- 1Department of Obstetrics, Gynecology and Reproductive Medicine, University of Maryland School of Medicine, Baltimore, MD, United States
- 2Marlene and Stewart Greenebaum Comprehensive Cancer Center, Baltimore, MD, United States
- 3Cellular and Molecular Medicine, Johns Hopkins University School of Medicine, Baltimore, MD, United States
- 4Department of Pathology, University of Maryland School of Medicine, Baltimore, MD, United States
- 5Baltimore Veterans Administration Medical Center, Baltimore, MD, United States
Eicosanoids, bio-active lipid molecules, evoke a multitude of biological effects that directly affect cancer cells and indirectly affect tumor microenvironment. An emerging role has been shown for eicosanoids in the pathogenesis of gynecological malignancies which include cancers of the vulva, vagina, cervix, uterine, and ovary. Eicosanoid biosynthesis pathways start at the metabolism of phospholipids by phospholipase A2 then proceeding to one of three pathways: the cyclooxygenase (COX), lipoxygenase (LOX), or P450 epoxygenase pathways. The most studied eicosanoid pathways include COX and LOX; however, more evidence is appearing to support further study of the P450 epoxygenase pathway in gynecologic cancers. In this review, we present the current knowledge of the role of COX, LOX and P450 pathways in the pathogenesis of gynecologic malignancies. Vulvar and vaginal cancer, the rarest subtypes, there is association of COX-2 expression with poor disease specific survival in vulvar cancer and, in vaginal cancer, COX-2 expression has been found to play a role in mucosal inflammation leading to disease susceptibility and transmission. Cervical cancer is associated with COX-2 levels 7.4 times higher than in healthy tissues. Additionally, HPV elevates COX-2 levels through the EGFR pathway and HIV promotes elevated COX-2 levels in cervical tissue as well as increases PGE2 levels eliciting inflammation and progression of cancer. Evidence supports significant roles for both the LOX and COX pathways in uterine cancer. In endometrial cancer, there is increased expression of 5-LOX which is associated with adverse outcomes. Prostanoids in the COX pathway PGE2 and PGF2α have been shown to play a significant role in uterine cancer including alteration of proliferation, adhesion, migration, invasion, angiogenesis, and the inflammatory microenvironment. The most studied gynecological malignancy in regard to the potential role of eicosanoids in tumorigenesis is ovarian cancer in which all three pathways have shown to be associated or play a role in ovarian tumorigenesis directly on the tumor cell or through modulation of the tumor microenvironment. By identifying the gaps in knowledge, additional pathways and targets could be identified in order to obtain a better understanding of eicosanoid signaling in gynecological malignancies and identify potential new therapeutic approaches.
Introduction
Eicosanoids, bio-active lipid molecules, elicit a wide range of biological effects that plays a role in physiological and pathophysiological conditions (Harizi et al., 2008; Buczynski et al., 2009; Chhonker et al., 2018). Eicosanoid driven processes have been shown to have increasing importance in the development, progression and metastasis of gynecological malignancies. Gynecological malignancies are cancers that initiate in the reproductive organs of women that include vulvar, vaginal, cervical, uterine, and ovarian cancers. The eicosanoid pathway in cancer has been reviewed in detail (Panigrahy et al., 2010; Wang and DuBois, 2010; Gomes et al., 2018; Umamaheswaran et al., 2018); briefly, arachidonic acid (AA) is liberated from membrane phospholipids by phospholipase A2 (PLA2) and metabolized by one of three pathways cyclooxygenase (COX), lipoxygenase (LOX) and P450 epoxygenase which then produce a wide range of prostanoids, leukotrienes, epoxyeicosatrienoic acids (EETs), and hydroxyeicosatetraenoic acids (HETEs) (Wang and DuBois, 2010; Gomes et al., 2018). The most prominent eicosanoids to be identified to play a role in cancer are those involved in the COX and LOX pathways (Jones et al., 2019); however, products of the cytochrome P450 epoxygenase pathway are shown to play an emerging role in angiogenesis, inflammation and cancer [as reviewed by (Panigrahy et al., 2010)]. In gynecological malignancies, eicosanoids can act directly on the cancer cells, indirectly in the tumor microenvironment and, in many cases, at the confluence of infectious diseases such as HIV and HPV, inflammation and carcinogenesis (De Nola et al., 2019). This review will highlight the role of eicosanoids in the development and progression of gynecological malignancies.
The cyclooxygenase (COX) pathway converts arachidonic acid to PGH2 which is then further converted to 5 different eicosanoids, prostaglandin E2 (PGE2), prostaglandin F2α (PGF2α), prostaglandin D2 (PGD2), Prostaglandin I2 (PGI2) (prostacyclin), and thromboxane (TXA2) by specific PG synthases which are then exported to signal through their cognate receptors (Wang and DuBois, 2010; Reader et al., 2011) (Figure 1). The COX pathway has been the main focus of research especially concerning PGE2. PGE2 binds to four different G protein-coupled receptors EP1-4 which signal to different downstream signaling pathways (Reader et al., 2011). In the lipoxygenase pathway both HETEs and leukotrienes are produced by multiple subfamilies of LOX enzymes: 5-, 8-, 12-, 15-LOX. 5- (ALOX5) and 12-LOX (ALOX12) have been reported to have pro-carcinogenic roles, whereas 15-LOX-2 (ALOX15B) may have an anticancer effect (Guo et al., 2011). The role of 15-LOX-1 (ALOX15) is controversial (as reviewed in Guo et al., 2011). The two major isoforms of 12-LOX are platelet (p12-LOX) and leukocyte (l12-LOX) LOX with pLOX defined as the main 12-LOX in humans. 12-LOX catalyzes the stereospecific oxygenation of AA to form 12(S)-hydroperoxyeicosatetraenoic acid (HPETE), which is converted to 12(S)-hydroxyeicosatetraenoic acid (12-HETE) (Wang and DuBois, 2010; Guo et al., 2011) (Figure 2). It has been hypothesized that HETEs can bind to GPCRs, with 3 identified thus far, and at higher concentrations they can also activate nuclear transcription factors peroxisome proliferator-activated receptors (PPARs) (Panigrahy et al., 2010; Choi and Bothwell, 2012; Powell and Rokach, 2015; Garcia et al., 2017; Hoxha and Zappacosta, 2020). In addition to HETEs, 5-LOX, and 5-LOX activating protein (FLAP) convert AA to a series of leukotrienes including LTA4, LTB4, LTC4, LTD4, and LTE4 that when exported can signal through cognate receptors including leukotriene B4 receptors (BLT) and cysteinyl leukotriene receptors (CysLTR) (Wang and DuBois, 2010).
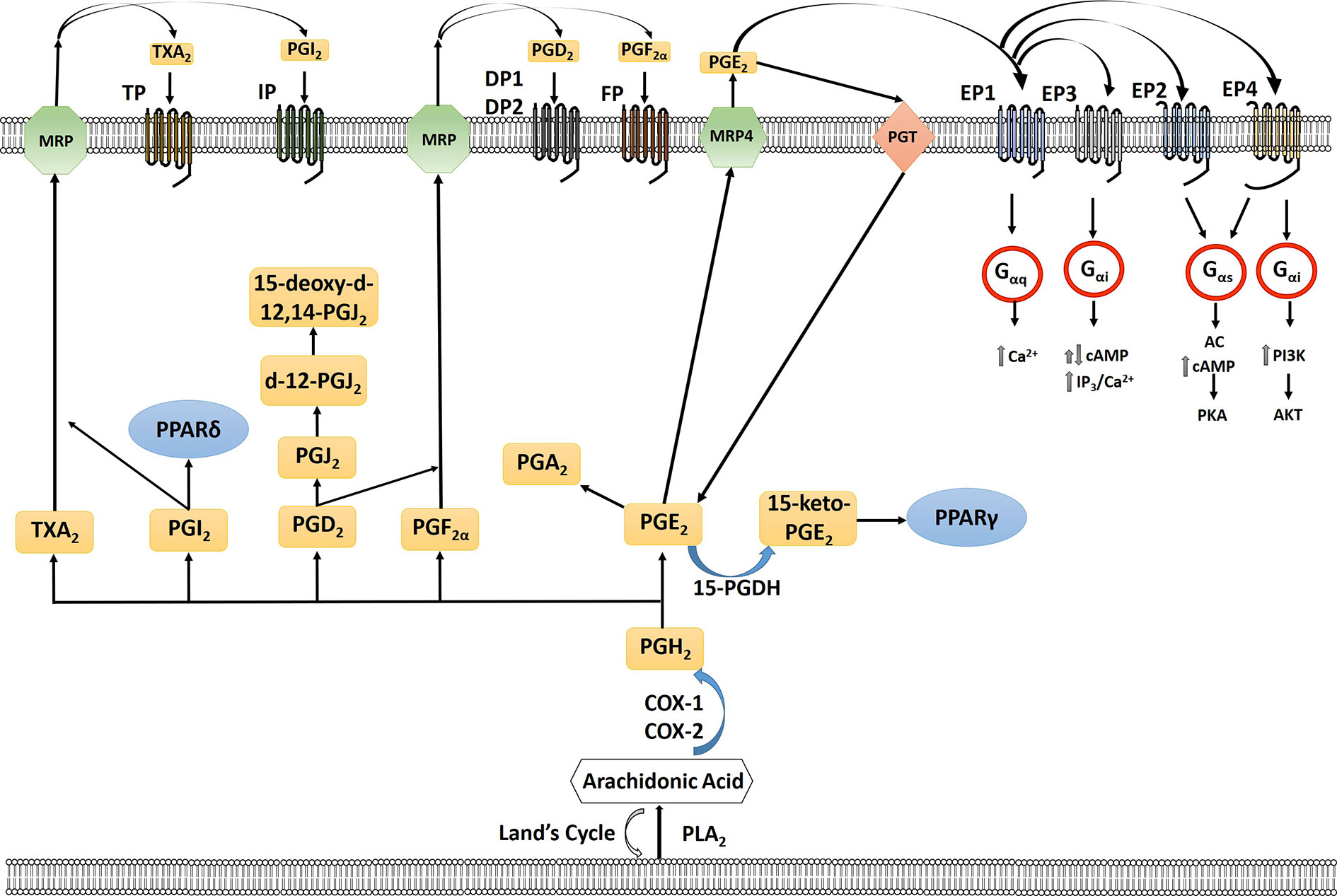
Figure 1 Arachidonic acid (AA) is liberated from the plasma membrane via phospholipase A2 (PLA2). AA can be recycled by the Lands cycle, which is a reacylation/deacylation cycle, that serves to keep the concentration of free AA at a low level. AA is converted by cyclooxygenase 1 or 2 (COX-1/COX-2) to PGH2 which is then converted to PGE2, PGF2α, PGD2, PGI2, or TXA2 by prostaglandin specific synthases. PGE2 is exported out of the cell by multidrug resistance-associated protein four (MRP4) where it can bind to its receptors, the E series of prostaglandin receptors on the plasma membrane, EP1-4. Each of the G-protein-coupled- receptors signal through a different intracellular pathway: EP1 leads to elevation of intracellular calcium through Gαq, EP3, which exists in multiple isoforms, can lead to different responses with the majority acting to inhibit cAMP through Gαi as well as an increase in IP3/intracellular calcium; EP2 and EP4 cause stimulation of cyclic AMP (cAMP) production and protein kinase A (PKA) by sequential activation of Gαs and adenylate cyclase (AC); EP4 can also activate phosphoinositide-3-kinase (PI3K) through Gαi. PGE2 is imported back into the cell through prostaglandin transporter (PGT) where it can either be re-exported or inactivated by 15-hydroxyprostaglandin dehydrogenase (15-PGDH) to 15-keto-PGE2. 15-keto-PGE2 can signal through PPARγ. PGE2 can be converted to PGA2 through a dehydration reaction. PGD2 through a series of dehydrogenation reactions creates PGJ2, delta-12-prostaglandin J2 (d-12-PGJ2) and 15-deoxy-delta12,14-prostaglandin J2 (15-d-PGJ2). PGI2 can either signal through PPARδ intracellularly or exported via multidrug resistance protein (MRP) to signal through the IP receptor. TXA2 is exported out of the cell via MRP to signal through the TP receptor. PGF2α is exported out of the cell via MRP where it can signal via the FP receptor on the cell surface.
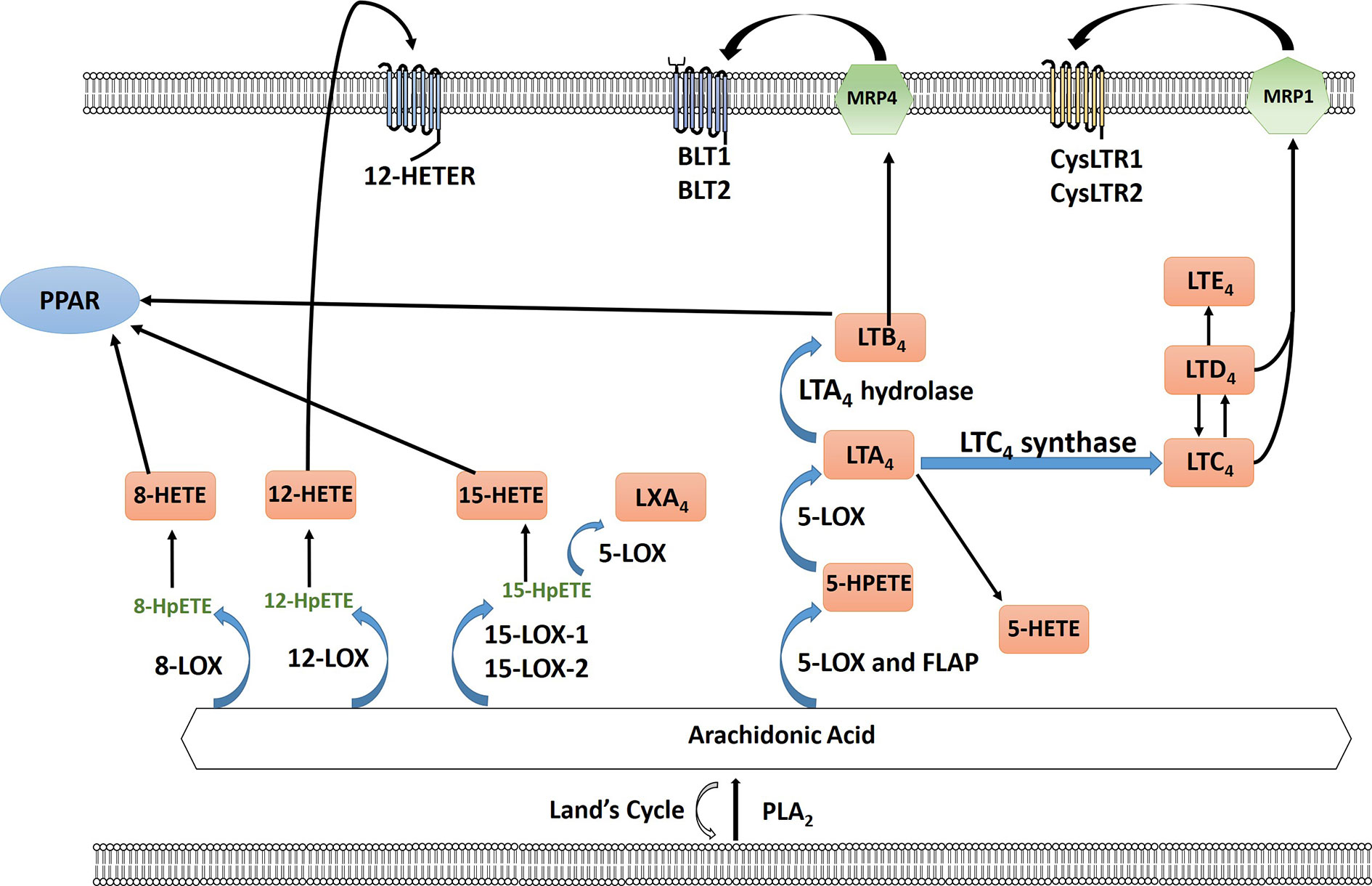
Figure 2 Arachidonic acid (AA) is liberated from the plasma membrane via phospholipase A2 (PLA2). AA can be recycled by the Lands cycle, which is a reacylation/deacylation cycle, that serves to keep the concentration of free AA at a low level. AA is converted by different lipoxygenase enzymes to form either hydroperoxyeicosatetraenoic acid (HPETEs) which is then reduced to the corresponding hydroxy compound. 12-HETE can signal through the G-protein-coupled receptor 12-HETE (12-HETER). 8-HETE, 15-HETE and leukotriene LTB4 can signal through PPAR. 5-LOX and 5-lipoxygenase activating protein (FLAP) convert AA to leukotrienes through a series of reactions using 5-LOX and as well as LTA4 hydrolase and LTC4 synthase including LTA4, LTB4, LTC4, LTD4, and LTE4. LTB4 is exported via multidrug resistance protein 4 (MRP4) to signal through leukotriene B4 receptor BLT1/2. LTC4 and LTD4 is exported via multidrug resistance protein 1 (MRP1) to bind to cysteinyl leukotriene receptor 1 or 2 (CysLTR1/2).
The cytochrome P450 (CYP) epoxygenase pathway, a largely neglected pathway in cancer, leads to the creation of regioisomeric epoxyeicosatrienoic acids (EETs) or HETEs (Panigrahy et al., 2010; Panigrahy et al., 2011). EETs are then metabolized by soluble epoxide hydrolase (sEH) to form the corresponding fatty acid diols (Wang et al., 2019) (Figure 3). Arachidonic acid is metabolized by the CYP ω-hydroxylases to 7-, 10-, 12-, 13-, 15-, 16-, 17-, 18-, 19-, and 20-HETEs, the principal metabolite being the pro-inflammatory 20-HETE (Panigrahy et al., 2010). Although EETs are primarily metabolized by sEH, a few studies have observed that the 5,6-EET and 8,9-EET are but substrates for COX-1 and COX-2 (Zhang et al., 1992; Carroll et al., 1993; Moreland et al., 2007; Rand et al., 2017). Three EET regioisomers were found to be substrates for COX, and EET substrate preference for both COX-1 and COX-2 were estimated as 8,9-EET > 5,6-EET > 11,12-EET, whereas 14,15-EET was inactive. 8,9-EET is metabolized by COX to form ct-8,9-E-11-HET (8,9,11-EHET) and ct-8,9-E-15-HET (8,9,15-EHET) (Choi and Bothwell, 2012; Rand et al., 2017; Rand et al., 2019). Receptors for EETs have only recently been identified. A low affinity receptor for 11,12-EET, GPR40, has been identified in vascular cells that upon stimulation can lead to increase in Cx43 and COX-2 expression in endothelial cells via ERK phosphorylation (Park et al., 2018). A high affinity binding protein that may be a receptor was identified in smooth muscle, endothelial and U937 cells that can bind 8,9-EET, 11,12-EET, and 14,15-EET (Chen et al., 2011). Is it not yet known if these receptors play a role in gynecological malignancies.
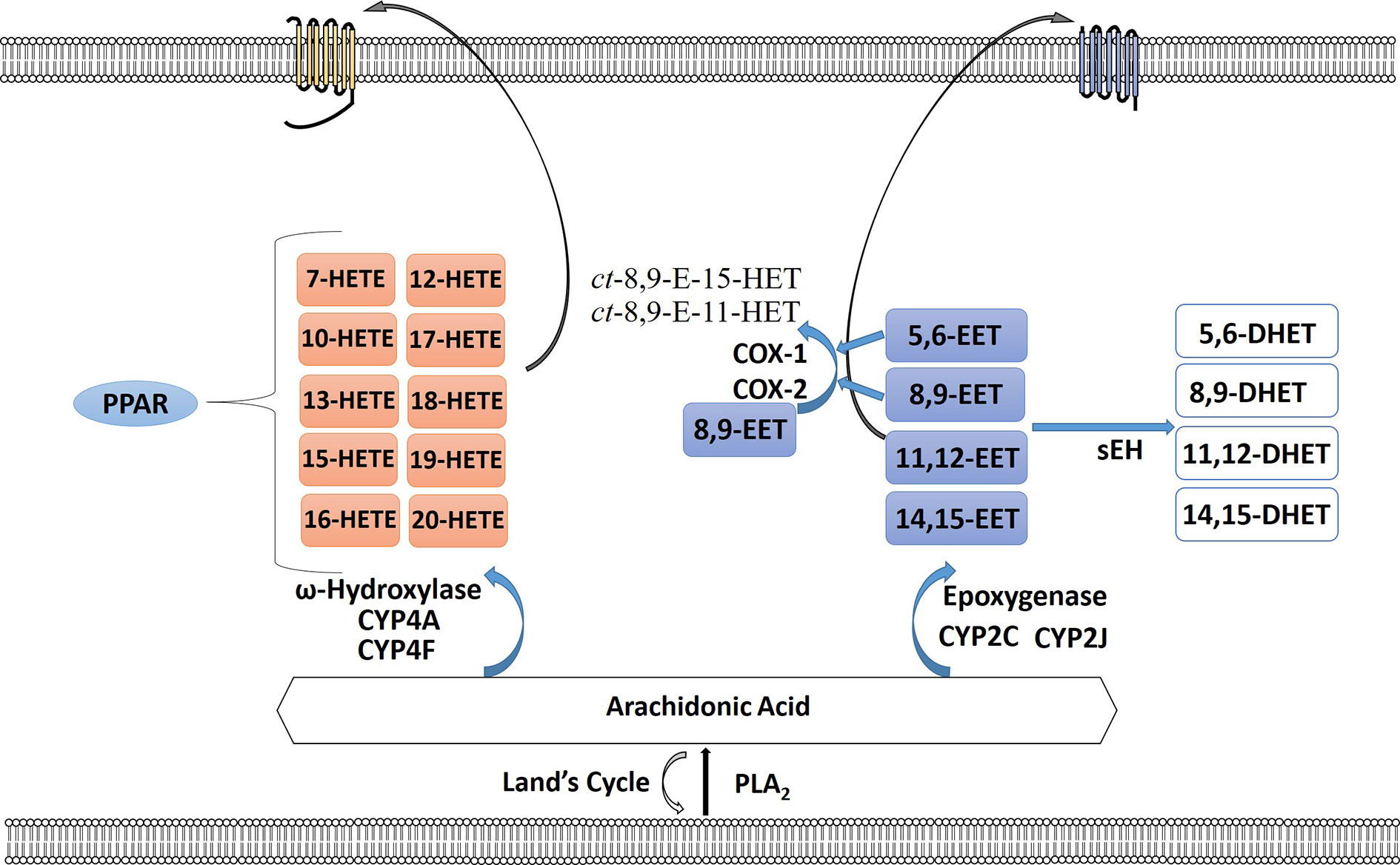
Figure 3 The cytochrome P450 epoxygenase pathway creates a series of regioisomeric epoxyeicosatrienoic acids (EETs) or hydroperoxyeicosatetraenoic (HETEs). Arachidonic acid (AA) is metabolized by the CYP ω-hydroxylases to 7-, 10-, 12-, 13-, 15-, 16-, 17-, 18-, 19-, and 20-HETEs. HETEs can bind to PPAR intracellularly or bind their receptor on the plasma membrane surface. EETs are metabolized by soluble epoxide hydrolase (sEH) to form the corresponding fatty acid diols. 5,6-EET and 8,9-EET are also substrates for COX-1 and COX-2 forming ct-8,9-E-11-HET and ct-8,9-E-15-HET. EETs can also signal through their cognate receptors.
Eicosanoids in Ovarian Cancer
In the United States, ovarian cancer is the second most common gynecologic cancer and leads to more deaths than any other cancer of the female reproductive tract (Desai et al., 2014; Torre et al., 2018). Epithelial ovarian cancer encompasses a heterogenous group that is differentiated by cell, site of origin, pathological grade, risk factors, prognosis, and treatment (Torre et al., 2018). And of those, epithelial ovarian cancer accounts for 90% of all ovarian cancer cases (Torre et al., 2018). Epithelial malignancies are further subdivided into type I or type II based on clinicopathologic features and molecular features. Type I ovarian cancers, with the exception of clear cell, are considered low grade and are thought to usually develop from extraovarian benign lesions that embed within the ovary. Type II ovarian cancers are high grade characterized by aggressive behavior, late stage at diagnosis and low survival (Prahm et al., 2015; Torre et al., 2018). Clear cell, mucinous, low grade endometrioid and low grade serous cancers are type I, whereas high grade serous, high grade endometrioid, carcinosarcomas, and undifferentiated cancers are type II tumors (Jayson et al., 2014; Nezhat et al., 2015; Beeghly-Fadiel et al., 2018).
Epoxygenase and Lipoxygenase Pathways in Ovarian Cancer
While the cyclooxygenase pathway is the most studied pathway in ovarian cancer, several studies have identified a role for the CYP P450 epoxygenase pathway. HETEs produced by CYP mono-oxygenases have been implicated in cancer development, particularly 20-HETE (Chen et al., 2005; Guo et al., 2006; Guo et al., 2007; Alexanian et al., 2009; Alexanian et al., 2012). Alexanian and colleagues have shown that CYP4A/4F genes were increased in ovarian cancer tissue compared to normal tissues and further more CYP4F2 protein and 20-HETE were also higher in ovarian cancer samples (Alexanian et al., 2012) thus demonstrating a potential role for 20-HETE in ovarian cancer which warrant additional mechanistic studies for this pathway.
The lipoxygenase pathway has been identified to play a role in ovarian cancer growth and progression (Figure 2). Women with increased levels of 8-HETE produced by 8-LOX and other free fatty acid metabolites were at higher risk of developing ovarian cancer in the ensuing decade implicating a role for inflammation in the initiation and promotion of ovarian cancer (Hada et al., 2019). 12-LOX expression is higher in high grade serous ovarian cancer compared to normal ovarian tissue. Ovarian cancer cell lines with increased expression of 12-LOX had increased production of 12-HETE. The addition of 12-HETE to ovarian cancer cells leads to an increase in cellular proliferation (Guo et al., 2011). 12-LOX-12-HETE pathway also inhibits apoptosis in ovarian cancer through activation of NF-kB pathway (Liu Q. et al., 2018). The 5-LOX enzyme leads to the creation of both 5-HETE and several leukotrienes. Wen and colleagues reported that hypoxia leads to an increase in 5-LOX metabolites and promotes migration and invasion of tumor associated macrophages and upregulation of MMP-7 (Wen et al., 2015). In cisplatin-resistant ovarian cancer cell line SKOV-3, upregulation of leukotriene B4 and its receptor leukotriene B4 receptor-2 (BLT2) leads to activation of STAT-3 and IL-6 and depletion of BLT2 increased cell sensitivity to cisplatin chemotherapy (Park et al., 2016). In addition, BLT2 also plays a key role in ovarian cancer invasiveness and metastasis (Seo et al., 2012). Transcriptomic analysis of tumor cells and tumor-associated macrophages (TAMs) from ascites identified a synergistic relationship between arachidonic acid, cytokines and disease recurrence (Reinartz et al., 2016). A network of lipid mediators between tumor cells and TAMs were identified which includes products of phospholipid hydrolysis, prostanoids and products of lipoxygenase pathway (Reinartz et al., 2016). These studies highlight the lipoxygenase pathway in modulation of both the tumor microenvironment as well as directly affecting the ovarian tumor cells in the progression of ovarian tumorigenesis.
Cyclooxygenase Pathway in Ovarian Cancer
The cyclooxygenase pathway is the most investigated pathway in ovarian cancers with studies identifying both COX-1 and COX-2 as potential mediators of pro-tumorigenic prostaglandin production. There has been conflicting evidence as to whether COX-1 or COX-2 plays a larger role in ovarian cancer tumorigenesis. Denkert et al. (2002) found that COX-2 expression was an independent prognostic factor for poor survival in ovarian surface epithelial tumors. Various groups have reported differences in COX-1 vs COX-2 expression in different ovarian cancer histological subtypes. Epithelial ovarian cancer demonstrates a high rate of COX-1 and COX-2 expression especially in non-mucinous tumors and a combination of high COX-1 but low COX-2 was associated with poor progression-free and overall survival (Khunnarong et al., 2010). COX-1 was found to be overexpressed in high grade serous ovarian tumors; whereas, COX-2 was highly expressed in endometrioid and mucinous tumors (Wilson et al., 2015). In a follow up study, COX-1 expression was higher in low and high grade serous tumors and type II tumors in comparison to type I tumors while COX-2 was more highly expressed in non-serous and type I tumors (Beeghly-Fadiel et al., 2018).
The association of elevated prostaglandins in the tissues and ascites from ovarian cancer was first reported almost 40 years ago. Prostaglandins PGE2, PGF2α and 6-keto-PGF1α (a degradation product of PGI2) were measured in advanced human ovarian cancer tissues (Bauknecht et al., 1985). In tumors that were resistant to chemotherapy, higher levels of these prostaglandins were detected compared to chemotherapy responsive tumors (Bauknecht et al., 1985). Ascitic fluids from ovarian cancer patients were compared to patients with benign gynecologic conditions and higher amounts of fatty acid palmitoleic acid were measured in cancer patients (Punnonen et al., 1986). Concentrations of PGE2, 6-keto-PGF1α, TxB2, cyclic adenosine monophosphate (cAMP) and cyclic guanosine monophosphate (cGMP) in ovarian tumor tissue were measured in 38 post-menopausal women with malignant or benign ovarian tumors and in six women without ovarian neoplasms (Heinonen and Metsä-Ketelä, 1988). PGE2 and TXB2 contents in ovarian cancer tissue were significantly (P < 0.05) higher than in normal ovarian tissue (Heinonen and Metsä-Ketelä, 1988). Ovarian cancer is more common in patients with elevated gonadotropins such as FSH and LH and they are detectable in ovarian tumor fluid. FSH/LH lead to increased PGE2 production through upregulation of the expression of COX-1 and COX-2 and activation of the PI3K/AKT pathway that ultimately leads to tumor cell migration and invasion (Lau et al., 2010).
Phospholipase A2 in Ovarian Cancer
Phospholipase A2 enzymes, which convert lipids to arachidonic acid, the substrate for the COX enzyme, have been implicated in several cancers. EOC ascites contains high levels of oncogenic lipid growth factors including lipid products produced by PLA2 such as lysophosphatidic acid (LPA) and lysophosphatidylcholine (LPC) (Cai et al., 2012). Human EOC ascites contained microvesicle-free cPLA2 and iPLA2 that were secreted in an ABC transporter dependent mechanism. Chen et al. (2018) reported that the ABCC1 inhibitor MK571 was the most effective inhibitor of PLA2 activity. However, ABCC4 is also inhibited by MK571 and we have found increased expression of ABCC4 (MRP4), the exporter for PGE2, to be overexpressed in multiple ovarian cancer cell lines and tumor tissue; therefore, it is possible that ABCC4 could also play a role in PLA2 secretion (Kochel and Fulton, 2015; Kochel et al., 2017; Ching et al., 2018). Treatment of ovarian cancer cells with chemotherapy drug VP16 causes release of AA resulting in elevation of PGE2 levels and enhancing the repopulation of ovarian cancer cells (Zhao et al., 2017). Berberine can block the caspase 3‐iPLA2‐AA‐COX‐2‐PGE2 pathway by inhibiting the expression of iPLA2 and COX‐2. PGE2 can lead to increased phosphorylation of FAK. Treatment with berberine reverse PGE2 induced FAK phosphorylation thereby leading to inhibition of VP16 induced ovarian cancer cell repopulation (Cui et al., 2017; Zhao et al., 2017). Targeting of cytosolic phospholipase A2 can increase efficacy of low-dose radiotherapy in ovarian cancer (Schulte et al., 2011). These studies demonstrate the role of PLA2 in treatment response and tumor promoting activities such as proliferation, migration and invasion and tumor growth in vivo (Schulte et al., 2011; Cai et al., 2012; Chen et al., 2018).
Prostanoids in Ovarian Cancer
PGE2 signals through four receptors of which two have been identified to play a role in ovarian cancer (Spinella et al., 2004a; Ching et al., 2018; Zhang et al., 2019). There is increased expression of EP4 in ovarian cancer cell lines and primary tumor tissue compared to normal ovarian cell lines and tissue (Ching et al., 2018; Fan et al., 2018). Treatment of SKOV-3 and MDAH-2774 with dmPGE2 lead to a dose dependent increase in COX-2, Bcl-2 and bax expression as well as an increase in proliferation and decrease in apoptosis (Munkarah et al., 2002). Bagnato’s group studied the relationship between endothelin-1, COX and EP receptors in ovarian cancer growth and invasion (Spinella et al., 2004b; Spinella et al., 2004a; Spinella et al., 2004c). Activation of the endothelian A receptor (ETAR) by endothelin-1 (ET-1) leads to an increase in COX-1 and COX-2 expression as well as PGE2 production (Spinella et al., 2004b). ET-1 induced PGE2 production then signals through EP2 and EP4 to stimulate VEGF production. Additionally, signaling through the EP4 receptor leads to an increase in ovarian cancer cell invasiveness (Spinella et al., 2004a). Signaling through the EP2 receptor leads to an increase in proliferation and invasion through the NFkB pathway (Zhang et al., 2019).
There is conflicting evidence on whether PGI2 is anti- or pro-tumoral prostanoid. PGI2 analog iloprost has been shown to have anti-inflammatory and anti-tumoral effects in lung cancer (Lo et al., 1998; Tennis et al., 2010). In ovarian cancer, iloprost inhibited migration and invasion through downregulation of MMP-2 expression (Ahn et al., 2018). In study using engineered OSE cells to form ovarian tumors, tumors demonstrated an increase in COX-1 expression but not COX-2 (Daikoku et al., 2005). Additionally, PGI1, not PGE2, was the major prostaglandin produced by these tumors (Daikoku et al., 2005). Treatment with COX-1 inhibitor SC-560 reduced cell proliferation and accelerated apoptosis; whereas, celecoxib had little effect on tumor growth. SC-560 also decreased PGI2 production by OSE cells and tumors. In a follow up study, Daikoku et al. (2007) demonstrated that aspirin (Cox-1 inhibitor) not only lowered PGI2 levels in T2 and OVCAR-3 cells, but it also attenuates the transactivation of PPARδ, which indicates the inhibition of PG production and PPARδ are downstream targets of COX-1 PGs. This suppression of COX-1- PPARδ signaling by aspirin lead to compromised tumor growth in mice; this result suggests the importance of this pathway to stimulate tumor growth and offers a promising target for ovarian cancer treatment (Daikoku et al., 2007).
Lipid mediators play an important role in both platelet and ovarian cancer functions. Tumor cells can act as agonists for platelets leading to an increase in platelet adhesion, aggregation and degranulation (Serhan et al., 2018). Meanwhile platelets can protect tumor cells from host defenses and increase tumor cell invasion and extravasation (Serhan et al., 2018). Lipid mediators that act as platelet agonists are often produced by tumor cells and are found in the tumor microenvironment. TXA2, PGD2, PGE2, and PGI2 are all important prostanoids for platelet function including activation of platelets, TXA2 and PGE2, or inhibiting platelet aggregation, PGI2 or PGD2 (Friedman et al., 2015; Contursi et al., 2017; Serhan et al., 2018). PGE2 and prostacyclin are found in the ovarian tumor microenvironment linking lipid signaling and platelets in ovarian cancer (Reinartz et al., 2016). In ovarian cancer patients with residual disease, plasma 6-keto-PGF1α (a metabolite of PGI2) in cancer patients (146.7 +/− 14.7 pg/ml, mean +/− SE) was higher (P less than 0.02) than that in the controls (85.3 +/− 9.2 pg/ml, n = 17). Also, the release of TXB2 (a metabolite of TXA2) during spontaneous clotting of the blood samples was greater in the patients than controls (Ylikorkala et al., 1983).
Cyclopentenone prostaglandins of the A and J series, PGA2, PGA1, and PGJ2, which are produced by dehydration of PGE2, PGE1, and PGD2, respectively, have various biological activities including both pro- (Oliva et al., 2003) and anti-tumoral effects (Sasaki and Fukushima, 1994; Straus and Glass, 2001). Sasaki demonstrated that ovarian cancer cell lines resistant to cisplatin, doxorubicin and L-phenylalanine mustard were sensitive to antitumor prostaglandins delta-7-prostaglandin A1 (d7-PGA1) and delta-12-prostaglandin J2 (d-12-PGJ2) (Sasaki et al., 1991) and a derivative of d7-PGA1, 13,14-dihydro-15-deoxy-delta7-prostaglandin-A1-methyl ester, exhibits antitumor activities against cisplatin resistant ovarian cancer cells (Sasaki et al., 1999). McClay and colleagues showed that d-12-PGJ2 can synergize with cisplatin and radiation thus increasing the efficacy of these treatments against ovarian cancer (McClay et al., 1996). 15-deoxy-delta12,14-prostaglandin J2 (15-d-PGJ2) has been shown to strongly induce apoptosis and autophagic cell death pathways and inhibit sirtuin in chemotherapy resistant cell lines (Jong et al., 2011; Tae et al., 2018). Interestingly, expression of PGD2 in high-grade serous ovarian cancer is an independent marker of good prognosis and is associated with increase in disease-free survival, the absence of relapse and sensitivity to platinum-based therapy (Alves et al., 2019) which further links the antitumor properties associated with the PGJ prostaglandins which are derived from PGD2. Given the activity of these metabolites against drug resistant ovarian cancer cell lines, which is what ultimately leads to mortality in patients, warrants additional studies on these compounds.
COX Enzymes in Ovarian Cancer
Several studies have been published supporting the role of COX-1 in ovarian cancer development and progression. Gupta et al. (2003) found increased expression of COX-1 mRNA and protein in ovarian cancer tissues compared to normal ovarian tissue. They also examined the link between the COX pathway and angiogenesis; ovarian epithelial cells with high COX-1 expression exhibit high levels of transcription factors including HIF-1α, VEGF, VEGF receptor: Flk-1. In a follow up study, a genetically engineered mouse model of ovarian EOC was used to evaluate whether COX-1 expression is linked to mutations in tumor suppressors p53 and Rb (Daikoku et al., 2006). In mice with these mutations, high COX-1 mRNA levels were detected in well differentiated serous epithelial neoplasms; COX-2 levels remain to be undetectable and this is consistent in situ tumor samples (Daikoku et al., 2006). In a follow up study, peroxisome proliferator-activated receptor δ (PPARδ), a downstream target of COX-1, is directly activated by PGI2 and transactivated by PGE2, stimulates cell proliferation and tumor growth in cancers like mammary and hepatocellular, and was found to be overexpressed in mouse ovarian cancer cell lines (Daikoku et al., 2007). COX-1 has been identified to also produce prostaglandins in ovarian cancer cell lines. Increased levels of COX-1 were identified in 3 out of 10 ovarian cancer cell lines, PGE2 production was also elevated in those cell lines (Kino et al., 2005). Non-selective COX inhibitor (indomethacin) and a selective COX-1 inhibitor (SC-569) significantly decreased PGE2 production; however, COX-2 inhibitors, NS-398 and rofecoxib, did not affect PGE2 production which suggests that COX-1 is regulating the production of PGE2 in these cell lines (Gupta et al., 2003; Kino et al., 2005; Rask et al., 2006).
In addition to COX-1, COX-2 has also been shown to play a role in ovarian cancer progression. COX-2 is constitutively expressed in various EOC cell lines; introduction of PGE2 increases COX-2 expression, increases proliferation as well as reduces apoptosis (Munkarah et al., 2002). COX-2 derived PGE2 also promotes ovarian cancer cell invasion through an epidermal growth factor (EGF) signaling mechanism in which a positive feedback loop leads to increases in COX-2, PGE2 and EGF (Qiu et al., 2014). COX-2 can also lead to an increase in pro-angiogenic proteins. The expression of nerve growth factor (NGF) is frequently overexpressed in EOC leading to an increase in VEGF, β-catenin/TCF-Lef, survivin, and MYC. Garrido et al. (2019) identified that this was due to a NGF-regulated increase in COX-2/PGE2 signaling. Dursun et al. (2005) hypothesize that the difference between invasive ovarian tumors and borderline ovarian tumors, which are less threatening in terms of survival, is the level of COX-2 expression. Overexpression of COX-2 stimulates prostaglandin production and cellular growth, reduces apoptosis enzymes, promotes mutagenesis, tumorigenesis, and angiogenesis (Dursun et al., 2005). COX-2 positive ovarian cancer cases also have higher expression of MDR1/P-glycoprotein (P-gp). P-gp is a drug efflux pump whose presence is associated with unfavorable significance in ovarian cancer treated with chemotherapy and may be upregulated by COX-2. Previously, Denkert et al. (2002) demonstrated that expression of COX-2 is an independent unfavorable prognostic factor in ovarian cancers. In a follow up study, they have extended these findings to demonstrate a positive correlation between COX-2 and P-gp expression in human ovarian carcinomas and unfavorable prognostic and predictive significance in tumors expressing COX-2 and/or P-gp (Surowiak et al., 2006). Combination treatment with cisplatin or docetaxel with NSAID, NS-398 or sulindac, can lead to dose-dependent enhancement of cytotoxicity which could be enhanced under hyperthermic conditions (41°C) (Barnes et al., 2007). Denkert et al. (2003) investigated COX enzyme dependent vs independent effects of the NSAID NS-398 on ovarian cancer cell lines that are either positive for COX-1/2, OVCAR-3, or negative for COX-1/2 expression, SKOV-3. They demonstrated that NS-398 could inhibit proliferation through induction of cell cycle arrest that is independent of COX-2 inhibition and the inhibitory effect could not be rescued with the addition of PGE2 (Denkert et al., 2003).
COX inhibitors have been explored as both a monotherapy and as well in combination with chemotherapeutics. Li and colleagues investigated the effect of COX-1 and COX-2 inhibitors as a monotherapy and as well as in combination with taxol on SKOV-3 xenograft mouse model (Li et al., 2008; Li et al., 2010; Li et al., 2011; Li et al., 2012a; Li et al., 2012b; Li et al., 2013). Nimesulide (COX-2 inhibitor) treatment of mice with SKOV-3 xenograft tumors leads to a reduction in microvessel density (MVD) and PGE2 levels but did not significantly decrease tumor growth (Li et al., 2008). This led Li to examine the effects of COX-1 inhibitor, SC-560, and COX-2 inhibitor, celecoxib, or dual inhibitor, indomethacin, on xenograft tumor growth. Monotherapy treatment was not effective in decreasing tumor volume; however, dual SC-560 and celecoxib inhibitor significantly reduced relative tumor volume (Li et al., 2012a). Single treatment with SC-560 or celecoxib also significantly prolonged survival of the mice compared to control. Previously, Gupta et al. (2003) reported that COX-1 and pro-angiogenic proteins were overexpressed in ovarian tumors and treatment with COX-1 inhibitor SC-560 but not COX-2 inhibitor celecoxib lead to a decrease in secretion of VEGF by OVCAR-3 cells in vitro. Li found similar results in their in vivo studies (Li et al., 2012a). Li and colleagues also investigated the combination of SC-560 and taxol as well as celecoxib and taxol on the effect of SKOV-3 tumor MVD, apoptosis and VEGF transcript levels (Li et al., 2012a). Taxol in combination with SC-560 was superior to taxol with celecoxib and while the authors did not provide an explanation for the observed differences in the two inhibitors, the potential remains for the possibility of off target or non-COX related effects of the inhibitors (Li et al., 2012a; Li et al., 2013). Using a combination of siRNAs against COX-1/COX-2, Denkert et al. (2003) examined the role COX-2-dependent and COX-2 independent effects of COX inhibitors on ovarian cancer proliferation. As opposed to the predominant role of COX-1 in SKOV-3 cells, in OVCAR-3 cells, COX-2 was responsible for PGE2 production and production could be inhibited with COX-2 inhibitor NS-398. COX-1/COX-2 inhibitors lead to a decrease in proliferation that was independent of COX activity and expression through induction of Go/G1 cell cycle arrest. Uddin et al. (2010) examined the molecular pathways involved in COX-2 inhibition in ovarian cancer. COX-2, but not COX-1, expression was associated with expression of pAKT in ovarian cancer tumor tissue. In MDAH2774 and SKOV3 cell lines, aspirin and NS-398 also lead to a decrease in proliferation and induction of apoptosis. While the authors did not explore specific EP receptors in this study, EP4 has been shown to activate PI3K/AKT (Hsu et al., 2017). Given the results of these studies the possibility exists that COX independent effects play a significant role in the function of COX inhibitors.
Cyclooxygenase in Ovarian Cancer Tumor Microenvironment
The innate and adaptive immune system plays an essential role in the progression and spread of cancer. The ovarian cancer microenvironment is highly immune suppressive (Preston et al., 2011). Natural killer cells (NK), part of the innate immune system, can lyse cells without first having to recognize specific antigens and have been reported to play an essential role in controlling metastasis in breast cancer with contradictory roles in ovarian cancer (Dong et al., 2006; Holt et al., 2012; Sun et al., 2018). Myeloid-derived suppressor cells (MDSC), which are a heterogenous population of macrophages, DCs and granulocytes at early stages of differentiation, is another driver of immune suppression with the ability to block local and systemic immune activation (Preston et al., 2011). TAMs are one of the most abundant immune cells in the tumor microenvironment (Colvin, 2014). These heterogenous cells can act to either stimulate or suppress the immune response and these opposing behaviors have been classified as either classically activated, M1, or alternatively activated, M2 (Colvin, 2014). M1-polarized macrophages produce pro-inflammatory and immunostimulatory cytokines, are involved in TH1 responses and are considered cancer killing; whereas, M2-polarized macrophages are immunosuppressive, associated with the TH2 response and are considered cancer promoting (Colvin, 2014). Dendritic cells (DC) can either activate adaptive immunity or tolerize T cells specific for tumor-associated antigens (Pardoll, 2015). Cytotoxic T cells (CTL) exhibit antitumor immune functions with CD8+ cytotoxic T cells generally are the primary mediators of antitumor immune response. Helper T cells, which are required to activate cytotoxic T cells, are functionally divided into subsets including TH1 and TH2 according to secretory cytokines and immunologic roles (Lee et al., 2019). The TH1/TH2 balance plays an essential role in immune response to cancer with TH1 eliciting an anti-tumor response whereas TH2 is pro-tumorigenic (Burkholder et al., 2014).
Mucin molecules (MUCs) are aberrantly secreted from ovarian cancer cells. There is a positive correlation with five year survival rate in patients with high density of M1 TAMS and a high M1/M2 ratio and therapeutic targeting against M2 inhibits disease progression (Luo et al., 2006; Mantovani et al., 2006; He et al., 2013). He et al. showed that an increased concentration of COX-2+ cancer cells, decreased ratio of M1/M2 TAMs, and an increased concentration of COX-2+ TAMs was associated with poor prognosis (He et al., 2013). Populations of M2 TAMs and COX-2+ TAMs frequently converged. There was also an association between COX-2+ cancer cells and COX-2+ TAMs (He et al., 2013). Expression of MUC2 can result in M2-polarization and induce TAM COX-2 expression. The polarization of M2 TAMs and expression of COX-2 can lead to an increase in PGE2 in the microenvironment by TAMs and cancer cells which can lead to an acceleration of ovarian cancer progression (He et al., 2013).
MDSCs expand during cancer and can suppress T-cell facilitated responses (Gabrilovich and Nagaraj, 2009; Ostrand-Rosenberg and Sinha, 2009; Rodríguez-Ubreva et al., 2017). Myeloid cell differentiation is highly dependent on DNA methylation changes and pro-inflammatory mediators such as PGE2 regulate MDSC accumulation (Sinha et al., 2007; Álvarez-Errico et al., 2015; Rodríguez-Ubreva et al., 2017). Rodríguez-Ubreva et al. (2017) demonstrated that ovarian cancer cells can induce differentiation of monocytes to MDSCs by causing hypermethylation of MDSC specific genes by signaling through the PGE2 EP2 and EP4 receptors leading to upregulation of DNMT3A. DCs and MDSCs show opposing roles in the immune system, suppression of DCs contributes to cancer progression while MDSCs suppress the ability of CD8+ T cells to mediate effective anti-tumor response (Rodríguez-Ubreva et al., 2017). COX-2-PGE2 leads to positive feedback signaling that results in blocking CD1α+ DC differentiation and leading to induction of CD14+CD33+CD34+ monocytic MDSCs (Obermajer et al., 2011). The frequency of CD11b(+)CD33(+) MDSCs in ovarian cancer are closely correlated with local PGE2 production. Inhibition of EP2 and EP4 or interruption of COX-2-PGE2 feedback using COX-2 inhibitors decreases the production of suppressive mediators and inhibitory functions of MDSCs from cancer patients.
Wong et al. (2016) observed an unexpected increase in both type-1 mediators, IFNγ and TNFα, induction of immune suppressive COX-2 and hyperactivation of MDSCs in the tumor microenvironment of ovarian cancer patients. The synergistic action of both IFNγ and TNFα which lead to increased MDSC activity and expression of IDO1, NOX2, IL-10 and COX-2 mRNA and protein was dependent on COX-2-PGE2 such that blockade of COX-2 was able to completely reverse enhanced suppressive ability of the hyperactivated MDSCs (Wong et al., 2016). Blockade of COX-2 or the EP4 receptor reduces expression of IDO1, an immunosuppressive enzyme, leading to an increase in CD3+ and CD8+ cells thus increasing T cells and control of tumor growth (Hennequart et al., 2017). While IL-10 and PGE2 were identified as having immunosuppressive capabilities in ascites from ovarian cancer patients, PGE2 was found to be the more important of the two that leads to impaired T cell stimulatory capacity of DC cells (Brencicova et al., 2017). Cytotoxic T cells directed against endogenously expressed antigens are key for antigen-specific cancer immunotherapy. COX-2-PGE2 was identified as resistance factors for suppression of antigen-induced interferon-gamma secretion of T cells and generation of factors that suppress the immune system leading to escape from immune surveillance and resistance to cellular immunotherapy (Göbel et al., 2014).
Maintenance of CTL, TH1 and NK cell-mediated type I immunity is key for effective antitumor responses. Liu et al. (2009) analyzed the pattern of CD8+ (CTL), CD57+ (NK), and CD1α+ (DC) infiltrating immune cells in intraepithelial or stromal spaces with survival and COX-1 and COX-2 expression. PGE2 can alter the TH1/TH2 balance, suppress DC function, and increase the number of tumor-infiltrating regulatory T cells (Liu et al., 2009). Higher levels of COX-2 expression occurred more frequently in serous ovarian carcinoma compared to other types (P<0.05) and higher levels of COX-1 or COX-2 tended to correlate with poor prognosis (n.s.) (Liu et al., 2009). COX may impact ovarian cancer prognosis and the pattern of tumor-infiltrating immune cells. Ovarian cancers were analyzed by hierarchical cluster analysis based on the number of tumor-infiltering immune cells and three different clusters were generated (Liu et al., 2009). Cluster 1 was characterized with the worst progression free survival and overall survival. Cluster 1 was characterized by higher expression of COX-1 and COX-2 compared to cluster 2 (P<0.05, respectively). The presence of intraepithelial CD8+ cells was negatively correlated with COX-1 and COX-2 expression (P<0.05 for both) (Liu et al., 2009). It is clear that PGE2 via COX-1/COX-2 expression plays a critical role in the ovarian cancer tumor microenvironment leading to immune suppression through multiple mechanisms. The COX/PGE2 pathway warrants additional studies including combining PGE2 EP4 inhibitors with immunotherapy in ovarian cancer.
Eicosanoids in Uterine Cancer
Uterine cancer is the fourth most common malignancy as well as the most common gynecologic malignancy (Siegel et al., 2019). There are two subgroups, Type I, endometrioid cancer, which is the most common, is associated with expression of hormonal receptors and, Type 2, unrelated to estrogen, and includes histological types such as serous, clear cell, mucinous and uterine sarcomas (Creutzberg and Fleming, 2016; Di Tucci et al., 2019).
Lipoxygenase in Uterine Cancer
The majority of the research published to date discusses the role of prostanoids in uterine cancer; however, the lipoxygenase pathway has been shown to be involved in type 2 endometrial cancer. ALOX5 (5-LOX) is generally reported to be absent in normal epithelial but is induced by pro-inflammatory stimuli and can be overexpressed in various epithelial cancers (Pidgeon et al., 2007; Wang and DuBois, 2010). In a study performed by Cummings et al. (2019), there is a significant increase in ALOX5 (5-LOX) mRNA expression in type II endometrial cancer compared to normal endometrium and elevated ALOX5 expression is associated with adverse outcomes. Given the limited number of studies available on the role of lipoxygenase as well as the lack of studies in the cytochrome P450 pathway in uterine cancer, these pathways are ones that should be evaluated in uterine cancer in the future.
Cyclooxygenase in Uterine Cancer
Uterine leiomyosarcoma (LMS) is the second most common subtype of uterine sarcoma with uterine leiomyomas comprising the most common benign pelvic tumors in women (Sabry and Al-Hendy, 2012; Kobayashi et al., 2013). Leiomyomas release prostaglandins including 6-keto-PGF1α, PGF2α and PGE2 (Rees and Turnbull, 1985). Endothelin (ET)-1 exerts survival effects in uterine leiomyoma cells and leads to increased protein expression of COX-2 and PGE2 production (Oyeniran and Tanfin, 2011). COX-2 expression was higher in uterine fibroids compared to healthy smooth muscle cells and selective COX-2 inhibitor celecoxib decreased fibroid cell proliferation and PGE2 secretion (Ke et al., 2013). Recently, in a study published by Reader et al. (2019), the role of EP4 was evaluated in LMS proliferation and migration. While minimal effects in proliferation was observed with monotherapy treatment of LMS with an EP4 inhibitor when combined with docetaxel treatment, we saw a sensitization of LMS cells to chemotherapeutic treatment (Reader et al., 2019). In addition to sensitization to chemotherapy, we also demonstrated a significant decrease in LMS cell migration. PI3K has been shown to play a significant role in the development of uterine cancer and activation of EP4 can lead to activation of the PI3K pathway (Figure 1) (Naumann, 2011).
One common inflammatory disease that affects approximately 10% of reproductive aged women is endometriosis, which causes chronic pain and infertility, and is defined as the presence of endometrium-like tissue outside the uterus (As-Sanie et al., 2019). Epidemiological, biological, and molecular data all indirectly suggest links between the endometriosis and endometrial cancer (Painter et al., 2018; As-Sanie et al., 2019). In 1992, Koike et al. (1992) identified increased production of 6-keto-PGF1α, TXB2, and PGE2 in endometrial cysts compared to non-endometrial cysts and normal ovaries. Additionally, the COX-2 pathway promotes survival, migration, and invasion of endometriotric cells and promotes angiogenesis during endometriosis progression (Banu et al., 2008; Jana et al., 2016). Inhibition of EP2 and EP4 leads to apoptosis of endometriotic cells as well as a decrease of human endometriotic epithelial and stromal cells through integrin-mediated mechanisms (Banu et al., 2009; Lee et al., 2013). As indicated in these studies a relationship exists between COX eicosanoids in the pathogenesis of endometriosis and therefore endometrial cancer.
COX-1 and COX-2 are important in normal reproduction as reviewed in (Sales and Jabbour, 2003). COX enzymes convert arachidonic acid to PGH2 which is then metabolized by specific synthases to thromboxanes and prostaglandins including PGE2 and PGF2α (Wang and DuBois, 2010). PGE2, EP2 and EP4 receptors work in an autocrine/paracrine role in the epithelial/endothelial cell function in normal human endometrium (Milne et al., 2001). During the menstrual cycle, signaling of EP2/EP4 receptors produced a greater response in proliferative tissue compared with early and midsecretory stage tissue. COX-2 expression is up-regulated in neoplastic epithelial and endothelial cells of endometrial carcinomas (Tong et al., 2000; Jabbour et al., 2001; Ferrandina et al., 2002; Uotila et al., 2002; Jabbour et al., 2006). Lower risk of endometrial cancer was reported when aspirin and other nonsteroidal anti-inflammatory agents were used in patients which could block production of prostaglandins (Black et al., 2014). The importance of COX-2 in the early stages of endometrial cancer development was shown using a conditional endometrial-specific phosphatase and tensin homologue gene (PTEN) knockout mouse model (Daikoku et al., 2008). Dual inhibition of COX-2 and mTORC1 signaling markedly reduces endometrial cancer progression (Daikoku et al., 2014). Paclitaxel resistance may be associated with COX-2 and multidrug resistance 1 (MDR1) expression, an efflux pump that can transport a wide range of compounds including chemotherapy out of the cell, in endometrial cancer (Hasegawa et al., 2013). Co-administration of COX-2 inhibitor etodolac with paclitaxel leads to a decrease in MDR1 expression which may enhance accumulation of MDR1 substrates such as paclitaxel thus leading to an increase in paclitaxel sensitivity (Hasegawa et al., 2013). However, recently Cummings, et al. using laser capture microdissection, whole genome expression analysis and liquid chromatography-tandem mass spectrometry, did not show an increase in COX isoform expression nor PGE2/PGF2α levels in neoplastic endometrium compared to sample matched epithelial tissue (Cummings et al., 2019). The authors do suggest that an increase in local levels of PGE2 and PGF2α could occur due to decreased catabolism, via downregulation of HPGD (Figure 1). There was an increase in EP3 (PTGER3) and EP4 (PTGER4) receptor gene expression in type I and type II endometrial cancers which could lead to an increase in local PGE2-EP3/EP4 mediated signaling. In addition, our group has observed an increase in EP4 expression in primary endometriod cancer tissue (Reader et al., 2016). The differences could be due to the fact that the tissues used for this study were microdissected instead using the entire tissue for analysis as well as the use of fewer clinical samples in previous studies.
Prostanoids in Uterine Cancer
The F and E prostanoid pathways have been shown to contribute to endometrial cancer phenotypes including proliferation, adhesion, migration, invasion, angiogenesis, and inflammatory microenvironment by binding with their respective G-protein coupled receptors (Sales et al., 2004b; Sales et al., 2005; Sales et al., 2008a; Keightley et al., 2010b; Wallace et al., 2010; Zhu et al., 2017). PGF2α exerts its action via FP receptors of which two isoforms exists generated via alternative mRNA splicing designated FPA and FPB. These isoforms are coupled to Gαq and can produce IP3 through activation of phospholipase C, intracellular calcium flux and activation of protein kinase C (Sales and Jabbour, 2003). PGF2α plays an essential role in parturition and regulation of expression of the oxytocin receptor. PGE2 can be converted to PGF2α adding a layer of crosstalk and complexity (Dozier et al., 2008) (Figure 4). Downstream signaling of FP and EP receptors leads to an increase in COX-2 expression (Fujino and Regan, 2003; Sales et al., 2008b). PGE2 can act as an agonist for FP receptors thus PGE2 and PGF2α when locally produced by endometrial adenocarcinomas can regulate tumor cell function in an autocrine/paracrine manner by the FP receptor leading to an induction of COX-2 expression (Sales et al., 2008b). PGF2α and FP receptor interaction enhances proliferation of endometrial epithelial cells (Sales et al., 2005; Sales et al., 2007). There is co-localization of COX-2 and the FP receptor within neoplastic epithelial cells of endometrial adenocarcinoma and activation of PGF2α-FP receptor lead to an increase in COX-2 expression through activation of ERK1/2 pathway which leads to a concomitant increase in PGF2α creating a positive feedback loop (Jabbour et al., 2005). Another positive feedback involving FGF2-FGFR1 interaction that results in elevated FGF2 and COX-2 expression and enhanced proliferation via the FGFR1 and ERK pathways (Sales et al., 2007). In addition to proliferative changes, activation of the FP receptor can lead to an increase in adhesion, migration and invasion of endometrial adenocarcinoma cells. Alterations in cell adhesion and increased migration occur through a αVβ3-ECM dependent mechanism after PGF2α-FP activation (Sales et al., 2008a) and regulation of endometrial adenocarcinoma cell invasion occurs via increased expression of disintegrin and metalloprotease with a thrombospondin repeat 1 (ADAMTS1) (Keightley et al., 2010b). Chemokines play an important role in normal uterine function; however, dysregulated chemokines can contribute to uncontrolled proliferation, migration and invasion and contribute to an inflammatory microenvironment (Kayisli et al., 2002). Chemokines CXCL8 and CXCL1 are both targets of PGF2α (Sales et al., 2009; Wallace et al., 2009). CXCL8 leads to increased proliferation of Ishikawa cells in vivo (Sales et al., 2009) while CXCL1induces neutrophil chemotaxis in vitro and in vivo (Wallace et al., 2009). The effects of these chemokines presumably also involve activation of the PGF2α receptor. A pro-angiogenic and anti-angiogenic relationship has been described as a result of PGF2α and FP receptor interactions (Sales et al., 2005; Keightley et al., 2010b; Keightley et al., 2010a). PGF2α and FP receptor activation enables endothelial cell network formation and proliferation via FGF2-FGFR1 signaling in vitro (Keightley et al., 2010a) and through transactivation of EGFR and secretion of VEGF leads to an increase in angiogenesis in vivo (Sales et al., 2005). Elevated COX-2 is associated with increased VEGF, PGE2 and angiogenesis in endometrial cancer and can be negatively regulated by miR-101 and while a specific receptor was not identified in this study it is feasible that FP and PGF2α could play a role by conversion of PGE2 to PGF2α (Dozier et al., 2008; Liu Y. et al., 2018).
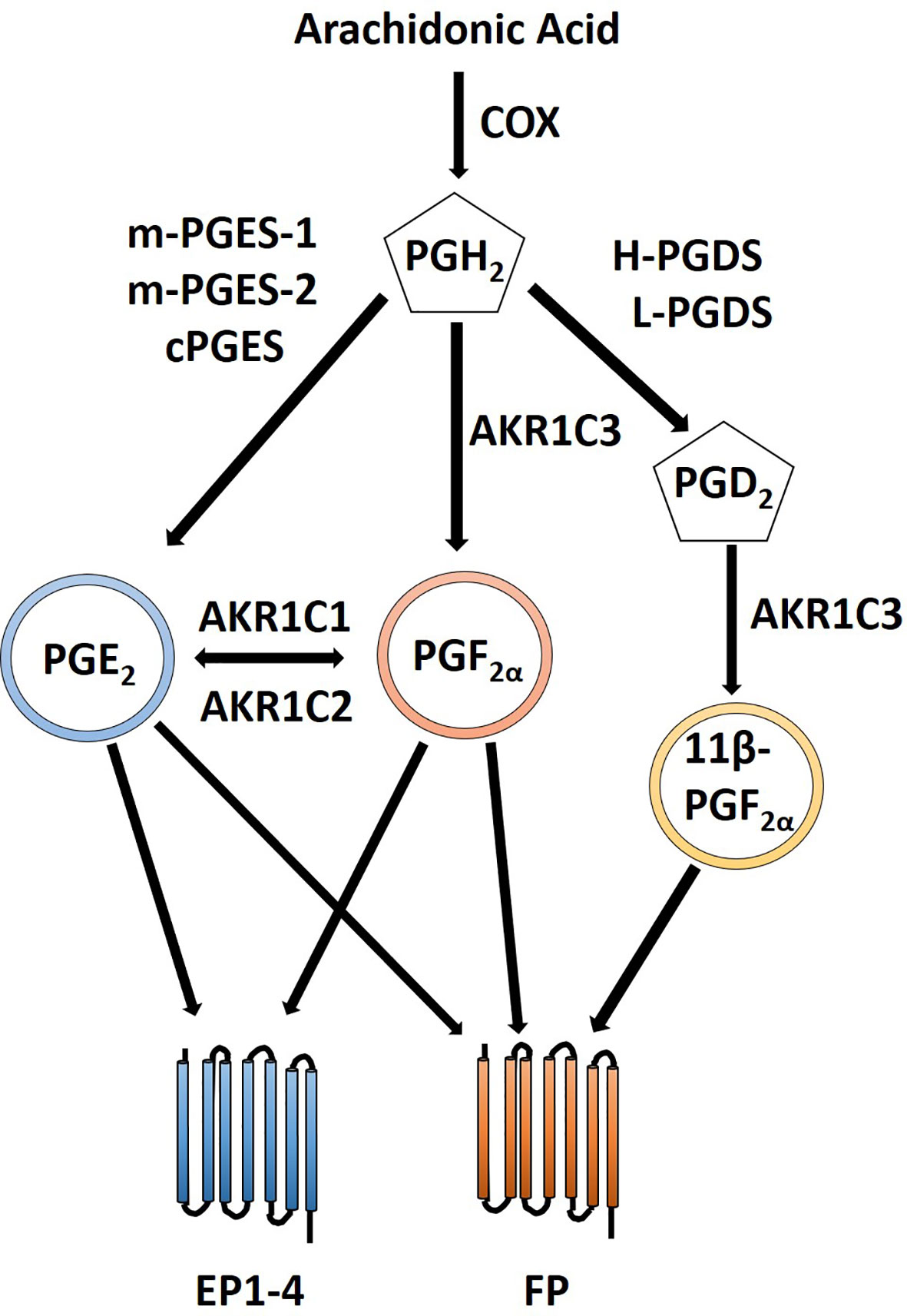
Figure 4 Crosstalk between PGE2 and PGF2α prostanoid pathways. PGE2 is created via conversion of PGH2 by PGE synthases including m-PGES-1, m-PGES-2 and cPGES. Likewise, PGF2α is formed by conversion of PGH2 by AKR1C3. Additionally, PGF2α can be created from PGE2 by AKR1C1 or AKR1C2. 11β-PGF2α, a stereoisomer of PGF2α, can also signal via the FP receptor and is created by the PGD2 prostanoid pathway with an additional conversion by AKR1C3. PGE2 can signal through its cognate PGE2 EP receptors as well as the through PGF2α FP receptor. Similarly, PGF2α can signal through its FP receptor as well as through PGE2 EP receptors.
Cyclooxygenase in Uterine Tumor Microenvironment
The production of PGE2 by malignant endometrial epithelial cells in the tumor microenvironment can cause an increase in COX-2 expression. In normal endometrial cells, activation of transcription via NF-kB and stabilization of COX-2 mRNA leads to production of PGE2 (Tamura et al., 2002). PGE2 signals through four different E series receptors EP1-EP4 which are linked to different intracellular signaling pathways (Figure 1). The expression of the EP1 receptor was only increased in endometriotic tissue compared to healthy endometrium and tumor tissue; and in the tumor stroma, the expression of EP1 in the tumor was lower than in both normal tissue and endometriosis (Zhu et al., 2018). There was no significant differences in EP1 staining in either epithelium or in stroma within histological stage, grading, metastatic and recurrent subtypes (Zhu et al., 2018). High expression of EP3 was associated with impaired progression free survival and inhibition of EP3 signaling lead to a decrease in proliferation and migration in RL95-2 endometrial cancer cells (Zhu et al., 2017). Compared to EP1 and EP3, the EP2 and EP4 receptors are the most prominent receptors in regard to playing a role in uterine cancer pathogenesis. Jabbour et al. (2001) demonstrated an increase in expression of EP2 and EP4 receptors in endometrial adenocarcinomas and signaling through these receptors resulted in significantly higher cAMP production in response to PGE2. Signaling of the EP2 receptor in endometrial adenocarcimoma leads to transactivation of EGFR through PKA and c-Src and induction of VEGF (Sales et al., 2004b). Abera et al. (2010) reported on crosstalk between FP and EP2 receptors that results in increased cAMP release via the Gαq-Ca2+-calmodulin pathway. Endogenous PGE2 and exogenous PGE2, through exposure of seminal plasma, leads to expression of FGF2, a potent mitogenic and angiogenic factor, though activation of EP2 (Battersby et al., 2007). Exposure to PGE2 leads to an increase in phosphorylation of Akt and tuberin, a tumor suppressor that negatively regulates cellular proliferation, with a concomitant decrease in total tuberin protein expression (Sales et al., 2004a). In this study, the authors used inhibitors to block PI3K/Akt signaling and while involvement of a specific EP receptor was not identified (Sales et al., 2004a) several studies have determined that the EP4 receptor can lead to activation of PI3K pathway (Xu et al., 2018; Lu et al., 2019; Osawa et al., 2020).
Based on the studies presented in this section, there is a significant role for prostanoid signaling in uterine cancer development and progression. It would be interesting to see if other prostanoids as well as eicosanoids in LOX and P450 pathways also play a role in uterine cancer. It would also be beneficial to see additional studies combining inhibition of specific prostanoid receptors with first-line therapies.
Eicosanoids in Cervical Cancer
Cervical cancer is one of the foremost contributors to worldwide gynecological malignancies and the top contributor to that in Southern Africa (Sales and Katz, 2012; Adefuye et al., 2014). In 2008, as reported by the International Agency for Research on Cancer, an estimated 493,243 women were diagnosed with cervical cancer annually, contributing to approximately 27,300 deaths (Sales and Katz, 2012). Cervical cancer primarily arises as a neoplasm that’s initiated by oncogenic variations of human papillomavirus (HPV) and of the 180 different genotypes of the infectious disease, 40 can be accredited to cause infection of the anogenital tract (Adefuye et al., 2014). The most common mode of transmission of HPV is through the exchanging of bodily fluids (primarily seminal fluid) during sexual intercourse (Adefuye et al., 2014). Not only does seminal fluid contribute to the transmission of HPV, but the presence of prominent concentrations of prostaglandins associated with seminal plasma (including PGE2) is likely to regulate inflammatory and tumorigenic pathways due to elevated expression of PGE2 receptors in cervical cancers (Adefuye et al., 2014). Increased binding of PGE2 to EP receptors can increase COX-2 expression as well as regulate target genes such as growth factors, angiogenic factors, prostaglandins, chemokines, and cytokines, which supports tumorigenesis, inflammation, and local changes in tissue architecture (Adefuye et al., 2014). Since COX-2 is controlled by growth factors, tumor promoters, oncogenes, and carcinogens, it is speculated that COX-2 expression is increased in a variety of cancers, including cervical (Saldivar et al., 2007). By producing prostaglandins, COX-2 is responsible for suppressing apoptosis and promoting tumor invasion (Saldivar et al., 2007). Colposcopically, within the same patient, COX-2 expression levels were measured at 83.1± 30.1 ng COX-2/mg protein in normal tissues versus 403.7± 175.6 in abnormal biopsies (CIN tissues). Inflammation is associated with carcinogenesis and in line with this association, Saldivar found that COX-2 levels were 3.7 times higher in specimen that were inflammation positive and of those specimens, COX-2 protein levels were 7.4 times higher than that in control biopsies (Saldivar et al., 2007). These data provide evidence that COX-2 may play an integral role in cervical cancer inflammation and tumorigenesis.
Upregulation of COX-2 can be attributed to HPV oncoproteins playing a role in cervical carcinogenesis. Kim and colleagues investigated the role of HPV 16, the most frequent genotype detected in cervical cancer tissue, E5 oncoprotein in cervical cancer development (Kim et al., 2009b). One-way E5 affects COX-2 levels is through EGFR activation; in HaCa T cells transfected with E5, there is activation of the EGFR pathway (Kim et al., 2009b). Transactivation of EGFR by protease-activated receptors are also known to have an effect on COX-2 upregulation (Almeida et al., 2018). Inhibition of EGFR activation lead to a decrease in COX-2 expression (Kim et al., 2009b). E5 also induces COX-2 mRNA expression; assessment of transcriptional activity determined that the COX-2 gene expression was regulated through binding of NF-κB and AP-1 to the COX-2 promoter (Kim et al., 2009b). Inhibition of NF- κB or AP-1 lead to significant decrease in COX-2 expression (Kim et al., 2009b). Bcl proteins are essential for cell clearance and when defective, can promote cancer (Alibek et al., 2014).
Sales and Katz (2012) have shown that PGE2 biosynthesized by COX-1 and COX-2 in HeLa cells, increases expression of pro-angiogenic factors that can exert paracrine activity on endothelial cells to promote blood supply for tumor growth and alter vascular permeability for the release and distribution of leucocytes and macrophages to surrounding tissues. The production of prostaglandins via elevated COX enzyme activity, act on G-protein couple receptors (GPCRs) to encourage tissue remodeling for tumors, angiogenesis, apoptosis inhibition, cell proliferation, and altered vascular permeability (Adefuye et al., 2014). Peng et al. (2019) examines anti-cancer activity of medicinal plant, Conyza blinii, through the downregulation of COX-2 and decrease in PGE2 levels. C. blinii acts as a NF-κB pathway inhibitor and it inhibits downstream gene expression of COX-2, which in turn decreases PGE2 production (Peng et al., 2019). In C. blinii treated mice, PGE2 levels in both serum and tumors were significantly lower. This decrease in prostaglandin activity has promising implication in inflammation and immunomodulation (Peng et al., 2019).
The Bcl family is important in regulating apoptosis according to environmental cues. Bcl-2 homologues act as oncoproteins that are anti-apoptotic and thus, integral for cancer initiation (Alibek et al., 2014). Viruses have been known to have developed a mechanism of apoptosis prevention in host cells through targeting Bcl-2 homologues. HPV protein E6 interacts with p53 in infected cells in order to prevent p53 from blocking the anti-apoptotic Bcl-2 protein (Alibek et al., 2014). It is unknown how viral protein E5 interacts with the Bcl family directly; however, it is known that as pro-apoptotic Bak and Bax proteins are decreased, Bcl-2 expression levels are increased thus inhibition of Bak and Bax is facilitated by E5 (Alibek et al., 2014). This inhibition is performed through ubiquitin-proteasome-dependent degradation, involving EGFR activation and subsequently COX-2 upregulation (Alibek et al., 2014).
In addition to the contribution of HPV in cervical cancer, HIV also poses a role in infection and inflammation through elevation of COX-2 expression as well as elevated PGE2 levels Fitzgerald and colleagues (Fitzgerald et al., 2012). HIV infected women are five times more likely to develop cervical cancer than HIV-negative women. HIV induces high COX-2 levels in a number of immune cells circulating and in tissue (Fitzgerald et al., 2012). Fitzgerald and colleagues hypothesized that HIV promotes COX-2 levels in cervical tissue and in turn increases systemic levels of PGE2 leading to inflammation and cancer (Fitzgerald et al., 2012). Women positive for HIV and negative HPV have significantly higher levels of COX-2 mRNA (+/−) (P<0.001) with additional increase in COX-2 observed in women positive for both HIV and HPV (+/+)(P<0.001). Similarly, urinary PGE-M levels were elevated in HIV positive women; HPV positive women did not exhibit significantly higher levels of PGE-M meaning HIV status was the only statistical predictor of PGE-M levels. Through this observation, it was concluded that COX-2 and PGE-M levels were positively correlated (P= 0.005) (Fitzgerald et al., 2012). This study confirms association of HIV with development of cervical cancer. The data presented in this section demonstrates a significant role for COX-2 and PGE2 in the pathogenesis of cervical cancer alone and in conjunction with infection by HPV or HIV.
Eicosanoids in Vulvar Cancer
Vulvar cancers are rare, accounting for only an estimated 6,070 cases and 1,280 deaths in the United States (US) in 2019 (Siegel et al., 2019). The majority are squamous cell carcinomas (Moroney et al., 2013). Vulvar cancer arises from two pathways (Zarcone et al., 1997). The most common mechanism involves chronic inflammation in older women from vulvar dystrophies such as lichen sclerosus with development of differentiated vulvar intraepithelial neoplasia (VIN) then keratinizing squamous cell carcinoma. In 25–30% of cases and often in younger women, pathogenesis is driven by human papillomavirus (HPV) leading to usual VIN or high-grade squamous intraepithelial neoplasia (HSIL) and basaloid or warty squamous cell carcinoma. HPV-related vulvar cancers may exhibit a predilection for localization to the perineum and carry a favorable prognosis (Hinten et al., 2018) compared to vulvar cancers that arise independent of HPV.
COX-2 expression has clinicopathologic relevance in vulvar cancer. Lee et al. (2007) found that COX-2 expression by immunohistochemistry and adjacent inflammatory cell infiltrate was higher in older compared to younger vulvar cancer patients (P = 0.002) (Lee et al., 2007). Interestingly, COX-2 expression was inversely correlated with differentiation. Fons et al. (2007) found that strong COX-2 expression was an independent predictor of poor disease-specific survival (HR 4.01, 95% CI 1.10-14.63, P = 0.035), as was lymphovascular space invasion, lymph node involvement, tumor size > 4 cm, and absence of apoptotic caspase-3.
Inhibition of COX-2 may potentiate the effects of chemotherapies used to treat vulvar cancer. Dual treatment of COX-2-expressing vulvar squamous cell carcinoma cell lines (A431 and SW962) with the COX-2 inhibitor celecoxib and cisplatin inhibited 49% of growth, compared to 25% with cisplatin monotherapy alone after 48 h (Kim et al., 2009a). Interestingly, exposure of the cells to either agent at 10 micromol/L or in combination increased COX-2 expression, putatively through downregulation of its negative regulator PI3K. Inhibition of PI3K has been shown to block activation of mitogen-activated kinase (MAPK) independent of EGFR in A431 cells (Graness et al., 2000), suggesting a prominent role for therapies targeting PI3K in vulvar cancer and as mentioned previously signaling through PGE2 receptor EP4 can lead to activation of PI3K. In squamous cell carcinoma of the head and neck, agonists of the PGE2 receptor EP4 promote migration through PI3K activation and Ca2+ influx (Osawa et al., 2020). EP4 antagonists are currently under study in phase IB trials of rectal cancer in conjunction with pre-operative chemoradiation (Adlai Nortye Biopharma CO, Ltd). Based on molecular mechanisms, it may be hypothesized that EP4 inhibition may one day merit study in vulvar cancers and other gynecologic malignancies treated with chemoradiation.
Eicosanoids in Vaginal Cancer
Vaginal cancer represents only 1–2% of gynecologic malignancies and is under-studied due to its rarity. There were only 5,350 US cases and 1,430 deaths from this disease in 2019 (Siegel et al., 2019). Approximately 10–50% of patients with vaginal cancer or its precursor lesion [high grade vaginal intraepithelial neoplasia (VAIN)] have a history of hysterectomy or radiation for cervical cancer. HPV infection and immunosuppression are strong risk factors for development. The majority arise in the upper third of the canal and involve the posterior wall and are squamous in histology. Less common subtypes include clear cell adenocarcinoma, which is characteristically associated with in utero diethylstilbestrol (DES) exposure or malignant degeneration of endometriosis, and embryonal rhabdomyosarcoma, the most common vaginal malignancy in children (Cardenes et al., 2013).
COX-2 expression has been found to be a prominent feature in mucosal inflammation of the vagina leading to disease susceptibility and transmission (Joseph et al., 2012), as well as rectovaginal endometriotic implants (Fagotti et al., 2004). Recently, we have observed lower expression of EP4 in vaginal tissues of patients with pelvic organ prolapse and higher expression in women with radiation-induced vaginal stenosis after treatment for gynecologic malignancy compared to healthy controls (Santayana et al., 2018). There also appears to be differential expression between luminal, basal, and intermediate (smooth muscle) layers of the vagina. Additional studies are underway to better elucidate the role of EP4 in both alterations of tissue integrity as well as its relevance in gynecologic malignancy, but this preliminary data raises interest in a possible pharmacologic role for use of EP4 inhibitors to modify a number of biologic processes in vaginal cancer.
Conclusion
This manuscript aimed to provide a comprehensive review of the role of eicosanoids in gynecological malignancies. With increasing evidence, it is demonstrated that eicosanoid driven processes are associated with the progression and spread of gynecological malignancies. These bio-active lipids have direct effects on cancer cells as well as indirect effects on the tumor microenvironment. Most of the studies reported in gynecological malignancies focused on the cyclooxygenase pathway especially the role of PGE2 and PGF2α and their receptors. The least reported pathway was the P450 cytochrome epoxygenase pathway in which only ovarian cancer had associative studies involving the pathway but with a lack of mechanistic investigation; thus, this pathway is one that should be examined more in the future for all gynecological malignancies.
Given the rare nature of vaginal and vulvar cancer, there is not an abundance of research available; however, the studies performed are directed towards the cyclooxygenase pathway and exploring all of the eicosanoid pathways would be of benefit in order to find much needed treatments and information related to tumorigenesis of these malignancies. It was demonstrated that the COX pathway plays a significant role in the pathogenesis of cervical cancer alone and in conjunction with HPV and HIV; however, there was a lack of mechanistic studies in interrogating which receptors are involved in the tumorigenic signaling pathways. In uterine cancer, it would be of benefit to see more studies performed on the potential for PGE2 receptors EP1 and EP3 in uterine cancer given that to date most of the investigations have been correlative.
In ovarian cancer, the data presented for the potential role for the lipoxygenase pathway in ovarian cancer tumorigenesis and modulation of the tumor microenvironment supports the need for additional studies including antagonizing the receptors for both leukotrienes and HETEs. Given the potential anti-neoplastic effects on some of the prostaglandins such as the cyclopentenone prostaglandins as well as the association of PGD2 with good prognosis in high grade serous ovarian cancer (Alves et al., 2019) as well as the positive effects of blocking PGE2 EP4 in modulating the tumor microenvironment (Hennequart et al., 2017), additional mechanistic studies exploring modulation of COX signaling downstream of the COX enzymes as a monotherapy and in combination with additional therapeutic interventions is warranted.
Overall there is a theme that inhibition of COX pathways leads to potentiation of chemotherapeutic treatment in multiple gynecological malignancies including ovarian (Li et al., 2012a; Li et al., 2013), uterine (Hasegawa et al., 2013; Reader et al., 2019) and vulvar (Kim et al., 2009a). Additional studies are warranted to explore the role of and the mechanism behind adding inhibition of prostanoid receptors to chemotherapy and other treatment modalities in order to conserve potential anti-neoplastic eicosanoid activities for development of novel treatments for gynecologic malignancies.
Author Contributions
PS, DR, GR, and JR contributed written sections and figures. MC and AF contributed edits. All authors contributed to the article and approved the submitted version.
Funding
The work presented has been supported by Department of Obstetrics, Gynecology, and Reproductive Medicine (JR, GR, DR), the Kaleidoscope of Hope Ovarian Cancer Foundation (JR), The Foundation for Women’s Cancer Research (DR), Maryland Department of Health’s Cigarette Restitution Fund Program (AF, JR), Baltimore Veterans Affairs Administration (AF) the STAR-PREP program of the National Institute of General Medical Sciences at the University of Maryland School of Medicine (MMC).
Conflict of Interest
The authors declare that the research was conducted in the absence of any commercial or financial relationships that could be construed as a potential conflict of interest.
References
Abera, A. B., Sales, K. J., Catalano, R. D., Katz, A. A., Jabbour, H. N. (2010). EP2 receptor mediated cAMP release is augmented by PGF 2 alpha activation of the FP receptor via the calcium-calmodulin pathway. Cell. Signal. 22, 71–79. doi: 10.1016/j.cellsig.2009.09.012
Adefuye, A., Katz, A. A., Sales, K. J. (2014).The regulation of inflammatory pathways and infectious disease of the cervix by seminal fluid. Available at: https://www.hindawi.com/journals/pri/2014/748740/ (Accessed January 8, 2020).
Adlai Nortye Biopharma CO, Ltd NCT03152370: Preoperative Radiotherapy and E7046 in Rectum Cancer (PRAER 1). Available at: www.clinicaltrials.gov/ct2/show/NCT3152370 (Accessed November 23, 2018).
Ahn, J.-H., Lee, K.-T., Choi, Y. S., Choi, J.-H. (2018). Iloprost, a prostacyclin analog, inhibits the invasion of ovarian cancer cells by downregulating matrix metallopeptidase-2 (MMP-2) through the IP-dependent pathway. Prostaglandins Other Lipid Mediat. 134, 47–56. doi: 10.1016/j.prostaglandins.2017.12.002
Alexanian, A., Rufanova, V. A., Miller, B., Flasch, A., Roman, R. J., Sorokin, A. (2009). Down-regulation of 20-HETE synthesis and signaling inhibits renal Adenocarcinoma cell proliferation and tumor growth. Available at: http://ar.iiarjournals.org/content/29/10/3819 (Accessed February 25, 2020).
Alexanian, A., Miller, B., Roman, R. J., Sorokin, A. (2012). 20-HETE-producing enzymes are up-regulated in human cancers. Cancer Genomics Proteomics 9, 163–169.
Alibek, K., Irving, S., Sautbayeva, Z., Kakpenova, A., Bekmurzayeva, A., Baiken, Y., et al. (2014). Disruption of Bcl-2 and Bcl-xL by viral proteins as a possible cause of cancer. Infect. Agents Cancer 9, 44. doi: 10.1186/1750-9378-9-44
Almeida, V. H., dos, S. I., Almendra, L. R., Rondon, A. M. R., Tilli, T. M., de, A. C., et al. (2018). Positive crosstalk between EGFR and the TF-PAR2 pathway mediates resistance to cisplatin and poor survival in cervical cancer. Oncotarget 9, 30594–30609. doi: 10.18632/oncotarget.25748
Álvarez-Errico, D., Vento-Tormo, R., Sieweke, M., Ballestar, E. (2015). Epigenetic control of myeloid cell differentiation, identity and function. Nat. Rev. Immunol. 15, 7–17. doi: 10.1038/nri3777
Alves, M. R., Do Amaral, N. S., Marchi, F. A., Silva, F., II, Da Costa, A. A. B. A., Carvalho, K. C., et al. (2019). Prostaglandin D2 expression is prognostic in high−grade serous ovarian cancer. Oncol. Rep. 41, 2254–2264. doi: 10.3892/or.2019.6984
As-Sanie, S., Black, R., Giudice, L. C., Gray Valbrun, T., Gupta, J., Jones, B., et al. (2019). Assessing research gaps and unmet needs in endometriosis. Am. J. Obstetrics Gynecol. 221, 86–94. doi: 10.1016/j.ajog.2019.02.033
Banu, S. K., Lee, J., Speights, V. O., Starzinski-Powitz, A., Arosh, J. A. (2008). Cyclooxygenase-2 regulates survival, migration, and invasion of human endometriotic cells through multiple mechanisms. Endocrinology 149, 1180–1189. doi: 10.1210/en.2007-1168
Banu, S. K., Lee, J., Speights, V. O., Starzinski-Powitz, A., Arosh, J. A. (2009). Selective inhibition of prostaglandin E2 receptors EP2 and EP4 induces apoptosis of human endometriotic cells through suppression of ERK1/2, AKT, NFkappaB, and beta-catenin pathways and activation of intrinsic apoptotic mechanisms. Mol. Endocrinol. 23, 1291–1305. doi: 10.1210/me.2009-0017
Barnes, A. P., Miller, B. E., Kucera, G. L. (2007). Cyclooxygenase inhibition and hyperthermia for the potentiation of the cytotoxic response in ovarian cancer cells. Gynecol. Oncol. 104, 443–450. doi: 10.1016/j.ygyno.2006.08.008
Battersby, S., Sales, K. J., Williams, A. R., Anderson, R. A., Gardner, S., Jabbour, H. N. (2007). Seminal plasma and prostaglandin E2 up-regulate fibroblast growth factor 2 expression in endometrial adenocarcinoma cells via E-series prostanoid-2 receptor-mediated transactivation of the epidermal growth factor receptor and extracellular signal-regulated kinase pathway. Hum. Reprod. 22, 36–44. doi: 10.1093/humrep/del328
Bauknecht, T., Siegel, A., Meerpohl, H. G., Zahradnik, H. P. (1985). Formation of prostaglandins by ovarian carcinomas. Prostaglandins 29, 665–672. doi: 10.1016/0090-6980(85)90128-5
Beeghly-Fadiel, A., Wilson, A. J., Keene, S., El Ramahi, M., Xu, S., Marnett, L. J., et al. (2018). Differential cyclooxygenase expression levels and survival associations in type I and type II ovarian tumors. J. Ovarian Res. 11, 17. doi: 10.1186/s13048-018-0389-9
Black, J. D., English, D. P., Roque, D. M., Santin, A. D. (2014). Targeted therapy in uterine serous carcinoma: an aggressive variant of endometrial cancer. Womens Health (Lond) 10, 45–57. doi: 10.2217/whe.13.72
Brencicova, E., Jagger, A. L., Evans, H. G., Georgouli, M., Laios, A., Attard Montalto, S., et al. (2017). Interleukin-10 and prostaglandin E2 have complementary but distinct suppressive effects on Toll-like receptor-mediated dendritic cell activation in ovarian carcinoma. PloS One 12, e0175712. doi: 10.1371/journal.pone.0175712
Buczynski, M. W., Dumlao, D. S., Dennis, E. A. (2009). Thematic review series: proteomics. an integrated omics analysis of eicosanoid biology. J. Lipid Res. 50, 1015–1038. doi: 10.1194/jlr.R900004-JLR200
Burkholder, B., Huang, R.-Y., Burgess, R., Luo, S., Jones, V. S., Zhang, W., et al. (2014). Tumor-induced perturbations of cytokines and immune cell networks. Biochim. Biophys. Acta (BBA) - Rev. Cancer 1845, 182–201. doi: 10.1016/j.bbcan.2014.01.004
Cai, Q., Zhao, Z., Antalis, C., Yan, L., Del Priore, G., Hamed, A. H., et al. (2012). Elevated and secreted phospholipase A2 activities as new potential therapeutic targets in human epithelial ovarian cancer. FASEB J. 26, 3306–3320. doi: 10.1096/fj.12-207597
Cardenes, H. R., Schiler, J. M., Emerson, R. (2013). “Vagina,” in Principles and Practice of Gynecologic Oncology. Eds. Barakat, R., Berchuck, A., Markman, M., Randall, M. (Lippincott Williams & Wilkins), 557–597.
Carroll, M. A., Balazy, M., Margiotta, P., Falck, J. R., McGiff, J. C. (1993). Renal vasodilator activity of 5,6-epoxyeicosatrienoic acid depends upon conversion by cyclooxygenase and release of prostaglandins. (Philadelphia: Wolters Kluwer Health/Lippincott Williams & Wilkins, ©2013) J. Biol. Chem. 268, 12260–12266.
Chen, P., Guo, M., Wygle, D., Edwards, P. A., Falck, J. R., Roman, R. J., et al. (2005). Inhibitors of Cytochrome P450 4A Suppress Angiogenic Responses. Am. J. Pathol. 166, 615–624. doi: 10.1016/S0002-9440(10)62282-1
Chen, Y., Falck, J. R., Manthati, V. L., Jat, J. L., Campbell, W. B. (2011). 20-Iodo-14,15-Epoxyeicosa-8Z-enoyl-3-azidophenylsulfonamide: Photoaffinity Labeling of a 14,15-Epoxyeicosatrienoic Acid Receptor. Biochemistry 50, 3840–3848. doi: 10.1021/bi102070w
Chen, Y., Yuan, X., Xiao, Z., Jin, H., Zhang, L., Liu, Z. (2018). Discovery of novel multidrug resistance protein 4 (MRP4) inhibitors as active agents reducing resistance to anticancer drug 6-Mercaptopurine (6-MP) by structure and ligand-based virtual screening. PloS One 13. doi: 10.1371/journal.pone.0205175
Chhonker, Y. S., Bala, V., Murry, D. J. (2018). Quantification of eicosanoids and their metabolites in biological matrices: a review. Bioanalysis 10, 2027–2046. doi: 10.4155/bio-2018-0173
Ching, M. M., Fan, C., Roque, D., Rao, G., Staats, P., Fulton, A., et al. (2018). Functional analysis of PGE2 pathway members EP4 and MRP4 in ovarian cancer. [abstract]. In: Proceedings of the AACR Conference: Addressing Critical Questions in Ovarian Cancer Research and Treatment; Oct 1-4, 2017. (Pittsburgh, PA. Philadelphia (PA): AACR), Clin. Cancer Res. 2018, 24(15_Suppl:Abstract nr A22).
Choi, J.-M., Bothwell, A. L. M. (2012). The Nuclear Receptor PPARs as important regulators of T-cell functions and autoimmune diseases. Mol. Cells 33, 217–222. doi: 10.1007/s10059-012-2297-y
Colvin, E. K. (2014). Tumor-associated macrophages contribute to tumor progression in ovarian cancer. Front. Oncol. 4, 137. doi: 10.3389/fonc.2014.00137
Contursi, A., Sacco, A., Grande, R., Dovizio, M., Patrignani, P. (2017). Platelets as crucial partners for tumor metastasis: from mechanistic aspects to pharmacological targeting. Cell. Mol. Life Sci. 74, 3491–3507. doi: 10.1007/s00018-017-2536-7
Creutzberg, C. L., Fleming, G. F. (2016). “Chapter 59 - Endometrial Cancer,” in Clinical Radiation Oncology, 4th ed. Eds. Gunderson, L. L., Tepper, J. E. (Philadelphia: Elsevier), 1203–1229.e7. doi: 10.1016/B978-0-323-24098-7.00059-9
Cui, L., Zhao, Y., Pan, Y., Zheng, X., Shao, D., Jia, Y., et al. (2017). Chemotherapy induces ovarian cancer cell repopulation through the caspase 3-mediated arachidonic acid metabolic pathway. Oncol. Targets Ther. 10, 5817–5826. doi: 10.2147/OTT.S150456
Cummings, M., Massey, K. A., Mappa, G., Wilkinson, N., Hutson, R., Munot, S., et al. (2019). Integrated eicosanoid lipidomics and gene expression reveal decreased prostaglandin catabolism and increased 5-lipoxygenase expression in aggressive subtypes of endometrial cancer. J. Pathol. 247, 21–34. doi: 10.1002/path.5160
Daikoku, T., Wang, D., Tranguch, S., Morrow, J. D., Orsulic, S., DuBois, R. N., et al. (2005). Cyclooxygenase-1 is a potential target for prevention and treatment of ovarian epithelial cancer. Cancer Res. 65, 3735–3744. doi: 10.1158/0008-5472.CAN-04-3814
Daikoku, T., Tranguch, S., Trofimova, I. N., Dinulescu, D. M., Jacks, T., Nikitin, A. Y., et al. (2006). Cyclooxygenase-1 Is Overexpressed in Multiple Genetically Engineered Mouse Models of Epithelial Ovarian Cancer. Cancer Res. 66, 2527–2531. doi: 10.1158/0008-5472.CAN-05-4063
Daikoku, T., Tranguch, S., Chakrabarty, A., Wang, D., Khabele, D., Orsulic, S., et al. (2007). Extracellular Signal-Regulated Kinase Is a Target of Cyclooxygenase-1-Peroxisome Proliferator-Activated Receptor-δ Signaling in Epithelial Ovarian Cancer. Cancer Res. 67, 5285–5292. doi: 10.1158/0008-5472.CAN-07-0828
Daikoku, T., Hirota, Y., Tranguch, S., Joshi, A. R., DeMayo, F. J., Lydon, J. P., et al. (2008). Conditional loss of uterine Pten unfailingly and rapidly induces endometrial cancer in mice. Cancer Res. 68, 5619–5627. doi: 10.1158/0008-5472.CAN-08-1274
Daikoku, T., Terakawa, J., Hossain, M. M., Yoshie, M., Cappelletti, M., Yang, P., et al. (2014). Mammalian target of rapamycin complex 1 and cyclooxygenase 2 pathways cooperatively exacerbate endometrial cancer. Am. J. Pathol. 184, 2390–2402. doi: 10.1016/j.ajpath.2014.05.023
De Nola, R., Menga, A., Castegna, A., Loizzi, V., Ranieri, G., Cicinelli, E., et al. (2019). The Crowded Crosstalk between Cancer Cells and Stromal Microenvironment in Gynecological Malignancies: Biological Pathways and Therapeutic Implication. Int. J. Mol. Sci. 20. doi: 10.3390/ijms20102401
Denkert, C., Köbel, M., Pest, S., Koch, I., Berger, S., Schwabe, M., et al. (2002). Expression of Cyclooxygenase 2 Is an Independent Prognostic Factor in Human Ovarian Carcinoma. Am. J. Pathol. 160, 893–903. doi: 10.1016/S0002-9440(10)64912-7
Denkert, C., Fürstenberg, A., Daniel, P. T., Koch, I., Köbel, M., Weichert, W., et al. (2003). Induction of G0/G1 cell cycle arrest in ovarian carcinoma cells by the anti-inflammatory drug NS-398, but not by COX-2-specific RNA interference. Oncogene 22, 8653–8661. doi: 10.1038/sj.onc.1206920
Desai, A., Xu, J., Aysola, K., Qin, Y., Okoli, C., Hariprasad, R., et al. (2014). Epithelial ovarian cancer: An overview. World J. Transl. Med. 3, 1–8. doi: 10.5528/wjtm.v3.i1.1
Di Tucci, C., Capone, C., Galati, G., Iacobelli, V., Schiavi, M. C., Di Donato, V., et al. (2019). Immunotherapy in endometrial cancer: new scenarios on the horizon. J. Gynecol. Oncol. 30:e46. doi: 10.3802/jgo.2019.30.e46
Dong, H. P., Elstrand, M. B., Holth, A., Silins, I., Berner, A., Trope, C. G., et al. (2006). NK- and B-cell infiltration correlates with worse outcome in metastatic ovarian carcinoma. Am. J. Clin. Pathol. 125, 451–458. doi: 10.1309/15B66DQMFYYM78CJ
Dozier, B. L., Watanabe, K., Duffy, D. M. (2008). Two Pathways for Prostaglandin F2α (PGF2α) Synthesis by the Primate Periovulatory Follicle. Reproduction 136, 53–63. doi: 10.1530/REP-07-0514
Dursun, P., Gultekin, M., Yuce, K., Ayhan, A. (2005). Lower expression of cyclooxygenase-2: is it associated with the development of borderline ovarian tumors? Med. Hypotheses 64, 273–278. doi: 10.1016/j.mehy.2004.06.031
Fagotti, A., Ferrandina, G., Fanfani, F., Legge, F., Lauriola, L., Gessi, M., et al. (2004). Analysis of cyclooxygenase-2 (COX-2) expression in different sites of endometriosis and correlation with clinico-pathological parameters. Hum. Reprod. 19, 393–397. doi: 10.1093/humrep/deh054
Fan, C., Reader, J., Rao, G., Staats, P., Ching, M. M., Fulton, A., et al. (2018). Abstract A50: EP4 receptor antagonism in paclitaxel-resistant ovarian clear cell carcinomas. Clin. Cancer Res. 24, A50–A50. doi: 10.1158/1557-3265.OVCA17-A50
Ferrandina, G., Legge, F., Ranelletti, F. O., Zannoni, G. F., Maggiano, N., Evangelisti, A., et al. (2002). Cyclooxygenase-2 expression in endometrial carcinoma. Cancer 95, 801–807. doi: 10.1002/cncr.10736
Fitzgerald, D. W., Bezak, K., Ocheretina, O., Riviere, C., Wright, T. C., Milne, G. L., et al. (2012). The effect of HIV and HPV coinfection on Cervical COX-2 expression and systemic Prostaglandin E2 Levels. Cancer Prev. Res. 5, 34–40. doi: 10.1158/1940-6207.CAPR-11-0496
Fons, G., Burger, M. P., Ten Kate, F. J., van der Velden, J. (2007). Identification of potential prognostic markers for vulvar cancer using immunohistochemical staining of tissue microarrays. Int. J. Gynecol. Pathol. 26, 188–193. doi: 10.1097/01.pgp.0000228140.94339.db
Friedman, E. A., Ogletree, M. L., Haddad, E. V., Boutaud, O. (2015). Understanding the role of prostaglandin E2 in regulating human platelet activity in health and disease. Thromb. Res. 136, 493–503. doi: 10.1016/j.thromres.2015.05.027
Fujino, H., Regan, J. W. (2003). Prostaglandin F(2alpha) stimulation of cyclooxygenase-2 promoter activity by the FP(B) prostanoid receptor. Eur. J. Pharmacol. 465, 39–41. doi: 10.1016/s0014-2999(03)01485-7
Gabrilovich, D., II, Nagaraj, S. (2009). Myeloid-derived suppressor cells as regulators of the immune system. Nat. Rev. Immunol. 9, 162–174. doi: 10.1038/nri2506
Garcia, V., Gilani, A., Shkolnik, B., Pandey, V., Zhang, F. F., Dakarapu, R., et al. (2017). 20-HETE signals through G-Protein-Coupled Receptor GPR75 (Gq) to affect vascular function and trigger hypertension. Circ. Res. 120, 1776–1788. doi: 10.1161/CIRCRESAHA.116.310525
Garrido, M. P., Hurtado, I., Valenzuela-Valderrama, M., Salvatierra, R., Hernández, A., Vega, M., et al. (2019). NGF-enhanced vasculogenic properties of epithelial ovarian cancer cells is reduced by inhibition of the COX-2/PGE2 Signaling Axis. Cancers (Basel) 11. doi: 10.3390/cancers11121970
Göbel, C., Breitenbuecher, F., Kalkavan, H., Hähnel, P. S., Kasper, S., Hoffarth, S., et al. (2014). Functional expression cloning identifies COX-2 as a suppressor of antigen-specific cancer immunity. Cell Death Dis. 5, e1568. doi: 10.1038/cddis.2014.531
Gomes, R. N., Felipe da Costa, S., Colquhoun, A. (2018). Eicosanoids and cancer. Clinics (Sao Paulo) 73. doi: 10.6061/clinics/2018/e530s
Graness, A., Hanke, S., Boehmer, F. D., Presek, P., Liebmann, C. (2000). Protein-tyrosine-phosphatase-mediated epidermal growth factor (EGF) receptor transinactivation and EGF receptor-independent stimulation of mitogen-activated protein kinase by bradykinin in A431 cells. Biochem. J. 347, 441–447. doi: 10.1042/0264-6021:3470441
Guo, M., Roman, R. J., Fenstermacher, J. D., Brown, S. L., Falck, J. R., Arbab, A. S., et al. (2006). 9L Gliosarcoma cell proliferation and tumor growth in rats are suppressed by N-Hydroxy-N′-(4-butyl-2-methylphenol) Formamidine (HET0016), a selective inhibitor of CYP4A. J. Pharmacol. Exp. Ther. 317, 97–108. doi: 10.1124/jpet.105.097782
Guo, A. M., Arbab, A. S., Falck, J. R., Chen, P., Edwards, P. A., Roman, R. J., et al. (2007). Activation of vascular Endothelial growth factor through reactive Oxygen species mediates 20-Hydroxyeicosatetraenoic acid-induced endothelial cell Proliferation. J. Pharmacol. Exp. Ther. 321, 18–27. doi: 10.1124/jpet.106.115360
Guo, A. M., Liu, X., Al-Wahab, Z., Maddippati, K. R., Ali-Fehmi, R., Scicli, A. G., et al. (2011). Role of 12-lipoxygenase in regulation of ovarian cancer cell proliferation and survival. Cancer Chemother. Pharmacol. 68, 1273–1283. doi: 10.1007/s00280-011-1595-y
Gupta, R. A., Tejada, L. V., Tong, B. J., Das, S. K., Morrow, J. D., Dey, S. K., et al. (2003).Cyclooxygenase-1 is overexpressed and promotes angiogenic growth factor production in ovarian cancer. Available at: http://cancerres.aacrjournals.org/content/63/5/906 (Accessed May 25, 2015).
Hada, M., Edin, M. L., Hartge, P., Lih, F. B., Wentzensen, N., Zeldin, D. C., et al. (2019). Prediagnostic serum levels of fatty acid metabolites and risk of ovarian cancer in the Prostate, Lung, Colorectal, and Ovarian (PLCO) cancer screening trial. Cancer Epidemiol. Biomarkers Prev. 28, 189–197. doi: 10.1158/1055-9965.EPI-18-0392
Harizi, H., Corcuff, J.-B., Gualde, N. (2008). Arachidonic-acid-derived eicosanoids: roles in biology and immunopathology. Trends Mol. Med. 14, 461–469. doi: 10.1016/j.molmed.2008.08.005
Hasegawa, K., Ishikawa, K., Kawai, S., Torii, Y., Kawamura, K., Kato, R., et al. (2013). Overcoming paclitaxel resistance in uterine endometrial cancer using a COX-2 inhibitor. Oncol. Rep. 30, 2937–2944. doi: 10.3892/or.2013.2790
He, Y., Zhang, M., Wu, X., Sun, X., Xu, T., He, Q., et al. (2013). High MUC2 expression in ovarian cancer is inversely associated with the M1/M2 ratio of tumor-associated Macrophages and patient survival time. PloS One 8. doi: 10.1371/journal.pone.0079769
Heinonen, P. K., Metsä-Ketelä, T. (1988). Prostanoids and cyclic nucleotides in malignant and benign ovarian tumors. Med. Oncol. Tumor Pharmacother. 5, 11. doi: 10.1007/BF03003177
Hennequart, M., Pilotte, L., Cane, S., Hoffmann, D., Stroobant, V., Plaen, E. D., et al. (2017). Constitutive IDO1 expression in human tumors is driven by Cyclooxygenase-2 and mediates intrinsic immune resistance. Cancer Immunol. Res. 5, 695–709. doi: 10.1158/2326-6066.CIR-16-0400
Hinten, F., Molijn, A., Eckhardt, L., Massuger, L. F., Quint, W., Bult, P., et al. (2018). Vulvar cancer: Two pathways with different localization and prognosis. Gynecol. Oncol. 149, 310–317. doi: 10.1016/j.ygyno.2018.03.003
Holt, D. M., Ma, X., Kundu, N., Collin, P. D., Fulton, A. M. (2012). Modulation of host natural killer cell functions in breast cancer via prostaglandin E2 receptors EP2 and EP4. J. Immunother. 35, 179–188. doi: 10.1097/CJI.0b013e318247a5e9
Hoxha, M., Zappacosta, B. (2020). CYP-derived eicosanoids: implications for rheumatoid arthritis. Prostaglandins Other Lipid Mediators 146:106405. doi: 10.1016/j.prostaglandins.2019.106405
Hsu, H.-H., Lin, Y.-M., Shen, C.-Y., Shibu, M. A., Li, S.-Y., Chang, S.-H., et al. (2017). Prostaglandin E2-induced COX-2 expressions via EP2 and EP4 signaling pathways in human LoVo colon cancer cells. Int. J. Mol. Sci. 18. doi: 10.3390/ijms18061132
Jabbour, H. N., Milne, S. A., Williams, A. R., Anderson, R. A., Boddy, S. C. (2001). Expression of COX-2 and PGE synthase and synthesis of PGE(2)in endometrial adenocarcinoma: a possible autocrine/paracrine regulation of neoplastic cell function via EP2/EP4 receptors. Br. J. Cancer 85, 1023–1031. doi: 10.1054/bjoc.2001.2033
Jabbour, H. N., Sales, K. J., Boddy, S. C., Anderson, R. A., Williams, A. R. W. (2005). A positive feedback loop that regulates cyclooxygenase-2 expression and prostaglandin F2alpha synthesis via the F-series-prostanoid receptor and extracellular signal-regulated kinase 1/2 signaling pathway. Endocrinology 146, 4657–4664. doi: 10.1210/en.2005-0804
Jabbour, H. N., Sales, K. J., Smith, O. P. M., Battersby, S., Boddy, S. C. (2006). Prostaglandin receptors are mediators of vascular function in endometrial pathologies. Mol. Cell. Endocrinol. 252, 191–200. doi: 10.1016/j.mce.2006.03.025
Jana, S., Chatterjee, K., Ray, A. K., DasMahapatra, P., Swarnakar, S. (2016). Regulation of Matrix Metalloproteinase-2 Activity by COX-2-PGE2-pAKT Axis promotes Angiogenesis in Endometriosis. PloS One 11, e0163540. doi: 10.1371/journal.pone.0163540
Jayson, G. C., Kohn, E. C., Kitchener, H. C., Ledermann, J. A. (2014). Ovarian cancer. Lancet 384, 1376–1388. doi: 10.1016/S0140-6736(13)62146-7
Jones, R. D., Liao, J., Tong, X., Xu, D., Sun, L., Li, H., et al. (2019). Epoxy-Oxylipins and Soluble Epoxide Hydrolase metabolic pathway as targets for NSAID-induced Gastroenteropathy and inflammation-associated Carcinogenesis. Front. Pharmacol. 10, 731. doi: 10.3389/fphar.2019.00731
Jong, E., Winkel, P., Poelstra, K., Prakash, J. (2011). Anticancer effects of 15d-Prostaglandin-J2 in Wild-Type and Doxorubicin-resistant ovarian cancer cells: novel actions on SIRT1 and HDAC. PloS One 6, e25192. doi: 10.1371/journal.pone.0025192
Joseph, T., Zalenskaya, I. A., Yousefieh, N., Schriver, S. D., Cote, L. C., Chandra, N., et al. (2012). Induction of cyclooxygenase (COX)-2 in human vaginal epithelial cells in response to TLR ligands and TNF-α. Am. J. Reprod. Immunol. 67, 482–490. doi: 10.1111/j.1600-0897.2011.01099.x
Kayisli, U. A., Mahutte, N. G., Arici, A. (2002). Uterine chemokines in reproductive physiology and pathology. Am. J. Reprod. Immunol. 47, 213–221. doi: 10.1034/j.1600-0897.2002.01075.x
Ke, X., Dou, F., Cheng, Z., Dai, H., Zhang, W., Qu, X., et al. (2013). High expression of cyclooxygenase-2 in uterine fibroids and its correlation with cell proliferation. Eur. J. Obstetrics Gynecol. Reprod. Biol. 168, 199–203. doi: 10.1016/j.ejogrb.2013.01.006
Keightley, M. C., Brown, P., Jabbour, H. N., Sales, K. J. (2010a). F-Prostaglandin receptor regulates endothelial cell function via fibroblast growth factor-2. BMC Cell Biol. 11:8. doi: 10.1186/1471-2121-11-8
Keightley, M. C., Sales, K. J., Jabbour, H. N. (2010b). PGF2α-F-prostanoid receptor signalling via ADAMTS1 modulates epithelial cell invasion and endothelial cell function in endometrial cancer. BMC Cancer 10, 488. doi: 10.1186/1471-2407-10-488
Khunnarong, J., Tangjitgamol, S., Manusirivithaya, S., Pataradool, K., Thavaramara, T., Leelahakorn, S. (2010). Expression of Cyclooxygenase-1 and 2 in Epithelial ovarian cancer: a Clinicopathologic study. World J. Oncol. 1, 19–27. doi: 10.4021/wjon2010.02.190w
Kim, S.-H., Kim, S.-H., Song, Y.-C., Song, Y.-S. (2009a). Celecoxib potentiates the anticancer effect of cisplatin on vulvar cancer cells independently of cyclooxygenase. Ann. N. Y. Acad. Sci. 1171 (1). doi: 10.1111/j.1749-6632.2009.04888.x
Kim, S.-H., Oh, J.-M., No, J.-H., Bang, Y.-J., Juhnn, Y.-S., Song, Y.-S. (2009b). Involvement of NF-κB and AP-1 in COX-2 upregulation by human papillomavirus 16 E5 oncoprotein. Carcinogenesis 30, 753–757. doi: 10.1093/carcin/bgp066
Kino, Y., Kojima, F., Kiguchi, K., Igarashi, R., Ishizuka, B., Kawai, S. (2005). Prostaglandin E2 production in ovarian cancer cell lines is regulated by cyclooxygenase-1, not cyclooxygenase-2. Prostaglandins Leukotrienes Essent. Fatty Acids 73, 103–111. doi: 10.1016/j.plefa.2005.04.014
Kobayashi, H., Uekuri, C., Akasaka, J., Ito, F., Shigemitsu, A., Koike, N., et al. (2013). The biology of uterine sarcomas: a review and update. Mol. Clin. Oncol. 1, 599–609. doi: 10.3892/mco.2013.124
Kochel, T. J., Fulton, A. M. (2015). Multiple drug resistance-associated protein 4 (MRP4), Prostaglandin Transporter (PGT), and 15-Hydroxyprostaglandin Dehydrogenase (15-PGDH) as determinants of PGE2 levels in cancer. Prostaglandins Other Lipid Mediat 0, 99–103. doi: 10.1016/j.prostaglandins.2014.11.003
Kochel, T. J., Reader, J. C., Ma, X., Kundu, N., Fulton, A. M. (2017). Multiple drug resistance-associated protein (MRP4) exports prostaglandin E2 (PGE2) and contributes to metastasis in basal/triple negative breast cancer. Oncotarget 8, 6540–6554. doi: 10.18632/oncotarget.14145
Koike, H., Egawa, H., Ohtsuka, T., Yamaguchi, M., Ikenoue, T., Mori, N. (1992). Eicosanoids production in endometriosis. Prostaglandins Leukot. Essent. Fatty Acids 45, 313–317. doi: 10.1016/0952-3278(92)90089-2
Lau, M.-T., Wong, A. S. T., Leung, P. C. K. (2010). Gonadotropins induce tumor cell migration and invasion by increasing cyclooxygenases expression and prostaglandin E(2) production in human ovarian cancer cells. Endocrinology 151, 2985–2993. doi: 10.1210/en.2009-1318
Lee, T. S., Jeon, Y. T., Kim, J.-W., Park, N., Park, I. (2007). Increased cyclooxygenase-2 expression associated with inflammatory cellular infiltration in elderly patient with vulvar cancer. Signal Transduction Pathways, Part C: Cell Signaling in Health and Disease, 143–153. doi: 10.1196/annals.1397.018
Lee, J., Banu, S. K., Burghardt, R. C., Starzinski-Powitz, A., Arosh, J. A. (2013). Selective inhibition of prostaglandin E2 receptors EP2 and EP4 inhibits adhesion of human endometriotic epithelial and stromal cells through suppression of integrin-mediated mechanisms. Biol. Reprod. 88, 77. doi: 10.1095/biolreprod.112.100883
Lee, H. L., Jang, J. W., Lee, S. W., Yoo, S. H., Kwon, J. H., Nam, S. W., et al. (2019). Inflammatory cytokines and change of Th1/Th2 balance as prognostic indicators for hepatocellular carcinoma in patients treated with transarterial chemoembolization. Sci. Rep. 9, 1–8. doi: 10.1038/s41598-019-40078-8
Li, W., Zhang, H., Xu, R., Zhuo, G., Hu, Y., Li, J. (2008). Effects of a selective cyclooxygenase-2 inhibitor, nimesulide, on the growth of ovarian carcinoma in vivo. Med. Oncol. 25, 172–177. doi: 10.1007/s12032-007-9016-0
Li, W., Ji, Z., Zhuo, G., Xu, R., Wang, J., Jiang, H. (2010). Effects of a selective cyclooxygenase-1 inhibitor in SKOV-3 ovarian carcinoma xenograft-bearing mice. Med. Oncol. 27, 98–104. doi: 10.1007/s12032-009-9179-y
Li, W., Wang, J., Jiang, H.-R., Xu, X.-L., Zhang, J., Liu, M.-L., et al. (2011). Combined Effects of Cyclooxygenase-1 and Cyclooxygenase-2 Selective Inhibitors on Ovarian Carcinoma in Vivo. Int. J. Mol. Sci. 12, 668–681. doi: 10.3390/ijms12010668
Li, W., Liu, M.-L., Cai, J.-H., Tang, Y.-X., Zhai, L.-Y., Zhang, J. (2012a). Effect of the combination of a cyclooxygenase-1 selective inhibitor and taxol on proliferation, apoptosis and angiogenesis of ovarian cancer in vivo. Oncol. Lett. 4, 168–174. doi: 10.3892/ol.2012.688
Li, W., Xu, X.-L., Zhang, J., Cai, J.-H., Tang, Y.-X. (2012b). Effects of cyclooxygenase inhibitors on survival time in ovarian cancer xenograft-bearing mice. Oncol. Lett. 4, 1269–1273. doi: 10.3892/ol.2012.929
Li, W., Tang, Y.-X., Wan, L., Cai, J.-H., Zhang, J. (2013). Effects of combining Taxol and cyclooxygenase inhibitors on the angiogenesis and apoptosis in human ovarian cancer xenografts. Oncol. Lett. 5, 923–928. doi: 10.3892/ol.2012.1086
Liu, M., Matsumura, N., Mandai, M., Li, K., Yagi, H., Baba, T., et al. (2009). Classification using hierarchical clustering of tumor-infiltrating immune cells identifies poor prognostic ovarian cancers with high levels of COX expression. Modern Pathol. 22, 373–384. doi: 10.1038/modpathol.2008.187
Liu, Q., Tan, W., Che, J., Yuan, D., Zhang, L., Sun, Y., et al. (2018). 12-HETE facilitates cell survival by activating the integrin-linked kinase/NF-κB pathway in ovarian cancer. Cancer Manag. Res. 10, 5825–5838. doi: 10.2147/CMAR.S180334
Liu, Y., Li, H., Zhao, C., Jia, H. (2018). MicroRNA-101 inhibits angiogenesis via COX-2 in endometrial carcinoma. Mol. Cell. Biochem. 448, 61–69. doi: 10.1007/s11010-018-3313-0
Lo, C.-J., Fu, M., Lo, F. R. (1998). Prostaglandin I2Analogue, Iloprost, down regulates Mitogen-activated protein kinases of macrophages. J. Surg. Res. 76, 159–164. doi: 10.1006/jsre.1998.5312
Lu, J.-W., Wang, W.-S., Zhou, Q., Gan, X.-W., Myatt, L., Sun, K. (2019). Activation of prostaglandin EP4 receptor attenuates the induction of cyclooxygenase-2 expression by EP2 receptor activation in human amnion fibroblasts: implications for parturition. FASEB J. 33, 8148–8160. doi: 10.1096/fj.201802642R
Luo, Y., Zhou, H., Krueger, J., Kaplan, C., Lee, S.-H., Dolman, C., et al. (2006). Targeting tumor-associated macrophages as a novel strategy against breast cancer. J. Clin. Invest. 116, 2132–2141. doi: 10.1172/JCI27648
Mantovani, A., Porta, C., Rubino, L., Allavena, P., Sica, A. (2006). Tumor-associated macrophages (TAMs) as new target in anticancer therapy. Drug Discovery Today: Ther. Strategies 3, 361–366. doi: 10.1016/j.ddstr.2006.07.001
McClay, E., Winski, P., Jones, J., Jennerette, J., Gattoni-Celli, S. (1996). Δ12-Prostaglandin-J2 is cytotoxic in human malignancies and synergizes with both cisplatin and radiation. Cancer Res. 56, 3866–3869.
Milne, S. A., Perchick, G. B., Boddy, S. C., Jabbour, H. N. (2001). Expression, localization, and signaling of PGE(2) and EP2/EP4 receptors in human nonpregnant endometrium across the menstrual cycle. J. Clin. Endocrinol. Metab. 86, 4453–4459. doi: 10.1210/jcem.86.9.7856
Moreland, K. T., Procknow, J. D., Sprague, R. S., Iverson, J. L., Lonigro, A. J., Stephenson, A. H. (2007). Cyclooxygenase (COX)-1 and COX-2 participate in 5,6-epoxyeicosatrienoic acid-induced contraction of rabbit intralobar pulmonary arteries. J. Pharmacol. Exp. Ther. 321, 446–454. doi: 10.1124/jpet.106.107904
Moroney, J. W., Kunos, C., Wilkinson, E. J., Levenback, C. (2013). “Vulva,” in Principles and Practive of Gynecologic Oncology (Lippincott Williams & Wilkins).
Munkarah, A. R., Morris, R., Baumann, P., Deppe, G., Malone, J., Diamond, M. P., et al. (2002). Effects of prostaglandin E(2) on proliferation and apoptosis of epithelial ovarian cancer cells. (Wolters Kluwer Health/Lippincott Williams & Wilkins, ©2013) J. Soc Gynecol. Investig. 9, 168–173. doi: 10.1177/107155760200900309
Naumann, R. W. (2011). The role of the phosphatidylinositol 3-kinase (PI3K) pathway in the development and treatment of uterine cancer. Gynecol. Oncol. 123, 411–420. doi: 10.1016/j.ygyno.2011.08.002
Nezhat, F. R., Apostol, R., Nezhat, C., Pejovic, T. (2015). New insights in the pathophysiology of ovarian cancer and implications for screening and prevention. Am. J. Obstet. Gynecol. 213, 262–267. doi: 10.1016/j.ajog.2015.03.044
Obermajer, N., Muthuswamy, R., Lesnock, J., Edwards, R. P., Kalinski, P. (2011). Positive feedback between PGE2 and COX2 redirects the differentiation of human dendritic cells toward stable myeloid-derived suppressor cells. Blood 118, 5498–5505. doi: 10.1182/blood-2011-07-365825
Oliva, J. L., Pérez-Sala, D., Castrillo, A., Martínez, N., Cañada, F. J., Boscá, L., et al. (2003). The cyclopentenone 15-deoxy-Δ12,14-prostaglandin J2 binds to and activates H-Ras. PNAS 100, 4772–4777. doi: 10.1073/pnas.0735842100
Osawa, K., Umemura, M., Nakakaji, R., Tanaka, R., Islam, R. M., Nagasako, A., et al. (2020). Prostaglandin E2 receptor EP4 regulates cell migration through Orai1. Cancer Sci. 111, 160–174. doi: 10.1111/cas.14247
Ostrand-Rosenberg, S., Sinha, P. (2009). Myeloid-derived suppressor cells: linking inflammation and cancer. J. Immunol. 182, 4499–4506. doi: 10.4049/jimmunol.0802740
Oyeniran, C., Tanfin, Z. (2011). MAPK14 cooperates with MAPK3/1 to regulate endothelin-1-mediated prostaglandin synthase 2 induction and survival in leiomyoma but not in normal myometrial cells. Biol. Reprod. 84, 495–504. doi: 10.1095/biolreprod.110.089011
Painter, J. N., O’Mara, T. A., Morris, A. P., Cheng, T. H. T., Gorman, M., Martin, L., et al. (2018). Genetic overlap between endometriosis and endometrial cancer: evidence from cross-disease genetic correlation and GWAS meta-analyses. Cancer Med. 7, 1978–1987. doi: 10.1002/cam4.1445
Panigrahy, D., Kaipainen, A., Greene, E. R., Huang, S. (2010). Cytochrome P450-derived eicosanoids: the neglected pathway in cancer. Cancer Metastasis Rev. 29, 723–735. doi: 10.1007/s10555-010-9264-x
Panigrahy, D., Greene, E. R., Pozzi, A., Wang, D. W., Zeldin, D. C. (2011). EET signaling in cancer. Cancer Metastasis Rev. 30, 525–540. doi: 10.1007/s10555-011-9315-y
Pardoll, D. (2015). Cancer and the Immune System: Basic Concepts and Targets for Intervention. Semin. Oncol. 42, 523–538. doi: 10.1053/j.seminoncol.2015.05.003
Park, J., Park, S. Y., Kim, J.-H. (2016). Leukotriene B4 receptor-2 contributes to chemoresistance of SK-OV-3 ovarian cancer cells through activation of signal transducer and activator of transcription-3-linked cascade. Biochim. Biophys. Acta 1863, 236–243. doi: 10.1016/j.bbamcr.2015.11.011
Park, S.-K., Herrnreiter, A., Pfister, S. L., Gauthier, K. M., Falck, B. A., Falck, J. R., et al. (2018). GPR40 is a low-affinity epoxyeicosatrienoic acid receptor in vascular cells. J. Biol. Chem. 293, 10675–10691. doi: 10.1074/jbc.RA117.001297
Peng, L., Hu, C., Zhang, C., Lu, Y., Man, S., Ma, L. (2019). Anti-cancer activity of Conyza blinii saponin against cervical carcinoma through MAPK/TGF-β/Nrf2 signaling pathways. J. Ethnopharmacol. 112503. doi: 10.1016/j.jep.2019.112503
Pidgeon, G. P., Lysaght, J., Krishnamoorthy, S., Reynolds, J. V., O’Byrne, K., Nie, D., et al. (2007). Lipoxygenase metabolism: roles in tumor progression and survival. Cancer Metastasis Rev. 26, 503–524. doi: 10.1007/s10555-007-9098-3
Powell, W. S., Rokach, J. (2015). Biosynthesis, biological effects, and receptors of hydroxyeicosatetraenoic acids (HETEs) and oxoeicosatetraenoic acids (oxo-ETEs) derived from arachidonic acid. Biochim. Biophys. Acta 1851, 340–355. doi: 10.1016/j.bbalip.2014.10.008
Prahm, K. P., Karlsen, M. A., Høgdall, E., Scheller, N. M., Lundvall, L., Nedergaard, L., et al. (2015). The prognostic value of dividing epithelial ovarian cancer into type I and type II tumors based on pathologic characteristics. Gynecol. Oncol. 136, 205–211. doi: 10.1016/j.ygyno.2014.12.029
Preston, C. C., Goode, E. L., Hartmann, L. C., Kalli, K. R., Knutson, K. L. (2011). Immunity and immune suppression in human ovarian cancer. Immunotherapy 3, 539–556. doi: 10.2217/imt.11.20
Punnonen, R., Seppälä, E., Punnonen, K., Heinonen, P. K. (1986). Fatty acid composition and arachidonic acid metabolites in ascitic fluid of patients with ovarian cancer. Prostaglandins Leukot. Med. 22, 153–158. doi: 10.1016/0262-1746(86)90084-3
Qiu, X., Cheng, J.-C., Chang, H.-M., Leung, P. C. K. (2014). COX2 and PGE2 mediate EGF-induced E-cadherin-independent human ovarian cancer cell invasion. Endocrine-Related Cancer 21, 533–543. doi: 10.1530/ERC-13-0450
Rand, A. A., Barnych, B., Morisseau, C., Cajka, T., Lee, K. S. S., Panigrahy, D., et al. (2017). Cyclooxygenase-derived proangiogenic metabolites of epoxyeicosatrienoic acids. Proc. Natl. Acad. Sci. U.S.A. 114, 4370–4375. doi: 10.1073/pnas.1616893114
Rand, A., Rajamani, A., Kodani, S., Harris, T., Schlatt, L., Barnych, B., et al. (2019). Epoxyeicosatrienoic acid (EET)-stimulated angiogenesis is mediated by epoxy hydroxyeicosatrienoic acids (EHETs) formed from COX-2. J Lipid Res 60 (12), 1996–2005. doi: 10.1194/jlr.M094219
Rask, K., Zhu, Y., Wang, W., Hedin, L., Sundfeldt, K. (2006). Ovarian epithelial cancer: a role for PGE2-synthesis and signalling in malignant transformation and progression. Mol. Cancer 5, 62. doi: 10.1186/1476-4598-5-62
Reader, J., Holt, D., Fulton, A. (2011). Prostaglandin E2 EP receptors as therapeutic targets in breast cancer. Cancer Metastasis Rev. 30, 449–463. doi: 10.1007/s10555-011-9303-2
Reader, J. C., Staats, P., Goloubeva, O., Jian, N., Roque, D. M., Matheny, M. E., et al. (2016). Abstract 5169: Functional studies of the prostaglandin E2 receptor EP4 in ovarian cancer. Cancer Res. 76, 5169–5169. doi: 10.1158/1538-7445.AM2016-5169
Reader, J., Harper, A. K., Legesse, T., Staats, P. N., Goloubeva, O., Rao, G. G., et al. (2019). EP4 and Class III β-Tubulin expression in uterine smooth muscle tumors: implications for prognosis and treatment. Cancers (Basel) 11. doi: 10.3390/cancers11101590
Rees, M. C., Turnbull, A. C. (1985). Leiomyomas release prostaglandins. Prostaglandins Leukot. Med. 18, 65–68. doi: 10.1016/0262-1746(85)90051-4
Reinartz, S., Finkernagel, F., Adhikary, T., Rohnalter, V., Schumann, T., Schober, Y., et al. (2016). A transcriptome-based global map of signaling pathways in the ovarian cancer microenvironment associated with clinical outcome. Genome Biol. 17, 108. doi: 10.1186/s13059-016-0956-6
Rodríguez-Ubreva, J., Català-Moll, F., Obermajer, N., Álvarez-Errico, D., Ramirez, R. N., Company, C., et al. (2017). Prostaglandin E2 leads to the acquisition of DNMT3A-dependent tolerogenic functions in human Myeloid-derived suppressor cells. Cell Rep. 21, 154–167. doi: 10.1016/j.celrep.2017.09.018
Sabry, M., Al-Hendy, A. (2012). Medical treatment of Uterine Leiomyoma. Reprod. Sci. 19, 339–353. doi: 10.1177/1933719111432867
Saldivar, J. S., Lopez, D., Feldman, R. A., Tharappel-Jacob, R., de la Rosa, A., Terreros, D., et al. (2007). COX-2 overexpression as a biomarker of early cervical carcinogenesis: a pilot study. Gynecol. Oncol. 107, S155–S162. doi: 10.1016/j.ygyno.2007.07.023
Sales, K. J., Jabbour, H. N. (2003). Cyclooxygenase enzymes and prostaglandins in pathology of the endometrium. Reproduction 126, 559–567. doi: 10.1530/rep.0.1260559
Sales, K. J., Katz, A. A. (2012). Inflammatory pathways in cervical cancer – the UCT contribution. South Afr. Med. J. 102, 493-496–496. doi: 10.7196/SAMJ.5532
Sales, K. J., Battersby, S., Williams, A. R. W., Anderson, R. A., Jabbour, H. N. (2004a). Prostaglandin E2 mediates phosphorylation and down-regulation of the tuberous sclerosis-2 tumor suppressor (tuberin) in human endometrial adenocarcinoma cells via the Akt signaling pathway. J. Clin. Endocrinol. Metab. 89, 6112–6118. doi: 10.1210/jc.2004-0892
Sales, K. J., Maudsley, S., Jabbour, H. N. (2004b). Elevated prostaglandin EP2 receptor in endometrial adenocarcinoma cells promotes vascular endothelial growth factor expression via cyclic 3’,5’-adenosine monophosphate-mediated transactivation of the epidermal growth factor receptor and extracellular signal-regulated kinase 1/2 signaling pathways. Mol. Endocrinol. 18, 1533–1545. doi: 10.1210/me.2004-0022
Sales, K. J., List, T., Boddy, S. C., Williams, A. R. W., Anderson, R. A., Naor, Z., et al. (2005). A novel angiogenic role for prostaglandin F2alpha-FP receptor interaction in human endometrial adenocarcinomas. Cancer Res. 65, 7707–7716. doi: 10.1158/0008-5472.CAN-05-0101
Sales, K. J., Boddy, S. C., Williams, A. R. W., Anderson, R. A., Jabbour, H. N. (2007). F-prostanoid receptor regulation of fibroblast growth factor 2 signaling in endometrial adenocarcinoma cells. Endocrinology 148, 3635–3644. doi: 10.1210/en.2006-1517
Sales, K. J., Boddy, S. C., Jabbour, H. N. (2008a). F-prostanoid receptor alters adhesion, morphology and migration of endometrial adenocarcinoma cells. Oncogene 27, 2466–2477. doi: 10.1038/sj.onc.1210883
Sales, K. J., Grant, V., Jabbour, H. N. (2008b). Prostaglandin E2 and F2alpha activate the FP receptor and up-regulate cyclooxygenase-2 expression via the cyclic AMP response element. Mol. Cell. Endocrinol. 285, 51–61. doi: 10.1016/j.mce.2008.01.016
Sales, K. J., Maldonado-Pérez, D., Grant, V., Catalano, R. D., Wilson, M. R., Brown, P., et al. (2009). Prostaglandin F2α-F-prostanoid receptor regulates CXCL8 expression in endometrial adenocarcinoma cells via the calcium–calcineurin–NFAT pathway. Biochim. Biophys. Acta (BBA) - Mol. Cell Res. 1793, 1917–1928. doi: 10.1016/j.bbamcr.2009.09.018
Santayana, C., Sun, W., Yang, P., Dick-Biascoechea, M., Johnson, H. W., Fan, C., et al. (2018). EP4 Receptor Expression in Pelvic Organ Prolapse and Radiation-Induced Vaginal Stenosis: A Novel Therapeutic Target. American Urogyencologic Society 39th Annual Scientific Meeting, October 9-13, 2018. (Chicago: IL Female Pelvic Medicine & Reconstructive Surgery: 9/10 2018) 24 (5S), S138–S157. doi: 10.1097/SPV.0000000000000616
Sasaki, H., Fukushima, M. (1994). Prostaglandins in the treatment of cancer. Anticancer Drugs 5, 131–138. doi: 10.1097/00001813-199404000-00002
Sasaki, H., Takada, K., Terashima, Y., Ekimoto, H., Takahashi, K., Tsuruo, T., et al. (1991). Human ovarian cancer cell lines resistant to cisplatin, doxorubicin, and L-phenylalanine mustard are sensitive to delta 7-prostaglandin A1 and delta 12-prostaglandin J2. Gynecol. Oncol. 41, 36–40. doi: 10.1016/0090-8258(91)90251-y
Sasaki, H., Niimi, S., Akiyama, M., Tanaka, T., Hazato, A., Kurozumi, S., et al. (1999). Antitumor activity of 13,14-dihydro-15-deoxy-delta7-prostaglandin-A1-methyl ester integrated into lipid microspheres against human ovarian carcinoma cells resistant to cisplatin in vivo. Cancer Res. 59, 3919–3922.
Schulte, R. R., Linkous, A. G., Hallahan, D. E., Yazlovitskaya, E. M. (2011). Cytosolic phospholipase A2 as a molecular target for the radiosensitization of ovarian cancer. Cancer Lett. 304, 137–143. doi: 10.1016/j.canlet.2011.02.015
Seo, J.-M., Park, S., Kim, J.-H. (2012). Leukotriene B4 Receptor-2 promotes invasiveness and metastasis of ovarian cancer cells through signal transducer and activator of transcription 3 (STAT3)-dependent Up-regulation of Matrix Metalloproteinase 2. J. Biol. Chem. 287, 13840–13849. doi: 10.1074/jbc.M111.317131
Serhan, K., Gartung, A., Panigrahy, D. (2018). Drawing a link between the thromboxane A2 pathway and the role of platelets and tumor cells in ovarian cancer. Prostaglandins Other Lipid Mediat. 137, 40–45. doi: 10.1016/j.prostaglandins.2018.06.001
Siegel, R. L., Miller, K. D., Jemal, A. (2019). Cancer statistic. CA: A Cancer J. Clin. 69, 7–34. doi: 10.3322/caac.21551
Sinha, P., Clements, V. K., Fulton, A. M., Ostrand-Rosenberg, S. (2007). Prostaglandin E2 promotes tumor progression by inducing myeloid-derived suppressor cells. Cancer Res. 67, 4507–4513. doi: 10.1158/0008-5472.CAN-06-4174
Spinella, F., Rosanò, L., Di Castro, V., Natali, P. G., Bagnato, A. (2004a). Endothelin-1-induced prostaglandin E2-EP2, EP4 signaling regulates vascular endothelial growth factor production and ovarian carcinoma cell invasion. J. Biol. Chem. 279, 46700–46705. doi: 10.1074/jbc.M408584200
Spinella, F., Rosanò, L., Di Castro, V., Nicotra, M. R., Natali, P. G., Bagnato, A. (2004b). Inhibition of cyclooxygenase-1 and -2 expression by targeting the endothelin a receptor in human ovarian carcinoma cells. Clin. Cancer Res. 10, 4670–4679. doi: 10.1158/1078-0432.CCR-04-0315
Spinella, F., Rosanò, L., Elia, G., Di Castro, V., Natali, P. G., Bagnato, A. (2004c). Endothelin-1 stimulates cyclooxygenase-2 expression in ovarian cancer cells through multiple signaling pathways: evidence for involvement of transactivation of the epidermal growth factor receptor. J. Cardiovasc. Pharmacol. 44 Suppl 1, S140–S143. doi: 10.1097/01.fjc.0000166255.12229.0d
Straus, D. S., Glass, C. K. (2001). Cyclopentenone prostaglandins: new insights on biological activities and cellular targets. Med. Res. Rev. 21, 185–210. doi: 10.1002/med.1006
Sun, Y., Yao, Z., Zhao, Z., Xiao, H., Xia, M., Zhu, X., et al. (2018). Natural killer cells inhibit metastasis of ovarian carcinoma cells and show therapeutic effects in a murine model of ovarian cancer. Exp. Ther. Med. 16, 1071–1078. doi: 10.3892/etm.2018.6342
Surowiak, P., Materna, V., Denkert, C., Kaplenko, I., Spaczyński, M., Dietel, M., et al. (2006). Significance of cyclooxygenase 2 and MDR1/P-glycoprotein coexpression in ovarian cancers. Cancer Lett. 235, 272–280. doi: 10.1016/j.canlet.2005.04.035
Tae, I. H., Park, E. Y., Dey, P., Son, J. Y., Lee, S.-Y., Jung, J. H., et al. (2018). Novel SIRT1 inhibitor 15-deoxy-Δ12,14-prostaglandin J2 and its derivatives exhibit anticancer activity through apoptotic or autophagic cell death pathways in SKOV3 cells. Int. J. Oncol. 53, 2518–2530. doi: 10.3892/ijo.2018.4561
Taek, S. L., Yong, T. J., Kim, J.-W., Won, J. K., Park, N., Park, I., et al. (2007). Increased cyclooxygenase-2 expression associated with inflammatory cellular infiltration in elderly patients with vulvar cancer. Signal Transduction Pathways Part C: Cell Signaling Health Dis. 1095, 143–153. doi: 10.1196/annals.1397.018
Tamura, M., Sebastian, S., Yang, S., Gurates, B., Ferrer, K., Sasano, H., et al. (2002). Up-regulation of cyclooxygenase-2 expression and prostaglandin synthesis in endometrial stromal cells by malignant endometrial epithelial cells. A paracrine effect mediated by prostaglandin E2 and nuclear factor-kappa B. J. Biol. Chem. 277, 26208–26216. doi: 10.1074/jbc.M201347200
Tennis, M. A., Vanscoyk, M., Keith, R. L., Winn, R. A. (2010). The role of prostacyclin in lung cancer. Trans. Res. 155, 57–61. doi: 10.1016/j.trsl.2009.06.010
Tong, B. J., Tan, J., Tajeda, L., Das, S. K., Chapman, J. A., DuBois, R. N., et al. (2000). Heightened expression of Cyclooxygenase-2 and Peroxisome proliferator-activated receptor-δ in human Endometrial Adenocarcinoma. Neoplasia 2, 483–490. doi: 10.1038/sj.neo.7900119
Torre, L. A., Trabert, B., DeSantis, C. E., Miller, K. D., Samimi, G., Runowicz, C. D., et al. (2018). Ovarian cancer statistics 2018. CA: A Cancer J. Clin. 68, 284–296. doi: 10.3322/caac.21456
Uddin, S., Ahmed, M., Hussain, A., Assad, L., Al-Dayel, F., Bavi, P., et al. (2010). Cyclooxygenase-2 inhibition inhibits PI3K/AKT kinase activity in epithelial ovarian cancer. Int. J. Cancer 126, 382–394. doi: 10.1002/ijc.24757
Umamaheswaran, S., Dasari, S. K., Yang, P., Lutgendorf, S. K., Sood, A. K. (2018). Stress, inflammation, and eicosanoids: an emerging perspective. Cancer Metastasis Rev. 37, 203–211. doi: 10.1007/s10555-018-9741-1
Uotila, P. J., Erkkola, R. U., Klemi, P. J. (2002). The expression of cyclooxygenase-1 and -2 in proliferative endometrium and endometrial adenocarcinoma. Ann. Med. 34, 428–433. doi: 10.1080/078538902321012379
Wallace, A. E., Sales, K. J., Catalano, R. D., Anderson, R. A., Williams, A. R. W., Wilson, M. R., et al. (2009). Prostaglandin F2alpha-F-prostanoid receptor signaling promotes neutrophil chemotaxis via chemokine (C-X-C motif) ligand 1 in endometrial adenocarcinoma. Cancer Res. 69, 5726–5733. doi: 10.1158/0008-5472.CAN-09-0390
Wallace, A. E., Gibson, D. A., Saunders, P. T. K., Jabbour, H. N. (2010). Inflammatory events in endometrial adenocarcinoma. J. Endocrinol. 206, 141–157. doi: 10.1677/JOE-10-0072
Wang, D., DuBois, R. N. (2010). Eicosanoids and cancer. Nat. Rev. Cancer 10, 181–193. doi: 10.1038/nrc2809
Wang, W., Zhang, J., Zhang, G. (2019). Cytochrome P450 monooxygenase-mediated eicosanoid pathway: a potential mechanistic linkage between dietary fatty acid consumption and colon cancer risk. Food Sci. Hum. Wellness 8, 337–343. doi: 10.1016/j.fshw.2019.11.002
Wen, Z., Liu, H., Li, M., Li, B., Gao, W., Shao, Q., et al. (2015). Increased metabolites of 5-lipoxygenase from hypoxic ovarian cancer cells promote tumor-associated macrophage infiltration. Oncogene 34, 1241–1252. doi: 10.1038/onc.2014.85
Wilson, A. J., Fadare, O., Beeghly-Fadiel, A., Son, D.-S., Liu, Q., Zhao, S., et al. (2015). Aberrant over-expression of COX-1 intersects multiple pro-tumorigenic pathways in high-grade serous ovarian cancer. Oncotarget 6, 21353–21368. doi: 10.18632/oncotarget.3860
Wong, J. L., Obermajer, N., Odunsi, K., Edwards, R. P., Kalinski, P. (2016). Synergistic COX2 induction by IFNγ and TNFα self-limits Type-1 immunity in the human tumor microenvironment. Cancer Immunol. Res. 4, 303–311. doi: 10.1158/2326-6066.CIR-15-0157
Xu, S., Zhou, W., Ge, J., Zhang, Z. (2018). Prostaglandin E2 receptor EP4 is involved in the cell growth and invasion of prostate cancer via the cAMP−PKA/PI3K−Akt signaling pathway. Mol. Med. Rep. 17, 4702–4712. doi: 10.3892/mmr.2018.8415
Ylikorkala, O., Kauppila, A., Viinikka, L. (1983). Prostacyclin and thromboxane in ovarian cancer: effect of cytostatics and prostaglandin synthesis inhibitors. Gynecol. Oncol. 16, 340–345. doi: 10.1016/0090-8258(83)90160-9
Zarcone, R., Mainini, G., Carfora, E., Cardone, A. (1997). Current etiopathogenetic views in vulvar cancer. Panminerva Med. 39, 30–34.
Zhang, J. Y., Prakash, C., Yamashita, K., Blair, I. A. (1992). Regiospecific and enantioselective metabolism of 8,9-epoxyeicosatrienoic acid by cyclooxygenase. Biochem. Biophys. Res. Commun. 183, 138–143. doi: 10.1016/0006-291x(92)91619-2
Zhang, X., Yan, K., Deng, L., Liang, J., Liang, H., Feng, D., et al. (2019). Cyclooxygenase 2 promotes proliferation and invasion in ovarian cancer cells via the PGE2/NF-κB pathway. Cell Transplant. 28, 1S–13S. doi: 10.1177/0963689719890597
Zhao, Y., Cui, L., Pan, Y., Shao, D., Zheng, X., Zhang, F., et al. (2017). Berberine inhibits the chemotherapy-induced repopulation by suppressing the arachidonic acid metabolic pathway and phosphorylation of FAK in ovarian cancer. Cell Prolif. 50, e12393. doi: 10.1111/cpr.12393
Zhu, J., Trillsch, F., Mayr, D., Kuhn, C., Rahmeh, M., Hofmann, S., et al. (2017). Prostaglandin receptor EP3 regulates cell proliferation and migration with impact on survival of endometrial cancer patients. Oncotarget 9, 982–994. doi: 10.18632/oncotarget.23140
Keywords: gynecological cancers, eicosanoid, lipoxygenase, epoxygenase, cyclooxygenase
Citation: Smith PG, Roque D, Ching MM, Fulton A, Rao G and Reader JC (2020) The Role of Eicosanoids in Gynecological Malignancies. Front. Pharmacol. 11:1233. doi: 10.3389/fphar.2020.01233
Received: 13 April 2020; Accepted: 28 July 2020;
Published: 26 August 2020.
Edited by:
Paola Patrignani, University of Studies G. d’Annunzio Chieti and Pescara, ItalyReviewed by:
Melania Dovizio, University of Studies G. d’Annunzio Chieti and Pescara, ItalyPallavi R. Devchand, University of Calgary, Canada
Copyright © 2020 Smith, Roque, Ching, Fulton, Rao and Reader. This is an open-access article distributed under the terms of the Creative Commons Attribution License (CC BY). The use, distribution or reproduction in other forums is permitted, provided the original author(s) and the copyright owner(s) are credited and that the original publication in this journal is cited, in accordance with accepted academic practice. No use, distribution or reproduction is permitted which does not comply with these terms.
*Correspondence: Jocelyn C. Reader, anJlYWRlckBzb20udW1hcnlsYW5kLmVkdQ==