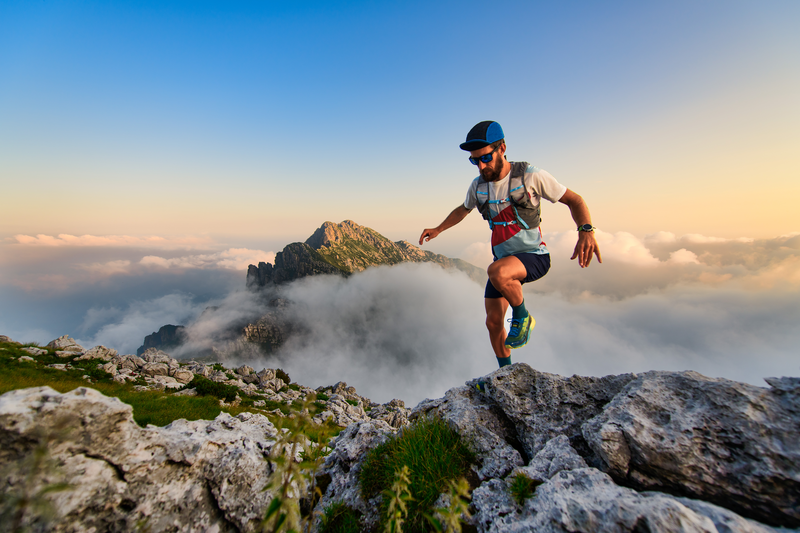
95% of researchers rate our articles as excellent or good
Learn more about the work of our research integrity team to safeguard the quality of each article we publish.
Find out more
ORIGINAL RESEARCH article
Front. Pharmacol. , 15 August 2019
Sec. Experimental Pharmacology and Drug Discovery
Volume 10 - 2019 | https://doi.org/10.3389/fphar.2019.00895
Introduction: Previous studies demonstrated that 35 mM QX-OH/10 mM Levobupivacaine (LL-1), a fixed-dose combination, produced a long-acting effect in rat local anesthesia models. All preclinical pharmacodynamic results indicated that LL-1 had potential for postsurgical pain treatment. The objective of this study was to investigate the pharmacokinetics of LL-1. Then, the possible mechanism of the extended duration by the combination was examined.
Methods and Results: All experiments were examined and approved by the Committee of Animal Care of the West China Hospital Sichuan University (Ethical approval number, 2015014A). The compound action potentials were recorded to verify the pharmacodynamic result in ex vivo. In frog sciatic nerve, LL-1 produced an effective inhibition with rapid onset time. The concentration-time profiles of LL-1 were determined in plasma and local tissues after sciatic nerve block. The maximum concentration of QX-OH and levobupivacaine were 727.22 ± 43.38 µg/g and 256.02 ± 28.52 µg/g in muscle, 634.26 ± 36.04 µg/g and 429.63 ± 48.64 µg/g in sciatic nerve, and 711.71 ± 25.14 ng/ml and 114.40 ± 10.19 ng/ml in plasma, respectively. The absorption of QX-OH into circulation was very rapid at 0.71 ± 0.06 h, which was faster than that of levobupivacaine (4.11 ± 0.39 h, p = 0.003). The half-time of QX-OH in plasma and local tissues had no significant difference (p = 0.329), with the values of 2.64 h, 3.20 h, and 3.79 h in plasma, muscle, and sciatic nerve, respectively. The elimination profile of levobupivacaine differed from that of QX-OH, which was slower eliminated from plasma (4.89 ± 1.77 h, p = 0.036) than from muscle (1.38 ± 0.60 h) or sciatic nerve (1.28 ± 0.74 h). When levobupivacaine was used alone, the Tmax in plasma was 1.07 ± 0.16 h. Interestingly, the Tmax of levobupivacaine in the plasma was increased by four times in combination with QX-OH (4.11 ± 0.39 h). Levobupivacaine promotes cellular QX-OH uptake.
Conclusion: The preclinical pharmacokinetic study of LL-1 in the rat plasma, muscle, and sciatic nerve was accomplished. Then, the possible mechanism of the prolonged duration was that QX-OH delayed the absorption of levobupivacaine from the injection site into circulation, and levobupivacaine accelerated QX-OH to accumulate into cells.
More than 80% of patients experience an acute pain after surgery, and in 75% of them, the pain severity is either moderate or extreme (Chou et al., 2016). The annual volume of surgeries has reached more than 300 million worldwide, suggesting that there are at least 200 million patients who will suffer an acute postsurgical pain every year (Weiser et al., 2016). Poor postsurgical pain management can cause a multitude of complications, including cardiopulmonary complications, prolonged hospital stays, development of chronic pain, and drug addiction (Kehlet et al., 2006). Multimodal analgesia is a primary pain control method in clinics that combines different analgesic drugs and techniques to generate a synergistic effect by utilizing distinct mechanisms of action and affecting different pain pathways (Kehlet and Dahl, 1993; Chou et al., 2016). Local anesthetics that provide adequate pain control with limited side effects are increasingly used in clinical practice (Wick et al., 2017). However, the short duration of local anesthetics typically requires continuous catheter infusion, which can be associated with complications, such as infection, dislodgement, leakage or nerve injury (Bomberg et al., 2016; Ilfeld, 2017). Thus, to develop a long-acting local anesthetic for postsurgical pain management is an unmet medical need (Santamaria et al., 2017).
In recent years, efforts to develop a novel long-acting local anesthetic included research on new drug molecules (Padera et al., 2006; Lim et al., 2007; Ries et al., 2009; Rodriguez-Navarro et al., 2011; Banerjee et al., 2015; Zhang et al., 2017b), adjuvant drugs (Kito et al., 1998; Sakamoto et al., 2016; Zhang et al., 2018), and sustained-release formulations (Ohri et al., 2013; Ramos Campos et al., 2013; Burbridge and Jaffe, 2015; Zhao et al., 2017b). In previous studies, we showed that QX-OH (Invention Patent, China, ZL201410688865.1), a quaternary lidocaine derivative, maintained the local anesthetic effect over a long period in rats (Zhang et al., 2017b). Furthermore, we found that the duration of QX-OH can be extended in combination with levobupivacaine (Levo-Bupi), and the longest duration with moderate local toxicity was achieved at the constant concentration ratio of 35 mM/10 mM (QX-OH/Levo-Bupi, denoted as LL-1) (Zhao et al., 2017a; Yin et al., 2019). In our previous work (Zhao et al., 2017a), LL-1 had a longer duration than Exparel™, a sustained-release formulation of bupivacaine, in rat models for sciatic nerve block and total knee surgery. These preclinical pharmacodynamic data indicated the potential of LL-1 as an effective treatment for postsurgical pain.
The objective of this study was to assess the pharmacokinetics of LL-1 in rats. We further investigated the underlying mechanism of the extended duration of the local anesthetic effect achieved by the fixed dose combination.
QX-OH (purity: 99.8%) was synthesized according to the protocol described in our previous study (Zhang et al., 2017b). QX-314, lidocaine, levobupivacaine, acetonitrile (HPLC grade), and formic acid (HPLC grade) were purchased from Sigma-Aldrich Co., Ltd. (MO, USA). Ropivacaine was purchased from the China National Institutes for Food and Drug Control. Isoflurane was bought from Hebei Yipin Pharmaceutical Co., Ltd. (Hebei, China). DMEM with high glucose, penicillin–streptomycin, and phosphate buffered saline (PBS) were purchased from Thermo Fisher Scientific Inc. Ringer solution was bought from Procell Life Science & Technology Co., Ltd. (Wuhan, China). The LL-1 was prepared by diluting the mixture of QX-OH powder and Levo-Bupi powder at ratio of 35 mM/10 mM in ultrapure water. Ultrapure water was prepared by Milli-Q® water purification system (Merck Millipore, Darmstadt, Germany).
The weight of young adult Sprague-Dawley rats at range of 270 to 330 g and frogs were purchased from Dossy Biological Technology Co., Ltd. (Chengdu, China). Rats were maintained in 5 per cage with separate ventilation, uncontrolled food and water supply, controlled temperature and humidity under 12-h light/dark cycle (lights on at 7:00 a.m.). The total number of animals used in the study was 160 for rat and 9 for frog. In each group, the blood samples were collected from 10 rats (half male and half female), respectively. To determine drug concentration in local tissues, 110 male rats were randomized into each time points (n = 5). Nine frogs were used for compound action potential measurement. All experiments were examined and approved by the Committee of Animal Care of the West China Hospital Sichuan University (Ethical approval number, 2015014A). The practices of experiment were depending on the Guidance on the Care and Use of Laboratory Animals (U.S. National Institutes of Health Publication Publications No.80-23, revised in 1996). All procedures were strictly executed depending on the standard practice for biosecurity and institutional safety published by West China Hospital Sichuan University.
In brief, rats were anesthetized by inhaling 1%–2% isoflurane mixed with oxygen through an inhouse-made mask. Then, the rats were placed at right lateral position and inserted vertically using a 23-gauge injector at the middle of the line from the greater trochanter to ischial tuberosity (Binshtok et al., 2007; Zhang et al., 2017b). Once the bone has been contacted, 200 μl drug was injected.
According to the China Food and Drug Administration guidance for preclinical pharmacokinetic study, there should be more than three sampling points adjacent to the time to reach the maximum concentration. In our preliminary experiment, the time to maximum concentration of Levo-Bupi, as a single drug in the rat sciatic nerve block, was 0.5 h. Thus, to obtain an accurate value of the maximum concentration of Levo-Bupi, we added another time point at 0.33 h. Therefore, 200 μl blood sample was harvested via caudal vein from 10 rats after administration (half males and half females) at time points for QX-OH and LL-1: 0, 0.16, 0.5, 0.67, 1, 2, 3, 4, 5, 6, 8, 9, and 10 h; for Levo-Bupi: 0, 0.16, 0.33, 0.5, 0.67, 1, 2, 3, 4, 5, 6, 8, 9, and 10 h. Blood samples were maintained in heparinized polypropylene tube separately and then centrifuged at 877 × g for 10 min to gain plasma within 1 h. All the samples were kept at -80°C.
On the basis of previous pharmacodynamic studies, nine sampling points were used for determining drug concentration in muscle and sciatic nerve for QX-OH and LL-1: 0.5, 1, 2, 3, 4, 5, 6, 7, and 8 h; four sampling points for Levo-Bupi: 0.5, 1, 2, and 3 h. Due to the destructive sampling, five male rats were sacrificed at each time point for collecting muscle and sciatic nerve nearby the injection site. Then all samples were weighed and frozen at -80°C.
According to previous study (Zhang et al., 2017a), plasma samples were deproteinized by acetonitrile solution with ropivacaine hydrochloride (internal standard, IS). The mixture was centrifuged for 10 min at 28,621 × g in 4°C after fully vortexed. The supernatant was diluted with ultrapure water (50:50, v/v). Muscle and sciatic nerve samples were homogenized by gentleMACS™ Dissociator (Miltenyi Biotec, Germany) and tissue grinder (model SCIENTZ-48, Ningbo, China), respectively. Tissue homogenate was mixed with IS solution by vortex and then centrifuged for 10 min at 28,621 × g. Then, the supernatant was diluted with ultrapure water at equal volume ratio. LC-MS/MS analysis system consist of Agilent 6460 triple quadrupole mass spectrometer and electrospray ionization source (Agilent Technologies, CA, USA). Chromatographic separation was adopted by Agilent Extend C18 column (100 mm × 3 mm, 3.5 μm) at 35°C with isocratic elution containing 0.05% formic acid and acetonitrile at the volume ratio of 78:22 at a flow rate of 0.3 ml/min. Mass spectrometry conditions were in a positive ionization mode: the sheath gas flow rate, 11.0 L/min; sheath gas heater temperature, 300°C; nebulizer pressure, 45 psi; capillary voltage, 3,500 V. MassHunter software was used to analyze data (B.04.00 Build 4.0.479.0, Agilent Technologies, USA).
The parameters of pharmacokinetics were analyzed by DAS® (Drug and Statistics, version 3.2.1, China) using noncompartmental model. In this study, the following pharmacokinetic parameters were calculated: the value of maximum concentration (Cmax) and the time to acquire (Tmax); the elimination half-life (t1/2); area under curve (AUC) from zero to the last time point (AUC0–last) and from zero to infinity (AUC0–∞); clearance (CL/F), and volume of distribution (Vd/F).
According to previous published researches (Matsushita et al., 2013; Ohtsubo et al., 2015). Nine of frogs were decapitated and then pithed. Then, the sciatic nerve was dissected from the lumbar plexus to the knee. The two ends of isolated sciatic nerves were carefully tied to the wires by threads and placed in Ringer solution (NaCl, 115.5 mmol/L; KCl, 2.0 mmol/L; CaCl2, 1.8 mmol/L; Na2HPO4, 1.3 mmol/L and NaH2PO4, 0.7 mmol/L; pH = 7.4).
The compound action potentials (CAPs) from frog sciatic nerve fibers was recorded by the biological function experiment system (BL-420F, Chengdu Techman Software, China). All practices were carried out at room temperature. The sciatic nerve was loosely placed in three platinum wires and then CAPs were recorded in air using a preamplifier. In this system, two of the platinum wires were used to record CAPs, and the other one was for stimulation. The parameters of stimulation were set as follows: intensity, 3 V; frequency, 1 Hz; rectangular pulses, 0.1 ms. The procedure should be performed quickly in order to avoid the nerve dry. The time of measurement should be less than 2 min. After CAPs examined, the sciatic nerve was put back into Ringer solution with drugs. In this study, we compared the amplitude of CAPs after using drugs with baseline to present the degree of inhibition from frog sciatic nerve, and which was analyzed using the following equation: CAPs amplitude (%) = CAPs measurement/CAPs baseline × 100%.
HT22 cell line, a sub-line derived from HT4 cells that were originally immortalized from primary mouse hippocampal neuron, were a generous gift from Dr. Huang (West China Second University Hospital, Sichuan University, China). HT22 cells were cultured in DMEM with high glucose (HyClone, Utah, USA) supplemented with 10% fetal bovine serum (Gibco, New York, USA) and 1% penicillin and streptomycin (HyClone, Utah, USA) in a humidified incubator with 5% CO2 and 95% air at 37°C.
According to previous study (Brenneis et al., 2014), cells were plated on 35 mm 6-well dishes (2.5 × 105 per well). When cells reached confluence at 70%–80%, the medium was removed, and plates washed with PBS (NaCl, 137.0 mmol/L; KCl, 2.7 mmol/L; KH2PO4, 1.5 mmol/L and Na2HPO4·12H2O, 8.0 mmol/L; pH = 7.4). Thirty wells were divided to incubate with LL-1, 35 mM QX-OH and 10 mM Levo-Bupi for 10 min, and then washed four times with PBS. Cells were translated to polypropylene tube. The cell suspensions were centrifuged at 72 × g and the supernatants were removed by pipette. All samples were frozen at -80°C before testing. Samples were homogenized with 100 µL of saline using a tissue grinder. After mixing 50 μl homogenate, 50 μl acetonitrile, and 10 μl IS, then the mixture was centrifuged for 10 min at 28,621 × g, and the supernatant was injected for analysis.
All statistical analyses were calculated through SPSS (version 23, SPSS, Chicago, IL, USA). All data should be examined by tests for standard normal distribution and homogeneity of variance. If the data are without normal distribution or equal variance, it should be analyzed by the method of nonparametric tests. For the CAPs amplitude, it used two-way repeated measures analysis of variance to compare the difference of drug and time, and then followed with the method of least significant difference (LSD) for pairwise comparison to compare the difference among drugs in the same time point. For the half-time and time to block CAPs, it was conducted by Kruskal–Wallis test due to its unequal variance, and the Cmax, Tmax and the cellular drug uptake assay were statistically analyzed using independent-sample T test. The standard of statistical significance was set at p < 0.05.
After sciatic nerve block, the LL-1 concentration-time profiles were determined in the plasma, sciatic nerve, and muscle. The data are presented in Figure 1, and the calculated pharmacokinetic parameters for LL-1 are shown in Table 1. The Cmax values of QX-OH and Levo-Bupi were 727.22 ± 43.38 and 256.02 ± 28.52 μg/g in muscle, 634.26 ± 36.04 and 429.63 ± 48.64 μg/g in sciatic nerve, and 711.71 ± 25.14 and 114.40 ± 10.19 ng/ml in plasma, respectively. The Cmax of QX-OH in sciatic nerve did not significantly differ from that in muscle (p = 0.058). In contrast, the Cmax of Levo-Bupi was higher in sciatic nerve (429.63 ± 48.64 μg/g) than that in muscle (256.02 ± 28.52 μg/g, p = 0.008). In LL-1, the absorption of QX-OH into circulation (0.71 ± 0.06 h) was more rapid than that of Levo-Bupi (4.11 ± 0.39 h, p = 0.003). The half-time of QX-OH in plasma and local tissues had no significant difference (p = 0.329), with the values of 2.64, 3.20, and 3.79 h in plasma, muscle, and sciatic nerve, respectively. The elimination profile of Levo-Bupi differed from that of QX-OH, which was slower eliminated from plasma (4.89 ± 1.77 h, p = 0.036) than from muscle (1.38 ± 0.60 h) or sciatic nerve (1.28 ± 0.74 h). The computed AUC values of QX-OH from zero to the last time point were 1,487.72 ± 82.20 μg*h/g in sciatic nerve, 1,188.29 ± 62.38 μg*h/g in muscle, and 1,913.72 ± 148.7 ng*h/ml in plasma. The AUC values of Levo-Bupi from zero to the last time point were 935.82 ± 74.35 μg*h/g in sciatic nerve, 417.58 ± 43.46 μg*h/g in muscle, and 651.23 ± 47.39 ng*h/ml in plasma.
Figure 1 Mean concentration-time profiles of LL-1 in plasma (A–B), sciatic nerve (C–D) and muscle (E–F) after sciatic nerve block in rats. (n = 10 for plasma, and n = 5 per time point for muscle and sciatic nerve). Data are expressed as mean ± SEM. Levo-Bupi, levobupivacaine; LL-1, 35 mM QX-OH/10 mM Levo-Bupi.
Table 1 Tissue and systemic pharmacokinetic parameters of LL-1 after sciatic nerve block in rats (Mean ± SEM).
In a previous study (Yu et al., 2002), the Cmax of bupivacaine was reached at 0.5 h after subcutaneous administration. However, the Tmax of Levo-Bupi in LL-1 was 4.11 ± 0.39 h. Therefore, we tested Levo-Bupi as a single drug in the rat sciatic nerve block model and determined its plasma concentration. The concentration-time profiles of Levo-Bupi are presented in Figure 2, and the calculated pharmacokinetic parameters are shown in Table 2. When Levo-Bupi was used alone, the Tmax in plasma was 1.07 ± 0.16 h (Figure 2A). Interestingly, the Tmax of Levo-Bupi in the plasma was increased by four times in combination with QX-OH (4.11 ± 0.39 h, p < 0.001). The Cmax of Levo-Bupi, used alone, (142.45 ± 13.04 ng/ml) did not significantly differ from that in LL-1 (p = 0.114). In contrast, Levo-Bupi, used alone, had a faster elimination (CL = 1.32 ± 0.08 L/h, p = 0.001; t1/2 = 2.61 ± 0.31 h, p = 0.199). In sciatic nerve block model, the drugs were administrated adjacent to sciatic nerve and then absorbed into circulation. Based on this assumption, we predicted that the absorption delay of Levo-Bupi was related to its accumulation in local tissues. So, we further measured the drug concentration in sciatic nerve and adjacent muscle. When Levo-Bupi was used as a single drug, its concentration in sciatic nerve went from 284.01 ± 32.25 to 4.64 ± 3.66 μg/g; in LL-1, the Levo-Bupi concentration was increased at 1.5 to 24 times in LL-1 at the same time points (422.06 ± 83.62 to 113.63 ± 55.91 μg/g, Figure 2B). Similarly, in the single-drug test, the Levo-Bupi concentration in muscle went from 102.25 ± 15.28 to 2.84 ± 3.25 μg/g, but was increased by 2 to 15 times in the LL-1 at the same time points (256.02 ± 42.78 to 41.42 ± 20.69 μg/g, Figure 2C). These results showed that QX-OH could promote Levo-Bupi accumulation in local tissues, which could delay its absorption into blood circulation.
Figure 2 Mean concentration-time profiles of Levobupivacaine in plasma (A), sciatic nerve (B), and muscle (C) after sciatic nerve block in rats. (n = 10 for plasma, and n = 5 per time point for muscle and sciatic nerve). Data are expressed as mean ± SEM. Levo-Bupi, levobupivacaine; LL-1, 35 mM QX-OH/10 mM Levo-Bupi.
Table 2 The pharmacokinetic parameters of Levobupivacaine in plasma after sciatic nerve block in rats (Mean ± SEM).
Local anesthetics are known to prevent the action potentials of nerve blocking the sensory and motor function (Miller et al., 2009). In local anesthesia animal models, administration of LL-1 was associated with longer duration and shorter onset time than administering the single drugs, QX-OH and Levo-Bupi, at the same concentration (Yin et al., 2019). Monitoring the CAPs in frog sciatic nerve fibers, we confirmed the effect of LL-1, 35 mM QX-OH, and 10 mM Levo-Bupi. The amplitude of CAPs was decreased with the exposure time (Figure 3A). Compared with QX-OH, the CAPs were affected significantly by LL-1 (p < 0.001) and Levo-Bupi (p < 0.001). Within the first 5 min, the CAPs amplitude was reduced to 16%, 34% and 85% by LL-1, Levo-Bupi and QX-OH, respectively. The soak time required to block CAPs was longer with QX-OH (27.50 ± 1.71 min, Figure 3B) than with LL-1 (10.83 ± 1.53 min, p = 0.003) or Levo-Bupi (14.17 ± 2.01 min, p = 0.034).
Figure 3 Effects of LL-1, QX-OH and Levobupivacaine on frog sciatic nerve CAPs. (A) the CAP amplitude; (B) The time to block CAPs, n = 6 in each group). Data are expressed as mean ± SEM. * p < 0.05, # p < 0.001. Levo-Bupi, levobupivacaine, LL-1: 35 mM QX-OH/10 mM Levo-Bupi.
The extended duration in pharmacodynamic study cannot be easily explained only by the slow absorption of Levo-Bupi. Local anesthetics block the action potentials by penetrating into cell and inhibiting the voltage-gated sodium channels (Narahashi et al., 1970; Fink, 1989; Butterworth and Strichartz, 1990). Hence, we tested whether Levo-Bupi can promote cellular QX-OH uptake. Levo-Bupi accelerated membrane permeation of QX-OH and its accumulation in the cells (Figure 4A, p < 0.001). However, the cellular uptake of Levo-Bupi had no difference (Figure 4B, p = 0.067).
Figure 4 The ratio of concentration by cellular drug uptake in HT22 cells (n = 10 in each group). (A) QX-OH; (B) Levo-Bupi. Data are expressed as mean ± SEM. # p < 0.001. Levo-Bupi, levobupivacaine; LL-1, 35 mM QX-OH/10 mM Levo-Bupi.
In this study, we performed the preclinical pharmacokinetic analysis of a novel long-acting local anesthetic, a fixed-dose combination of QX-OH/Levo-Bupi, in rat plasma, muscle, and sciatic nerve. We further explored the underlying mechanism of the extended duration of local anesthetic effects produced by the combination. The pharmacokinetic studies were performed in the sciatic nerve block model.
Previous reports indicated that LL-1 had a longer duration with a rapid onset (Zhao et al., 2017a; Yin et al., 2019). The duration of one drug is closely related to its pharmacokinetic feature. Many factors can affect the pharmacokinetics of a local anesthetic, such as the molecular structure, physicochemical properties, method of administration, local blood flow, and drug–drug interaction. In this study, we proved that QX-OH delayed the absorption of Levo-Bupi from local tissues into circulation. Meanwhile, Levo-Bupi accelerated QX-OH to accumulate into cells. There are two possible reasons for the delayed absorption of Levo-Bupi, decreased local blood flow or drug–drug interactions. In clinical practice, epinephrine, a vasoconstrictor agent, is used to increase the duration of local anesthesia. In the inferior alveolar nerve block (Keesling and Hinds, 1963; Dagher et al., 1997), the Tmax of Levo-Bupi was doubled by combining it with epinephrine in the transversus abdominis plane block (Corvetto et al., 2012). Thus, epinephrine extended the Tmax of local anesthetics by decreasing local blood flow. The Tmax of Levo-Bupi in the plasma was increased by four times after combine with QX-OH. However, the underlying mechanism of the effect of QX-OH is not fully understood. We considered that this effect might have been generated by a decreased local blood flow. However, in a recent study, Jang, et al. mixed lidocaine with trivalent PO43- to generate a lidocaine/multivalent ion complex by ionic interaction, which promoted a prolonged period of lidocaine release and a long duration of nerve blockade (Jang et al., 2017). Meanwhile, Yin, et al. investigated the effect of differently charged liposomes on the duration of QX-314. Anionic liposomes produced a longer duration of the nerve blockage when compared with the cationic and neutral liposomes. Interestingly, QX-314 was attached to the surface of anionic liposomes by electrostatic interactions (Yin et al., 2017). Similar to the results with QX-OH, the Tmax of Levo-Bupi with in combination with QX-314 was extended to 4 h in the sciatic nerve block (Supplementary Figure 1A). However, lidocaine did not have a similar effect on the absorption of Levo-Bupi (Supplementary Figure 1B). In solution, traditional local anesthetics, such as lidocaine and bupivacaine, are in a rapid equilibrium between the uncharged and charged status. Based on the chemical structures, the most important difference between the traditional local anesthetics and QX-OH and QX-314 is that the latter two compounds are permanently charged in solution. Meanwhile, Levo-Bupi owns an ione electron pair in the nitrogen atom of tertiary ammonium group. Thus, we considered whether the underlying mechanism of QX-OH-induced the delayed absorption of Levo-Bupi is ionic interactions.
In the Results section, we demonstrated that Levo-Bupi accelerated the cellular accumulation of QX-OH, which was an important mechanism to extend the duration of LL-1. In previous study, bupivacaine enhanced the cellular uptake of QX-314 in RAW, N1E and CHO cells (Brenneis et al., 2014). In addition, bupivacaine, a transient receptor potential (TRP) channels agonist, promote QX-314 to permeate the membrane via TRP channels (Meyers et al., 2003; Binshtok et al., 2007; Chung et al., 2008; Banke and Wickenden, 2009; Chen et al., 2009; Kim et al., 2010; Rivera-Acevedo et al., 2011; Stueber et al., 2016). Based on an analogical molecular structure with QX-314, we considered that QX-OH might be accumulated into cellular through TRP channels as QX-314 which was assist of Levo-Bupi as a TRP channels agonist.
The preclinical pharmacokinetic study of a novel long-acting local anesthetic, a fixed-dose combination of QX-OH/Levo-Bupi, in rat plasma and local tissues was accomplished. Then, the prolonged nerve blockade may be related to the effect of interaction between QX-OH and Levo-Bupi. QX-OH delayed the absorption of Levo-Bupi from the local tissue into circulation, and Levo-Bupi accelerated QX-OH to accumulate into cells.
The raw data supporting the conclusions of this manuscript will be made available by the authors, without undue reservation, to any qualified researcher.
YZ, QY, JL, and WZ conceived and designed the project. YZ, QY, DG, YK, and JY performed the experiments. YZ and WZ analyzed the data. YZ wrote the manuscript. JL and WZ revised the manuscript.
This work was supported by the Post-Doctor Research Project, West China Hospital, Sichuan University (2019HXBH012) and the National Science and Technology Major Project, Ministry of Science and Technology of the People’s Republic of China (No. 2014ZX09101001-003).
The authors declare that the research was conducted in the absence of any commercial or financial relationships that could be construed as a potential conflict of interest.
We are grateful to LinQiao Tang and Yan Wang from the Core facility of West China Hospital for their technical supports.
The Supplementary Material for this article can be found online at: https://www.frontiersin.org/articles/10.3389/fphar.2019.00895/full#supplementary-material
Figure S1 | Drug-time curve of QX-314/Levo-Bupi (35 mM/10 mM, A) and Lidocaine/Levo-Bupi (35 mM/10 mM, B) in plasma after sciatic nerve block in rats. (n = 10 in each group). Data are expressed as mean ± SEM. Levo-Bupi: levobupivacaine
Banerjee, M., Baranwal, A., Saha, S., Saha, A., Priestley, T. (2015). EN3427: a novel cationic aminoindane with long-acting local anesthetic properties. Anesth Analg. 120, 941–949. doi: 10.1213/ANE.0000000000000629
Banke, T. G., Wickenden, A. D. (2009). Intracellular zinc irritates TRPA1. Nat. Chem. Biol. 5, 141–142. doi: 10.1038/nchembio0309-141
Binshtok, A. M., Bean, B. P., Woolf, C. J. (2007). Inhibition of nociceptors by TRPV1-mediated entry of impermeant sodium channel blockers. Nature 449, 607–610. doi: 10.1038/nature06191
Bomberg, H., Kubulus, C., Herberger, S., Wagenpfeil, S., Kessler, P., Steinfeldt, T., et al. (2016). Tunnelling of thoracic epidural catheters is associated with fewer catheter-related infections: a retrospective registry analysis. Br. J. Anaesth. 116, 546–553. doi: 10.1093/bja/aew026
Brenneis, C., Kistner, K., Puopolo, M., Jo, S., Roberson, D., Sisignano, M., et al. (2014). Bupivacaine-induced cellular entry of QX-314 and its contribution to differential nerve block. Br. J. Pharmacol. 171, 438–451. doi: 10.1111/bph.12466
Burbridge, M., Jaffe, R. A. (2015). Exparel(R): a new local anesthetic with special safety concerns. Anesth Analg. 121, 1113–1114. doi: 10.1213/ANE.0000000000000822
Butterworth, J. F. T., Strichartz, G. R. (1990). Molecular mechanisms of local anesthesia: a review. Anesthesiology 72, 711–734. doi: 10.1097/00000542-199004000-00022
Chen, J., Kim, D., Bianchi, B. R., Cavanaugh, E. J., Faltynek, C. R., Kym, P. R., et al. (2009). Pore dilation occurs in TRPA1 but not in TRPM8 channels. Mol. Pain 5, 3. doi: 10.1186/1744-8069-5-3
Chou, R., Gordon, D. B., De Leon-Casasola, O. A., Rosenberg, J. M., Bickler, S., Brennan, T., et al. (2016). Management of postoperative pain: a clinical practice guideline from the american pain society, the american society of regional anesthesia and pain medicine, and the american society of anesthesiologists’ committee on regional anesthesia, executive committee, and administrative council. J. Pain 17, 131–157. doi: 10.1016/j.jpain.2015.12.008
Chung, M. K., Guler, A. D., Caterina, M. J. (2008). TRPV1 shows dynamic ionic selectivity during agonist stimulation. Nat. Neurosci. 11, 555–564. doi: 10.1038/nn.2102
Corvetto, M. A., Echevarria, G. C., De La Fuente, N., Mosqueira, L., Solari, S., Altermatt, F. R. (2012). Comparison of plasma concentrations of levobupivacaine with and without epinephrine for transversus abdominis plane block. Reg. Anesth. Pain Med. 37, 633–637. doi: 10.1097/AAP.0b013e31826c330a
Dagher, F. B., Yared, G. M., Machtou, P. (1997). An evaluation of 2% lidocaine with different concentrations of epinephrine for inferior alveolar nerve block. J. Endod. 23, 178–180. doi: 10.1016/S0099-2399(97)80271-3
Fink, B. R. (1989). The long and the short of conduction block. Anesth Analg. 68, 553–555. doi: 10.1213/00000539-198905000-00002
Ilfeld, B. M. (2017). Continuous peripheral nerve blocks: an update of the published evidence and comparison with novel, alternative analgesic modalities. Anesth Analg. 124, 308–335. doi: 10.1213/ANE.0000000000001581
Jang, Y. J., Lee, J. H., Seo, T. B., Oh, S. H. (2017). Lidocaine/multivalent ion complex as a potential strategy for prolonged local anesthesia. Eur. J. Pharm. Biopharm. 115, 113–121. doi: 10.1016/j.ejpb.2017.02.007
Keesling, G. R., Hinds, E. C. (1963). Optimal concentration of epinephrine in lidocaine solutions. J. Am. Dent. Assoc. 66, 337–340. doi: 10.14219/jada.archive.1963.0108
Kehlet, H., Dahl, J. B. (1993). The value of “multimodal” or “balanced analgesia” in postoperative pain treatment. Anesth Analg. 77, 1048–1056. doi: 10.1213/00000539-199311000-00030
Kehlet, H., Jensen, T. S., Woolf, C. J. (2006). Persistent postsurgical pain: risk factors and prevention. Lancet 367, 1618–1625. doi: 10.1016/S0140-6736(06)68700-X
Kim, H. Y., Kim, K., Li, H. Y., Chung, G., Park, C. K., Kim, J. S., et al. (2010). Selectively targeting pain in the trigeminal system. Pain 150, 29–40. doi: 10.1016/j.pain.2010.02.016
Kito, K., Kato, H., Shibata, M., Adachi, T., Nakao, S., Mori, K. (1998). The effect of varied doses of epinephrine on duration of lidocaine spinal anesthesia in the thoracic and lumbosacral dermatomes. Anesth Analg. 86, 1018–1022. doi: 10.1213/00000539-199805000-00021
Lim, T. K., Macleod, B. A., Ries, C. R., Schwarz, S. K. (2007). The quaternary lidocaine derivative, QX-314, produces long-lasting local anesthesia in animal models in vivo. Anesthesiology 107, 305–311. doi: 10.1097/01.anes.0000270758.77314.b4
Matsushita, A., Ohtsubo, S., Fujita, T., Kumamoto, E. (2013). Inhibition by TRPA1 agonists of compound action potentials in the frog sciatic nerve. Biochem. Biophys. Res. Commun. 434, 179–184. doi: 10.1016/j.bbrc.2013.02.127
Meyers, J. R., Macdonald, R. B., Duggan, A., Lenzi, D., Standaert, D. G., Corwin, J. T., et al. (2003). Lighting up the senses: FM1-43 loading of sensory cells through nonselective ion channels. J. Neurosci. 23, 4054–4065. doi: 10.1523/JNEUROSCI.23-10-04054.2003
Miller, R. D., Eriksson, L. I., Fleisher, L. A., Wiener-Kronish, J. P., Young, W. L. (2009). Anesthesia. (Elsevier Health Sciences), 916–919.
Narahashi, T., Frazier, T., Yamada, M. (1970). The site of action and active form of local anesthetics. I. Theory and pH experiments with tertiary compounds. J. Pharmacol. Exp. Ther. 171, 32–44.
Ohri, R., Wang, J. C., Blaskovich, P. D., Pham, L. N., Costa, D. S., Nichols, G. A., et al. (2013). Inhibition by local bupivacaine-releasing microspheres of acute postoperative pain from hairy skin incision. Anesth Analg. 117, 717–730. doi: 10.1213/ANE.0b013e3182a00851
Ohtsubo, S., Fujita, T., Matsushita, A., Kumamoto, E. (2015). Inhibition of the compound action potentials of frog sciatic nerves by aroma oil compounds having various chemical structures. Pharmacol. Res. Perspect. 3, e00127. doi: 10.1002/prp2.127
Padera, R. F., Tse, J. Y., Bellas, E., Kohane, D. S. (2006). Tetrodotoxin for prolonged local anesthesia with minimal myotoxicity. Muscle Nerve 34, 747–753. doi: 10.1002/mus.20618
Ramos Campos, E. V., Silva De Melo, N. F., Guilherme, V. A., De Paula, E., Rosa, A. H., De Araujo, D. R., et al. (2013). Preparation and characterization of poly(epsilon-caprolactone) nanospheres containing the local anesthetic lidocaine. J. Pharm. Sci. 102, 215–226. doi: 10.1002/jps.23350
Ries, C. R., Pillai, R., Chung, C. C., Wang, J. T., Macleod, B. A., Schwarz, S. K. (2009). QX-314 produces long-lasting local anesthesia modulated by transient receptor potential vanilloid receptors in mice. Anesthesiology 111, 122–126. doi: 10.1097/ALN.0b013e3181a9160e
Rivera-Acevedo, R. E., Pless, S. A., Ahern, C. A., Schwarz, S. K. (2011). The quaternary lidocaine derivative, QX-314, exerts biphasic effects on transient receptor potential vanilloid subtype 1 channels in vitro. Anesthesiology 114, 1425–1434. doi: 10.1097/ALN.0b013e318216ea0c
Rodriguez-Navarro, A. J., Berde, C. B., Wiedmaier, G., Mercado, A., Garcia, C., Iglesias, V., et al. (2011). Comparison of neosaxitoxin versus bupivacaine via port infiltration for postoperative analgesia following laparoscopic cholecystectomy: a randomized, double-blind trial. Reg. Anesth. Pain Med. 36, 103–109. doi: 10.1097/AAP.0b013e3182030662
Sakamoto, B., Harker, G., Eppstein, A. C., Gwirtz, K. (2016). Efficacy of local anesthetic with dexamethasone on the quality of recovery following total extraperitoneal bilateral inguinal hernia repair: a randomized clinical trial. JAMA Surg. 151, 1108–1114. doi: 10.1001/jamasurg.2016.2905
Santamaria, C. M., Woodruff, A., Yang, R., Kohane, D. S. (2017). Drug delivery systems for prolonged duration local anesthesia. Mater. Today (Kidlington) 20, 22–31. doi: 10.1016/j.mattod.2016.11.019
Stueber, T., Eberhardt, M. J., Hadamitzky, C., Jangra, A., Schenk, S., Dick, F., et al. (2016). Quaternary lidocaine derivative qx-314 activates and permeates human trpv1 and trpa1 to produce inhibition of sodium channels and cytotoxicity. Anesthesiology 124, 1153–1165. doi: 10.1097/ALN.0000000000001050
Weiser, T. G., Haynes, A. B., Molina, G., Lipsitz, S. R., Esquivel, M. M., Uribe-Leitz, T., et al. (2016). Size and distribution of the global volume of surgery in 2012. Bull. World Health Organ 94, 201–209F. doi: 10.2471/BLT.15.159293
Wick, E. C., Grant, M. C., Wu, C. L. (2017). Postoperative multimodal analgesia pain management with nonopioid analgesics and techniques: a review. JAMA Surg. 152, 691–697. doi: 10.1001/jamasurg.2017.0898
Yin, Q., Li, J., Zheng, Q., Yang, X., Lv, R., Ma, L., et al. (2017). The quaternary lidocaine derivative QX-314 in combination with bupivacaine for long-lasting nerve block: efficacy, toxicity, and the optimal formulation in rats. PLoS One 12, e0174421. doi: 10.1371/journal.pone.0174421
Yin, Q., Zhang, Y., Lv, R., Gong, D., Ke, B., Yang, J., et al. (2019). A fixed-dose combination, QXOH/levobupivacaine, produces long-acting local anesthesia in rats without additional toxicity. Front. Pharmacol. 10, 243. doi: 10.3389/fphar.2019.00243
Yu, H. Y., Li, S. D., Sun, P. (2002). Kinetic and dynamic studies of liposomal bupivacaine and bupivacaine solution after subcutaneous injection in rats. J. Pharm. Pharmacol. 54, 1221–1227. doi: 10.1211/002235702320402062
Zhang, W., Xu, W., Ning, C., Li, M., Zhao, G., Jiang, W., et al. (2018). Long-acting hydrogel/microsphere composite sequentially releases dexmedetomidine and bupivacaine for prolonged synergistic analgesia. Biomaterials 181, 378–391. doi: 10.1016/j.biomaterials.2018.07.051
Zhang, Y., Gong, D., Zheng, Q., Liu, J., Zhang, W. (2017a). LC-MS/MS method for preclinical pharmacokinetic study of QX-OH, a novel long-acting local anesthetic, in sciatic nerve blockade in rats. J. Pharm. Biomed. Anal. 146, 161–167. doi: 10.1016/j.jpba.2017.07.028
Zhang, Y., Yang, J., Yin, Q., Yang, L., Liu, J., Zhang, W. (2017b). QX-OH, a QX-314 derivative agent, produces long-acting local anesthesia in rats. Eur. J. Pharm. Sci. 105, 212–218. doi: 10.1016/j.ejps.2017.05.039
Zhao, W., Yang, J., Zhang, Y., Liu, J., Zhang, W. (2017a). QX-OH/Levobupivacaine: fixed-dose combination to provide a long-acting postoperative pain of knee surgery in rodents. Eur. J. Pharm. Sci. 111, 418–424. doi: 10.1016/j.ejps.2017.10.025
Keywords: preclinical drug development, preclinical pharmacokinetics, long-acting local anesthetic, fixed-dose combination, QX-OH
Citation: Zhang Y, Yin Q, Gong D, Kang Y, Yang J, Liu J and Zhang W (2019) The Preclinical Pharmacological Study of a Novel Long-Acting Local Anesthetic, a Fixed-Dose Combination of QX-OH/Levobupivacaine, in Rats. Front. Pharmacol. 10:895. doi: 10.3389/fphar.2019.00895
Received: 16 May 2019; Accepted: 16 July 2019;
Published: 15 August 2019.
Edited by:
Salvatore Salomone, University of Catania, ItalyReviewed by:
Paola Badino, University of Turin, ItalyCopyright © 2019 Zhang, Yin, Gong, Kang, Yang, Liu and Zhang. This is an open-access article distributed under the terms of the Creative Commons Attribution License (CC BY). The use, distribution or reproduction in other forums is permitted, provided the original author(s) and the copyright owner(s) are credited and that the original publication in this journal is cited, in accordance with accepted academic practice. No use, distribution or reproduction is permitted which does not comply with these terms.
*Correspondence: WenSheng Zhang, emhhbmdfd3NAc2N1LmVkdS5jbg==
Disclaimer: All claims expressed in this article are solely those of the authors and do not necessarily represent those of their affiliated organizations, or those of the publisher, the editors and the reviewers. Any product that may be evaluated in this article or claim that may be made by its manufacturer is not guaranteed or endorsed by the publisher.
Research integrity at Frontiers
Learn more about the work of our research integrity team to safeguard the quality of each article we publish.