- Swinburne Centre for Human Psychopharmacology, Swinburne University, Melbourne, VIC, Australia
Brain aging is a complex and multifactorial process broadly involving changes in the brain’s structure, neuronal activity, and biochemical profile. These changes in brain function have also been linked to age-associated variations in cognitive function. Recent research has suggested a role of increased oxidative stress and reduced cognition in older people. Therefore, studies that examine the effects of antioxidants on cognitive performance are important, particularly in the context of an increase in elderly populations in most Western countries. One such antioxidant, Pycnogenol, is a standardized plant-based extract obtained from the bark of the French maritime pine and has a long historical use to treat inflammation and improve health. More recently, Pycnogenol has been subjected to more than 100 research trials. In vitro and animal studies using the standardized extract have indicated a multimodal action of Pycnogenol, and several human studies have shown improvements in cognitive function after chronic administration. In this paper, we review these studies in the context of understanding both biological and cognitive changes due to Pycnogenol and evaluate possibilities of Pycnogenol to improve neurocognitive function.
Introduction
The purpose of this paper is to present mechanistic and efficacy studies relating to the antioxidative properties of the patented French maritime pine bark extract, trading under the name Pycnogenol® (PYC; Pinus pinaster ssp. atlantica, Pinaceae: Horphag Research Ltd., UK, Geneva, Switzerland) and how these mechanisms support cognitive function in aging. PYC is utilized as a nutritional supplement and as a bioactive remedy for several chronic diseases. Human clinical trials have demonstrated that PYC enhances domains of cognitive ability, and in vitro and in vivo animal and human studies have assessed its mechanisms of action, particularly its strong antioxidative properties as well as anti-inflammatory and vascular functions. Cost-effective interventions with therapeutic potential to sustain and maintain an individual’s cognitive ability and to alter the biochemical profile and symptom severity in different disease states have stimulated interest within the scientific community and are important targets, particularly for older populations.
What is Pycnogenol?
PYC is a plant-based extract derived from the bark of the French maritime pine. It grows along the coastal southwest of France. Pine bark extracts have been used traditionally and date back at least to Hippocrates (inflammation) around 400 bc and Hans Minner around 1479 to treat wounds (Drehsen, 1999). The standardized extract is composed of phenolic compounds, divided between monomers (catechin, epicatechin, and taxifolin), condensed flavonoids (classed as procyanidins/proanthocyanidins) and phenolic acids (cinnamic acids and other glycosides) (Petrassi et al., 2000; Rohdewald, 2005; refer to Figure 1). After being ingested, these phenolic compounds undergo biotransformation and are broken down in the colon by microbial enzymes yielding smaller, bioavailable molecules that can then be absorbed by the colon into the bloodstream and transported to tissues and organs (Trebatická and Ďuračková, 2015). The nutritional preparation is extracted from crushed bark, followed by a patented extraction process (Rohdewald, 2002). The quality and purity of the raw bark are assessed by a French independent regulatory and control body, The French Association of Norms Association Française des Normes, AFNOR (Association Française des Normes, AFNOR: Packer et al., 1999) and conforms to the monograph “Maritime pine extract” in the United States Pharmacopeia (USP). PYC is available in most countries as an over-the-counter product, in tablet or capsule form in doses varying from 20 to 100 mg (Rohdewald, 2002), and has demonstrated good tolerability with very few side effects and a high level of safety (Rohdewald, 2005). Based on a recent update by the American Botanical Council of the Scientific and Clinical Monograph for Pycnogenol (The American Botanical Council, 2019), it is stated that an independent panel of toxicology experts has classified PYC as generally recognized as safe (GRAS) based on clinical safety and preclinical toxicology data. Additionally, while there are no known contraindications for PYC, it is recommended that children under 6 years of age should not use PYC, and PYC should not be taken during the first 3 months of pregnancy as a precaution. The data of 91 human clinical studies (6,849 people) have informed the safety PYC whereby the frequency of adverse events (AEs) is 2.4%; and in healthy participants, the global AE rate is 0.1%. Furthermore, the AEs reported in the clinical trials were unrelated to the duration of use or dose of PYC. The most commonly reported AE was gastrointestinal discomfort; it is therefore recommended that PYC is taken with food. Other reported AEs are dizziness, headache, and nausea.
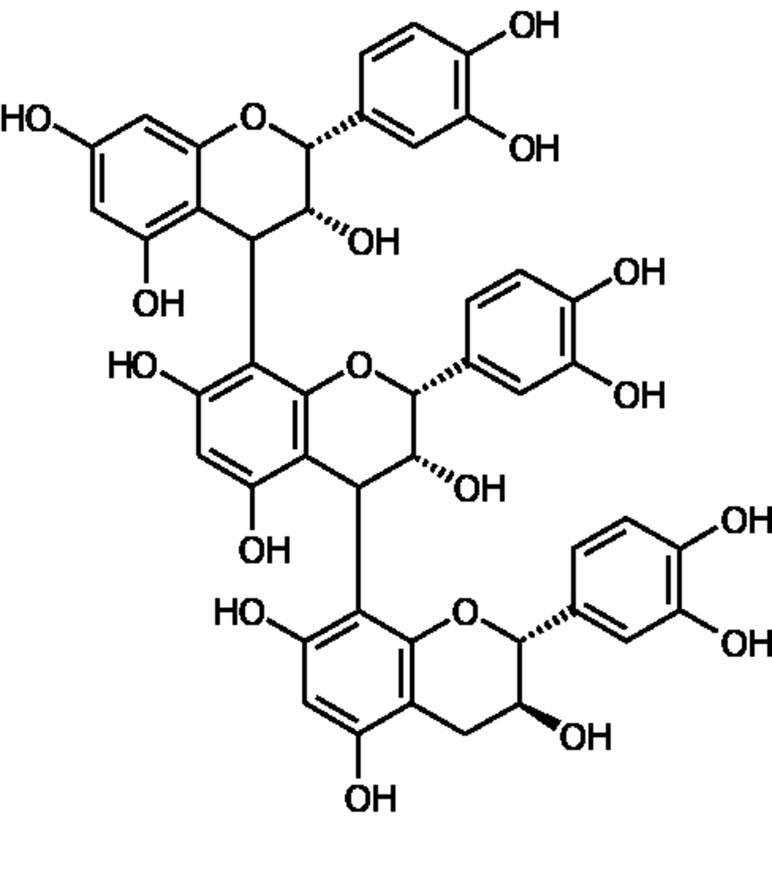
Figure 1 Structural representation of a procyanidin molecule consisting of catechin and epicatechin subunits which are the main constituents of Pycnogenol (PYC). The catechin and epicatechin units can be linked by different bonds, the main being C4-C8 bonds and C4-C6 bonds as well as C4-C8 bonds with different chain lengths up to dodecamers.
Because of its composition of multiple constituents, PYC has several actions that give rise to its reported multiple health benefits. PYC has been widely studied and has shown to have promising effects in improving conditions including diabetes (Spadea and Balestrazzi, 2001; Liu et al., 2004a; Liu et al., 2004b), cardiovascular health (Fitzpatrick et al., 1998; Liu et al., 2000; Valls et al., 2016), asthma (Hosseini et al., 2001; Lau et al., 2004; Shin et al., 2013), osteoarthritis (Farid et al., 2007; Belcaro et al., 2008a; Belcaro et al., 2008b), sexual disorders (Stanislavov and Nikolova, 2003; Aoki et al., 2012; Kobori, 2015), venous insufficiency (Petrassi et al., 2000; Cesarone et al., 2010; Gulati, 2014), and neurological disorders including attention-deficit hyperactivity disorder (Chovanová et al., 2006; Dvořáková et al., 2006; Trebatická et al., 2006) and cognitive impairment (Maimoona et al., 2011). For the purposes of this review, we characterize the antioxidative activity of PYC, how this activity relates to aging, and how these mechanisms support cognitive function.
Cognitive Function and Oxidative Stress in the Aging Brain
Cognition is a collective term generally referring to mental processes requiring the ability to remember and recall information and use information logically as well as abstractly and tasks that require sustained attention (Budson and Price, 2005). While cognitive impairment or deterioration is a hallmark of advancing age, the outcomes are more insidious where there are functional losses that impact an individual’s potential to lead an active, productive, and healthy lifestyle. Alterations in brain structure, neural activity, and biochemical profile are believed to contribute to the associated functional losses of reduced cognitive ability (Sohal and Orr, 2012). Particularly, an increase in oxidative stress, elevated inflammation, and damage to the vasculature all play a role in age-associated cognitive decline (Teunissen et al., 2003; Ansari and Scheff, 2010; Jomova et al., 2010; Waldstein and Elias, 2015). Oxidative stress is an imbalance in the production of free radicals [reactive oxygen species (ROS)] and the antioxidant system. In the elderly, age-related oxidative stress is multifactorial, marked by increased production of free radicals, reduced activities of antioxidant enzymes, diminished antioxidant levels, and weakened repair of oxidative damage (Rybka et al., 2011). During aging, the presence of persistent oxidative stress and lack of antioxidant support increases the production of amyloid-β plaques in the brain, increasing the risk of developing neurodegenerative disease, mild cognitive impairment, and Alzheimer’s disease (AD) (Smith et al., 2002; Mariani et al., 2005).
Aging impacts the brain’s capacity to scavenge free radicals such as ROS because of reduced antioxidant defenses, making oxidative stress a significant feature of general aging and age-related neurodegenerative disorders like AD (Cadenas and Davies, 2000; Valko et al., 2007). Increased production of ROS with or without decreased antioxidant defenses leads to damage of macromolecules, impaired organ function, and disease development (Finkel and Holbrook, 2000). The human brain is highly susceptible to oxidative damage. This is due to several factors, particularly because of its 1) high oxygen metabolic activity. The human brain consumes 20% of the body’s oxygen metabolism, providing energy for neuronal activity (Raichle et al., 2001). This dynamic metabolic exchange must be stable for normal brain function. Dysfunctional oxygen metabolism is the hallmark of neurodegenerative disorders associated with cognitive decline (Watts et al., 2018); 2) high concentration of polyunsaturated fatty acids (PUFAs). The biochemical process of excessive free radical-mediated peroxidation of PUFA causes destruction to cell membranes, structure, and function (Syslová et al., 2014); 3) cytotoxic action of the excitatory amino acid glutamate. Glutamate receptors are found on the surface of cells and therefore can only exert its excitatory function through extracellular fluid (Zhou and Danbolt, 2014). If glutamate receptors are excessively activated, the increased glutamate can overstimulate nerve cells, depolarizing them (known as excitotoxicity) and eventually leading to cell death (Kritis et al., 2015); 4) compared with other organs of the human body, the brain has reduced antioxidant mechanisms due to lower glutathione peroxidase (GPx) and catalase (CAT) (Packer, 1992). Both GPx and CAT reduce hydrogen peroxide into water and oxygen to assist in maintaining beneficial levels of ROS to prevent damage to molecular bonds, cell membranes, and DNA (Basu, 2010).
Additionally, essential biometals such as copper, iron, and zinc are involved in several biological processes and are essential for the survival of living organisms. These biometals, particularly iron and copper, act by increasing the speed of chemical reactions due to their oxidizing and reducing powers (redox potential) (Greenough et al., 2013). It is because of this process, where oxygen is transported and/or metabolized, that free radical formation (superoxide and hydroxyl radicals) and other ROS, formed via the Fenton reaction, increases the risk of oxidative stress in the brain (Winterbourn, 1995; Jomova and Valko, 2011). This breakdown or dyshomeostasis of biometals whereby antioxidant protection is overwhelmed in favor of elevated concentration of metals leads to a state of neurotoxicity with damaging interaction to proteins, lipid peroxidation, and DNA, all evidence of increased oxidative stress in the AD affected brain (Jomova et al., 2010; Jomova and Valko, 2011). Oxidative stress has been suggested to be not only an early feature of AD but also the mechanism that leads to the disease state (Ansari and Scheff, 2010). The cognitive impairments experienced by those with AD severely impact the individual’s ability to function on a daily basis. They are unable to remember new information and perform complex tasks, have problems with language functions as well as visuospatial abilities, and lose the ability to make good judgments (McKhann et al., 2011).
Due to these mechanisms of aging and hallmarks of neurodegenerative diseases, it may be beneficial for older individuals to consume dietary interventions with antioxidant properties to maintain cognitive function and reduce the risk of increased oxidative stress (Simpson et al., 2015). In light of this, the antioxidant mechanisms of PYC are discussed below.
Antioxidative Activity of Pycnogenol
Due to its antioxidant and various biomodulating effects, PYC has potential benefits in improving cognitive function because it acts as a regulator and protects cells against oxidative stress by 1) being a potent free radical scavenger; 2) protecting DNA from damage; 3) increasing the synthesis of antioxidant enzymes; and 4) protecting other endogenous antioxidants (vitamin C, vitamin E, and glutathione) from oxidative damage (Packer et al., 1999; Rohdewald, 2002). Demonstrating its antioxidant actions, PYC had been shown to decrease oxidative damage to DNA in children with attention deficit hyperactivity disorder (Chovanová et al., 2006). In a group of patients suffering from erectile dysfunction, plasma antioxidant activity significantly increased total cholesterol, and low-density lipoprotein cholesterol decreased in response to PYC intervention (Ďuračková et al., 2003). Experiments on cultured neuroblastoma cells exposed to oxidative stress toxicity (acrolein induced) have showed that pre-treatment with PYC significantly diminished the ensuing cytotoxicity, protein damage, lipid peroxidation, and cell death (Ansari et al., 2008). Furthermore, in animal models of dementia, PYC and vitamin E mitigated cognitive deficits and oxidative damage in the cerebral cortex and hippocampus (Ishrat et al., 2009). Clinical studies have demonstrated the antioxidant function of PYC. For example, a 6-week PYC intervention (150 mg/day) in healthy adults resulted in a significant increase in plasma oxygen radical absorbance capacity (ORAC), a biomarker of antioxidant activity (Devaraj et al., 2002). Additionally, in smokers who largely have higher oxidative stress than non-smokers, PYC supplementation (50 mg/day) reduced plasma levels of reactive oxidative species (derivatives of the reactive oxygen metabolites test) and increased antioxidant potential (biological antioxidant potential test) (Belcaro et al., 2013).
Researchers have also revealed additional pharmacological actions that may benefit conditions associated with cognitive impairments. One study showed that PYC not only exerts antioxidant activity but also increases plasma high-density lipoprotein and decreases serum low-density lipoprotein, indicating additional benefits on the lipid profile (Stough et al., 2012b). A study of erythrocyte membrane fluidity found increased membrane surface fluidity in response to PYC as well as a further outcome of protection against lipid peroxidation either from chelating metal ions or quenching free radicals, or both (Sivonová et al., 2004). As it is known that lipoproteins are associated with cognitive impairments in the elderly (Van Exel et al., 2002), this mechanism may also be important in improving cognition. PYC has also demonstrated a potential role in protecting genetic damage through its antimutagenic effects (Križková et al., 2008). Attenuation of DNA cleavage has been seen in beta-amyloid-induced apoptosis in rodent models (Peng et al., 2002). However, since research into the genetic effects of PYC is in its infancy, these findings need to be further explored to determine the clinical use of these genetic mechanisms. Findings from these studies further support the multiple roles PYC may have as a potential cognitive enhancer (Liu et al., 2000).
Mechanistically, PYC targets free radicals derived from both oxygen and nitrogen. While at higher concentrations nitric oxide (NO) is detrimental and contributes to neuroinflammation and neurodegeneration, at normal physiological levels, the role of NO is integral within the brain for neurotransmission (modulates serotonin, dopamine, and norepinephrine), neuromodulation, and synaptic plasticity (Dvořáková et al., 2006). The balance of NO production within the brain is therefore integral to cognitive health. Animal experiments have demonstrated that learning and memory increase NO production within the brain (Paul and Ekambaram, 2011). PYC either inhibits or increases vasodilation by assisting in the regulation of endothelial cell production of NO, which increases blood flow as well as oxygenation and transport of nutrients around the body to all organs including the brain (Fitzpatrick et al., 1998; Finkel and Holbrook, 2000). This action of relaxing blood vessels and making them more flexible not just benefits the brain but also reduces demand on the cardiovascular system and entire body due to better circulation.
The effects of PYC on stimulated macrophage cell cultures to mimic the immune response identified that the most active metabolite of PYC, M1 (δ-(3,4-dihydroxyphenyl)-γ-valerolactone), mediated anti-inflammatory effects by impeding nitric oxide generation and inducible nitric oxide synthase (iNOS) upregulation production (Uhlenhut and Högger, 2012). Furthermore, M1 has been identified to accumulate in erythrocytes, monocytes, and endothelial cells with this cellular uptake and has the ability to cross the blood–brain barrier (BBB) possibly via the GLUT-1-type glucose transporter (Uhlenhut and Högger, 2012; Kurlbaum et al., 2013). The M1 metabolite is a by-product of PYC consumption formed in the gut from catechins (Kurlbaum et al., 2013). An in vitro study utilizing cultured B16 melanoma cells found that while PYC suppressed reactive species in their assays, glutathione was enhanced (Kim et al., 2008). The most important intracellular antioxidant in the human body is glutathione (GSH). GSH is a unique tripeptide made from glutamate, cysteine, and glycine found predominantly in the cytosol, mitochondria, and cell nucleus (Berk et al., 2008). GSH has many roles; for example, it acts as a detoxifier, and the ratio of reduced to oxidized glutathione (GSH:GSSG) forms a marker of intracellular antioxidant redox potential to regulate drug detoxification and elimination; second, GSH’s antioxidant properties protect proteins, lipids, and nucleic acids from free radicals, peroxides, and toxins (Wu and Batist, 2013). Interestingly, the facilitated uptake of M1 into cells involves further metabolism by conjugation with GSH in erythrocytes (Kurlbaum et al., 2013). Therefore, the antioxidative properties of PYC make it particularly novel in reducing oxidative stress in the brain than do other endogenous antioxidants, which are unable to cross the BBB (Iuga et al., 2011).
Several studies discussed confirm PYC’s antioxidant and free radical scavenging effects and potential use in improving cognitive function (see Figure 2). Future studies are required to investigate the modulation of GSH metabolism in response to PYC administration and further elucidate the role of M1 and GSH. Interestingly, neuroimaging studies have not yet been performed as a method to investigate GSH metabolism and cognitive effects in relation to PYC administration. Given that oxidative stress is known to affect neuroplasticity, attempts to normalize such impairments are of great therapeutic value in aging and cognition research. Neuroimaging is a non-invasive modality that may assist in answering structural, functional, and mechanistic questions, complementary to the in vivo animal, histological, and hematological studies and human clinical trials that have been discussed in response to PYC intervention. We now review studies that have investigated the efficacy of PYC as a cognitive enhancer in humans.
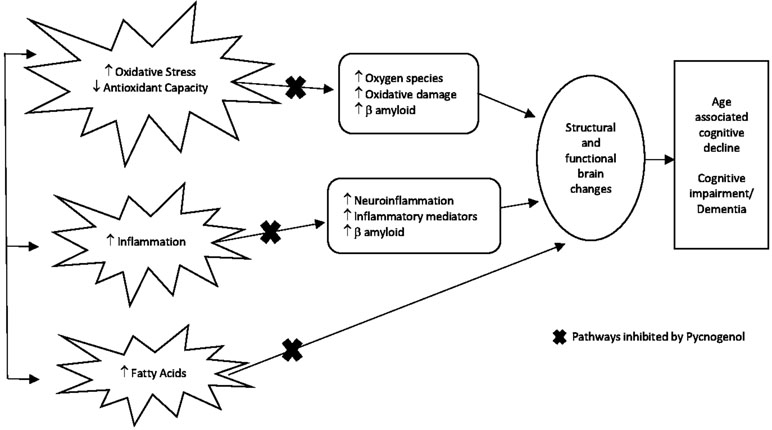
Figure 2 Proposed mechanism of action of PYC as a targeted therapy for preventing cognitive decline. With increasing age, inflammation-reduced antioxidant metabolism leading to increased oxidative stress and damage to fatty acids are common mechanisms that over time can impact on the brain causing structural and functional changes culminating in the outcome of age-associated cognitive decline, cognitive impairment, and/or dementia. PYC potentially inhibits these mechanisms as represented by the x in the diagram due to its scavenging ability to free radicals and protection of proteins (biomolecules) against oxidative damage (Packer et al., 1999; Rohdewald, 2002; Ansari et al., 2008), neuron protection from β amyloid-induced apoptonis (Peng et al., 2002; Gulati, 2014), anti-inflammatory effects (Lau et al., 2004), and reduction of fatty acids (Sivonová et al., 2004).
Pycnogenol as a Cognitive Enhancer
Preliminary findings from clinical trials show promising cognitive-enhancing effects of PYC in healthy adults. Luzzi et al. (2011) found that in a group of young healthy university students, an 8-week PYC intervention (100 mg/day) when taken in conjunction with a standardized health plan resulted in improvements in sustained attention, memory, and executive functioning. Students in this study who were taking PYC also showed improvements on mood parameters (alertness, contentedness, and reduced anxiety) and performed significantly better on actual university exams than did the control group. In a similar study, Belcaro and colleagues (2014) examined the effects of a 12-week intervention comprising PYC (150 mg/day) and a healthy lifestyle plan (controlled diet and lifestyle suggestions) in young healthy professionals (aged 33 and 55 years) who had elevated oxidative stress at baseline. These authors found significant improvements in those who were taking PYC on mood (alertness and attention) and cognitive parameters (episodic memory and spatial working memory) and a 30.4% reduction in the oxidative stress marker, with no changes seen in controls. Furthermore, a 3-month daily PYC intervention (150 mg/day) resulted in improvements in working memory, but not in concentration or psychomotor abilities, in healthy, elderly individuals and concomitant decreases in plasma levels of the oxidative stress marker F2-isoprostanes (Ryan et al., 2008). Findings from these studies are promising, and trials with larger sample sizes are currently being conducted to confirm these potential benefits of PYC in preventing cognitive decline (Stough et al., 2012b).
Conclusions
Converging evidence suggests that the biomodulating effects of PYC improve several mechanisms that may underpin cognition including vascular, anti-inflammatory, neuroprotective, and antioxidant processes. Research into PYC for improving cognitive function is growing with preliminary clinical studies indicating benefits on several cognitive domains including attention, memory, and executive functioning. Future clinical studies using PYC in larger sample sizes and over longer treatment conditions are needed to further support its use as a treatment for improving cognitive function and to further elucidate the antioxidant mechanisms supporting cognitive health and/or improvement (Stough et al., 2012a). Research investigating the role of M1 in relation to GSH as well as the effect of PYC on brain metabolites (e.g., Simpson et al., 2019) is an area of study that shows promise in elucidating the in vivo brain effects and mechanisms of PYC. Furthermore, neuroimaging techniques to investigate brain structure, function, and metabolism in response to PYC is warranted.
Author Contributions
TS, CK, and CS all contributed to the design, writing, and editing of the manuscript.
Funding
The authors thank the Australian Research Council Discovery Grant (DP1093825) to CS, with additional funding from Horphag and philanthropic grants from Doug Mitchell and Roderic O’Connor, while working on this manuscript. TS received an Australian Government Research Training Program Scholarship.
Conflict of Interest Statement
The authors conduct studies using Pycnogenol® that are funded by Horphag. Horphag had no role in the preparation of the manuscript or decision to publish.
References
Ansari, M. A., Keller, J. N., Scheff, S. W. (2008). Protective effect of Pycnogenol in human neuroblastoma SH-SY5Y cells following acrolein-induced cytotoxicity. Free. Radic. Biol. Med. 45 (11), 1510–1519. doi: 10.1016/j.freeradbiomed.2008.08.025
Ansari, M. A., Scheff, S. W. (2010). Oxidative stress in the progression of Alzheimer disease in the frontal cortex. J. Neuropathol. Exp. Neurol. 69 (2), 155–167. doi: 10.1097/NEN.0b013e3181cb5af4
Aoki, H., Nagao, J., Ueda, T., Strong, J. M., Schonlau, F., Yu-Jing, S., et al. (2012). Clinical assessment of a supplement of Pycnogenol® and l-arginine in Japanese patients with mild to moderate erectile dysfunction. Phytother. Res. 26 (2), 204–207. doi: 10.1002/ptr.3462
Basu, S. (2010). Fatty acid oxidation and isoprostanes: oxidative strain and oxidative stress. Prostaglandins Leukot. Essent. Fatty Acids 82 (4–6), 219–225. doi: 10.1016/j.plefa.2010.02.031
Belcaro, G., Cesarone, M. R., Errichi, S., Zulli, C., Errichi, B. M., Vinciguerra, G., et al. (2008a). Variations in C-reactive protein, plasma free radicals and fibrinogen values in patients with osteoarthritis treated with Pycnogenol®. Redox Rep. 13 (6), 271–276. doi: 10.1179/135100008X309019
Belcaro, G., Cesarone, M. R., Errichi, S., Zulli, C., Errichi, B. M., Vinciguerra, G., et al. (2008b). Treatment of osteoarthritis with Pycnogenol®. Phytother. Res. 22 (4), 518–523. doi: 10.1002/ptr.2376
Belcaro, G., Hu, S., Cesarone, M. R., Dugall, M. (2013). A controlled study shows daily intake of 50 mg of French pine bark extract (Pycnogenol®) lowers plasma reactive oxygen metabolites in healthy smokers. Minerva Med. 104 (4), 439–446.
Belcaro, G., Luzzi, R., Dugall, M., Ippolito, E., Saggino, A. (2014). Pycnogenol® improves cognitive function, attention, mental performance and specific professional skills in healthy professionals aged 35-55. J. Neurosurg. Sci. 58 (4), 239–248.
Berk, M., Copolov, D., Dean, O., Lu, K., Jeavons, S., Schapkaitz, I., et al. (2008). N-Acetyl cysteine as a glutathione precursor for schizophrenia—a double-blind, randomized, placebo-controlled trial. Biol. Psychiatry. 64 (5), 361–368. doi: 10.1016/j.biopsych.2008.03.004
Budson, A. E., Price, B. H. (2005). Memory dysfunction. N. Engl. J. Med. 352 (7), 692–699. doi: 10.1056/NEJMra041071
Cadenas, E., Davies, K. J. A. (2000). Mitochondrial free radical generation, oxidative stress, and aging. Free Radic. Biol. Med. 29 (3–4), 222–230. doi: 10.1016/S0891-5849(00)00317-8
Cesarone, M. R., Belcaro, G., Rohdewald, P., Pellegrini, L., Ledda, A., Vinciguerra, G., et al. (2010). Improvement of signs and symptoms of chronic venous insufficiency and microangiopathy with Pycnogenol®: a prospective, controlled study. Phytomedicine 17 (11), 835–839. doi: 10.1016/j.phymed.2010.04.009
Chovanová, Z., Muchová, J., Sivoňová, M., Dvořáková, M., Žitňanová, I., Waczulíková, I., et al. (2006). Effect of polyphenolic extract, Pycnogenol®, on the level of 8-oxoguanine in children suffering from attention deficit/hyperactivity disorder. Free Radic. Res. 40 (9), 1003–1010. doi: 10.1080/10715760600824902
Devaraj, S., Vega-Lopez, S., Kaul, N., Schonlau, F., Rohdewald, P., Jialal, I. (2002). Supplementation with a pine bark extract rich in polyphenols increases plasma antioxidant capacity and alters the plasma lipoprotein profile. Lipids 37 (10), 931–934. doi: 10.1007/s11745-006-0982-3
Drehsen, G. (1999). “20-From ancient pine bark uses to Pycnogenol” in Antioxidant food supplements in human health. Eds. Packer, L., Hiramatsu, M., Yoshikawa, T. (San Diego: Academic Press), 311–322.
Ďuračková, Z., Trebatický, B., Novotný, V., Žitňanová, I., Breza, J. (2003). Lipid metabolism and erectile function improvement by Pycnogenol®, extract from the bark of Pinus pinaster in patients suffering from erectile dysfunction—a pilot study. Nutr. Res. 23 (9), 1189–1198. doi: 10.1016/S0271-5317(03)00126-X
Dvořáková, M., Sivoňová, M., Trebatická, J., Škodáček, I., Waczuliková, I., Muchová, J., et al. (2006). The effect of polyphenolic extract from pine bark, Pycnogenol® on the level of glutathione in children suffering from attention deficit hyperactivity disorder (ADHD). Redox Rep. 11 (4), 163–172. doi: 10.1179/135100006X116664
Farid, R., Mirfeizi, Z., Mirheidari, M., Rezaieyazdi, Z., Mansouri, H., Esmaelli, H., et al. (2007). Pycnogenol supplementation reduces pain and stiffness and improves physical function in adults with knee osteoarthritis. Nutr. Res. 27 (11), 692–697. doi: 10.1016/j.nutres.2007.09.007
Finkel, T., Holbrook, N. J. (2000). Oxidants, oxidative stress and the biology of ageing. Nature 408 (6809), 239–247. doi: 10.1038/35041687
Fitzpatrick, D. F., Bing, B., Rohdewald, P. (1998). Endothelium-dependent vascular effects of Pycnogenol. J. Cardiovasc. Pharmacol. Ther. 32 (4), 509–515. doi: 10.1097/00005344-199810000-00001
Greenough, M. A., Camakaris, J., Bush, A. I. (2013). Metal dyshomeostasis and oxidative stress in Alzheimer’s disease. Neurochem. Int. 62 (5), 540–555. doi: 10.1016/j.neuint.2012.08.014
Gulati, O. P. (2014). Pycnogenol® in chronic venous insufficiency and related venous disorders. Phytother. Res. 28 (3), 348–362. doi: 10.1002/ptr.5019
Hosseini, S., Pishnamazi, S., Sadrzadeh, S. M. H., Farid, F., Farid, R., Watson, R. R. (2001). Pycnogenol® in the management of asthma. J. Med. Food 4 (4), 201–209. doi: 10.1089/10966200152744472
Ishrat, T., Parveen, K., Hoda, M. N., Khan, M. B., Yousuf, S., Ansari, M. A., et al. (2009). Effects of Pycnogenol and vitamin E on cognitive deficits and oxidative damage induced by intracerebroventricular streptozotocin in rats. Behav Pharmacol. 20 (7), 567–575. doi: 10.1097/FBP.0b013e32832c7125
Iuga, C., Alvarez-Idaboy, J. R., Vivier-Bunge, A. (2011). ROS initiated oxidation of dopamine under oxidative stress conditions in aqueous and lipidic environments. J. Phys. Chem. 115 (42), 12234–12246. doi: 10.1021/jp206347u
Jomova, K., Valko, M. (2011). Advances in metal-induced oxidative stress and human disease. Toxicology 283 (2), 65–87. doi: 10.1016/j.tox.2011.03.001
Jomova, K., Vondrakova, D., Lawson, M., Valko, M. (2010). Metals, oxidative stress and neurodegenerative disorders. Mol. Cell. Biochem. 345 (1-2), 91–104. doi: 10.1007/s11010-010-0563-x
Kim, Y. J., Kang, K. S., Yokozawa, T. (2008). The anti-melanogenic effect of Pycnogenol by its anti-oxidative actions. Food Cosmet. Toxicol. 46 (7), 2466–2471. doi: 10.1016/j.fct.2008.04.002
Kobori, Y., Suzuki, K., Iwahata, T., Shin, T., Sadaoka, Y., Sato, R., et al. (2015). Improvement of seminal quality and sexual function of men with oligoasthenoteratozoospermia syndrome following supplementation with l-arginine and Pycnogenol®. Arch. Ital. Urol. Androl. 87 (3), 190–193. doi: 10.4081/aiua.2015.3.190
Kritis, A. A., Stamoula, E. G., Paniskaki, K. A., Vavilis, T. D. (2015). Researching glutamate-induced cytotoxicity in different cell lines: a comparative/collective analysis/study. Front. Cell. Neurosci. 9, 91–91. doi: 10.3389/fncel.2015.00091
Križková, L., Chovanová, Z., Ďuračková, Z., Krajčovič, J. (2008). Antimutagenic in vitro activity of plant polyphenols: Pycnogenol® and Ginkgo biloba extract (EGb 761). Phytother. Res. 22 (3), 384–388. doi: 10.1002/ptr.2331
Kurlbaum, M., Mülek, M., Högger, P. (2013). Facilitated uptake of a bioactive metabolite of maritime pine bark extract (Pycnogenol) into human erythrocytes. PLoS ONE 8 (4), e63197. doi: 10.1371/journal.pone.0063197
Lau, B. H. S., Riesen, S. K., Truong, K. P., Lau, E. W., Rohdewald, P., Barreta, R. A. (2004). Pycnogenol® as an adjunct in the management of childhood asthma. J. Asthma 41 (8), 825–832. doi: 10.1081/JAS-200038433
Liu, F., Lau, B. H., Peng, Q., Shah, V. (2000). Pycnogenol protects vascular endothelial cells from beta-amyloid-induced injury. Biol. Pharm. Bull. 23 (6), 735–737. doi: 10.1248/bpb.23.735
Liu, X., Wei, J., Tan, F., Zhou, S., Würthwein, G., Rohdewald, P. (2004a). Antidiabetic effect of Pycnogenol® French maritime pine bark extract in patients with diabetes type II. Life Sci. 75 (21), 2505–2513. doi: 10.1016/j.lfs.2003.10.043
Liu, X., Zhou, H.-J., Rohdewald, P. (2004b). French maritime pine bark extract Pycnogenol dose-dependently lowers glucose in type 2 diabetic patients. Diabetes Care 27 (3), 839. doi: 10.2337/diacare.27.3.839
Luzzi, R., Belcaro, G., Zulli, C., Cesarone, M. R., Cornelli, U., Dugall, M., et al. (2011). Pycnogenol® supplementation improves cognitive function, attention and mental performance in students. Panminerva Med. 53 ( Suppl 1), 75–82.
Maimoona, A., Naeem, I., Saddiqe, Z., Jameel, K. (2011). A review on biological, nutraceutical and clinical aspects of French maritime pine bark extract. J. Ethnopharmacol. 133 (2), 261–277. doi: 10.1016/j.jep.2010.10.041
Mariani, E., Polidori, M. C., Cherubini, A., Mecocci, P. (2005). Oxidative stress in brain aging, neurodegenerative and vascular diseases: an overview. J. Chromatogr. B 827, 65–75. doi: 10.1016/j.jchromb.2005.04.023
McKhann, G. M., Knopman, D. S., Chertkow, H., Hyman, B. T., Jack, C. R., Jr., Kawas, C. H., et al. (2011). The diagnosis of dementia due to Alzheimer’s disease: recommendations from the National Institute on Aging–Alzheimer’s Association workgroups on diagnostic guidelines for Alzheimer’s disease. Alzheimers Dement. 7 (3), 263–269. doi: 10.1016/j.jalz.2011.03.005
Packer, L. (1992). “Free radical scavengers and antioxidants in prophylaxy and treatment of brain diseases” in Free radicals in the brain. Eds. Packer, L., Prilipko, L., Christensen, Y. (Berlin, Heidelberg: Springer), 1–20.
Packer, L., Rimbach, G., Virgili, F. (1999). Antioxidant activity and biologic properties of a procyanidin-rich extract from pine (Pinus maritima) bark, Pycnogenol. Free Radic. Biol. Med. 27 (5-6), 704–724. doi: 10.1016/S0891-5849(99)00090-8
Paul, V., Ekambaram, P. (2011). Involvement of nitric oxide in learning & memory processes. Indian J. Med. Res. 133 (5), 471–478.
Peng, Q. L., Buz’Zard, A. R., Lau, B. H. (2002). Pycnogenol protects neurons from amyloid-beta peptide-induced apoptosis. Brain Res. Mol. Brain Res 104 (1), 55–65. doi: 10.1016/S0169-328X(02)00263-2
Petrassi, C., Mastromarino, A., Spartera, C. (2000). Pycnogenol® in chronic venous insufficiency. Phytomedicine 7 (5), 383–388. doi: 10.1016/S0944-7113(00)80059-8
Raichle, M. E., MacLeod, A. M., Snyder, A. Z., Powers, W. J., Gusnard, D. A., Shulman, G. L. (2001). A default mode of brain function. Proc. Natl. Acad. Sci. U.S.A. 98 (2), 676–682. doi: 10.1073/pnas.98.2.676
Rohdewald, P. (2002). A review of the French maritime pine bark extract (Pycnogenol), a herbal medication with a diverse clinical pharmacology. Int. J. Clin. Pharmacol. Ther. 40 (4), 158–168. doi: 10.5414/CPP40158
Rohdewald, P. (2005). “Pycnogenol®, French maritime pine bark extract,” in Encyclopedia of dietary supplements. Eds. Coates, P., Blackman, M., Cragg, G., Levine, M., Moss, J., White, J. (New York: Marcel Dekker), 545–553. doi: 10.1201/b13959-57
Ryan, J., Croft, K., Mori, T., Wesnes, K., Spong, J., Downey, L., et al. (2008). An examination of the effects of the antioxidant Pycnogenol® on cognitive performance, serum lipid profile, endocrinological and oxidative stress biomarkers in an elderly population. J. Psychopharmacol. 22 (5), 553–562. doi: 10.1177/0269881108091584
Rybka, J., Kupczyk, D., Kędziora-Kornatowska, K., Pawluk, H., Czuczejko, J., Szewczyk-Golec, K., et al. (2011). Age-related changes in an antioxidant defense system in elderly patients with essential hypertension compared with healthy controls. Redox Rep. 16 (2), 71–77. doi: 10.1179/174329211X13002357050897
Shin, I.-S., Shin, N.-R., Jeon, C.-M., Hong, J.-M., Kwon, O.-K., Kim, J.-C., et al. (2013). Inhibitory effects of Pycnogenol® (French maritime pine bark extract) on airway inflammation in ovalbumin-induced allergic asthma. Food Cosmet. Toxicol. 62, 681–686. doi: 10.1016/j.fct.2013.09.032
Simpson, T., Deleuil, S., Echeverria, N., Komanduri, M., Macpherson, H., Suo, C., et al. (2019). The Australian Research Council Longevity Intervention (ARCLI) study protocol (ANZCTR12611000487910) addendum: neuroimaging and gut microbiota protocol. Nutr. J. 18 (1), 1. doi: 10.1186/s12937-018-0428-9
Simpson, T., Pase, M., Stough, C. (2015). Bacopa monnieri as an antioxidant therapy to reduce oxidative stress in the aging brain. Evid Based Complement. Alternat. Med. 2015, 615384. doi: 10.1155/2015/615384
Sivonová, M., Waczulíková, I., Kilanczyk, E., Hrnciarová, M., Bryszewska, M., Klajnert, B., et al. (2004). The effect of Pycnogenol on the erythrocyte membrane fluidity. Gen. Physiol. Biophys. 23 (1), 39–51.
Smith, M. A., Casadesus, G., Joseph, J. A., Perry, G. (2002). Amyloid-β and τ serve antioxidant functions in the aging and Alzheimer brain. Free Radic. Biol. Med. 33 (9), 1194–1199. doi: 10.1016/S0891-5849(02)01021-3
Sohal, R. S., Orr, W. C. (2012). The redox stress hypothesis of aging. Free Radic. Biol. Med. 52, 539–555. doi: 10.1016/j.freeradbiomed.2011.10.445
Spadea, L., Balestrazzi, E. (2001). Treatment of vascular retinopathies with Pycnogenol®. Phytother. Res. 15 (3), 219–223. doi: 10.1002/ptr.853
Stanislavov, R., Nikolova, V. (2003). Treatment of erectile dysfunction with Pycnogenol and l-arginine. J. Sex. Marital. Ther. 29 (3), 207–213. doi: 10.1080/00926230390155104
Stough, C., Pase, M., Cropley, V., Myers, S., Nolidin, K., King, R., et al. (2012a). A randomized controlled trial investigating the effect of Pycnogenol and Bacopa CDRI08 herbal medicines on cognitive, cardiovascular, and biochemical functioning in cognitively healthy elderly people: the Australian Research Council Longevity Intervention (ARCLI) study protocol (ANZCTR12611000487910). Nutr. J. 11 (1), 11. doi: 10.1186/1475-2891-11-11
Stough, C. K., Pase, M. P., Cropley, V., Myers, S., Nolidin, K., King, R., et al. (2012b). A randomized controlled trial investigating the effect of Pycnogenol and Bacopa CDRI08 herbal medicines on cognitive, cardiovascular, and biochemical functioning in cognitively healthy elderly people: the Australian Research Council Longevity Intervention (ARCLI) study protocol (ANZCTR12611000487910). Nutr J. 11, 11. doi: 10.1186/1475-2891-11-11
Syslová, K., Böhmová, A., Mikoška, M., Kuzma, M., Pelclová, D., Kačer, P. (2014). Multimarker screening of oxidative stress in aging. Oxid. Med. Cell. Longev. 2014, 14. doi: 10.1155/2014/562860
Teunissen, C. E., van Boxtel, M. P. J., Bosma, H., Bosmans, E., Delanghe, J., De Bruijn, C., et al. (2003). Inflammation markers in relation to cognition in a healthy aging population. J. Neuroimmunol. 134 (1–2), 142–150. doi: 10.1016/S0165-5728(02)00398-3
The American Botanical Council (2019). Scientific and clinical monograph for Pycnogenol (French maritime pine bark extract) Pinus pinaster Aiton subsp. atlantica [Fam. Pinaceae]. 2019 Update).
Trebatická, J., Ďuračková, Z. (2015). Psychiatric disorders and polyphenols: can they be helpful in therapy? Oxid. Med. Cell. Longev. 2015, 248529. doi: 10.1155/2015/248529
Trebatická, J., Kopasová, S., Hradecná, Z., Cinovsky, K., Skodácek, I., Suba, J., et al. (2006). Treatment of ADHD with French maritime pine bark extract, Pycnogenol. Eur. Child. Adolesc. Psychiatry 15 (6), 329–335. doi: 10.1007/s00787-006-0538-3
Uhlenhut, K., Högger, P. (2012). Facilitated cellular uptake and suppression of inducible nitric oxide synthase by a metabolite of maritime pine bark extract (Pycnogenol). Free Radic. Biol. Med. 53 (2), 305–313. doi: 10.1016/j.freeradbiomed.2012.04.013
Valko, M., Leibfritz, D., Moncol, J., Cronin, M. T. D., Mazur, M., Telser, J. (2007). Free radicals and antioxidants in normal physiological functions and human disease. Int. J. Biochem. Cell Biol. 39, 44–84. doi: 10.1016/j.biocel.2006.07.001
Valls, R.-M., Llauradó, E., Fernández-Castillejo, S., Puiggrós, F., Solà, R., Arola, L., et al. (2016). Effects of low molecular weight procyanidin rich extract from French maritime pine bark on cardiovascular disease risk factors in stage-1 hypertensive subjects: randomized, double-blind, crossover, placebo-controlled intervention trial. Phytomedicine 23 (12), 1451–1461. doi: 10.1016/j.phymed.2016.08.007
Van Exel, E., de Craen, A. J. M., Gussekloo, J., Houx, P., Bootsma-van der Wiel, A., Macfarlane, P. W., et al. (2002). Association between high-density lipoprotein and cognitive impairment in the oldest old. Ann. Neurol. 51 (6), 716–721. doi: 10.1002/ana.10220
Waldstein, S. R., Elias, M. F. (2015). Neuropsychology of cardiovascular disease. New York: Routledge, Taylor & Francis. doi: 10.4324/9781315851877
Watts, M. E., Pocock, R., Claudianos, C. (2018). Brain energy and oxygen metabolism: emerging role in normal function and disease. Front Mol. Neurosci. 11, 216–216. doi: 10.3389/fnmol.2018.00216
Winterbourn, C. C. (1995). Toxicity of iron and hydrogen peroxide: the Fenton reaction. Toxicol. Lett. 82-83, 969–974. doi: 10.1016/0378-4274(95)03532-X
Wu, J. H., Batist, G. (2013). Glutathione and glutathione analogues; therapeutic potentials. Biochim. Biophys. Acta. Gen. Subj. 1830 (5), 3350–3353. doi: 10.1016/j.bbagen.2012.11.016
Keywords: Pycnogenol®, aging, cognition, memory, RCT—randomized controlled trial
Citation: Simpson T, Kure C and Stough C (2019) Assessing the Efficacy and Mechanisms of Pycnogenol® on Cognitive Aging From In Vitro Animal and Human Studies. Front. Pharmacol. 10:694. doi: 10.3389/fphar.2019.00694
Received: 19 December 2018; Accepted: 28 May 2019;
Published: 03 July 2019.
Edited by:
Luc Pieters, University of Antwerp, BelgiumReviewed by:
Petra Högger, Julius-Maximilians-Universität Würzburg, GermanyZdenka Ďuračková, Comenius University, Slovakia
Copyright © 2019 Simpson, Kure and Stough. This is an open-access article distributed under the terms of the Creative Commons Attribution License (CC BY). The use, distribution or reproduction in other forums is permitted, provided the original author(s) and the copyright owner(s) are credited and that the original publication in this journal is cited, in accordance with accepted academic practice. No use, distribution or reproduction is permitted which does not comply with these terms.
*Correspondence: Tamara Simpson, tsimpson@swin.edu.au