- 1Department of Basic Sciences, School of Dentistry of Araçatuba, São Paulo State University (UNESP), São Paulo, Brazil
- 2Programa Multicêntrico de Pós-graduação em Ciências Fisiológicas, School of Dentistry of Araçatuba, São Paulo State University (UNESP), São Paulo, Brazil
- 3Department of Stomatology, Bauru School of Dentistry, University of São Paulo (USP), Bauru, Brazil
- 4Department of Biological Sciences, Bauru School of Dentistry, University of São Paulo (USP), Bauru, Brazil
The aim of this study was to characterize the role of local RAS (renin–angiotensin system) in the inflammatory response of normal (N) and diabetic (D) mice with periodontal disease (PD). Diabetes Mellitus (DM) was induced by peritoneal injection of streptozotocin in Balb/c mice. PD was induced by ligature around the first molar in both N and D, irrespective of whether they were treated with aliskiren (50 mg/kg, Alisk). Mandibles were harvested for histomorphometric analyses, and gingival tissue (GT) was collected to evaluate gene expression and extracellular matrix components (ECM). Immunohistochemical (IHC) analyses were used to localize RAS in GT. The production of C-reactive protein (CRP), IL-1β, CXCL2, and CCL8 was evaluated by enzyme-linked immunosorbent assay (ELISA). Renin was found to exacerbate the inflammation and periodontal bone loss at 14 days after PD, and Alisk inhibited this process in GT of N and D. PD increased CRP, CXCL2, CCL8, and IL-1β production in both animals. Alisk could inhibit CRP, CXCL2, and CCL8 primarily in D animals. However, only CCL8 was decreased in N animals after Alisk pretreatment. PD enhanced expression and production of AGT, ACE, AT1R, and AT2R in both N and D. AT1R expression was higher in D with PD, and AT2R expression was higher in N with PD. ACE2 and receptor Mas (MasR) expression and production was elevated in the control group of both animals. PD inhibited ACE2 in N but not in D. MasR expression was unaffected in both N and D with PD. Alisk reduced expression and production of all RAS components in GT of both animals, except for ACE2 in N. RAS staining was observed in all layers of epithelium, basal cell layer, and lamina propria and was higher in N with PD. Col1a1, Col1a2, Col3a1, and fibronectin (Fn1) were increased in both animals with PD. Alisk inhibited Col1a1 and Fn in both animals, Col1a2 was decreased only in D, while levels of Col3a1 remained unchanged in all animal groups. In conclusion, these data demonstrated the presence and functional role of local RAS in GT, exacerbating the inflammatory response, periodontal bone loss, and wound healing processes in both N and D animal groups. In addition, Alisk was able to significantly reduce gingival inflammation, excessive wound healing processes, and periodontal bone loss.
Introduction
Systemic renin–angiotensin system (RAS) is an endocrine axis responsible for modulating a variety of physiological and pathological processes such as blood pressure, hydroelectrolytic balance, inflammation, and oxidative stress (Zablocki and Sadoshima, 2013; Itinteang et al., 2015; Wang et al., 2015; Xue et al., 2016). The classical RAS consists of circulating renin, acting on angiotensinogen (AGT) to produce angiotensin I (AngI), which in turn is converted into angiotensin II (AngII) by angiotensin-converting enzyme (ACE). Some studies have demonstrated the presence of local RAS components in oral tissues such as guinea pig and rabbit gingival fibroblasts (Ohuchi et al., 2002; Ohuchi et al., 2004) and ferret gingiva in vivo (Berggreen and Heyeraas, 2003). Recent studies have demonstrated an association between diabetes and RAS, particularly a prominent involvement of AngII in diabetic complications, such as nephropathy and cardiovascular dysfunction (Ribeiro-Oliveira et al., 2008). One of the most important landmarks was the discovery of local RAS as described by Santos et al. (2009), where the existence of local RAS in rat gingival tissue was demonstrated for the first time. These authors reported that antagonizing AT1R and renin can significantly prevent periodontal bone loss induced by PD in rats (Santos et al., 2015). Moreover, recent studies have shown that RAS blockers are able to reduce the inflammation and, therefore, decrease the expression of matrix metalloproteinases (MMPs) in RANK/RANKL axis and cathepsin K in a rat model of PD (Araújo et al., 2013). Furthermore, our knowledge about this concept is growing due to increase in the number of studies that have described treatment of mice with ACE inhibitor preventing bone loss Gonçalves-Zillo et al. (2013), the protective effect of ACE inhibitors in PD-induced and arthritis-associated alveolar bone loss (Queiroz-Junior et al., 2015), and the mechanism by which AT1R inhibitor prevents inflammation and alveolar bone loss in periodontitis (Li et al., 2018).
Periodontal disease (PD) has a high prevalence worldwide and is considered to be the second largest cause of dental pathology in human population (Mumghamba et al., 1995). It is characterized by a chronic infection leading to destruction of periodontal tissues, resulting in loss of connective soft tissue and bone and formation of periodontal pockets that eventually cause teeth loss (Okada and Murakami, 1998; Bascones-Martinez et al., 2011). Most of these disease pathologies are caused due to an exacerbated inappropriate immune response. The circulating cytokines and chemokines have been detected at elevated levels in the gingival crevicular fluid (GCF) and saliva of patients who have PD, making them putative biomarkers of the disease (Lamster, 1997; Gemmell et al., 2001; Sexton et al., 2011; Stadler et al., 2016; Aldahlawi and Youssef, 2018). CXCL2-like-related chemokines are powerful neutrophil chemoattractants and are involved in many immune responses including wound healing and periodontitis. Elevated levels of CXCL2 leads to increased recruitment of neutrophils to periodontal ligaments in diabetic mice with induced PD (Greer et al., 2016; Zheng et al., 2018). Another important chemokine is CCL8, which is involved in leukocyte cell activation and is associated with the severity of periodontitis (Panezai et al., 2017). The association of periodontitis with systemic inflammation diseases has been a target of various studies to understand the mechanisms involved in the process.
Diabetes has been confirmed as a major risk factor for periodontitis (Khader et al., 2006; Chávarry et al., 2009). Diabetic patients have impaired immune functioning, especially due to polymorphonuclear cells, which when present reduce phagocytic and microbicidal functions in the patient’s body (Drachman et al., 1966). The decrease in cellular functions occurring in diabetes is due to high concentrations of glucose to which the host cells are exposed, reducing immunological function and making the host more susceptible to develop a variety of pathologies including periodontitis (Loe, 1993; Marhoffer et al., 1992; Geerlings and Hoepelman, 1999; Shah and Hux, 2003; Muller et al., 2005). In our study, we used a direct renin inhibitor available for clinical use. This pharmacological modulation may be a useful tool to understand the effects of this drug in the interaction between RAS, PD, and systemic disease, thereby improving the quality of life of individuals with these pathologies. Thus, the aim of this study was to characterize the local RAS and the role of renin in inflammatory response of normal (N) and diabetic mice (D) after experimental PD was induced. We observed that renin exacerbates the inflammatory response and periodontal bone loss in PD-induced mice, and this effect is more intense in hyperglycemic animals. Furthermore, we verified the existence of the components of local RAS in the gingival tissue as well as their role in tissue repair.
Materials and methods
Animals, Ethical Aspects, and Experimental Design
Animal care and experimental protocols were in accordance with the Brazil’s National Council for Animal Experiments Control and were approved by the Ethics Committee on Animal Use from School of Dentistry of Araçatuba (UNESP) (Process FOA-00106-2016). Efforts were made to minimize animal suffering and reduce the number of subjects. Thirty six adult male Balb/c mice (30 ± 5 g) from the Animal Facility of the Department of Basic Sciences (School of Dentistry of Araçatuba, UNESP) were used. Animals were housed in individually ventilated cages in an environment with controlled light (12 h light/dark cycle), temperature (22 ± 2°C), and humidity (55 ± 5%), offered with standard pellet diet and drinking water ad libitum. The animals were randomly divided into six experimental groups (n = 12), as follows: Normal Control, Normal with PD, aliskiren-treated normal animals with PD, Diabetic Control, Diabetic with PD, and aliskiren-treated diabetic animals with PD.
Diabetes Induction
For diabetes induction, mice received 200 mg/kg streptozotocin intraperitoneally (Sigma-Aldrich, St. Louis, MO, USA) diluted in citrate buffer (100 mM, pH 4.5). After 7 days, glycemia levels were measured by a glucometer (One Touch Ultra 2, Johnson & Johnson Medical, Milpitas, CA, USA) and animals were considered to be diabetic when values are shown to be 250 mg/dl or higher (Kim et al., 2014).
Periodontal Disease Induction
PD was induced by bilateral insertion of a ligature around the lower first molar, kept for 14 days, as previously described (Bonato et al., 2012). Briefly, the animals were anesthetized with 80 mg/kg ketamine hydrochloride (Cetamim, Syntec; Hortolândia, São Paulo, Brazil) associated with 10 mg/kg xylazine hydrochloride (Calmium, AgenerUnião; Embu-Guaçu, São Paulo, Brazil) and were placed in the ventral decubitus position at a dental table for rodents, with oral retractors supported on the incisive teeth. A 4-0 silk thread (Shalon; Goiânia, Goiás, Brazil) was wrapped around the first inferior molars, carefully pushing the ligature into the gingival sulcus, and knotting mesially. Control groups did not receive the ligature. However, they were anesthetized and manipulated similarly to undergo the same stress as the others.
Aliskiren Treatment
Aliskiren was daily administered, at 50 mg/kg on PBS pH 7.4, as described by Santos et al. (2015) using an oral gavage, starting 1 day before PD induction and maintained until euthanasia. Control groups were manipulated similarly and received only PBS pH 7.4 to undergo the same stress as the others.
Euthanasia and Sample Harvest
On day 15 after PD induction, the animals were euthanized by halothane (Tanohalo; Cristália, Itapira, SP, Brazil) by overdose inhalation. The presence of bilateral ligature was evaluated, and the animals in which it was absent were excluded from the study. The marginal gingival was surgically harvested in clean condition, immediately frozen in liquid nitrogen, subsequently stored at −80°C until used for cytokine quantification and gene expression analyses, or fixed for histological processing. Hemi-mandibles were also harvested to evaluate the alveolar bone lost by histological methods.
Histomorphological Analysis
Gingival tissue and hemi-mandible were fixed in 10% formaldehyde buffered solution for 48 h. Tissue was washed under tap water for 24 h, and hemi-mandibles were decalcified with 10% ethylenediaminetetraacetic acid solution for 60 days. After this, tissue was dehydrated and embedded in paraffin and 5-µm-thick sections longitudinally were obtained. To determine bone resorption, the total area of furcation region and the alveolar bone area within were measured with the software ImageJ (Version 1.47, National Institutes of Health, Bethesda, MD, USA). Finally, the alveolar bone percentage was calculated.
Immunohistochemical Analysis
Paraffin sections were obtained as described above and immunostained to determine the expression of renin (Renin), angiotensinogen (AGT), angiotensin I converting enzyme 1 (ACE), angiotensin I converting enzyme 2 (ACE2), angiotensin II receptor type 1 (AT1R), angiotensin II receptor type 2 (AT2R), and receptor Ag1-7 Mas (MasR). Primary antibodies that were used (Santa Cruz Biotechnology, Santa Cruz, CA, USA) included rabbit polyclonal anti-renin (1:50), rabbit polyclonal anti-AGT (1:50), rabbit polyclonal anti-ACE (1:50), anti-ACE2 (1:50), goat polyclonal anti-AT1R (1:250), anti-AT2R (1:100), and goat polyclonal anti-MasR (1:75). Antibodies binging was detected using Histofine® Simple Stain™ Mouse MAX PO (goat and rabbit) (Nichirei Biosciences Inc.; Tokyo, Japan) and 3,3’-diaminobenzidine-tetrahydrochloride (Dako Corp., Carpinteria, CA, USA) as chromogenic substrate. The procedure was completed using Harry’s hematoxylin for counterstaining. All assays were accompanied by a negative control where primary antibody was not used. Positive controls were performed on paraffin-embedded longitudinal sections of kidney for Renin, ACE, ACE2, AT1R, AT2R, and MasR and longitudinal sections of heart for AGT. For immunohistochemical (IHC) analysis, five sections from each group were randomly selected to examine the immunolabeling in gingival epithelium and connective tissue and were performed by three independent and blind researchers. In brief, expression was based on intensity of the immunostaining [negative (−); weak (+); moderate (++); strong (+++)], as described by Santos et al. (2015) with some modifications. Representative slides were photographed with a digital camera (Olympus, XC50, Tokyo, Japan), which was coupled to a light microscope (Olympus, BX53, Tokyo, Japan).
Cytokines Quantification by Enzyme-Linked Immunosorbent Assay
The gingival samples from the same animal were pooled together and sonicated until total tissue was disintegrated in lysis buffer (PBS pH 7.4 plus protease inhibitor cocktail), which was then centrifuged, and the supernatant was used for quantification of C-reactive protein (CRP), IL-1β, CXCL2, and CCL8 by sandwich enzyme-linked immunosorbent assay (ELISA). Each pool was considered n = 1, and final experimental number was n = 5. Antibodies and standards were purchased from R&D Systems (Minneapolis, MN, USA), and antibody concentrations that were used had been previously standardized. Assays were performed in 96-well microplates coated with the respective capture antibodies for 18 h at 4°C. Nonspecific binding sites were blocked with blocking buffer (PBS plus 1% bovine serum albumin) for 2 h at room temperature. Samples and standards were loaded and incubated for 2 h at room temperature under constant agitation. Respective biotinylated antibody was added and incubated for 1 h at room temperature. Horseradish peroxidase-conjugated streptavidin in blocking buffer was added and incubated for 1 h at room temperature, followed by addition of chromogenic substrate solution (3,3′,5,5′-tetramethylbenzidine). Reaction was conducted for 30 min and stopped with 4 M sulfuric acid, and OD was measured at wavelength 450 nm. Plates were washed four times with washing buffer (PBS pH 7.4, with 0.1% Tween 20) after each step. Standard curve consisted of two-fold serial dilution of respective recombinant cytokines in blocking buffer (ranging from 2000 to 1.95 pg/ml), and their concentrations in samples were calculated in relation to the standard curve.
Gene Expression Analysis by Real-Time Reverse Transcription Polymerase Chain Reaction
Gingival tissue was homogenized with TRIzol reagent (Invitrogen, Thermo Fisher Scientific; Carlsbad, CA, USA), and total RNA was extracted following the manufacturer’s instructions. Samples were treated with DNAse I (Sigma-Aldrich), RNA was quantified by fluorometry with commercial kit (Quant-iTRiboGreen RNA Assay Kit, Invitrogen), and samples were reverse transcribed to complementary DNA with High Capacity RNA-to-cDNA™ Kit (Applied Biosystems, Thermo Fisher Scientific; Foster City, California, USA), according to the manufacturer’s instructions.
Gene expression analysis of RAS and tissue repair targets was performed by real-time reverse transcription polymerase chain reaction (qRT-PCR), using StepOnePlus™ Real-Time PCR Systems and TaqMan™ Gene Expression Assays (FAM fluorophore reporter/nonfluorescent quencher MGB) (Applied Biosystems, Thermo Fisher Scientific), and assay references are listed in the following table. The relative amount of transcripts was determined by the 2−(∆∆CT) method, with target expression normalization done with glyceraldehyde-3-phosphate dehydrogenase as housekeeping gene, and normal control NC as the calibrator.
Statistical Analysis
After passing the Shapiro–Wilk normality distribution test, data were analyzed by one-way ANOVA, followed by Sidak post hoc test. The data are represented as column graphs, plotted with mean and standard error. Non-parametric data (IHC analysis) are represented as a box plot graph, plotted with median and minimum and maximum values. Such data were analyzed by non-parametric Kruskal–Wallis test followed by the Dunn’s post hoc test. For all analyses, p < 0.05 was considered statistically significant and multiple comparisons comprised of (a) Control vs. PD, (b) PD vs. Alisk-treated animals with PD, and (c) Normal vs. Diabetic in the same experimental condition. All analysis was carried out in the statistical software Graph Pad Prism v7.0 (GraphPad Software Inc.; San Diego, California, USA).
Results
Renin Is Related to PD-Induced Periodontal Bone Loss and Inflammatory Response in a Diabetic Mouse
Firstly, we assessed the PD-induced bone loss in the first molar furcation region by histomorphometry on HE-stained mandible sections. Groups with PD had significant bone loss compared to Control. As expected diabetic mice presented more severe periodontal destruction compared to normal mice with PD (Figure 1C, D, and G). Treatment with aliskiren (50 mg/kg, Alisk) was able to significantly reduce periodontal bone loss, in both conditions of normal and diabetic with PD, as compared to non-treated mice (Figure 1C vs 1E, and Figure 1D vs 1F).
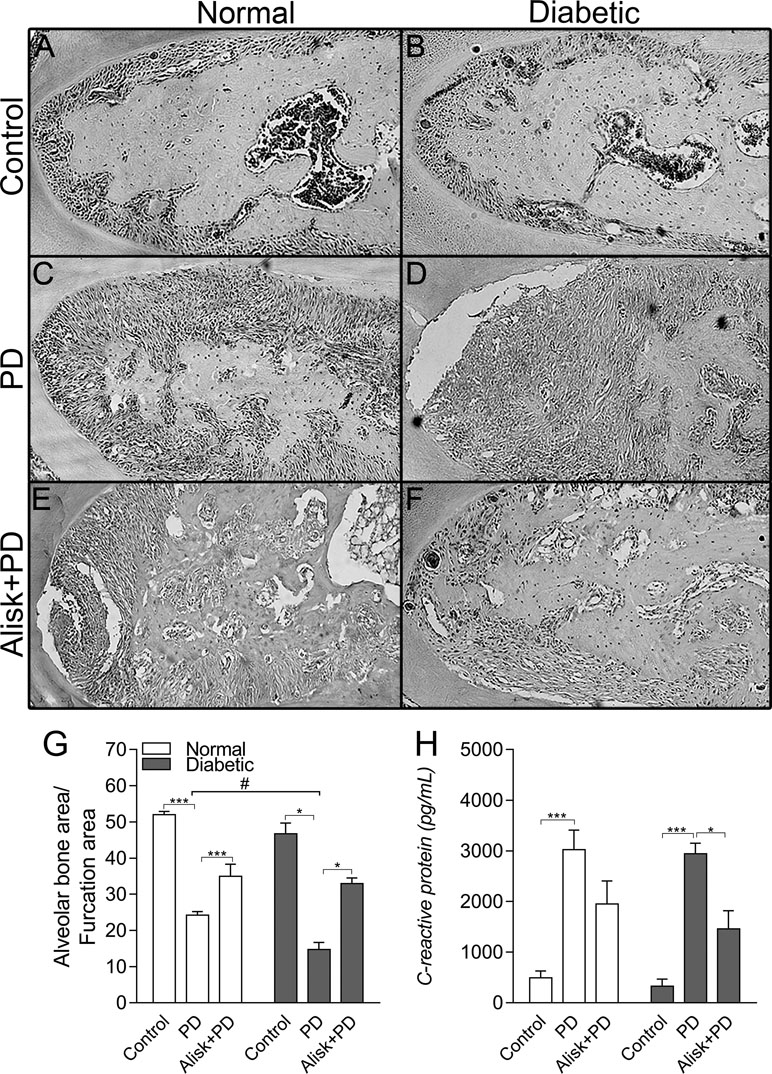
Figure 1 Renin contributes to the periodontal bone loss and inflammation response in normal and diabetic mice 14 days after PD. Histological sections of the lower first molars from the normal mice [control (A), normal with PD (C), normal with PD treated with aliskiren (Alisk) (E)], diabetic mice [control (B), diabetic with PD (D), and diabetic with PD and treated with Alisk (F)]. Percentage of alveolar bone area per μm2 computed using Image J (G) software. C-reactive protein production by gingival fibroblast in normal and diabetic mice 14 days after PD (H). Data are represented as mean ± SEM (n = 10). Significant values are represented as ***p < 0.001 and *p < 0.05 (Control vs. PD; PD vs. Alisk + PD; and PD vs. PD+Alisk), and #p < 0.05 (diabetic + PD vs normal + PD).
Inflammatory response was evaluated by gingival CRP production by ELISA, which showed to be elevated in both normal and diabetic PD groups. Alisk treatment could significantly reduce it only in diabetic animals group (Figure 1H).
Renin Modulates CXCL2 and CCL8 Production Induced by PD on Gingival Tissue in Diabetic Mice
Next, we evaluated IL-1β and chemokine production by ELISA in gingival tissue from normal and diabetic mice with PD for both treated and not treated with Alisk groups. PD caused an increase in CXCL2 and CCL8, in both animals, compared to its respective control. Alisk treatment significantly reduced CCL8 production but not CXCL2 in normal mice, while in diabetic mice Alisk was able to significantly reduce both CXCL2 and CCL8 production (Figure 2A and B). Interleukin-1β production increased after PD induction, but Alisk did not modify the effect in either group of animals (Figure 2C).
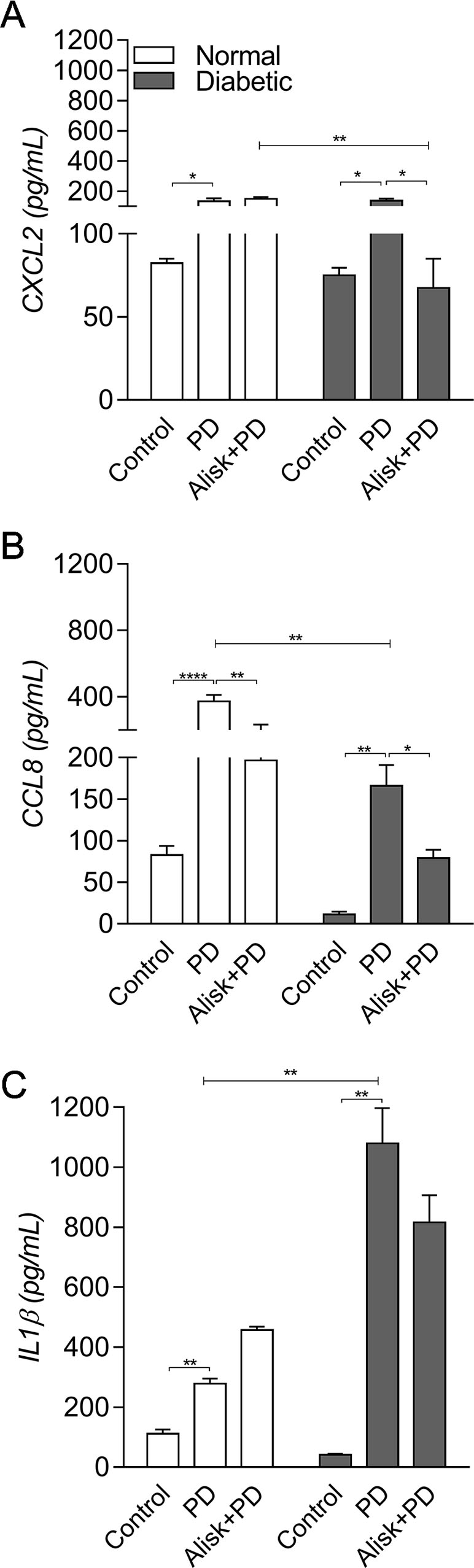
Figure 2 Production of CXCL2, CCL8, and IL-1β by gingival tissue from normal and diabetic mice 14 days after PD. (A) CXCL2, (B) CCL8, and (C) IL1β by ELISA. Data represented as mean ± SEM (n = 10). Statistical differences represented by *p < 0.05, **p < 0.01, and ****p < 0.0001 (Control vs. PD; PD vs. Alisk + PD; and PD vs. PD+Alisk).
PD-Induced RAS Component Expression May be Modulated by Renin
With an aim to investigate whether the RAS components are produced in local gingival tissue and are involved in the inflammatory process in the PD-induced events, we next evaluated the gene expression of Renin, AGT, ACE, AT1R, AT2R, ACE2, and MasR by qRT-PCR in gingival tissue from normal and diabetic mice with PD for both groups in which mice were treated or not treated with Alisk.
First of all, we observed that renin expression was undetectable in the gingival tissue by qRT-PCR (data not shown). PD increased AGT and ACE expression in both normal and diabetic mice, compared to control. Alisk treatment significantly decreased AGT expression in both conditions, while ACE expression was decreased only in diabetic treated animals (Figure 3A and B). Angiotensin II (AngII) receptors, AT1R and AT2R, are expressed in greater amount in diabetic animal, as compared to normal animal. PD did not alter AT1R expression in normal mice but it was significantly increased in diabetic mice. Alisk treatment significantly reduced its expression in normal mice and significantly in diabetic mice. PD increased AT2R expression but more pronouncedly in normal than in diabetic mice, while Alisk treatment decreased this response in both normal and diabetic mice (Figure 3C and D).
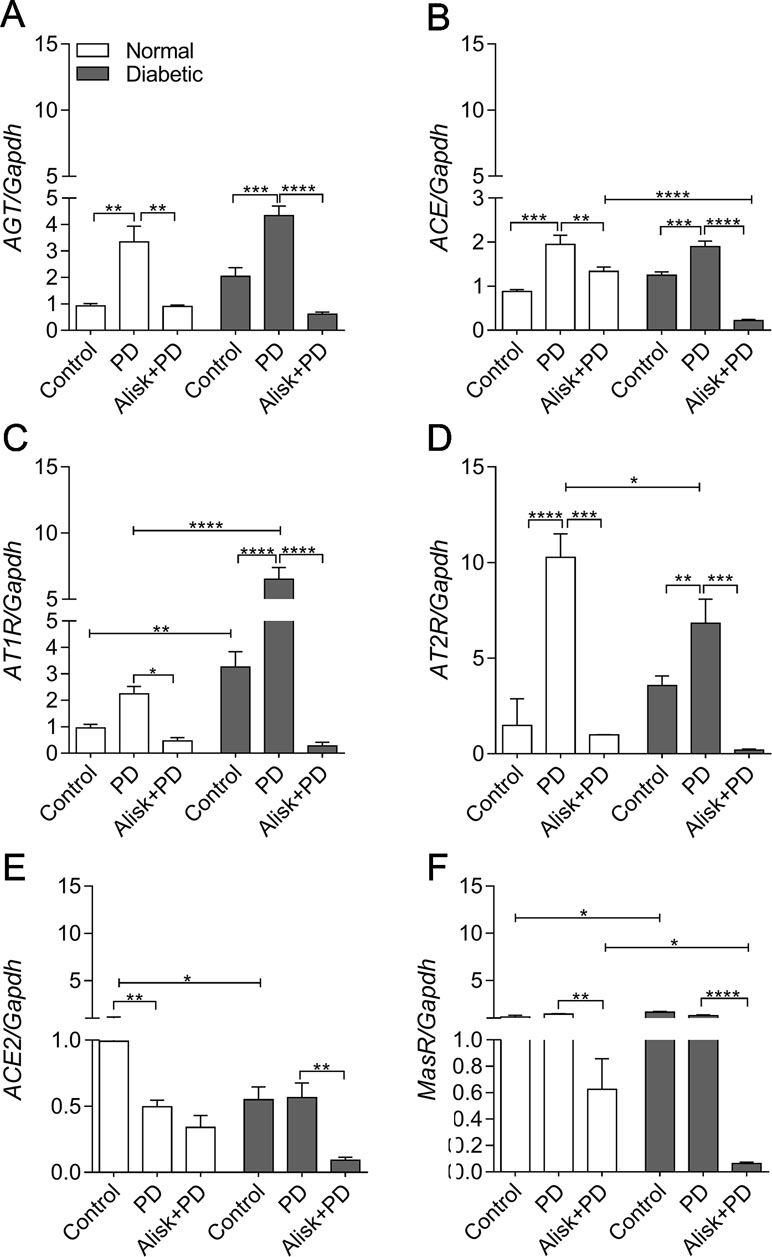
Figure 3 AGT, ACE, AT1R, AT2R, ACE2, and MasR gene expression of gingival tissue from normal and diabetic mice 14 days after PD. (A) AGT, (B) ACE, (C) AT1R, (D) AT2R, (E) ACE2, and (F) MasR in gingival tissue of control, normal mice with PD (PD), normal mice with PD treated with aliskiren (Alisk + PD), control, diabetic mice with PD (PD), diabetic mice with PD treated with aliskiren (Alisk + PD) by real-time RT-PCR. Results express mean ± SEM, normalized by fold change. Significant values are represented by *p < 0.001, **p < 0.05, ***p < 0.005, and ****p < 0.0001 (Control vs. PD, PD vs. Alisk+ PD, PD vs. PD, PD+Alisk vs. PD+Alisk).
Animals in both control and PD groups showed increased expression of ACE2, but it remained unaltered in diabetic group. Alisk significantly reduced its expression only in diabetic mice (Figure 3E). Angiotensin 1–7 receptor, MasR, was also evaluated, and it was observed to have higher expression in control animals. PD was able to reduce the expression only in diabetic mice, and Alisk treatment reduced the expression in both animals, but significantly in diabetic mice (Figure 3F).
Renin Inhibition Reduces the Production of RAS Components in Gingival Tissue
To confirm the gene expression profile, we analyzed the presence and localization of proteins of RAS components by IHC in gingival tissue in the proposed experimental conditions. Furthermore, we used pharmacological agents to block the renin component. Our results demonstrated positive immunolabeling for Renin, AGT, ACE, ACE2, AT1R, AT2R, and MasR, confirming the presence of local RAS in gingival tissue; however, the immunolabeling profile of each target was different.
In normal mice, the IR for AGT was found moderate in the epithelium and basal cell layer of gingival in control animals, but weak in the lamina propria (Figure 4a.1). In PD-induced animals, we observed moderate IR in the gingival epithelium, weak IR in basal cell layer, and negative IR in lamina propria of the gingival (Figure 4a.2). In PD animals treated with Alisk group, the IR was negative in the epithelium and basal cell layer of gingival and weak in the lamina propria (Figure 4a.3). In diabetic animals, the IR for AGT was absent in the control group but was strong in PD animals and moderate in PD animals treated with Alisk (Figure 4a.4, a.5, and a.6). In control group of normal mice, IR for rennin was weak in gingival epithelium, basal cell layer, and lamina propria (Figure 4b.1). Induction of PD and Alisk treatment did not induce any alteration in the IR (Figure 4b.2 and b.3). In diabetic mice, we did not find any immunostaining for renin in any part of the gingival tissue (Figure 4b.4, b.5, and b.6). Weak IR for ACE was found in the epithelium and basal cell layer of the gingival tissue but was negative in the lamina propria in the control group of normal mice (Figure 4c.1). In PD group, the IR for ACE was moderate to strong in the epithelium, basal cell layer, and lamina propria. Alisk did not produce any changes in the IR in gingival tissue when compared to PD group (Figure 4c.2 and c.3). In diabetic mice, the immunostaining in the gingival epithelium of the control group was negative, in the basal cell layer was weak, and in the lamina propria was moderate (Figure 4c.4). In PD group, IR was negative in the gingival epithelium and moderate in the basal cell layer and lamina propria of the gingival tissue (Figure 4c.5). Pre-treatment with Alisk induced a weak IR in the gingival epithelium and was able to inhibit the IR for ACE in the basal cell layer and lamina propria of the gingival tissue (Figure 4c.6). IR for AT1R was found to be weak in the epithelium, basal cell layer, and lamina propria of the gingival tissue in normal mice (Figure 4d.1). In PD group, IR was negative in the epithelium, strong in the basal cell layer, and moderate in the lamina propria (Figure 4d.2). Alisk was able to reverse IR in the epithelium and decrease the IR in the basal cell layer and lamina propria of the gingival tissue (Figure 4d.3). In diabetic mice, AT1R IR was weak in the epithelium and basal cell layer and moderate in the lamina propria of gingival tissue in the control group (Figure 4d.4). In PD group, IR was negative in the epithelium, moderate in the basal cell layer, and decreased in the lamina propria of the gingival tissue (Figure 4d.5). Alisk increased the immunostaining for AT1R in the epithelium, decreased in the basal cell layer, and maintained in the lamina propria (Figure 4d.6). AT2R IR was found to be moderate in the epithelium, basal cell layer, and lamina propria in the control group of normal mice (Figure 4e.1). In PD group, IR was observed to be weak in the epithelium and strong in the basal cell layer and lamina propria of the gingival tissue (Figure 4e.2). Alisk inhibited IR in the epithelium and basal cell layer and maintained in the lamina propria of the gingival tissue (Figure 4e.3). In diabetic mice, IR for AT2R was weak in epithelium, basal cell layer, and lamina propria in the control group in the normal mice (Figure 4e.4). In PD group, IR was increased since it was negative in the epithelium, weak in the basal cell layer, and moderate in the lamina propria of the gingival epithelium (Figure 4e.5). In the presence of Alisk, the IR for PD group did not change in the whole gingival tissue (Figure 4e.6). For ACE2, IR was found negative in the epithelium and moderate in the basal cell layers and lamina propria of the gingival tissue in the normal mice (Figure 4f.1). PD increased IR in the epithelium, basal cell layer, and lamina propria (Figure 4f.2). Pre-treatment of the Alisk did not modify IR in the whole gingival tissue (Figure 4f.3). In diabetic mice, IR was observed to be negative in the epithelium and weak in the basal cell layer and lamina propria of the gingival tissue (Figure 4f.4). Immunostaining for PD group was weak in the epithelium and moderate in the basal cell layer and lamina propria (Figure 4f.5). Alisk treatment was able to inhibit the IR in the whole gingival tissue in these mice (Figure 4f.6). In relation to MasR, IR was not found in the epithelium and was weak in the basal cell layer and lamina propria of the gingival tissue in the control group of normal mice (Figure 4g.1). PD increased the IR in the epithelium but maintained in the basal cell layer and lamina propria (Figure 4g.2). Addition of Alisk inhibited the IR in the epithelium but did not change it in the basal cell layer and lamina propria of the gingival tissue of normal mice (Figure 4g.3). In diabetic mice, IR for MasR was negative in the epithelium and weak in the basal cell layer and lamina propria of the gingival tissue (Figure 4g.4). PD increased the IR in the epithelium but not in the basal cell layer and lamina propria (Figure 4g.5). Alisk did not alter the IR for MasR in whole gingival tissue (Figure 4g.6).
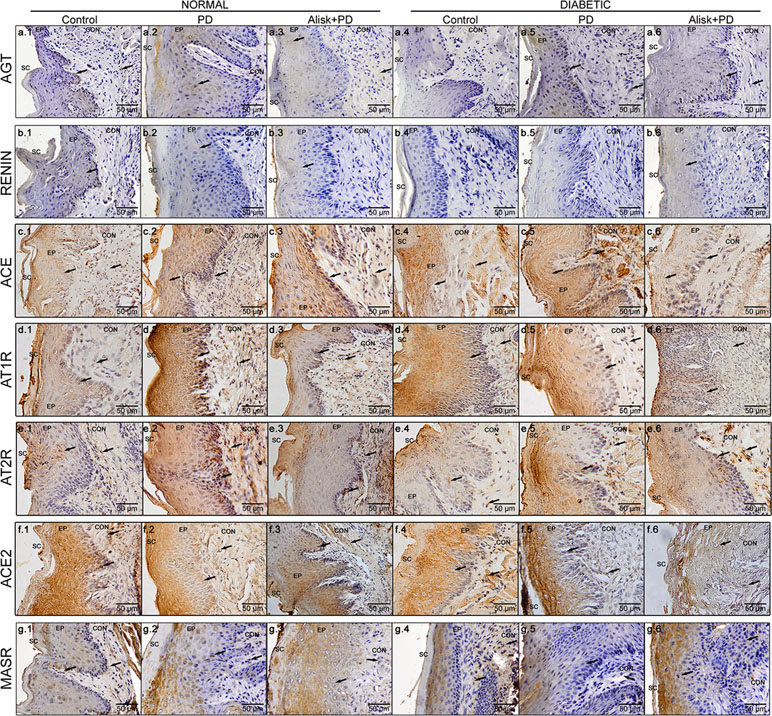
Figure 4 Immunoreactivity for AGT, renin, ACE, AT1R, AT2R, ACE2, and MasR in gingival tissue of normal and diabetic mice 14 days after PD. AGT production of normal mice (a.1 control, a.2 with PD, and a.3 with Alisk + PD) and diabetic mice (a.4 control, a.5 with PD, and a.6 with Alisk + PD); Renin production of normal mice (b.1 control, b.2 with PD, and b.3 with Alisk +PD), and diabetic mice (b.4 control, b.5 with PD, and b.6 with Alisk+PD); ACE production of normal mice (c.1 control, c.2 with PD, and c.3 with Alisk + PD), and diabetic mice (c.4 control, c.5 with PD, and c.6 with Alisk + PD); AT1R production of normal mice (d.1 control, d.2 with PD, and d.3 with Alisk +PD), and diabetic mice (d.4 control, d.5 with PD, and d.6 with Alisk+PD); AT2R production of normal mice (e.1 control, e.2 with PD, and e.3 with Alisk + PD), and diabetic mice (e.4 control, e.5 with PD, and e.6 with Alisk + PD); ACE2 production of normal mice (f.1 control, f.2 with PD, and f.3 with Alisk +PD), and diabetic mice (f.4 control, f.5 with PD, and f.6 with Alisk+PD); MasR production of normal mice (g.1 control, g.2 with PD, and g.3 with Alisk +PD), and diabetic mice (g.4 control, g.5 with PD, and g.6 with Alisk+PD). All images are at 20× magnification, and scale bars indicate a distance of 50 µm. Brow staining indicates positive IR. Abbreviations: sc, superficial cells; ep, epithelium; BL, basal cell layer; LP, lamina propria.
Renin Modulates Col1a1, Col1a2, and Fibronectin mRNA Expression on Gingival Tissue in Diabetic Mice After PD
Aiming to accumulate evidence for modulation of renin in the tissue repair markers, their expression was evaluated in gingival tissue in normal and diabetic mice with PD, with or without treatment with Alisk. PD increased Col1a1, Col1a2, Col3a1, and Fn expression in normal and diabetic mice, when compared to respective control (Figure 5A–E). In normal mice, Alisk treatment reduced the expression of Col1a1 and Fn1 (Figure 5A, D, and E), while in diabetic mice, Alisk reduced Col1a1, Col1a2, and Fn expression (Figure 5B). TGF-β was increased in the control animals, and PD induction caused a decrease in the mRNA expression. Alisk did not produce any effect on its levels (Figure 5E).
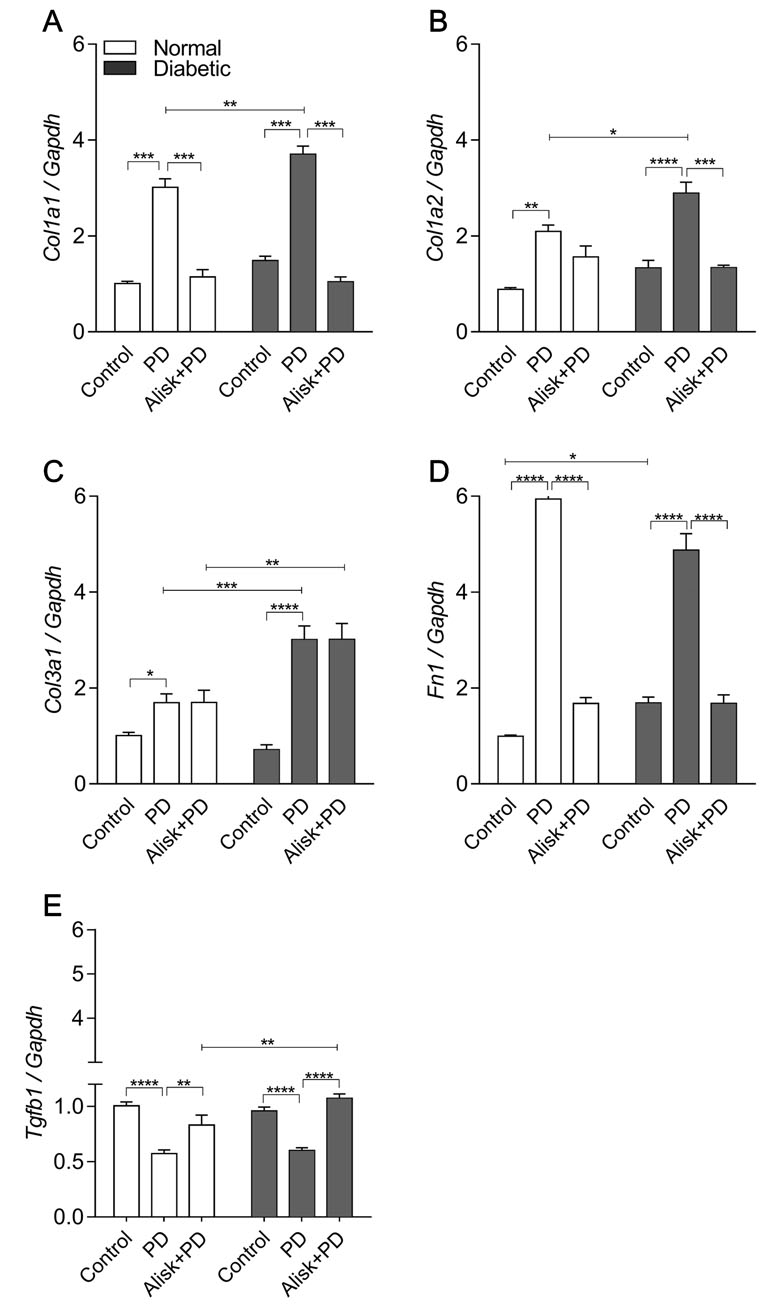
Figure 5 Col1a1, Col1a2, Col3a1, Fn1, and Tgfb1 gene expression of gingival tissue from normal and diabetic mice at 14 days after PD regardless of the fact if they were treated or not with aliskiren. (A) Col1a1, (B) Col1a2, (C) Col3a1, (D) Fn1, and (E) Tgfb1 in gingival tissue of control normal, normal with PD, normal with PD treated with aliskiren, control diabetic, diabetic with PD, and diabetic with PD treated with aliskiren by real-time RT-PCR. Results express mean ± SEM, normalized by fold change. Significant values are represented by p < 0.001, **p < 0.05, ***p < 0.005, and ****p < 0.0001 (Control vs. PD, PD vs. Alisk+PD, PD vs. PD, and PD+Alisk vs. PD+Alisk).
Discussion
The results of our study demonstrated, for the first time, that renin causes exacerbation of inflammatory response and periodontal bone loss, primarily in diabetic mice. Furthermore, we confirmed the existence of components of local RAS in the gingival tissue of mice, as well as their involvement in the regulation of the components of extracellular matrix proteins after PD.
Renin is the rate-limiting enzyme of RAS, which can thereby stimulate the inflammation, vasopressor effects, and oxidative stress and induce fibrosis by cytokine released and chemokines mediating tissue inflammatory process (Campbell, 1987; Paul, 2006). Aliskiren is the first renin inhibitor to reach the market. It lowers elevated blood pressure efficiently by decreasing plasma and/or local renin activity (Jensen et al., 2008). The Alisk in the dose of 50 mg/kg improved bone alveolar loss and decreased inflammatory process. Corroborating with the literature, the dose used in the present study was also observed to decrease blood pressure, and renin–angiotensin II level in mice and attenuated steatosis and inflammation in mice (Lee et al., 2013; Wang et al., 2015; Chen et al., 2017). Furthermore, other study using the same dose of Alisk reduced levels of TNF-α and IL-6 in mice (Patel et al., 2013). IR for renin was found in gingival tissue of control normal mice but not in diabetic mice, but mRNA expression was undetectable. Although speculative, it is possible that circulating renin may be involved in the local process as a compensatory mechanism.
In the present study, renin-induced periodontal bone loss was observed in both animals; however, Alisk was able to reverse it more significantly in diabetic mice. Studies have demonstrated that AngII produced by bone cells is capable of stimulating osteoclastogenesis in MC3T3-E1 cells of calvaria (Hatton et al., 1997) and that the local RAS plays an important role in RANKL/OPG signaling modulating the bone metabolism of hypertensive and osteoporotic patients (Aoki et al., 2015; Shuai et al., 2015). Xue et al. (2016) demonstrated that renin inhibitor Alisk exhibited beneficial effects on trabecular bone of ovariectomy-induced osteoporotic mice. Thus, our results highlight, for the first time, the hypothesis that RAS might be contributing to the periodontal bone loss of diabetic mice after PD. The mechanism involved in this process is in progress for further study.
This study has also demonstrated the role of renin in the leukocyte recruitment during inflammatory process and/or infection. Alisk was able to prevent CRP production, which is an acute inflammatory protein that increases up to 1,000-fold at sites of infection or inflammation. Recently, there is growing evidence that CRP plays an important role in inflammatory processes and host responses to infection including the complement pathway, apoptosis, phagocytosis, nitric oxide release, and the production of cytokines, particularly IL-6 and TNF-α (Sproston and Ashworth, 2018). In our study, we observed elevated CRP production in the gingival tissue after 14 days of PD. Possible reasons for this can be, firstly, animals used in this study had PD suggesting presence of bacteria causing local inflammation. Antigens from bacteria can go into the circulation and activate hepatocytes; thereby, releasing CRP animals had PD, suggesting the presence of bacteria in the local of inflammation and antigens from bacteria going to the circulation and activating hepatocytes releasing CRP. Secondly, leukocytes recruited primarily by macrophages and lymphocytes can also be releasing local CRP (Sproston and Ashworth, 2018). Studies have confirmed CRP to be an important PD-induced gingival inflammation marker, which is already associated with leukocyte infiltrate (Bansal et al., 2014; Kumar et al., 2013), prothrombotic effects (Pasceri et al., 2000), and up regulation of AT1R (Wang et al., 2003). As an inference of our results, we suggest an important role of RAS in the PD-triggered inflammation (Li et al., 2011; Zhao et al., 2013). The inhibition of CRP by Alisk mainly in diabetic animals is possibly due to the capacity of this inhibitor to lead to decrease in the monocytes adhesion, inflammation, and oxidative stress (Pacurari et al., 2014).
A correct balance between neutrophils and monocytes/macrophages is crucial for the inflammation reduction and tissue repair attempts (Chapple et al., 1998; Cekici et al., 2014). It has already been shown that diabetes does not reduce leukocyte recruitment but impairs phagocytic ability of leukocytes and bacterial clearance (Pettersson et al., 2011). Thus, these differences in the chemokine production observed in the present study may represent one of the mechanisms responsible for an increased periodontal destruction in diabetics. The higher production of IL-1β in diabetic mice suggests that this cytokine is an important marker of severity of PD, which in presence of hyperglycemia induces activation of ACEs, causing local immune dysfunction and cytokine imbalance promoting elevation of IL-1β (Polak and Shapira, 2018). Alisk prevented increased production of CXCL2 in diabetics, and CCL8 in both animals. It suggests that, at least, renin is modulating CXCL2 and CCL8 production. Studies have already shown that AngII/Agtr1 axis is involved in neutrophil recruitment in different experimental models through CXC chemokines (Nabah et al., 2004; Shimada et al., 2011). Few studies relate RAS to CCL8, but an in vitro study has shown that AngII can stimulate CCL8 expression in human macrophage (Tone et al., 2007). In vitro studies have already shown that IL-1β attenuates renin expression via the p42/44 MAPK–STAT3 pathway (Liu et al., 2006). Our results show negative IR for renin production and expression in the gingival after 14 days of PD, which corroborates with previous results. Furthermore, elevated AngII alters systemic and local levels of pro- and anti-inflammatory cytokines such as IL-1β, IL-6, and TNF-α (Zhang et al., 2009). Conclusively, our data corroborates to literature, evidencing an important role of RAS in the cytokine/chemokine production, mainly in diabetic model. This helps in explaining the increased PD consequences and pronounces protective effects of Alisk on CXCL2 and CCL8. However, this has no effect on IL-β production possibly acting via a different regulatory mechanism; that is, it is not dependent on renin expression and/or production. Furthermore, IL-1β as an important pro-inflammatory cytokine is able to coordinate the fibroblast activation to produce other cytokines and/or chemokines as CXCL2 or CCL8 promoting a cascade mechanism (O’Hara et al., 2012; Gao et al., 2014; Alomar et al., 2015).
Aiming to confirm a possible correlation between RAS and PD in the gingival tissue, we further investigated the gene expression and protein production of RAS components in gingival tissue. Both qPCR and IHC experimental analyses confirmed the presence of RAS components in the normal and diabetic mice gingival tissue after 14 days of PD with different characteristics depending on the target evaluated.
Hepatic AGT is the major source of circulating AGT protein. However, the gingival tissue also produces local AGT as observed in our study. Regulation of AGT expression by cytokines has been shown in multiple organs and isolated immune cells (Brasier et al., 1990; Corvol et al., 1997; O’Leary et al., 2016). If this result can be applied to this study, then it seems reasonable to assume that CXCL2, CCL8, or IL-1β could be stimulating AGT production from gingival tissue after PD in both normal and diabetic mice. Therefore, PD-induced AGT production suggests that there is an increase in all angiotensin peptides, including AngII, which also plays a pro-inflammatory action. However, this needs further investigation. In the current study, Alisk significantly decreased AGT gene expression and protein production in both normal and diabetic mice. This is probably caused by reducing levels of renin and consequently circulating AngII, which, besides being a cytokine, regulates AGT production as has been already shown in a previous study (Kobori et al., 2001). Recent studies have shown that Alisk is effective in decreasing the progression of diabetes in db/db mice reducing proteinuria and renal inflammation and oxidative stress (Zhou et al., 2015).
In the present study, we found, for the first time, ACE expression and protein production in gingival tissue in both normal and diabetic mice after PD. The presence of ACE was also observed in human gingival fibroblasts, human periodontal ligament fibroblasts, and gingival tissue of rats with PD in serum, lung, heart, and liver of mice (Santos et al., 2015; Roca-Ho et al., 2017). Alisk was more effective in reducing ACE levels in diabetic mice to levels even below than what are seen in control group. This is possible as Alisk might be blocking the generation of AngI from AGT by causing renin inhibition, thereby reducing all angiotensin peptides. This might be promoting a down regulation of ACE due to feedback mechanism, as shown by previous other studies (Schunkert et al., 1992; Sadjadi et al., 2005). Information regarding regulation of ACE by inflammatory mediators is less; however, in an adjuvant induced arthritis model, the expression of ACE was found to be decreased in heart (Hanafy et al., 2011). For this reason, it is possible to speculate that the inflammatory mediators produced during PD in our model can be modulating ACE production.
The majority of ACE-derived angiotensin II actions mainly depend on their binding to their AT1R receptor, although there is also some affinity for the AT2R receptor. In the current study, for the first time, we found AT1R and AT2R expression and protein production in gingival tissue of normal and diabetic mice after PD. It has already been seen in normal rats with PD by Santos et al., 2009, 2015. AT1R and AT2R show similar properties of AngII binding but different genomic structure, localization, tissue-specific expression, and regulation (de Gasparo et al., 2000). Although it is speculative, the higher AT1R and AT2R expression and protein production seen in the gingival tissue of normal and diabetic mice after PD can be happening because of increased cytokine and chemokine release by gingival fibroblast, thereby increasing AngII production by acting on their specific receptors (Forrester et al., 2018; Sato et al., 2018). Furthermore, the exacerbated consequences of PD in diabetic animals, which present higher AT1R response, can be related to bone loss and inflammation promotion, combined to a higher AT2R response, which shows protective effects in inflammation and tissue injury (Benndorf et al., 2009; Abadir et al., 2011; Dhande et al., 2015). This suggests an altered endogenous mechanism in the diabetic condition. Renin also regulates these AngII receptors, as Alisk was able to significantly reduce AT1R and AT2R in both normal and diabetic mice. Studies with clinical screening have shown that the use of inhibitors of RAS components reduces the incidence of cardiovascular complications and inflammation in diabetic patients (Lewis et al., 2001). Taken together, these data suggest that local and/or systemic renin can be involved in the expression and production of AT1R and AT2R. Alisk presented a protective effect in the gingival tissue, possibly by reducing AngII levels and, consequently, the inflammatory process that is responsible to trigger AT1R activation and hence altering the local RAS in periodontitis.
In the current study, we demonstrated for the first time mRNA expression and protein production of ACE2 and MasR in gingival tissue of normal and diabetic mice after 14 days of PD. ACE2 is able to convert AngII into Ang1–7, which acts through MasR. The activation of this receptor, in turn, shows opposite effect to AngII/AT1R axis, comprising an important endogenous mechanism that eliminates AngII produced and counteracts its effects (Rodrigues Prestes et al., 2017). Our results showed high expression of ACE2 in both animals. However, in diabetic mice, this expression was lower as compared to normal mice. These results are in agreement with previous studies that have also observed that ACE2 was reduced in streptozotocin-induced diabetic animals regulating the tissue and plasma level of AngII, preventing development of cardiovascular disease (Tikellis et al., 2008; Tikellis et al., 2012). PD decreased ACE2 expression in normal mice, but not in diabetic mice. This suggests that, although speculative, cytokines released during the local gingival inflammatory process were able to inhibit the production of ACE2 primarily in normal mice, as ACE2 can be reduced in diabetic mice. Studies showed that the activation of the ACE2/Ang-(1–7)/MasR axis can modulate the expression of pro-inflammatory cytokines in a model of pulmonary hypertension. Indeed, there was decreased expression of TNF-α, IL-1β, IL-6, MCP-1, and TGF-β and increased expression of the anti-inflammatory cytokine IL-10 (Ferreira et al., 2009; Yamazato et al., 2009; Shenoy et al., 2010). In the present study, the inflammatory response provoked by PD in diabetic mice was more pronounced when compared to normal mice. For this reason, it is possible that some imbalance occurs in the ACE2/Ang1–7 axis promoting a poor prognosis in diabetic mice with PD. Alisk decreased the ACE2 mRNA expression and protein production in diabetic mice, but not in normal mice, suggesting that renin production can also be involved in this modulation. Studies have demonstrated that the pathogenesis of diabetes is mediated by an upregulation of ACE and a downregulation of ACE2, suggesting a compensatory mechanism (Mizuiri et al., 2008).
In the current study, although speculative, it is possible that AT2R can be modulating the MasR, since, when AT2R expression is decreased, MasR expression is increased in control group. In the presence of PD, AT2R has a huge enhancement in expression possibly because MasR remained equal to control group. Previous studies conducted in the rat stroke model point out presence of AngII/AT2R signaling in the brain, which enhances Ang-(1–7)/MasR, antagonizing repressor response after stroke (Chang and Wei, 2015). Additionally, Patel et al. (2017), using obese rat kidney, observed that AT2R and MasR can colocalize and are functionally interdependent in terms of stimulation by nitric oxide (NO). In the present study, we observed that renin is involved in the modulation of MasR, more effectively in diabetic mice. Studies showed that the novel renin inhibitor Alisk antagonizes the stroke-induced repressor response by reducing AngII, Ang-(1–7), and AngIV levels (Chang and Wei, 2015). Corroborating with the present study, our data showed that AT1R, AT2R, ACE2, and MasR were decreased after Alisk treatment, suggesting the reduction in their response.
The RAS operates predominantly through AngII, which is a major regulator of fibroblast homeostasis (Brilla, 2000). Type 1 collagen and type 3 collagen have already been related to PD progression and other inflammatory conditions (Lorencini et al., 2009). Since gingival tissue has lots of fibroblast producing ECM, it is of utmost importance to our study, whereas after PD, the collagen production was increased. Studies have already reported increased collagen expression in PD model as a compensatory mechanism and repair effort due to increased matrix degradation (Larjava et al., 1989; Larjava et al., 1990). AngII has already been shown to stimulate collagen synthesis in cardiac fibroblasts (Lijnen et al., 2001) and diabetic skin fibroblasts (Ren et al., 2013). Besides that, AngII leads to dose-dependent collagen production within the myocardium and has emerged as a key mediator of myocardial fibrosis (Brilla et al., 1994). However, studies have demonstrated that AT1R inhibitor, but not AT2R inhibitor, was able to decrease Col 1, Col 3, and TGF-β in diabetic skin fibroblasts, suggesting that this effect is mediated through AT1R stimulation independent of AT2R (Ren et al., 2013). In this study, Alisk reduced Col1a1 expression, in both animals, and Col1a2 expression only in diabetic mice, suggesting that both are regulated by renin. On the other hand, Col3a1 expression was not altered, suggesting that it is not regulated by renin mechanism. Fn1 expression, also an extracellular matrix glycoprotein related to tissue repair and cell adhesion/migration (Ren et al., 2013), was found to be significantly increased after PD in normal and diabetic mice, suggesting that its production is mediated by renin release. Recent studies have already shown that AngII stimulates Fn synthesis in proximal tubular (Alexander et al., 2014) and collecting duct cells (Cuevas et al., 2015) by different pathway. An important point is that wound healing process occurs in three phases: inflammation, proliferation, and remodeling. However, some abnormality and imbalance in the production and destruction of collagens can lead to formation of hypertrophic scars and keloids (Mari et al., 2015). In one study, patients with hypertrophic scars and keloids were treated with AngII receptor antagonist and ACE inhibitors and they were effective to inhibit them (Niazi et al., 2018). For these reasons, it is possible to speculate that Alisk can be used as a pharmacological tool to inhibit the excess of Col1 and Fn1 production during the PD. Further investigation needs to be conducted to warrant and support this.
In the present study, PD significantly reduced TGF-β1 expression in both animal groups. Previous studies have demonstrated that although TGF-β1 levels are elevated in moderate disease, progression of PD can decrease this response in fluid samples obtained from the periodontal pockets (Skalerič et al., 1997). Then, this can explain the reduction of TGF-β in gingival tissue of animals after 14 days. Thus, ambiguous role of TGF-β1 in periodontal wound healing remains unclear. Studies have shown that concentration of TGF-β1 in the gingival tissue exhibits dynamic changes associated with the progression of experimental periodontal inflammation (Skalerič et al., 1997; Ko et al., 1999; Shah and Hux, 2003; Muller et al., 2005; Gürkan et al., 2006; Ribeiro-Oliveira et al., 2008). The levels of TGF-β1 in gingival tissue may be valuable to detect the inflammatory reaction in the periodontal tissue. In addition, Alisk was able to reverse this response, increasing these levels mainly in diabetic mice. These results suggest that TGF-β1 expression is dependent on renin and possibly on its derivatives such as AngII-dependent mechanisms, being able to participate in this response. Further studies are necessary to elucidate the relationship between RAS and TGF-β production in PD progression.
In summary, the results suggest that local RAS in gingival tissue not only does exist but also is functional in normal and diabetic mice in periodontal tissue, modulating the inflammatory and wound healing process. Furthermore, inhibiting the renin enzymatic activity can improve periodontal bone loss and inflammatory response and exacerbate wound healing process, mainly in diabetes. Renin inhibitor can be a possible tool to prevent bone and tissue implication during the PD during the diabetes.
Data Availability Statement
The raw data supporting the conclusions of this manuscript will be made available by the authors, without undue reservation, to any qualified researcher.
Ethics Statement
We certify that the study entitled “Renin–angiotensin system and periodontal bone metabolism: involvement of microRNAs and mast cells in inflammatory response in animal models of hypertension and diabetes,” Protocol FOA no. 00974-2016, under the supervision of Sandra Helena Penha de Oliveira presents an experimental protocol in accordance with the Ethical Principles of Animal Experimentation and its implementation was approved by CEUA on April 19, 2017.
Author Contributions
SO conceived and designed the experiments. VB, SF, BR, MF, DQ, and CB performed the experiments. SO, VB, SF, and DQ analyzed the data. SO, CS, and VL contributed reagents/materials/analysis tools. SO, VB, and DQ wrote the paper.
Funding
This work was supported by two types of research grants as follows: The São Paulo Research Foundation [FAPESP-2015/03965-2 (SHPO, CFS, VSL), 2018/04989-0 (MNF), 2018/04476-3 (BSR), 2017/05873-3 (SCTF)] and the Coordination for the Improvement of Higher Education Personnel (CAPES) (VGBB, DPQ, CTB).
Conflict of Interest Statement
The authors declare that the research was conducted in the absence of any commercial or financial relationships that could be construed as a potential conflict of interest.
Acknowledgments
The authors express their gratitude to Professor Dr. Edilson Ervolino for his assistance with histological analysis with mice gingival tissue and his help with the histological technique.
References
Abadir, P. M., Walston, J. D., Carey, R. M., Siragy, H. M. (2011). Angiotensin II Type 2 receptors modulate inflammation through signal transducer and activator of transcription proteins 3 phosphorylation and TNFα production. J. Interf. Cytokine Res. 31 (6), 471–474. doi: 10.1089/jir.2010.0043
Aldahlawi, S., and Youssef AR, S. S. (2018). Evaluation of chemokine CXCL10 in human gingival crevicular fluid, saliva, and serum as periodontitis biomarker. J. Inflamm. Res. 31, 389–396. doi: 10.2147/JIR.S177188
Alexander, L. D., Ding, Y., Alagarsamy, S., Cui, X. (2014). Angiotensin II stimulates fibronectin protein synthesis via a Gβγ/arachidonic acid-dependent pathway. Am. J. Physiol. Ren. Physiol. 307 (3), F287–302. doi: 10.1152/ajprenal.00094.2014
Alomar, S. Y., Zaibi, M. S., Kępczyńska, M. A., Gentili, A., Alkhuriji, A., Mansour, L., et al. (2015). PCR array and protein array studies demonstrate that IL-1 β (interleukin-1 β) stimulates the expression and secretion of multiple cytokines and chemokines in human adipocytes. Arch. Physiol. Biochem. 121, 187–193. doi: 10.3109/13813455.2015.1087034
Amemiya, S., Ishihara, T., Higashida, K., Kusano, S., Ohyama, K., Kato, K. (1990). Altered synthesis of renin in patients with insulin-dependent diabetes: plasma prorenin as a marker predicting the evolution of nephropathy. Diabetes Res. Clin. Pract. 10 (2), 115–122. doi: 10.1016/0168-8227(90)90032-O
Aoki, M., Kawahata, H., Sotobayashi, D., Yu, H., Moriguchi, A., Nakagami, H., et al. (2015). Effect of angiotensinII receptor blocker, olmesartan, on turnover of bone metabolism in bedridden elderly hypertensive women with disuse syndrome. Geriatr. Gerontol. Int. 15 (8), 1064–1072. doi: 10.1111/ggi.12406
Araújo, A. A., Souza, T. O., Moura, L. M., Brito, G. A. C., Aragão, K. S., Araújo, L. S., et al. (2013). Effect of telmisartan on levels of IL-1, TNF-α, down-regulated COX-2, MMP-2, MMP-9 and RANKL/RANK in an experimental periodontitis model. J. Clin. Periodontol. 40 (12), 1104–1111. doi: 10.1111/jcpe.12160
Asano, K., Takahashi, N., Ushiki, M., Monya, M., Aihara, F., Kuboki, E., et al. (2015). Intestinal CD169 + macrophages initiate mucosal inflammation by secreting CCL8 that recruits inflammatory monocytes. Nat. Commun. 6, 7802. doi: 10.1038/ncomms8802
Bansal, T., Pandey, A., Deepa, D., Asthana, A. K. (2014). C-reactive protein (CRP) and its association with periodontal disease: a brief review. J. Clin. Diagnostic Res. 8 (7), ZE21–24. doi: 10.7860/JCDR/2014/8355.4646
Bascones-Martinez, A., Matesanz-Perez, P., Escribano-Bermejo, M., González-Moles, M., Bascones-Ilundain, J., Meurman, J. H. (2011). Periodontal disease and diabetes-Review of the literature. Med. Oral Patol. Oral Cir. Bucal. 16 (6), e722–729. doi: 10.4317/medoral.17032
Benndorf, R. A., Krebs, C., Hirsch-Hoffmann, B., Schwedhelm, E., Cieslar, G., Schmidt-Haupt, R., et al. (2009). Angiotensin II type 2 receptor deficiency aggravates renal injury and reduces survival in chronic kidney disease in mice. Kidney Int. 75 (10), 1039–1049. doi: 10.1038/ki.2009.2
Berggreen, E., Heyeraas, K. J. (2003). Role of K+ATPchannels, endothelin A receptors, and effect of angiotensin II on blood flow in oral tissues. J. Dent. Res. 82 (1), 33–37. doi: 10.1177/154405910308200108
Bonato, C. F., Amaral, C. C. F., Belini, L., Salzedas, L. M. P., Oliveira, S. H. P. (2012). Hypertension favors the inflammatory process in rats with experimentally induced periodontitis. J. Periodontal Res. 47 (6), 783–792. doi: 10.1111/j.1600-0765.2012.01496.x
Brasier, A. R., Ron, D., Tate, J. E., Habener, J. F. (1990). A family of constitutive C/EBP-like DNA binding proteins attenuate the IL-1 alpha induced, NF kappa B mediated trans-activation of the angiotensinogen gene acute-phase response element. EMBO J. 9 (12), 3933–3944. doi: 10.1002/j.1460-2075.1990.tb07614.x
Brilla, C. G. (2000). Renin-angiotensin-aldosterone system and myocardial fibrosis: editorial. Cardiovasc. Res. 47 (1), 1–3. doi: 10.1016/S0008-6363(00)00092-4
Brilla, C. G., Zhou, G., Matsubara, L., Weber, K. T. (1994). Collagen metabolism in cultured adult rat cardiac fibroblasts: response to angiotensin II and aldosterone. J. Mol. Cell. Cardiol. 26 (7), 809–820. doi: 10.1006/jmcc.1994.1098
Campbell, D. J. (1987). Circulating and tissue angiotensin systems. J. Clin. Invest. 79 (1), 1–6. doi: 10.1172/JCI112768
Cekici, A., Kantarci, A., Hasturk, H., Van Dyke, T. E. (2014). Inflammatory and immune pathways in the pathogenesis of periodontal disease. Periodontol. 2000. 64 (1), 57–80. doi: 10.1111/prd.12002
Chang, Y., Wei, W. (2015). Angiotensin II in inflammation, immunity and rheumatoid arthritis. Clin. Exp. Immunol. 179 (2), 137–145. doi: 10.1111/cei.12467
Chapple, C. C., Srivastava, M., Hunter, V. (1998). Failure of macrophage activation in destructive periodontal disease. J. Pathol. 186 (3), 281–286. doi: 10.1002/(SICI)1096-9896(1998110)186:3<281::AID-PATH200>3.0.CO;2-7
Chávarry, N. G. M., Vettore, M. V., Sansone, C., Sheiham, A. (2009). The relationship between diabetes mellitus and destructive periodontal disease: a meta-analysis. Oral Health Prev. Dent. 7 (2), 107–127. doi: 10.3290/j.ohpd.a15518
Chen, Y., Meng, G., Bai, W., Ma, Y., Xie, L., Altaf, N., et al. (2017). Aliskiren protects against myocardial ischaemia-reperfusion injury via an endothelial nitric oxide synthase dependent manner. Clin. Exp. Pharmacol. Physiol. 44, 266–274. doi: 10.1111/1440-1681.12692
Corvol, P., Soubrier, F., Jeunemaitre, X. (1997). Molecular genetics of the renin-angiotensin-aldosterone system in human hypertension. Pathol Biol. 45 (3), 229–239. doi: 10.1007/s10569-014-9554-7
Cuevas, C. A., Gonzalez, A. A., Inestrosa, N. C., Vio, C. P., Prieto, M. C. (2015). Angiotensin II increases fibronectin and collagen I through the β-catenin-dependent signaling in mouse collecting duct cells. Am. J. Physiol. Physiol. 308 (4), F358–365. doi: 10.1152/ajprenal.00429.2014
de Gasparo, M., Catt, K. J., Inagami, T., Wright, J. W., Unger, T. (2000). International Union of Pharmacology. XXIII. The angiotensin II receptors. Pharmacol. Rev. 52 (3), 415–472.
Dhande, I., Ma, W., Hussain, T. (2015). Angiotensin AT2receptor stimulation is anti-inflammatory in lipopolysaccharide-activated THP-1 macrophages via increased interleukin-10 production. Hypertens. Res. 38 (1), 21–29. doi: 10.1038/hr.2014.132
Drachman, R. H., Root, R. K., Wood, W. B. (1966). Studies on the effect of experimental nonketotic diabetes mellitus on antibacterial defense: I. Diabetes Antibact. Def. 124 (2), 227–240. doi: 10.1084/jem.124.2.227
Ferreira, A. J., Shenoy, V., Yamazato, Y., Sriramula, S., Francis, J., Yuan, L., et al. (2009). Evidence for angiotensin-converting enzyme 2 as a therapeutic target for the prevention of pulmonary hypertension. Am. J. Respir. Crit. Care Med. 179 (11), 1048–1054. doi: 10.1164/rccm.200811-1678OC
Forrester, S. J., Booz, G. W., Sigmund, C. D., Coffman, T. M., Kawai, T., Rizzo, V., et al. (2018). Angiotensin II signal transduction: an update on mechanisms of physiology and pathophysiology. Physiol. Rev. 98 (3), 1627–1738. doi: 10.1152/physrev.00038.2017
Gao, D., Madi, M., Ding, C., Fok, M., Steele, T., Ford, C., et al. (2014). Interleukin-1β mediates macrophage-induced impairment of insulin signaling in human primary adipocytes. Am. J. Physiol. Metab. 307, E289–E304. doi: 10.1152/ajpendo.00430.2013
Geerlings, S. E., Hoepelman, A. I. M. (1999). Immune dysfunction in patients with diabetes mellitus (DM). FEMS Immunol. Med. Microbiol. 26 (3–4), 259–265. doi: 10.1016/S0928-8244(99)00142-X
Gemmell, E., Carter, C. L., Seymour, G. J. (2001). Chemokines in human periodontal disease tissues. Clin. Exp. Immunol. 125 (1), 134–141. doi: 10.1046/j.1365-2249.2001.01511.x
Gonçalves-Zillo, T. O., Pugliese, L. S., Sales, V. M. T., Da Silva Mori, M. A., Squaiella-Baptistão, C. C., Longo-Maugéri, I. M., et al. (2013). Increased bone loss and amount of osteoclasts in kinin B1 receptor knockout mice. J. Clin. Periodontol. 40 (7), 653–660. doi: 10.1111/jcpe.12097
Greer, A., Irie, K., Hashim, A., Leroux, B. G., Chang, A. M., Curtis, M. A., et al. (2016). Site-specific neutrophil migration and CXCL2 expression in periodontal tissue. J. Dent. Res. 95 (8), 946–952. doi: 10.1177/0022034516641036
Gürkan, A., Emingil, G., Çinarcik, S., Berdeli, A. (2006). Gingival crevicular fluid transforming growth factor-β1 in several forms of periodontal disease. Arch. Oral Biol. 51 (10), 906–912. doi: 10.1016/j.archoralbio.2006.04.008
Hanafy, S., Tavasoli, M., Jamali, F. (2011). Inflammation alters angiotensin converting enzymes (ACE and ACE-2) balance in rat heart. Inflammation 34 (6), 609–613. doi: 10.1007/s10753-010-9269-1
Hatton, R., Stimpel, M., Chambers, T. J. (1997). Angiotensin II is generated from angiotensin I by bone cells and stimulates osteoclastic bone resorption in vitro. J. Endocrinol. 152 (1), 5–10. doi: 10.1677/joe.0.1520005
Itinteang, T., Marsh, R., Davis, P. F., Tan, S. T. (2015). Angiotensin II causes cellular proliferation in infantile haemangioma via angiotensin II receptor. J. Clin. Pathol. 68 (5), 346–350. doi: 10.1136/jclinpath-2014-202794
Jensen, C., Herold, P., Brunner, H. R. (2008). Aliskiren: the first renin inhibitor for clinical treatment. Nat. Rev. Drug Discov. 7 (5), 399–410. doi: 10.1038/nrd2550
Khader, Y. S., Dauod, A. S., El-Qaderi, S. S., Alkafajei, A., Batayha, W. Q. (2006). Periodontal status of diabetics compared with nondiabetics: a meta-analysis. J. Diabetes Complications 20 (1), 59–68. doi: 10.1016/j.jdiacomp.2005.05.006
Kim, J. J., Choi, J., Lee, M. K., Kang, K. Y., Paik, M. J., Jo, S. K., et al. (2014). Immunomodulatory and antidiabetic effects of a new herbal preparation (HemoHIM) on streptozotocin-induced diabetic mice. Evidence-based Complement. Altern. Med. 2014, 461685. doi: 10.1155/2014/461685
Ko, W. L., Wang, J. C., Chen, C. C., Wu, Y. M., Tsai, C. C. (1999). TGF-beta 1 in the experimentally induced inflammatory periodontal tissues in miniature swines. Kaohsiung J Med Sci 15, 315–321.
Kobori, H., Harrison-Bernard, L. M., Navar, L. G. (2001). Enhancement of angiotensinogen expression in angiotensin II-dependent hypertension. Hypertension 37 (5), 1329–1335. doi: 10.1161/01.HYP.37.5.1329
Kumar, S., Shah, S., Budhiraja, S., Desai, K., Shah, C. M. D. (2013). The effect of periodontal treatment on C-reactive protein: a clinical study. J Nat Sci Biol Med. 4, 379–382. doi: 10.4103/0976-9668.116991
Lamster, I. B. (1997). Evaluation of components of gingival crevicular fluid as diagnostic tests. Ann. Periodontol. 2 (1), 123–137. doi: 10.1902/annals.1997.2.1.123
Larjava, H., Sandberg, M., Happonen, R. P., Vuorio, E. (1990). Differential localization of type I and type III procollagen messenger ribonucleic acids in inflamed periodontal and periapical connective tissues by in situ hybridization. Lab Invest. 62 (1), 96–103.
Larjava, H., Sandberg, M., Vuorio, E. (1989). Altered distribution of type I collagen mRNA in periodontal disease. J. Periodontal Res. 24 (3), 171–177. doi: 10.1111/j.1600-0765.1989.tb02002.x
Lee, K.-C., Chan, C.-C., Yang, Y.-Y., Hsieh, Y.-C., Huang, Y.-H., Lin, H.-C. (2013). Aliskiren attenuates steatohepatitis and increases turnover of hepatic fat in mice fed with a methionine and choline deficient diet. PLoS One 8, e77817. doi: 10.1371/journal.pone.0077817
Lewis, E. J., Hunsicker, L. G., Clarke, W. R., Berl, T., Pohl, M. A., Lewis, J. B., et al. (2001). Renoprotective effect of the angiotensin-receptor antagonist irbesartan in patients with nephropathy due to type 2 diabetes. N. Engl. J. Med. 345 (12), 851–860. doi: 10.1056/NEJMoa011303
Li, J., Xiao, X., Wei, W., Ding, H., Yue, Y., Tian, Y., et al. (2018). Inhibition of angiotensin II receptor I prevents inflammation and bone loss in periodontitis. J. Periodontol. 90 (2), 208–216. doi: 10.1002/JPER.17-0753
Li, M., Liu, J., Han, C., Wang, B., Pang, X. M. J. (2011). Angiotensin II induces the expression of c-reactive protein via MAPK-dependent signal pathway in U937 macrophages. Cell Physiol Biochem 27, 63–70. doi: 10.1159/000325206
Lijnen, P. J., Petrov, V. V., Fagard, R. H. (2001). Angiotensin II-induced stimulation of collagen secretion and production in cardiac fibroblasts is mediated via angiotensin II subtype 1 receptors. JRAAS - J. Renin-Angiotensin-Aldosterone Syst. 2 (2), 117–122. doi: 10.3317/jraas.2001.012
Liu, X., Shi, Q., Sigmund, C. D. (2006). Interleukin-1β attenuates renin gene expression via a mitogen-activated protein kinase kinase-extracellular signal-regulated kinase and signal transducer and activator of transcription 3-dependent mechanism in As4.1 cells. Endocrinology 147 (12), 6011–6018. doi: 10.1210/en.2006-0129
Loe, H. (1993). Periodontal disease: the sixth complication of diabetes mellitus. Diabetes Care. 16 (1), 329–334. doi: 10.2337/diacare.16.1.329
Lorencini, M., Silva, J. A. F., Almeida, C. A., Bruni-Cardoso, A., Carvalho, H. F., Stach-Machado, D. R. (2009). A new paradigm in the periodontal disease progression: gingival connective tissue remodeling with simultaneous collagen degradation and fibers thickening. Tissue Cell 41 (1), 43–50. doi: 10.1016/j.tice.2008.07.001
Marhoffer, W., Stein, M., Maeser, E., Federlin, K. (1992). Impairment of polymorphonuclear leukocyte function and metabolic control of diabetes. Diabetes Care 15 (2), 256–260. doi: 10.2337/diacare.15.2.256
Mari, W., Alsabri, S. G., Tabal, N., Younes, S., Sherif, A., Simman, R. (2015). Novel insights on understanding of keloid scar: article review. J. Am. Coll. Clin. Wound Spec. 7 (1–3), 1–7. doi: 10.1016/j.jccw.2016.10.001
Mizuiri, S., Hemmi, H., Arita, M., Ohashi, Y., Tanaka, Y., Miyagi, M., et al. (2008). Expression of ACE and ACE2 in individuals with diabetic kidney disease and healthy controls. Am. J. Kidney Dis. 51 (4), 613–623. doi: 10.1053/j.ajkd.2007.11.022
Muller, L. M. A. J., Gorter, K. J., Hak, E., Goudzwaard, W. L., Schellevis, F. G., Hoepelman, A. I. M., et al. (2005). Increased risk of common infections in patients with type 1 and type 2 diabetes mellitus. Clin. Infect. Dis. 41 (3), 281–288. doi: 10.1086/431587
Mumghamba, E. G. S., Markkanen, H. A., Honkala, E. (1995). Risk factors for periodontal diseases in Ilala, Tanzania. J. Clin. Periodontol. 22 (5), 347–354. doi: 10.1111/j.1600-051X.1995.tb00160.x
Nabah, Y. N. A., Mateo, T., Estellés, R., Mata, M., Zagorski, J., Sarau, H., et al. (2004). Angiotensin II induces neutrophil accumulation in vivo through generation and release of CXC chemokines. Circulation 110 (23), 3581–3586. doi: 10.1161/01.CIR.0000148824.93600.F3
Niazi, F., Hooshyar, S. H., Hedayatyanfard, K., Ziai, S. A., Doroodgar, F., Niazi, S., et al. (2018). Detection of angiotensin II and AT1 receptor concentrations in keloid and hypertrophic scar. J Clin Aesthet Dermatol 11, 36–39.
O’Hara, A., Lim, F.-L., Mazzatti, D. J., Trayhurn, P. (2012). Stimulation of inflammatory gene expression in human preadipocytes by macrophage-conditioned medium: upregulation of IL-6 production by macrophage-derived IL-1β. Mol. Cell. Endocrinol. 349, 239–247. doi: 10.1016/j.mce.2011.10.031
O’Leary, R., Penrose, H., Miyata, K., Satou, R. (2016). Macrophage-derived interleukin 6 contributes to angiotensin II mediated angiotensinogen stimulation in renal proximal tubular cells. Am. J. Physiol. Ren. Physiol. 310 (10), F1000–1007. doi: 10.1152/ajprenal.00482.2015
Ohuchi, N., Hayashi, K., Koike, K., Kizawa, Y., Kusama, T., Ohsawa, M., et al. (2004). Pharmacological properties of angiotensin II receptors in cultured rabbit gingival fibroblasts. Comp. Biochem. Physiol. -C Toxicol. Pharmacol. 137 (3), 281–289. doi: 10.1016/j.cca.2004.02.003
Ohuchi, N., Koike, K., Sano, M., Kusama, T., Kizawa, Y., Hayashi, K., et al. (2002). Proliferative effects of angiotensin II and endothelin-1 on guinea pig gingival fibroblast cells in culture. Comp. Biochem. Physiol. -C Toxicol. Pharmacol. 132 (4), 451–460. doi: 10.1016/S1532-0456(02)00098-4
Okada, H., Murakami, S. (1998). Cytokine expression in periodontal health and disease. Crit. Rev. Oral Biol. Med. 9 (3), 248–266. doi: 10.1177/10454411980090030101
Pacurari, M., Kafoury, R., Tchounwou, P. B., Ndebele, K. (2014). The renin-angiotensin-aldosterone system in vascular inflammation and remodeling. Int. J. Inflam. 2014, 689360. doi: 10.1155/2014/689360
Panezai, J., Ghaffar, A., Altamash, M., Sundqvist, K. G., Engström, P. E., Larsson, A. (2017). Correlation of serum cytokines, chemokines, growth factors and enzymes with periodontal disease parameters. PLoS One 12 (11), e0188945 doi: 10.1371/journal.pone.0188945
Pasceri, V., Willerson, J. T., Yeh, E. T. H. (2000). Direct proinflammatory effect of C-reactive protein on human endothelial cells. Circulation 102 (18), 2165–2168. doi: 10.1161/01.CIR.102.18.2165
Patel, R. B., Pawar, V. D., Prajapati, K. D., Sonara, B. M., Deshpande, S. S., Shah, G. B., et al. (2013). Anti-nociceptive and anti-allodynic activity of aliskiren in various pain models. Eur. J. Pharmacol. 708, 80–87. doi: 10.1016/j.ejphar.2013.03.036
Patel, S. N., Ali, Q., Samuel, P., Steckelings, U. M., Hussain, T. (2017). Angiotensin II type 2 receptor and receptor mas are colocalized and functionally interdependent in obese zucker rat kidney. Hypertension 70 (4), 831–838. doi: 10.1161/HYPERTENSIONAHA.117.09679
Paul, M. (2006). Physiology of local renin-angiotensin systems. Physiol. Rev. 86 (3), 747–803. doi: 10.1152/physrev.00036.2005
Pettersson, U. S., Christoffersson, G., Massena, S., Ahl, D., Jansson, L., Henriksnäs, J., et al. (2011). Increased recruitment but impaired function of leukocytes during inflammation in mouse models of type 1 and type 2 diabetes. PLoS One 6 (7), e22480. doi: 10.1371/journal.pone.0022480
Polak, D., Shapira, L. (2018). An update on the evidence for pathogenic mechanisms that may link periodontitis and diabetes. J. Clin. Periodontol. 45 (2), 150–166. doi: 10.1111/jcpe.12803
Queiroz-Junior, C. M., Silveira, K. D., de Oliveira, C. R., Moura, A. P., Madeira, M. F. M., Soriani, F. M., et al. (2015). Protective effects of the angiotensin type 1 receptor antagonist losartan in infection-induced and arthritis-associated alveolar bone loss. J. Periodontal Res. 50 (6), 814–823. doi: 10.1111/jre.12269
Ren, M., Hao, S., Yang, C., Zhu, P., Chen, L., Lin, D., et al. (2013). Angiotensin II regulates collagen metabolism through modulating tissue inhibitor of metalloproteinase-1 in diabetic skin tissues. Diabetes Vasc. Dis. Res. 10 (5), 426–435. doi: 10.1177/1479164113485461
Ribeiro-Oliveira, A., Nogueira, A. I., Pereira, R. M., Vilas Boas, W. W., Souza dos Santos, R. A., Simões e Silva, A. C. (2008). The renin-angiotensin system and diabetes: an update. Vasc. Health Risk Manag. 4 (4), 787–803. doi: 10.2147/VHRM.S1905
Roca-Ho, H., Riera, M., Palau, V., Pascual, J., Soler, M. J. (2017). Characterization of ACE and ACE2 expression within different organs of the NOD mouse. Int. J. Mol. Sci. 18 (3), E563. doi: 10.3390/ijms18030563
Rodrigues Prestes, T. R., Rocha, N. P., Miranda, A. S., Teixeira, A. L., Simoes-e-Silva, A. C. (2017). The anti-inflammatory potential of ACE2/angiotensin-(1-7)/mas receptor axis: evidence from basic and clinical research. Curr. Drug Targets 18 (11), 1301–1313. doi: 10.2174/1389450117666160727142401
Sadjadi, J., Kramer, G. L., Yu, C. H., Welborn, M. B., Modrall, J. G. (2005). Angiotensin II exerts positive feedback on the intrarenal renin-angiotensin system by an angiotensin converting enzyme-dependent mechanism. J. Surg. Res. 129 (2), 272–277. doi: 10.1016/j.jss.2005.04.044
Santos, C. F., Akashi, A. E., Dionísio, T. J., Sipert, C. R., Didier, D. N., Greene, A. S., et al. (2009). Characterization of a local renin-angiotensin system in rat gingival tissue. J. Periodontol. 80 (1), 130–139. doi: 10.1902/jop.2009.080264
Santos, C. F., Morandini, A. C., Dionísio, T. J., Faria, F. A., Lima, M. C., Figueiredo, C. M., et al. (2015). Functional local renin-angiotensin system in human and rat periodontal tissue. PLoS One 10 (8), e0134601. doi: 10.1371/journal.pone.0134601
Sato, A., Ueda, C., Kimura, R., Kobayashi, C., Yamazaki, Y., Ebina, K. (2018). Angiotensin II induces the aggregation of native and oxidized low-density lipoprotein. Eur. Biophys. J. 47 (1), 1–9. doi: 10.1007/s00249-017-1208-8
Schunkert, H., Ingelfinger, J. R., Jacob, H., Jackson, B., Bouyounes, B., Dzau, V. J. (1992). Reciprocal feedback regulation of kidney angiotensinogen and renin mRNA expressions by angiotensin II. Am. J Physiol. 263 (5 Pt 1), E863–869. doi: 10.1152/ajpendo.1992.263.5.E863
Sexton, W. M., Lin, Y., Kryscio, R. J., Dawson, D. R., Ebersole, J. L., Miller, C. S. (2011). Salivary biomarkers of periodontal disease in response to treatment. J. Clin. Periodontol. 38 (5), 434–441. doi: 10.1111/j.1600-051X.2011.01706.x
Shah, B. R., Hux, J. E. (2003). Quantifying the risk of infectious diseases for people with diabetes. Diabetes Care 26 (2), 510–513. doi: 10.2337/diacare.26.2.510
Shenoy, V., Ferreira, A. J., Qi, Y., Fraga-Silva, R. A., Díez-Freire, C., Dooies, A., et al. (2010). The angiotensin-converting enzyme 2/angiogenesis-(1-7)/Mas axis confers cardiopulmonary protection against lung fibrosis and pulmonary hypertension. Am. J. Respir. Crit. Care Med. 182 (8), 1065–1072. doi: 10.1164/rccm.200912-1840OC
Shimada, K., Murayama, T., Yokode, M., Kita, T., Fujita, M., Kishimoto, C. (2011). Olmesartan, a novel angiotensin II type 1 receptor antagonist, reduces severity of atherosclerosis in apolipoprotein E deficient mice associated with reducing superoxide production. Nutr. Metab. Cardiovasc. Dis. 21 (9), 672–678. doi: 10.1016/j.numecd.2009.12.016
Shuai, B., Yang, Y. P., Shen, L., Zhu, R., Xu, X. J., Ma, C., et al. (2015). Local renin-angiotensin system is associated with bone mineral density of glucocorticoid-induced osteoporosis patients. Osteoporos. Int. 26 (3), 1063–1071. doi: 10.1007/s00198-014-2992-y
Skalerič, U., Kramar, B., Petelin, M., Pavlica, Z., Wahl, S. M. (1997). Changes in TGF-β1levels in gingiva, crevicular fluid and serum associated with periodontal inflammation in humans and dogs. Eur. J. Oral Sci. 105 (2), 136–142. doi: 10.1111/j.1600-0722.1997.tb00192.x
Sproston, N. R., Ashworth, J. J. (2018). Role of C-reactive protein at sites of inflammation and infection. Front. Immunol. 9, 754. doi: 10.3389/fimmu.2018.00754
Stadler, A. F., Angst, P. D. M., Arce, R. M., Gomes, S. C., Oppermann, R. V., Susin, C. (2016). Gingival crevicular fluid levels of cytokines/chemokines in chronic periodontitis: a meta-analysis. J. Clin. Periodontol. 43 (9), 727–745. doi: 10.1111/jcpe.12557
Tikellis, C., Bialkowski, K., Pete, J., Sheehy, K., Su, Q., Johnston, C., et al. (2008). ACE2 deficiency modifies renoprotection afforded by ACE inhibition in experimental diabetes. Diabetes 57 (4), 1018–1025. doi: 10.2337/db07-1212
Tikellis, C., Pickering, R., Tsorotes, D., Du, X.-J., Kiriazis, H., Nguyen-Huu, T.-P., et al. (2012). Interaction of diabetes and ACE2 in the pathogenesis of cardiovascular disease in experimental diabetes. Clin. Sci. 123 (8), 519–529. doi: 10.1042/CS20110668
Tone, A., Shikata, K., Ogawa, D., Sasaki, S., Nagase, R., Sasaki, M., et al. (2007). Changes of gene expression profiles in macrophages stimulated by angiotensin II—angiotensin II induces MCP-2 through AT1-receptor. JRAAS - J. Renin-Angiotensin-Aldosterone Syst. 8 (1), 45–50. doi: 10.3317/jraas.2007.007
Wang, C. H., Li, S. H., Weisel, R. D., Fedak, P. W. M., Dumont, A. S., Szmitko, P., et al. (2003). C-reactive protein upregulates angiotensin type 1 receptors in vascular smooth muscle. Circulation 107 (13), 1783–1790. doi: 10.1161/01.CIR.0000061916.95736.E5
Wang, T. N., Chen, X., Li, R., Gao, B., Mohammed-Ali, Z., Lu, C., et al. (2015). SREBP-1 mediates angiotensin II-induced TGF-1 upregulation and glomerular fibrosis. J. Am. Soc. Nephrol. 26 (8), 1839–1854. doi: 10.1681/ASN.2013121332
Xue, B., Yu, Y., Zhang, Z., Guo, F., Beltz, T. G., Thunhorst, R. L., et al. (2016). Leptin mediates high-fat diet sensitization of angiotensin II-elicited hypertension by upregulating the brain renin-angiotensin system and inflammation. Hypertension 67 (5), 970–976. doi: 10.1161/HYPERTENSIONAHA.115.06736
Yamazato, Y., Ferreira, A. J., Hong, K. H., Sriramula, S., Francis, J., Yamazato, M., et al. (2009). Prevention of pulmonary hypertension by angiotensin-converting enzyme 2 gene transfer. Hypertension 54 (2), 365–371. doi: 10.1161/HYPERTENSIONAHA.108.125468
Zablocki, D., Sadoshima, J. (2013). Angiotensin II and oxidative stress in the failing heart. Antioxid. Redox Signal. 19 (10), 1095–1109. doi: 10.1089/ars.2012.4588
Zenobia, C., Luo, X. L., Hashim, A., Abe, T., Jin, L., Chang, Y., et al. (2013). Commensal bacteria-dependent select expression of CXCL2 contributes to periodontal tissue homeostasis. Cell. Microbiol. 15 (8), 1419–1426. doi: 10.1111/cmi.12127
Zhang, J., Qu, H. Y., Song, J., Wei, J., Jiang, S., Wang, L., et al. (2017). Enhanced hemodynamic responses to angiotensin II in diabetes are associated with increased expression and activity of AT1 receptors in the afferent arteriole. Physiol. Genomics. 49 (10), 531–540. doi: 10.1152/physiolgenomics.00025.2017
Zhang, L., Du, J., Hu, Z., Han, G., Delafontaine, P., Garcia, G., et al. (2009). IL-6 and serum amyloid A synergy mediates angiotensin II-induced muscle wasting. J. Am. Soc. Nephrol. 20 (3), 604–612. doi: 10.1681/ASN.2008060628
Zhao, J., Liu, J., Pang, X., Wang, S., Wu, D., Zhang, X., et al. (2013). Angiotensin II induces C-reactive protein expression via AT1-ROS-MAPK-NF-κB signal pathway in hepatocytes. Cell. Physiol. Biochem. 32 (3), 569–580. doi: 10.1159/000354461
Zheng, Y., Ley, S. H., Hu, F. B. (2018). Global aetiology and epidemiology of type 2 diabetes mellitus and its complications. Nat. Rev. Endocrinol. 14 (2), 88–98. doi: 10.1038/nrendo.2017.151
Keywords: renin–angiotensin system, diabetes, periodontal disease, inflammation, renin, collagen, cytokine, chemokines
Citation: Oliveira SHP, Brito VGB, Frasnelli SCT, Ribeiro BdS, Ferreira MN, Queiroz DP, Beltan CT, Lara VS and Santos CF (2019) Aliskiren Attenuates the Inflammatory Response and Wound Healing Process in Diabetic Mice With Periodontal Disease. Front. Pharmacol. 10:708. doi: 10.3389/fphar.2019.00708
Received: 22 February 2019; Accepted: 31 May 2019;
Published: 04 July 2019.
Edited by:
Dieter Steinhilber, Goethe-Universität Frankfurt am Main, GermanyReviewed by:
Jiiang-Huei Jeng, National Taiwan University, TaiwanAstrid Parenti, University of Florence, Italy
Copyright © 2019 Oliveira, Brito, Frasnelli, Ribeiro, Ferreira, Queiroz, Beltan, Lara and Santos. This is an open-access article distributed under the terms of the Creative Commons Attribution License (CC BY). The use, distribution or reproduction in other forums is permitted, provided the original author(s) and the copyright owner(s) are credited and that the original publication in this journal is cited, in accordance with accepted academic practice. No use, distribution or reproduction is permitted which does not comply with these terms.
*Correspondence: Sandra Helena Penha Oliveira, sandra.hp.oliveira@unesp.br