- School of Chinese Medicine, Li Ka Shing Faculty of Medicine, The University of Hong Kong, Hong Kong, Hong Kong
The dysregulation of hepatic lipid metabolism is one of the hallmarks in many liver diseases including alcoholic liver diseases (ALD) and non-alcoholic fatty liver diseases (NAFLD). Hepatic inflammation, lipoperoxidative stress as well as the imbalance between lipid availability and lipid disposal, are direct causes of liver steatosis. The application of herbal medicines with anti-oxidative stress and lipid-balancing properties has been extensively attempted as pharmaceutical intervention for liver disorders in experimental and clinical studies. Although the molecular mechanisms underlying their hepatoprotective effects warrant further exploration, increasing evidence demonstrated that many herbal medicines are involved in regulating lipid accumulation processes including hepatic lipolytic and lipogenic pathways, such as mitochondrial and peroxisomal β-oxidation, the secretion of very low density lipoprotein (VLDL), the non-esterified fatty acid (NEFA) uptake, and some vital hepatic lipogenic enzymes. Therefore, in this review, the pathways or crucial mediators participated in the dysregulation of hepatic lipid metabolism are systematically summarized, followed by the current evidences and advances in the positive impacts of herbal medicines and natural products on the lipid metabolism pathways are detailed. Furthermore, several herbal formulas, herbs or herbal derivatives, such as Erchen Dection, Danshen, resveratrol, and berberine, which have been extensively studied for their promising potential in mediating lipid metabolism, are particularly highlighted in this review.
Introduction
Generally, liver regulates lipid metabolism by three major processes: (1) uptake free fatty acids from circulation, and de novo fatty acid synthesis (FAS); (2) lipid storage, including converting fatty acids into triglyceride (TG) and other lipid droplets, which are subsequently exported to adipose tissue or stored in liver; and (3) lipid consumption, including lipolysis, β-oxidation, and the generation of lipoproteins (Reddy and Rao, 2006; Musso et al., 2009; Ponziani et al., 2015; Mato et al., 2019). These processes are presented in Figure 1. Correct control of lipid level is critical for cellular and organismal homeostasis, while interferences with the lipogenic pathways are accompanied with a variety of metabolic syndromes. The disorders of lipid metabolism, such as decreased β‐oxidation, enhanced lipolysis, and secretion of very low-density lipoprotein (VLDL), as well as altered pathways involved in the FAS, drive the accumulation of lipid droplets into the hepatocytes, eventually leading to the development of hepatic steatosis, which is a common pathological feature in various liver diseases (Reddy and Rao, 2006; Nguyen et al., 2008; Tessari et al., 2009; Perla et al., 2017).
The most prevalent liver diseases resulting from lipid metabolism disorder are alcoholic and non-alcoholic fatty liver diseases. Except difference in alcohol consumption, alcoholic and non-alcoholic fatty liver diseases show similar pathological process, which is characterized by long-term excessive fat accumulation in the liver (Younossi, 2019). They represent a wide range of liver injury, from simple fatty liver through steatosis with necrosis and inflammation to fibrosis and cirrhosis (Lomonaco et al., 2013; Heeboll et al., 2018). In particular, non-alcoholic fatty liver diseases (NAFLD), as the metabolic diseases induced by obesity and type 2 diabetes mellitus, are the second leading causes of death globally, becoming a heavy economic burden in many countries due to the high prevalence (Albhaisi and Sanyal, 2018; Al-Dayyat et al., 2018). Since inordinate lipid metabolism is intensively involved in fatty liver diseases progression, reducing lipid accumulation is a major target of development of pharmaceutical agents for various liver diseases (Ipsen et al., 2018). Simvastatin has been used as lipid-lowing drug in patients with hyperlipidemia (Aronow, 2006). However, it shows side effects, such as constipation headaches, nausea, myopathy, elevated blood sugar, and even liver damage. As a matter of fact, there is currently no satisfying therapeutic drug for fatty liver diseases (Issa et al., 2018; Moctezuma-Velazquez, 2018).
Over the past decades, due to the positive efficacy and minimal side effects, herbal medicines, and natural products have obtained increasing attention as alternative therapeutic agents for liver disorders and dyslipidemia (Xiao et al., 2013; Yao et al., 2016; Liu Q. et al., 2017). Growing evidence from preclinical studies suggests that many herbs and isolated compounds could inhibit the progression of hepatic steatosis (Dong et al., 2012; Liu Z. L. et al., 2013). A variety of mechanisms have been demonstrated to be implicated in preventing hepatic steatosis, including reducing lipogenesis, enhancing β-oxidation, increasing insulin sensitivity, suppressing oxidative stress, and inhibiting activation of inflammatory pathways (Dong et al., 2012; Yao et al., 2016). In recent studies, sterol regulatory elementbinding protein 1c (SREBP-1c), peroxisome proliferator activated receptor α (PPARα), AMP-activated protein kinase (AMPK) and sirtuin 1 (SIRT1) signaling pathways have been highlighted as crucial molecular targets of action mechanisms by which herbal medicines regulate hepatic lipid metabolism (Liu Z. L. et al., 2013). In this review, herbal medicines involved in regulating hepatic lipolytic and lipogenic pathways, such as mitochondrial and peroxisomal β-oxidation, the secretion of very low-density lipoprotein (VLDL), the non-esterified fatty acid (NEFA) uptake, and some vital hepatic lipogenic enzymes are summarized. Current clinical evidences and meta-analysis in the positive impacts of herbal medicines on the hepatic lipid metabolism pathways have also been reviewed. Furthermore, several herbal formulae, herbs or herbal derivatives, such as Erchen Dection, Danshen, resveratrol, and berberine which have been extensively studied for their promising potential in mediating lipid metabolism, are particularly highlighted in this review. This review aims to update and summarize current evidence from laboratory and clinic studies to provide alternative and complementary medical therapies with the regulatory property of hepatic lipid metabolism to current pharmaceuticals for the treatment of liver diseases.
Herbal Medicines and Natural Products Regulate on the Hepatic Lipid Metabolism Pathways
Increasing evidence indicated that many herbs, natural products, and their derived compounds could inhibit the progression of hepatic steatosis. A variety of mechanisms have been demonstrated to be implicated in preventing hepatic steatosis and modulating lipid metabolism by herbs, including anti-oxidative stress, anti-inflammation, reducing hepatocyte fatty acid uptake and trafficking, reducing hepatic de novo lipogenesis, increasing lipolysis, induction of lipophagy, enhancing fatty acid β-oxidation. In particular, SREBP-1c, PPARα, AMPK, and SIRT1 signaling pathways have been highlighted as crucial molecular targets of action mechanisms by which herbal medicines regulate hepatic lipid metabolism. In Table 1, we reviewed the effects and mechanisms of herbs and some natural products on fatty liver diseases from recent studies. In the following section, we will discuss herbs that attenuate hepatic steatosis via reducing hepatocyte fatty acid uptake and trafficking, reducing hepatic de novo lipogenesis, increasing lipolysis, induction of lipophagy, and enhancing fatty acid β-oxidation in detail.
Reducing Hepatocyte Fatty Acid Uptake and Trafficking
Nonesterified fatty acids (NEFAs) and glycerol are generated and released from adipose tissue via lipolysis (Kawano and Cohen, 2013). Then NEFAs enter into hepatocytes principally through CD36, and fatty acid transports (FATPs)(Kawano and Cohen, 2013). Several mediators have been demonstrated to play a role in regulating CD36 and FATPs, such as pregnane X receptor (PXR), which impact the hepatocyte fatty acid uptake. Increasing evidence has shown that a variety of herbs and natural compounds attenuate hepatic steatosis via modulating genes for fatty acid uptake.
Scutellarin, one of the Traditional Chinese Medicines (TCM) used for liver diseases and diabetes, was found to reduce insulin-dependent lipid accumulation and the mRNA expression of CD36 in HepG2 cells-treated with palmitic acid (Luan et al., 2019). Several other TCM and isolated compounds, babaodan, licorice extract, polyphenol-enriched fraction from Herba Erigerontis, and magnesium lithospermate B, reduced hepatic CD36 expression in mice fed with High Fat Diet (HFD) (Wu and Wang, 2012; Wang et al., 2016; Sheng et al., 2019). Dansameum reduced the expression level of CD36 in liver of apolipoprotein E-Knockout mice with NAFLD (Ahn et al., 2019). In another mice model of NAFLD induced by high-fat and high-cholesterol diet, gypenosides which are a type of TCM extracted from plants downregulated CD36 level in the liver, alleviating the progression of hepatic steatosis (Huang et al., 2019). Berberine attenuated fat accumulation in the liver partially via suppressing the expression of FATP gene in HFD-fed mice (Zhou et al., 2019).
Reducing Hepatic De Novo Lipogenesis
De novo lipogenesis in the liver is tightly controlled by metabolic hormones such as insulin, and glucose level (Wang Y. et al., 2015). In the normal physiological status, high level of glucose promotes the secretion of insulin, activates carbohydrate-responsive element-binding protein (ChREBP), and meanwhile, provides substrate to facilitate lipogenesis in the liver (Wang Y. et al., 2015). In terms of insulin, it activates sterol regulatory element-binding protein 1c (SREBP-1c) to up-regulate lipogenic enzymes, and then promotes de novo lipogenesis (Eissing et al., 2013; Chao et al., 2019). Figure 2 shows the overview of lipogenesis in hepatocytes. Herbs and isolated natural compounds have been demonstrated by animal studies and in vitro studies to alleviate hepatic steatosis by ChREBP pathway and insulin-SREBP-1c pathway, as well as other factors, such as AMPK, PPARγ, SIRT1, inflammatory cytokines, immuno-modulation, and microRNAs. We summarized medicinal herbs and isolated natural compounds from recent literatures with the effects of reducing hepatic lipogenesis in Table 2 and discussed some representative studies in detail as following.
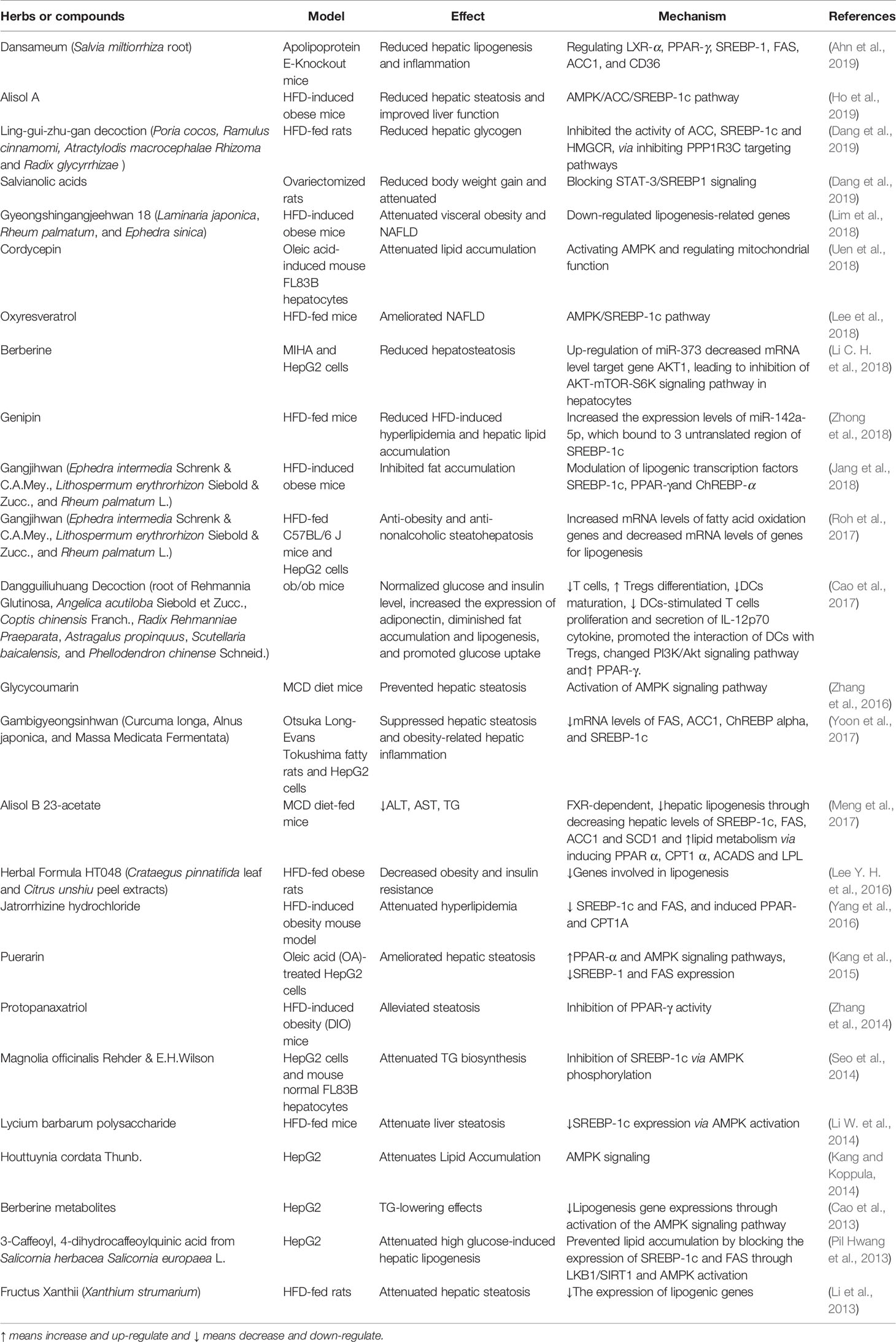
Table 2 Medicinal herbs and isolated natural compounds with the effect of hepatic lipogenesis reduction.
Magnolia officinalis Rehder & E.H.Wilson, Houttuynia cordata Thunb., 3-Caffeoyl, 4-dihydrocaffeoylquinic acid from Salicornia europaea L., puerarin and four kinds metabolites of berberine attenuated lipid accumulation in HepG2 cells in vitro via down-regulation of lipogenesis gene expressions through activation of the AMPK signaling pathway (Cao et al., 2013) (Pil Hwang et al., 2013; Kang and Koppula, 2014). Gyeongshingangjeehwan 18 (an herbal drug composed of Laminaria japonica, Rheum palmatum, and Ephedra sinica), Herbal Formula HT048 (Citrus unshiu and Crataegus pinnatifida), Fructus Xanthii (Xanthium sibiricum Patr.), Lycium barbarum polysaccharide, Jatrorrhizine hydrochloride, oxyresveratrol, and alisol A isolated from Rhizoma alismatis (Oriental Waterplantain Tuber.) attenuated liver steatosis in HFD-fed animals via regulating lipogenic genes, predominantly relating with downregulation of SREBP-1c expression via AMPK activation (Li et al., 2013; Li W. et al., 2014; Lee Y. H. et al., 2016; Yang et al., 2016; Lee et al., 2018; Lim et al., 2018; Ho et al., 2019). Gangjihwan, a polyherbal composition of Ephedra intermedia Schrenk & C.A.Mey., Lithospermum erythrorhizon Siebold & Zucc., and Rheum palmatum L., showed anti-obesity and anti-nonalcoholic steatohepatosis effects in HFD-fed mice. Lipogenic transcription factors, SREBP-1c, PPAR-γ, and ChREBP alpha were involved in the action mechanism (Jang et al., 2018); (Roh et al., 2017). Molecular targets of FAS, ACC1, ChREBP alpha, and SREBP-1c were also found to be involved in the underlying mechanism of anti-hepatic steatosis and anti-obesity-related hepatic inflammation effect of Gambigyeongsinhwan in Otsuka Long-Evans Tokushima fatty rats and HepG2 cells (Yoon et al., 2017).
Glycycoumarin, a representative of coumarin compounds isolated from licorice, and Alisol B 23-acetate exert ability of reducing hepatic lipogenesis in methionine-choline-deficient (MCD) diet-fed mice (Meng et al., 2017; Zhang E. et al., 2019). MCD diet is a classical dietary model of non-alcoholic steato-hepatitis. With the lack of methionine and choline and high sucrose (40%) and fat (10%), impaired hepatic mitochondrial β-oxidation and very low-density lipoprotein (VLDL) synthesis are observed in mice (Ibrahim et al., 2016). Glycycoumarin activated AMPK signaling pathway to reduce lipogenesis. Alisol B 23-acetate, a natural triterpenoid derived from TCM Rhizoma alismatis (Oriental Waterplantain Tuber.), decreased hepatic lipogenesis via FXR-dependent pathway. It decreased hepatic levels of SREBP-1c, FAS, ACC1 and SCD1, and promoted lipid metabolism via inducing PPAR α, CPT1 α, ACADS, and LPL (Meng et al., 2017). In an apolipoprotein E-knockout mice model, Dansameum (Salvia miltiorrhiza root), a kind of Korean polyherbal medicine, reduced hepatic lipogenesis, and inflammation via regulating PPAR-γ, SREBP-1c, FAS, ACC1, and CD36 (Ahn et al., 2019).
Dangguiliuhuang Decoction, a TCM formula composed of radix rehmanniae (root of Rehmannia Glutinosa), angelica (Angelica acutiloba Siebold et Zucc.), Coptis chinensis Franch., Radix Rehmanniae Praeparata (Rehmannia root), Astragalus propinquus (the root of astragalus membranaceus), Chinese skullcap (Scutellaria baicalensis) and Phellodendron amurense (Phellodendron chinense Schneid.), is used for the treatment of autoimmune diseases and diabetes (Cao et al., 2017; Cao et al., 2018). In a study of ob/ob mice model, it normalized glucose and insulin level, diminished fat accumulation and lipogenesis, increased the expression of adiponectin, and promoted glucose uptake (Cao et al., 2017). It showed modulation abilities on inflammation and immune response. Dangguiliuhuang Decoction (composition as listed above) promoted the shift of pro-inflammatory to anti-inflammatory cytokines. Furthermore, it decreased T cells proliferation while increased regulatory T cells (Tregs) differentiation, reduced dendritic cells (DCs) maturation and secretion of IL-12p70 cytokine, decreased DCs-stimulated T cells proliferation, and promoted, the interaction of DCs with Tregs. In adipocytes and hepatocytes as well as DCs and T cells, Dangguiliuhuang Decoction treatment altered PI3K/Akt signaling pathway and increased PPAR-γ expression, indicating the ameliorated glucose and lipid metabolism (Cao et al., 2017).
MicroRNA (miR), a small non-coding RNA molecule, has been recently demonstrated to play a role in mediating the anti-hepatic steatosis effects of natural compounds derived from herbs. Berberine reduced steatosis in MIHA and HepG2 cells by mechanism associating with up-regulation of miR-373, which decreased its mRNA level target gene AKT serine/threonine kinase 1 (AKT1), resulting in the suppression of AKT-mTOR-S6K signaling pathway in hepatocytes (Cao et al., 2018). Genipin reduced HFD-induced hyperlipidemia and hepatic lipid accumulation in mice via increasing the expression levels of miR-142a-5p, which bound to 3’-untranslated region of SREBP-1c, thus leading to the inhibition of lipogenesis (Zhong et al., 2018).
Increasing Lipolysis
Lipolysis is the catabolic process of hydrolytic cleavage of ester bonds in TG, leading to the production of fatty acids and glycerol, which could be further utilized for β-oxidation and subsequent ATP generation (Lass et al., 2011). It predominantly occurs in adipose tissues, but also in the liver, with different physiological functions. Dietary fat is digested into the gut lymphatic system as chylomicrons, which arrives at the liver through the circulation and release NEFAs through lipolysis which mediated mainly by lipoprotein lipase (LPL) (Rui, 2014). Other lipolytic enzymes contributing to hepatic TG metabolism include adiponutrin/patatin-like phospholipase domain containing 3 (PNPLA3) (Kumashiro et al., 2013), lysosomal acid lipase (LAL) (Quiroga and Lehner, 2018), arylacetamide deacetylase (Lo et al., 2010), hepatic lipase (HL)(Chatterjee and Sparks, 2011) and some members of the carboxylesterase family. In adipose tissue, inhibition of lipolysis improves glucose metabolism and insulin sensitivity, whereas in liver tissue, increasing lipolysis facilitates the attenuation of hepatic steatosis.
As far from now, limited herbs were found to show regulatory effect on hepatic lipolysis. Lavatera critica (Cornish mallow), a green leafy vegetable, attenuated hepatic lipid accumulation induced by HFD via reversing lipolysis genes acetyl-CoA carboxylase (Veeramani et al., 2017). Nitraria retusa (Forssk.) Asch. ethanolic extract modulated the lipolysis-lipogenesis balance in the liver of db/db mice (Veeramani et al., 2017). Caffeic acid upregulated the phosphorylation of AMPK and its primary downstream targeting enzyme, acetyl-CoA carboxylase, to promote the lipolysis in HepG2 cells with oleic acid administration (Liao et al., 2014). Polygonatum stenophyllum (PS) Maxim. rhizome showed efficacy on menopausal obesity by activating lipolysis-related genes including hormone-sensitive lipase (HSL) and adipose triglyceride lipase (ATGL) (Lee J. E. et al., 2016). Mulberry (Fructus Mori) water extracts promoted hepatic lipolysis and protected liver from steatosis in obesity (Peng et al., 2011). More herbs or natural compounds exerted effects on lipolysis in adipose tissues and attenuated hepatic steatosis via liver-adipose tissue crosstalk, which are not going to be discussed in detail here.
Induction of Lipophagy
In addition to lipolysis, lipid breakdown can also be accessed via lipophagy, a special kind of autophagy to degrade lipid droplets (Singh and Cuervo, 2012; Kounakis et al., 2019). It is a process that the double membrane wraps lipid droplets and sends them to lysosomes to form autolysosomes for degradation of excessive lipid droplets deposited in cells (Liu and Czaja, 2013; Ward et al., 2016). It plays a vital role in maintaining the cellular steady state. During the early stage of NAFLD, lipophagy is activated in response to acute increase in lipid availability, thus reduce lipid deposition (Czaja, 2016; Ipsen et al., 2018). However, in the condition of such as long-lasting high fat dieting, hepatic lipophagy is impaired when lipids are sustained overwhelmed (Kwanten et al., 2014; Czaja, 2016; Ipsen et al., 2018). Growing evidence raised from recent studies indicate that lipophagy is partially suppressed in patients and animal models of NAFLD and restoring lipophagy may slow the progression of hepatic steatosis. Lipophagy could be activated by various approaches, such as mTOR and AMPK-targeting agents. Glycycoumarin, a representative of coumarin compounds isolated from licorice, mitigated hepatic steatosis partially through AMPK-mediated lipophagy in a murine model of NAFLD induced by MCD diet (Zhang et al., 2016). Dioscin is a saponin extracted and isolated from Polygonatum zanlanscianense Pamp. It has been proposed as a healthcare product against hepatic fibrosis with remarkable ability to inhibit the expression of p-mTOR/mTOR level and sequentially promote autophagy (Xu et al., 2017). In another study, Bergamot polyphenol fraction prevents NAFLD via stimulation of lipophagy in cafeteria dietinduced rat model of metabolic syndrome. The increased levels of LC3 and Beclin 1, and concomitant reduction of SQSTM1/p62 proved the promoted lipophagy with the treatment of Bergamot polyphenol fraction (Parafati et al., 2015). Increasing number of herbs or natural products have been demonstrated to exert significant effects on regulating lipophagy in the liver. Current understanding of mechanisms associated with autophagy/lipophagy of herbal medicines and natural products in preventing and treating NAFLD has been well reviewed in Zhang et al. (2018), which could be referred for further reading.
In alcoholic liver diseases (ALD), upon acute consumption of alcohol, lipophagy is activated in hepatocytes, serving as a defensive mechanism against injury to steatosis (Yan et al., 2019). However, it is impaired by chronic alcohol exposure, which is likely due to the activation of mTOR signaling and decreased lysosomal biogenesis in hepatocytes (Kounakis et al., 2019; Yang L. et al., 2019). There are growing number of herbs and natural products have been found to protect liver from injury induced by alcohol by mechanism of lipophagy stimulation. Corosolic acid, a compound derived from the leaves of Langertroemia speciosa L Pers., protected the liver from alcoholic-induced liver injury partially via restoring hepatic lipophagy due to mTORC1 suppression after AMPK activation (Guo et al., 2016). Another natural compound, quercitin, which is extensively found in many fruits and herbal plants, remarkably reversed the alcohol-induced blockade of TFEB nuclear localization, via restoring lysosome function and autophagic flux in livers of ethanol-fed C57BL6 mice (Li et al., 2019). Salvianolic acid A, a phenolic carboxylic acid extracted from Salvia miltiorrhiza Bunge, reduced hepatic steatosis induced by alcohol administration in rats. The action mechanism is attributed to enhanced autophagosome-lysosome fusion after restoring lysosomal cathepsin activities (Shi et al., 2018).
As a matter of fact, the field of lipophagy in liver diseases has yet to be fully developed. Its pathological role in different stages and circumstances of various liver disorders still needs to be revealed. Nevertheless, current studies concerning lipophagy have already provided new insights on lipid metabolism and energy homeostasis in the liver. It represents a promising path forward to the therapeutic of hepatic steatosis. Pharmaceutic agents including herbs, natural products or compounds targeting lipophagy in the liver deserve to be further investigated in future basic and clinic researches.
Enhancing Fatty Acid β-Oxidation
Fatty acid could be oxidized by β-oxidation, α-oxidation, omega-oxidation, and peroxisomal oxidation, among which β-oxidation is the major type occurring in the mitochondria matrix (Wanders et al., 2015). In β-oxidation, two carbon subunits from fatty acids are removed repeatedly until the fatty acid carbon chain is fully degraded to form acetyl-CoA, which is further oxidized to carbon dioxide and H2O in the tricarboxylic acid cycle (TCA) (Canbay et al., 2007). β-oxidation plays a vital role in hepatic lipid consumption. A variety of proteins and enzymes are involved in the process of mitochondrial fatty acid β-oxidation, such as plasma membrane fatty acid binding protein (FABPpm) (Furuhashi and Hotamisligil, 2008), fatty acid transport protein (FATP) (Ouali et al., 2000), carnitine acylcarnitine translocase (CACT) (Pierre et al., 2007), carnitine palmitoyltransferases 1 and 2 (CPT1/2), etc. (Bonnefont et al., 2004; Houten and Wanders, 2010). More importantly, mitochondrial fatty acid β-oxidation is regulated by both transcriptional and posttranscriptional mechanisms. Peroxisome proliferator-activated receptors (PPARs) are activated by fatty acids, having specific roles in physiology of different tissues (Yu et al., 2003; Lamichane et al., 2018). In liver, PPARα controls many genes involved in mitochondrial fatty acid β-oxidation (Lamichane et al., 2018). In terms of posttranscriptional mechanism, the inhibition of CPT1 by malonyl-CoA is a vital regulatory step. The levels of malonyl-CoA in hepatocytes are regulated via degradation induced by malonyl-CoA decarboxylase and via production by acetyl-CoA carboxylase (ACC)(Park et al., 2002). PPARs-mediated activation persuades transcription of malonyl-CoA decarboxylase, and phosphorylated AMPK inactivated ACC (Saha and Ruderman, 2003). They stimulate mitochondrial fatty acid β-oxidation by reducing malonyl-CoA levels. Additionally, peroxisome proliferator activated receptor gamma coactivator 1-alpha (PGC-1α) has also been regarded as a factor of posttranscriptional regulation of β-oxidation (Fernandez-Marcos and Auwerx, 2011). The activation of PGC-1α is mediated by AMPK via SIRT1-mediated deacetylation (Canto and Auwerx, 2009).
Many herbs and active compounds protect liver from steatosis via regulation of fatty acid β-oxidation. Herbacetin is a dietary flavonoid with plenty of pharmacological activities. Its anti-hyperglycemic and anti-hyperlipidemic properties was associated with up-regulation of CPT to enhanced β-oxidation and hepatic lipid metabolism (Veeramani et al., 2018). Acteoside, a major compound isolated from leaves of Aloysia citriodora Palau (syn. Lippia triphylla), promoted lipolysis and fatty acid oxidation by enhancing mRNA expression level of adipose triglyceride lipase (ATGL) and CPT-1, and thus improved hepatic lipid metabolism (Zhang Y. et al., 2019). Cordycepin enhanced β-oxidation and suppressed lipid accumulation via regulating AMPK pathway and mitochondrial fusion in hepatocytes (Uen et al., 2018).
In China, the modified Longdan Xiegan Tang (mLXT, composed of Scutellaria baicalnsis Geprgi, Gardenia jasminoides, Adenophora capillaris, Akebia quinate, Plantago asiatica, Angelica sinensis, Rehmannia glutinosa, Alisma plantago-aquatica, Bupleurum gibraltaicum, and Glycyrrhiza uralensis) has been used clinically for various liver diseases such as NAFLD. It was found to activate hepatic expression of PPAR α and its target genes associated with fatty acid β-oxidation (Ren et al., 2019). Babaodan, a TCM, up-regulated the expression of CPT-1 and PPAR α in liver of HFD-fed mice with NAFLD, leading to the enhanced β-oxidation (Sheng et al., 2019). Rosa rugosa Thunb., another TCM, is used for treatment of cardiovascular diseases and diabetes, hypertension, hyperlipidemia, and inflammation. R. rugosa flavonoids, the major components in R. rugosa Thunb., were observed to up-regulate the mRNA expression of PPAR α and its downstream gene of acyl-coenzyme A oxidas X (ACOX) in a mouse model of hypertriglyceridemia (Baiyisaiti et al., 2019). Thereby, R. rugosa flavonoids could reduce TG in hepatocytes via rising β-oxidation. Gynura procumbens Merr., one of precious medicinal herbs of Asterceaes, up-regulated the mRNA expression of genes involved in β-oxidation, including PPAR α, CPT1 α, ACOX, fatty acid-binding proteins 5 (FABP5), stearoyl-coenzyme A desaturase-1 (SCD-1), glycerol-3-phosphate acyltransferase (mGPAT), microsomal triglyceride transfer protein (MTTP), to increase β-oxidation and efflux of fatty acids in liver of mice fed with MCD diet, and consequently decreased hepatic lipid accumulation (Liu Y. Y. et al., 2019). An herbal formula Gyeongshingangjeehwan 18 (GGEx18), composed of Laminaria japonica Aresch (Laminariaceae), Rheum palmatum L. (Polygonaceae) and Ephedra sinica Stapf (Ephedraceae), has traditionally been described to against obesity and related metabolic disease such as dyslipidemia. In HFD-fed mice receiving GGEx18, genes related to hepatic fatty acid β-oxidation was higher compared to mice fed with only HFD (Lim et al., 2018).
Evidence from recent studies has also indicated that some natural compounds promoted fatty acid oxidation by regulating the AMPK/PGC-1α signaling pathway. Yellow pigments, monascin, and ankaflavin, as secondary metabolites derived from monascus-fermented products, could reduce fatty acid accumulation partly mediated by the AMPK signaling activation and enhancement of β-oxidation by PGC-1α (Hsu et al., 2014). Myricetin, a natural flavonol with many biological activities, decreased PGC-1α acetylation through SIRT1 activation, and thus enhanced mitochondrial activity, suggesting its potential role in regulating hepatic lipid metabolism (Jung et al., 2017).
Clinical Trials
Given to the encouraging effects of herbal medicines on liver diseases, plenty of clinical trials have been extensively performed. The potential therapeutic benefits of herbal medicines in patients with NAFLD have been reviewed in several papers in recent years (Xiao et al., 2013; Bedi et al., 2016; Perumpail et al., 2018). In present review, we focused on the efficacy of herbal medicines to mediate lipid metabolism and attenuate hepatic steatosis.
Dava Al-Balgham, as one of the traditional medicine products composed of Nigella sativa L., Pistacia lentiscus L., Zataria multiflora Boiss. (ZM), and Trachyspermum ammi, was tested for its effect on NAFLD by a randomized, double-blinded, placebo-controlled trial with 76 NAFLD patients. Placebo or Dava Al-Balgham were consumed with each meal for three months. The results showed that Dava Al-Balgham could cause weight loss and have anti-hypolipidemic effect (Hormati et al., 2019).
The effect of Z. multiflora supplementation on NAFLD was studied by a randomized double-blind placebo-controlled clinical trial. Total 85 patients with NAFLD were treated with ZM powder (700 mg) or placebo twice daily for 3 months. However, no significant difference between two ZM-treated groups and placebo groups regarding ALT, TNF-α, grade of fatty liver in ultrasonography, lipid profiles, and high sensitive C-reactive protein (hs-CRP), while it could improve insulin resistance in patients with NAFLD. Further studies with larger sample size and longer duration are recommended (Zamani et al., 2018).
A 12-weeks randomized, controlled, double-blind trial included with was 44 NAFLD patients, was performed to evaluate the efficacy of Capparis spinosa L. on disease regression of NAFLD. Patients are randomly divided into control (n=22) or caper (n=22) group. The caper group was treated with 40-50 g caper fruit pickles with meals every day. Results obtained after treatment of 12 weeks indicated that the grade of fatty liver and serum lipoproteins were improved by C. spinosa administration (Khavasi et al., 2017).
We further checked the registered clinical trials about testing effects of the herbs and natural products on fatty liver via the website of www.clinialtrial.gov. The intensively studied herbs and derived compounds are resveratrol, ginseng, and ginger, which were discussed in detail in following. Other herbs and some natural products that are undergoing or were performed clinical trials on fatty liver diseases are listed in Table 3.
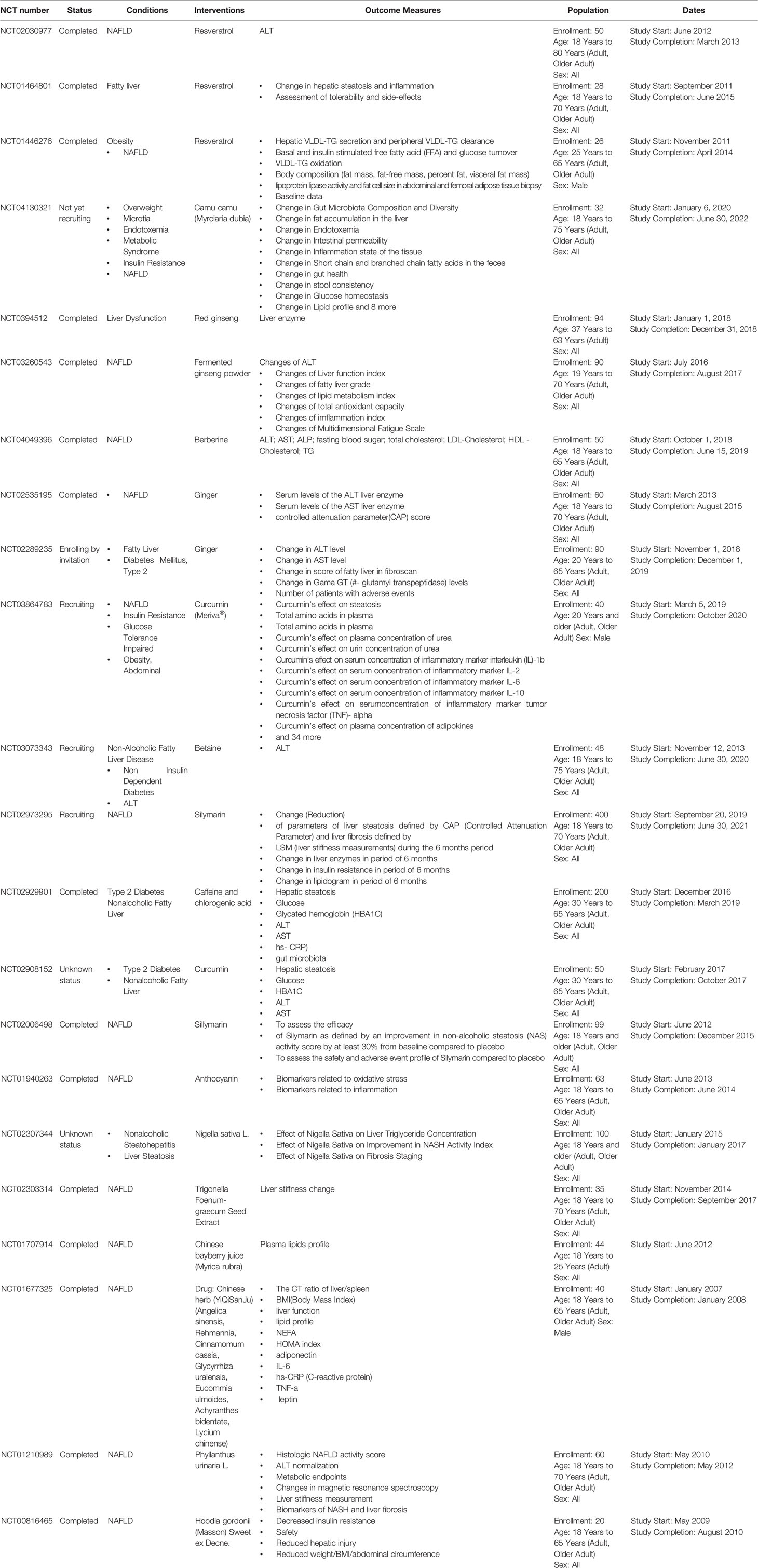
Table 3 Registered clinical trials of herbs and natural products on fatty liver diseases (Referred to http://www.ClinicalTrials.gov website).
Resveratrol is a stilbenoid and a phytoalexin generated by several plants, such as red grapes in response to stimuli (Hasan and Bae, 2017). It is an activator of AMPK and SIRT1, and thus has a critical role in promoting fat breakdown and removal from the liver, preventing liver damage and inhibiting the progression of NAFLD (Shang et al., 2008; Charytoniuk et al., 2017; Theodotou et al., 2019). Resveratrol has been involved in three trials (NCT01446276; NCT01464801; NCT02030977) included patients of fatty liver, NAFLD, and obesity.
Another herb, ginseng, has been traditionally used for more than 2,000 years with various biological effects. A great deal of preclinical studies have demonstrated the protective effects of ginseng on liver diseases, including ALD and NAFLD. Korean Red Ginseng (Panax ginseng) (Park et al., 2017) enhanced the decreased phosphorylation of AMPK induced by ethanol consumption. Notably, it reduced the accumulation of fat in hepatocytes caused by ethanol via regulation of SREBP-1, SIRT-1 and PPAR-α (Huu Tung et al., 2012; Park et al., 2017). Clinical trial (NCT0394512) has been performed to study the effect of red ginseng on liver dysfunction. Fermented ginseng powder has also been tested to study its efficacy on NAFLD (NCT03260543).
Ginger is the root of Zingiber officinale Roscoe and is one of the most used spices in many countries (Huu Tung et al., 2012). It contains active compounds, such as shogaol, gingerol, zingerone, and β-bisabolene. It has been shown that ginger can reduce insulin resistance and serum TG level in patients with Type II diabetes and hyperlipidemia (Arablou et al., 2014). In a randomized, double-blind, placebo-controlled clinical trial with 44 patients of NAFLD, ginger supplementation significantly reduced the levels of ALT, inflammatory cytokines, γ-glutamyl transferase, as well as hepatic steatosis grade and the insulin resistance index in comparison to the control group. Another clinical trial of ginger supplement on fatty liver or Type 2 Diabetes Mellitus is still undergoing (NCT02289235).
Meta-Analysis Studies
HuoXueHuaYu (HXHY), a TCM formula, has been widely used in clinic for patients with NAFLD. Cai et al. performed a meta-analysis of randomized controlled trial of HXHY in NAFLD. There are 13 studies involving 1429 patients which 654 patients receiving conventional treatment group and 775 patients belonged to HXHY group. HXHY showed better ability on lowing TC and TG levels than that of conversational treatment. HXHY might be an effective and safe therapy for NAFLD, and trials with rigorous design, multicenter, large-scale, and high-quality worldwide are still expected (Cai et al., 2019).
Erchen Decoction (ECD), a TCM formula, is often used in the therapy of various diseases. A meta-analysis of the efficacy of ECD for the treatment of NAFLD by PRISMA systematic review standard has been performed. Seven randomized controlled trial with a total of 1951 participants were included in this study. The analysis results showed that patients with ECD treatment showed an improved status compared to the conventional treatment. Longer follow-up periods and larger-scale randomized controlled trial are still required to evaluate the efficacy of ECD in NAFLD (Li et al., 2017).
The efficiency and safety of a famous TCM Danshen in the treatment of NAFLD has also been analyzed by a meta-analysis study. Eight randomized controlled trials with 800 patients of NAFLD were identified. The results indicated that Danshen had improved total effectiveness rate, lower level of TC, TG, LDL, ALT, and AST, suggesting that Danshen may have potential effects on NAFLD, while multicenter large-sample randomized clinical trials are still expected to confirm the efficacy and safety of Danshen (Peng et al., 2016).
Another study performed by Narjes et al. on 2017 has evaluated the efficiency of all kinds of TCM on the treatment of NAFLD. Literature were searched on China National Knowledge and PubMed from 1995 to 2010. Total 5904 patients from 62 randomized controlled trials were included for meta-analysis. Results showed that TCM had a better effect on the normalization of ALT level and disappearance of radiological steatosis for the patients of NAFLD. Finally, authors concluded that TCM is of modest benefit to the therapy of NAFLD (Shi et al., 2012).
Conclusions and Perspectives
Due to the positive efficacy and minimal side effects, herbal medicines have obtained increasing attention as alternative therapeutic agents for liver disorders and dyslipidemia. Increasing evidence from laboratory studies suggests that many herbs, natural products, and derived compounds could inhibit the progression of hepatic steatosis. A variety of mechanisms have been demonstrated to be implicated in preventing hepatic steatosis and modulating lipid metabolism by herbs, including reducing hepatocyte fatty acid uptake and trafficking, reducing hepatic de novo lipogenesis, increasing lipolysis, inducing lipophagy, enhancing fatty acid β-oxidation. In particular, SREBP-1c, PPARα, AMPK, and SIRT1 signaling pathways have been highlighted as crucial molecular targets of action mechanisms by which herbal medicines regulate hepatic lipid metabolism. Current clinical evidences and meta-analysis showing the positive impacts of herbal medicines on the hepatic lipid metabolism pathways are still not strong enough. Further multicenter large-sample randomized clinical trials are still required to confirm the efficacy and safety of herbal medicines on hepatic lipid metabolism. Herbs mix and single medical plants as well as their components have been widely applied in the treatment of NAFLD. We consider the main actor should be the active components. For both herbs mix and single medical plants, they are containing many compounds, which may act synergistically in ways to enhance the therapeutic effects. Identifying the active components in herbs is a crucial and significant subject for the development of TCM. Currently, network pharmacology-based strategy has been extensively used for the prediction of the active components from herbs. Network pharmacology is an approach based on systems biology, poly-pharmacology, and molecular networks, to analyze relationships between drugs and diseases in recent decade, which has attracted considerable attention among Chinese medicine researchers for its ability in predicting and illustrating interactive relationships between numerous components and targets of herbal medicines. Network-based pharmacological analysis is a desirable approach as well as a good tool of in silico prediction for investigating the mechanisms of action for herbs and formulae and their potential bioactive components at molecular and systematic levels, which renders more effective subsequent exploration with experimental approaches. With the promising and effective prediction, subsequently validation experiments in laboratory and bench would be performed to confirm their pivotal role. In conclusion, herbal medicines have the potency to be alternative and complementary medical therapies to current pharmaceuticals for the treatment of liver diseases with lipid metabolism disorder.
Author Contributions
YF designed and conceived the study. SL and YF retrieved and analyzed the data, and drafted the manuscript. SL, YX, WG, FC, CZ, HT, and NW discussed and revised the manuscript. All authors confirmed final version of the manuscript.
Funding
This research was partially supported by the Research Council of the University of Hong Kong (project codes: 104004092 and 104004460), Wong’s donation (project code: 200006276), a donation from the Gaia Family Trust of New Zealand (project code: 200007008), the Research Grants Committee (RGC) of Hong Kong, HKSAR (project codes: 740608, 766211, 17152116, and 17121419), and Health and Medical Research Fund (project codes: 15162961, 16171511, and 16172751).
Conflict of Interest
The authors declare that the research was conducted in the absence of any commercial or financial relationships that could be construed as a potential conflict of interest.
References
Ahn, S. H., Lee, K. P., Kim, K., Choi, J. Y., Park, S. Y., Cheon, J. H. (2019). Dansameum regulates hepatic lipogenesis and inflammation in vitro and in vivo. Food Sci. Biotechnol. 28 (5), 1543–1551. doi: 10.1007/s10068-019-00579-8
Albhaisi, S., Sanyal, A. (2018). Recent advances in understanding and managing non-alcoholic fatty liver disease. F1000Res 7. doi: 10.12688/f1000research.14421.1
Al-Dayyat, H. M., Rayyan, Y. M., Tayyem, R. F. (2018). Non-alcoholic fatty liver disease and associated dietary and lifestyle risk factors. Diabetes Metab. Syndr. 12 (4), 569–575. doi: 10.1016/j.dsx.2018.03.016
Ansari, A., Bose, S., Patra, J. K., Shin, N. R., Lim, D. W., Kim, K. W., et al. (2018). A Controlled Fermented Samjunghwan Herbal Formula Ameliorates Non-alcoholic Hepatosteatosis in HepG2 Cells and OLETF Rats. Front. Pharmacol. 9, 596. doi: 10.3389/fphar.2018.00596
Arablou, T., Aryaeian, N., Valizadeh, M., Sharifi, F., Hosseini, A., Djalali, M. (2014). The effect of ginger consumption on glycemic status, lipid profile and some inflammatory markers in patients with type 2 diabetes mellitus. Int. J. Food Sci. Nutr. 65 (4), 515–520. doi: 10.3109/09637486.2014.880671
Aronow, W. S. (2006). Management of hyperlipidemia with statins in the older patient. Clin. Interv. Aging 1 (4), 433–438. doi: 10.2147/ciia.2006.1.4.433
Ayuob, N. N., Abdel-Hamid, A., Helal, G. M. M., Mubarak, W. A. (2019). Thymoquinone reverses nonalcoholic fatty liver disease (NAFLD) associated with experimental hypothyroidism. Rom. J. Morphol. Embryol. 60 (2), 479–486.
Baiyisaiti, A., Wang, Y., Zhang, X., Chen, W., Qi, R. (2019). Rosa rugosa flavonoids exhibited PPARalpha agonist-like effects on genetic severe hypertriglyceridemia of mice. J. Ethnopharmacol. 240, 111952. doi: 10.1016/j.jep.2019.111952
Bedi, O., Bijjem, K. R. V., Kumar, P., Gauttam, V. (2016). Herbal Induced Hepatoprotection and Hepatotoxicity: A Critical Review. Indian J. Physiol. Pharmacol. 60 (1), 6–21.
Bonnefont, J. P., Djouadi, F., Prip-Buus, C., Gobin, S., Munnich, A., Bastin, J. (2004). Carnitine palmitoyltransferases 1 and 2: biochemical, molecular and medical aspects. Mol. Aspects Med. 25 (5-6), 495–520. doi: 10.1016/j.mam.2004.06.004
Cai, Y., Liang, Q., Chen, W., Chen, M., Chen, R., Zhang, Y., et al. (2019). Evaluation of HuoXueHuaYu therapy for nonalcoholic fatty liver disease: a systematic review and meta-analysis of randomized controlled trial. BMC Complement Altern. Med. 19 (1), 178. doi: 10.1186/s12906-019-2596-3
Canbay, A., Bechmann, L., Gerken, G. (2007). Lipid metabolism in the liver. Z. Gastroenterol. 45 (1), 35–41. doi: 10.1055/s-2006-927368
Canto, C., Auwerx, J. (2009). PGC-1alpha, SIRT1 and AMPK, an energy sensing network that controls energy expenditure. Curr. Opin. Lipidol. 20 (2), 98–105. doi: 10.1097/MOL.0b013e328328d0a4
Cao, S., Zhou, Y., Xu, P., Wang, Y., Yan, J., Bin, W., et al. (2013). Berberine metabolites exhibit triglyceride-lowering effects via activation of AMP-activated protein kinase in Hep G2 cells. J. Ethnopharmacol. 149 (2), 576–582. doi: 10.1016/j.jep.2013.07.025
Cao, H., Tuo, L., Tuo, Y., Xia, Z., Fu, R., Liu, Y., et al. (2017). Immune and Metabolic Regulation Mechanism of Dangguiliuhuang Decoction against Insulin Resistance and Hepatic Steatosis. Front. Pharmacol. 8, 445. doi: 10.3389/fphar.2017.00445
Cao, H., Li, S., Xie, R., Xu, N., Qian, Y., Chen, H., et al. (2018). Exploring the Mechanism of Dangguiliuhuang Decoction Against Hepatic Fibrosis by Network Pharmacology and Experimental Validation. Front. Pharmacol. 9, 187. doi: 10.3389/fphar.2018.00187
Chao, H. W., Chao, S. W., Lin, H., Ku, H. C., Cheng, C. F. (2019). Homeostasis of Glucose and Lipid in Non-Alcoholic Fatty Liver Disease. Int. J. Mol. Sci. 20 (2). doi: 10.3390/ijms20020298
Charytoniuk, T., Drygalski, K., Konstantynowicz-Nowicka, K., Berk, K., Chabowski, A. (2017). Alternative treatment methods attenuate the development of NAFLD: A review of resveratrol molecular mechanisms and clinical trials. Nutrition 34, 108–117. doi: 10.1016/j.nut.2016.09.001
Chatterjee, C., Sparks, D. L. (2011). Hepatic lipase, high density lipoproteins, and hypertriglyceridemia. Am. J. Pathol. 178 (4), 1429–1433. doi: 10.1016/j.ajpath.2010.12.050
Choi, J. Y., Kwon, E. Y., Choi, M. S. (2019). Elucidation of the Metabolic and Transcriptional Responses of an Oriental Herbal Medicine, Bangpungtongseong-san, to Nonalcoholic Fatty Liver Disease in Diet-Induced Obese Mice. J. Med. Food 22 (9), 928–936. doi: 10.1089/jmf.2018.4383
Cui, Y., Chang, R., Zhang, T., Zhou, X., Wang, Q., Gao, H., et al. (2019). Chinese Herbal Formula (CHF03) Attenuates Non-Alcoholic Fatty Liver Disease (NAFLD) Through Inhibiting Lipogenesis and Anti-Oxidation Mechanisms. Front. Pharmacol. 10, 1190. doi: 10.3389/fphar.2019.01190
Czaja, M. J. (2016). Function of Autophagy in Nonalcoholic Fatty Liver Disease. Dig. Dis. Sci. 61 (5), 1304–1313. doi: 10.1007/s10620-015-4025-x
Dang, Y., Hao, S., Zhou, W., Zhang, L., Ji, G. (2019). The traditional Chinese formulae Ling-gui-zhu-gan decoction alleviated non-alcoholic fatty liver disease via inhibiting PPP1R3C mediated molecules. BMC Complement Altern. Med. 19 (1), 8. doi: 10.1186/s12906-018-2424-1
Dong, H., Lu, F. E., Zhao, L. (2012). Chinese herbal medicine in the treatment of nonalcoholic fatty liver disease. Chin. J. Integr. Med. 18 (2), 152–160. doi: 10.1007/s11655-012-0993-2
Eissing, L., Scherer, T., Todter, K., Knippschild, U., Greve, J. W., Buurman, W. A., et al. (2013). De novo lipogenesis in human fat and liver is linked to ChREBP-beta and metabolic health. Nat. Commun. 4, 1528. doi: 10.1038/ncomms2537
Feng, Q., Liu, W., Baker, S. S., Li, H., Chen, C., Liu, Q., et al. (2017). Multi-targeting therapeutic mechanisms of the Chinese herbal medicine QHD in the treatment of non-alcoholic fatty liver disease. Oncotarget 8 (17), 27820–27838. doi: 10.18632/oncotarget.15482
Feng, W. W., Kuang, S. Y., Tu, C., Ma, Z. J., Pang, J. Y., Wang, Y. H., et al. (2018). Natural products berberine and curcumin exhibited better ameliorative effects on rats with non-alcohol fatty liver disease than lovastatin. BioMed. Pharmacother. 99, 325–333. doi: 10.1016/j.biopha.2018.01.071
Fernandez-Marcos, P. J., Auwerx, J. (2011). Regulation of PGC-1alpha, a nodal regulator of mitochondrial biogenesis. Am. J. Clin. Nutr. 93 (4), 884S–8890.
Furuhashi, M., Hotamisligil, G. S. (2008). Fatty acid-binding proteins: role in metabolic diseases and potential as drug targets. Nat. Rev. Drug Discovery 7 (6), 489–503. doi: 10.1038/nrd2589
Guo, X., Cui, R., Zhao, J., Mo, R., Peng, L., Yan, M. (2016). Corosolic acid protects hepatocytes against ethanol-induced damage by modulating mitogen-activated protein kinases and activating autophagy. Eur. J. Pharmacol. 791, 578–588. doi: 10.1016/j.ejphar.2016.09.031
Guo, Y., Li, J. X., Mao, T. Y., Zhao, W. H., Liu, L. J., Wang, Y. L. (2017). Targeting Sirt1 in a rat model of high-fat diet-induced non-alcoholic fatty liver disease: Comparison of Gegen Qinlian decoction and resveratrol. Exp. Ther. Med. 14 (5), 4279–4287. doi: 10.3892/etm.2017.5076
Hasan, M., Bae, H. (2017). “An Overview of Stress-Induced Resveratrol Synthesis in Grapes: Perspectives for Resveratrol-Enriched Grape Products. Molecules 22 (2). doi: 10.3390/molecules22020294
Heeboll, S., Vilstrup, H., Gronbaek, H. (2018). “[Treatment of non-alcoholic fatty liver disease]. Ugeskr Laeger 180 (31).
Ho, C., Gao, Y., Zheng, D., Liu, Y., Shan, S., Fang, B., et al. (2019). Alisol A attenuates high-fat-diet-induced obesity and metabolic disorders via the AMPK/ACC/SREBP-1c pathway. J. Cell Mol. Med. 23 (8), 5108–5118. doi: 10.1111/jcmm.14380
Hormati, A., Tooiserkany, F., Mohammadbeigi, A., Aliasl, F., Dehnavi, H. M. (2019). Effect of an Herbal Product on the Serum Level of Liver Enzymes in Patients with Non-Alcoholic Fatty Liver Disease: A Randomized, Double-Blinded, Placebo-Controlled Trial. Iranian Red Crescent Med. J. 21 (7), 7. doi: 10.5812/ircmj.91024
Houten, S. M., Wanders, R. J. (2010). A general introduction to the biochemistry of mitochondrial fatty acid beta-oxidation. J. Inherit. Metab. Dis. 33 (5), 469–477. doi: 10.1007/s10545-010-9061-2
Hsu, W. H., Chen, T. H., Lee, B. H., Hsu, Y. W., Pan, T. M. (2014). Monascin and ankaflavin act as natural AMPK activators with PPARalpha agonist activity to down-regulate nonalcoholic steatohepatitis in high-fat diet-fed C57BL/6 mice. Food Chem. Toxicol. 64, 94–103. doi: 10.1016/j.fct.2013.11.015
Huang, L., Cheng, Y., Huang, K., Zhou, Y., Ma, Y., Zhang, M. (2018). Ameliorative effect of Sedum sarmentosum Bunge extract on Tilapia fatty liver via the PPAR and P53 signaling pathway. Sci. Rep. 8 (1), 8456.
Huang, X., Chen, W., Yan, C., Yang, R., Chen, Q., Xu, H., et al. (2019). Gypenosides improve the intestinal microbiota of non-alcoholic fatty liver in mice and alleviate its progression. BioMed. Pharmacother. 118, 109258. doi: 10.1016/j.biopha.2019.109258
Huu Tung, N., Uto, T., Morinaga, O., Kim, Y. H., Shoyama, Y. (2012). Pharmacological effects of ginseng on liver functions and diseases: a minireview. Evid. Based Complement Alternat. Med. 2012, 173297.
Ibrahim, S. H., Hirsova, P., Malhi, H., Gores, G. J. (2016). Animal Models of Nonalcoholic Steatohepatitis: Eat, Delete, and Inflame. Dig. Dis. Sci. 61 (5), 1325–1336. doi: 10.1007/s10620-015-3977-1
Ipsen, D. H., Lykkesfeldt, J., Tveden-Nyborg, P. (2018). Molecular mechanisms of hepatic lipid accumulation in non-alcoholic fatty liver disease. Cell Mol. Life Sci. 75 (18), 3313–3327. doi: 10.1007/s00018-018-2860-6
Issa, D., Patel, V., Sanyal, A. J. (2018). Future therapy for non-alcoholic fatty liver disease. Liver Int. 38 Suppl 1, 56–63. doi: 10.1111/liv.13676
Jang, J., Jung, Y., Chae, S., Cho, S. H., Yoon, M., Yang, H., et al. (2018). Gangjihwan, a polyherbal composition, inhibits fat accumulation through the modulation of lipogenic transcription factors SREBP1C, PPARgamma and C/EBPalpha. J. Ethnopharmacol. 210, 10–22. doi: 10.1016/j.jep.2017.08.024
Jung, H. Y., Lee, D., Ryu, H. G., Choi, B. H., Go, Y., Lee, N., et al. (2017). Myricetin improves endurance capacity and mitochondrial density by activating SIRT1 and PGC-1alpha. Sci. Rep. 7 (1), 6237.
Kang, H., Koppula, S. (2014). Houttuynia cordata attenuates lipid accumulation via activation of AMP-activated protein kinase signaling pathway in HepG2 cells. Am. J. Chin. Med. 42 (3), 651–664. doi: 10.1142/S0192415X14500426
Kang, O. H., Kim, S. B., Mun, S. H., Seo, Y. S., Hwang, H. C., Lee, Y. M., et al. (2015). Puerarin ameliorates hepatic steatosis by activating the PPARalpha and AMPK signaling pathways in hepatocytes. Int. J. Mol. Med. 35 (3), 803–809. doi: 10.3892/ijmm.2015.2074
Kawano, Y., Cohen, D. E. (2013). Mechanisms of hepatic triglyceride accumulation in non-alcoholic fatty liver disease. J. Gastroenterol. 48 (4), 434–441. doi: 10.1007/s00535-013-0758-5
Khalil, M., Khalifeh, H., Baldini, F., Salis, A., Damonte, G., Daher, A., et al. (2019). Antisteatotic and antioxidant activities of Thymbra spicata L. extracts in hepatic and endothelial cells as in vitro models of non-alcoholic fatty liver disease. J. Ethnopharmacol. 239, 111919.
Khavasi, N., Somi, M. H., Khadem, E., Faramarzi, E., Ayati, M. H., Fazljou, S. M. B., et al. (2017). Effect of Daily Caper Fruit Pickle Consumption on Disease Regression in Patients with Non-Alcoholic Fatty Liver Disease: a Double-Blinded Randomized Clinical Trial. Adv. Pharm. Bull. 7 (4), 645–650. doi: 10.15171/apb.2017.077
Kounakis, K., Chaniotakis, M., Markaki, M., Tavernarakis, N. (2019). Emerging Roles of Lipophagy in Health and Disease. Front. Cell Dev. Biol. 7, 185. doi: 10.3389/fcell.2019.00185
Kumashiro, N., Yoshimura, T., Cantley, J. L., Majumdar, S. K., Guebre-Egziabher, F., Kursawe, R., et al. (2013). Role of patatin-like phospholipase domain-containing 3 on lipid-induced hepatic steatosis and insulin resistance in rats. Hepatology 57 (5), 1763–1772. doi: 10.1002/hep.26170
Kwanten, W. J., Martinet, W., Michielsen, P. P., Francque, S. M. (2014). Role of autophagy in the pathophysiology of nonalcoholic fatty liver disease: a controversial issue. World J. Gastroenterol. 20 (23), 7325–7338. doi: 10.3748/wjg.v20.i23.7325
Lamichane, S., Dahal Lamichane, B., Kwon, S. M. (2018). Pivotal Roles of Peroxisome Proliferator-Activated Receptors (PPARs) and Their Signal Cascade for Cellular and Whole-Body Energy Homeostasis. Int. J. Mol. Sci. 19 (4). doi: 10.3390/ijms19040949
Lass, A., Zimmermann, R., Oberer, M., Zechner, R. (2011). Lipolysis - a highly regulated multi-enzyme complex mediates the catabolism of cellular fat stores. Prog. Lipid Res. 50 (1), 14–27. doi: 10.1016/j.plipres.2010.10.004
Lee, J. E., Kim, E. J., Kim, M. H., Hong, J., Yang, W. M. (2016). Polygonatum stenophyllum improves menopausal obesity via regulation of lipolysis-related enzymes. J. Nat. Med. 70 (4), 789–796. doi: 10.1007/s11418-016-1018-9
Lee, Y. H., Jin, B., Lee, S. H., Song, M., Bae, H., Min, B. J., et al. (2016). Herbal Formula HT048 Attenuates Diet-Induced Obesity by Improving Hepatic Lipid Metabolism and Insulin Resistance in Obese Rats”. Molecules 21 (11). doi: 10.3390/molecules21111424
Lee, J. H., Baek, S. Y., Jang, E. J., Ku, S. K., Kim, K. M., Ki, S. H., et al. (2018). Oxyresveratrol ameliorates nonalcoholic fatty liver disease by regulating hepatic lipogenesis and fatty acid oxidation through liver kinase B1 and AMP-activated protein kinase. Chem. Biol. Interact. 289, 68–74. doi: 10.1016/j.cbi.2018.04.023
Li, X., Li, Z., Xue, M., Ou, Z., Liu, M., Yang, M., et al. (2013). Fructus Xanthii attenuates hepatic steatosis in rats fed on high-fat diet. PloS One 8 (4), e61499. doi: 10.1371/journal.pone.0061499
Li, W., Li, Y., Wang, Q., Yang, Y. (2014). Crude extracts from Lycium barbarum suppress SREBP-1c expression and prevent diet-induced fatty liver through AMPK activation. BioMed. Res. Int. 2014, 196198.
Li, Y., Zhao, J., Zheng, H., Zhong, X., Zhou, J., Hong, Z. (2014). Treatment of Nonalcoholic Fatty Liver Disease with Total Alkaloids in Rubus aleaefolius Poir through Regulation of Fat Metabolism. Evid. Based Complement Alternat. Med. 2014, 768540. doi: 10.1155/2014/768540
Li, Z., Xu, J., Zheng, P., Xing, L., Shen, H., Yang, L., et al. (2015). Hawthorn leaf flavonoids alleviate nonalcoholic fatty liver disease by enhancing the adiponectin/AMPK pathway. Int. J. Clin. Exp. Med. 8 (10), 17295–17307.
Li, W. S., Wu, Y., Ge, W. Z., Fan, L., Sun, W. (2017). A herbal formula Erchen decoction for non-alcoholic fatty liver disease: a systematic review and meta-analysis of randomized controlled trials. Int. J. Clin. Exp. Med. 10 (6), 9110–9116.
Li, C. H., Tang, S. C., Wong, C. H., Wang, Y., Jiang, J. D., Chen, Y. (2018). Berberine induces miR-373 expression in hepatocytes to inactivate hepatic steatosis associated AKT-S6 kinase pathway. Eur. J. Pharmacol. 825, 107–118. doi: 10.1016/j.ejphar.2018.02.035
Li, Y. Y., Tang, D., Du, Y. L., Cao, C. Y., Nie, Y. Q., Cao, J., et al. (2018). Fatty liver mediated by peroxisome proliferator-activated receptor-alpha DNA methylation can be reversed by a methylation inhibitor and curcumin. J. Dig. Dis. 19 (7), 421–430. doi: 10.1111/1751-2980.12610
Li, Y. Y., Chen, M., Wang, J., Guo, X. P., Xiao, L., Liu, P. Y., et al. (2019). Quercetin ameliorates autophagy in alcohol liver disease associated with lysosome through mTOR-TFEB pathway. J. Funct. Foods 52, 177–185. doi: 10.1016/j.jff.2018.10.033
Liang, Z. E., Zhang, Y. P., Tang, K. R., Deng, Y. J., Liang, Y. Q., Liang, S., et al. (2019). Anti-inflammation effect via TLR4-mediated MyD88-dependent and -independent signalling pathways in non-alcoholic fatty liver disease rats: Chinese herb formula. Int. J. Clin. Exp. Med. 12 (3), 2265–2277.
Liao, C. C., Ou, T. T., Huang, H. P., Wang, C. J. (2014). The inhibition of oleic acid induced hepatic lipogenesis and the promotion of lipolysis by caffeic acid via up-regulation of AMP-activated kinase. J. Sci. Food Agric. 94 (6), 1154–1162. doi: 10.1002/jsfa.6386
Lim, J., Lee, H., Ahn, J., Kim, J., Jang, J., Park, Y., et al. (2018). The polyherbal drug GGEx18 from Laminaria japonica, Rheum palmatum, and Ephedra sinica inhibits hepatic steatosis and fibroinflammtion in high-fat diet-induced obese mice. J. Ethnopharmacol. 225, 31–41. doi: 10.1016/j.jep.2018.06.034
Liu, K., Czaja, M. J. (2013). Regulation of lipid stores and metabolism by lipophagy. Cell Death Differ. 20 (1), 3–11. doi: 10.1038/cdd.2012.63
Liu, L., Yang, M., Lin, X., Li, Y., Liu, C., Yang, Y., et al. (2013). Modulation of hepatic sterol regulatory element-binding protein-1c-mediated gene expression contributes to Salacia oblonga root-elicited improvement of fructose-induced fatty liver in rats. J. Ethnopharmacol. 150 (3), 1045–1052. doi: 10.1016/j.jep.2013.10.020
Liu, Z. L., Xie, L. Z., Zhu, J., Li, G. Q., Grant, S. J., Liu, J. P. (2013). Herbal medicines for fatty liver diseases. Cochrane Database Syst. Rev. (8), CD009059. doi: 10.1002/14651858.CD009059.pub2
Liu, Q., Zhu, L., Cheng, C., Hu, Y. Y., Feng, Q. (2017). Natural Active Compounds from Plant Food and Chinese Herbal Medicine for Nonalcoholic Fatty Liver Disease. Curr. Pharm. Des. 23 (34), 5136–5162.
Liu, X., Tong, W., Zhao, X., Zhang, H., Tang, Y., Deng, X. (2017). Chinese herb extract improves liver steatosis by promoting the expression of high molecular weight adiponectin in NAFLD rats. Mol. Med. Rep. 16 (4), 5580–5586. doi: 10.3892/mmr.2017.7284
Liu, Y. L., Lin, L. C., Tung, Y. T., Ho, S. T., Chen, Y. L., Lin, C. C., et al. (2017). Rhododendron oldhamii leaf extract improves fatty liver syndrome by increasing lipid oxidation and decreasing the lipogenesis pathway in mice. Int. J. Med. Sci. 14 (9), 862–870. doi: 10.7150/ijms.19553
Liu, X., Xie, Z. H., Liu, C. Y., Zhang, Y. (2019). Effect of Chinese Herbal Monomer Hairy Calycosin on Nonalcoholic Fatty Liver Rats and its Mechanism. Comb. Chem. High Throughput Screen 22 (3), 194–200. doi: 10.2174/1386207322666190411112814
Liu, Y. Y., You, J. J., Xu, W., Zhai, T., Du, C. Y., Chen, Y., et al. (2019). Gynura procumbens aqueous extract alleviates nonalcoholic steatohepatitis through CFLAR-JNK pathway in vivo and in vitro. Chin. Herb. Medicines 11 (4), 369–378. doi: 10.1016/j.chmed.2019.09.005
Lo, V., Erickson, B., Thomason-Hughes, M., Ko, K. W., Dolinsky, V. W., Nelson, R., et al. (2010). Arylacetamide deacetylase attenuates fatty-acid-induced triacylglycerol accumulation in rat hepatoma cells. J. Lipid Res. 51 (2), 368–377. doi: 10.1194/jlr.M000596
Lomonaco, R., Sunny, N. E., Bril, F., Cusi, K. (2013). Nonalcoholic fatty liver disease: current issues and novel treatment approaches. Drugs 73 (1), 1–14. doi: 10.1007/s40265-012-0004-0
Lu, X., Yuan, Z. Y., Yan, X. J., Lei, F., Jiang, J. F., Yu, X., et al. (2016). Effects of Angelica dahurica on obesity and fatty liver in mice. Chin. J. Nat. Med. 14 (9), 641–652. doi: 10.1016/S1875-5364(16)30076-0
Luan, H., Huo, Z., Zhao, Z., Zhang, S., Huang, Y., Shen, Y., et al. (2019). Scutellarin, a modulator of mTOR, attenuates hepatic insulin resistance by regulating hepatocyte lipid metabolism via SREBP-1c suppression. Phytother. Res. doi: 10.1002/ptr.6582
Ma, J., Zhao, D., Wang, X., Ma, C., Feng, K., Zhang, S., et al. (2019). LongShengZhi Capsule Reduces Established Atherosclerotic Lesions in apoE-Deficient Mice by Ameliorating Hepatic Lipid Metabolism and Inhibiting Inflammation. J. Cardiovasc. Pharmacol. 73 (2), 105–117. doi: 10.1097/FJC.0000000000000642
Mandal, S., Mukhopadhyay, S., Bandhopadhyay, S., Sen, G., Biswas, T. (2014). 14-Deoxyandrographolide alleviates ethanol-induced hepatosteatosis through stimulation of AMP-activated protein kinase activity in rats. Alcohol 48 (2), 123–132. doi: 10.1016/j.alcohol.2013.11.005
Mato, J. M., Alonso, C., Noureddin, M., Lu, S. C. (2019). Biomarkers and subtypes of deranged lipid metabolism in non-alcoholic fatty liver disease. World J. Gastroenterol. 25 (24), 3009–3020. doi: 10.3748/wjg.v25.i24.3009
Meng, Q., Duan, X. P., Wang, C. Y., Liu, Z. H., Sun, P. Y., Huo, X. K., et al. (2017). Alisol B 23-acetate protects against non-alcoholic steatohepatitis in mice via farnesoid X receptor activation. Acta Pharmacol. Sin. 38 (1), 69–79. doi: 10.1038/aps.2016.119
Moctezuma-Velazquez, C. (2018). Current treatment for non-alcoholic fatty liver disease. Rev. Gastroenterol. Mex. 83 (2), 125–133. doi: 10.1016/j.rgmxen.2018.05.014
Musso, G., Gambino, R., Cassader, M. (2009). Recent insights into hepatic lipid metabolism in non-alcoholic fatty liver disease (NAFLD). Prog. Lipid Res. 48 (1), 1–26. doi: 10.1016/j.plipres.2008.08.001
Nguyen, P., Leray, V., Diez, M., Serisier, S., Le Bloc’h, J., Siliart, B., et al. (2008). Liver lipid metabolism. J. Anim. Physiol. Anim. Nutr. (Berl.) 92 (3), 272–283. doi: 10.1111/j.1439-0396.2007.00752.x
Ouali, F., Djouadi, F., Merlet-Benichou, C., Riveau, B., Bastin, J. (2000). Regulation of fatty acid transport protein and mitochondrial and peroxisomal beta-oxidation gene expression by fatty acids in developing rats. Pediatr. Res. 48 (5), 691–696. doi: 10.1203/00006450-200011000-00023
Parafati, M., Lascala, A., Morittu, V. M., Trimboli, F., Rizzuto, A., Brunelli, E., et al. (2015). Bergamot polyphenol fraction prevents nonalcoholic fatty liver disease via stimulation of lipophagy in cafeteria diet-induced rat model of metabolic syndrome. J. Nutr. Biochem. 26 (9), 938–948. doi: 10.1016/j.jnutbio.2015.03.008
Park, H., Kaushik, V. K., Constant, S., Prentki, M., Przybytkowski, E., Ruderman, N. B., et al. (2002). Coordinate regulation of malonyl-CoA decarboxylase, sn-glycerol-3-phosphate acyltransferase, and acetyl-CoA carboxylase by AMP-activated protein kinase in rat tissues in response to exercise. J. Biol. Chem. 277 (36), 32571–32577. doi: 10.1074/jbc.M201692200
Park, H. J., Han, J. M., Kim, H. G., Choi, M. K., Lee, J. S., Lee, H. W., et al. (2013). Chunggan extract (CGX), methionine-and choline-deficient (MCD) diet-induced hepatosteatosis and oxidative stress in C57BL/6 mice. Hum. Exp. Toxicol. 32 (12), 1258–1269. doi: 10.1177/0960327113485253
Park, H., Hwang, Y. H., Kim, D. G., Jeon, J., Ma, J. Y. (2015). Hepatoprotective effect of herb formula KIOM2012H against nonalcoholic fatty liver disease. Nutrients 7 (4), 2440–2455. doi: 10.3390/nu7042440
Park, T. Y., Hong, M., Sung, H., Kim, S., Suk, K. T. (2017). Effect of Korean Red Ginseng in chronic liver disease. J. Ginseng Res. 41 (4), 450–455. doi: 10.1016/j.jgr.2016.11.004
Peng, C. H., Liu, L. K., Chuang, C. M., Chyau, C. C., Huang, C. N., Wang, C. J. (2011). Mulberry water extracts possess an anti-obesity effect and ability to inhibit hepatic lipogenesis and promote lipolysis. J. Agric. Food Chem. 59 (6), 2663–2671. doi: 10.1021/jf1043508
Peng, H., He, Y., Zheng, G., Zhang, W., Yao, Z., Xie, W. (2016). Meta-analysis of traditional herbal medicine in the treatment of nonalcoholic fatty liver disease. Cell Mol. Biol. (Noisy-le-grand) 62 (4), 88–95.
Perla, F. M., Prelati, M., Lavorato, M., Visicchio, D., Anania, C. (2017). The Role of Lipid and Lipoprotein Metabolism in Non-Alcoholic Fatty Liver Disease. Children (Basel) 4 (6). doi: 10.3390/children4060046
Perumpail, B. J., Li, A. A., Iqbal, U., Sallam, S., Shah, N. D., Kwong, W., et al. (2018). Potential Therapeutic Benefits of Herbs and Supplements in Patients with NAFLD. Diseases 6 (3). doi: 10.3390/diseases6030080
Pierre, G., Macdonald, A., Gray, G., Hendriksz, C., Preece, M. A., Chakrapani, A. (2007). Prospective treatment in carnitine-acylcarnitine translocase deficiency. J. Inherit. Metab. Dis. 30 (5), 815. doi: 10.1007/s10545-007-0518-x
Pil Hwang, Y., Gyun Kim, H., Choi, J. H., Truong Do, M., Tran, T. P., Chun, H. K., et al. (2013). 3-Caffeoyl, 4-dihydrocaffeoylquinic acid from Salicornia herbacea attenuates high glucose-induced hepatic lipogenesis in human HepG2 cells through activation of the liver kinase B1 and silent information regulator T1/AMPK-dependent pathway. Mol. Nutr. Food Res. 57 (3), 471–482. doi: 10.1002/mnfr.201200529
Ponziani, F. R., Pecere, S., Gasbarrini, A., Ojetti, V. (2015). Physiology and pathophysiology of liver lipid metabolism. Expert Rev. Gastroenterol. Hepatol. 9 (8), 1055–1067. doi: 10.1586/17474124.2015.1056156
Qian, W., Cai, X., Zhang, X., Wang, Y., Qian, Q., Hasegawa, J. (2016). Effect of Daisaikoto on Expressions of SIRT1 and NF-kappaB of Diabetic Fatty Liver Rats Induced by High-Fat Diet and Streptozotocin. Yonago Acta Med. 59 (2), 149–158.
Qiu, P., Li, X., Kong, D. S., Li, H. Z., Niu, C. C., Pan, S. H. (2015). Herbal SGR Formula Prevents Acute Ethanol-Induced Liver Steatosis via Inhibition of Lipogenesis and Enhancement Fatty Acid Oxidation in Mice. Evid. Based Complement Alternat. Med. 2015, 613584. doi: 10.1155/2015/613584
Quiroga, A. D., Lehner, R. (2018). Pharmacological intervention of liver triacylglycerol lipolysis: The good, the bad and the ugly. Biochem. Pharmacol. 155, 233–241. doi: 10.1016/j.bcp.2018.07.005
Reddy, J. K., Rao, M. S. (2006). Lipid metabolism and liver inflammation. II. Fatty liver disease and fatty acid oxidation. Am. J. Physiol. Gastrointest Liver Physiol. 290 (5), G852–G858.
Ren, L., Sun, D., Zhou, X., Yang, Y., Huang, X., Li, Y., et al. (2019). Chronic treatment with the modified Longdan Xiegan Tang attenuates olanzapine-induced fatty liver in rats by regulating hepatic de novo lipogenesis and fatty acid beta-oxidation-associated gene expression mediated by SREBP-1c, PPAR-alpha and AMPK-alpha. J. Ethnopharmacol. 232, 176–187. doi: 10.1016/j.jep.2018.12.034
Roh, J. S., Lee, H., Lim, J., Kim, J., Yang, H., Yoon, Y., et al. (2017). Effect of Gangjihwan on hepatic steatosis and inflammation in high fat diet-fed mice. J. Ethnopharmacol. 206, 315–326. doi: 10.1016/j.jep.2017.06.008
Rui, L. (2014). Energy metabolism in the liver. Compr. Physiol. 4 (1), 177–197. doi: 10.1002/cphy.c130024
Saha, A. K., Ruderman, N. B. (2003). Malonyl-CoA and AMP-activated protein kinase: an expanding partnership. Mol. Cell Biochem. 253 (1-2), 65–70. doi: 10.1023/A:1026053302036
Seo, M. S., Hong, S. W., Yeon, S. H., Kim, Y. M., Um, K. A., Kim, J. H., et al. (2014). Magnolia officinalis attenuates free fatty acid-induced lipogenesis via AMPK phosphorylation in hepatocytes. J. Ethnopharmacol. 157, 140–148. doi: 10.1016/j.jep.2014.09.031
Shang, J., Chen, L. L., Xiao, F. X., Sun, H., Ding, H. C., Xiao, H. (2008). Resveratrol improves non-alcoholic fatty liver disease by activating AMP-activated protein kinase. Acta Pharmacol. Sin. 29 (6), 698–706. doi: 10.1111/j.1745-7254.2008.00807.x
Sheng, X., Wang, M., Lu, M., Xi, B., Sheng, H., Zang, Y. Q. (2011). Rhein ameliorates fatty liver disease through negative energy balance, hepatic lipogenic regulation, and immunomodulation in diet-induced obese mice. Am. J. Physiol. Endocrinol. Metab. 300 (5), E886–E893. doi: 10.1152/ajpendo.00332.2010
Sheng, D., Zhao, S., Gao, L., Zheng, H., Liu, W., Hou, J., et al. (2019). BabaoDan attenuates high-fat diet-induced non-alcoholic fatty liver disease via activation of AMPK signaling. Cell Biosci. 9, 77. doi: 10.1186/s13578-019-0339-2
Shi, K. Q., Fan, Y. C., Liu, W. Y., Li, L. F., Chen, Y. P., Zheng, M. H. (2012). Traditional Chinese medicines benefit to nonalcoholic fatty liver disease: a systematic review and meta-analysis. Mol. Biol. Rep. 39 (10), 9715–9722. doi: 10.1007/s11033-012-1836-0
Shi, L. J., Shi, L., Song, G. Y., Zhang, H. F., Hu, Z. J., Wang, C., et al. (2013). Oxymatrine attenuates hepatic steatosis in non-alcoholic fatty liver disease rats fed with high fructose diet through inhibition of sterol regulatory element binding transcription factor 1 (Srebf1) and activation of peroxisome proliferator activated receptor alpha (Pparalpha). Eur. J. Pharmacol. 714 (1-3), 89–95. doi: 10.1016/j.ejphar.2013.06.013
Shi, X., Sun, R. M., Zhao, Y., Fu, R., Wang, R. W., Zhao, H. Y., et al. (2018). Promotion of autophagosome-lysosome fusion via salvianolic acid A-mediated SIRT1 up-regulation ameliorates alcoholic liver disease. Rsc Adv. 8 (36), 20411–20422. doi: 10.1039/C8RA00798E
Singh, R., Cuervo, A. M. (2012). Lipophagy: connecting autophagy and lipid metabolism. Int. J. Cell Biol. 2012, 282041. doi: 10.1155/2012/282041
Sun, F., Xie, M. L., Zhu, L. J., Xue, J., Gu, Z. L. (2009). Inhibitory effect of osthole on alcohol-induced fatty liver in mice. Dig. Liver Dis. 41 (2), 127–133. doi: 10.1016/j.dld.2008.01.011
Sun, X. H., Zhang, L. D., Wei, W. (2019). A study on the mechanism of adipokine in non-alcoholic fatty liver in rats treated by four herbs decoction. Eur. J. Inflammation 17, 8. doi: 10.1177/2058739219853970
Tessari, P., Coracina, A., Cosma, A., Tiengo, A. (2009). Hepatic lipid metabolism and non-alcoholic fatty liver disease. Nutr. Metab. Cardiovasc. Dis. 19 (4), 291–302. doi: 10.1016/j.numecd.2008.12.015
Theodotou, M., Fokianos, K., Moniatis, D., Kadlenic, R., Chrysikou, A., Aristotelous, A., et al. (2019). Effect of resveratrol on non-alcoholic fatty liver disease. Exp. Ther. Med. 18 (1), 559–565. doi: 10.3892/etm.2019.7607
Uen, W. C., Shi, Y. C., Choong, C. Y., Tai, C. J. (2018). Cordycepin suppressed lipid accumulation via regulating AMPK activity and mitochondrial fusion in hepatocytes. J. Food Biochem. 42 (5), 7. doi: 10.1111/jfbc.12569
Veeramani, C., Alsaif, M. A., Al-Numair, K. S. (2017). Lavatera critica, a green leafy vegetable, controls high fat diet induced hepatic lipid accumulation and oxidative stress through the regulation of lipogenesis and lipolysis genes. BioMed. Pharmacother. 96, 1349–1357. doi: 10.1016/j.biopha.2017.11.072
Veeramani, C., Alsaif, M. A., Al-Numair, K. S. (2018). Herbacetin, a flaxseed flavonoid, ameliorates high percent dietary fat induced insulin resistance and lipid accumulation through the regulation of hepatic lipid metabolizing and lipid-regulating enzymes. Chem. Biol. Interact. 288, 49–56. doi: 10.1016/j.cbi.2018.04.009
Wanders, R. J., Waterham, H. R., Ferdinandusse, S. (2015). Metabolic Interplay between Peroxisomes and Other Subcellular Organelles Including Mitochondria and the Endoplasmic Reticulum. Front. Cell Dev. Biol. 3, 83.
Wang, Y. L., Liu, L. J., Zhao, W. H., Li, J. X. (2015). Intervening TNF-alpha via PPARgamma with Gegenqinlian Decoction in Experimental Nonalcoholic Fatty Liver Disease. Evid. Based Complement Alternat. Med. 2015, 715638.
Wang, Y., Viscarra, J., Kim, S. J., Sul, H. S. (2015). Transcriptional regulation of hepatic lipogenesis. Nat. Rev. Mol. Cell Biol. 16 (11), 678–689. doi: 10.1038/nrm4074
Wang, Y. P., Wat, E., Koon, C. M., Wong, C. W., Cheung, D. W., Leung, P. C., et al. (2016). The beneficial potential of polyphenol-enriched fraction from Erigerontis Herba on metabolic syndrome. J. Ethnopharmacol. 187, 94–103. doi: 10.1016/j.jep.2016.04.040
Wang, S. J., Chen, Q., Liu, M. Y., Yu, H. Y., Xu, J. Q., Wu, J. Q., et al. (2019). Regulation effects of rosemary (Rosmarinus officinalis Linn.) on hepatic lipid metabolism in OA induced NAFLD rats. Food Funct. 10 (11), 7356–7365.
Ward, C., Martinez-Lopez, N., Otten, E. G., Carroll, B., Maetzel, D., Singh, R., et al. (2016). Autophagy, lipophagy and lysosomal lipid storage disorders. Biochim. Biophys. Acta 1861 (4), 269–284. doi: 10.1016/j.bbalip.2016.01.006
Wu, W. Y., Wang, Y. P. (2012). Pharmacological actions and therapeutic applications of Salvia miltiorrhiza depside salt and its active components. Acta Pharmacol. Sin. 33 (9), 1119–1130. doi: 10.1038/aps.2012.126
Xiao, J., Fai So, K., Liong, E. C., Tipoe, G. L. (2013). Recent advances in the herbal treatment of non-alcoholic Fatty liver disease. J. Tradit. Complement Med. 3 (2), 88–94. doi: 10.4103/2225-4110.110411
Xu, L., Yin, L., Tao, X., Qi, Y., Han, X., Xu, Y., et al. (2017). Dioscin, a potent ITGA5 inhibitor, reduces the synthesis of collagen against liver fibrosis: Insights from SILAC-based proteomics analysis. Food Chem. Toxicol. 107 (Pt A), 318–328. doi: 10.1016/j.fct.2017.07.014
Yan, S., Khambu, B., Hong, H., Liu, G., Huda, N., Yin, X. M. (2019). Autophagy, Metabolism, and Alcohol-Related Liver Disease: Novel Modulators and Functions. Int. J. Mol. Sci. 20 (20). doi: 10.3390/ijms20205029
Yang, W., She, L., Yu, K., Yan, S., Zhang, X., Tian, X., et al. (2016). Jatrorrhizine hydrochloride attenuates hyperlipidemia in a high-fat diet-induced obesity mouse model. Mol. Med. Rep. 14 (4), 3277–3284. doi: 10.3892/mmr.2016.5634
Yang, L., Lin, W., Nugent, C. A., Hao, S., Song, H., Liu, T., et al. (2017). Lingguizhugan Decoction Protects against High-Fat-Diet-Induced Nonalcoholic Fatty Liver Disease by Alleviating Oxidative Stress and Activating Cholesterol Secretion. Int. J. Genomics 2017, 2790864. doi: 10.1155/2017/2790864
Yang, J. M., Sun, Y., Wang, M., Zhang, X. L., Zhang, S. J., Gao, Y. S., et al. (2019). Regulatory effect of a Chinese herbal medicine formula on non-alcoholic fatty liver disease. World J. Gastroenterol. 25 (34), 5105–5119. doi: 10.3748/wjg.v25.i34.5105
Yang, L., Yang, C., Thomes, P. G., Kharbanda, K. K., Casey, C. A., McNiven, M. A., et al. (2019). Lipophagy and Alcohol-Induced Fatty Liver. Front. Pharmacol. 10, 495. doi: 10.3389/fphar.2019.00495
Yang, X. X., Wang, X., Shi, T. T., Dong, J. C., Li, F. J., Zeng, L. X., et al. (2019). Mitochondrial dysfunction in high-fat diet-induced nonalcoholic fatty liver disease: The alleviating effect and its mechanism of Polygonatum kingianum. BioMed. Pharmacother. 117, 109083. doi: 10.1016/j.biopha.2019.109083
Yang, Y., Li, J., Wei, C., He, Y., Cao, Y., Zhang, Y., et al. (2019). Amelioration of nonalcoholic fatty liver disease by swertiamarin in fructose-fed mice. Phytomedicine 59, 152782. doi: 10.1016/j.phymed.2018.12.005
Yao, H., Qiao, Y. J., Zhao, Y. L., Tao, X. F., Xu, L. N., Yin, L. H., et al. (2016). Herbal medicines and nonalcoholic fatty liver disease. World J. Gastroenterol. 22 (30), 6890–6905. doi: 10.3748/wjg.v22.i30.6890
Yoon, S., Kim, J., Lee, H., Lee, H., Lim, J., Yang, H., et al. (2017). The effects of herbal composition Gambigyeongsinhwan (4) on hepatic steatosis and inflammation in Otsuka Long-Evans Tokushima fatty rats and HepG2 cells. J. Ethnopharmacol. 195, 204–213. doi: 10.1016/j.jep.2016.11.020
Younossi, Z. M. (2019). Non-alcoholic fatty liver disease - A global public health perspective. J. Hepatol. 70 (3), 531–544. doi: 10.1016/j.jhep.2018.10.033
Yu, S., Rao, S., Reddy, J. K. (2003). Peroxisome proliferator-activated receptors, fatty acid oxidation, steatohepatitis and hepatocarcinogenesis. Curr. Mol. Med. 3 (6), 561–572. doi: 10.2174/1566524033479537
Zamani, N., Shams, M., Nimrouzi, M., Zarshenas, M. M., Abolhasani Foroughi, A., Fallahzadeh Abarghooei, E., et al. (2018). The effects of Zataria multiflora Boiss. (Shirazi thyme) on nonalcoholic fatty liver disease and insulin resistance: A randomized double-blind placebo-controlled clinical trial. Complement Ther. Med. 41, 118–123. doi: 10.1016/j.ctim.2018.09.010
Zar Kalai, F., Han, J., Ksouri, R., Abdelly, C., Isoda, H. (2014). Oral administration of Nitraria retusa ethanolic extract enhances hepatic lipid metabolism in db/db mice model ‘BKS.Cg-Dock7(m)+/+ Lepr(db/)J’ through the modulation of lipogenesis-lipolysis balance. Food Chem. Toxicol. 72, 247–256. doi: 10.1016/j.fct.2014.07.029
Zhang, Y., Si, Y., Zhai, L., Yang, N., Yao, S., Sang, H., et al. (2013). Celastrus orbiculatus Thunb. ameliorates high-fat diet-induced non-alcoholic fatty liver disease in guinea pigs. Pharmazie 68 (10), 850–854.
Zhang, Y., Yu, L., Cai, W., Fan, S., Feng, L., Ji, G., et al. (2014). Protopanaxatriol, a novel PPARgamma antagonist from Panax ginseng, alleviates steatosis in mice. Sci. Rep. 4, 7375. doi: 10.1038/srep07375
Zhang, E., Yin, S., Song, X., Fan, L., Hu, H. (2016). Glycycoumarin inhibits hepatocyte lipoapoptosis through activation of autophagy and inhibition of ER stress/GSK-3-mediated mitochondrial pathway. Sci. Rep. 6, 38138. doi: 10.1038/srep38138
Zhang, L., Yao, Z., Ji, G. (2018). Herbal Extracts and Natural Products in Alleviating Non-alcoholic Fatty Liver Disease via Activating Autophagy. Front. Pharmacol. 9, 1459. doi: 10.3389/fphar.2018.01459
Zhang, E., Yin, S., Zhao, S., Zhao, C., Yan, M., Fan, L., et al. (2019). Protective effects of glycycoumarin on liver diseases. Phytother. Res. doi: 10.1002/ptr.6598
Zhang, Y., Liu, M., Chen, Q., Wang, T., Yu, H., Xu, J., et al. (2019). Leaves of Lippia triphylla improve hepatic lipid metabolism via activating AMPK to regulate lipid synthesis and degradation. J. Nat. Med. 73 (4), 707–716. doi: 10.1007/s11418-019-01316-5
Zheng, Y., Wang, M., Zheng, P., Tang, X., Ji, G. (2018). Systems pharmacology-based exploration reveals mechanisms of anti-steatotic effects of Jiang Zhi Granule on non-alcoholic fatty liver disease. Sci. Rep. 8 (1), 13681.
Zhong, H., Chen, K., Feng, M., Shao, W., Wu, J., Chen, K., et al. (2018). Genipin alleviates high-fat diet-induced hyperlipidemia and hepatic lipid accumulation in mice via miR-142a-5p/SREBP-1c axis. FEBS J. 285 (3), 501–517. doi: 10.1111/febs.14349
Zhou, W., Rahimnejad, S., Lu, K., Wang, L., Liu, W. (2019). Effects of berberine on growth, liver histology, and expression of lipid-related genes in blunt snout bream (Megalobrama amblycephala) fed high-fat diets. Fish Physiol. Biochem. 45 (1), 83–91. doi: 10.1007/s10695-018-0536-7
Zhu, M., Hao, S., Liu, T., Yang, L., Zheng, P., Zhang, L., et al. (2017). Lingguizhugan decoction improves non-alcoholic fatty liver disease by altering insulin resistance and lipid metabolism related genes: a whole trancriptome study by RNA-Seq. Oncotarget 8 (47), 82621–82631. doi: 10.18632/oncotarget.19734
Keywords: herbal medicines, natural products, lipid metabolism, fatty liver, lipolysis, lipogenesis
Citation: Li S, Xu Y, Guo W, Chen F, Zhang C, Tan HY, Wang N and Feng Y (2020) The Impacts of Herbal Medicines and Natural Products on Regulating the Hepatic Lipid Metabolism. Front. Pharmacol. 11:351. doi: 10.3389/fphar.2020.00351
Received: 31 December 2019; Accepted: 09 March 2020;
Published: 24 March 2020.
Edited by:
Min Ye, Peking University, ChinaReviewed by:
Helen Skaltsa, National and Kapodistrian University of Athens, GreeceGiuseppe Annunziata, University of Naples Federico II, Italy
Copyright © 2020 Li, Xu, Guo, Chen, Zhang, Tan, Wang and Feng. This is an open-access article distributed under the terms of the Creative Commons Attribution License (CC BY). The use, distribution or reproduction in other forums is permitted, provided the original author(s) and the copyright owner(s) are credited and that the original publication in this journal is cited, in accordance with accepted academic practice. No use, distribution or reproduction is permitted which does not comply with these terms.
*Correspondence: Yibin Feng, eWZlbmdAaGt1Lmhr