- 1Institute of Pharmacology, Università Cattolica del Sacro Cuore, Roma, Italy
- 2Department of Physiology and Pharmacology “V. Erspamer,” Sapienza University of Rome, Rome, Italy
- 3Department of Clinical and Experimental Medicine, University of Foggia, Foggia, Italy
- 4Fondazione Policlinico Universitario A. Gemelli IRCCS, Roma, Italy
Over the last few years, several preclinical studies have shown that some herbal products, such as ferulic acid, Ginkgo biloba, and resveratrol, exert neuroprotective effects through the modulation of the heme oxygenase/biliverdin reductase system. Unfortunately, sufficient data supporting the shift of knowledge from preclinical studies to humans, particularly in neurodegenerative diseases, are not yet available in the literature. The purpose of this review is to summarize the studies and the main results achieved on the potential therapeutic role of the interaction between the heme oxygenase/biliverdin reductase system with ferulic acid, G. biloba, and resveratrol. Some critical issues have also been reported, mainly concerning the safety profile and the toxicological sequelae associated to the supplementation with the herbs mentioned above, based on both current literature and specific reports issued by the competent Regulatory Authorities.
Background
At the end of Sixties, three skilled scientists from the University of California San Francisco Medical Center first described and characterized the enzymatic activity of heme oxygenase (HO), a microsomal enzyme catalyzing the oxidative cleavage of hemoproteins’ prosthetic group in equimolar amounts of ferrous iron, carbon monoxide (CO) and biliverdin (BV) (Tenhunen et al., 1968; Tenhunen et al., 1969). Following this initial description of the enzymatic activity, there were many other findings, including the inducible and constitutive nature of HO (HO-1 and HO-2, respectively), as well as the full characterization of biliverdin reductase (BVR), a cytosolic enzyme that works in combination with HO and reduces BV into bilirubin (BR). A significant contribution to these discoveries was provided by Mahin Maines and her research group [see (Maines, 1988; Maines, 1997; Maines, 2005) and references therein] who, recently, have deepened the field by describing pleiotropic effects of BVR in terms of modulation of numerous cytoprotective signaling pathways [see (Maines and Gibbs, 2005; Kapitulnik and Maines, 2009; Gibbs et al., 2015) and references therein].
Following these observations, the HO/BVR system has been studied worldwide by scientists who have gradually discovered its enormous potential. Just to give an idea of the attention aroused, suffice it to say that since 1969, more than 15300 papers have been published containing the keyword “heme oxygenase” (source: PubMed, accessed on August 8, 2019), while, concerning “biliverdin reductase,” the first studies appeared in 1965 reaching, to date, more than 450 papers (source: PubMed, accessed on August 8, 2019). The numbers mentioned so far have been achieved thanks to the many studies that have described the involvement of the HO/BVR system in the pathogenesis of Alzheimer’s disease (AD), Parkinson’s disease (PD), atherosclerosis and other cardiovascular disorders, kidney diseases, diabetes, etc. The molecular mechanisms through which the HO-1/BVR system exerts neuroprotective effects mainly depends on the down-stream effectors CO and BR. In the nervous system, CO has been shown to modulate synaptic plasticity, neuropeptide secretion, and neurogenesis (Verma et al., 1993; Mancuso et al., 1998; Mancuso et al., 2010; Choi, 2018), whereas BR interacts with reactive oxygen species (ROS), reactive nitrogen species (RNS) and nitric oxide (NO) to prevent neurotoxicity due to free radical injury (Mancuso et al., 2006a; Mancuso et al., 2008; Barone et al., 2009b; Mancuso et al., 2012a). While the antioxidant and cytoprotective effects of the HO/BVR system and its by-products have been extensively described in literature, scarce attention has been dedicated to a critical examination of their potentially toxic effects. Our research group has contributed to this field by describing the cytotoxic effects of CO either through the inhibition of the stress axis under pro-inflammatory conditions or production of pro-inflammatory prostaglandins (PG) (e.g., PGE2) in rat hypothalamus [see (Mancuso et al., 1997; Mancuso et al., 2006c; Mancuso et al., 2010) and references therein]. Moreover, we have also explored the cytoprotective vs cytotoxic effects of the interaction between BR and NO [see (Mancuso et al., 2006b; Mancuso, 2017) and references therein; (Barone et al., 2009b)]. Hyman Schipper’s group, instead, decisively contributed to the discovery of the neurotoxic role of HO-derived iron in mitochondrial dysfunctions and in the genesis of oxidative stress-induced damage in neurons and glial cells [see (Schipper, 2004; Schipper et al., 2009; Schipper et al., 2019) and references therein]. Finally, Shigeki Shibahara and Kazuhiko Igarashi, with collaborators, discovered the importance of HO-1 gene repression, through the transcription factor Bach1, to reduce cellular toxic effects due to iron and CO accumulation as in the case of strong and long-lasting pro-oxidant conditions (Shibahara, 2003; Shibahara et al., 2003; Igarashi and Sun, 2006). These findings contributed to increase the awareness of the risks associated with the uncontrolled activation of the HO/BVR system.
A particularly detailed aspect, starting from the early 2000s, was the interaction between HO-1 and products of herbal origin, and caffeic acid derivatives were the first to be studied (Scapagnini et al., 2002); only later, many studies have appeared in the literature concerning the ability of several other herbal products to induce HO-1 (Mancuso et al., 2012b; Mancuso and Santangelo, 2014; Fetoni et al., 2015; Mancuso and Santangelo, 2017). However, as detailed below, the up-regulation of HO-1 by herb-derived nutritional supplements is claimed as a beneficial mechanism through which they exert neuroprotective outcomes. In this regard, however, it is worth underlining how not always the fine involvement of HO-1 in the claimed neuroprotective effects of herbal products has been studied, but frequently HO-1 induction has been considered rather as a mere biomarker of activation of the cell stress response. In our opinion, the aspects that are still weak and are worth investigating, with regard to the effects of herbal products through HO-1 up-regulation, are the following: (i) the correlation between the dose/concentration of herbal product capable of inducing HO-1 in vitro and its effective concentration in the target organ within in vivo studies; (ii) the extent and duration of HO-1 induction, considering that, as previously mentioned, the accumulation of products, such as iron and CO can become toxic to cells, (iii) loss of specificity of the obtained results, as nearly all the studied herbal products induced HO-1 and (iv) the reduced number of studies that evaluated the BVR modulation, through herbal products.
The purpose of this review is to provide a critical overview of the potential therapeutic role of ferulic acid, Ginkgo biloba, and resveratrol via the modulation of the HO/BVR system; the reason why the attention has been focused on these herbal products depends on the consistent number of articles published on this topic through the years strong enough to substantiate a potential interest towards clinics. The safety profile of ferulic acid, G. biloba, and resveratrol has been also evaluated with the purpose to provide a complete overview of the risk/benefit balance of a chronic supplementation with these agents.
Ferulic Acid
Ferulic acid {[(E)-3-(4-hydroxy-3-methoxy-phenyl)prop-2-enoic acid)], FA, Figure 1} belongs to the family of phenolic acids and is highly abundant in fruits and vegetables. Furthermore, FA is also a component of Chinese medicinal herbs, such as Angelica sinensis, Cimicifuga racemosa, and Ligusticum chuangxiong. The main pharmacokinetic parameters of FA are shown in Table 1.
Over the past years, several studies have shown that FA acts as a strong antioxidant not only by a direct free radical-scavenging mechanism, but largely by enhancing the cell stress response through the up-regulation of the HO/BVR system (Barone et al., 2009a; Mancuso and Santangelo, 2014). On the other hand, literature data have highlighted the contribution of FA-induced HO-1 up-regulation to numerous biological effects in several preclinical models (see Table 2).
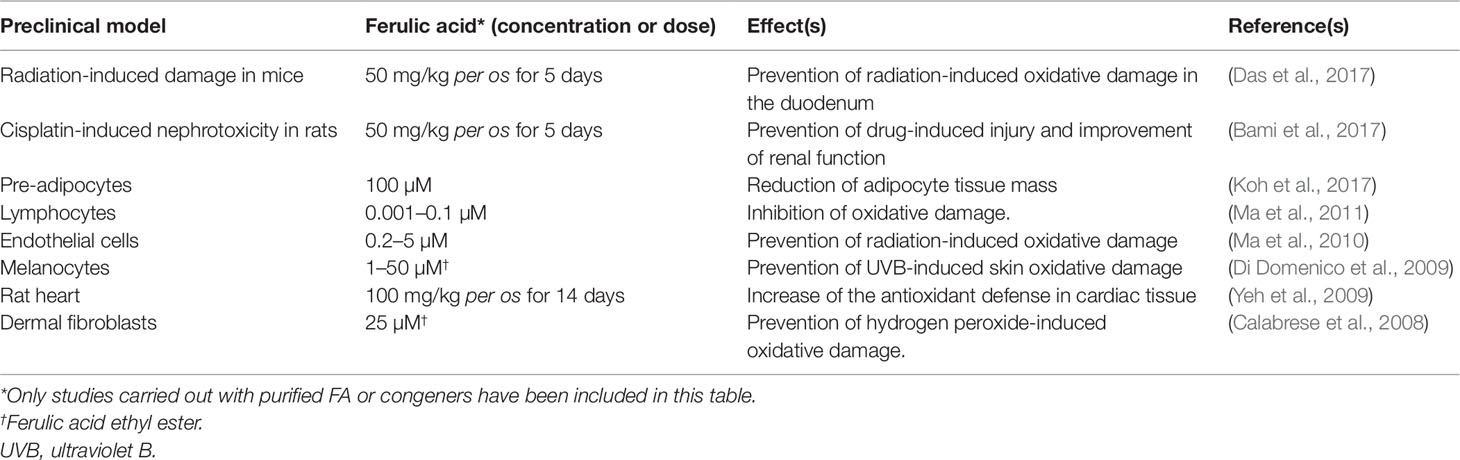
Table 2 Contribution of HO-1 up-regulation to the biological effects of ferulic acid (FA) in preclinical in vitro and in vivo models.
Ferulic acid and its ethyl ester [5–50 µM or 150 mg/kg intraperitoneal (i.p.)] were reported to over-express HO-1 in rat neurons and gerbil synaptosomes resulting in a significant neuroprotective effect on ROS- and glucose oxidase- related oxidative damage (Kanski et al., 2002; Scapagnini et al., 2004; Joshi et al., 2006). In the neuronal cell line SH-SY5Y, FA (1–10 µM) exerted marked neuroprotective effects against trimethyltin-induced damage by increasing the expression of HO-1; the translocation of the transcriptional inducer nuclear factor erythroid 2-related factor 2 (Nrf2) from cytosol to the nucleus has been described as the molecular mechanism underlining the FA-induced HO-1 up-regulation (Catino et al., 2016). Moreover, CO and BR have been identified as the by-products of HO and BVR activities responsible for the antioxidant and neuroprotective effects of FA (Catino et al., 2016). Through the specific up-regulation of the Nrf2/HO-1 system, FA (3–30 µM) counteracted lead-induced inhibition of neurite outgrow in PC12 cells (Yu et al., 2016). As shown by Ma et al. (Ma et al., 2011), the extracellular signal-regulated kinase (ERK) has a role in mediating the FA-activation of Nrf2/HO-1 since its blockade counteracts the nuclear translocation and transcriptional activity of Nrf2 on the HO-1 gene. Quite recently, our research group has demonstrated how FA exerts neuroprotective effects not only under pro-oxidant conditions, but also during psychosocial stress. As shown by Mhillaj et al. (Mhillaj et al., 2018), FA (150 mg/kg i.p.) enhances long-term memory in rats exposed to novelty-induced emotional arousal through the up-regulation of HO-1 in the hippocampus and frontal cortex; it is worth mentioning the finding that FA also over-expressed HO-2 in the frontal cortex. CO is responsible for this nootropic effect of FA, whereas BV does not have any significant effect (Mhillaj et al., 2018).
Ferulic acid (150 mg/kg i.p. for 4 days) up-regulated HO-1 in the organ of Corti of guinea pigs exposed to acoustic trauma; FA-induced improvement of the auditory function was counteracted by the HO inhibitor zinc-protoporphyrin-IX and paralleled the time-course of FA-induced HO-1 overexpression, thus supporting the hypothesis that the neuroprotective effect of this phenolic acid was due to the induction of cytoprotective HO-1 (Fetoni et al., 2010). Interestingly, FA (21.61 mg/kg for 3 weeks intragastric) increased the HO-1 expression and counteracted visible light-induced retinal degeneration in pigmented rabbits (Wang et al., 2016b).
Ferulic acid has also been complexed with tacrine, one of the earliest drugs developed for AD therapy and later discarded for severe hepatotoxicity. Tacrine-FA (2–100 µM) has been shown to prevent β-amyloid (Aβ) aggregation, ROS formation, and apoptosis in PC12 cells; in addition, tacrine-FA (2–20 mg/kg intragastric) improved cognitive skills in a mouse model of AD (Pi et al., 2012). In a subsequent paper, Huang et al. (Huang et al., 2012) showed how tacrine-FA inhibits oxidative stress-induced damage by over-expressing HO-1, through Nrf2 translocation, in HT22 cells.
Ferulic acid has an enviable safety profile, since no significant toxicities have been reported in humans; furthermore, FA’s modulation of drug metabolizing enzymes is negligible (see https://toxnet.nlm.nih.gov. Accessed on June 27, 2019). However, female rats treated with the highest tolerated dose of tacrine-FA have shown both glycogen depletion and HO-1 induction and cytochrome-P450 (CYP) CYP1A1, 2B1, and 3A2 up-regulation in the liver, mainly due to the tacrine moiety (Lupp et al., 2010). A careful risk/benefit analysis of the pharmacological and safety profiles of tacrine-FA is required.
Ginkgo Biloba
Gingko biloba is a plant growing in the mountainous valleys of Eastern China. G. biloba extracts contain several active compounds, including flavonoids, terpenes, organic acids, and polyphenols, the most important being flavonol-glycosides (primarily quercetin, kaempferol, and isorhamnetin) and terpene-lactones; the latter are further divided into diterpenes (ginkgolides) and sesquiterpenes (bilobalide) (Figure 2). For a summary of the pharmacokinetic parameters and biological effects of G. biloba, see Tables 1 and 3, respectively.
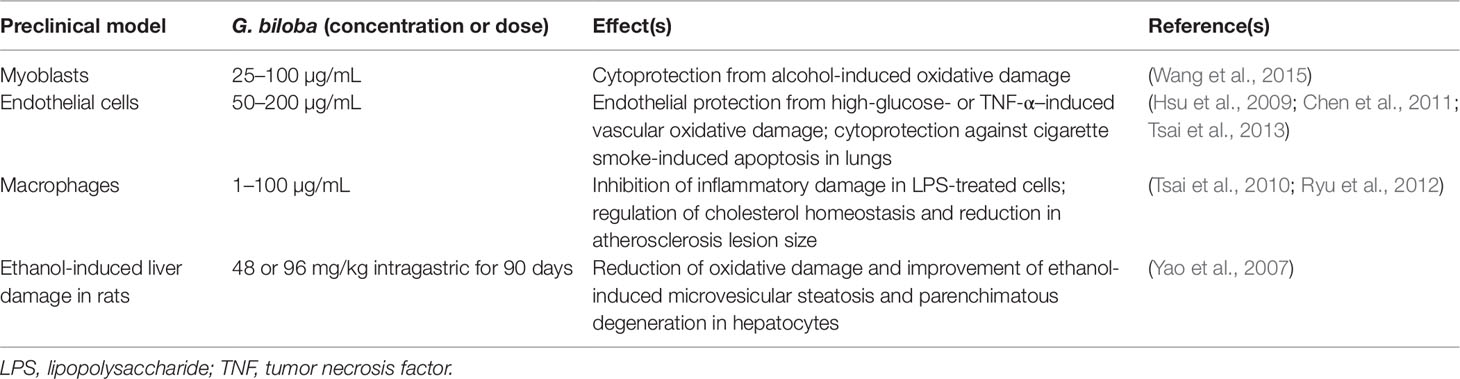
Table 3 Contribution of HO-1 up-regulation to the biological effects of Ginkgo biloba in preclinical in vitro and in vivo models.
Through the induction of HO-1, ginkgolides A, B, and C [1, 3, and 10 mg/kg by intravenous route (i.v.)] decreased neurological deficits and brain infarct volume in rats exposed to ischemia/reperfusion damage (Zhang et al., 2018). In a similar experimental setting the G. biloba extract EGb 761 [containing flavonol-glycosides (24%), ginkgolides A, B, and C (2.8–3.4%), and bilobalide (2.6–3.2%)] at the dosage of 100 mg/kg per os for 7 days before or 4-24 h after damage reduced cortical infarct volume and stimulated the proliferation of neuronal stem/progenitor cells via HO-1 overexpression in mice with permanent middle cerebral artery occlusion (Shah et al., 2011; Nada and Shah, 2012; Nada et al., 2014). The same research group confirmed the neuroprotective effects of G. biloba in the hippocampus of mice pre-treated with EGb 761 (100 mg/kg per os for 7 days) and then susceptible to bilateral common carotid artery occlusion (Tulsulkar and Shah, 2013). The contribution of HO-1 to the neuroprotective effects of EGb 761 (100 mg/kg per os) was also demonstrated in HO-1 knockout male mice with middle cerebral artery occlusion (Saleem et al., 2008). Ginkgolide B (1–50 µM or 10 mg/kg i.p.) prevented cisplatin-induced damage in HEI-OC1 auditory cells and rats through HO-1 induction (Ma et al., 2015). Interestingly, in order to improve brain penetration, G. biloba has been complexed with phosphatidylcholine (Carini et al., 2001); this novel formulation exhibited neuroprotective effects by increasing catalase, superoxide dismutase, glutathione peroxidase, and glutathione reductase activities in rat brain (Naik et al., 2006). Regrettably, there are no studies addressing the potential role of this novel formulation on HO-1. Worth mentioning is the nootropic effects of a novel formulation of G. biloba (120 mg/day per os) complexed with phosphatidylserine administered to healthy volunteers for 7 days (Kennedy et al., 2007).
As far as neurodegenerative disorders, no evidence has been found in literature dealing with the involvement of HO-1 and/or BVR in G. biloba-related neuroprotection. Indeed, the neuroprotective effects of EGb 761 have been extensively reported in both AD (Augustin et al., 2009; Liu et al., 2015; Wan et al., 2016) or PD rodent models (Kim et al., 2004; Rojas et al., 2012; El-Ghazaly et al., 2015) and in humans. Over the last 2–3 years, some retrospective analyses, meta-analyses, and systematic reviews on the neurotherapeutic effects of G. biloba extracts in subjects with dementia, including AD, have been published and the conclusions are the following: (i) over 12 months treatment, EGb 761 and donepezil showed similar effects in cognitive decline in patients aged 80 years or older affected by AD (Rapp et al., 2018); (ii) EGb 761 (240 mg/day per os for 22–24 weeks) had a better performance than placebo in 1,598 patients with dementia (probable AD with or without cerebrovascular disease and probable vascular dementia) (Savaskan et al., 2018); (iii) doses lower than 200 mg/day did not have any remarkable clinical effects in demented people (Yuan et al., 2017) and (iv) G. biloba extract (240 mg/day per os) also showed neuroprotective effects in subjects with mild cognitive impairment (Zhang et al., 2016; Kandiah et al., 2019). Unfortunately, there are no clinical studies on the neuroprotective effects of G. biloba in PD patients, therefore no conclusions can be drawn.
Although the safety profile of G. biloba extracts is acceptable, few mild adverse effects, including mild gastrointestinal complaints, headaches, and allergic reactions have been reported (Kleijnen and Knipschild, 1992). However, important interactions between G. biloba and common drugs should be underlined. G. biloba is an inducer of CYP2C19 and, through this mechanism, it has been shown to reduce omeprazole plasma levels in individuals sharing the poor metabolizer phenotype (Yin et al., 2004) and to increase metabolism and reduce plasma concentrations of the antiepileptic drugs, valproic acid, and phenytoin, thus increasing the risk of fatal seizures (Kupiec and Raj, 2005). A greater risk of bleeding has been reported in people taking G. biloba and aspirin or warfarin (Agbabiaka et al., 2017). G. biloba has been discouraged in people taking selective serotonin-reuptake inhibitors for the increased risk of developing serotonin syndrome (Kreijkamp-Kaspers et al., 2015). Lastly, ginkgo flavonol-glycosides caused a coma in an 80-year-old AD patient taking trazodone, probably by stimulating the CYP3A4 activity which increases the transformation of trazodone into the active metabolite 1-(m-chlorophenyl) piperazine and up-regulates GABAergic activity in the brain (Galluzzi et al., 2000).
Resveratrol
Resveratrol (3,5,4’-trihydroxy-trans-stilbene, (Figure 3) is a phytoalexin found in grapes, cranberries, peanuts, and some beverages (Mancuso et al., 2007). However, wine is considered the main source of resveratrol since the solubility of the latter in ethanol is about 1,667-times higher than that in water (Weiskirchen and Weiskirchen, 2016). Also based on the interest aroused by the so-called “French paradox” over the last few years, the interaction resveratrol-HO-1 has been extensively studied for its beneficial effects in several preclinical models of disease (Table 4).
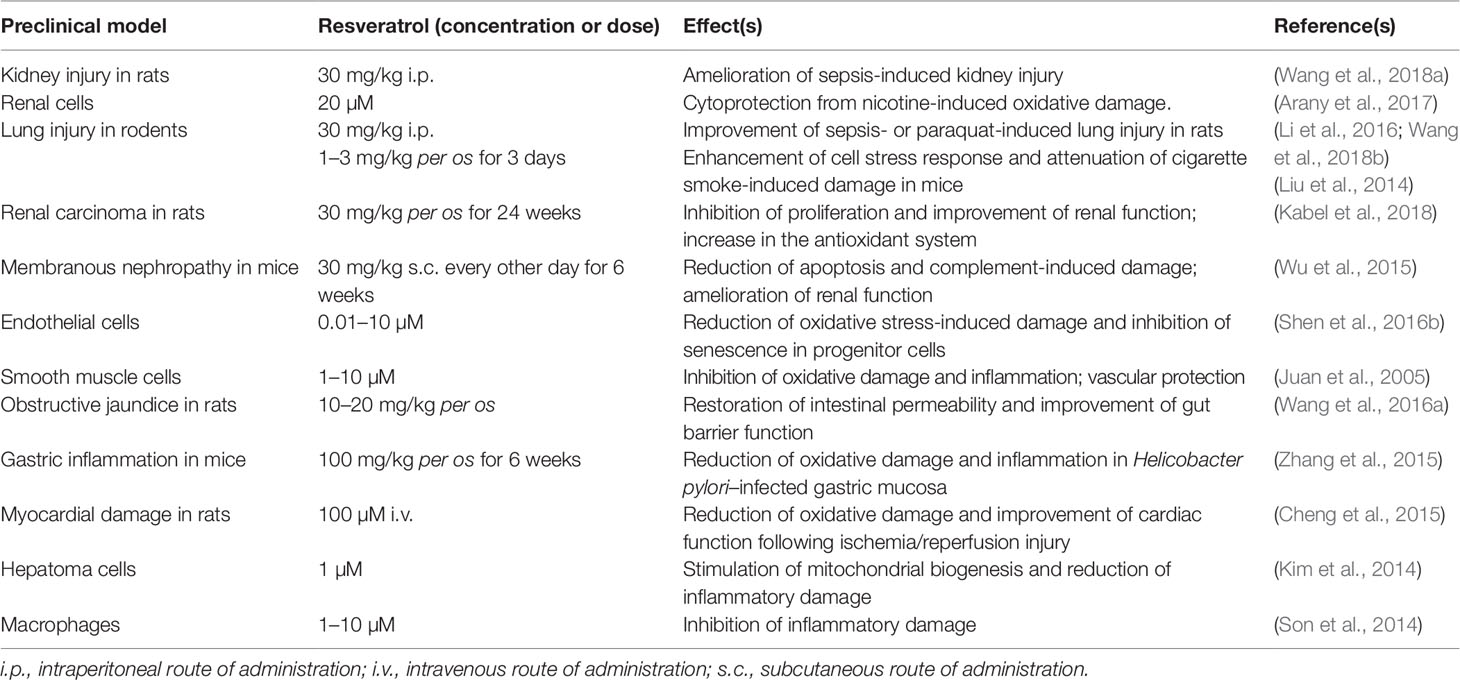
Table 4 Contribution of HO-1 up-regulation to the biological effects of resveratrol in preclinical in vitro and in vivo models.
With regard to the neuroprotective effects, earlier studies showed that resveratrol (5–100 µM) up-regulates HO-1 in primary cultures of mouse neurons (Zhuang et al., 2003). Resveratrol (15 µM), was also found to increase HO-1 expression in neuron-like PC12 cells through both phosphoinositide 3-kinase and MEK1/2 activities (Chen et al., 2005). Quite recently, resveratrol (10 µM) has exhibited a marked protective effect on primary rat oligodendrocyte progenitor cells exposed to lipopolysaccharide through the modulation of Nrf2/HO-1 pathway and the enhancement of cell stress response (Rosa et al., 2018). Neuroprotective effects, due to HO-1 up-regulation, have also been shown in C6 astroglial cells and in hippocampal primary rat astrocytes treated with 100 µM resveratrol and then exposed to neurotoxicants, such as buthionine sulfoximine, azide, or ammonia, for 3–24 h (Bobermin et al., 2015; Bellaver et al., 2016; Arus et al., 2017).
Resveratrol (20–40 mg/kg i.p. for 7 days) significantly enhanced HO-1 expression and prevented both cerebral edema and infarction in seven-day-old rat pups exposed to hypoxic/ischemic injury secondary to unilateral carotid artery ligation (Gao et al., 2018). Similar results have been obtained in adult male rats treated with resveratrol (15–30 mg/kg i.p. for 7 days) and undergoing middle cerebral artery occlusion; in these animals, HO-1 induction was paralleled by down-regulation of caspase-3 and improvement of neuronal viability (Ren et al., 2011). Resveratrol (1–100 µM) increased HO-1 expression in both rat neural stem cells and primary rat cortical neurons exposed to glucose deprivation/reoxygenation injury (an in vitro experimental model mimicking cerebral artery occlusion and reperfusion injury) along with a strong enhancement of cell stress response and a marked reduction of apoptotic cell death (Shen et al., 2016a; Yang et al., 2018). As far as AD is concerned, resveratrol (10–40 µM) has been shown to counteract Aβ-induced oxidative stress in PC12 cells through the overexpression of Nrf2/HO-1 system (Hui et al., 2018). In Aβ-treated rats, resveratrol [100 µM/5 µL by intracerebroventricular route (i.c.v.)] up-regulated hippocampal HO-1, reduced neuronal death in the same area, and improved spatial memory (Huang et al., 2011). In SH-SY5Y cells exposed to rotenone, resveratrol (10–20 µM) induced HO-1 expression and prevented dopaminergic cell death by autophagy (Lin et al., 2014).
Studies in humans have found that resveratrol (25 mg per os) has a low bioavailability and reaches plasma concentrations of about 40 nM within 2 h from administration (Goldberg et al., 2003; Walle et al., 2004); plasma concentrations up to 2.4 µM are reached if the dose of resveratrol increases up to 1 g (Almeida et al., 2009). For a summary of resveratrol pharmacokinetics see Table 1. In order to improve bioavailability, resveratrol has been complexed with solid lipid nanoparticles (SLN) or gold-conjugated nanoparticles (Park et al., 2016; Yadav et al., 2018). Among these formulations, SLN increased by about 4-times the brain levels of resveratrol, which was able to up-regulate HO-1 and improve cognitive decline in rats with permanent bilateral common carotid artery occlusion (Yadav et al., 2018). Unfortunately, there are no studies in humans on the effective concentrations of resveratrol in the brain and a brain concentration greater than 2.4 µM is unlikely, by considering the plasma concentrations reached in the studies mentioned above and the presence of the blood-brain barrier. This suggests that most of the preclinical studies on the neuroprotective effects of resveratrol cannot be applied to humans. As far as the role of wine as the source of resveratrol is concerned, considering both the annual consumption of red and white wines in France (31.7 vs 11.7 L, respectively) and the amount of resveratrol contained in red and white wines (2 and 0.5 mg/L, respectively), it is possible to calculate an amount of resveratrol “drunk” of about 0.2 mg/day, 5000-times less than the highest daily dosage (1 g/day) claimed to give rise to pharmacological effects (Weiskirchen and Weiskirchen, 2016).
It is worth mentioning the document released by the EFSA regarding the request by the European Commission on the safety of trans-resveratrol as a novel food (Efsa, 2016). In this document, the EFSA established that the 38 clinical studies provided in support of the claim do not lead to any conclusion about the efficacy of resveratrol, at doses up to 5 g/day for either acute (4 days) or chronic administration (4–12 weeks), in the treatment of metabolic diseases or cancer. The EFSA pointed out the heterogeneity of the resveratrol doses, the low number of individuals recruited, the uncontrolled experimental design, and the exploratory character of several studies.
In some clinical studies, patients supplemented with resveratrol reported mild adverse effects, such as diarrhea and hot flushes. As far as the interaction with drugs-metabolizing enzymes is concerned, resveratrol has been shown to inhibit CYP3A4, CYP2D6, and CYP2C9 and to induce CYP1A2 in vitro. No significant changes in plasma levels of common drugs have been reported so far (Efsa, 2016).
Conclusions
In illustrating the interactions between the HO/BVR system with FA, G. biloba, and resveratrol, we have attempted to address some critical points presented in the Introduction, discussing each issue to the best of our knowledge.
An initial consideration concerns the janus face of the HO-1/BVR by-products. As mentioned in the Introduction, both CO and BR have important pleiotropic and neuroprotective effects in the nervous system, but they may become toxic in the case of a disproportionate production or under conditions of redox imbalance (Mancuso et al., 1997; Mancuso et al., 2010; Mancuso, 2017). The potential toxic effect due to an over activation of the HO/BVR system has also been supported by the discovery of the importance of HO-1 gene repression in order to preserve cell homeostasis and integrity (Shibahara, 2003; Shibahara et al., 2003; Palozza et al., 2006). For this reason, the long-lasting induction of HO-1 due to chronic supplementation with herbal products may be a double-edged sword and the possibility of neurotoxicity must be carefully considered.
Regarding the effective dose correlation in vitro and blood or tissue concentrations found in vivo, resveratrol is an indisputable example. Both the bioavailability data through wine consumption and the EFSA opinion on anti-inflammatory efficacy do not justify the turmoil often generated by the media regarding the beneficial properties of resveratrol in red wine. In this regard, the antioxidant, antithrombotic, and metabolic effects of ethanol per se often are not mentioned; moreover, red wines usually contain ethanol in greater concentration than white wines and the beneficial effects claimed for the former can be due, once again, to ethanol (Goldberg et al., 1995; Criqui, 1998; Belleville, 2002). Focusing on the beneficial effects of moderate ethanol consumption would also allow a greater responsibility on the consumers’ part towards their own health, because of the pathological effects of uncontrolled alcohol intake are well known. On the contrary, if attention is focused on resveratrol, of which only the beneficial effects are artificially advertised, the need for a controlled intake of red wine is lost since the attention is driven towards a natural compound.
The risk linked to the effects on drug metabolizing enzymes and the health consequences in case of concomitant drug intake, concepts that are very often neglected also by health professionals, should be emphasized. Particularly dangerous are the interactions between G. biloba and CYP or other phase II enzymes, which could increase the risk of toxicity in patients treated with drugs, such as valproic acid, trazodone, talinolol, warfarin, etc. It is worth mentioning that the lack of reliable data on the neuroprotective effects of resveratrol and G. biloba challenges their potential beneficial effects in the treatment of neurodegenerative diseases. In this regard, more attention is required for FA for which, to our knowledge, there are no clinical studies confirming the promising neuroprotective results obtained on preclinical models. The few clinical studies available, addressing the kinetics in most cases, have been performed using foods rich in FA and this does not always allow to ascertain the actual dose administered and any confounding effects related to other active compounds present. Actually, only one randomized and double-blind study on hyperlipidemic patients (Bumrungpert et al., 2018) has appeared in the literature and shows the hypolipemic, anti-inflammatory, and antioxidant effects of FA (1 g/day per os for 6 weeks).
These considerations lead to the conclusion that there is not sufficient evidence on the efficacy of these herbal products in neurodegenerative diseases and that further efforts and many attempts are recommended and requested by doctors, researchers, and other health professionals to bridge this gap for the benefit of patients and their families.
Author Contributions
Conceptualization: CM, VC, LT. Data curation: CM, EM. Writing–original draft preparation: CM, EM. Writing–review and editing: CM, EM, VC, LT.
Conflict of Interest
The authors declare that the research was conducted in the absence of any commercial or financial relationships that could be construed as a potential conflict of interest.
Acknowledgments
This work was supported by Università Cattolica del Sacro Cuore grant “Fondi Ateneo” to CM.
References
Agbabiaka, T. B., Wider, B., Watson, L. K., Goodman, C. (2017). Concurrent use of prescription drugs and herbal medicinal products in older adults: a systematic review. Drugs Aging 34, 891–905. doi: 10.1007/s40266-017-0501-7
Almeida, L., Vaz-Da-Silva, M., Falcao, A., Soares, E., Costa, R., Loureiro, A. I., et al. (2009). Pharmacokinetic and safety profile of trans-resveratrol in a rising multiple-dose study in healthy volunteers. Mol. Nutr. Food Res. 53 Suppl 1, S7–15. doi: 10.1002/mnfr.200800177
Arany, I., Hall, S., Faisal, A., Dixit, M. (2017). Nicotine exposure augments renal toxicity of 5-aza-cytidine through p66shc: prevention by resveratrol. Anticancer Res. 37, 4075–4079. doi: 10.21873/anticanres.11793
Arus, B. A., Souza, D. G., Bellaver, B., Souza, D. O., Goncalves, C. A., Quincozes-Santos, A., et al. (2017). Resveratrol modulates GSH system in C6 astroglial cells through heme oxygenase 1 pathway. Mol. Cell Biochem. 428, 67–77. doi: 10.1007/s11010-016-2917-5
Augustin, S., Rimbach, G., Augustin, K., Schliebs, R., Wolffram, S., Cermak, R. (2009). Effect of a short- and long-term treatment with Ginkgo biloba extract on amyloid precursor protein levels in a transgenic mouse model relevant to Alzheimer’s disease. Arch. Biochem. Biophys. 481, 177–182. doi: 10.1016/j.abb.2008.10.032
Bami, E., Ozakpinar, O. B., Ozdemir-Kumral, Z. N., Koroglu, K., Ercan, F., Cirakli, Z., et al. (2017). Protective effect of ferulic acid on cisplatin induced nephrotoxicity in rats. Environ. Toxicol. Pharmacol. 54, 105–111. doi: 10.1016/j.etap.2017.06.026
Barone, E., Calabrese, V., Mancuso, C. (2009a). Ferulic acid and its therapeutic potential as a hormetin for age-related diseases. Biogerontology 10, 97–108. doi: 10.1007/s10522-008-9160-8
Barone, E., Trombino, S., Cassano, R., Sgambato, A., De Paola, B., Di Stasio, E., et al. (2009b). Characterization of the S-denitrosylating activity of bilirubin. J. Cell Mol. Med. 13, 2365–2375. doi: 10.1111/j.1582-4934.2009.00680.x
Bellaver, B., Bobermin, L. D., Souza, D. G., Rodrigues, M. D., De Assis, A. M., Wajner, M., et al. (2016). Signaling mechanisms underlying the glioprotective effects of resveratrol against mitochondrial dysfunction. Biochim. Biophys. Acta 1862, 1827–1838. doi: 10.1016/j.bbadis.2016.06.018
Belleville, J. (2002). The French paradox: possible involvement of ethanol in the protective effect against cardiovascular diseases. Nutrition 18, 173–177. doi: 10.1016/S0899-9007(01)00721-3
Bobermin, L. D., Hansel, G., Scherer, E. B., Wyse, A. T., Souza, D. O., Quincozes-Santos, A., et al. (2015). Ammonia impairs glutamatergic communication in astroglial cells: protective role of resveratrol. Toxicol. In Vitro 29, 2022–2029. doi: 10.1016/j.tiv.2015.08.008
Bumrungpert, A., Lilitchan, S., Tuntipopipat, S., Tirawanchai, N., Komindr, S. (2018). Acid supplementation improves lipid profiles, oxidative stress, and inflammatorystatus in hyperlipidemic subjects: a randomized, double-blind, placebo-controlledClin. Trial Nutrients. Nutrients 10. (6), e713 doi: 10.3390/nu10060713
Calabrese, V., Calafato, S., Puleo, E., Cornelius, C., Sapienza, M., Morganti, P., et al. (2008). Redox regulation of cellular stress response by ferulic acid ethyl ester in human dermal fibroblasts: role of vitagenes. Clin. Dermatol. 26, 358–363. doi: 10.1016/j.clindermatol.2008.01.005
Carini, M., Aldini, G., Rossoni, G., Morazzoni, P., Facino, R. M. (2001). Complexation of Ginkgo biloba extract with phosphatidylcholine improves cardioprotective activity and increases the plasma antioxidant capacity in the rat. Planta Med. 67, 326–330. doi: 10.1055/s-2001-14313
Catino, S., Paciello, F., Miceli, F., Rolesi, R., Troiani, D., Calabrese, V., et al. (2016). Ferulic acid regulates the Nrf2/Heme oxygenase-1 system and counteracts trimethyltin-induced neuronal damage in the human neuroblastoma cell line SH-SY5Y. Front. Pharmacol. 6, 305. doi: 10.3389/fphar.2015.00305
Chen, C. Y., Jang, J. H., Li, M. H., Surh, Y. J. (2005). Resveratrol upregulates heme oxygenase-1 expression via activation of NF-E2-related factor 2 in PC12 cells. Biochem. Biophys. Res. Commun. 331, 993–1000. doi: 10.1016/j.bbrc.2005.03.237
Chen, J. S., Huang, P. H., Wang, C. H., Lin, F. Y., Tsai, H. Y., Wu, T. C., et al. (2011). Nrf-2 mediated heme oxygenase-1 expression, an antioxidant-independent mechanism, contributes to anti-atherogenesis and vascular protective effects of Ginkgo biloba extract. Atherosclerosis 214, 301–309. doi: 10.1016/j.atherosclerosis.2010.11.010
Cheng, L., Jin, Z., Zhao, R., Ren, K., Deng, C., Yu, S. (2015). Resveratrol attenuates inflammation and oxidative stress induced by myocardial ischemia-reperfusion injury: role of Nrf2/ARE pathway. Int. J. Clin. Exp. Med. 8, 10420–10428.
Choi, Y. K. (2018) Role of Carbon Monoxide in Neurovascular Repair Processing. Biomol. Ther. (Seoul) 26, 93–100. doi: 10.4062/biomolther.2017.144
Criqui, M. H. (1998). Do known cardiovascular risk factors mediate the effect of alcohol on cardiovascular disease? Novartis Found Symp. 216, 159–172. doi: 10.1002/9780470515549.ch10
Das, U., Sengupta, A., Biswas, S., Adhikary, A., Dey Sharma, R., Chakraborty, A., et al. (2017). Alteration of murine duodenal morphology and redox signalling events by reactive oxygen species generated after whole body gamma-irradiation and its prevention by ferulic acid. Free Radic. Res. 51, 886–910. doi: 10.1080/10715762.2017.1388916
Di Domenico, F., Perluigi, M., Foppoli, C., Blarzino, C., Coccia, R., De Marco, F., et al. (2009). Protective effect of ferulic acid ethyl ester against oxidative stress mediated by UVB irradiation in human epidermal melanocytes. Free Radic. Res. 43, 365–375. doi: 10.1080/10715760902777329
EFSA (2016). Safety of synthetic trans-resveratrol as a novel food pursuant to Regulation (EC) No 258/97. EFSA J. 14, 4368. doi: 10.2903/j.efsa.2016.4368
El-Ghazaly, M. A., Sadik, N. A., Rashed, E. R., Abd-El-Fattah, A. A. (2015). Neuroprotective effect of EGb761(R) and low-dose whole-body gamma-irradiation in a rat model of Parkinson’s disease. Toxicol. Ind. Health 31, 1128–1143. doi: 10.1177/0748233713487251
Fetoni, A. R., Mancuso, C., Eramo, S. L., Ralli, M., Piacentini, R., Barone, E., et al. (2010). In vivo protective effect of ferulic acid against noise-induced hearing loss in the guinea-pig. Neuroscience 169, 1575–1588. doi: 10.1016/j.neuroscience.2010.06.022
Fetoni, A. R., Paciello, F., Rolesi, R., Eramo, S. L., Mancuso, C., Troiani, D., et al. (2015). Rosmarinic acid up-regulates the noise-activated Nrf2/HO-1 pathway and protects against noise-induced injury in rat cochlea. Free Radic. Biol. Med. 85, 269–281. doi: 10.1016/j.freeradbiomed.2015.04.021
Galluzzi, S., Zanetti, O., Binetti, G., Trabucchi, M., Frisoni, G. B. (2000). Coma in a patient with Alzheimer’s disease taking low dose trazodone and gingko biloba. J. Neurol. Neurosurg. Psychiatry 68, 679–680. doi: 10.1136/jnnp.68.5.679a
Gao, Y., Fu, R., Wang, J., Yang, X., Wen, L., Feng, J. (2018). Resveratrol mitigates the oxidative stress mediated by hypoxic-ischemic brain injury in neonatal rats via Nrf2/HO-1 pathway. Pharm. Biol. 56, 440–449. doi: 10.1080/13880209.2018.1502326
Gibbs, P. E., Miralem, T., Maines, M. D. (2015). Biliverdin reductase: a target for cancer therapy? Front. Pharmacol. 6, 119. doi: 10.3389/fphar.2015.00119
Goldberg, D. M., Hahn, S. E., Parkes, J. G. (1995). Beyond alcohol: beverage consumption and cardiovascular mortality. Clin. Chim. Acta 237, 155–187. doi: 10.1016/0009-8981(95)06069-P
Goldberg, D. M., Yan, J., Soleas, G. J. (2003). Absorption of three wine-related polyphenols in three different matrices by healthy subjects. Clin. Biochem. 36, 79–87. doi: 10.1016/S0009-9120(02)00397-1
Hsu, C. L., Wu, Y. L., Tang, G. J., Lee, T. S., Kou, Y. R. (2009). Ginkgo biloba extract confers protection from cigarette smoke extract-induced apoptosis in human lung endothelial cells: Role of heme oxygenase-1. Pulm. Pharmacol. Ther. 22, 286–296. doi: 10.1016/j.pupt.2009.02.003
Huang, T. C., Lu, K. T., Wo, Y. Y., Wu, Y. J., Yang, Y. L. (2011). Resveratrol protects rats from Abeta-induced neurotoxicity by the reduction of iNOS expression and lipid peroxidation. PLoS One 6, e29102. doi: 10.1371/journal.pone.0029102
Huang, W. Y., Chao, X. J., Ouyang, Y., Liu, A. M., He, X. X., Chen, M. H., et al. (2012). Tacrine-6-ferulic acid, a novel multifunctional dimer against Alzheimer’s disease, prevents oxidative stress-induced neuronal death through activating Nrf2/ARE/HO-1 pathway in HT22 cells. CNS Neurosci. Ther. 18, 950–951. doi: 10.1111/cns.12010
Hui, Y., Chengyong, T., Cheng, L., Haixia, H., Yuanda, Z., Weihua, Y. (2018). Resveratrol attenuates the cytotoxicity induced by amyloid-beta1-42 in PC12 cells by upregulating heme oxygenase-1 via the PI3K/Akt/Nrf2 Pathway. Neurochem. Res. 43, 297–305. doi: 10.1007/s11064-017-2421-7
Igarashi, K., Sun, J. (2006). The heme-Bach1 pathway in the regulation of oxidative stress response and erythroid differentiation. Antioxid. Redox Signal 8, 107–118. doi: 10.1089/ars.2006.8.107
Joshi, G., Perluigi, M., Sultana, R., Agrippino, R., Calabrese, V., Butterfield, D. A. (2006). In vivo protection of synaptosomes by ferulic acid ethyl ester (FAEE) from oxidative stress mediated by 2,2-azobis(2-amidino-propane)dihydrochloride (AAPH) or Fe(2+)/H(2)O(2): insight into mechanisms of neuroprotection and relevance to oxidative stress-related neurodegenerative disorders. Neurochem. Int. 48, 318–327. doi: 10.1016/j.neuint.2005.11.006
Juan, S. H., Cheng, T. H., Lin, H. C., Chu, Y. L., Lee, W. S. (2005). Mechanism of concentration-dependent induction of heme oxygenase-1 by resveratrol in human aortic smooth muscle cells. Biochem. Pharmacol. 69, 41–48. doi: 10.1016/j.bcp.2004.09.015
Kabel, A. M., Atef, A., Estfanous, R. S. (2018). Ameliorative potential of sitagliptin and/or resveratrol on experimentally-induced clear cell renal cell carcinoma. Biomed. Pharmacother. 97, 667–674. doi: 10.1016/j.biopha.2017.10.149
Kandiah, N., Ong, P. A., Yuda, T., Ng, L. L., Mamun, K., Merchant, R. A., et al. (2019). Treatment of dementia and mild cognitive impairment with or without cerebrovascular disease: Expert consensus on the use of Ginkgo biloba extract, EGb 761((R)). CNS Neurosci. Ther. 25, 288–298. doi: 10.1111/cns.13095
Kanski, J., Aksenova, M., Stoyanova, A., Butterfield, D. A. (2002). Ferulic acid antioxidant protection against hydroxyl and peroxyl radical oxidation in synaptosomal and neuronal cell culture systems in vitro: structure-activity studies. J. Nutr. Biochem. 13, 273–281. doi: 10.1016/S0955-2863(01)00215-7
Kapitulnik, J., Maines, M. D. (2009). Pleiotropic functions of biliverdin reductase: cellular signaling and generation of cytoprotective and cytotoxic bilirubin. Trends Pharmacol. Sci. 30, 129–137. doi: 10.1016/j.tips.2008.12.003
Kennedy, D. O., Haskell, C. F., Mauri, P. L., Scholey, A. B. (2007). Acute cognitive effects of standardised Ginkgo biloba extract complexed with phosphatidylserine. Hum. Psychopharmacol. 22, 199–210. doi: 10.1002/hup.837
Kim, M. S., Lee, J. I., Lee, W. Y., Kim, S. E. (2004). Neuroprotective effect of Ginkgo biloba L. extract in a rat model of Parkinson’s disease. Phytother. Res. 18, 663–666. doi: 10.1002/ptr.1486
Kim, S. K., Joe, Y., Zheng, M., Kim, H. J., Yu, J. K., Cho, G. J., et al. (2014). Resveratrol induces hepatic mitochondrial biogenesis through the sequential activation of nitric oxide and carbon monoxide production. Antioxid. Redox Signal 20, 2589–2605. doi: 10.1089/ars.2012.5138
Kleijnen, J., Knipschild, P. (1992). Ginkgo biloba. Lancet 340, 1136–1139. doi: 10.1016/0140-6736(92)93158-J
Koh, E. J., Kim, K. J., Seo, Y. J., Choi, J., Lee, B. Y. (2017). Modulation of HO-1 by ferulic acid attenuates adipocyte differentiation in 3T3-L1 Cells. Mol. 22 (5), e745 doi: 10.3390/molecules22050745
Kreijkamp-Kaspers, S., Mcguire, T., Bedford, S., Loadsman, P., Pirotta, M., Moses, G., et al. (2015). Your questions about complementary medicines answered: gingko biloba. Aust. Fam. Physician 44, 565–566.
Kupiec, T., Raj, V. (2005). Fatal seizures due to potential herb-drug interactions with Ginkgo biloba. J. Anal. Toxicol. 29, 755–758. doi: 10.1093/jat/29.7.755
Li, S., Zhao, G., Chen, L., Ding, Y., Lian, J., Hong, G., et al. (2016). Resveratrol protects mice from paraquat-induced lung injury: The important role of SIRT1 and NRF2 antioxidant pathways. Mol. Med. Rep. 13, 1833–1838. doi: 10.3892/mmr.2015.4710
Lin, T. K., Chen, S. D., Chuang, Y. C., Lin, H. Y., Huang, C. R., Chuang, J. H., et al. (2014). Resveratrol partially prevents rotenone-induced neurotoxicity in dopaminergic SH-SY5Y cells through induction of heme oxygenase-1 dependent autophagy. Int. J. Mol. Sci. 15, 1625–1646. doi: 10.3390/ijms15011625
Liu, H., Ren, J., Chen, H., Huang, Y., Li, H., Zhang, Z., et al. (2014). Resveratrol protects against cigarette smoke-induced oxidative damage and pulmonary inflammation. J. Biochem. Mol. Toxicol. 28, 465–471. doi: 10.1002/jbt.21586
Liu, X., Hao, W., Qin, Y., Decker, Y., Wang, X., Burkart, M., et al. (2015). Long-term treatment with Ginkgo biloba extract EGb 761 improves symptoms and pathology in a transgenic mouse model of Alzheimer’s disease. Brain Behav. Immun. 46, 121–131. doi: 10.1016/j.bbi.2015.01.011
Lupp, A., Appenroth, D., Fang, L., Decker, M., Lehmann, J., Fleck, C. (2010). Tacrine-NO donor and tacrine-ferulic acid hybrid molecules as new anti-Alzheimer agents: hepatotoxicity and influence on the cytochrome P450 system in comparison to tacrine. Arzneimittelforschung 60, 229–237.
Ma, W., Hu, J., Cheng, Y., Wang, J., Zhang, X., Xu, M. (2015). Ginkgolide B protects against cisplatin-induced ototoxicity: enhancement of Akt-Nrf2-HO-1 signaling and reduction of NADPH oxidase. Cancer Chemother. Pharmacol. 75, 949–959. doi: 10.1007/s00280-015-2716-9
Ma, Z. C., Hong, Q., Wang, Y. G., Liang, Q. D., Tan, H. L., Xiao, C. R., et al. (2011). Ferulic acid induces heme oxygenase-1 via activation of ERK and Nrf2. Drug Discov. Ther. 5, 299–305. doi: 10.5582/ddt.2011.v5.6.299
Ma, Z. C., Hong, Q., Wang, Y. G., Tan, H. L., Xiao, C. R., Liang, Q. D., et al. (2010). Ferulic acid protects human umbilical vein endothelial cells from radiation induced oxidative stress by phosphatidylinositol 3-kinase and extracellular signal-regulated kinase pathways. Biol. Pharm. Bull. 33, 29–34. doi: 10.1248/bpb.33.29
Maines, M. D. (1988). Heme oxygenase: function, multiplicity, regulatory mechanisms, and clinical applications. FASEB J. 2, 2557–2568. doi: 10.1096/fasebj.2.10.3290025
Maines, M. D. (1997). The heme oxygenase system: a regulator of second messenger gases. Annu. Rev. Pharmacol. Toxicol. 37, 517–554. doi: 10.1146/annurev.pharmtox.37.1.517
Maines, M. D. (2005). New insights into biliverdin reductase functions: linking heme metabolism to cell signaling. Physiology (Bethesda) 20, 382–389. doi: 10.1152/physiol.00029.2005
Maines, M. D., Gibbs, P. E. (2005). 30 some years of heme oxygenase: from a “molecular wrecking ball” to a “mesmerizing” trigger of cellular events. Biochem. Biophys. Res. Commun. 338, 568–577. doi: 10.1016/j.bbrc.2005.08.121
Mancuso, C. (2017). Bilirubin and brain: A pharmacological approach. Neuropharmacology 118, 113–123. doi: 10.1016/j.neuropharm.2017.03.013
Mancuso, C., Barone, E., Guido, P., Miceli, F., Di Domenico, F., Perluigi, M., et al. (2012a). Inhibition of lipid peroxidation and protein oxidation by endogenous and exogenous antioxidants in rat brain microsomes in vitro. Neurosci. Lett. 518, 101–105. doi: 10.1016/j.neulet.2012.04.062
Mancuso, C., Bates, T. E., Butterfield, D. A., Calafato, S., Cornelius, C., De Lorenzo, A., et al. (2007). Natural antioxidants in Alzheimer’s disease. Expert Opin. Investig. Drugs 16, 1921–1931. doi: 10.1517/13543784.16.12.1921
Mancuso, C., Bonsignore, A., Capone, C., Di Stasio, E., Pani, G. (2006a). Albumin-bound bilirubin interacts with nitric oxide by a redox mechanism. Antioxid. Redox Signal 8, 487–494. doi: 10.1089/ars.2006.8.487
Mancuso, C., Capone, C., Ranieri, S. C., Fusco, S., Calabrese, V., Eboli, M. L., et al. (2008). Bilirubin as an endogenous modulator of neurotrophin redox signaling. J. Neurosci. Res. 86, 2235–2249. doi: 10.1002/jnr.21665
Mancuso, C., Navarra, P., Preziosi, P. (2010). Roles of nitric oxide, carbon monoxide, and hydrogen sulfide in the regulation of the hypothalamic-pituitary-adrenal axis. J. Neurochem. 113, 563–575. doi: 10.1111/j.1471-4159.2010.06606.x
Mancuso, C., Pani, G., Calabrese, V. (2006b). Bilirubin: an endogenous scavenger of nitric oxide and reactive nitrogen species. Redox Rep. 11, 207–213. doi: 10.1179/135100006X154978
Mancuso, C., Perluigi, M., Cini, C., De Marco, C., Giuffrida Stella, A. M., Calabrese, V. (2006c). Heme oxygenase and cyclooxygenase in the central nervous system: a functional interplay. J. Neurosci. Res. 84, 1385–1391. doi: 10.1002/jnr.21049
Mancuso, C., Preziosi, P., Grossman, A. B., Navarra, P. (1997). The role of carbon monoxide in the regulation of neuroendocrine function. Neuroimmunomodulation 4, 225–229. doi: 10.1159/000097340
Mancuso, C., Santangelo, R. (2014). Ferulic acid: pharmacological and toxicological aspects. Food Chem. Toxicol. 65, 185–195. doi: 10.1016/j.fct.2013.12.024
Mancuso, C., Santangelo, R. (2017). Panax ginseng and Panax quinquefolius: From pharmacology to toxicology. Food Chem. Toxicol. 107, 362–372. doi: 10.1016/j.fct.2017.07.019
Mancuso, C., Siciliano, R., Barone, E., Preziosi, P. (2012b). Natural substances and Alzheimer’s disease: from preclinical studies to evidence based medicine. Biochim. Biophys. Acta 1822, 616–624. doi:10.1016/j.bbadis.2011.09.004
Mancuso, C., Tringali, G., Grossman, A., Preziosi, P., Navarra, P. (1998). The generation of nitric oxide and carbon monoxide produces opposite effects on the release of immunoreactive interleukin-1beta from the rat hypothalamus in vitro: evidence for the involvement of different signaling pathways. Endocrinology 139, 1031–1037. doi: 10.1210/endo.139.3.5822
Mhillaj, E., Catino, S., Miceli, F. M., Santangelo, R., Trabace, L., Cuomo, V., et al. (2018). Ferulic acid improves cognitive skills through the activation of the heme oxygenase system in the rat. Mol. Neurobiol. 55, 905–916. doi: 10.1007/s12035-017-0381-1
Nada, S. E., Shah, Z. A. (2012). Preconditioning with Ginkgo biloba (EGb 761(R)) provides neuroprotection through HO1 and CRMP2. Neurobiol. Dis. 46, 180–189. doi: 10.1016/j.nbd.2012.01.006
Nada, S. E., Tulsulkar, J., Shah, Z. A. (2014). Heme oxygenase 1-mediated neurogenesis is enhanced by Ginkgo biloba (EGb 761(R)) after permanent ischemic stroke in mice. Mol. Neurobiol. 49, 945–956. doi: 10.1007/s12035-013-8572-x
Naik, S. R., Pilgaonkar, V. W., Panda, V. S. (2006). Evaluation of antioxidant activity of Ginkgo biloba phytosomes in rat brain. Phytother. Res. 20, 1013–1016. doi: 10.1002/ptr.1976
Palozza, P., Serini, S., Curro, D., Calviello, G., Igarashi, K., Mancuso, C. (2006). beta-Carotene and cigarette smoke condensate regulate heme oxygenase-1 and its repressor factor Bach1: relationship with cell growth. Antioxid. Redox Signal 8, 1069–1080. doi: 10.1089/ars.2006.8.1069
Park, S. Y., Chae, S. Y., Park, J. O., Lee, K. J., Park, G. (2016). Gold-conjugated resveratrol nanoparticles attenuate the invasion and MMP-9 and COX-2 expression in breast cancer cells. Oncol. Rep. 35, 3248–3256. doi: 10.3892/or.2016.4716
Pi, R., Mao, X., Chao, X., Cheng, Z., Liu, M., Duan, X., et al. (2012). Tacrine-6-ferulic acid, a novel multifunctional dimer, inhibits amyloid-beta-mediated Alzheimer’s disease-associated pathogenesis in vitro and in vivo. PLoS One 7, e31921. doi: 10.1371/journal.pone.0031921
Rapp, M., Burkart, M., Kohlmann, T., Bohlken, J. (2018). Similar treatment outcomes with Ginkgo biloba extract EGb 761 and donepezil in Alzheimer’s dementia in very old age: a retrospective observational study. Int. J. Clin. Pharmacol. Ther. 56, 130–133. doi: 10.5414/CP203103
Ren, J., Fan, C., Chen, N., Huang, J., Yang, Q. (2011). Resveratrol pretreatment attenuates cerebral ischemic injury by upregulating expression of transcription factor Nrf2 and HO-1 in rats. Neurochem. Res. 36, 2352–2362. doi: 10.1007/s11064-011-0561-8
Rojas, P., Montes, P., Rojas, C., Serrano-Garcia, N., Rojas-Castaneda, J. C. (2012). Effect of a phytopharmaceutical medicine, Ginko biloba extract 761, in an animal model of Parkinson’s disease: therapeutic perspectives. Nutrition 28, 1081–1088. doi: 10.1016/j.nut.2012.03.007
Rosa, P. M., Martins, L.a.M., Souza, D. O., Quincozes-Santos, A. (2018). Glioprotective Effect of Resveratrol: an emerging therapeutic role for oligodendroglial cells. Mol. Neurobiol. 55, 2967–2978. doi: 10.1007/s12035-017-0510-x
Ryu, E. Y., Park, A. J., Park, S. Y., Park, S. H., Eom, H. W., Kim, Y. H., et al. (2012). Inhibitory effects of Ginkgo biloba extract on inflammatory mediator production by Porphyromonas gingivalis lipopolysaccharide in murine macrophages via Nrf-2 mediated heme oxygenase-1 signaling pathways. Inflammation 35, 1477–1486. doi: 10.1007/s10753-012-9461-6
Saleem, S., Zhuang, H., Biswal, S., Christen, Y., Dore, S. (2008). Ginkgo biloba extract neuroprotective action is dependent on heme oxygenase 1 in ischemic reperfusion brain injury. Stroke 39, 3389–3396. doi: 10.1161/STROKEAHA.108.523480
Savaskan, E., Mueller, H., Hoerr, R., Von Gunten, A., Gauthier, S. (2018). Treatment effects of Ginkgo biloba extract EGb 761(R) on the spectrum of behavioral and psychological symptoms of dementia: meta-analysis of randomized controlled trials. Int. Psychogeriatr. 30, 285–293. doi: 10.1017/S1041610217001892
Scapagnini, G., Butterfield, D. A., Colombrita, C., Sultana, R., Pascale, A., Calabrese, V. (2004). Ethyl ferulate, a lipophilic polyphenol, induces HO-1 and protects rat neurons against oxidative stress. Antioxid. Redox Signal 6, 811–818. doi: 10.1089/1523086041798079
Scapagnini, G., Foresti, R., Calabrese, V., Giuffrida Stella, A. M., Green, C. J., Motterlini, R. (2002). Caffeic acid phenethyl ester and curcumin: a novel class of heme oxygenase-1 inducers. Mol. Pharmacol. 61, 554–561. doi: 10.1124/mol.61.3.554
Schipper, H. M. (2004). Heme oxygenase-1: transducer of pathological brain iron sequestration under oxidative stress. Ann. N. Y. Acad. Sci. 1012, 84–93. doi: 10.1196/annals.1306.007
Schipper, H. M., Song, W., Tavitian, A., Cressatti, M. (2019). The sinister face of heme oxygenase-1 in brain aging and disease. Prog. Neurobiol. 172, 40–70. doi: 10.1016/j.pneurobio.2018.06.008
Schipper, H. M., Song, W., Zukor, H., Hascalovici, J. R., Zeligman, D. (2009). Heme oxygenase-1 and neurodegeneration: expanding frontiers of engagement. J. Neurochem. 110, 469–485. doi: 10.1111/j.1471-4159.2009.06160.x
Shah, Z. A., Nada, S. E., Dore, S. (2011). Heme oxygenase 1, beneficial role in permanent ischemic stroke and in Gingko biloba (EGb 761) neuroprotection. Neuroscience 180, 248–255. doi: 10.1016/j.neuroscience.2011.02.031
Shen, C., Cheng, W., Yu, P., Wang, L., Zhou, L., Zeng, L., et al. (2016a). Resveratrol pretreatment attenuates injury and promotes proliferation of neural stem cells following oxygen-glucose deprivation/reoxygenation by upregulating the expression of Nrf2, HO-1 and NQO1 in vitro. Mol. Med. Rep. 14, 3646–3654. doi: 10.3892/mmr.2016.5670
Shen, X., Wang, M., Bi, X., Zhang, J., Wen, S., Fu, G., et al. (2016b). Resveratrol prevents endothelial progenitor cells from senescence and reduces the oxidative reaction via PPARgamma/HO1 pathways. Mol. Med. Rep. 14, 5528–5534. doi: 10.3892/mmr.2016.5929
Shibahara, S. (2003). The heme oxygenase dilemma in cellular homeostasis: new insights for the feedback regulation of heme catabolism. Tohoku J. Exp. Med. 200, 167–186. doi: 10.1620/tjem.200.167
Shibahara, S., Nakayama, M., Kitamuro, T., Udono-Fujimori, R., Takahashi, K. (2003). Repression of heme oxygenase-1 expression as a defense strategy in humans. Exp. Biol. Med. (Maywood) 228, 472–473. doi: 10.1177/15353702-0322805-08
Son, Y., Chung, H. T., Pae, H. O. (2014). Differential effects of resveratrol and its natural analogs, piceatannol and 3,5,4’-trans-trimethoxystilbene, on anti-inflammatory heme oxigenase-1 expression in RAW264.7 macrophages. Biofactors 40, 138–145. doi: 10.1002/biof.1108
Tenhunen, R., Marver, H. S., Schmid, R. (1968). The enzymatic conversion of heme to bilirubin by microsomal heme oxygenase. Proc. Natl. Acad. Sci. U. S. A. 61, 748–755. doi: 10.1073/pnas.61.2.748
Tenhunen, R., Marver, H. S., Schmid, R. (1969). Microsomal heme oxygenase. Characterization of the enzyme. J. Biol. Chem. 244, 6388–6394.
Tsai, H. Y., Huang, P. H., Lin, F. Y., Chen, J. S., Lin, S. J., Chen, J. W. (2013). Ginkgo biloba extract reduces high-glucose-induced endothelial reactive oxygen species generation and cell adhesion molecule expression by enhancing HO-1 expression via Akt/eNOS and p38 MAP kinase pathways. Eur. J. Pharm. Sci. 48, 803–811. doi: 10.1016/j.ejps.2013.01.002
Tsai, J. Y., Su, K. H., Shyue, S. K., Kou, Y. R., Yu, Y. B., Hsiao, S. H., et al. (2010). EGb761 ameliorates the formation of foam cells by regulating the expression of SR-A and ABCA1: role of haem oxygenase-1. Cardiovasc. Res. 88, 415–423. doi: 10.1093/cvr/cvq226
Tulsulkar, J., Shah, Z. A. (2013). Ginkgo biloba prevents transient global ischemia-induced delayed hippocampal neuronal death through antioxidant and anti-inflammatory mechanism. Neurochem. Int. 62, 189–197. doi: 10.1016/j.neuint.2012.11.017
Ude, C., Schubert-Zsilavecz, M., Wurglics, M. (2013). Ginkgo biloba extracts: a review of the pharmacokinetics of the active ingredients. Clin. Pharmacokinet. 52, 727–749. doi: 10.1007/s40262-013-0074-5
Verma, A., Hirsch, D. J., Glatt, C. E., Ronnett, G. V., Snyder, S. H. (1993). Carbon monoxide: a putative neural messenger. Science 259, 381–384. doi: 10.1126/science.7678352
Walle, T., Hsieh, F., Delegge, M. H., Oatis, J. E. Jr., and Walle, U. K. (2004). High absorption but very low bioavailability of oral resveratrol in humans. Drug Metab. Dispos. 32, 1377–1382. doi: 10.1124/dmd.104.000885
Wan, W., Zhang, C., Danielsen, M., Li, Q., Chen, W., Chan, Y., et al. (2016). EGb761 improves cognitive function and regulates inflammatory responses in the APP/PS1 mouse. Exp. Gerontol. 81, 92–100. doi: 10.1016/j.exger.2016.05.007
Wang, J., Zhang, L., Zhang, Y., Luo, M., Wu, Q., Yu, L., et al. (2015). Transcriptional upregulation centra of HO-1 by EGB via the MAPKs/Nrf2 pathway in mouse C2C12 myoblasts. Toxicol. In Vitro 29, 380–388. doi: 10.1016/j.tiv.2014.10.015
Wang, N., Han, Q., Wang, G., Ma, W. P., Wang, J., Wu, W. X., et al. (2016a). Resveratrol Protects Oxidative Stress-Induced Intestinal Epithelial Barrier Dysfunction by Upregulating Heme Oxygenase-1 Expression. Dig. Dis. Sci. 61, 2522–2534. doi: 10.1007/s10620-016-4184-4
Wang, P., Sang, S. (2018). Metabolism and pharmacokinetics of resveratrol and pterostilbene. Biofactors 44, 16–25. doi: 10.1002/biof.1410
Wang, Y., Feng, F., Liu, M., Xue, J., Huang, H. (2018a). Resveratrol ameliorates sepsis-induced acute kidney injury in a pediatric rat model via Nrf2 signaling pathway. Exp. Ther. Med. 16, 3233–3240. doi: 10.3892/etm.2018.6533
Wang, Y., Huo, Y., Zhao, L., Lu, F., Wang, O., Yang, X., et al. (2016b). Cyanidin-3-glucoside and its phenolic acid metabolites attenuate visible light-induced retinal degeneration in vivo via activation of Nrf2/HO-1 pathway and NF-kappaB suppression. Mol. Nutr. Food Res. 60, 1564–1577. doi: 10.1002/mnfr.201501048
Wang, Y., Wang, X., Zhang, L., Zhang, R. (2018b). Alleviation of Acute Lung Injury in Rats with Sepsis by Resveratrol via the Phosphatidylinositol 3-Kinase/Nuclear Factor-Erythroid 2 Related Factor 2/Heme Oxygenase-1 (PI3K/Nrf2/HO-1) Pathway. Med. Sci. Monit. 24, 3604–3611. doi: 10.12659/MSM.910245
Weiskirchen, S., Weiskirchen, R. (2016). Resveratrol: How Much Wine Do You Have to Drink to Stay Healthy? Adv. Nutr. 7, 706–718. doi: 10.3945/an.115.011627
Wu, C. C., Huang, Y. S., Chen, J. S., Huang, C. F., Su, S. L., Lu, K. C., et al. (2015). Resveratrol ameliorates renal damage, increases expression of heme oxygenase-1, and has anti-complement, anti-oxidative, and anti-apoptotic effects in a murine model of membranous nephropathy. PLoS One 10, e0125726. doi: 10.1371/journal.pone.0125726
Yadav, A., Sunkaria, A., Singhal, N., Sandhir, R. (2018). Resveratrol loaded solid lipid nanoparticles attenuate mitochondrial oxidative stress in vascular dementia by activating Nrf2/HO-1 pathway. Neurochem. Int. 112, 239–254. doi: 10.1016/j.neuint.2017.08.001
Yang, J., Huang, J., Shen, C., Cheng, W., Yu, P., Wang, L., et al. (2018). Resveratrol Treatment in Different Time-Attenuated Neuronal Apoptosis After Oxygen and Glucose Deprivation/Reoxygenation via Enhancing the Activation of Nrf-2 Signaling Pathway In Vitro. Cell Transplant 27, 1789–1797. doi: 10.1177/0963689718780930
Yao, P., Li, K., Song, F., Zhou, S., Sun, X., Zhang, X., et al. (2007). Heme oxygenase-1 upregulated by Ginkgo biloba extract: potential protection against ethanol-induced oxidative liver damage. Food Chem. Toxicol. 45, 1333–1342. doi: 10.1016/j.fct.2007.01.016
Yeh, C. T., Ching, L. C., Yen, G. C. (2009). Inducing gene expression of cardiac antioxidant enzymes by dietary phenolic acids in rats. J. Nutr. Biochem. 20, 163–171. doi: 10.1016/j.jnutbio.2008.01.005
Yin, O. Q., Tomlinson, B., Waye, M. M., Chow, A. H., Chow, M. S. (2004). Pharmacogenetics and herb-drug interactions: experience with Ginkgo biloba and omeprazole. Pharmacogenetics 14, 841–850. doi: 10.1097/00008571-200412000-00007
Yu, C. L., Zhao, X. M., Niu, Y. C. (2016). Ferulic Acid Protects Against Lead Acetate-Induced Inhibition of Neurite Outgrowth by Upregulating HO-1 in PC12 Cells: Involvement of ERK1/2-Nrf2 Pathway. Mol. Neurobiol. 53, 6489–6500. doi: 10.1007/s12035-015-9555-x
Yuan, Q., Wang, C. W., Shi, J., Lin, Z. X. (2017). Effects of Ginkgo biloba on dementia: An overview of systematic reviews. J. Ethnopharmacol. 195, 1–9. doi: 10.1016/j.jep.2016.12.005
Zhang, H. F., Huang, L. B., Zhong, Y. B., Zhou, Q. H., Wang, H. L., Zheng, G. Q., et al. (2016). An Overview of Systematic Reviews of Ginkgo biloba Extracts for Mild Cognitive Impairment and Dementia. Front. Aging Neurosci. 8, 276. doi: 10.3389/fnagi.2016.00276
Zhang, W., Song, J. K., Yan, R., Li, L., Xiao, Z. Y., Zhou, W. X., et al. (2018). Diterpene ginkgolides protect against cerebral ischemia/reperfusion damage in rats by activating Nrf2 and CREB through PI3K/Akt signaling. Acta Pharmacol. Sin. 39, 1259–1272. doi: 10.1038/aps.2017.149
Zhang, X., Jiang, A., Qi, B., Ma, Z., Xiong, Y., Dou, J., et al. (2015). Resveratrol Protects against Helicobacter pylori-Associated Gastritis by Combating Oxidative Stress. Int. J. Mol. Sci. 16, 27757–27769. doi: 10.3390/ijms161126061
Keywords: ferulic acid, Ginkgo biloba, heme oxygenase, neuroprotection, resveratrol
Citation: Mhillaj E, Cuomo V, Trabace L and Mancuso C (2019) The Heme Oxygenase/Biliverdin Reductase System as Effector of the Neuroprotective Outcomes of Herb-Based Nutritional Supplements. Front. Pharmacol. 10:1298. doi: 10.3389/fphar.2019.01298
Received: 27 August 2019; Accepted: 10 October 2019;
Published: 11 November 2019.
Edited by:
Claudio Viegas Jr., Federal University of Alfenas, BrazilReviewed by:
Caterina Scuderi, Sapienza University of Rome, ItalyScott Smid, University of Adelaide, Australia
Copyright © 2019 Mhillaj, Cuomo, Trabace and Mancuso. This is an open-access article distributed under the terms of the Creative Commons Attribution License (CC BY). The use, distribution or reproduction in other forums is permitted, provided the original author(s) and the copyright owner(s) are credited and that the original publication in this journal is cited, in accordance with accepted academic practice. No use, distribution or reproduction is permitted which does not comply with these terms.
*Correspondence: Cesare Mancuso, cesare.mancuso@unicatt.it