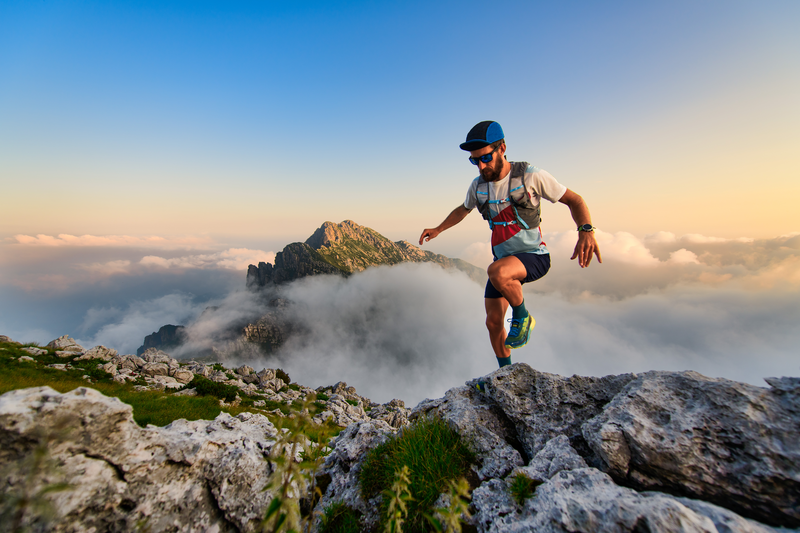
95% of researchers rate our articles as excellent or good
Learn more about the work of our research integrity team to safeguard the quality of each article we publish.
Find out more
OPINION article
Front. Pharmacol. , 30 January 2020
Sec. Experimental Pharmacology and Drug Discovery
Volume 10 - 2019 | https://doi.org/10.3389/fphar.2019.01691
We currently have no approved drugs for the treatment of Ebola virus disease (EVD) or post-Ebola syndrome (PES). A substantial proportion of patients presenting for treatment die, including healthcare workers (HCWs) with hospital-acquired infections. More than 28,000 people were suspected or confirmed with EVD and 11,000 died in the West Africa outbreak during 2014–2016. A new EVD epicenter developed in the Democratic Republic of Congo in mid-2018, and it is likely that new outbreaks will occur in the future. The low survival rate discourages patients from presenting for treatment, and the occupational risk discourages HCWs from caring for highly infectious patients. A substantial proportion of survivors complain of chronic symptoms, such as eye problems (47%) and arthralgias (64%)—a condition that has been termed PES (Wilson et al., 2018). Inflammation may play a role in both the uveitis, which can result in blindness (Shantha et al., 2017) and in arthritis (Amissah-Arthur et al., 2017). Availability of an efficacious adjunctive treatment drug would save lives, increase the number of people presenting for treatment, and increase the willingness of HCWs to care for patients. An efficacious drug for adjunctive treatment of PES could decrease morbidity suffered by survivors. To date, most research efforts have focused on vaccine for prevention and either antivirals or antibody preparations for treatment. However, given the extensive inflammatory component of EVD, adjunctive therapy to decrease inflammation—but not globally downregulate the host immune response in a manner that could be detrimental (e.g., steroids)—may hold promise for better outcomes for those infected. Although drugs such as acetylsalicylic acid, ibuprofen, indomethacin, and celecoxib are also broadly anti-inflammatory, they all inhibit cyclooxygenase and can interfere with platelet aggregation—a characteristic that would be disqualifying for use with hemorrhagic fevers.
“Cytokine storm,” a burst in production of inflammatory cytokines, is thought by many to be integral to EVD pathogenesis, and high levels of tumor necrosis factor (TNF)-α, interleukin (IL)-6, IL-10, IL-1β, macrophage inflammatory protein (MIP)-1α, MIP-1β, and macrophage chemoattractant protein (MCP)-1 are associated with fatal infections (Ruibal et al., 2016; Vernet et al., 2017). Nuclear factor of activated T cells (NFAT) is thought to be the key transcriptional regulator of inflammatory mediators (Madelain et al., 2018). Knock-out mice with dampened cytokine response (Tim-1 -/-) are considerably more likely to survive Ebola virus challenge than their wild-type counterparts, despite a limited impact on viremia (Younan et al., 2017).
We questioned whether the inflammation associated with cytokine storm could be countered pharmaceutically—and having some familiarity with one antimicrobial (minocycline) with significant anti-inflammatory properties as well as documented antiviral activity—we searched PubMed for articles describing cytokine activity during EVD and during minocycline use.
Minocycline is an FDA-approved semisynthetic tetracycline with an established safety profile that has been used for 40 years in the treatment of acne and rosacea (Cullen and Cohan, 1976; Hersle and Gisslen, 1976), and more recently, for multidrug resistant Acinetobacter (Lashinsky et al., 2017). It appears to have activity against certain viral pathogens: It inhibits H7N9 replication in vitro (Josset et al., 2014), attenuates stimulation of interferon-related gene and TRAILΨ in human dendritic cells and PBMCs exposed to HIV or influenza virus (Drewes et al., 2014), reduces West Nile Virus titers in brain-derived cell types in a dose-dependent manner (Michaelis et al., 2007), reduces Japanese encephalitis-induced damage in neuronal cell cultures (Mishra et al., 2009), and, based on molecular dynamics, may possibly inhibit the binding of Congo Crimean hemorrhagic fever virus to host nucleoprotein during cell infection—a host protein that is believed to be pivotal to viral replication (Sharifi et al., 2017). In a randomized controlled trial of patients with dengue hemorrhagic fever, compared to patients who received standard-of-care supportive treatment, those who also received the related tetracycline class antibiotic—doxycycline—had significantly lower mortality [20.9% vs 11.2% (p < 0.05)] and lower TNF and IL6 levels on days 3, 5, and 7 (p < 0.05 for all) (Fredeking et al., 2015). Table 1 compares the effects of Ebola virus and minocycline on selected biomarkers including important cytokines and chemokines.
As shown in our table, the anti-inflammatory activity of minocycline opposes those of many gene products of Ebola virus. It also selectively impairs NFAT-mediated transcriptional activation (Szeto et al., 2011). Due to its small size and lipophilic nature, minocycline may reach potentially therapeutic concentrations in tissue compartments for which antibiotic penetration is typically difficult, such as the eye (Abcouwer et al., 2013; Scholz et al., 2015) and joints (McEvoy, 2016). Such spaces appear to be capable of harboring Ebola virus (Varkey et al., 2015; Steptoe et al., 2017; Subissi et al., 2018) and are thought to contribute to the chronic sequelae seen in PES (Shantha et al., 2017; PREVAIL III Study Group, 2019; Heydari-Kamjani et al., 2019). However, pharmacokinetic/pharmacodynamic (PK/PD) data are lacking that would confirm minocycline penetration into such spaces. As previously mentioned, inflammation may play a role in both the potentially blinding uveitis and arthritis of PES. Although there are animal data to suggest that minocycline may have anti-inflammatory effects in the eye (Scholz et al., 2015) and human data to suggest anti-inflammatory activity in joints (Pradier et al., 2018), it is not known whether it has direct antiviral activity against Ebola virus.
Given minocycline’s broad anti-inflammatory activity against cytokines/chemokines that appear to be pathologically upregulated by Ebola virus and the safety history, relative availability, and affordability of minocycline, we feel it should be investigated as an adjunctive therapy in animal models of acute EVD. Given that it appears to cross into protected spaces where it may have anti-inflammatory activity, we feel it should also be investigated as adjunctive therapy for chronic sequelae of EVD (i.e., PES). It is likely that the anti-inflammatory benefit would be greater in certain infected populations (starting treatment early vs late in the infection), and it is also possible that, for some, downregulation of inflammation in general could impair host clearance of the virus. These important issues are amenable to investigation in animal models.
KH performed the literature review and drafted the table and text. MP helped to synthesize the tabular data and edited the text. JB reviewed the information and edited the text.
Use of trade names and commercial sources is for identification only and does not imply endorsement. This is an opinion piece based on literature review. No experiments have been conducted or data collected at this time for the potential adjunct treatment of Ebola virus disease or post-Ebola syndrome.
The authors declare that the research was conducted in the absence of any commercial or financial relationships that could be construed as a potential conflict of interest.
We would like to thank Dr. Conrad Quinn of the Centers for Disease Control and Prevention for listening to and asking questions about the concept and offering editorial advice.
Abcouwer, S. F., Lin, C. M., Shanmugam, S., Muthusamy, A., Barber, A. J., Antonetti, D. A. (2013). Minocycline prevents retinal inflammation and vascular permeability following ischemia-reperfusion injury. J. Neuroinflammation 10, 149. doi: 10.1186/1742-2094-10-149
Amissah-Arthur, M. B., Poller, B., Tunbridge, A., Adebajo, A. (2017). Musculoskeletal manifestations of Ebola virus. Rheumatology 57 (1), 28–31. doi: 10.1093/rheumatology/kex082%JRheumatology
Ashraf, T., Jiang, W., Hoque, M. T., Henderson, J., Wu, C., Bendayan, R. (2014). Role of anti-inflammatory compounds in human immunodeficiency virus-1 glycoprotein120-mediated brain inflammation. J. Neuroinflammation 11, 91. doi: 10.1186/1742-2094-11-91
Baize, S., Leroy, E. M., Georges, A. J., Georges-Courbot, M. C., Capron, M., Bedjabaga, I., et al. (2002). Inflammatory responses in Ebola virus-infected patients. Clin. Exp. Immunol. 128 (1), 163–168. doi: 10.1046/j.1365-2249.2002.01800.x
Chu, H. C., Lin, Y. L., Sytwu, H. K., Lin, S. H., Liao, C. L., Chao, Y. C. (2005). Effects of minocycline on Fas-mediated fulminant hepatitis in mice. Br. J. Pharmacol. 144 (2), 275–282. doi: 10.1038/sj.bjp.0706079
Cilloniz, C., Ebihara, H., Ni, C., Neumann, G., Korth, M. J., Kelly, S. M., et al. (2011). Functional genomics reveals the induction of inflammatory response and metalloproteinase gene expression during lethal Ebola virus infection. J. Virol. 85 (17), 9060–9068. doi: 10.1128/jvi.00659-11
Cullen, S. I., Cohan, R. H. (1976). Minocycline therapy in acne vulgaris. Cutis 17 (6), 1208–1210. 14.
Czerny, C., Kholmukhamedov, A., Theruvath, T. P., Maldonado, E. N., Ramshesh, V. K., Lehnert, M., et al. (2012). Minocycline decreases liver injury after hemorrhagic shock and resuscitation in mice. HPB Surg. World J. Hepatic Pancreatic Biliary Surg. 2012, 259512. doi: 10.1155/2012/259512
Drewes, J. L., Szeto, G. L., Engle, E. L., Liao, Z., Shearer, G. M., Zink, M. C., et al. (2014). Attenuation of pathogenic immune responses during infection with human and simian immunodeficiency virus (HIV/SIV) by the tetracycline derivative minocycline. PloS One 9 (4), e94375. doi: 10.1371/journal.pone.0094375
Fortier, L. A., Motta, T., Greenwald, R. A., Divers, T. J., Mayr, K. G. (2010). Synoviocytes are more sensitive than cartilage to the effects of minocycline and doxycycline on IL-1alpha and MMP-13-induced catabolic gene responses. J. Orthopaedic Res. Off. Publ. Orthopaedic Res. Soc. 28 (4), 522–528. doi: 10.1002/jor.21006
Fredeking, T. M., Zavala-Castro, J. E., Gonzalez-Martinez, P., Moguel-Rodriguez, W., Sanchez, E. C., Foster, M. J., et al. (2015). Dengue patients treated with doxycycline showed lower mortality associated to a reduction in IL-6 and TNF levels. Recent patents on anti-infective drug discovery. Recent Pat. Antiinfect. Drug Discov. 10, 1, 51–58. doi: 10.2174/1574891x10666150410153839
Geisbert, T. W., Hensley, L. E., Larsen, T., Young, H. A., Reed, D. S., Geisbert, J. B., et al. (2003). Pathogenesis of Ebola hemorrhagic fever in cynomolgus macaques: evidence that dendritic cells are early and sustained targets of infection. Am. J. Pathol. 163 (6), 2347–2370. doi: 10.1016/s0002-9440(10)63591-2
Gupta, M., MacNeil, A., Reed, Z. D., Rollin, P. E., Spiropoulou, C. F. (2012). Serology and cytokine profiles in patients infected with the newly discovered Bundibugyo ebolavirus. Virology 423 (2), 119–124. doi: 10.1016/j.virol.2011.11.027
Hensley, L. E., Young, H. A., Jahrling, P. B., Geisbert, T. W. (2002). Proinflammatory response during Ebola virus infection of primate models: possible involvement of the tumor necrosis factor receptor superfamily. Immunol. Lett. 80 (3), 169–179. doi: 10.1016/s0165-2478(01)00327-3
Hersle, K., Gisslen, H. (1976). Minocycline in acne vulgaris: a double-blind study. Curr. Ther. Res. Clin. Exp. 19 (3), 339–342.
Heydari-Kamjani, M., Demory Beckler, M., Kesselman, M. M. (2019). Reconsidering the use of minocycline in the preliminary treatment regime of rheumatoid arthritis. Cureus 11 (8), e5351. doi: 10.7759/cureus.5351
Hou, Y., Ryu, C. H., Park, K. Y., Kim, S. M., Jeong, C. H., Jeun, S. S. (2013). Effective combination of human bone marrow mesenchymal stem cells and minocycline in experimental autoimmune encephalomyelitis mice. Stem Cell Res. Ther. 4 (4), 77. doi: 10.1186/scrt228
Huang, C., Li, R., Zeng, Q., Ding, Y., Zou, Y., Mao, X., et al. (2012). Effect of minocycline postconditioning and ischemic postconditioning on myocardial ischemia-reperfusion injury in atherosclerosis rabbits. J. Huazhong University Sci. Technol. Med. Sci. = Hua zhong ke ji da xue xue bao Yi xue Ying De wen ban = Huazhong keji daxue xuebao Yixue Yingdewen ban 32 (4), 524–529. doi: 10.1007/s11596-012-0090-y
Hutchinson, K. L., Rollin, P. E. (2007). Cytokine and chemokine expression in humans infected with Sudan Ebola virus. J. Infect. Dis. 196 Suppl 2, S357–S363. doi: 10.1086/520611
Josset, L., Zeng, H., Kelly, S. M., Tumpey, T. M., Katze, M. G. (2014). Transcriptomic characterization of the novel avian-origin influenza A (H7N9) virus: specific host response and responses intermediate between avian (H5N1 and H7N7) and human (H3N2) viruses and implications for treatment options. mBio 5 (1), e01102–e01113. doi: 10.1128/mBio.01102-13
Lashinsky, J. N., Henig, O., Pogue, J. M., Kaye, K. S. (2017). Minocycline for the treatment of multidrug and extensively drug-resistant a. baumannii: A review. Infect. Dis. Ther. 6 (2), 199–211. doi: 10.1007/s40121-017-0153-2
Ledeboer, A., Sloane, E. M., Milligan, E. D., Frank, M. G., Mahony, J. H., Maier, S. F., et al. (2005). Minocycline attenuates mechanical allodynia and proinflammatory cytokine expression in rat models of pain facilitation. Pain 115 (1-2), 71–83. doi: 10.1016/j.pain.2005.02.009
Madelain, V., Baize, S., Jacquot, F., Reynard, S., Fizet, A., Barron, S., et al. (2018). Ebola viral dynamics in nonhuman primates provides insights into virus immuno-pathogenesis and antiviral strategies. Nat. Commun. 9 (1), 4013. doi: 10.1038/s41467-018-06215-z
Mahanty, S., Gupta, M., Paragas, J., Bray, M., Ahmed, R., Rollin, P. E. (2003). Protection from lethal infection is determined by innate immune responses in a mouse model of Ebola virus infection. Virology 312 (2), 415–424. doi: 10.1016/s0042-6822(03)00233-2
Masocha, W., Rottenberg, M. E., Kristensson, K. (2006). Minocycline impedes African trypanosome invasion of the brain in a murine model. Antimicrob. Agents Chemother. 50 (5), 1798–1804. doi: 10.1128/aac.50.5.1798-1804.2006
McEvoy, T. (2016). Minocycline: rheumatoid arthritis. Hospital Pharm. 51 (7), 535–538. doi: 10.1310/hpj5107-535
Michaelis, M., Kleinschmidt, M. C., Doerr, H. W., Cinatl, J., Jr. (2007). Minocycline inhibits West Nile virus replication and apoptosis in human neuronal cells. J. Antimicrob. Chemother. 60 (5), 981–986. doi: 10.1093/jac/dkm307
Mishra, M. K., Ghosh, D., Duseja, R., Basu, A. (2009). Antioxidant potential of minocycline in japanese encephalitis virus infection in murine neuroblastoma cells: correlation with membrane fluidity and cell death. Neurochem. Int. 54 (7), 464–470. doi: 10.1016/j.neuint.2009.01.022
Pradier, M., Robineau, O., Boucher, A., Titecat, M., Blondiaux, N., Valette, M., et al. (2018). Suppressive antibiotic therapy with oral tetracyclines for prosthetic joint infections: a retrospective study of 78 patients. Rheumatology 46 (1), 39–47. doi: 10.1007/s15010-017-1077-1
PREVAIL III Study Group (2019). A longitudinal study of ebola sequelae in liberia. N. Engl. J. Med. 380, 10, 924–934. doi: 10.1056/NEJMoa1805435
Rollin, P. E., Bausch, D. G., Sanchez, A. (2007). Blood chemistry measurements and D-Dimer levels associated with fatal and nonfatal outcomes in humans infected with Sudan Ebola virus. J. Infect. Dis. 196 Suppl 2, S364–S371. doi: 10.1086/520613
Rubins, K. H., Hensley, L. E., Wahl-Jensen, V., Daddario DiCaprio, K. M., Young, H. A., Reed, D. S., et al. (2007). The temporal program of peripheral blood gene expression in the response of nonhuman primates to Ebola hemorrhagic fever. Genome Biol. 8 (8), R174. doi: 10.1186/gb-2007-8-8-r174
Ruibal, P., Oestereich, L., Ludtke, A., Becker-Ziaja, B., Wozniak, D. M., Kerber, R., et al. (2016). Unique human immune signature of Ebola virus disease in Guinea. Nature 533 (7601), 100–104. doi: 10.1038/nature17949
Sanchez, A., Lukwiya, M., Bausch, D., Mahanty, S., Sanchez, A. J., Wagoner, K. D., et al. (2004). Analysis of human peripheral blood samples from fatal and nonfatal cases of Ebola (Sudan) hemorrhagic fever: cellular responses, virus load, and nitric oxide levels. J. Virol. 78 (19), 10370–10377. doi: 10.1128/jvi.78.19.10370-10377.2004
Scholz, R., Sobotka, M., Caramoy, A., Stempfl, T., Moehle, C., Langmann, T. (2015). Minocycline counter-regulates pro-inflammatory microglia responses in the retina and protects from degeneration. J. Neuroinflammation 12, 209. doi: 10.1186/s12974-015-0431-4
Schwartz, J., Holmuhamedov, E., Zhang, X., Lovelace, G. L., Smith, C. D., Lemasters, J. J. (2013). Minocycline and doxycycline, but not other tetracycline-derived compounds, protect liver cells from chemical hypoxia and ischemia/reperfusion injury by inhibition of the mitochondrial calcium uniporter. Toxicol. Appl. Pharmacol. 273 (1), 172–179. doi: 10.1016/j.taap.2013.08.027
Shantha, J. G., Crozier, I., Yeh, S. (2017). An update on ocular complications of Ebola virus disease. Curr. Opin. Ophthalmol. 28 (6), 600–606. doi: 10.1097/icu.0000000000000426
Sharifi, A., Amanlou, A., Moosavi-Movahedi, F., Golestanian, S., Amanlou, M. (2017). Tetracyclines as a potential antiviral therapy against Crimean Congo hemorrhagic fever virus: Docking and molecular dynamic studies. Comput. Biol. Chem. 70, 1–6. doi: 10.1016/j.compbiolchem.2017.06.003
Steptoe, P. J., Scott, J. T., Baxter, J. M., Parkes, C. K., Dwivedi, R., Czanner, G., et al. (2017). Novel retinal lesion in ebola survivors, sierra leone, 2016. Emerging Infect. Dis. 23 (7), 1102–1109. doi: 10.3201/eid2307.161608
Subissi, L., Keita, M., Mesfin, S., Rezza, G., Diallo, B., Van Gucht, S., et al. (2018). Ebola virus transmission caused by persistently infected survivors of the 2014-2016 outbreak in west africa. J. Infect. Dis. 218 (suppl_5), S287–Ss91. doi: 10.1093/infdis/jiy280
Suzuki, H., Sugimura, Y., Iwama, S., Suzuki, H., Nobuaki, O., Nagasaki, H., et al. (2010). Minocycline prevents osmotic demyelination syndrome by inhibiting the activation of microglia. J. Am. Soc. Nephrol. JASN 21 (12), 2090–2098. doi: 10.1681/asn.2010040438
Szeto, G. L., Brice, A. K., Yang, H. C., Barber, S. A., Siliciano, R. F., Clements, J. E. (2010). Minocycline attenuates HIV infection and reactivation by suppressing cellular activation in human CD4+ T cells. J. Infect. Dis. 201 (8), 1132–1140. doi: 10.1086/651277
Szeto, G. L., Pomerantz, J. L., Graham, D. R., Clements, J. E. (2011). Minocycline suppresses activation of nuclear factor of activated T cells 1 (NFAT1) in human CD4+ T cells. J. Biol. Chem. 286 (13), 11275–11282. doi: 10.1074/jbc.M110.210518
Tai, K., Iwasaki, H., Ikegaya, S., Ueda, T. (2013). Minocycline modulates cytokine and chemokine production in lipopolysaccharide-stimulated THP-1 monocytic cells by inhibiting IkappaB kinase alpha/beta phosphorylation. Transl. Res. J. Lab. Clin. Med. 161 (2), 99–109. doi: 10.1016/j.trsl.2012.10.001
Tang, X. N., Wang, Q., Koike, M. A., Cheng, D., Goris, M. L., Blankenberg, F. G., et al. (2007). Monitoring the protective effects of minocycline treatment with radiolabeled annexin V in an experimental model of focal cerebral ischemia. J. Nucl. Med. Off. Publ. Soc. Nucl. Med. 48 (11), 1822–1828. doi: 10.2967/jnumed.107.041335
Varkey, J. B., Shantha, J. G., Crozier, I., Kraft, C. S., Lyon, G. M., Mehta, A. K., et al. (2015). Persistence of ebola virus in ocular fluid during convalescence. N. Engl. J. Med. 372 (25), 2423–2427. doi: 10.1056/NEJMoa1500306
Vernet, M. A., Reynard, S., Fizet, A., Schaeffer, J., Pannetier, D., Guedj, J., et al. (2017). Clinical, virological, and biological parameters associated with outcomes of Ebola virus infection in Macenta, Guinea. JCI Insight 2 (6), e88864. doi: 10.1172/jci.insight.88864
Villinger, F., Rollin, P. E., Brar, S. S., Chikkala, N. F., Winter, J., Sundstrom, J. B., et al. (1999). Markedly elevated levels of interferon (IFN)-gamma, IFN-alpha, interleukin (IL)-2, IL-10, and tumor necrosis factor-alpha associated with fatal Ebola virus infection. J. Infect. Dis. 179 Suppl;1, S188–S191. doi: 10.1086/514283
Wauquier, N., Becquart, P., Padilla, C., Baize, S., Leroy, E. M. (2010). Human fatal zaire ebola virus infection is associated with an aberrant innate immunity and with massive lymphocyte apoptosis. PLoS Negl. Trop. Dis. 4 (10), e837. doi: 10.1371/journal.pntd.0000837
Wilson, H. W., Amo-Addae, M., Kenu, E., Ilesanmi, O. S., Ameme, D. K., Sackey, S. O. (2018). Post-ebola syndrome among ebola virus disease survivors in montserrado county, liberia 2016. BioMed Res. Int. 2018, 1909410. doi: 10.1155/2018/1909410
Wu, K. L., Chan, S. H., Chan, J. Y. (2012). Neuroinflammation and oxidative stress in rostral ventrolateral medulla contribute to neurogenic hypertension induced by systemic inflammation. J. Neuroinflammation 9, 212. doi: 10.1186/1742-2094-9-212
Yang, L. P., Zhu, X. A., Tso, M. O. (2007). Minocycline and sulforaphane inhibited lipopolysaccharide-mediated retinal microglial activation. Mol. Vision 13, 1083–1093.
Keywords: Ebola virus disease, minocycline, drug discovery, cytokine, chemokine, hepatoprotection
Citation: Hendricks K, Parrado MG and Bradley J (2020) Opinion: An Existing Drug to Assess In Vivo for Potential Adjunctive Therapy of Ebola Virus Disease and Post-Ebola Syndrome. Front. Pharmacol. 10:1691. doi: 10.3389/fphar.2019.01691
Received: 31 October 2019; Accepted: 24 December 2019;
Published: 30 January 2020.
Edited by:
Salvatore Salomone, University of Catania, ItalyReviewed by:
Sina Bavari, United States Army Medical Research Institute of Infectious Diseases (USAMRIID), United StatesCopyright © 2020 Hendricks, Parrado and Bradley. This is an open-access article distributed under the terms of the Creative Commons Attribution License (CC BY). The use, distribution or reproduction in other forums is permitted, provided the original author(s) and the copyright owner(s) are credited and that the original publication in this journal is cited, in accordance with accepted academic practice. No use, distribution or reproduction is permitted which does not comply with these terms.
*Correspondence: Katherine Hendricks, S2F0ZUBIZWFsdGhLQVAuY29t
Disclaimer: All claims expressed in this article are solely those of the authors and do not necessarily represent those of their affiliated organizations, or those of the publisher, the editors and the reviewers. Any product that may be evaluated in this article or claim that may be made by its manufacturer is not guaranteed or endorsed by the publisher.
Research integrity at Frontiers
Learn more about the work of our research integrity team to safeguard the quality of each article we publish.