of Croton gratissimus var. gratissimus (Euphorbiaceae), and Cuscuta hyalina Roth ex Schult. (Convolvulaceae) showed promising activity against P. falciparum and Leishmania donovani.
The genus Croton comprises over 1300 species that are widely distributed throughout tropical and subtropical regions of the world. Croton species have been used traditionally in Africa, South Asia, and Latin America for the treatment of infections and digestive disorders (Wu and Zhao, 2004; Xu et al., 2018). In Sudan, C. gratissimus, locally known as Um-Geleigla, has been used traditionally for the treatment of hypertension and malaria (Mohamed et al., 2009). The main secondary metabolites include flavonoids, terpenoids, and essential oil (Ngadjui et al., 2002; Aderogba et al., 2011; Yagi et al., 2016). Previous studies have demonstrated that the roots of C. gratissimus possessed antiplasmodial activity in vivo (Okokon and Nwafor, 2009). Cembranolide diterpenes isolated from the leaves were found to be active when tested against P. falciparum (Langat et al., 2011).
The genus Cuscuta comprises over 200 species distributed worldwide. They are stem obligate holoparasitic plants possessing neither roots nor fully expanded leaves. The interaction between parasite and host is established through haustoria (Kaiser et al., 2015). Different Cuscuta species have been used in traditional Indian and Chinese medicine. Cytotoxic, antioxidant, and antimicrobial activities have been reported (Ahmad et al., 2017). Previous phytochemical investigations of the genus Cuscuta identified flavonoids, lignans, alkaloids, fatty acids, and essential oil (Donnapee et al., 2014; Ahmad et al., 2017). The phytochemistry and antiparasitic activity of C. hyalina has not been studied.
In an earlier screening of Sudanese medicinal plants for antiprotozoal activity, the ethanolic extracts of Croton gratissimus fruits and Cuscuta hyalina stems had been found to exhibit in vitro antiprotozoal activity against axenic L. donovani (MHOM/ET/67/L82). Subsequent liquid- liquid partitioning against petroleum ether, chloroform, and ethyl acetate located the activity in the chloroform portion (Mahmoud et al., 2020b). We here report on the targeted isolation and structure elucidation of compounds responsible for the activity, and on their in vitro activity against T. b. rhodesiense (STIB 900), axenic and intramacrophage amastigotes of L. donovani (MHOM/ET/67/L82), and P. falciparum (NF54).
Materials and Methods
General Experimental Procedures
HPLC-grade methanol and acetonitrile from Macron Fine Chemicals (Avantor Performance Materials), and water from Milli-Q water purification systems (Merck Millipore) were used for HPLC separations. For fractionation and preparative separation, technical grade solvents from Scharlau (Scharlab S. L.) were used after distillation. Silica gel 60 F254 coated aluminum TLC plates were obtained from Merck. Silica gel (230–400 µm, Merck) and Sephadex LH-20 (25–100 µm, Sigma-Aldrich) were used for open column chromatography. Optical rotation was measured in methanol using a JASCO P-2000 digital polarimeter equipped with a sodium lamp (589 nm) and a temperature-controlled microcell (10 cm). UV and ECD spectra were recorded in methanol on a Chirascan CD spectrometer (Applied Photophysics) using 110 QS 1 mm path precision cells (Hellma Analytics). NMR spectra were recorded on a Bruker Avance III NMR spectrometer operating at 500.13 MHz for 1H and 125.77 MHz for 13C. 1H NMR, COSY, HSQC, HMBC, and NOESY spectra were measured at 23°C in a 1 mm TXI probe with a z-gradient, using standard Bruker pulse sequences. Spectra were analyzed by Bruker TopSpin 3.5 pl 7 and ACDLabs Spectrus Processor. NMR spectra were recorded in DMSO-d6 (99.9 atom % D; Armar Chemicals).
HPLC-PDA-ELSD-ESIMS data were recorded in positive- and negative-ion mode (scan range of m/z 200–1,500) on a Shimadzu LC-MS/MS 8030 triple quadrupole MS system, connected via a T-splitter (1:10) to a Shimadzu HPLC system consisting of degasser, binary mixing pump, autosampler, column oven, and a diode array detector and to an Alltech 3300 ELSD detector. Separation was achieved on a SunFire C18 (3.5 μm, 150 × 3.0 mm i.d.) column equipped with a guard column (10 mm × 3.0 mm i.d.) (Waters). Data acquisition and processing were performed with LabSolution software.
Microfractionation was carried out with the same HPLC instrument connected via a T split to an FC204 fraction collector (Gilson) with only UV detection, using a SunFire C18 (3.5 μm, 150 mm × 3.0 mm i.d.) column equipped with a guard column (10 mm × 3.0 mm i.d.) (Waters).
Semipreparative HPLC separations were carried out with an Agilent HP 1100 Series system consisting of a quaternary pump, autosampler, column oven, and a diode array detector. SunFire C18 (5 μm, 10 × 150 mm i.d.) columns (Waters) were used for separations. Chemstation software was used for data acquisition and processing. Preparative separations were carried out on a Puriflash 4100 system (Interchim) or a Reveleris PREP purification system (Büchi). Sephadex LH-20 (110 cm × 3 cm; 25–100 µm) and silica gel (40 cm × 5 cm, 230–400 mesh) columns were used.
All handling of infectious agents (L. donovani, T. b. rhodesiense, P. falciparum) was performed under strict biosafety level 2 conditions under notification A000275 to the Swiss Federal Office of Public Health.
Plant Material
Croton gratissimus var. gratissimus fruits and Cuscuta hyalina Roth ex Schult. stems were obtained from the Herbarium of the Faculty of Pharmacy, University of Science and Technology, Omdurman, Sudan. The taxonomic identity was confirmed by the Medicinal and Aromatic Plants Research Institute, Sudan and voucher specimens (CZFCHL02 and ChSCHL 02) were deposited. Plant materials were dried at room temperature and milled before extraction.
Extraction
Powdered materials of C. gratissimus fruits and C. hyalina stems (500 g each), respectively, were extracted with 1 L of 70% ethanol and kept in a magnet rod shaker for 24 h. The extraction procedure was repeated three times for each herbal drug. Extracts were filtered and dried under reduced pressure. For each plant, the ethanolic extract was suspended in water and partitioned successively with petroleum ether, chloroform, and ethyl acetate. Three repetitive partitioning procedures, each with 500 ml of either solvent were performed. This afforded 3.5 and 1.2 g of the chloroform extracts of C. gratissimus fruits and C. hyalina stems, respectively.
Microfractionation
HPLC-based microfractionation of the chloroform extracts of C. gratissimus fruits and C. hyalina stems was performed [H2O + 0.1% formic acid (A), MeCN + 0.1% formic acid (B); 0→100% B (0–30 min), 100% B (30–40 min); flow rate 0.4 ml/min; sample concentration 10 mg/ml in DMSO; injection volume twice 35 μl] by collecting 1-min fractions from minute 1 to minute 40 into a 96-deepwell plate. After drying of plates in a Genevac EZ-2 evaporator, microfractions were tested for their antiprotozoal activity according to previously established protocols (Potterat and Hamburger, 2013; Potterat and Hamburger, 2014).
Preparative Isolation
The chloroform fraction (3.5 g) of C. gratissimus fruits was fractionated by column chromatography (CC) on Sephadex LH-20 (110 × 3 cm; 25–100 µm) using methanol as eluent at a flow rate of 1 ml/min. A total of 19 fractions (A-S) were combined based on TLC patterns (silica gel; CH2CL2−MeOH, 90:10, 75:25, and 50:50, respectively; detection with 1% ethanolic vanillin and 10% sulfuric acid, followed by heating at 105°C). Fractions were submitted to HPLC-PDA-ELSD-MS analysis to track peaks previously detected in the active time windows of the activity profile.
Fraction M (36 mg) was submitted to semipreparative RP-HPLC [H2O (A), CH3CN (B); 43% B (0–22 min), 43→100% B (22–27 min), 100% B (27–30 min), flow rate 4 ml/min; sample concentration 50 mg/ml in DMSO; injection volume 50 μl], yielding quercetin-3,3′,4′-trimethylether (1, 0.3 mg, tR 10.2 min), ayanin (2, 21.9 mg, tR 16.7 min), and retusin (3, 0.4 mg, tR 28.8 min).
Fraction O (15 mg) was submitted to semipreparative RP-HPLC [H2O (A), CH3CN (B); 35% B (0–34 min), 35→100% B (34–40 min), 100% B (40–45 min), flow rate 4 ml/min; sample concentration 50 mg/ml in DMSO; injection volume 50 μl], to afford naringenin (4, 0.51 mg, tR 9.6 min), quercetin-3,4′-dimethylether (5, 1.9 mg, tR 12.1 min), and quercetin-3,7-dimethylether (6, 7.1 mg, tR 20.5 min).
Fraction K (26.6 mg) was purified by semipreparative RP-HPLC [H2O (A), CH3CN (B), both containing 0.1% formic acid; 10→32% B (0–30 min), 32→100% B (30–35 min), 100% B (35–40 min), flow rate 4 ml/min; sample concentration 50 mg/ml in DMSO; injection volume 50 μl], to afford 3-methoxy-4-hydroxybenzoic acid (7, 0.63 mg, tR 10.9 min).
Fraction C (100.6 mg) was purified by semipreparative RP-HPLC [H2O (A), CH3CN (B), both containing 0.1% formic acid; 10→17% B (0–20 min), 17→100% B (20–25 min), 100% B (25–30 min), flow rate 4 ml/min; sample concentration 50 mg/ml in DMSO; injection volume 50 μl], to afford laudanine (8, 0.41 mg, tR 9.1 min), and laudanosine (9, 0.63 mg, tR 14.5 min).
The chloroform fraction (1.9 g) of C. hyalina stems was fractionated by CC on silica gel (40 × 5 cm, 230–400 mesh), using a gradient of CH2CL2−MeOH (99:1 to 0:100) as mobile phase. A total of 16 fractions (A-P) were combined based on TLC patterns (silica gel; CH2CL2−MeOH, 99:1, 90:10, and 80:20, respectively; detection with 1% ethanolic vanillin and 10% sulfuric acid, followed by heating). Fractions were submitted to HPLC-PDA-ELSD-MS analyses to track peaks previously detected in the active time windows of the activity profile.
Fraction B (52.7 mg) was purified by semipreparative RP-HPLC [H2O (A), CH3CN (B); 25→70% B (0–30 min), 70→100% B (30–33 min), 100% B (33–40 min), flow rate 4 ml/min; sample concentration 50 mg/ml in DMSO; injection volume 50 μl], to afford pinoresinol (10, 7.8 mg, tR 10.2 min), isorhamnetin (11, 3.5 mg, tR 13.6 min), pseudosemiglabrin (12, 2.2 mg, tR 23.5 min). Kaempferol (13) was identified by co-injection of a reference standard (Sigma-Aldrich).
Quercetin-3,3′,4′-trimethylether (1): amorphous solid; 1H and 13C NMR, see Table S1,
Supporting Information; ESIMS m/z 345 [M + H]+.
Ayanin (2): amorphous solid; 1H and 13C NMR, see Table S1, Supporting Information; ESIMS m/z 345 [M + H]+.
Retusin (3): amorphous solid; 1H and 13C NMR, see Table S1, Supporting Information; ESIMS m/z 359 [M + H]+.
Naringenin (4): amorphous solid; 1H and 13C NMR, see Table S2, Supporting Information; ESIMS m/z 273 [M + H]+.
Quercetin-3,4′-dimethylether (5): amorphous solid; 1H and 13C NMR, see Table S2, Supporting Information; ESIMS m/z 331 [M + H]+.
Quercetin-3,7-dimethylether (6): amorphous solid; 1H and 13C NMR, see Table S2, Supporting Information; ESIMS m/z 331 [M + H]+.
3-Methoxy-4-hydroxybenzoic acid (7): amorphous solid; 1H and 13C NMR, see Table S3, Supporting Information; ESIMS m/z 169 [M + H]+.
R-Laudanine (8): amorphous solid; [α]25D -6.6 (c 0.04, MeOH); UV λmax (MeOH) (log ϵ) 226 (0.07), 291 (0.01) nm; ECD (MeOH, c 3.5 × 10−4 M, 1 mm path length) λmax(Δϵ) 214 (−0.56), 241 (−0.47), 290 (−0.39); 1H and 13C NMR, see Table S4, Supporting Information; ESIMS m/z 344 [M + H]+.
R-Laudanosine (9): amorphous solid; [α]25D −62.5 (c 0.04, MeOH); UV λmax (MeOH) (log ϵ) 201 (0.75), 226 (0.18), 279 (0.06) nm; ECD (MeOH, c 1.4 × 10−4 M, 1 mm path length) λmax(Δϵ) 211 (−19.43), 241 (−6.67), 290 (−3.38); 1H and 13C NMR, see Table S4, Supporting Information; ESIMS m/z 358 [M + H]+.
(+)-(7S,7′S,8R,8′R)-Pinoresinol (10): amorphous solid; [α]25D 69.0 (c 0.10, MeOH); UV λmax (MeOH) (log ϵ) 202 (0.70), 232 (0.10) nm; ECD (MeOH, c 7.0 × 10−5 M, 1 mm path length) λmax(Δϵ) 207 (+21.78) nm; 1H and 13C NMR, see Table S5, Supporting Information; ESIMS m/z 359 [M + H]+.
Isorhamnetin (11): amorphous solid; 1H and 13C NMR, see Table S5, Supporting Information; ESIMS m/z 317 [M + H]+.
(-)-(3′′S,4′′R,5′′S)-Pseudosemiglabrin (12): amorphous solid; [α]25D −410.0 (c 0.05, MeOH); UV λmax (MeOH) (log ϵ) 212 (0.73), 255 (0.49), 309 (0.43) nm; ECD (MeOH, c 2.6 × 10−4 M, 1 mm path length) λmax(Δϵ) 206 (−12.84), 215 (+3.69), 226 (−10.40), 257 (−11.01), 275 (−7.99) nm; 1H and 13C NMR, see Table S6, Supporting Information; ESIMS m/z 393 [M + H]+.
Kaempferol (13): identified by co-injection of a reference standard (Sigma-Aldrich).
Sample Preparation
Compounds were dissolved in DMSO (10 mg/ml) and warmed up to 40°C and/or sonicated if necessary. These DMSO stocks were kept at −20°C. For each assay, a fresh dilution to 100 µg/ml in medium was prepared. This was used to prepare the serial dilutions directly in the 96-well assay plates. Since DMSO is cytotoxic, the maximum DMSO concentration in the test was 1%.
Activity Against Leishmania donovani Axenic Amastigotes
Amastigotes of L. donovani strain MHOM/ET/67/L82 were grown under an atmosphere of 5% CO2 in air in axenic culture at 37°C in SM medium (Cunningham, 1977) at pH 5.4 supplemented with 10% heat-inactivated fetal bovine serum. 50 µL of culture medium was added in the wells of a 96-well plate and serial drug dilutions of eleven three-fold dilution steps covering a final range from 100 to 0.002 μg/ml were prepared. 50 µL culture medium with 2 × 105 amastigotes from axenic culture were added to each well. After 70 h of incubation the plates were inspected under an inverted microscope to assure growth of the controls and sterile conditions. 10 μl of resazurin (12.5 mg resazurin dissolved in 100 ml distilled water) were added to each well and the plates incubated for another 2 h. Then the plates were read with a Spectramax Gemini XS microplate fluorometer (Molecular Devices Cooperation, Sunnyvale, CA, USA) using an excitation wavelength of 536 nm and an emission wavelength of 588 nm. Data were analyzed using the software Softmax Pro (Molecular Devices Cooperation, Sunnyvale, CA, USA). Decrease of fluorescence (= inhibition) was expressed as percentage of the fluorescence of untreated control cultures and plotted against the drug concentrations. From the sigmoidal inhibition curves the IC50 values were calculated. Miltefosine was used as positive control drug. Assays were performed in two independent replicates at least.
Activity Against Leishmania donovani Intramacrophage Amastigotes
Macrophages were isolated from the mouse (CDI) peritoneal cavity (Zhang et al., 2008) using 2% starch solution as eliciting agent injected 2 day prior to cell harvest. Animal work was carried out according to the rules and regulations for the protection of animal rights (“Tierschutzverordnung”) of the Swiss “Bundesamt für Veterinärwesen” (License number 2374). Mouse peritoneal macrophages (4 × 104 in 100 µL RPMI 1640 medium with 10% heat-inactivated FBS) were seeded into wells of a 96-well plate. After 24 h, 2 × 105 amastigote L. donovani in 100 µL were added. The amastigotes were taken from an axenic amastigote culture grown at pH 5.4. The medium containing free amastigote forms was removed after 24 h and replaced with fresh medium. The washing step was repeated and afterward the serial drug dilution was prepared with at least 6 dilution steps. Compounds were dissolved in DMSO at 10 mg/ml and further diluted in medium. After 96 h of incubation at 37°C under a 5% CO2 atmosphere, the medium was removed, and cells were fixed by adding 50 µl 4% formaldehyde solution followed by a staining with a 5 µM DRAQ5 solution. Plates were imaged in ImageXpress XLS (MD) microscope using a 20× air objective (635 nm excitation: 690/50 emission). 9 images were collected per well. Automated image analysis was performed with a script developed on Meta Xpress Software (MD). Three outputs were provided for each well: i) number of host cell nuclei; ii) numbers of infected and non-infected host cells; iii) number of parasite nuclei per infected host cell. The IC50 values were calculated based on the infection rate and the numbers of intracellular amastigotes. The cytotoxicity to macrophages was determined in parallel, and IC50 values were calculated based on the numbers of surviving, uninfected macrophages. Miltefosine was used as control. Assays were performed in two independent replicates at least.
Activity Against Trypanosoma brucei rhodesiense STIB900
The stock was originally isolated from a Tanzanian patient and adapted to axenic culture conditions after several mouse passages and cloned. Minimum Essential Medium (50 µl) supplemented with 25 mM HEPES, 1 g/L additional glucose, 1% MEM non-essential amino acids (100×), 0.2 mM 2-mercaptoethanol, 1 mM Na-pyruvate (Baltz et al., 1985) and 15% heat inactivated horse serum was added to each well of a 96-well microtiter plate. Serial drug dilutions of eleven 3-fold dilution steps covering a range from 100 to 0.002 μg/ml were prepared. Then 4 × 103 bloodstream forms of T. b. rhodesiense STIB 900 in 50 µL were added to each well and the plate incubated for 70 h at 37°C and under a 5% CO2 atmosphere. 10 µL resazurin solution (resazurin, 12.5 mg in 100 ml double-distilled water) was then added to each well and incubation continued for a further 2–4 h (Räz et al., 1997). Plates were read with a Spectramax Gemini XS microplate fluorometer (Molecular Devices Cooperation, Sunnyvale, CA, USA) using an excitation wavelength of 536 nm and an emission wavelength of 588 nm. Softmax Pro program (Molecular Devices Cooperation, Sunnyvale, CA, USA) was used for data analyses and IC50 values were calculated by linear regression (Huber and Koella, 1993), and 4-parameter logistic regression from the sigmoidal dose inhibition curves. Melarsoprol (Arsobal Sanofi-Aventis, received from WHO) was used as control. Assays were performed in two independent replicates at least.
Activity Against Plasmodium falciparum
In vitro activity against the erythrocytic stages of P. falciparum was determined using a 3H-hypoxanthine incorporation assay (Desjardins et al., 1979), using the drug sensitive NF54 strain (Ponnudurai et al., 1981). Compounds were dissolved in DMSO at 10 mg/ml and further diluted in medium before addition to parasite cultures incubated in RPMI 1640 medium without hypoxanthine, supplemented with HEPES (5.94 g/L), NaHCO3 (2.1 g/L), neomycin (100 U/ml), AlbumaxR (5 g/L), and washed human red cells A+ at 2.5% haematocrit (0.3% parasitemia). Serial drug dilutions of 11 three-fold dilution steps covering a range from 100 to 0.002 μg/ml were prepared. The 96-well plates were incubated in a humidified atmosphere at 37°C; 4% CO2, 3% O2, 93% N2. After 48 h, 50 μl of 3H-hypoxanthine (=0.5 μCi) was added to each well of the plate. The plates were incubated for a further 24 h under the same conditions. The plates were then harvested with a Betaplate™ cell harvester (Wallac, Zurich, Switzerland), the red blood cells transferred onto a glass fibre filter, and lysed with distilled water. The dried filters were inserted into a plastic foil with 10 ml of scintillation fluid and counted in a Betaplate™ liquid scintillation counter (Wallac, Zurich, Switzerland). IC50 values were calculated from sigmoidal inhibition curves by linear regression using Microsoft Excel. Chloroquine (Sigma C6628) was used as control. Assays were performed in two independent replicates at least.
In Vitro Cytotoxicity With L-6 Cells
Assays were performed in 96-well microtiter plates, each well containing 100 µl of RPMI 1640 medium supplemented with 1% L-glutamine (200 mM) and 10% fetal bovine serum, and 4000 L-6 cells (a primary cell line derived from rat skeletal myoblasts) (Ahmed et al., 1994). Serial drug dilutions of 11 three-fold dilution steps covering a range from 100 to 0.002 μg/ml were prepared 24 h post seeding L-6 cells. The plates were incubated for 70 h and inspected under an inverted microscope to assure growth of the controls and sterile conditions. 10 µl of resazurin was then added to each well and the plates incubated for another 2 h. Then the plates were read with a Spectramax Gemini XS microplate fluorometer (Molecular Devices Cooperation, Sunnyvale, CA, USA) using an excitation wavelength of 536 nm and an emission wavelength of 588 nm. The IC50 values were calculated by linear regression and four-parameter logistic regression from the sigmoidal dose inhibition curves using SoftmaxPro software (Molecular Devices Cooperation, Sunnyvale, CA, USA). Podophyllotoxin (Sigma P4405) was used as positive control. All assays were performed in two independent replicates at least. Activities of all compounds were expressed in µM using the formula:
Results and Discussion
Extraction and HPLC-Based Activity Profiling
The methanolic extracts of Croton gratissimus var. gratissimus fruits and Cuscuta hyalina Roth ex Schult. stems had been previously found to exhibit antiprotozoal activity (Mahmoud et al., 2020b). The antileishmanial activity displayed by the chloroform fractions of the two plants was tracked by HPLC-based activity profiling, a procedure combining analytical separation with on-line spectroscopy and time-based microfractionation for bioactivity testing (Potterat and Hamburger, 2013; Potterat and Hamburger, 2014). One-minute microfractions were collected and tested for L. donovani growth inhibition. The HPLC−ESIMS (positive base peak chromatograms) trace and the corresponding antileishmanial activity profiles for C. gratissimus and C. hyalina are shown in Figures 1 and 2. Major antileishmanial activity and a series of distinct peaks in the HPLC-ESIMS trace were observed in the time window between 18 and 24 min for C. gratissimus, and between 13 and 17 min for C. hyalina.
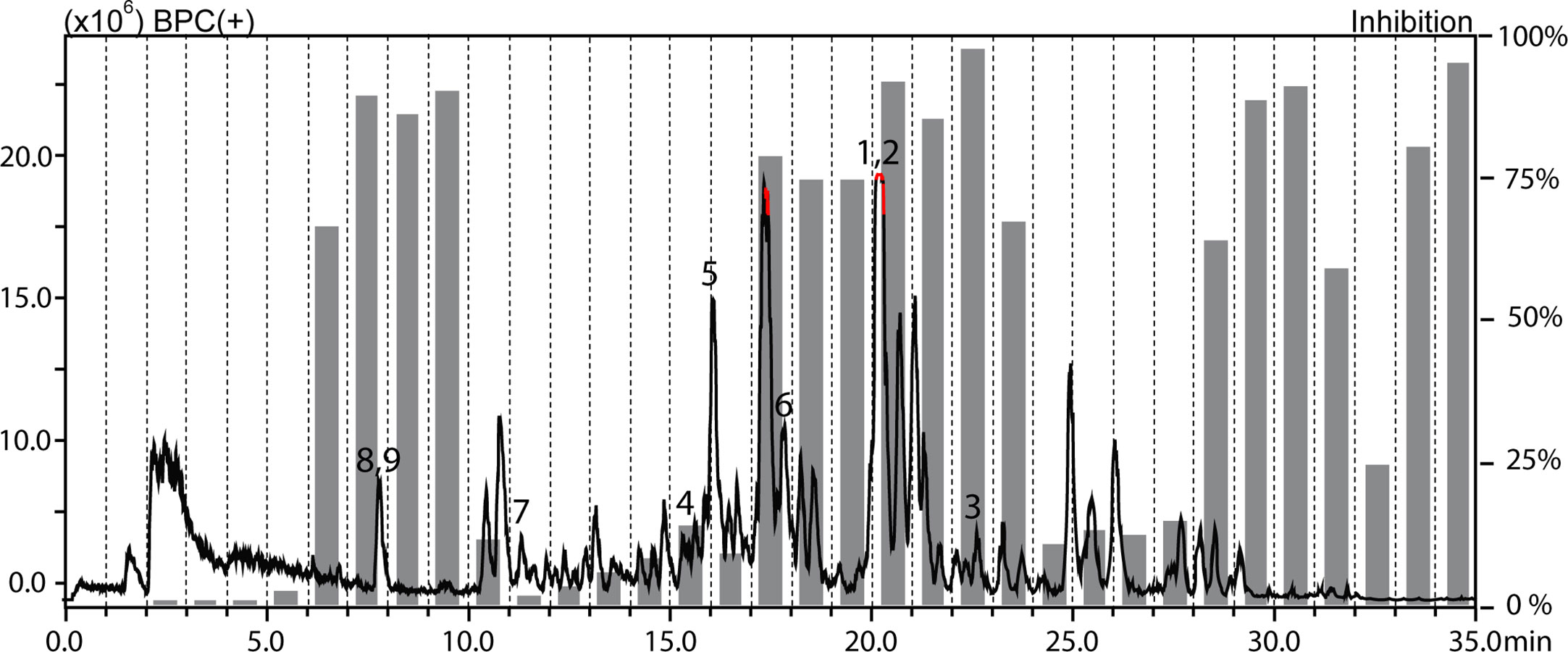
Figure 1 HPLC-based activity profiling of the chloroform fraction of Croton gratissimus var. gratissimus against axenic amastigotes of L. donovani. The ESIMS (positive base peak chromatogram) of a separation of 300 μg of fraction on an analytical RP-HPLC column is shown. Activities of 1-min microfractions are shown with grey columns, and are expressed as percent growth inhibition compared to untreated parasites. Bold numbers in the chromatogram refer to compounds 1–9
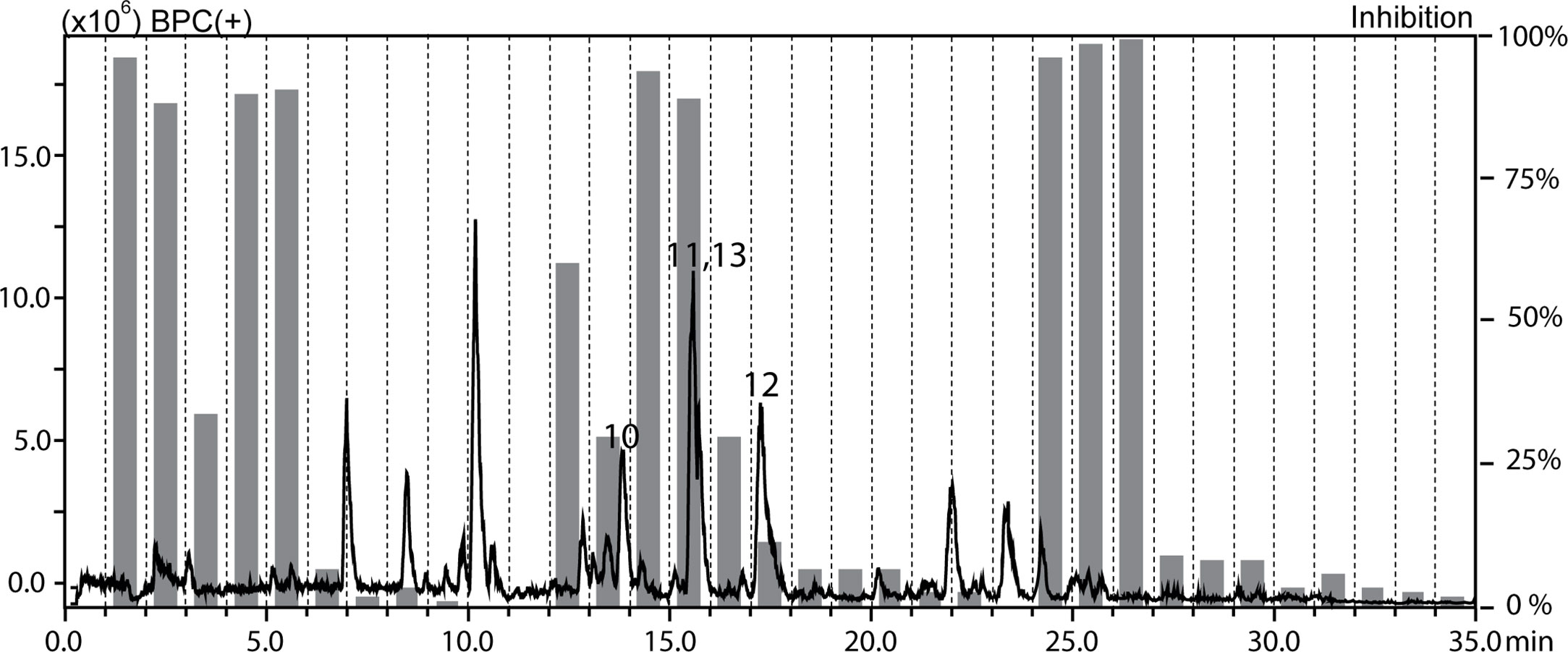
Figure 2 HPLC-based activity profiling of the chloroform fraction of Cuscuta hyalina Roth ex Schult. against axenic amastigotes of L. donovani. The ESIMS (positive base peak chromatogram) and activity profile (gray bars) are shown. Bold numbers in the chromatogram refer to compounds 10–13.
Compound Isolation and Structure Elucidation
Separation of the chloroform fraction of C. gratissimus on a Sephadex LH-20 column yielded 19 subfractions (A-S). Based on the HPLC−PDA-ESIMS analysis, subfractions M, O, K, and C were found to contain peaks associated with the active time window. Further purification by semipreparative RP-HPLC afforded compounds 1–3 from subfraction M, 4–6 from subfraction O, 7 from subfraction K, and 8 and 9 from subfraction C.
By means of 1D and 2D NMR data (Tables S1-S4; Supporting Information), six flavonoids were identified as quercetin-3,3′,4′-trimethylether (1) (Urbatsch et al., 1976), ayanin (2) (Matsuda et al., 2002), retusin (3) (Matsuda et al., 2002), naringenin (4) (Ibrahim et al., 2003), quercetin-3,4′-dimethyl ether (5) (Barberá et al., 1986a), quercetin-3,7-dimethylether (6) (Wang et al., 1989), along with 3-methoxy-4-hydroxybenzoic acid (7) (Crestini et al., 2006), and the two benzyltetrahydroisoquinoline alkaloids laudanine (8) (Janssen et al., 1990) and laudanosine (9) (Janssen et al., 1990). For naringenin (4), an optical rotation close to 0 and the absence of a Cotton effect (CE) in the ECD indicated a 1:1 mixture of R- and S-stereoisomers. The absolute configuration of 8 and 9 was determined as R based on the optical rotation ([α]25D -6.6 (c 0.04, MeOH) for 8 (Frydman et al., 1958) and [α]25D -62.5 (c 0.04, MeOH) for 9 (Ruiz-Olalla et al., 2015)). Moreover, the ECD spectra of both compounds showed two negative cotton effects (CEs) at 210 to 215 nm and 240 to 242 nm which were in good agreement with calculated spectra of the R-stereoisomers (Figure S1 and S2, Supporting Information).
Compounds 1–9 (Figure 3) are reported here for the first time from C. gratissimus, but some have been previously identified in other Croton species, such as ayanin (2) and quercetin-3,7-dimethylether (6) from C. schiedeanus (Guerrero et al., 2002), quercetin-3,4′-dimethylether (5) from C. arboreus (Aguilar-Guadarrama and Rios, 2004), 3-methoxy-4-hydroxybenzoic acid (7) from C. tonkinensis (Kuo et al., 2013), and R-laudanine (8) and R-laudanosine (9) from leaves and stems of C. celtidifolius (Amaral and Barnes, 1997).
Preparative chromatography on silica gel of the chloroform fraction of C. hyalina yielded 16 subfractions (A-P). Peaks associated with the active time window were detected in subfraction B. Further separation by semipreparative RP-HPLC afforded compounds 10–12 (Figure 3).
Based on the NMR data (Tables S5 and S6, Supporting Information), compounds were identified as the lignan pinoresinol (10) (Abe and Yamauchi, 1988) and as flavonoids isorhamnetin (11) (Barberá et al., 1986b) and (-)-pseudosemiglabrin (12) (Waterman and Khalid, 1980). In addition, kaempferol (13) was identified by dereplication with a reference compound. The absolute configuration of 10 and 12 was established based on their optical activity and ECD spectra. For compound 10, the optical rotation [α]25D + 69.0 (c 0.10, MeOH) and the positive cotton effect at 207 nm (Δϵ +21.78) in the ECD spectrum indicated a (+)-(7S,7′S,8R,8′R) configuration of pinoresinol (Figure S3, Supporting Information). The optical rotation [α]25D -410.0 (c 0.05, MeOH) of 12 indicated (-)-pseudosemiglabrin. The ECD spectrum showed four negative CEs at 206 (Δϵ -12.84), 226 (Δϵ -10.40), 257 (Δϵ -11.01), 275 (Δϵ -7.99) nm, and a positive CE at 215 nm (Δϵ +3.69). This was in agreement with calculated spectra for the 3′′S,4′′R,5′′S stereoisomer (Figure S4, Supporting Information), and opposite to the ECD data published for (+)-pseudosemiglabrin (Pirrung and Lee, 1995). However, the assignment of C-5′′ as S by Pirrung and Lee was incorrect. The absolute configuration of (-)-pseudosemiglabrin (12) was thus assigned as 3′′S,4′′R,5′′S.
Kaempferol (13) and isorhamnetin (11) have been previously reported from different Cuscuta species (Ahmad et al., 2017), while pinoresinol has been identified in C. chinensis (Yahara et al., 1994). To the best of our knowledge, this is the first report on isolation of pseudosemiglabrin (12) from Cuscuta species.
Activity Against Leishmania donovani Axenic and Intracellular Amastigotes
All compounds were tested for their activity against L. donovani (MHOM/ET/67/L82) axenic amastigotes (Table 1). Quercetin-3,7-dimethylether (6) has shown the highest activity (IC50 4.5 ± 0.3 µM), followed by ayanin (2) (IC50 8.2 ± 1.6 µM). Both compounds exhibited similar selectivity indices (SI 12.3), which were the highest among the tested compounds.
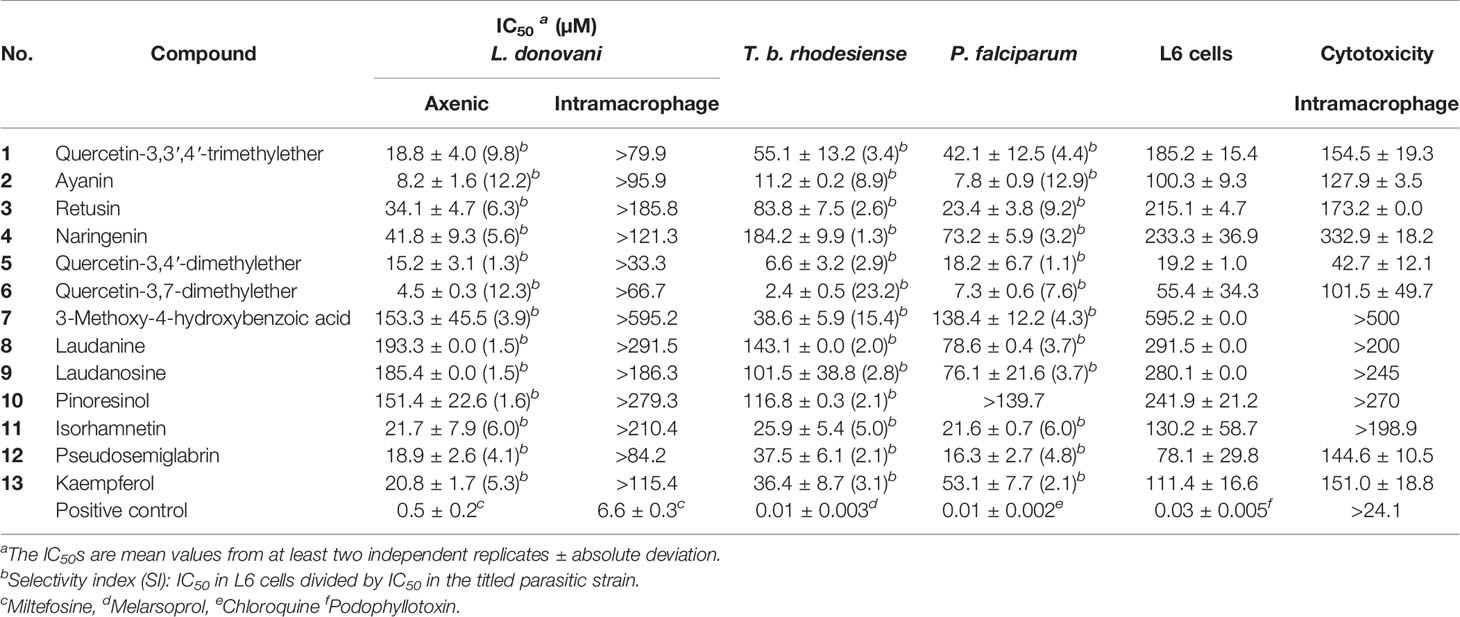
Table 1 In vitro activity of compounds 1–13 against T. b. rhodesiense (STIB 900), L. donovani (MHOM-ET-67/L82) axenic and intracellular amastigotes, P. falciparum (NF54), and cytotoxicity in L6 cells and intramacrophages.
Compounds 1 and 5 from C. gratissimus, and 11–13 from C. hyalina exhibited IC50 values in the range of 15 to 22 µM against axenic amastigotes. These compounds showed varying degrees of cytotoxicity in L6 rat skeletal cells. Moderate selectivity (selectivity indices (SI) of 4–6) toward L. donovani axenic amastigotes was observed for compounds 11–13, while quercetin-3,4′-dimethylether (5) showed the highest toxicity (SI 1.3).
The benzyltetrahydroisoquinoline alkaloids laudanine (8) and laudanosine (9), and the furofurano lignan pinoresinol (10) showed only marginal activity against L. donovani axenic amastigotes (IC50 ˃ 150 µM). The weak activity of the alkaloids was in agreement with previous reports (García Díaz et al., 2019).
After a first testing against axenic amastigotes, compounds were tested against L. donovani amastigotes in mouse macrophages. However, in this more elaborate and more physiological model none of the compounds showed activity (Table 1). In general, IC50 values for the intramacrophage form are higher than those for the axenic amastigotes (Berry et al., 2018). This loss of activity in the intracellular model could be due to poor cellular permeability of the compounds, binding to cytosolic proteins in the host cell, or metabolism in the host cell phagolysosome (Burchmore and Barrett, 2001; De Rycker et al., 2013; Berry et al., 2018).
Activity Against Trypanosoma brucei rhodesiense
All compounds were also tested for their in vitro activity against the blood stream form of T. brucei rhodesiense (STIB 900) (Table 1). As for L. donovani, quercetin-3,7-dimethylether (6) was the most active (IC50 2.4 ± 0.5 µM) and most selective (SI 23.2). Quercetin-3,4′-dimethyl ether (5) was also active (IC50 6.6 ± 3.2 µM) but had a low selectivity (SI 2.9). Ayanin (2) had an IC50 of 11.2 ± 0.2 µM with moderate cytotoxicity (SI 8.9), and isorhamnetin (11) an IC50 of 25.9 ± 5.4 µM and a SI of 5.0. Kaempferol (13), pseudosemiglabrin (12) and 3-methoxy-4-hydroxybenzoic acid (7) showed marginal activity (IC50 36–39 µM), and IC50 ˃ 80 µM were determined for retusin (3), naringenin (4), laudanine (8), laudanosine (9) and pinoresinol (10).
Activity Against Plasmodium falciparum
In vitro activity against the erythrocytic stages of the P. falciparum drug sensitive strain NF54 was determined for all compounds (Table 1). Ayanin (2) and quercetin-3,7-dimethylether (6) showed IC50 values of 7 to 8 µM, but 2 exhibited a higher selectivity index (SI 12.9) than 6 (SI 7.6). Quercetin-3,4′-dimethylether (5) was also rather active (IC50 <20 µM) but equally showed cytotoxic in L6 cells. Isorhamnetin (11) and pseudosemiglabrin (12) showed IC50 around 20 µM against P. falciparum and a moderate degree of selectivity towards the parasite (SI ~ 5). 3-Methoxy-4-hydroxybenzoic acid (7), and pinoresinol (10) were the least active among the tested compounds.
Correlation Between Chemical Structure of Isolated Flavonoids and Antiprotozoal Activity
Of the isolated compounds, only flavones showed notable activity (Table 1). From a comparison of flavones 1–3, 5, 6, 10, and 13 the following conclusions can be drawn: Compounds with a hydroxyl group at C-3′ (2, 5, and 6) were the most active against the three parasites, whereby a catechol moiety as in 6 further increased the activity. Free hydroxyl groups at C-3 or C-7 (as in 5, 11, and 13) had not result in significant in vitro activity. Compounds 2 and 6 exhibited the highest selectivity, while 5 showed significant cytotoxicity in L6 cells leading to a low SI.
Naringenin (4) displayed the weakest antiparasitic activity among the tested flavonoids. The presence of a double bond between C-2 and C-3 has been previously found to be essential for antiparasitic activity (Tasdemir et al., 2006). Overall, our results were in agreement with previous structure-activity studies of flavonoids (Tasdemir et al., 2006).
The influence of a balance between antioxidant and prooxidant properties of flavonoids on antiparasitic activity, and a correlation with their chemical structure has been investigated with the aid of QSAR models (Baldim et al., 2017). Compounds that displayed moderate to higher antitrypanosomal activity shared structural features, such as Δ2,3 unsaturation, presence of a hydroxyl group at C-3, a carbonyl group at C-4, and a catechol moiety in ring B. Our results were in line with these findings. To the best of our knowledge, the antitrypanosomal activities of quercetin-3,7-dimethylether (6) and ayanin (2) are here reported for the first time.
Data Availability Statement
The raw data supporting the conclusions of this article will be made available by the authors, without undue reservation.
Author Contributions
Conceived and designed the experiments: AM, MK, MH. Performed the experiments: AM, OD, MK. Analyzed the data: OD, PM, MH, SK. Wrote the paper: AM, OD, PM, MK, MH, SK.
Funding
This work was supported by grants to AM by the Amt für Ausbildungsbeiträge Basel (www.hochschulen.bs.ch/ueber-uns/organisation/amtausbildungsbeitraege.html) and the Emilia Guggenheim-Schnurr Foundation (www.ngib.ch/stiftung-egs). The funders had no role in study design, data collection and analysis, decision to publish, or preparation of the manuscript.
Conflict of Interest
The authors declare that the research was conducted in the absence of any commercial or financial relationships that could be construed as a potential conflict of interest.
Acknowledgments
We wish to thank M. Cal, R. Rocchetti and S. Marki for help with antiparasitic drug testing, S. Abdelgaffar for help with preparation of extracts, and professors Suad Sulaiman and Marcel Tanner for their mentorship. We gratefully acknowledge financial support by the Amt für Ausbildungsbeiträge Basel and the Emilia Guggenheim-Schnurr Foundation.
Supplementary Material
The Supplementary Material for this article can be found online at: https://www.frontiersin.org/articles/10.3389/fphar.2020.01246/full#supplementary-material
References
Abe, F., Yamauchi, T. (1988). 9α-Hydroxypinoresinol, 9α-hydroxymedioresinol and related lignans from Allamanda neriifolia. Phytochemistry 27, 575–577. doi: 10.1016/0031-9422(88)83144-3
Aderogba, M. A., McGaw, L. J., Bezabih, M., Abegaz, B. M. (2011). Isolation and characterisation of novel antioxidant constituents of Croton zambesicus leaf extract. Nat. Prod. Res. 25, 1224–1233. doi: 10.1080/14786419.2010.532499
Aguilar-Guadarrama, A. B., Rios, M. Y. (2004). Three new sesquiterpenes from Croton arboreous. J. Nat. Prod. 67, 914–917. doi: 10.1021/np030485f
Ahmad, A., Tandon, S., Xuan, T. D., Nooreen, Z. (2017). A Review on Phytoconstituents and Biological activities of Cuscuta species. Biomed. Pharmacother. Biomed. Pharmacother. 92, 772–795. doi: 10.1016/j.biopha.2017.05.124
Ahmed, S. A., Gogal, R. M., Walsh, J. E. (1994). A new rapid and simple non-radioactive assay to monitor and determine the proliferation of lymphocytes: an alternative to [3H]thymidine incorporation assay. J. Immunol. Methods 170, 211–224. doi: 10.1016/0022-1759(94)90396-4
Amaral, A. C., Barnes, R. A. (1997). Alkaloids of Croton celtidifolius. Planta Med. 63, 485. doi: 10.1055/s-2006-957745
Baldim, J. L., de Alcântara, B. G. V., Domingos, O., da, S., Soares, M. G., Caldas, I. S., et al. (2017). The Correlation between Chemical Structures and Antioxidant, Prooxidant, and Antitrypanosomatid Properties of Flavonoids. Oxid. Med. Cell. Longev. 2017:3789856. doi: 10.1155/2017/3789856
Baltz, T., Baltz, D., Giroud, C., Crockett, J. (1985). Cultivation in a semi-defined medium of animal infective forms of Trypanosoma brucei, T. equiperdum, T. evansi, T. rhodesiense, and XXXT. gambiense. EMBO J. 4, 1273–1277. doi: 10.1002/j.1460-2075.1985.tb03772.x
Barberá, O., Marco, J. A., Sanz, J. F., Sánchez-Parareda, J. (1986a). 3-Methoxyflavones and coumarins from Artemisia incanescens. Phytochemistry 25, 2357–2360. doi: 10.1016/S0031-9422(00)81695-7
Barberá, O., Sanz, J. F., Sánchez-Parareda, J., Marco, J. A. (1986b). Further flavonol glycosides from Anthyllis onobrychioides. Phytochemistry 25, 2361–2365. doi: 10.1016/S0031-9422(00)81696-9
Berry, S. L., Hameed, H., Thomason, A., Maciej-Hulme, M. L., Saif Abou-Akkada, S., Horrocks, P., et al. (2018). Development of NanoLuc-PEST expressing Leishmania mexicana as a new drug discovery tool for axenic- and intramacrophage-based assays. PloS Negl. Trop. Dis. 12, e0006639. doi: 10.1371/journal.pntd.0006639
Burchmore, R. J., Barrett, M. P. (2001). Life in vacuoles–nutrient acquisition by Leishmania amastigotes. Int. J. Parasitol. 31, 1311–1320. doi: 10.1016/S0020-7519(01)00259-4
Crestini, C., Caponi, M. C., Argyropoulos, D. S., Saladino, R. (2006). Immobilized methyltrioxo rhenium (MTO)/H2O2 systems for the oxidation of lignin and lignin model compounds. Bioorg. Med. Chem. 14, 5292–5302. doi: 10.1016/j.bmc.2006.03.046
Cunningham, I. (1977). New culture medium for maintenance of tsetse tissues and growth of trypanosomatids. J. Protozool. 24, 325–329. doi: 10.1111/j.1550-7408.1977.tb00987.x
De Rycker, M., Hallyburton, I., Thomas, J., Campbell, L., Wyllie, S., Joshi, D., et al. (2013). Comparison of a high-throughput high-content intracellular Leishmania donovani assay with an axenic amastigote assay. Antimicrob. Agents Chemother. 57, 2913–2922. doi: 10.1128/AAC.02398-12
Desjardins, R. E., Canfield, C. J., Haynes, J. D., Chulay, J. D. (1979). Quantitative assessment of antimalarial activity in vitro by a semiautomated microdilution technique. Antimicrob. Agents Chemother. 16, 710–718. doi: 10.1128/aac.16.6.710
Donnapee, S., Li, J., Yang, X., Ge, A., Donkor, P. O., Gao, X., et al. (2014). Cuscuta chinensis Lam.: A systematic review on ethnopharmacology, phytochemistry and pharmacology of an important traditional herbal medicine. J. Ethnopharmacol. 157, 292–308. doi: 10.1016/j.jep.2014.09.032
Frydman, B., Bendisch, R., Deulofeu, V. (1958). A synthesis of laudanine and (±)-pseudocodamine: Resolution into the optical isomers. Tetrahedron 4, 342–350. doi: 10.1016/0040-4020(58)80055-1
García Díaz, J., Tuenter, E., Escalona Arranz, J. C., Llauradó Maury, G., Cos, P., Pieters, L. (2019). Antimicrobial activity of leaf extracts and isolated constituents of Croton linearis. J. Ethnopharmacol. 236, 250–257. doi: 10.1016/j.jep.2019.01.049
Guerrero, M. F., Puebla, P., Carrón, R., Martín, M. L., San Román, L. (2002). Quercetin 3,7-dimethyl ether: a vasorelaxant flavonoid isolated from Croton schiedeanus Schlecht. J. Pharm. Pharmacol. 54, 1373–1378. doi: 10.1211/002235702760345455
Huber, W., Koella, J. C. (1993). A comparison of three methods of estimating EC50 in studies of drug resistance of malaria parasites. Acta Trop. 55, 257–261. doi: 10.1016/0001-706x(93)90083-n
Ibrahim, A.-R. S., Galal, A. M., Ahmed, M. S., Mossa, G. S. (2003). O-demethylation and sulfation of 7-methoxylated flavanones by Cunninghamella elegans. Chem. Pharm. Bull. (Tokyo) 51, 203–206. doi: 10.1248/cpb.51.203
Janssen, R. H., Wijkens, P., Kruk, C., Biessels, H. W., Menichini, F., Theuns, H. G. (1990). Assignments of1H and13C NMR resonances of some isoquinoline alkaloids. Phytochemistry 29, 3331–3339. doi: 10.1016/0031-9422(90)80210-8
Kaiser, B., Vogg, G., Fürst, U. B., Albert, M. (2015). Parasitic plants of the genus Cuscuta and their interaction with susceptible and resistant host plants. Front. Plant Sci. 6, 45. doi: 10.3389/fpls.2015.00045
Khalid, S. A. (2012). Natural product-based drug discovery against neglected diseases with special reference to African natural resources, in Drug Discovery in African Natural Resources. Eds. Chabile, K., Davies-Coleman, M., Masimirembwa, C. (Berlin, Heidelberg: Springer). doi: 10.1007/978-3-642-28175-4_9
Kuo, P.-C., Yang, M.-L., Hwang, T.-L., Lai, Y.-Y., Li, Y.-C., Thang, T. D., et al. (2013). Anti-inflammatory diterpenoids from Croton tonkinensis. J. Nat. Prod. 76, 230–236. doi: 10.1021/np300699f
Langat, M. K., Crouch, N. R., Smith, P. J., Mulholland, D. A. (2011). Cembranolides from the leaves of Croton gratissimus. J. Nat. Prod. 74, 2349–2355. doi: 10.1021/np2002012
Mahmoud, A. B., Danton, O., Kaiser, M., Han, S., Moreno, A., Abd Algaffar, S., et al. (2020a). Lignans, Amides, and Saponins from Haplophyllum tuberculatum and Their Antiprotozoal Activity. Molecules 25, 2825. doi: 10.3390/molecules25122825
Mahmoud, A. B., Mäser, P., Kaiser, M., Hamburger, M., Khalid, S. (2020b). Mining Sudanese Medicinal Plants for Antiprotozoal Agents. Front. Pharmacol. 11, 865. doi: 10.3389/fphar.2020.00865
Matsuda, H., Morikawa, T., Toguchida, I., Yoshikawa, M. (2002). Structural requirements of flavonoids and related compounds for aldose reductase inhibitory activity. Chem. Pharm. Bull. (Tokyo) 50, 788–795. doi: 10.1248/cpb.50.788
Mohamed, I. E., El Nur, E. E., Choudhary, M. I., Khan, S. N. (2009). Bioactive natural products from two Sudanese medicinal plants Diospyros mespiliformis and Croton zambesicus. Rec. Nat. Prod. 3 (4), 198–203.
Ngadjui, B. T., Abegaz, B. M., Keumedjio, F., Folefoc, G. N., Kapche, G. W. F. (2002). Diterpenoids from the stem bark of Croton zambesicus. Phytochemistry 60, 345–349. doi: 10.1016/s0031-9422(02)00034-1
Okokon, J. E., Nwafor, P. A. (2009). Antiplasmodial activity of root extract and fractions of Croton zambesicus. J. Ethnopharmacol. 121, 74–78. doi: 10.1016/j.jep.2008.09.034
Pirrung, M. C., Lee, Y. R. (1995). Total synthesis and absolute configuration of pseudosemiglabrin, a platelet aggregation antagonist, and its diastereomer semiglabrin. J. Am. Chem. Soc 117, 4814–4821. doi: 10.1021/ja00122a011
Ponnudurai, T., Leeuwenberg, A. D., Meuwissen, J. H. (1981). Chloroquine sensitivity of isolates of Plasmodium falciparum adapted to in vitro culture. Trop. Geograph. Med. 33 (1), 50–54.
Potterat, O., Hamburger, M. (2013). Concepts and technologies for tracking bioactive compounds in natural product extracts: generation of libraries, and hyphenation of analytical processes with bioassays. Nat. Prod. Rep. 30, 546–564. doi: 10.1039/c3np20094a
Potterat, O., Hamburger, M. (2014). Combined use of extract libraries and HPLC-based activity profiling for lead discovery: potential, challenges, and practical considerations. Planta Med. 80, 1171–1181. doi: 10.1055/s-0034-1382900
Räz, B., Iten, M., Grether-Bühler, Y., Kaminsky, R., Brun, R. (1997). The Alamar Blue assay to determine drug sensitivity of African trypanosomes (T.b. rhodesiense and T.b. gambiense) in vitro. Acta Trop. 68, 139–147. doi: 10.1016/s0001-706x(97)00079-x
Ruiz-Olalla, A., Würdemann, M. A., Wanner, M. J., Ingemann, S., van Maarseveen, J. H., Hiemstra, H. (2015). Organocatalytic enantioselective pictet–spengler approach to biologically relevant 1-benzyl-1, 2, 3, 4-tetrahydroisoquinoline alkaloids. J. Org. Chem. 80, 5125–5132. doi: 10.1021/acs.joc.5b00509
Schmidt, T. J., Khalid, S. A., Romanha, A. J., Alves, T. M., Biavatti, M. W., Brun, R., et al. (2012). The potential of secondary metabolites from plants as drugs or leads against protozoan neglected diseases - part II. Curr. Med. Chem. 19, 2176–2228. doi: 10.2174/092986712800229087
Stuart, K., Brun, R., Croft, S., Fairlamb, A., Gürtler, R. E., McKerrow, J., et al. (2008). Kinetoplastids: related protozoan pathogens, different diseases. J. Clin. Invest. 118, 1301–1310. doi: 10.1172/JCI33945
Tasdemir, D., Kaiser, M., Brun, R., Yardley, V., Schmidt, T. J., Tosun, F., et al. (2006). Antitrypanosomal and antileishmanial activities of flavonoids and their analogues: in vitro, in vivo, structure-activity relationship, and quantitative structure-activity relationship studies. Antimicrob. Agents Chemother. 50, 1352–1364. doi: 10.1128/AAC.50.4.1352-1364.2006
Urbatsch, L. E., Mabry, T. J., Miyakado, M., Ohno, N., Yoshioka, H. (1976). Flavonol methyl ethers from Ericameria diffusa. Phytochemistry 15 (3), 440–441. doi: 10.1016/S0031-9422(00)86853-3
Wang, Y., Hamburger, M., Gueho, J., Hostettmann, K. (1989). Antimicrobial flavonoids from Psiadia trinervia and their methylated and acetylated derivatives. Phytochemistry 28, 2323–2327. doi: 10.1016/S0031-9422(00)97976-7
Waterman, P. G., Khalid, S. A. (1980). The major flavonoids of the seed of Tephrosia apollinea. Phytochemistry 19, 909–915. doi: 10.1016/0031-9422(80)85137-5
WHO | World Health Organization (n.d.). WHO. Available at: http://www.who.int/neglected_diseases/diseases/en/ (Accessed 3.4.20).
Wu, X., Zhao, Y. (2004). Advance on chemical composition and pharmacological action of Croton L. Nat. Prod. Res. Develop. 16 (5), 467–472.
Xu, W.-H., Liu, W.-Y., Liang, Q. (2018). Chemical Constituents from Croton Species and Their Biological Activities. Molecules 23 (9), 2333. doi: 10.3390/molecules23092333
Yagi, S., Babiker, R., Tzanova, T., Schohn, H. (2016). Chemical composition, antiproliferative, antioxidant and antibacterial activities of essential oils from aromatic plants growing in Sudan. Asian Pac. J. Trop. Med. 9, 763–770. doi: 10.1016/j.apjtm.2016.06.009
Yahara, S., Domoto, H., Sugimura, C., Nohara, T., Niiho, Y., Nakajima, Y., et al. (1994). An alkaloid and two lignans fromCuscuta chinensis. Phytochemistry 37, 1755–1757. doi: 10.1016/S0031-9422(00)89605-3
Keywords: Croton gratissimus, Cuscuta hyalina, antiprotozoal activity, HPLC activity profiling, flavonoids
Citation: Mahmoud AB, Danton O, Kaiser M, Khalid S, Hamburger M and Mäser P (2020) HPLC-Based Activity Profiling for Antiprotozoal Compounds in Croton gratissimus and Cuscuta hyalina. Front. Pharmacol. 11:1246. doi: 10.3389/fphar.2020.01246
Received: 13 May 2020; Accepted: 29 July 2020;
Published: 14 August 2020.
Edited by:
Banasri Hazra, Jadavpur University, IndiaReviewed by:
Vijay Kumar Prajapati, Central University of Rajasthan, IndiaChiranjib Pal, West Bengal State University, India
Copyright © 2020 Mahmoud, Danton, Kaiser, Khalid, Hamburger and Mäser. This is an open-access article distributed under the terms of the Creative Commons Attribution License (CC BY). The use, distribution or reproduction in other forums is permitted, provided the original author(s) and the copyright owner(s) are credited and that the original publication in this journal is cited, in accordance with accepted academic practice. No use, distribution or reproduction is permitted which does not comply with these terms.
*Correspondence: Abdelhalim Babiker Mahmoud, halim.mahmoud@unibas.ch; Pascal Mäser, pascal.maeser@swisstph.ch