- 1Therapeutic and Pharmacology Department, Health and Human Science Research, Academic Unit of Human Medicine and Health Sciences, Autonomous University of Zacatecas, Zacatecas, Mexico
- 2Molecular Biology Unit, Research Institute of University Hospital La Princesa (IP), Madrid, Spain
- 3Department and Nephrology, Research Institute of University Hospital La Princesa (IP), Madrid, Spain
- 4Research Institute of La Paz (IdiPAZ), University Hospital La Paz, Madrid, Spain
- 5Renal research network REDINREN, Madrid, Spain
- 6Molecular Biology Research Centre Severo Ochoa, Spanish Council for Scientific Research (CSIC), Madrid, Spain
Epithelial-to-mesenchymal transition (EMT) is a self-regulated physiological process required for tissue repair that, in non-controled conditions may lead to fibrosis, angiogenesis, loss of normal organ function or cancer. Although several molecular pathways involved in EMT regulation have been described, this process does not have any specific treatment. This article introduces a systematic review of effective natural plant compounds and their extract that modulates the pathological EMT or its deleterious effects, through acting on different cellular signal transduction pathways both in vivo and in vitro. Thereby, cryptotanshinone, resveratrol, oxymatrine, ligustrazine, osthole, codonolactone, betanin, tannic acid, gentiopicroside, curcumin, genistein, paeoniflorin, gambogic acid and Cinnamomum cassia extracts inhibit EMT acting on transforming growth factor-β (TGF-β)/Smads signaling pathways. Gedunin, carnosol, celastrol, black rice anthocyanins, Duchesnea indica, cordycepin and Celastrus orbiculatus extract downregulate vimectin, fibronectin and N-cadherin. Sulforaphane, luteolin, celastrol, curcumin, arctigenin inhibit β-catenin signaling pathways. Salvianolic acid-A and plumbagin block oxidative stress, while honokiol, gallic acid, piperlongumine, brusatol and paeoniflorin inhibit EMT transcription factors such as SNAIL, TWIST and ZEB. Plectranthoic acid, resveratrol, genistein, baicalin, polyphyllin I, cairicoside E, luteolin, berberine, nimbolide, curcumin, withaferin-A, jatrophone, ginsenoside-Rb1, honokiol, parthenolide, phoyunnanin-E, epicatechin-3-gallate, gigantol, eupatolide, baicalin and baicalein and nitidine chloride inhibit EMT acting on other signaling pathways (SIRT1, p38 MAPK, NFAT1, SMAD, IL-6, STAT3, AQP5, notch 1, PI3K/Akt, Wnt/β-catenin, NF-κB, FAK/AKT, Hh). Despite the huge amount of preclinical data regarding EMT modulation by the natural compounds of plant, clinical translation is poor. Additionally, this review highlights some relevant examples of clinical trials using natural plant compounds to modulate EMT and its deleterious effects. Overall, this opens up new therapeutic alternatives in cancer, inflammatory and fibrosing diseases through the control of EMT process.
Introduction
Epithelial-to-mesenchymal transition (EMT) is a tightly regulated physiological process implicated in tissue repair and in embryogenesis (Thiery et al., 2009). During EMT, epithelial cells undergo multiple morphologic, biochemical and genetic rearranges that gradually enable them to acquire a mesenchymal phenotype (Kalluri and Weinberg, 2009; Savagner, 2010; Kong et al., 2015).
We could consider two types of EMT, a physiological and a pathological EMT. The main characteristic of the physiological EMT is its ability to self-regulate, and it is related to embryonic development, organ formation, wound healing and tissue regeneration (Kalluri, 2009). In contrast, pathological EMT usually accompanies diseases and does not self-regulate. In this last case, EMT is an irreversible process and contributes to the failure of diseased organs (Thiery et al., 2009), which justifies the scientific investment into controlling such a process. Pathological EMT is present in many inflammatory and immunological diseases and leads to tissue fibrosis, angiogenesis, loss of organ function, cancer progression and metastasis (Corvol et al., 2009; Lee et al., 2006; Kalluri, 2009).
Although the involvement of EMT in some organ fibrosis, such as of the kidney, is controversial, more evidence about the role of pathological EMT has been reported in other organ fibrosis including that of the lung, the peritoneum and the heart, as well as in cancer progression (Yáñez-Mó et al., 2003; Rastaldi, 2006; Thiery et al., 2009; von Gise and Pu, 2012; Hertig et al., 2008).
EMT starts with the dissociation of intercellular junctions and the loss of microvilli and apical-basal polarity, followed bythe acquirement of a front to back polarity and an increased migratory capacity. In the latest stages of EMT, the cell increases its capacity to degrade the basement membrane and to invade the fibrotic compact zone. Cells that have undergone a mesenchymal conversion possess increased capacity to synthesize extracellular matrix (ECM) components as well as a large number of pro-inflammatory, fibrotic and angiogenic factors, including vascular endothelial growth factor (VEGF), inducing angiogenesis (Boutet et al., 2006; Yoo et al., 2006; Lopez-Cabrera, 2014; Grande et al., 2015; Lovisa et al., 2015; Cho et al., 2017; Kida et al., 2007).
To control the pathological EMT, many drugs and molecular measures with variable results have been tested, but this therapeutic target remains a challenge for current medicine (Aguilera et al., 2005). Targeting EMT can be a really interesting weapon for the treatment of many fibroproliferative, cardiovascular and autoimmune diseases, and other pathologies such as cancer.
Molecular Mechanisms Involved in EMT Regulation
Mechanistically, EMT is a complex, dynamic and progressive process that affects the cellular architecture and requires a deep molecular reprogramming with new biochemical instructions (Lopez-Cabrera, 2014). The EMT process results from an integration of diverse signals transduction pathways and multiple triggering factors, including inflammatory cytokines, advanced glycation end products (AGEs), oxidative stress and hypoxia, transforming growth factor-β1 (TGF-β1)/Smads pathway, tyrosine kinase receptors, delta-like jagged Notch, caveolin (cav)-1, angiotensin receptor and integrins (Figure 1). A master molecule in the EMT induction appears to be TGF-β, nontheless the number of molecules and routes implicated in EMT is still growing (Lopez-Cabrera, 2014). The binding of the TGF-β1 to its “primary receptor” (receptor type II) permits the recruitment, trans-phosphorylation, and activation of the “signaling receptor” (receptor type I), also known as activin receptor-like kinase 5 (ALK5). Then, ALK5 is able to exert its activity to phosphorylate Smad2 and Smad3 (Masszi and Kapus, 2011). These receptor-activated Smads (R-Smads) form heterodimers with Smad4, a common mediator of the Smad pathways. These resulting Smad heterocomplexes are translocated into the nucleus, where they bind directly to DNA to regulate the transcription of target genes (Lopez-Cabrera, 2014). Another group of Smads, known as “inhibitory Smads” (e.g. Smad7), control TGF-β1-induced Smad signaling by preventing the phosphorylation and/or nuclear translocation of R-Smads and inducing receptor heterocomplex degradation (Lopez-Cabrera, 2014).
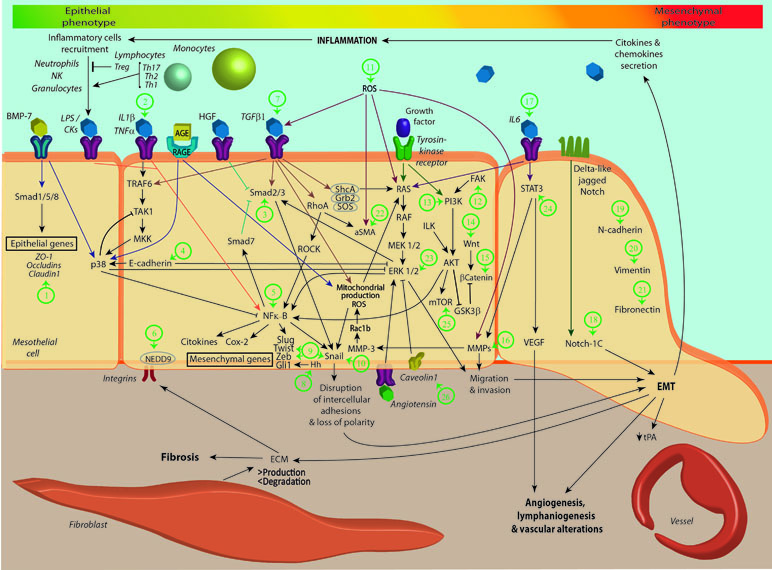
Figure 1 Key events during the development of epithelial-to-mesenchymal transition (EMT) and target pathways for therapeutic use of natural plant compounds (NPCs). The diagram shows key essential steps for the EMT course. Briefly, BMP7 and hepatocyte growth factor (HGF) are responsible for maintaining the cellular epithelial phenotype. Both molecules maintain E-cadherin and Smads1, 5, 7 and 8 expressions, and block Smads 2 and 3. On the other hand, inflammatory conditions triggered by exogenous or endogenous factors lead to a dysregulation of Th17/Treg equilibrium with IL6 synthesis that stimulates NFκB and induces EMT. Advanced glycation end products (AGEs) induce proteins structural crosslink, stimulate inflammatory response (monocytes), oxidative stress and finally uprregulation of Snail, leading to EMT. These effects are mediated through specific receivers called RAGES. Reactive oxigen species (ROS) generate oxidation and stimulate TGF-β, αSMA, RAS/RAF/MEK/ERK cascade and matrix metalloproteinases (MMPs) upregulation initiating cell migration. TGF-β initiates the classic route of EMT induction overexpressing Snail, Slug, N-Cadherin, Smads 2 and 3 and downregulating E-Cadherin and Smads 1, 5, 7 and 8. TGF-β also stimulates RhoA/ROCK and finally NFκB. Growth factors also stimulate the RAS/RAF/MEK/ERK cascade and MMPs upregulation, again inducing cell migration and invasion. Likewise they stimulate the P13k/ integrin-linked kinase (ILK)/ protein kinase B (Akt) cascade and GSK3β is blocked. Inflammatory cytokines such as IL6 also stimulates signal transducer and activator of transcription 3 (STAT3), vascular endothelial growth factor (VEGF) and MMPs inducing migration, invasion and angiogenesis. Finally Notch-1C directly induces mesenchymal genes such as N-Cadherin. The numbers in green indicate where each NPC acts. Many of them have an effect on several molecules or pathways. Depending on the target route over which the corresponding NPC acts, we have defined the following groups (green numbers). Group 1 acts by increasing ZO-1 expression: (Plumbagin), Group 2 acts by decreasing IL-1β action: (Baicalin), Group 3 acts by blocking Smad 2/3 phosphorilation; Paeoniflorin, Eupatolide, Gallic acid, Cairicoside E. Group 4 acts by up-regulating E-cadherin expression: α-solanine, Osthole, coumarin, Betanin, Cinnamomum cassia extracts, Genisteín, Withaferin A, Gedunin, Celastrus orbiculatus extract, Celastrol, Black rice anthocyanins, Duchesnea extracts, Cordycepin, Nitidine chloride, Phoyunnanin E, Epicatechin-3-gallate, Honokiol, Gallic acid, Piperlongumine, Brusatol, Berberine. Group 5 acts by inhibiting NFk-β signaling: Honokiol, Parthenolide, Baicalin and baicalein Group 6 acts by inhibiting NEDD9/Rac1 signaling: Acid plectranthoic. Group 7 acts by blocking TGF-β-1 signaling: Cryptotanshinone, Resveratrol, Oximatrina, Ligustrazina, Osthole, coumarin, Codonolactone, Betanin, Tannic acid, Cinnamomum cassia extracts Cairicoside E, Gentiopicroside, Genistein, Paeoniflorin, Gambogic acid, Arctigenin, Curcumin, Baicalin and baicalein, Baicalin and Cairicoside-E. Group 8 acts by downregulating hedgehog (Hn) signaling: Resveratrol, Sedum sarmentosum Bunge and extract Nitidine chloride Group 9 acts by decreasing Twist and ZEB expression: Resveratrol, Paeoniflorin, Jatrophone, Gedunin, Nitidine chloride, Plumbagin, Honokiol, Phoyunnanin E, Gallic acid, Piperlongumine, Brusatol, Nimbolide, Baicalin and baicalein. Group 10 acts by downregulation Snail expression: Resveratrol, Osthole, coumarin, Paeoniflorin, Gedunin, Celastrol, Nitidine chloride, Plumbagin, Phoyunnanin E, Piperlongumine, Berberine and Nimbolide. Group 11 acts by Inhibit Nrf2-mediated oxidative stress signaling pathway: Betanin, Salvianolic-acid-A and Plumbagin. Group 12 acts by Suppressing focal adhesion kinase (FAK)/AKT signaling: Phoyunnanin, Epicatechin-3-gallate, Gigantol and Eupatolide. Group 13 acts by blocking P13K/Akt cascade: Berberine, Nimbolide, and Curcumin. Group 14 acts by blocking Wnt/β-catenin Wnt signaling: Withaferin A, Jatrophone, Ginsenoside-Rb1 and Withaferin-A. Group 15 acts by down-regulated β-Catenin expression: Celastrol, Sulforaphane, Arctigenin, Plumbagin, Curcumin and Luteolin. Group 16 acts by down-regulating MMPs expression: α-solanine, Resveratrol, Cinnamomum cassia extracts, Paeoniflorin and Celastrus orbiculatus extract. Group 17 acts by inhibiting IL-6 activity: Baicalin and Polyphyllin-I. Group 18 acts by blocking Notch-1 signaling: Gedunin, Nimboliden and Luteolin. Group 19 acts by down-regulating N-cadherin expression: Tannic acid, Paeoniflorin, Gedunin, Celastrus orbiculatus extract, Celastrol Duchesnea indica, Cordycepin, Nitidine chloride, Honokiol, Phoyunnanin E, Gigantol, Gallic acid, Berberine and Nimbolide. Group 20 acts by down-regulating Vimentin expression: α-solanine Tannic acid, Cinnamomum cassia extracts, Paeoniflorin, Withaferin A, Jatrophone, Gedunin, Celastrus orbiculatus extract, Celastrol Black rice anthocyanins, Duchesnea indica, Nitidine chloride, Plumbagin, Phoyunnanin E, Gigantol, Gallic acid, Berberine and Nimbolide. Group 21 acts by down-regulation Fibronectin expression: Tannic acid, Cinnamomum cassia extracts, Jatrophone, Withaferin A, Black rice anthocyanins, Duchesnea indica, Epicatechin-3-gallate, Gallic acid and Berberine. Group 22 acts by down-regulating α-SMA expression: Betanin, Celastrol and Salvianolic-acid-A. Group 23 acts by blocking extracellular signal-regulated kinase (ERK) signaling: Arctigenin, Gigantol, Eupatolide and Nimbolide. Group 24 acts by blocking STAT- 3 signaling: Honokiol and Polyphyllin-I. Group 25 acts by blocking mammalian target of rapamycin (mTOR) signaling: Nimbolide. Group 26 acts by suppressing the cav-1 phosphorylation, stabilizating β-catenin: curcumin.
Among the R-Smads, Smad-3 appears to be the key mediator in TGF-β-induced fibrosis and EMT (Zhou et al., 2010). In this context, it has been shown that the inhibition of Smad3 activation and nuclear translocation blocks EMT (Zhou et al., 2010) and tissue fibrosis (Sato et al., 2003). Translocated Smad-3 into the nucleus controls TGF-β-responsive genes encoding integrin-linked kinase (ILK) (Massagué, 2000). The activation of ILK by β1-integrins lead to protein kinase B (Akt) and glycogen synthase kinase-3 (GSK-3)-beta phosphorylation (Massagué and Wotton, 2000).
Phosphorylated-Akt activates nuclear factor-κB (NF-κB) (Tan et al., 2002), which induces the expression of Smad-7 (Bitzer et al., 2000) emphasizing the self-regulated nature of the whole EMT process (Figure 1). On the other hand, phosphorylated-GSK-3β is inactive, what subsequently stabilizes β-catenin, released from the adherens junction, and activator protein-1 (AP-1) (D’Amico et al., 2000). When stabilized, β-catenin per se may induce EMT (Kim et al., 2002a), while AP-1 activates matrix metalloproteinase (MMP)-9 expression inducing the invasion of the ECM (Troussard et al., 2000).
The Smad-dependent pathways are not the only ways by which TGF-β1 regulate the EMT process. Smad-independent pathways also participate in TGF-β1-induced EMT. These pathways can either potentiate or modulate the outcome of TGF-β1-induced Smad signaling (Lopez-Cabrera, 2014). Amain Smad-independent signalling pathway activated by TGF-β1/receptor I interaction is the Ras homolog gene family member A (RhoA)/rho-associated, coiled-coil-containing protein kinase 1 (ROCK1) pathway. This route regulates cytoskeleton remodelling and cellular migration and invasion. RhoA also induces the expression of alpha smooth muscle actin (α-SMA) in a ROCK-independent manner (Masszi et al., 2003).
TGF-β1 also activates the H-Ras/Raf/extracellular signal-regulated kinase (ERK) pathway, necessary for the induction of transcription factor SNAIL1 expression and of EMT (Peinado et al., 2003; Barberà et al., 2004; Huber et al., 2004), cooperating with fibroblast growth factor (FGF), a potent inducer of the mentioned route (Peinado et al., 2003). SNAIL1 regulates EMT by inhibiting E-cadherin (Cano et al., 2000; Poser et al., 2001) and by inducing growth arrest and survival, which confer advantage to migrating transdifferentiated cells (Vega et al., 2004).
The Notch signalling pathway is another EMT-activating route, able to induce SNAIL1 and SNAIL2 expression, down-regulating E-cadherin. The TGFβ/Smads classical pathway is able to cooperate with different signaling routes. The association of the tumor necrosis factor (TNF)-receptor associated factor 6 (TRAF6) with the TGFβ receptor heterocomplex activates TGFβ-activated kinase 1 (TAK1) and, as a result, p38 and c-Jun N-terminal kinase (JNK) (Thiery et al., 2009). Other stimuli such as advanced glycation endproducts AGEs are able to induce EMT by acting on specific cellular receptors (RAGE) (Chen et al., 2016b). Reactive oxygen species (ROS) can also directly activate TGF-β, the production of ECM, MMP and RAS (Thiery et al., 2009) (Figure 1). Finally, the activation of mammalian target of rapamycin (mTOR) induces inflammatory processes mediated by T helper 17 (Th17) cells, TH17, which in turn also triggers EMT (Liappas et al., 2015).
Active Substances Derived from Plants Capable of Regulating EMT
Natural plant compounds (NPCs) have been used for many years as a source of therapeutic substances and a structural basis for drug elaboration. Unique architectures that can lead to novel therapeutic agents are provided by nature (Newman and Cragg, 2007; Song et al., 2014; Khan and Gurav, 2017).
NPCs are bioactive elements isolated from natural sources (plants) that can regulate the EMT through anti-inflammatory, anti-fibrotic or antioxidant mechanisms (Boldbaatar et al., 2017). Bioactive natural components are presented in this review which delves into their mechanisms of action against EMT (Table 1).
Arctigenin (ARC). It has been proposed as an anti-inflammatory and anti-cancer substance. In human lung cancer cells, ARC has been shown to inhibit TGF-β-induced phosphorylation, smad2/3 transcriptional activity, snail and N-cadherin expression, by contrast increasing the expression of E-cadherin in dose and time dependent manners. It blocks ERK-phosphorylation and β-catenin transcriptional activity. Through these mechanisms, ARC represses TGF-β-induced EMT (Xu et al., 2017).
Baicalin and baicalein. These molecules significantly decreased the TGF-β1-mediated EMT, by reducing the Slug expression and NF-κB signaling pathway in mammary epithelial cells. Likewise, both molecules decremented growth and cell migration capacities of human breast cancer cells (Chung et al., 2015). In the same way, baicalin also inhibited SMADs 2 and 3 phosphorylation and suppressed migration and invasion in pancreatic cancer cells (Zheng et al., 2016).
Berberine. It exhibits strong anti-cancer, anti-inflammatory, and anti-microbial effects (Tillhon et al., 2012). Kou et al. detected that berberine markedly upregulated E-cadherin and downregulated N-cadherin, fibronectin and vimentin expressions. Cadherin-bound β-catenin, which is required for cell adhesion, was also inhibited. The therapeutic espectrum of berberine also involved the downregulation of Snail, Slug and zinc finger E-box binding homeobox 1 (Zeb1) as well as the regulation of PI3K/Akt and retinoic acid receptor alpha and beta (RARα/RARβ) signaling, acting on the proliferation capacity of various cancer cells (Liu et al., 2015; Kou et al., 2016).
Betanin. It presents powerful anti-oxidative and anti-inflammatory activities. Studies from Sutariya and Brijesh showed that betanin reduces streptozotocin (STZ) fibrosis induced in diabetic nephropathy model, by modulating EMT associated markers such as of TGF-β, type IV collagen, α-SMA and E-cadherin expression. Therefore, betanin can efficiently suppress renal fibrosis in diabetic nephropathy and may slow down the advancing to end-stage renal disease by regulating TGF-β pathway (Sutariya and Brijesh, 2017).
Black rice anthocyanins (BRACs). These NPCs are extracted from the black rice, considered as a healthy food due to its effects on the liver and gastrointestinal tract (Kong et al., 2012). Anthocyanins happen to have potential beneficial effects such as antioxidant, anti-inflammatory, anti-cancerous and anti-metastatic effects (Hou, 2003; Sehitoglu et al., 2014). Zhou et al. observed that focal adhesion kinase (FAK) signaling pathway plays a function in the anti-metastatic properties of BRACs, decreasing the adhesion, migration and invasion of human HER-2-positive breast cancer cells in vitro. Likewise, these NPCs significantly modified the morphology of those cells from a mesenchymal to an epithelial phenotype. BRACs elevated the expression of E-cadherin and reduced the expression of fibronectin and vimentin (Zhou et al., 2017).
Brusatol (BR). This NPC strongly inhibits pancreatic tumor action in vitro (Zhao et al., 2011). Research sugests that BR could sensitize the current first-line chemotherapeutic agents to pancreatic cancer via inhibition of the EMT process. It has been proven that BR increased E-cadherin while decreasing vimentin protein expressions, and also reducing Twist mRNA expression (Lu et al., 2017).
Carnosol (CAR) is a naturally occurring phenolic diterpene located in several Mediterranean herbs and is a main component of rosemary (Rosmarinus officinalis L). It has been reported that the CAR anti-proliferative actions is preferentially directed towards cancer cells, as reported in both animal and in vitro models. Furthermore, CAR presented a favourable therapeutic option in glioblastoma cells (Johanson, 2011; Vergara et al., 2014; Giacomelli et al., 2016). CAR could interfere with the diverse processes implicated in cancer resistance and aggressiveness, such as cancer stem cells (CSC) formation, proliferation and self-renewal. Fascinatingly, also diminished the influence of the cancer microenvironment by reducing the cytokine- induced EMT that underlies the possession of the mesenchymal phenotype. Likewise, CAR had the ability to reactivate the p53 functionality promoting CSC proliferation control and decreasing EMT was highlighted for the first time (Giacomelli et al., 2017).
It also possesses anti-cancer effects on several tumor types. It has shown to promote apoptotic cell death through p53 functional reactivation and to control the TNF-α/TGF-β-induced EMT, counteracting the effects of the cytokine on EMT master regulator genes (Slug, Snail, Twist and ZEB1). It has also been demonstrated that CAR is able to modulate the activation of miR-200c, a key player in the EMT process. Finally, CAR increase the temozolomide anti-proliferative effects in vitro (Giacomelli et al., 2017).
Cairicoside E (CE). It has been published that this compound down-regulates the Aquaporin-5 (AQP5) expression and suppressed the EMT process in colon-rectal cancer cells. Research suggested that TGF-β1 increased the expression of AQP5 and activated the EMT byincreasing the expressions of p-Smad2/3, while silence of AQP5 with CE blocked the levels of p-Smad2/3 (Chen et al., 2017a).
Celastrol. It is a pharmacologically active element that demonstrates significant therapeutic actions in chronic inflammatory, autoimmune, cancer, and neurodegenerative disorders (Allison et al., 2001; Dai et al., 2010; Ge et al., 2010; Venkatesha et al., 2011; Wong et al., 2012). Recently, Divya et al. suggested that celastrol decreased the N‐cadherin, snail, slug, vimentin and β‐catenin expression in a Bleomycin‐induced lung fibrosis rat model. They, likewise, feature this anti-EMT effect to the inhibition of heat shock protein 90 inhibition (Divya et al., 2018). Other research established that celastrol suppresses inflammatory reactions, as well as regulates oncogenic proteins including β-catenin. Celastrol also decreases pro-inflammatory cytokines (TNF-α, IL-1β and IL-6) serum concentrations, down-regulates cyclooxygenase 2 (COX-2), inducible nitric oxide synthase (iNOS), N-cadherin, Vimentin and Snail expressions, inactivates NF-κB and upregulates E-cadherin (Lin et al., 2015).
Celastrus orbiculatus Thunb extract (COE). The extracts from the stems of this plant constitute 11 compounds (Li et al., 2012a). The ethyl acetate of COE constrains the proliferation, EMT (targeting VEGF, activating MAPK and inhibiting Akt signaling pathways), invasion and metastatic faculties of tumor cells (Qian et al., 2012; Zhang et al., 2012a). Moreover, COE is used in the antiinflammatory and analgesic handling of various diseases. In human gastric cancer AGS cells, it has been observed that Cofilin 1, Ncadherin, vimentin, MMP2 and MMP9 proteins expressions were significantly reduced by COE, whereas the Ecadherin expression was increased (Wang et al., 2017b).
Cinnamomum cassia extracts (CCE). Fifteen compounds were isolated from the bark extract of C. cassia growing in China (Zhao et al., 2013). These extracts were shown to diminish the TGF-β1-induced motility and invasive capacities of A549 and H1299 cells by inhibiting MMP-2 and urokinase-type plasminogen activator. Moreover, they impaired cell adhesion associated with collagen production. CCE also down-regulated vimentin and fibronectin and upregulated E-cadherin expressions (Lin et al., 2017).
Codonolactone (CLT). It inhibited the expression of acquired EMT’s mesenchymal markers such as N-cadherin and vimentin in a dose-dependent manner in in vivo and in vitro experiments in breast cancer. Likewise, it blocked the expression of transcription factors Snail, Slug, Twist-1 (TGF-β signaling) and the Runx2 phosphorylation (Fu et al., 2016).
Cordycepin. Its properties have been evaluated on a human oral squamous cell carcinoma xenograft model, through its administration in a regular, low-dose upregulated E-cadherin and downregulated N-cadherin protein expressions, inhibiting EMT (Su et al., 2017).
Cryptotanshinone (CTS). It exhibits multiple pharmacological benefits, involving anti-cancer (Li et al., 2015; Zhu et al., 2016), anti-oxidative stress (Jin et al., 2013), and anti-cardiac fibrosis properties via downregulation of COX-2, NADPH oxidase 2 and 4 and MMP-2 (Ma et al., 2012; Ma et al., 2014). The antifibrotic mechanism proposed for CTS is the inhibition of Smad2 phosphorylation. Although it did not inhibit Smads 3 and 4 or mitogen-activated protein kinase (MAPK) signals, the ECM accumulation was importantly reduced in a renal fibrosis model (Wang et al., 2017c).
Curcumin. This NPC inhibit EMT in CoCl2-treated hepatocytes. This action might be due to its capacity to diminish TGF-β-R1 expression in these cells. This effect suggests a disruption on the downstream signal transduction transmitted by SMADs pathway (Kong et al., 2015). Moreover, it was found that SMADs2 and 3 phosphorylation was inhibited by curcumin, pointing its powerful action blocking upstream the EMT pathway signaling. Another mechanism by which curcumin inhibits these pathways is by suppressing the cav-1 phosphorylation, stabilizating β-catenin (Sun et al., 2014). Recently, it has been published that curcumin inhibits the superoxide dismutase-induced invasion and migration of pancreatic cancer cells by inhibiting the PI3K/Akt/NF-κB signaling pathway (Li et al., 2018b). Curcumin was not related to toxicity including high dose administration, in human clinical trials (Gupta et al., 2013; Hewlings and Kalman, 2017).
Jatrophone (JA). This diterpene shows a broad assortment of biological actions, counting antitumoral, cytotoxic, anti-inflammatory, anti-malarial and fungicidal properties (Devappa et al., 2010). It has been demostrated that JA reduces mRNA expression of Slug, fibronectin and vimentin, but not ZEB1, and also exhibits an anti-proliferative and anti-migratory effect acting on Wnt/β-catenin signaling in triple-negative breast cancer (Fatima et al., 2017).
Duchesnea extracts.Duchesnea chrysantha and Duchesnea indica belong to the Rosaceae family, and their extracts show a diversity of biological properties, involving anti-biotic, anti-oxidative, anti-inflammatory and some cytotoxic features (Kim et al., 2002b; Kim et al., 2007). These extracts involve a range of chemical compounds such as triterpenes, triterpene glycodides, flavonoid glycodides and sterols (Lee et al., 1994; Qiao et al., 2009). An in vivo research showed that tumor growth was importantly diminished in BALB/c nude mouse xenograft model orally treated with Duchesnea indica extracts (DIE) (Chen et al., 2017c). In the same study, DIE also inhibited highly metastatic cells by reducing the secretions of MMP‐2 and urokinase‐type plasminogen activator (uPA) (Chen et al., 2017c). It was also able to decrease the cell adhesion capacity, down-regulate the N-cadherin, fibronectin, and vimentin and increase the E-cadherin expression (Kim et al., 2002b; Kim et al., 2007; Chen et al., 2017c). Another protective mechanism related with DIE is its antioxidant action which can also modulate the EMT (Hu et al., 2009; Hu et al., 2011).
Epicatechin-3-gallate (ECG). It elicits several anti-oxidant and anti-inflammatory activities and is one of the four types of catechins mainly detected in green tea, together with epicatechin, epigallocatechin and epigallocatechin-3-gallate (EGCG) (Chowdhury et al., 2016). In human lung cancer cells, ECG also reverts the TGF-β1-induced EMT by upregulating epithelial markers (E-cadherin) and downregulating mesenchymal markers (fibronectin). Moreover, it also phosphorylates FAK. Based on these facts, it has been recommended that ECG may be administered as an effective agent against TGF-β1-induced EMT (Huang et al., 2016).
Eupatolide. It shows anti-inflammatory, anti-proliferative and anti-migratory effects (Lee et al., 2010a; Kim et al., 2013). It has also been suggested that eupatolide might be employed as an inhibitor of the TGF-β1 signaling pathway to suppress EMT (Wrighton et al., 2009). Moreover, eupatolide suppress TGF-β1-induced EMT via downregulation of Smad3 phosphorylation and decreasing the TGF-β type 1 receptor (Boldbaatar et al., 2017).
Gallic acid.In vivo experiments with this NPC diminished vascular calcification, cardiac hypertrophy, cardiac fibrosis and hypertension. Gallic acid also inhibited pathological changes in the lungs, such as pulmonary fibrosis (Kee et al., 2014; Ryu et al., 2016). Moreover, it reduced the expression of fibrosis-related genes, including collagen types I and III, fibronectin, connective tissue growth factor (CTGF), and Smad3. In a mouse model, Garlic acid blocked the of EMT-related genes expression, such as N-cadherin, vimentin, Snail, and TWIST1 (Jin et al., 2017).
Gambogic acid (GA). It has been proved in vitro that this compound reverses TGF-β1-mediated EMT and endothelial–mesenchymal transition (EndoMT) in human lung fibroblasts (HLF-1). It also prevents pulmonary fibrosis in vivo and attenuates the EMT by modulating the TGFβ1/Smad3 pathway (Qu et al., 2016).
Gedunin. It has been shown to have potential anti-cancer activity (Kamath et al., 2009; Patwardhan et al., 2013; Hao et al., 2014). Recent research suggests that gedunin inhibits EMT by reducing the expression of the mesenchymal markers N-Cadherin, Slug, Snail, Vimentin, Notch 1 and 2, and Zeb whereas increasing the E-cadherin expression (Subramani et al., 2017).
Genistein (GEN). Soybeans and most soy products contain large amounts of isoflavones called soy phytoestrogens, and one of the most concentrated is the GEN (Lee et al., 2012). GEN is a phytoestrogen known for its chemopreventive effects in several types of cancers (Kim et al., 2015). It suppresses the EMT response induced by 17β-estradiol and two estrogens-like compounds, bisphenol-A and nonylphenol. Thus, it reduces the protein expressions of vimentin, cathepsin D, and MMP-2, increases E-cadherin expression and downregulates TGF-β. (Kim et al., 2015). In ovarian cancer derived cells, GEN inhibits the NF-κB and Akt signaling pathways, playing important roles in keeping the homeostatic balance between cell survival and apoptosis. It has been considered as a potencial antiangiogenic, antioxidant and anticancer agent (Han et al., 2012; Dai et al., 2015).
Gentiopicroside (GPS). It has been proved that in bronchoalveolar cells isolated from fluids of lungs pulmonary fibrosis in a mouse model, GPS decreased the levels of proinflammatory cytokines, including TNF-α and IL-1β, and downregulated TGF-β1 and CTGF expression. In vitro, GPS inhibited the EMT of A549 cells stimulated by TGF-β1 to induce transdifferentiation at a dose-dependent manner (Chen et al., 2017b).
Gigantol. It has been described to have anti-proliferative, anti-apoptosis and anti-metastatic properties (Charoenrungruang et al., 2014; Klongkumnuankarn et al., 2015). Recent publications suggest that gigantol considerably reduces lung cancer cells’ viability in a detached condition. It also shrinkages EMT biomarkers including N-cadherin, vimentin and Slug, leading to a meaningful suppression of AKT, ERK, and cav-1 survival pathways (Unahabhokha et al., 2016).
Ginsenoside. It has been registered in pharmacopeias for thousands of years due to its abundant content of saponins. One of the most extensively known saponins in the rhizome of ginseng is ginsenoside-Rb1 (Jia and Zhao 2009). In vivo studies mention that Rb1 showed cardioprotective, hepatoprotective and anti-inflammatory effects (Wang et al., 2008; Li et al., 2012b; Cheng et al., 2013; Hou et al., 2014). Likewise, it inhibits cell proliferation, angiogenesis and apoptosis stimulation (Zheng et al., 2013; Lee et al., 2016). A recent publication shows that ginsenoside-Rb1, especially its metabolite compound K, particularly sensitize cancer stem/tumor-initiating cells from ovarian cancer to chemotherapy through the inhibition of Wnt/β-catenin signaling and EMT (Deng et al., 2017).
Honokiol (HNK). It has been associated with anti-tumor and more recently anti-EMT effects (Fujita et al., 1973; Lee et al., 2005; Ahn et al., 2006; Sheu et al., 2008; Arora et al., 2011; Arora et al., 2012; Nagalingam et al., 2012). For instance, in breast cancer cells, Avtanski et al. demonstrated that HNK inhibited signal transducer and activator of transcription 3 (Stat3) phosphorylation and transactivation activity and Zeb1 expression, which plays a main role in EMT initiation. More than that, HNK induces an increase in E‐cadherin (Avtanski et al., 2014). Additionally, it has been published that HNK inhibits EMT motility and migration by targeting cellular FLICE (FADD-like, IL-1β-converting enzyme)-inhibitory protein (c-FLIP), considered a master anti-apoptotic regulator in non-small-cell lung cancer (Lv et al., 2016).
Isoviolanthin extracted from the leaves of Dendrobium officinale inhibits transforming growth factor (TGF)-β1-induced EMT in hepatocellular carcinoma (HCC) cells, it is the most significant constituents responsible for the antimetastasis activity of Dendrobium officinale. Recent publications report that isoviolanthin targets the TGF-β/Smad and PI3K/Akt/mTOR pathways to repress TGF-β1-induced EMT phenotypes in HepG2 and Bel-7402 HCC cells. Furthermore, these results confirm that isoviolanthin could be a favorable natural compound with low toxicity for the treatment of metastatic HCC by affecting TGF-β1-induced EMT (Xing et al., 2018).
Ligustrazine (LIG). In a model of renal tubulointerstitial fibrosis, LIG showed pleyotropic effects acting at different levels of EMT induction. LIG decreased the mRNA expression of TGF-β1, CTGF, monocyte chemoattractant protein-1 (MCP-1) and osteopontin, and, subsequently cytokeratin-18 expression decreased. Mainly, this molecule increased the expression of the natural inhibitors of TGF-β, hepatocyte growth factor (HGF) and bone morphogenetic protein (BMP)-7 (Yuan et al., 2012).
Luteolin. Many biological properties of luteolin, such as anti-inflammation, anti-allergy, antioxidant, anticancer and anti-microbial effects have been described (Chung et al., 2001; Chen et al., 2007; Lin et al., 2008). In breast cancer (in vivo and in vitro), epithelial markers such as E-cadherin and claudin were upregulated in response to luteolin while mesenchymal markers N-cadherin, vimentin, Snail and Slug were downregulated at dose-dependent manner. Researchers found that these positive effects of luteolin were extinguished by overexpression of β-catenin, indicating that downregulation of β-catenin expression may mediate the inhibitory effects of luteolin on EMT (Lin et al., 2017). Same results were found by Zang et al., who described that other pathways such as Notch1 were also blocked by Luteolin (Zang et al., 2017).
Nimbolide. Recent studies indicate that treatment with this agent reduces the expression of Notch-2, N-cadherin, vimentin and transcription factors (Snail, Slug and Zeb) in pancreatic cancer cell lines. Moreover, nimbolide treatment likewise increased the expression of E-cadherin. Additionally, the generation of ROS mediated by nimbolide reduces cell proliferation (via reduction of PI3K/AKT/mTOR and ERK signaling) and metastasis (via reduction of EMT, invasion, migration and colony forming abilities) through mitochondrial-mediated apoptotis but not through autophagy (Bodduluru et al., 2014; Hao et al., 2014; Subramani et al., 2016).
Nitidine chloride (NC). It has been shown to exert antimalarial (Bouquet et al., 2012), anti-inflammatory (Wang et al., 2012), anti-angiogenic (Chen et al., 2012), and anticancer effects (Fang et al., 2013). Likewise, NC inhibited the cellular migration and invasion through suppression of FAK-associated pathway in breast cancer metastasis (Sun et al., 2014). It has also been recently proposed that inactivation of Hedgehog signaling pathway by NC led to significantly decreased Smo and Gli expressions, targeting breast cancer metastasis. Thus, NC could be suitable for the prevention and treatment of breast cancer through dual-blocking EMT (Sun et al., 2016).
Osthole. It inhibits growth and metastasis in many types of cancer (Kao et al., 2012; Zhang et al., 2012b; Ding et al., 2014). It has also been proposed that osthole mediated the EMT by downregulating Snail and cell-invasive capability, suppressing the TGF-β/Akt/MAPK pathway (Wen et al., 2015).
Oxymatrine (OM). Many studies have proved that OM shows an anti-fibrotic effect on liver, pulmonary, myocardial and skin scar tissue fibrosis through inhibition of the TGF-β1/Smad signaling cascade (Shi and Li, 2005; Chen et al., 2008; Wu et al., 2008; Shen et al., 2011; Fan et al., 2012; Liu et al., 2012). Thus, Liu et al. demonstrated that OM inhibits the high glucose-induced renal tubular EMT, decreasing the degradation of SnoN mediated by a E3 ubiquitin ligase (Arkadia), and that promotes EMT amplifying TGF-β signalling through Smad7 degradation (Liu et al., 2016).
Paeoniflorin (PF). Pharmacological reports have shown that it prevents pulmonary EMT inhibiting collagen type-I synthesis, downregulating Snail and Slug and up-regulatining Smad7. These properties provide PF a protective action against cellular transdifferentiation in a lung bleomycin-induced fibrosis model in mice (Ji et al., 2016). It has also been demonstrated that PF down-regulates TGF-β1, maintains BMP-7 expression and inhibits Smad2/3 in a renal fibrosis model (Zeng et al., 2013). Likewise, PF blocks EMT in gliblastoma cells, and reduces TGF-β, Snail, N-cadherin, Vimentin and MMP2/9 expression at doses depended manner (Wang et al., 2018).
Paeonol. It is an aspirin analogue extracted from numerous medicinal herbs including Moutan Cortex, Cynanchi paniculati Radix et rhizome, and Paeoniae Radix rubra. Paeonol was discovered to present comprehensive pharmacological activities, such as antioxidant, anti-inflammatory, anti-aging, and anti-cancer activities (Chou, 2003; Zhang et al., 2015). Another author reported that paeonol influenced antioxidative stress activity in endothelial cells by controlling the expressions of Sirt1. It has been described, too, that paeonol ameliorated colitis related colorectal cancer by suppressing cytokine-induced EMT and NF-jB activation (Lin et al., 2014). Likewise, suggested that paeonol inactivated ERK and TGF-beta1/Smad pathway leading to regulation of relevant EMT markers. These results suggest that paeonol might be developed as a potential agent used for oxidative stress injury and EMT in premalignant lesion (Yang et al., 2018).
Parthenolide (PTL). It has been conventionally used for the treatment of headaches and arthritis. Recent analyses suggest that PTL is a valuable antitumor and anti-inflammatory NPC, and it was evaluated in clinical studies for leukemia and neurological tumors (Ghantous et al., 2013). These effects of PTL in tumors and inflammatory diseases primarily happen via the inhibition of NF-κB signaling pathways (Hehner et al., 1999). Current studies have established that PTL inhibit pulmonary fibrosis increasing E-Cadherin and decreasing vimentin NF-κB and Snail expression in TGF-β1-treated primary lung epithelial cells (Li et al., 2018a).
Plectranthoic acid (PA). It induces cell cycle arrest and apoptosis in prostate cancer cells (Akhtar et al., 2016). Recent research demonstrates that PA-exposed cells exhert considerably reduced cell migration capacity and a reversal of TGF-β induced EMT, representing the potential effectiveness of PA against prostate cancer, throughout regulation of Rac1 signaling (Akhtar et al., 2018).
Phoyunnanin-E. Recent publications suggest that Phoyunnanin E decreased the E-cadherin to N-cadherin switch and reduced upregulation of mesenchymal markers such as vimentin and snail, as well as slug expression. Phoyunnanin-E has also been shown to inhibit migration and growth and promote EMT suppression, reduce migratory-associated integrins αv and β3, and suppress FAK/AKT cascade, which subsequently suppressed downstream migratory proteins in lung cancer cells (Petpiroon et al., 2017).
Piperlongumine (PL). It has been identified as a powerful cytotoxic element highly selective to cancer cells (Raj et al., 2011; Bezerra et al., 2013; Liu et al., 2014; Zheng et al., 2016; Zhou et al., 2016). PL has also been demonstrated to accurately suppress bladder cancer development both in vitro and in vivo, via inhibition of EMT. Thereby, the expression of EMT-associated factors such as Slug, β-catenin, zeb1, N-Cadherin, Claudin-1, and zonula occludens-1 (ZO-1) were importantly decreased (Liu et al., 2017).
Plumbagin (PLB). It presents anti-inflammatory, anti-atherosclerotic, anti-bacterial, anti-fungal, and anti-cancer properties shown both in vitro and in vivo (Padhye et al., 2012). The anti-EMT effect of the PLB can be vinculated by its ability to adjust epithelial adherent junctions in human tongue squamous carcinoma cells. PLB also boosted the expression of E-cadherin and decreased of N-cadherin in these cells. Moreover, it reduced the expression of Snail, Slug, TCF-8/zeb1, β-catenin, and vimentin, whereas increased the expression of claudin-1 and ZO-1. Notably, PLB inhibited the translocation of nuclear factor erythroid 2-related factor (Nrf2) from cytosol to nucleus, causing an inhibition in the expression of downstream targets (Pan et al., 2015).
Polyphyllin (PP) I. It has been broadly investigated for its anti-inflammatory and anti-cancer activities. PPI exhibited inhibitory effect on various cancer types, involving hepatocarcinoma (Ong et al., 2008), non-small cell lung cancer (Kong et al., 2010), osteosarcoma (Chang et al., 2015), chronic myeloid leukemia (Wu et al., 2014), ovarian cancer (Gu et al., 2016) and glioma cells (Yu et al., 2014). Recent investigation described that PPI was capable to reverse EMT in osteosarcoma cells (Chang et al., 2015). Likewise, ZH-2, a compound derived from PP VII, exherts anti-chemoresistance properties through inhibiting EMT (He et al., 2016). In anacquired-erlotinib-resistant cell line, PPI inhibited IL-6/STAT3 signaling pathways and stimulates epithelial marker expression, reversing EMT. Significantly, PPI exhibited an inhibitory effect on epidermal growth factor (EGF) receptor tyrosine kinase inhibitors, which has a mutagenic and pro-EMT action in non-small cell lung cancer (Lou et al., 2017).
Resveratrol (RSV). RSV has been published to have many pharmacological activities, such as protection against coronary heart disease, anti-inflammatory properties, chemo-prevention of cancer, anti-oxidative and antiasthmatic effects (Frémont, 2000; Wallerath et al., 2002; Aggarwal et al., 2004; Bisht et al., 2010). It can be obtained from grapes, wine, mulberries and peanuts (Shakibaei et al., 2009). It also reduced renal injury and renal fibrosis by suppressing the inflammatory activity and by inhibiting lipid peroxidation (Chander and Chopra, 2005; De Jesus et al., 2007). The inhibitory effect of RSV on EMT has been demostrated in prostate (Li et al., 2014), ovarian (Baribeau et al., 2014),breast (Tsai et al., 2013) and pancreatic cancer (Li et al., 2013). Recent papers show that RSV inhibits EMT in renal tubular cells by antagonizing the hedgehog signaling pathway (Bai et al., 2014b). Likewise, Gao et al. suggested that RSV prevents from cancer cell invasion and metastasis in vitro by inhibiting the hedgehog pathway and EMT (Gao et al., 2015). In this context, RSV downregulates the EMT-inducting transcription factor (including Zeb-1, Slug and Snail) to reduce migration and invasion in pancreatic cancer cells (Shankar et al., 2011). EGF is a well-known EMT-inducer in human breast cancer cells (Ackland et al., 2003; Vergara et al., 2011). RSV blocks EGF-induced EMT by repressing EGF-induced ERK (Vergara et al., 2011). Furthermore, it is known that renal injury has a close relationship with the development of renal fibrosis and, during this process, tubular epithelial cells in the kidney undergo EMT via upregulating β-catenin/lymphoid enhancer-binding factor 1 (LEF1) signaling and MMP-7 (Liu, 2004; Shibata et al., 2009). A current study using RSV showed that this product attenuated renal injury and fibrosis through inhibition of EMT. Authors suggested that this inhibition was due to the fact that RSV up-regulated sirtuin 1 (SIRT1), which deacetylated Smad4 and inhibited the expression of MMP-7 (Xiao et al., 2016). Other findings also demonstrate that RSV modulates EMT by suppressing MMP-2 and MMP-9 via MAPK and NF-κb signals in lung cancer invasion and metastatic cells (Yang et al., 2009; Liu et al., 2010a). Moreover, RSV has been recently shown to limit EMT by controlling gene expression at post-transcriptional level (it favors the epithelial-type alternative splicing of pre-mRNAs that encode crucial factors in adhesion and migration, and enhances the expression of some RNA-Binding Proteins) (Moshiri et al., 2017). It also inhibits TGF-β1-induced EMT and suppresses lung cancer invasion and metastasis (Wang et al., 2013)
Salvianolic acid A (SAA). It exerts many pharmacological actions, such as myocardial protection, anti-thrombosis, anti-fibrosis, and the prevention of diabetes complications (Ho and Hong, 2011; Xu et al., 2014). Investigations have revealed that SAA treatment effectively decreased lung parenchymal injury and collagen deposition and diminished the apoptosis and lung fibrosis on a pulmonary arterial hypertension rat model. Furthermore, in pulmonary tissue, SAA treatment upregulated BMP type II receptor (BMPRII) expression and augmented the Smad1/5 phosphorylation. Both molecules showed an anti-EMT effect (Chen et al., 2016a). An anti-EndoMT capacity was also discovered in bleomycin-induced pulmonary fibrosis in mice, acting on Nrf2/HO-1 signaling pathway (Chen et al., 2017d).
Sedum sarmentosum Bunge (SSBE). Pharmacological reports have shown that SSBE has significant antiinflammatory, anti-tumor and anti-angiogenic effects (Oh et al., 2004; Morikawa et al., 2007; Ninomiya et al., 2007; Jung et al., 2008). Other authors demonstrated that SSBE has marked effects against renal fibrosis (Bai et al., 2014a; Bai et al., 2014b), down-regulating hedgehog signaling pathway (which promotes renal fibrogenesis fostering the formation of myofibroblasts from different cell types through an EMT process). SSBE also reduced the ECM accumulation and angiogenesis (Bai et al., 2017).
Sulforaphane. Numerous studies have observed the effects of sulforaphane in control of tumor generation or cancer progression, such as in lung, breast and prostate (Amjad et al., 2015; Atwell et al., 2015; Jiang et al., 2016), and also digestive system neoplasms (Jeon et al., 2011; Kim et al., 2015). Other authors demonstrated that reduced expression of the micro RNA miR-616-5p, transcriptionally induced by sulforaphane management, contributes to the suppression of EMT in non-small cell lung cancer and in lung cancer metastasis through the miR-616-5p/GSK3β/β-catenin signaling pathway (Wang et al., 2017a).
Tannic acid (TA). This molecule acts upstairs in the EMT induction process, in lung epithelial cells. It reduces the expression of TGF-β and N-cadherin and decreases the SMADs 2 and 3 phosphorylation and the production of ECM (fibronectin and vimentin). Moreover, cell proliferation in G0/G1 phase and the mitogenic activity of protein kinase (ERK1/2, JNK1/2, and p38) also decrease (Pattarayan et al., 2018).
Withaferin-A (WA). Pharmacological reports have shown anti-cancer effects in rodent experiments (Padmavathi et al., 2005; Garodia et al., 2007; Widodo et al., 2007). Withanolide-D (witha-D) is an active element of WA that partially inhibits EMT acting on Wnt/β-catenin signaling and recovering E-Cadherin expression in a human pancreatic tumour cell line (Chaurasiya et al., 2008; Sarkar et al., 2014).
Alpha-Solanine. This NPC presents pharmacological activities involving anti-proliferation, anti-apoptosis and anti-angiogenesis (Mohsenikia et al., 2013). Alpha-solanine also reduced ERK and PI3K/Akt phosphorylation. Likewise, this component also reduces the expression of MMP-2/9 and vimentin and induces the expression of E-cadherin (Shen et al., 2014).
Potential Therapeutic Effects of Natural Plants Compounds
Currently, there is growing evidence for potential plant-derived compounds as inhibitors in several stages of tumourgenesis and inflammatory and fibrosis processes. In several clinical trials it has been demonstrated that NPCs have elicited anti‐aging, anti‐cancer and other health‐enhancing effects. A key target of the effects of NPCs may be in suppressing oxidative stress and the induction of 5′AMP-activated Kinase (AMPK), or suppression of the WNT/beta-catenin, PI3K/Akt/mTOR and RAS/MEK/ERK signaling pathways, among others, which results in cell death or prevents aging, diabetes, cardiovascular, cancer and other diseases (McCubrey et al., 2017).
One NPC is Berberine, which has been tested in a wide spectrum of clinical applications. Oral administration of berberine significantly reduced the familial adenomatous polyposis patients’ polyp size along with the inhibition of cyclin D1 expression in polyp samples. These statements suggest that berberine inhibits colon tumour formation through inhibition of Wnt/β-catenin signalling and might be a favorable drug for the prevention of colon cancer (Zhang et al., 2013; Farooqi et al., 2019). Additionally, it has been described that Berberine shows an extensive array of pharmacological effects, being effective against gastroenteritis, abdominal pain and diarrhea, and having antimicrobial, antidiabetic and antiinflammatory properties (Imanshahidi and Hosseinzadeh, 2008; Kulkarni and Dhir, 2010; Vuddanda et al., 2010). Another beneficial effect of berberine has been reported on the treatment of type II diabetes (Yin et al., 2008). This natural compound has an explicit potential as a drug in a wide spectrum of already defined clinical purposes (Tillhon et al., 2012). Numerous pharmacological reports have suggested the cardiovascular effects of berberine and B. vulgaris, such as preventing ischemia induced ventricular tachyarrhythmia, improving cardiac contractility and lowering peripheral vascular resistance and blood pressure (Marin-Neto et al., 1988).
Likewise, RSV is being examined in many clinical trials, on age-related disease, cancer, cardiovascular problems, chronic renal insufficiency and other disorders (Boocock et al., 2007; Brown et al., 2010; la Porte et al., 2010; Howells et al., 2011; Popat et al., 2013).
Clinical trials show that RSV has been shown to activate sirtuins and such activation is able to explain most of the beneficial properties of the mediterranean diet (MD). While observational studies and meta-analysis have demonstrated an antiageing effect of MD accompanied by a reduced risk of age-related pathologies, such as cardiovascular, metabolic and neurodegenerative diseases, as well as cancer (Russo et al., 2014; Gliemann et al., 2016).
Other studies that involved healthy volunteers established that RSV synchronized the carcinogen metabolizing enzyme cytochrome P450 and phase II detoxification enzymes, which repressed carcinogen metabolism and subsequently prevented carcinogenesis (Chow et al., 2010).
In the same way, Curcumin is being evaluated in numerous clinical trials for various disorders such as acute kidney injury, neurodegenerative diseases, cancer cardiovascular abnormalities, psychiatric disorders, osteoarthritis, type 2 diabetes mellitus, ulcerative colitis, rheumatoid arthritis, lupus nephritis, multiple sclerosis and other health problems (Allegra et al., 2017; White and Lee, 2019; Yang et al., 2019). Its efficacy appears to be related to the induction of glutathione S-transferase enzymes, inhibition of prostaglandin E2 (PGE2) production, or the suppression of oxidative DNA adduct formation. Oral curcumin was administered to patients with advanced colorectal cancer refractory to standard chemotherapies to explore its pharmacodynamics in humans (Sharma et al., 2004). In this study, the authors concluded that administration of 0.5 to 3.6 g/day for up to 4 months is associated with mild diarrhea as its only toxicity, and that a dosis of 3.6 g/day generates detectable levels of parent compound and conjugates in plasma and urine, causing inhibition of PGE2 production in blood leukocytes measured ex vivo. They proposed that an oral dose of 3.6 g/day is suitable for evaluation in Phase II trials (Sharma et al., 2004). In fact, curcumin has been found to be safe when administered at doses up to 10 g/day. All of these studies suggest that curcumin has enormous potential in the prevention and therapy of cancer (Aggarwal et al., 2003). Another study showed that curcumin is not toxic to humans up to 8 g/day when taken orally for 3 months (Cheng et al., 2001).
Likewise, epigallocatechin-3-gallate (EGCG) have been studied in a wide range of illnesses related to excessive oxidative stress, involving cancers, cardiovascular diseases, metabolic syndromes, diabetes, cerebral ischemic stroke, lung diseases, and neurodegenerative disorders (Chowdhury et al., 2016). Recently, EGCG has been studied for management and prevention of various kidney diseases, which are usually associated with oxidative stress and inflammation (Bao and Peng, 2016; Kanlaya and Thongboonkerd, 2019).
Meanwhile, baicalin decreases blood lipids and inflammation in patients with coronary artery disease and rheumatoid arthritis, supporting its further clinical application (Hang et al., 2018). This NPC exhibits high clinical value, having anti-inflammatory, anti-arrhythmic and anti-hypertensive effects (Huang et al., 2005; Huang et al., 2006; Dinda et al., 2017).
The therapeutic usefulness and anti-inflammatory properties of celastrol have been studied in numerous inflammatory diseases, involving rheumatoid arthitis, ankylosing spondylitis, systemic lupus erythematosus, inflammatory bowel disease, osteoarthritis, allergies, and skin inflammation (Cascão et al., 2017). Celastrol exhibits beneficial effects decreasing cardiovascular symptoms involving hypertension. Researchers investigated the treatment outcome against preeclampsia with a combined use of celastrol and nifedipine in clinical trials. A total of 626 patients with preeclampsia were enrolled, screened, and assigned randomly to groups receiving either nifedipine + placebo or nifedipine + celastrol orally. This study provides evidence for the potential role of celastrol serving as an effective and safe adjuvant to oral nifedipine against hypertension in patients with preeclampsia (Xiao et al., 2017). The therapeutic effects such as the anti-inflammatory, anticancer, and neuroprotective properties of celastrol can be mainly attributed to its capacity to inhibit NF-κB, a central player in inflammation, cancer and neurodegenerative diseases (Cascão et al., 2017).
Clinical investigation shows that gallic acid (GA) inhibits oxidative stress in diabetic patients. A small amount of GA prevents oxidative DNA injury and decreases markers which reflect inflammation and augmented risks of cancer and cardiovascular diseases (Ferk et al., 2018).
Clinical reports in asthma patients show that Genistein exerts antioxidant effects and could inhibit the pathway of NF-κB and TNF-α in these patients (Liu et al., 2010b).
It has been reported that Ginsenoside Rb1 (GS-Rb1) treatment was efficient in decreasing the extent of oxidative stress and inflammation in chronic kidney disease, whereas persistent deterioration was observed in the placebo group. Thus, extended treatments using GS-Rb1 may represent an interesting approach to slow the development of this disease at early stages (Xu et al., 2017).
Limitations
Although NPCs are promising therapeutic agents, they need in vivo studies (animal models) mainly analyzing the specificity of their therapeutic action as well as toxic, mutagenic or side effects. Scientists must identify the components of each extract as well as the therapeutically active molecule/s. Moreover, previous prospective clinical trials are mandatory to recommend their use in clinical practice.
Conclusion
EMT is a physiological and self-regulated process of tissue repair. However, pathologic EMT is characterized by its irreversibility and loss of self-regulation being a pathogenic part of many diseases. Thus, EMT is a therapeutic target with no established treatment yet. Natural products appear as therapeutic alternatives that need deep studies to be used in humans. Synergy and antagonism with other agents and interactions with prescription drugs should be studied in order to develop clinical trials.
The use of natural plant compounds versus standard drugs offers therapeutic advantages, such as the potential lower price and ease of being obtained, as they do not need to be artificially syntethized. Moreover, some of them are usually employed in the diet, like curcumin, although other routes of administration should be analyzed to calculate potential doses. Moreover, although many new drugs are made by synthetic chemistry and novel approaches to drug discovery such as combinatorial chemistry and computer-based design have been developed, they cannot replace the role of plant compounds in drug discovery, serving as chemical templates for the design and synthesis of new therapeutical drugs.
The relevance of this study lies on the necessity of finding effective therapies against EMT, which is a process involved in many diseases.
Author Contributions
All the authors contributed to and approved the final manuscript.
Conflict of Interest Statement
The authors declare that the research was conducted in the absence of any commercial or financial relationships that could be construed as a potential conflict of interest.
Acknowledgments
This work was supported by grant SAF2013-47611R from the Ministerio de Economia y Competitividad and by grant S2010/BMD-2321 (FIBROTEAM Consortium) from Comunidad Autónoma de Madrid to ML-C from Fondo de Investigaciones Sanitarias (FIS) and European Regional Development found (FEDER), PI 15/00598 Instituto Carlos-III to AA. REDinREN is a group in which Spanish authors are involved, contributing to development of PD.
Thanks to the Academic Unit of Human Medicine and the Area of Health Sciences of the Autonomous University of Zacatecas, for their support in the Academic Stay in the Molecular Biology Unit at the Universitary Hospital La Princesa. Madrid, Spain. We want to thank Cristobal de los Rios, Associate Researcher from Institute-Foundation Teófilo Hernando, Pharmacology and Therapeutics Department, Autonomous University of Madrid and Institute of Health Research from Hospital la Princesa, (Madrid, Spain), for providing us the chemical structures of the natural products included in this publication.
References
Ackland, M. L., Newgreen, D. F., Fridman, M., Waltham, M. C., Arvanitis, A., Minichiello, J., et al. (2003). Epidermal growth factor-induced epitheliomesenchymal transition in human breast carcinoma cells. Lab. Invest. 83, 435–448. doi: 10.1097/01.LAB.0000059927.97515.FD
Aggarwal, B. B., Bhardwaj, A., Aggarwal, R. S., Seeram, N. P., Shishodia, S., Takada, Y. (2004). Role of resveratrol in prevention and therapy of cancer: preclinical and clinical studies. Anticancer Res. 24 (5A), 2783–2840.
Aggarwal, B. B., Kumar, A., Bharti, A. C. (2003). Anticancer potential of curcumin: preclinical and clinical studies. Anticancer Res. 23 (1A), 363–98.
Aguilera, A., Yáñez-Mo, M., Selgas, R., Sánchez-Madrid, F., López-Cabrera, M. (2005). Epithelial to mesenchymal transition as a triggering factor of peritoneal membrane fibrosis and angiogenesis in peritoneal dialysis patients. Curr. Opin. Invest. Drugs 6 (3), 262–268.
Ahn, K. S., Sethi, G., Shishodia, S., Sung, B., Arbiser, J. L., Aggarwal, B. B. (2006). Honokiol potentiates apoptosis, suppresses osteoclastogenesis, and inhibits invasion through modulation of nuclear factor-kappaB activation pathway. Mol. Cancer Res. MCR 4, 621–633. doi: 10.1158/1541-7786.MCR-06-0076
Akhtar, N., Syed, D. N., Khan, M. I., Adhami, V. M., Mirza, B., Mukhtar, H. (2016). The pentacyclic triterpenoid, plectranthoic acid, a novel activator of AMPK induces apoptotic death in prostate cancer cells. Oncotarget 7, 3819. doi: 10.18632/oncotarget.6625
Akhtar, N., Syed, D. N., Lall, R. K., Mirza, B., Mukhtar, H. (2018). Targeting epithelial to mesenchymal transition in prostate cancer by a novel compound, plectranthoic acid, isolated from Ficus microcarpa. Mol. Carcinog. 57, 653–663. doi: 10.1002/mc.22790
Allegra, A., Innao, V., Russo, S., Gerace, D., Alonci, A., Musolino, C. (2017). Anticancer activity of curcumin and its analogues: preclinical and clinical studies. Cancer Invest. 35 (1), 1–22. doi: 10.1080/07357907.2016.1247166
Allison, A. C., Cacabelos, R., Lombardi, V. R., Alvarez, X. A., Vigo, C. (2001). Celastrol, a potent antioxidant and anti-inflammatory drug, as a possible treatment for Alzheimer’s disease. Prog. Neuropsychopharmacol. Biol. Psychiatry 25 (7), 1341–1357. doi: 10.1016/S0278-5846(01)00192-0
Amjad, A. I., Parikh, R. A., Appleman, L. J., Hahm, E. R., Singh, K., Singh, S. V. (2015). Broccoli-derived sulforaphane and chemoprevention of prostate cancer: from bench to bedside. Curr. Pharmacol. Rep. 1, 382–390. doi: 10.1007/s40495-015-0034-x
Arora, S., Bhardwaj, A., Srivastava, S. K., Singh, S., McClellan, S., Wang, B., et al. (2011). Honokiol arrests cell cycle, induces apoptosis, and potentiates the cytotoxic effect of gemcitabine in human pancreatic cancer cells. PLoS One 6, e21573. doi: 10.1371/journal.pone.0021573
Arora, S., Singh, S., Piazza, G. A., Contreras, C. M., Panyam, J., Singh, A. P. (2012). Honokiol: a novel natural agent for cancer prevention and therapy. Curr. Mol. Med. 12, 1244–1252. doi: 10.2174/156652412803833508
Atwell, L. L., Beaver, L. M., Shannon, J., Williams, D. E., Dashwood, R. H., Ho, E. (2015). Epigenetic regulation by sulforaphane: opportunities for breast and prostate cancer chemoprevention. Curr. Pharmacol. Rep. 1 (2), 102–111. doi: 10.1007/s40495-014-0002-x
Avtanski, D. B., Nagalingam, A., Bonner, M. Y., Arbiser, J. L., Saxena, N. K., Sharma, D. (2014). Honokiol inhibits epithelial—mesenchymal transition in breast cancer cells by targeting signal transducer and activator of transcription 3/Zeb1/E-cadherin axis. Mol. Oncol. 8 (3), 565–580. doi: 10.1016/j.molonc.2014.01.004
Bai, Y., Lu, H., Hu, L., Hong, D., Ding, L., Chen, B. (2014a). Effect of sedum sarmentosum BUNGE extract on aristolochic acid-induced renal tubular epithelial cell injury. J. Pharmacol. Sci. 124, 445–456. doi: 10.1254/jphs.13216FP
Bai, Y., Lu, H., Wu, C. Z., Liang, Y., Wang, S. L., Lin, C. C., et al. (2014b). Resveratrol inhibits epithelial-mesenchymal transition and renal fibrosis by antagonizing the hedgehog signaling pathway. Biochem. Pharmacol. 92, 484–493. doi: 10.1016/j.bcp.2014.09.002
Bai, Y., Wu, C., Hong, W., Zhang, X., Liu, L., Chen, B. (2017). Anti-fibrotic effect of Sedum sarmentosum Bunge extract in kidneys via the hedgehog signaling pathway. Mol. Med. Rep. 16 (1), 737–745. doi: 10.3892/mmr.2017.6628
Bao, H., Peng, A. (2016). The green tea polyphenol (-)-epigallocatechin-3- gallate and its beneficial roles in chronic kidney disease. J. Transl. Int. Med. 4, 99–103. doi: 10.1515/jtim-2016-0031
Barberà, M. J., Puig, I., Domínguez, D., Julien-Grille, S., Guaita-Esteruelas, S., Peiró, S., et al. (2004). Regulation of snail transcription during epithelial to mesenchymal transition of tumor cells. Oncogene 23 (44), 7345–7354. doi: 10.1038/sj.onc.1207990
Baribeau, S., Chaudhry, P., Parent, S., Asselin, E. (2014). Resveratrol inhibits cisplatin-induced epithelial-to-mesenchymal transition in ovarian cancer cell lines. PLos One 9, e86987. doi: 10.1371/journal.pone.0086987
Bezerra, D. P., Pessoa, C., de Moraes, M. O., Saker-Neto, N., Silveira, E. R., Costa-Lotufo, L. V. (2013). Overview of the therapeutic potential of piplartine (piperlongumine). Eur. J. Pharm. Sci. 48 (3), 453–463. doi: 10.1016/j.ejps.2012.12.003
Bhadra, K., Kumar, G. S. (2011). Therapeutic potential of nucleic acid-binding isoquinoline alkaloids: binding aspects and implications for drug design. Med. Res. Rev. 31, 821–862. doi: 10.1002/med.20202
Bisht, K., Wagner, K. H., Bulmer, A. C. (2010). Curcumin, resveratrol and flavonoids as anti-inflammatory, cyto- and DNA-protective dietary compounds. Toxicology 278, 88–100. doi: 10.1016/j.tox.2009.11.008
Bitzer, M., von Gersdorff, G., Liang, D., Dominguez-Rosales, A., Beg, A. A., Rojkind, M., et al. (2000). A mechanism of supression of TGF-b/SMAD signaling by NF-κB/Re1A. Gene Dev. 14 (2), 187–197.
Boocock, D. J., Faust, G. E., Patel, K. R., Schinas, A. M., Brown, V. A., Ducharme, M. P., et al. (2007). Phase I dose escalation pharmacokinetic study in healthy volunteers of resveratrol, a potential cancer chemopreventive agent. Cancer Epidemiol. Biomarkers Prev. 16, 1246–1252. doi: 10.1158/1055-9965.EPI-07-0022
Bodduluru, L. N., Kasala, E. R., Thota, N., Barua, C. C., Sistla, R. (2014). Chemopreventive and therapeutic effects of nimbolide in cancer: the underlying mechanisms. Toxicol. In Vitro 28, 1026–1035. doi: 10.1016/j.tiv.2014.04.011
Boldbaatar, A., Lee, S., Han, S., Jeong, A. L., Ka, H. I., Buyanravjikh, S., et al. (2017). Eupatolide inhibits the TGF-β1-induced migration of breast cancer cells via downregulation of SMAD3 phosphorylation and transcriptional repression of ALK5. Oncol. Lett. 14 (5), 6031–6039. doi: 10.3892/ol.2017.6957
Bouquet, J., Rivaud, M., Chevalley, S., Deharo, E., Jullian, V., Valentin, A. (2012). Biological activities of nitidine, a potential anti-malarial lead compound. Malar. J. 11, 67. doi: 10.1186/1475-2875-11-67
Boutet, A., De Frutos, C. A., Maxwell, P. H., Mayol, M. J., Romero, J., Nieto, M. A. (2006). Snail activation disrupts tissue homeostasis and induces fibrosis in the adult kidney. EMBO J. 25 (23), 5603–5613. doi: 10.1038/sj.emboj.7601421
Brown, V. A., Patel, K. R., Viskaduraki, M., Crowell, J. A., Perloff, M., Booth, T. D., et al. (2010). Repeat dose study of the cancer chemopreventive agent resveratrol in healthy volunteers: safety, pharmacokinetics, and effect on the insulin-like growth factor axis. Cancer Res. 70 (22), 9003–9011. doi: 10.1158/0008-5472.CAN-10-2364
Cano, A., Pérez-Moreno, M. A., Rodrigo, I., Locascio, A., Blanco, M. J., del Barrio, M. G., et al. (2000). The transcription factor snail controls epithelial-mesenchymal transitions by repressing E-cadherin expression. Nat. Cell Biol. 2, 76–83. doi: 10.1038/35000025
Cascão, R., Fonseca, J. E., Moita, L. F. (2017). Celastrol: a spectrum of treatment opportunities in chronic diseases. Front. Med. (Lausanne) 15 (4), 69. doi: 10.3389/fmed.2017.00069
Chander, V., Chopra, K. (2005). Role of nitric oxide in resveratrol-induced renal protective effects of ischemic preconditioning. J. Vasc. Surg. 42, 1198–1205. doi: 10.1016/j.jvs.2005.08.032
Chang, C. C., Ling, X. H., Hsu, H. F., Wu, J. M., Wang, C. P., Yang, J. F., et al. (2016). Siegesbeckia orientalis extract inhibits TGFβ1-induced migration and invasion of endometrial cancer cells. Moléculas 21, 8. doi: 10.3390/molecules21081021
Chang, J., Wang, H., Wang, X., Zhao, Y., Zhao, D., Wang, C., et al. (2015). Molecular mechanisms of Polyphyllin I-induced apoptosis and reversal of the epithelial–mesenchymal transition in human osteosarcoma cells. J. Ethnopharmacol. 170, 117–127. doi: 10.1016/j.jep.2015.05.006
Charoenrungruang, S., Chanvorachote, P., Sritularak, B. (2014). Gigantol induced apoptosis in lung cancer cell through mitochondrial dependent pathway. TJPS 38 (2), 67–73. http://www.thaiscience.info/journals/Article/TJPS/10963058.pdf
Chaurasiya, N. D., Uniyal, G. C., Lal, P., Misra, L., Sangwan, N. S., Tuli, R., et al. (2008). Analysis of withanolides in root and leaf of Withania somnifera by HPLC with photodiode array and evaporative light scattering detection. Phytochem. Anal. 19, 148–154. doi: 10.1002/pca.1029
Chen, C., Ma, T., Zhang, C., Zhang, H., Bai, L., Kong, L., et al. (2017a). Down-regulation of aquaporin 5-mediated epithelial-mesenchymal transition and anti-metastatic effect by natural product Cairicoside E in colorectal cancer. Mol. Carcinog. 56 (12), 2692–2705. doi: 10.1002/mc.22712
Chen, C., Wang, Y. Y., Wang, Y. X., Cheng, M. Q., Yin, J. B., Zhang, X., et al. (2017b). Gentiopicroside ameliorates bleomycin-induced pulmonary fibrosis in mice via inhibiting inflammatory and fibrotic process. Biochem. Biophys. Res. Commun. 495 (4), 2396–2403. doi: 10.1016/j.bbrc.2017.12.112
Chen, P. N., Yang, S. F., Yu, C. C., Lin, C. Y., Huang, S. H., Chu, S. C., et al. (2017c). Duchesnea indica extract suppresses the migration of human lung adenocarcinoma cells by inhibiting epithelial–mesenchymal transition. Environ. Toxicol. 32 (8), 2053–2063. doi: 10.1002/tox.22420
Chen, Y., Yuan, T., Zhang, H., Yan, Y., Wang, D., Fang, L., et al. (2017d). Activation of Nrf2 attenuates pulmonary vascular remodeling via inhibiting endothelial-to-mesenchymal transition: an insight from a plant polyphenol. Int. J. Biol. Sci. 13 (8), 1067–1081. doi: 10.7150/ijbs.20316
Chen, C. Y., Peng, W. H., Tsai, K. D., Hsu, S. L. (2007). Luteolin suppresses inflammation-associated gene expression by blocking NF-kappaB and AP-1 activation pathway in mouse alveolar macrophages. Life Sci. 81, 1602–1614. doi: 10.1016/j.lfs.2007.09.028
Chen, J., Wang, J., Lin, L., He, L., Wu, Y., Zhang, L., et al. (2012). Inhibition of STAT3 signaling pathway by nitidine chloride suppressed the angiogenesis and growth of human gastric cancer. Mol. Cancer Ther. 11 (2), 277–287. doi: 10.1158/1535-7163.MCT-11-0648
Chen, X., Sun, R., Hu, J., Mo, Z., Yang, Z., Liao, D., et al. (2008). Attenuation of Bleomycin-induced lung Fibrosis by oxymatrine is associated with regulation of fibroblast proliferation and collagen production in primary culture. Basic Clin. Pharmacol. Toxicol. 103 (3), 278–286. doi: 10.1111/j.1742-7843.2008.00287.x
Chen, Y., Yuan, T., Zhang, H., Wang, D., Yan, Y., Niu, Z., et al. (2016a). Salvianolic acid A attenuates vascular remodeling in a pulmonary arterial hypertension rat model. Acta Pharmacol. Sin. 37 (6), 772–782. doi: 10.1038/aps.2016.22
Chen, X. L., Bai, Y. J., Hu, Q. R., Lvzhen, Huang, Li, X. X. (2016b). Advanced glycation end products induced the epithelial-mesenchymal transition in retinal pigment epithelial cells via ERK activation. Int. J. Clin. Exp. Pathol. 9 (4), 4891–4900. www.ijcep.com /ISSN:1936-2625/IJCEP0024078
Cheng, A. L., Hsu, C. H., Lin, J. K., Hsu, M. M., Ho, Y. F., Shen, T. S., et al. (2001). Phase I clinical trial of curcumin, a chemopreventive agent, in patients with high-risk or pre-malignant lesions. Anticancer Res. 21 (4B), 2895–2900.
Cheng, W., Wu, D., Zuo, Q., Wang, Z., Fan, W. (2013). Ginsenoside Rb1 prevents interleukin-1 beta induced inflammation and apoptosis in human articular chondrocytes. Int. Orthop. 37, 2065–2070. doi: 10.1007/s00264-013-1990-6
Cho, H. S., Kim, J. H., Jang, H. N., Lee, T. W., Jung, M. H., Kim, T. H., et al. (2017). Alpha-lipoic acid ameliorates the epithelial mesenchymal transition induced by unilateral ureteral obstruction in mice. Sci. Rep. 7, 46065. doi: 10.1038/srep46065
Chou, T. C. (2003). Anti-inflammatory and analgesic effects of paeonol in carrageenan-evoked thermal hyperalgesia. Br. J. Pharmacol. 139 (6), 1146–1152. doi: 10.1038/sj.bjp.0705360
Chow, H. H., Garland, L. L., Hsu, C. H., Vining, D. R., Chew, W. M., Miller, J. A., et al. (2010). Resveratrol modulates drug- and carcinogen-metabolizing enzymes in a healthy volunteer study. Cancer Prev. Res. (Phila.) 3 (9), 1168–75. doi: 10.1158/1940-6207.CAPR-09-0155
Chowdhury, A., Sarkar, J., Chakraborti, T., Pramanik, P. K., Chakraborti, S. (2016). Protective role of epigallocatechin-3-gallate in health and disease: a perspective. Biomed. Pharmacother. 78, 50–59. doi: 10.1016/j.biopha.2015.12.013
Chung, H., Choi, H. S., Seo, E. K., Kang, D. H., Oh, E. S. (2015). Baicalin and baicalein inhibit transforming growth factor-beta1-mediated epithelial-mesenchymal transition in human breast epithelial cells. Biochem. Biophys. Res. Commun. 458 (3), 707–713. doi: 10.1016/j.bbrc.2015.02.032
Chung, J. G., Hsia, T. C., Kuo, H. M., Li, Y. C., Lee, Y. M., Lin, S. S., Hung, C. F. (2001). Inhibitory actions of luteolin on the growth and arylamine N-acetyltransferase activity in strains of Helicobacter pylori from ulcer patients. Toxicol. In Vitro 15, 191–198. doi: 10.1016/S0887-2333(01)00015-7
Corvol, H., Flamein, F., Epaud, R., Clement, A., Guillot, L. (2009). Lung alveolar epithelium and interstitial lung disease. Int. J. Biochem. Cell Biol. 41 (8–9), 1643–1651. doi: 10.1016/j.biocel.2009.02.009
D’Amico, M., Hulit, J., Amanatullah, D. F., Zafonte, B. T., Albanese, C., Bouzahzah, B., et al. (2000). The integrin-linked kinase regulates the cyclin D1 gene through glycogen synthase kinase 3b and cAM-responsive element-binding proteindependent pathways. J. Biol. Chem. 275, 32649–32657. doi: 10.1074/jbc.M000643200
Dai, Y., Desano, J., Tang, W., Meng, X., Meng, Y., Burstein, E., et al. (2010). Natural proteasome inhibitor celastrol suppresses androgen-independent prostate cancer progression by modulating apoptotic proteins and NF-kappaB. PLoS One 5 (12), e14153. doi: 10.1371/journal.pone.0014153
Dai, W., Wang, F., He, L., Lin, C., Wu, S., Chen, P., et al. (2015). Genistein inhibits hepatocellular carcinoma cell migration by reversing the epithelial-mesenchymal transition: partial mediation by the transcription factor NFAT1. Mol. Carcinog. 54 (4), 301–311. doi: 10.1002/mc.22100
Deng, S., Wong, C. K. C., Lai, H. C., Wong, A. S. T. (2017). Ginsenoside-Rb1 targets chemotherapy-resistant ovarian cancer stem cells via simultaneous inhibition of Wnt/β-catenin signaling and epithelial-to-mesenchymal transition. Oncotarget 8 (16), 25897–25914. doi: 10.18632/oncotarget.13071
Devappa, R. K., Makkar, H. P., Becker, K. (2010). Nutritional, biochemical, and pharmaceutical potential of proteins and peptides from jatropha: review. J. Agric. Food Chem. 58, 6543–6555. doi: 10.1021/jf100003z
Dinda, B., Dinda, S., DasSharma, S., Banik, R., Chakraborty, A., Dinda, M. (2017). Therapeutic potentials of baicalin and its aglycone, baicalein against inflammatory disorders. Eur. J. Med. Chem. 131, 68–80. doi: 10.1016/j.ejmech.2017.03.004
Ding, Y., Lu, X., Hu, X., Ma, J., Ding, H. (2014). Osthole inhibits proliferation and induces apoptosis in human osteosarcoma cells. Int. J. Clin. Pharmacol. Ther. 52, 112–117. doi: 10.5414/CP201923
Divya, T., Velavan, B., Sudhandiran, G. (2018). Regulation of transforming growth factor-β/Smad-mediated epithelial-mesenchymal transition by celastrol provides protection against Bleomycin-induced pulmonary fibrosis. Basic Clin. Pharmacol. Toxicol. 123 (2), 122–129. doi: 10.1111/bcpt.12975
Fan, D. L., Zhao, W. J., Wang, Y. X., Han, S. Y., Guo, S. (2012). Oxymatrine inhibits collagen synthesis in keloid fibroblasts via inhibition of transforming growth factor-β1/Smad signaling pathway. Int. J. Dermatol. 51 (4), 463–472. doi: 10.1111/j.1365-4632.2011.05234.x
Fang, Z., Tang, Y., Jiao, W., Xing, Z., Guo, Z., Wang, W., et al. (2013). Nitidine chloride inhibits renal cancer cell metastasis via suppressing AKT signaling pathway. Food Chem. Toxicol. 60, 246–251. doi: 10.1016/j.fct.2013.07.062
Farooqi, A. A., Qureshi, M. Z., Khalid, S., Attar, R., Martinelli, C., Sabitaliyevich, U. Y., et al. (2019). Regulation of cell signaling pathways by Berberine in different cancers: searching for missing pieces of an incomplete jig-saw puzzle for an effective cancer therapy. Cancers (Basel). 11 (4), E478. doi: 10.3390/cancers11040478
Fatima, I., El-Ayachi, I., Taotao, L., Lillo, M. A., Krutilina, R., Seagroves, T. N., et al. (2017). The natural compound Jatrophone interferes with Wnt/β-catenin signaling and inhibits proliferation and EMT in human triple-negative breast cancer. PLoS One 12 (12), e0189864. doi: 10.1371/journal.pone.0189864
Ferk, F., Kundi, M., Brath, H., Szekeres, T., Al-Serori, H., Mišík, M., et al. (2018). Gallic acid improves health-associated biochemical parameters and prevents oxidative damage of DNA in type 2 diabetes patients: results of a placebo-controlled pilot study. Mol. Nutr. Food Res. 62 (4), 1–30. doi: 10.1002/mnfr.201700482
Frémont, L. (2000). Biological effects of resveratrol. Life Sci. 66 (8), 663–673. doi: 10.1016/S0024-3205(99)00410-5
Fu, J., Ke, X., Tan, S., Liu, T., Wang, S., Ma, J., et al. (2016). The natural compound codonolactone attenuates TGF-β1-mediated epithelial-to-mesenchymal transition and motility of breast cancer cells. Oncol. Rep. 35 (1), 117–126. doi: 10.3892/or.2015.4394
Fujita, M., Itokawa, H., Sashida, Y. (1973). Studies on the components of Magnolia obovata Thunb. III. Occurrence of magnolol and honokiol in M. obovata and other allied plants. Yakugaku Zasshi 93, 429–434. doi: 10.1248/yakushi1947.93.4_429
Gao, Q., Yuan, Y., Gan, H. Z., Peng, Q. (2015). Resveratrol inhibits the hedgehog signaling pathway and epithelial-mesenchymal transition and suppresses gastric cáncer invasion and metastasis. Oncol. Lett. 9 (5), 2381–2387. doi: 10.3892/ol.2015.2988
Garodia, P., Ichikawa, H., Malani, N., Sethi, G., Aggarwal, B. B. (2007). From ancient medicine to modern medicine: ayurvedic concepts of health and their role in inflammation and cancer. J. Soc. Integr. Oncol. 5, 25–37. doi: 10.2310/7200.2006.029
Ge, P., Ji, X., Ding, Y., Wang, X., Fu, S., Meng, F., et al. (2010). Celastrol causes apoptosis and cell cycle arrest in rat glioma cells. Neurol. Res. 32 (1), 94–100. doi: 10.1179/016164109X12518779082273
Giacomelli, C., Natali, L., Trincavelli, M.L., Daniele, S., Bertoli, A., Flamini, G., et al. (2016). New insights into the anticancer activity of carnosol: p53 reactivation in the U87MG human glioblastoma cell line. Int. J. Biochem. Cell. Biol. 74, 95–108. doi: 10.1016/j.biocel.2016.02.019
Giacomelli, C., Daniele, S., Natali, L., Iofrida, C., Flamini, G., Braca, A., et al. (2017). Carnosol controls the human glioblastoma stemness features through the epithelial-mesenchymal transition modulation and the induction of cancer stem cell apoptosis. Sci. Rep. 7 (1), 15174. doi: 10.1038/s41598-017-15360-2
Ghantous, A., Sinjab, A., Herceg, Z., Darwiche, N. (2013). Parthenolide: from plant shoots to cancer roots. Drug Discov. Today 18 (17–18), 894–905. doi: 10.1016/j.drudis.2013.05.005
Grande, M. T., Sanchez-Laorden, B., Lopez-Blau, C., De Frutos, C. A., Boutet, A., Arevalo, M., et al. (2015). Snail1-induced partial epithelial-tomesenchymal transition drives renal fibrosis in mice and can be targeted to reverse established disease. Nat. Med. 21, 989–997. doi: 10.1038/nm.3901
Gu, L., Feng, J., Zheng, Z., Xu, H., Yu, W. (2016). Polyphyllin I inhibits the growth of ovarian cancer cells in nude mice. Oncol. Lett. 12, 4969–4974. doi: 10.3892/ol.2016.5348
Gupta, S. C., Patchva, S., Aggarwal, B. B. (2013). Therapeutic roles of curcumin: lessons learned from clinical trials. AAPS J. 15 (1), 195–218. doi: 10.1208/s12248-012-9432-8
Gliemann, L., Nyberg, M., Hellsten, Y. (2016). Effects of exercise training and resveratrol on vascular health in aging. Free Radic. Biol. Med. 98, 165–176. doi: 10.1016/j.freeradbiomed.2016.03.037
Han, L., Zhang, H. W., Zhou, W. P., Chen, G. M., Guo, K. J. (2012). The effects of genistein on transforming growth factor-β1-induced invasion and metastasis in human pancreatic cancer cell line Panc-1 in vitro. Chin. Med. (Eng) 125 (11), 2032–2040.
Hang, Y., Qin, X., Ren, T., Cao, J. (2018). Baicalin reduces blood lipids and inflammation in patients with coronary artery disease and rheumatoid arthritis: a randomized, double-blind, placebo-controlled trial. Lipids Health Dis. 17 (1), 146. doi: 10.1186/s12944-018-0797-2
Hao, F., Kumar, S., Yadav, N., Chandra, D. (2014). Neem components as potential agents for cancer prevention and treatment. Biochim. Biophys. Acta 1846, 247–257. doi: 10.1016/j.bbcan.2014.07.002
Hao, J., Zhu, H., Zhang, Z., Yang, S., Li, H. (2015). Identification of anthocyanins in black rice (Oryza sativa L). by UPLC/Q-TOF-MS and their in vitro and in vivo antioxidant activities. J. Cereal Sci. 64, 92–99. doi: 10.1016/j.jcs.2015.05.003
He, D. X., Li, G. H., Gu, X. T., Zhang, L., Mao, A. Q., Wei, J., et al. (2016). A new agent developed by biotransformation of polyphyllin VII inhibits chemoresistance in breast cancer. Oncotarget 7, 31814–31824. doi: 10.18632/oncotarget.6674
Hehner, S. P., Hofmann, T. G., Dröge, W., Schmitz, M. L. (1999). The antiinflammatory sesquiterpene lactone parthenolide inhibits NF-kappa B by targeting the I kappa B kinase complex. J. Immunol. 163 (10), 5617–5623.
Hertig, A., Anglicheau, D., Verine, J., Pallet, N., Touzot, M., Ancel, P. Y., et al. (2008). Early epithelial phenotypic changes predict graft fibrosis. J. Am. Soc. Nephrol. 19 (8), 1584–1591. doi: 10.1681/ASN.2007101160
Hewlings, S. J., Kalman, D. S. (2017). Curcumin: a review of its’ effects on human health. Foods 6 (10), 92. doi: 10.3390/foods6100092
Howells, L. M., Berry, D. P., Elliott, P. J., Jacobson, E. W., Hoffmann, E., Hegarty, B., et al. (2011). Phase I randomized, double-blind pilot study of micronized resveratrol (SRT501) in patients with hepatic metastases—safety, pharmacokinetics, and pharmacodynamics. Cancer Prev. Res. (Phila.) 4 (9), 1419–25. doi: 10.1158/1940-6207.CAPR-11-0148
Ho, J. H., Hong, C. Y. (2011). Salvianolic acids: small compounds with multiple mechanisms for cardiovascular protection. J. Biomed. Sci. 18, 30. doi: 10.1186/1423-0127-18-30
Hou, D. X. (2003). Potential mechanisms of cancer chemoprevention by anthocyanins. Curr. Mol. Med. 3, 149–159. doi: 10.2174/1566524033361555
Hou, Y. L., Tsai, Y. H., Lin, Y. H., Chao, J. C. (2014). Ginseng extract and ginsenoside Rb1 attenuate carbon tetrachloride-induced liver fibrosis in rats. BMC Complement. Altern. Med. 14, 415. doi: 10.1186/1472-6882-14-415
Hu, W., Han, W., Huang, C., Wang, M. H. (2011). Protective effect of the methanolic extract from Duchesnea indica against oxidative stress in vitro and in vivo. Environ. Toxicol. Pharmacol. 31 (1), 42–50. doi: 10.1016/j.etap.2010.09.004
Hu, W., Shen, W., Wang, M. H. (2009). Free radical scavenging activity and protective ability of methanolic extract from Duchesnea indica against protein oxidation and DNA damage. J. Food Sci. Nutr. 14, 77–282. doi: 10.3746/jfn.2009.14.4.277
Huang, S. F., Horng, C. T., Hsieh, Y. S., Hsieh, Y. H., Chu, S. C., Chen, P. N. (2016). Epicatechin-3-gallate reverses TGF-β1-induced epithelial-to-mesenchymal transition and inhibits cell invasion and protease activities in human lung cancer cells. Food Chem. Toxicol. 94, 1–10. doi: 10.1016/j.fct.2016.05.009
Huang, W. H., Lee, A. R., Yang, C. H. (2006). Antioxidative and anti-inflammatory activities of polyhydroxyflavonoids of Scutellaria baicalensis GEORGI. Biosci. Biotechnol. Biochem. 70 (10), 2371–2380.
Huang, Y., Tsang, S. Y., Yao, X., Chen, Z. Y. (2005). Biological properties of baicalein in cardiovascular system. Curr. Drug Targets Cardiovasc. Haematol. Disord. 5 (2), 177–184. doi: 10.2174/1568006043586206
Hui, H., Tang, G., Go, V. L. (2009). Hypoglycemic herbs and their action mechanisms. Chin Med. 4, 11. doi: 10.1186/1749-8546-4-11
Huber, M. A., Azoitei, N., Baumann, B., Grünert, S., Sommer, A., Pehamberger, H., et al. (2004). NF-kB is essential for epithelial–mesenchymal transition and metastasis in a model of breast cancer progression. J. Clin. Invest. 114, 569–581. doi: 10.1172/JCI200421358
Imanshahidi, M., Hosseinzadeh, H. (2008). Pharmacological and therapeutic effects of Berberis vulgaris and its active constituent, berberine. Phytother. Res. 22 (8), 999–1012. doi: 10.1002/ptr.2399
Jeon, Y. K., Yoo, D. R., Jang, Y. H., Jang, S. Y., Nam, M. J. (2011). Sulforaphane induces apoptosis in human hepatic cancer cells through inhibition of 6-phosphofructo-2-kinase/fructose-2,6-biphosphatase 4, mediated by hypoxia inducible factor-1-dependent pathway. Biochim. Biophys. Acta 1814, 1340–1348. doi: 10.1016/j.bbapap.2011.05.015
Ji, Q., Liu, X., Han, Z., Zhou, L., Sui, H., Yan, L., et al. (2015). Resveratrol suppresses epithelial-to-mesenchymal transition in colorectal cancer through TGF-β1/Smads signaling pathway mediated Snail/E-cadherin expression. BMC Cancer 15, 97. doi: 10.1186/s12885-015-1119-y
Ji, Y., Dou, Y., Zhao, Q., Zhang, J., Yang, Y., Wang, T., et al. (2016). Paeoniflorin suppresses TGF-β mediated epithelial mesenchymal transition in pulmonary fibrosis through a Smad- dependent pathway. Acta Pharmacol. Sin. 37 (6), 794–804. doi: 10.1038/aps.2016.36
Jia, L., Zhao, Y. (2009). Current evaluation of the millennium phytomedicine- ginseng (I): etymology, pharmacognosy, phytochemistry, market and regulations. Curr. Med. Chem. 16, 2475–2484. doi: 10.2174/092986709788682146
Jiang, L. L., Zhou, S. J., Zhang, X. M., Chen, H. Q., Liu, W. (2016). Sulforaphane suppresses in vitro and in vivo lung tumorigenesis through downregulation of HDAC activity. Biomed. Pharmacother. 78, 74–80. doi: 10.1016/j.biopha.2015.11.007
Jin, H. J., Xie, X. L., Ye, J. M., Li, C. G. (2013). TanshinoneIIA and cryptotanshinone protect against hypoxia-induced mitochondrial apoptosis in H9c2 cells. PLoS One 8 (1), e51720. doi: 10.1371/journal.pone.0051720
Jin, L., Piao, Z. H., Sun, S., Liu, B., Ryu, Y., Choi, S. Y., et al. (2017). Gallic acid attenuates pulmonary fibrosis in a mouse model of transverse aortic contraction-induced heart failure. Vascul. Pharmacol. 99, 74–82. doi: 10.1016/j.vph.2017.10.007
Jo, E., Park, S. J., Choi, Y. S., Jeon, W. K., Kim, B. C. (2015). Kaempferol suppresses transforming growth factor-β1-induced epithelial-to-mesenchymal transition and migration of A549 lung cancer cells by inhibiting Akt1-mediated phosphorylation of Smad3 at Threonine-179. Neoplasia 17, 525–537. doi: 10.1016/j.neo.2015.06.004
Johanson, J. J. (2011). Carnosol: a promising anti-cancer and infammatory agent. Cancer Lett. 305 (1), 1–7. doi: 10.1016/j.canlet.2011.02.005
Jung, H. J., Kang, H. J., Song, Y. S., Park, E. H., Kim, Y. M., Lim, C. J. (2008). Anti-inflammatory, anti-angiogenic and anti-nociceptive activities of Sedum sarmentosum extract. J. Ethnopharmacol. 116, 138–143. doi: 10.1016/j.jep.2007.11.014
Kalluri, R. (2009). EMT: when epithelial cells decide to become mesenchymal-like cells. J. Clin. Invest. 119 (6), 1417–1419. doi: 10.1172/JCI39675
Kalluri, R., Weinberg, R. A. (2009). The basics of epithelial-mesenchymal transition. J. Clin. Invest. 119, 1420–1428. doi: 10.1172/JCI39104
Kamath, S. G., Chen, N., Xiong, Y., Wenham, R., Apte, S., Humphrey, M., et al. (2009). Gedunin, a novel natural substance, inhibits ovarian cancer cell proliferation. Int. J. Gynecol. Cancer 19, 1564–1569. doi: 10.1111/IGC.0b013e3181a83135
Kanlaya, R., Thongboonkerd, V. (2019). Protective effects of epigallocatechin-3-gallate from green tea in various kidney diseases. Adv. Nutr. 110 (1), 112–121. doi: 10.1093/advances/nmy077
Kao, S. J., Su, J. L., Chen, C. K., Yu, M. C., Bai, K. J., Chang, J. H., et al. (2012). Osthole inhibits the invasive ability of human lung adenocarcinoma cells via suppression of NF-kappaB-mediated matrix metalloproteinase-9 expression. Toxicol. Appl. Pharmacol. 261, 105–115. doi: 10.1016/j.taap.2012.03.020
Kee, H. J., Cho, S. N., Kim, G. R., Choi, S. Y., Ryu, Y., Kim, I. K., et al. (2014). Gallic acid inhibits vascular calcification through the blockade of BMP2-Smad1/5/8 signaling pathway. Vasc. Pharmacol. 63 (2), 71–78. doi: 10.1016/j.vph.2014.08.005
Khan, A. L., Hussain, J., Hamayun, M., Gilani, S. A., Ahmad, S., Rehman, G., et al. (2010). Secondary metabolites from Inula britannica L. and their biological activities. Molecules 15 (3), 1562–1577. doi: 10.3390/molecules15031562
Khan, T., Gurav, P. (2017). PhytoNanotechnology: enhancing delivery of plant based anti-cancer drugs. Front Pharmacol. 8, 1002. doi:10.3389/fphar.2017.01002
Kida, Y., Asahina, K., Teraoka, H., Gitelman, I., Sato, T. (2007). Twist relates to tubular epithelial-mesenchymal transition and interstitial fibrogenesis in the obstructed kidney. J. Histochem. Cytochem. 55 (7), 661–673. doi: 10.1369/jhc.6A7157.2007
Kim, D. H., Sung, B., Kang, Y. J., Hwang, S. Y., Kim, M. J., Yoon, J. H., et al. (2015). Sulforaphane inhibits hypoxia-induced HIF-1alpha and VEGF expression and migration of human colon cancer cells. Int. J. Oncol. 47, 2226–2232. doi: 10.3892/ijo.2015.3200
Kim, K., Lu, Z., Hay, E. D. (2002a). Direct evidence for a role of beta-catenin/LEF-1 signaling pathway in induction of EMT. Cell Biol. Int. 26, 463–476. doi: 10.1006/cbir.2002.0901
Kim, I. G., Jung, I. L., Oh, T. J., Kim, K. C., Shim, H. W. (2002b). Polysaccharide-enriched fraction isolated from Duchesnea chrysantha protects against oxidative damage. Biotechnol. Lett. 24, 1299–1305. doi: 10.1023/A:1019812202099
Kim, N., Hwangbo, C., Lee, S., Lee, J. H. (2013). Eupatolide inhibits PDGF-induced proliferation and migration of aortic smooth muscle cells through ROS-dependent heme oxygenase-1 induction. Phytother. Res. 27, 1700–1707. doi: 10.1002/ptr.4924
Kim, K. C., Kim, J. S., Son, J. K., Kim, I. G. (2007). Enhanced induction of mitochondrial damage and apoptosis in human leukemia HL-60 cells by the Ganoderma lucidum and Duchesnea chrysantha extracts. Cancer Lett. 246, 210–217. doi: 10.1016/j.canlet.2006.02.014
Kim, Y. S., Choi, K. C., Hwang, K. A. (2015). Genistein suppressed epithelial-mesenchymal transition and migration efficacies of BG-1 ovarian cancer cells activated by estrogenic chemicals via estrogen receptor pathway and downregulation of TGF-beta signaling pathway. Phytomedicine 22, 993–999. doi: 10.1016/j.phymed.2015.08.003
Klongkumnuankarn, P., Busaranon, K., Chanvorachote, P., Sritularak, B., Jongbunprasert, V., Likhitwitayawuid, K. (2015). Cytotoxic and Antimigratory activities of phenolic compounds from dendrobium brymerianum. J. Evid. Based Complement. Altern Med. 2015, 1–9. doi: 10.1155/2015/350410
Kong, D., Zhang, F., Shao, J., Wu, L., Zhang, X., Chen, L., et al. (2015). Curcumin inhibits cobalt chloride-induced epithelial-to-mesenchymal transition associated with interference with TGF-β/Smad signaling in hepatocytes. Lab. Invest. 95 (11), 1234–1245. doi: 10.1038/labinvest.2015.107
Kong, M., Fan, J., Dong, A., Cheng, H., Xu, R. (2010). Effects of polyphyllin I on growth inhibition of human non-small lung cancer cells and in xenograft. Acta Biochim. Biophys. Sin. 42, 827–833. doi: 10.1093/abbs/gmq091
Kong, S., Kim, D. J., Oh, S. K., Choi, I. S., Jeong, H. S., Lee, J. (2012). Black rice bran as an ingredient in noodles: chemical and functional evaluation. J. Food Sci. 77, C303–C307. doi: 10.1111/j.1750-3841.2011.02590.x
Kou, Y., Li, L., Li, H., Tan, Y., Li, B., Wang, K., et al. (2016). Berberine suppressed epithelial mesenchymal transition through cross-talk regulation of PI3K/AKT and RARα/RARβ in melanoma cells. Biochem. Biophys. Res. Commun. 479 (2), 290–296. doi: 10.1016/j.bbrc.2016.09.061
Kulkarni, S. K., Dhir, A. (2010). Berberine: a plant alkaloid with therapeutic potential for central nervous system disorders. Phytother. Res. 24, 317–324. doi: 10.1002/ptr.2968
la Porte, C., Voduc, N., Zhang, G., Seguin, I., Tardiff, D., Singhal, N., et al. (2010). Steady-State pharmacokinetics and tolerability of trans-resveratrol 2000 mg twice daily with food, quercetin and alcohol (ethanol) in healthy human subjects. Clin. Pharmacokinet. 49 (7), 449–454. doi: 10.2165/11531820-000000000-00000
Lee, D. G., Jang, S. I., Kim, Y. R., Yang, K. E., Yoon, S. J., Lee, Z. W., et al. (2016). Anti-proliferative effects of ginsenosides extracted from mountain ginseng on lung cancer. Chin. J. Integr. Med. 22 (5), 344–352. doi: 10.1007/s11655-014-1789-8
Lee, J., Hahm, E. R., Marcus, A. I., Singh, S. V. (2013). Withaferin a inhibits experimental epithelial-mesenchymal transition in MCF-10A cells and suppresses vimentin protein level in vivo in breast tumors. Mol. Carcinog. 54 (6), 417–429. doi: 10.1002/mc.22110
Lee, J., Hwangbo, C., Lee, J. J., Seo, J., Lee, J. H. (2010a). The sesquiterpene lactone eupatolide sensitizes breast cancer cells to TRAIL through down-regulation of c-FLIP expression. Oncol. Rep. 23, 229–237. doi: 10.3892/or_00000628
Lee, J., Tae, N., Lee, J. J., Kim, T., Lee, J. H. (2010b). Eupatolide inhibits lipopolysaccharide-induced COX-2 and iNOS expression in RAW264.7 cells by inducing proteasomal degradation of TRAF6. Eur. J. Pharmacol. 636, 173–180. doi: 10.1016/j.ejphar.2010.03.021
Lee, J., Jung, E., Park, J., Jung, K., Lee, S., Hong, S., et al. (2005). Anti-inflammatory effects of magnolol and honokiol are mediated through inhibition of the downstream pathway of MEKK-1 in NF-kappaB activation signaling. Planta Med. 71, 338–343. doi: 10.1055/s-2005-864100
Lee, J. M., Dedhar, S., Kalluri, R., Thompson, E. W. (2006). The epithelial-mesenchymal transition: new insights in signaling, development, and disease. J. Cell Biol. 172 (7), 973-981.
Lee, J. Y., Kim, H. S., Song, Y. S. (2012). Genistein as a potential anticancer agent against ovarian cancer. J. Tradit. Complement. Med. 2 (2), 96–104. doi: 10.1016/S2225-4110(16)30082-7
Lee, I. R., Yang, M. Y. (1994). Phenolic compounds from Duchesnea chrysantha and their cytotoxic activities in human cancer cell. Arch. Pharm. Res. 17, 476–479. doi: 10.1007/BF02979129
Li, J., Chong, T., Wang, Z., Chen, H., Li, H., Cao, J., et al. (2014). A novel anti-cancer effect of resveratrol: reversal of epithelial-mesenchymal transition in prostate cancer cells. Mol. Med. Rep. 10, 1717–1724. doi: 10.3892/mmr.2014.2417
Li, J., Yang, J., Lü, F., Qi, Y. T., Liu, Y. Q., Sun, Y., et al. (2012a). Chemical constituents from the stems of Celastrus orbiculatus. Chin. J. Nat. Med. 10 (4), 279–283. ISSN 1875-5364. doi: 10.3724/SP.J.1009.2012.00279
Li, J., Shao, Z. H., Xie, J. T., Wang, C. Z., Ramachandran, S., Yin, J. J., et al. (2012b). The effects of ginsenoside Rb1 on JNK in oxidative injury in cardiomyocytes. Arch. Pharm. Res. 35, 1259–1267. doi: 10.1007/s12272-012-0717-3
Li, W., Ma, J., Ma, Q., Li, B., Han, L., Liu, J., et al. (2013). Resveratrol inhibits the epithelial-mesenchymal transition of pancreatic cancer cells via suppression of the PI-3 K/Akt/NF-κB pathway. Curr. Med. Chem. 20, 4185–4194. doi: 10.2174/09298673113209990251
Li, W., Saud, S. M., Young, M. R., Colburn, N. H., Hua, B. (2015). Cryptotanshinone, a Stat3 inhibitor, suppresses colorectal cancer proliferation and growth in vitro. Mol. Cell Biochem. 406 (1–2), 63–73. doi: 10.1007/s11010-015-2424-0
Li, X. H., Xiao, T., Yang, J. H., Qin, Y., Gao, J. J., Liu, H. J., et al. (2018a). Parthenolide attenuated bleomycin-induced pulmonary fibrosis via the NF-κB/Snail signaling pathway. Respir. Res. 19 (1), 111. doi: 10.1186/s12931-018-0806-z
Li, W., Jiang, Z., Xiao, X., Wang, Z., Wu, Z., Ma, Q., et al. (2018b). Curcumin inhibits superoxide dismutase-induced epithelial-to-mesenchymal transition via the PI3K/Akt/NF-κB pathway in pancreatic cancer cells. Int. J. Oncol. 52, 1593–1602. doi: 10.3892/ijo.2018.4295
Liappas, G., Gónzalez-Mateo, G. T., Majano, P., Sánchez-Tomero, J. A., Ruiz-Ortega, M., Rodrigues Díez, R., et al. (2015). T helper 17/regulatory T Cell balance and experimental models of peritoneal dialysis-induced damage. Biomed. Res. Int. 2017, 6130208. doi: 10.1155/2017/6130208
Lin, C. Y., Hsieh, Y. H., Yang, S. F., Chu, S. C., Chen, P. N., Hsieh, Y. S. (2017). Cinnamomum cassia extracts reverses TGF-β1-induced epithelial–mesenchymal transition in human lung adenocarcinoma cells and suppresses tumor growth in vivo. Environ. Toxicol. 32 (7), 1878–1887. doi: 10.1002/tox.22410
Lin, D., Kuang, G., Wan, J., Zhang, X., Li, H., Gong, X., et al. (2016). Luteolin suppresses the metastasis of triple-negative breast cancer by reversing epithelial-to-mesenchymal transition via downregulation of β-catenin expression. Oncol. Rep. 37 (2), 895–902. doi: 10.3892/or.2016.5311
Lin, X., Yi, Z., Diao, J., Shao, M., Zhao, L., Cai, H., et al. (2014). ShaoYao decoction ameliorates colitis-associated col- orectal cancer by downregulating proinflammatory cytokines and promoting epithelial-mesenchymal transition. J. Transl. Med. 12, 105. doi: 10.1186/1479-5876-12-105
Lin, Y., Shi, R., Wang, X., Shen, H. M. (2008). Luteolin, a flavonoid with potential for cancer prevention and therapy. Curr. Cancer Drug Targets 8, 634–646. doi: 10.2174/156800908786241050
Lin, L., Sun, Y., Wang, D., Zheng, S., Zhang, J., Zheng, C. (2015). Celastrol ameliorates ulcerative colitis-related colorectal cancer in mice via suppressing inflammatory responses and epithelial-mesenchymal transition. Front. Pharmacol. 6, 320. doi: 10.3389/fphar.2015.00320
Liu, D., Qiu, X. Y., Wu, X., Hu, D. X., Li, C. Y., Yu, S. B., et al. (2017). Piperlongumine suppresses bladder cancer invasion via inhibiting epithelial mesenchymal transition and F-actin reorganization. Biochem. Biophys. Res. Commun. 494 (1–2), 165–172. doi: 10.1016/j.bbrc.2017.10.061
Liu, L., Lu, W., Ma, Z., Li, Z. (2012). Oxymatrine attenuates bleomycin-induced pulmonary fibrosis in mice via the inhibition of inducible nitric oxide synthase expression and the TGF-β/Smad signaling pathway. Int. J. Mol. Med. 29 (5), 815–822. doi: 10.3892/ijmm.2012.923
Liu, Q. R., Liu, J. M., Chen, Y., Xie, X. Q., Xiong, X. X., Qiu, X. Y., et al. (2014). Piperlongumine inhibits migration of glioblastoma cells via activation of ROS-dependent p38 and JNK signaling pathways. Oxidative Med. Cell. Longev. 2014, 653732. doi: 10.1155/2014/653732
Liu, Y. (2004). Epithelial to mesenchymal transition in renal fibrogenesis: pathologic significance, molecular mechanism, and therapeutic intervention. J. Am. Soc. Nephrol. 15, 1–12. doi: 10.1097/01.ASN.0000106015.29070.E7
Liu, C., Wang, Z., Song, Y., Wu, D., Zheng, X., Li, P., et al. (2015). Effects of berberine on amelioration of hyperglycemia and oxidative stress in high glucose and high fat diet-induced diabetic hamsters in vivo. Biomed. Res. Int. 2015, 313808. doi: 10.1155/2015/313808
Liu, L., Wang, Y., Yan, R., Li, S., Shi, M., Xiao, Y., et al. (2016). Oxymatrine inhibits renal tubular EMT induced by high glucose via upregulation of snoN and inhibition of TGF-β1/Smad signaling pathway. PLoS One 11 (3), e0151986. doi: 10.1371/journal.pone.0151986
Liu, P. L., Tsai, J. R., Charles, A. L., Hwang, J. J., Chou, S. H., Ping, Y. H., et al. (2010a). Resveratrol inhibits human lung adenocarcinoma cell metastasis by suppressing heme oxygenase 1-mediated nuclear factor-kappaB pathway and subsequently downregulating expression of matrix metalloproteinases. Mol. Nutr. Food Res. 54 (Suppl. 2), S196–S204. doi: 10.1002/mnfr.200900550
Liu, X. J., Zhao, J., Gu, X. Y. (2010b). The effects of genistein and puerarin on the activation of nuclear factor-kappaB and the production of tumor necrosis factor-alpha in asthma patients. Pharmazie 65 (2), 127–131.
Lopez-Cabrera, M. (2014). Mesenchymal conversion of mesothelial cells is a key event in the pathophysiology of the peritoneum during peritoneal dialysis. Adv. Med. 2014, 473134. doi: 10.1155/2014/473134
Lou, W., Chen, Y., Zhu, K. Y., Deng, H., Wu, T., Wang, J. (2017). Polyphyllin I Overcomes EMT-associated resistance to erlotinib in lung cancer cells via IL-6/STAT3 pathway inhibition. Biol. Pharm. Bull. 40 (8), 1306–1313. doi: 10.1248/bpb.b17-00271
Lovisa, S., LeBleu, V. S., Tampe, B., Sugimoto, H., Vadnagara, K., Carstens, J. L., et al. (2015). Epithelial-to-mesenchymal transition induces cell cycle arrest and parenchymal damage in renal fibrosis. Nat. Med. 21, 998–1009. doi: 10.1038/nm.3902
Lu, Z., Lai, Z.-Q., Leung, A. W. N., Leung, P. S., Li, Z.-S., Lin, Z.-X. (2017). Exploring brusatol as a new anti-pancreatic cancer adjuvant: biological evaluation and mechanistic studies. Oncotarget 8 (49), 84974–84985. doi: 10.18632/oncotarget.17761
Lu, L., Qin, Y., Chen, C., Guo, X. (2018). Beneficial effects exerted by paeonol in the management of atherosclerosis. Oxid. Med. Cell Longev. 2018, 1098617. doi: 10.1155/2018/1098617
Lv, X. Q., Qiao, X. R., Su, L., Chen, S. Z. (2016). Honokiol inhibits EMT-mediated motility and migration of human non-small cell lung cancer cells in vitro by targeting c-FLIP. Acta Pharmacol. Sin. 37 (12), 1574–1586. doi: 10.1038/aps.2016.81
Ma, S., Yang, D., Wang, K., Tang, B., Li, D., Yang, Y. (2012). Cryptotanshinone attenuates isoprenaline-induced cardiac fibrosis in mice associated with upregulation and activation of matrix metalloproteinase-2. Mol. Med. Rep. 6 (1), 145–150. doi: 10.3892/mmr.2012.866
Ma, Y., Li, H., Yue, Z., Guo, J., Xu, S., Xu, J., et al. (2014). Cryptotanshinone attenuates cardiac fibrosis via downregulation of COX-2, NOX-2, and NOX-4. J. Cardiovasc. Pharmacol. 64 (1), 28–37. doi: 10.1097/FJC.0000000000000086
Marin-Neto, J. A., Maciel, B. C., Secches, A. L., Gallo, L. (1988). Cardiovascular effects of berberine in patients with severe congestive heart failure. Clin. Cardiol. 11, 253–260. doi: 10.1002/clc.4960110411
Massagué, J., Wotton, D. (2000). Transcriptional control by the TGF-b/Smad signaling. EMBO 19, 1745–1754. doi: 10.1093/emboj/19.8.1745
Massagué, J. (2000). How cells read TGF-beta signals. Nat. Rev. Mol. Cell Biol. 1, 169–178. doi: 10.1038/35043051
Masszi, A., Di Ciano, C., Sirokmány, G., Arthur, W. T., Rotstein, O. D., Wang, J., et al. (2003). Central role for Rho in TGF-beta1-induced alpha-smooth muscle actin expresion during epithelial-mesenchymal transition. Am. J. Physiol. Renal Physiol. 284, F911–F924. doi: 10.1152/ajprenal.00183.2002
Masszi, A., Kapus, A. (2011). Smaddening complexity: the role of Smad3 in epithelial-myofibroblast transition. Cells Tissues Organs 193, 41–52. doi: 10.1159/000320180
McCubrey, J. A., Lertpiriyapong, K., Steelman, L. S., Abrams, S. L., Yang, L. V., Murata, et al. (2017). Effects of resveratrol, curcumin, berberine and other nutraceuticals on aging, cancer development, cancer stem cells and microRNAs. Aging (Albany N. Y.) 9, 1477–1536. doi: 10.18632/aging.101250
Mohsenikia, M., Alizadeh, A. M., Khodayari, S., Khodayari, H., Kouhpayeh, S. A., Karimi, A., et al. (2013). The protective and therapeutic effects of alpha-solanine on mice breast cancer. Eur. J. Pharmacol. 718, 1–9. doi: 10.1016/j.ejphar.2013.09.015
Morikawa, T., Zhang, Y., Nakamura, S., Matsuda, H., Muraoka, O., Yoshikawa, M. (2007). Bioactive constituents from Chinese natural medicines. XXII. Absolute structures of new megastigmane glycosides, sedumosides E1, E2, E3, F1, F2 and G, from Sedum sarmentosum (Crassulaceae). Chem. Pharm. Bull. 55, 435–441. doi: 10.1248/cpb.55.435
Moshiri, A., Puppo, M., Rossi, M., Gherzi, R., Briata, P. (2017). Resveratrol limits epithelial to mesenchymal transition through modulation of KHSRP/hnRNPA1-dependent alternative splicing in mammary gland cells. Biochim. Biophys. Acta Gene Regul Mech. 1860 (3), 291–298. doi: 10.1016/j.bbagrm.2017.01.001
Nagalingam, A., Arbiser, J. L., Bonner, M. Y., Saxena, N. K., Sharma, D. (2012). Honokiol activates AMP-activated protein kinase in breast cancer cells via an LKB1-dependent pathway and inhibits breast carcinogenesis. Breast Cancer Res. 14 (1), R35.
Newman, D. J., Cragg, G. M. (2007). Natural products as sources of new drugs over the last 25 years. J. Nat. Prod. 70, 461–477. doi: 10.1021/np068054v
Nieto, M. A., Huang, R. Y., Jackson, R. A., Thiery, J. P. (2016). EMT: 2016. Cell 166, 21–45. doi: 10.1016/j.cell.2016.06.028
Ninomiya, K., Morikawa, T., Zhang, Y., Nakamura, S., Matsuda, H., Muraoka, O., et al. (2007). Bioactive constituents from Chinese natural medicines. XXIII. Absolute structures of new megastigmane glycosides, sedumosides A(4), A(5), A(6), H, and I, and hepatoprotective megastigmanes from Sedum sarmentosum. Chem. Pharm. Bull. 55, 1185–1191. doi: 10.1248/cpb.55.1185
Oh, H., Kang, D. G., Kwon, J. W., Kwon, T. O., Lee, S. Y., Lee, D. B., et al. (2004). Isolation of angiotensin converting enzyme (ACE) inhibitory flavonoids from Sedum sarmentosum. Biol. Pharm. Bull. 27, 2035–2037. doi: 10.1248/bpb.27.2035
Ong, R. C., Lei, J., Lee, R. K., Cheung, J. Y., Fung, K. P., Lin, C., et al. (2008). Polyphyllin D induces mitochondrial fragmentation and acts directly on the mitochondria to induce apoptosis in drugresistant HepG2 cells. Cancer Lett. 261, 158–164. doi: 10.1016/j.canlet.2007.11.005
Padhye, S., Dandawate, P., Yusufi, M., Ahmad, A., Sarkar, F. H. (2012). Perspectives on medicinal properties of plumbagin and its analogs. Med. Res. Rev. 32 (6), 1131–1158. doi: 10.1002/med.20235
Padmavathi, B., Rath, P. C., Rao, A. R., Singh, R. P. (2005). Roots of Withania somnifera inhibit forestomach and skin carcinogenesis in mice. Evid. Based Complement. Alternat. Med. 2, 99–105. doi: 10.1093/ecam/neh064
Pan, S.-T., Qin, Y., Zhou, Z.-W., He, Z.-X., Zhang, X., Yang, T., et al. (2015). Plumbagin suppresses epithelial to mesenchymal transition and stemness via inhibiting Nrf2-mediated signaling pathway in human tongue squamous cell carcinoma cells. Drug Des. Dev. Ther. 9, 5511–5551. doi: 10.2147/DDDT.S89621
Pattarayan, D., Sivanantham, A., Krishnaswami, V., Loganathan, L., Palanichamy, R., Natesan, S., et al. (2018). Tannic acid attenuates TGF-β1-induced epithelial-to-mesenchymal transition by effectively intervening TGF-β signaling in lung epithelial cells. J. Cell Physiol. 233 (3), 2513–2525. doi: 10.1002/jcp.26127
Patwardhan, C. A., Fauq, A., Peterson, L. B., Miller, C., Blagg, B. S., Chadli, A. (2013). Gedunin inactivates the co-chaperone p23 protein causing cancer cell death by apoptosis. J. Biol. Chem. 288, 7313–7325. doi: 10.1074/jbc.M112.427328
Peinado, H., Quintanilla, M., Cano, A. (2003). Transforming growth factor b-1 induces snail transcription factor in epithelial cell lines. J. Biol. Chem. 278, 21113–21123. doi: 10.1074/jbc.M211304200
Peng, C. H., Chyau, C. C., Wang, C. J., Lin, H. T., Huang, C. N., Ker, Y. B. (2016). Abelmoschus esculentus fractions potently inhibited the pathogenic targets associated with diabetic renal epithelial to mesenchymal transition. Food Funct. 7 (2), 728–740. doi: 10.1039/C5FO01214G
Petpiroon, N., Sritularak, B., Chanvorachote, P. (2017). Phoyunnanin E inhibits migration of non-small cell lung cancer cells via suppression of epithelial-to-mesenchymal transition and integrin αv and integrin β3. BMC Complement. Altern. Med. 17, 553. doi: 10.1186/s12906-017-2059-7
Popat, R., Plesner, T., Davies, F., Cook, G., Cook, M., Elliott, P., et al. (2013). A phase 2 study of SRT501 (resveratrol) with bortezomib for patients with relapsed and or refractory multiple myeloma. Br. J. Haematol. 160 (5), 714–717. doi: 10.1111/bjh.12154
Poser, I., Dominguez, D., de Herreros, A. G., Varnai, A., Buettner, R., Bosserhoff, A. K. (2001). Loss of E-cadherin expression in melanoma cells involves up-regulation of the transcriptional repressor snail. J. Biol. Chem. 276, 24661–24666. doi: 10.1074/jbc.M011224200
Qian, Y. Y., Zhang, H., Hou, Y., Yuan, L., Li, G. Q., Guo, S. Y., et al. (2012). Celastrus orbiculatus extract inhibits tumor angiogenesis by targeting vascular endothelial growth factor signaling pathway and shows potent antitumor activity in hepatocarcinomas in vitro and in vivo. Chin. J. Integr. Med. 18, 752–760. doi: 10.1007/s11655-011-0819-7
Qiao, W., Yao, Z., Zhang, W., Duan, H. Q. (2009). Two new triterpenes from Duchesnea indica. Chin. Chem. Lett. 20, 572–575. doi: 10.1016/j.cclet.2008.12.052
Qu, Y., Zhang, G., Ji, Y., Zhua, H., Lv, C., Jiang, W. (2016). Protective role of gambogic acid in experimental pulmonary fibrosis in vitro and in vivo. Phytomedicine 23 (4), 350–358. doi: 10.1016/j.phymed.2016.01.011
Raj, L., Ide, T., Gurkar, A. U., Foley, M., Schenone, M., Li, X., et al. (2011). Selective killing of cancer cells by a small molecule targeting the stress response to ROS. Nature 475, 231e234. doi: 10.1038/nature10167
Rastaldi, M. P. (2006). Epithelial-mesenchymal transition and its implications for the development of renal tubulointerstitial fibrosis. J. Nephrol. 19 (4), 407–412.
Russo, M. A., Sansone, L., Polletta, L., Runci, A., Rashid, M. M., De Santis, E., et al. (2014). Sirtuins and resveratrol-derived compounds: a model for understanding the beneficial effects of the Mediterranean diet. Endocr. Metab. Immune Disord. Drug Targets 14 (4), 300–308. Review. doi: 10.2174/1871530314666140709093305
Ryu, Y., Jin, L., Kee, H. J., Piao, Z. H., Cho, J. Y., Kim, G. R., et al. (2016). Gallic acid prevents isoproterenol-induced cardiac hypertrophy and fibrosis through regulation of JNK2 signaling and Smad3 binding activity, Sci. Rep. 6, 34790. doi: 10.1038/srep34790
Sarkar, S., Mandal, C., Sangwan, R., Mandal, C. (2014). Coupling G2/M arrest to the Wnt/β-catenin pathway restrains pancreatic adenocarcinoma. Endocr. Relat. Cancer 21 (1), 113–125. doi: 10.1530/ERC-13-0315
Sato, M., Muragaki, Y., Saika, S., Roberts, A. B., Ooshima, A. (2003). Targeted disruption of TGF-beta1/Smad3 signaling protects against renal tubulointerstitial fibrosis induced by unilateral ureteral obstruction. J. Clin. Invest. 112 (10), 1486–1494. doi: 10.1172/JCI200319270
Savagner, P. (2010). The epithelial-mesenchymal transition (EMT) phenomenon. Ann. Oncol. 21 (Suppl 7), vii89–vii92. doi: 10.1093/annonc/mdq292
Sehitoglu, M. H., Farooqi, A. A., Qureshi, M. Z., Butt, G., Aras, A. (2014). Anthocyanins: targeting of signaling networks in cancer cells. Asian Pac. J. Cancer Prev. 15, 2379–2381. doi: 10.7314/APJCP.2014.15.5.2379
Shakibaei, M., Harikumar, K. B., Aggarwal, B. B. (2009). Resveratrol addiction: to die or not to die. Mol. Nutr. Food Res. 53, 115–128. doi: 10.1002/mnfr.200800148
Shankar, S., Nall, D., Tang, S. N., Meeker, D., Passarini, J., Sharma, J., et al. (2011). Resveratrol inhibits pancreatic cancer stem cell characteristics in human and KrasG12D transgenic mice by inhibiting pluripotency maintaining factors and epithelial-mesenchymal transition. PLoS One 316 (1), e16530. doi: 10.1371/journal.pone.0016530
Sharma, R. A., Euden, S. A., Platton, S. L., Cooke, D. N., Shafayat, A., Hewitt, H. R., et al. (2004). Phase I clinical trial of oral curcumin: biomarkers of systemic activity and compliance. Clin. Cancer Res. 10, 6847–6854. doi: 10.1158/1078-0432.CCR-04-0744
Shen, K. H., Liao, A. C., Hung, J. H., Lee, W. J., Hu, K. C., Lin, P. T., et al. (2014). α-Solanine inhibits invasion of human prostate cancer cell by suppressing epithelial-mesenchymal transition and MMPs expression. Molecules 1119 (8), 11896–11914. doi: 10.3390/molecules190811896
Shen, X. C., Yang, Y. P., Xiao, T. T., Peng, J., Liu, X. D. (2011). Protective effect of oxymatrine on myocardial fibrosis induced by acute myocardial infarction in rats involved in TGF-β1-Smad signal pathway. J. Asian Nat. Prod. Res. 13 (3), 215–224. doi: 10.1080/10286020.2010.550883
Sheu, M. L., Chiang, C. K., Tsai, K. S., Ho, F. M., Weng, T. I., Wu, H. Y., et al. (2008). Inhibition of NADPH oxidase-related oxidative stress-triggered signaling by honokiol suppresses high glucose-induced human endothelial cell apoptosis. Free Radic. Biol. Med. 44, 2043–2050. doi: 10.1016/j.freeradbiomed.2008.03.014
Shi, G. F., Li, Q. (2005). Effects of oxymatrine on experimental hepatic fibrosis and its mechanism in vivo. World J. Gastroenterol. 11 (2), 268–271. doi: 10.3748/wjg.v11.i2.268
Shibata, S., Marushima, H., Asakura, T., Matsuura, T., Eda, H., Aoki, K., et al. (2009). Three-dimensional culture using a radial flow bioreactor induces matrix metalloprotease 7-mediated EMT-like process in tumor cells via TGFbeta1/Smad pathway. Int. J. Oncol. 34 (5), 1433–1448.
Song, Y. H., Sun, H., Zhang, A., Yan, G., Han, Y., Wang, X. (2014). Plant-derived natural products as leads to anti-cancer drugs. J. Med. Plant Herb. Ther. Res. 2, 6–15.
Su, N.-W., Wu, S.-H., Chi, C.-W., Liu, C.-J., Tsai, T.-H., Chen, Y.-J. (2017). Metronomic cordycepin therapy prolongs survival of oral cancer-bearing mice and inhibits epithelial-mesenchymal transition. Molecules 22, 629. doi: 10.3390/molecules22040629
Subramani, R., Gonzalez, E., Arumugam, A., Nandy, S., Gonzalez, V., Medel, J., et al. (2016). Nimbolide inhibits pancreatic cancer growth and metastasis through ROS-mediated apoptosis and inhibition of epithelial-to-mesenchymal transition. Sci. Rep. 6, 19819. doi: 10.1038/srep19819
Subramani, R., Gonzalez, E., Nandy, S. B., Arumugam, A., Camacho, F., Medel, J., et al. (2017). Gedunin inhibits pancreatic cancer by altering sonic hedgehog signaling pathway. Oncotarget 8 (7), 10891–10904. doi: 10.18632/oncotarget.8055
Sun, L. N., Chen, Z. X., Liu, X. C., Liu, H. Y., Guan, G. J., Liu, G. (2014). Curcumin ameliorates epithelial-to mesenchymal transition of podocytes in vivo and in vitro via regulating caveolin-1. Biomed. Pharmacother. 68 (8), 1079–1088. doi: 10.1016/j.biopha.2014.10.005
Sun, M., Zhang, N., Wang, X., Cai, C., Cun, J., Li, Y., et al. (2014). Nitidine chloride induces apoptosis, cell cycle arrest, and synergistic cytotoxicity with doxorubicin in breast cancer cells. Tumour Biol. 35 (10), 10201–10212. doi: 10.1007/s13277-014-2327-9
Sun, M., Zhang, N., Wang, X., Li, Y., Qi, W., Zhang, H., et al. (2016). Hedgehog pathway is involved in nitidine chloride induced inhibition of epithelial-mesenchymal transition and cancer stem cells-like properties in breast cancer cells. Cell Biosci. 6, 44. doi: 10.1186/s13578-016-0104-8
Sutariya, Brijesh, S. (2017). Betanin, isolated from fruits of Opuntia elatior Mill attenuates renal fibrosis in diabetic rats through regulating oxidative stress and TGF-β pathway. J. Ethnopharmacol. 198, 432–443. doi: 10.1016/j.jep.2016.12.048
Tan, C., Mui, A., Dedhar, S. (2002). Integrin-linked kinase regulate inducible nitric oxide synthase and cyclooxygenase-2 expression in an NF-kB-dependent manner. J. Biol. Chem. 277, 3109–3116. doi: 10.1074/jbc.M108673200
Thiery, J. P., Acloque, H., Huang, R. Y., Nieto, M. A. (2009). Epithelialmesenchymal transitions in development and disease. Cell 139, 871–890. doi: 10.1016/j.cell.2009.11.007
Tillhon, M., Guaman Ortiz, L. M., Lombardi, P., Scovassi, A. I. (2012). Berberine: new perspectives for old remedies. Biochem. Pharmacol. 84, 1260–1267. doi: 10.1016/j.bcp.2012.07.018
Troussard, A. A., Costello, P., Yoganathan, T. N., Kumagai, S., Roskelley, C. D., Dedhar, S. (2000). The integrin linked kinase (ILK) induces an invasive phenotype via AP-1 transcription factordependent upregulation of matrix metalloproteinase 9 (MMP-9). Oncogene 16, 5444–5452. doi: 10.1038/sj.onc.1203928
Tsai, J. H., Hsu, L. S., Lin, C. L., Hong, H. M., Pan, M. H., Way, T.-D., et al. (2013). 3,5,4′-Trimethoxystilbene, a natural methoxylated analog of resveratrol, inhibits breast cancer cell invasiveness by downregulation of PI3K/Akt and Wnt/beta-catenin signaling cascades and reversal of epithelial-mesenchymal transition. Toxicol. Appl. Pharmacol. 272, 746–756. doi: 10.1016/j.taap.2013.07.019
Unahabhokha, T., Chanvorachote, P., Pongrakhananon, V. (2016). The attenuation of epithelial to mesenchymal transition and induction of anoikis by gigantol in human lung cancer H460 cells. Tumour Biol. 37 (7), 8633–8641. doi: 10.1007/s13277-015-4717-z
Vega, S., Morales, A. V., Ocaña, O. H., Valdés, F., Fabregat, I., Nieto, M. A. (2004). Snail blocks the cell cycle and confers resistance to cell death. Gene Dev. 18, 1131–1143. doi: 10.1101/gad.294104
Venkatesha, S. H., Yu, H., Rajaiah, R., Tong, L., Moudgil, K. D. (2011). Celastrus-derived celastrol suppresses autoimmune arthritis by modulating antigen-induced cellular and humoral effector responses. J. Biol. Chem. 286 (17), 15138–15146. doi: 10.1074/jbc.M111.226365
Vergara, D., Valente, C. M., Tinelli, A., Siciliano, C., Lorusso, V., Acierno, R., et al. (2011). Resveratrol inhibits the epidermal growth factor-induced epithelial mesenchymal transition in MCF-7 cells. Cancer Lett. 310 (1), 1–8. doi: 10.1016/j.canlet.2011.04.009
Vergara, D., et al. (2014). Antitumor activity of the dietary diterpene carnosol against a panel of human cancer cell lines. Food Funct. 5, 1261–1269. doi: 10.1039/c4fo00023d
von Gise, A., Pu, W. T. (2012). Endocardial and epicardial epithelial to mesenchymal transitions in heart development and disease. Circ. Res. 110 (12), 1628–1645. doi: 10.1161/CIRCRESAHA.111.259960
Vuddanda, P. R., Chakraborty, S., Singh, S. (2010). Berberine: a potential phytochemical with multispectrum therapeutic activities. Expert Opin. Invest. Drugs 19, 1297–1307. doi: 10.1517/13543784.2010.517745
Wallerath, T., Deckert, G., Ternes, T., Anderson, H., Li, H., Witte, K., et al. (2002). Resveratrol, a polyphenolic phytoalexin present in red wine, enhances expression and activity of endothelial nitric oxide synthase. Circulation 106 (13), 1652–1658. doi: 10.1161/01.CIR.0000029925.18593.5C
Wang, J., Qiao, L., Li, Y., Yang, G. (2008). Ginsenoside Rb1 attenuates intestinal ischemia-reperfusion-induced liver injury by inhibiting NF-κB activation. Exp. Mol. Med. 40, 686–698. doi: 10.3858/emm.2008.40.6.686
Wang, Z., Jiang, W., Zhang, Z., Qian, M., Du, B. (2012). Nitidine chloride inhibits LPS-induced inflammatory cytokines production via MAPK and NF-kappaB pathway in RAW 264.7 cells. J. Ethnopharmacol. 144 (1), 145–150. doi: 10.1016/j.jep.2012.08.041
Wang, H., Zhang, H., Tang, L., Chen, H., Wu, C., Zhao, M., et al. (2013). Resveratrol inhibits TGF-β1-induced epithelial-to-mesenchymal transition and suppresses lung cancer invasion and metástasis. Toxicología 303, 139–146. doi: 10.1016/j.tox.2012.09.017
Wang, D., Zou, Y., Zhuang, X., Chen, S., Lin, Y., Li, W., et al. (2017a). Sulforaphane suppresses EMT and metastasis in human lung cancer through miR-616-5p-mediated GSK3β/β-catenin signaling pathways. Acta Pharmacol. Sin. 38 (2), 241–251. doi: 10.1038/aps.2016.122
Wang, H., Gu, H., Feng, J., Qian, Y., Yang, L., Jin, F., et al. (2017b). Celastrus orbiculatus extract suppresses the epithelial-mesenchymal transition by mediating cytoskeleton rearrangement via inhibition of the Cofilin 1 signaling pathway in human gastric cancer. Oncol. Lett. 14 (3), 2926–2932. doi: 10.3892/ol.2017.6470
Wang, W., Zhou, P. H., Hu, W., Xu, C. G., Zhou, X. J., Liang, C. Z., et al. (2017c). Cryptotanshinone hinders renal fibrosis and epithelial transdifferentiation in obstructive nephropathy by inhibiting TGF β1/Smad3/integrin β1 signal. Oncotarget 9 (42), 26625–26637. doi: 10.18632/oncotarget.23803
Wang, Z., Liu, Z., Yu, G., Nie, X., Jia, W., Liu, R., et al. (2018). Paeoniflorin inhibits migration and invasion of human glioblastoma cells via suppression transforming growth factor β-induced epithelial–mesenchymal transition. Neurochem. Res. 43 (3), 760–774. doi: 10.1007/s11064-018-2478-y
Wen, Y. C., Lee, W. J., Tan, P., Yang, S. F., Hsiao, M., Lee, L. M., et al. (2015). By inhibiting snail signaling and miR-23a-3p, osthole suppresses the EMT-mediated metastatic ability in prostate cancer. Oncotarget 6 (25), 21120–21136. doi: 10.18632/oncotarget.4229
Widodo, N., Kaur, K., Shrestha, B. G., Takagi, Y., Ishii, T., Wadhwa, R., et al. (2007). Selective killing of cancer cells by leaf extract of Ashwagandha: identification of a tumor-inhibitory factor and the first molecular insights to its effect. Clin. Cancer Res. 13, 2298–2306. doi: 10.1158/1078-0432.CCR-06-0948
White, C. M., Lee, J. Y. (2019). The impact of turmeric or its curcumin extract on nonalcoholic fatty liver disease: a systematic review of clinical trials. Pharm. Pract. (Granada) 17 (1), 1350. doi: 10.18549/PharmPract.2019.1.1350
Wong, K. F., Yuan, Y., Luk, J. M. (2012). Tripterygium wilfordii bioactive compounds as anticancer and anti-inflammatory agents. Clin. Exp. Pharmacol. Physiol. 39 (3), 311–320. doi: 10.1111/j.1440-1681.2011.05586.x
Wrighton, K. H., Lin, X., Feng, X. H. (2009). Phospho-control of TGF-beta superfamily signaling. Cell Res. 19, 8–20. doi: 10.1038/cr.2008.327
Wu, L., Li, Q., Liu, Y. (2014). Polyphyllin D induces apoptosis in K562/A02 cells through G2/M phase arrest. J. Pharm. Pharmacol. 66, 713–721. doi: 10.1111/jphp.12188
Wu, X., Zeng, W. Z., Jiang, M. D., Qin, J. P., Xu, H. (2008). Effect of Oxymatrine on the TGF beta-Smad signaling pathway in rats with CCl4-induced hepatic fibrosis. World J. Gastroenterol. 14 (13), 2100–2105. doi: 10.3748/wjg.14.2100
Xiao, Z., Chen, C., Meng, T., Zhang, W., Zhou, Q. (2016). Resveratrol attenuates renal injury and fibrosis by inhibiting transforming growth factor-beta pathway on matrix metalloproteinase 7. Exp. Biol. Med. 241, 140–146. doi: 10.1177/1535370215598401
Xiao, S., Zhang, M., Liang, Y., Wang, D. (2017). Celastrol synergizes with oral nifedipine to attenuate hypertension in preeclampsia: a randomized, placebo-controlled, and double blinded trial. J. Am. Soc. Hypertens. 11 (9), 598–603. doi: 10.1016/j.jash.2017.07.004
Xing, S., Yu, W., Zhang, X., Luo, Y., Lei, Z., Huang, D., et al. (2018). Isoviolanthin extracted from Dendrobium officinale reverses TGF-β1-mediated epithelial-mesenchymal transition in hepatocellular carcinoma cells via deactivating the TGF-β/Smad and PI3K/Akt/mTOR signaling pathways. Int. J. Mol. Sci. 19 (6), E1556. doi: 10.3390/ijms19061556
Xu, T., Wu, X., Chen, Q., Zhu, S., Liu, Y., Pan, D., et al. (2014). The anti-apoptotic and cardioprotective effects of salvianolic acid a on rat cardiomyocytes following ischemia/reperfusion by DUSP-mediated regulation of the ERK1/2/JNK pathway. PLoS One 9 (7), e102292. doi: 10.1371/journal.pone.0102292
Xu, Y., Lou, Z., Lee, S. H. (2017). Arctigenin represses TGF-β-induced epithelial mesenchymal transition in human lung cancer cells. Biochem. Biophys. Res. Commun. 493 (2), 934–939. doi: 10.1016/j.bbrc.2017.09.117
Xu, Q., Zong, L., Chen, X., Jiang, Z., Nan, L., Li, J., et al. (2015). Resveratrol in the treatment of pancreatic cancer. Ann. N. Y. Acad. Sci. 1348, 10–19. doi: 10.1111/nyas.12837
Xu, J., Wang, J. H., Xue, W. (2009). Clinical analysis of the preventive effect of Schisandra chinensis on anti-tuberculosis therapy induced liver injury. J. Yichun College 36 (6), 65–67.
Yáñez-Mó, M., Lara-Pezzi, E., Selgas, R., Ramírez-Huesca, M., Domínguez-Jiménez, C., Jiménez-Heffernan, J. A., et al. (2003). Peritoneal dialysis and epithelial-to-mesenchymal transition of mesothelial cells. N. Engl. J. Med. 348 (5), 403–413.
Yan, F., Zhang, G. H., Feng, M., Zhang, W., Zhang, J. N., Dong, W. Q., et al. (2015). Glucagon-like Peptide 1 protects against hyperglycemic-induced endothelial-to-mesenchymal transition and improves myocardial dysfunction by suppressing Poly(ADP-Ribose) polymerase 1 activity. Mol. Med. 21, 15–25. doi: 10.2119/molmed.2014.00259
Yang, Y. T., Weng, C. J., Ho, C. T., Yen, G. C. (2009). Resveratrol analog-3,5,4-trimethoxytrans-stilbene inhibits invasion of human lung adenocarcinoma cells by suppressing the MAPK pathway and decreasing matrix metalloproteinase-2 expression. Mol. Nutr. Food Res. 53, 407–416. doi: 10.1002/mnfr.200800123
Yang, L., Xing, S., Wang, K., Yi, H., Du, B. (2018). Paeonol attenuates aging MRC-5 cells and inhibits epithelial-mesenchymal transition of premalignant HaCaT cells induced by aging MRC-5 cell-conditioned medium. Mol. Cell Biochem. 439 (1–2), 117–129. doi: 10.1007/s11010-017-3141-7
Yang, M., Akbar, U., Mohan, C. (2019). Curcumin in autoimmune and rheumatic diseases. Nutrients 2, 11(5). doi: 10.3390/nu11051004
Yin, J., Xing, H., Ye, J. (2008). Efficacy of berberine in patients with type 2 diabetes mellitus. Metabolism 57 (5), 712–717. doi: 10.1016/j.metabol.2008.01.013
Yoo, K. H., Thornhill, B. A., Forbes, M. S., Coleman, C. M., Marcinko, E. S., Liaw, L., et al. (2006). Osteopontin regulates renal apoptosis and interstitial fibrosis in neonatal chronic unilateral ureteral obstruction. Kidney Int. 70 (10), 1735–41. doi: 10.1038/sj.ki.5000357
Yu, Q., Li, Q., Lu, P., Chen, Q. (2014). Polyphyllin D induces apoptosis in U87 human glioma cells through the c-Jun NH2-terminal kinase pathway. J. Med. Food 17, 1036–1042. doi: 10.1089/jmf.2013.2957
Yuan, T., Chen, Y., Zhang, H., Fang, L., Du, G. (2017). Salvianolic acid A, a component of salvia miltiorrhiza, attenuates endothelial–mesenchymal transition of HPAECs induced by hypoxia. Am. J. Chin. Med. 45 (6), 1185–1200. doi: 10.1142/S0192415X17500653
Yuan, X. P., Liu, L. S., Fu, Q., Wang, C. X. (2012). Effects of ligustrazine on ureteral obstruction-induced renal tubulointerstitial fibrosis. Phytother. Res. 26 (5), 697–703. doi: 10.1002/ptr.3630
Zang, M. D., Hu, L., Fan, Z. Y., Wang, H. X., Zhu, Z. L., Cao, S., et al. (2017). Luteolin suppresses gastric cancer progression by reversing epithelial-mesenchymal transition via suppression of the Notch signaling pathway. J. Transl. Med. 15 (1), 52. doi: 10.1186/s12967-017-1151-6
Zeng, J., Dou, Y., Guo, J., Wu, X., Dai, Y. (2013). Paeoniflorin de Paeonia lactiflora previene Fibrosis intersticial renal inducida por obstrucción ureteral unilateral en ratones. Fitomedicina 20 (8–9), 753–759. doi: 10.1016/j.phymed.2013.02.010
Zhang, H., Qian, Y., Liu, Y., Li, G., Cui, P., Zhu, Y., et al. (2012a). Celastrus orbiculatus extract induces mitochon- drial-mediated apoptosis in human hepatocellular carcinoma cells. J. Tradit. Chin. Med. 32, 621–626. doi: 10.1016/S0254-6272(13)60081-3
Zhang, L., Jiang, G., Yao, F., He, Y., Liang, G., Zhang, Y., et al. (2012b). Growth inhibition and apoptosis induced by osthole, a natural coumarin, in hepatocellular carcinoma. PLoS One 7 (5), e37865. doi: 10.1371/journal.pone.0037865
Zhang, J., Cao, H., Zhang, B., Cao, H., Xu, X., Ruan, H., et al. (2013). Berberine potently attenuates intestinal polyps growth in ApcMin mice and familial adenomatous polyposis patients through inhibition of Wnt signalling. J. Cell. Mol. Med. 17 (11), 1484–1493. doi: 10.1111/jcmm.12119
Zhang, L., Tao, L., Shi, T., Zhang, F., Sheng, X., Cao, Y., et al. (2015). Paeonol inhibits B16F10 melanoma metastasis in vitro and in vivo via disrupting proin- flammatory cytokines-mediated NF-kappaB and STAT3 pathways. IUBMB Life 67, 778–788. doi: 10.1002/iub.1435
Zhang, Z., Liu, T., Yu, M., Li, K., Li, W. (2018). The plant alkaloid tetrandrine inhibits metastasis via autophagy-dependent Wnt/β-catenin and metastatic tumor antigen 1 signaling in human liver cancer cells. J. Exp. Clin. Cancer Res. 37, 7. doi: 10.1186/s13046-018-0678-6
Zhao, M., Lau, S. T., Leung, P. S., Che, C. T., Lin, Z. X. (2011). Seven quassinoids from Fructus Bruceae with cytotoxic effects on pancreatic adenocarcinoma cell lines. Phytother. Res. 25 (12), 1796–800. doi: 10.1002/ptr.3477
Zhao, K., Yiang, Y., Xue, P.-F., Tu, P.-F. (2013). Chemical constituents from barks of Cinnamomum cassia growing in China. Chin. Tradit. Herb. Drugs 44, 2358–2363. doi: 10.7501/j.issn.0253-2670.2013.17.005
Zheng, J., Son, D. J., Gu, S. M., Woo, J. R., Ham, Y. W., Lee, H. P., et al. (2016). Piperlongumine inhibits lung tumor growth via inhibition of nuclear factor kappa B signaling pathway. Sci. Rep. 6, 26357. doi: 10.1038/srep26357
Zheng, Y., Nan, H., Hao, M., Song, C., Zhou, Y., Gao, Y. (2013). Antiproliferative effects of protopanaxadiol ginsenosides on human colorectal cancer cells. Biomed. Rep. 1, 555–558. doi: 10.3892/br.2013.104
Zhou, L. X., Liang, L., Zhang, L., Yang, N., Nagao, H., Wu, C., et al. (2016). MiR-27a-3p functions as an oncogene in gastric cancer by targeting BTG2. Oncotarget 7, 51943–51954. doi: 10.18632/oncotarget.10460
Zhou, Y., Mao, H., Li, S., Cao, S., Li, Z., Zhuang, S., et al. (2010). HSP72 inhibits Smad3 activation and nuclear translocation in renal epithelial-tomesenchymal transition. J. Am. Soc. Nephrol. 21, 598–609. doi: 10.1681/ASN.2009050552
Zhou, J., Zhu, Y. F., Chen, X. Y., Han, B., Li, F., Chen, J. Y., et al. (2017). Black rice-derived anthocyanins inhibit HER-2-positive breast cancer epithelial-mesenchymal transition-mediated metastasis in vitro by suppressing FAK signaling. Int. J. Mol. Med. 40 (6), 1649–1656. doi: 10.3892/ijmm.2017.3183
Zhu, Z., Zhao, Y., Li, J., Tao, L., Shi, P., Wei, Z., et al. (2016). Cryptotanshinone, a novel tumor angiogenesis inhibitor, destabilizes tumor necrosis factor-α mRNA via decreasing nuclear-cytoplasmic translocation of RNA-binding protein HuR. Mol. Carcinog. 55 (10), 1399–1410. doi: 10.1002/mc.22383
Keywords: natural plants compounds, epithelial-to-mesenchymal transition (EMT), anti-fibrotic, anti-inflammatory, anti-oxidant agent
Citation: Avila-Carrasco L, Majano P, Sánchez-Toméro JA, Selgas R, López-Cabrera M, Aguilera A and Mateo GG (2019) Natural Plants Compounds as Modulators of Epithelial-to-Mesenchymal Transition. Front. Pharmacol. 10:715. doi: 10.3389/fphar.2019.00715
Received: 10 March 2019; Accepted: 05 June 2019;
Published: 30 July 2019.
Edited by:
Raffaele Strippoli, Sapienza University of Rome, ItalyReviewed by:
Marco Cordani, IMDEA Nanociencia, SpainGautam Sethi, National University of Singapore, Singapore
Copyright © 2019 Avila-Carrasco, Majano, Sánchez-Toméro, Selgas, López-Cabrera, Aguilera and González Mateo. This is an open-access article distributed under the terms of the Creative Commons Attribution License (CC BY). The use, distribution or reproduction in other forums is permitted, provided the original author(s) and the copyright owner(s) are credited and that the original publication in this journal is cited, in accordance with accepted academic practice. No use, distribution or reproduction is permitted which does not comply with these terms.
*Correspondence: Lorena Avila-Carrasco, bGFjZG9jX0Bob3RtYWlsLmNvbQ==
†These authors have contributed equally to this work.
‡In memoriam.