- 1School of Traditional Chinese Medicine, Capital Medical University, Beijing, China
- 2School of Pharmaceutical Sciences, Capital Medical University, Beijing, China
- 3State Key Laboratory of Dao-di Herbs, National Resource Center for Chinese Materia Medica, China Academy of Chinese Medical Sciences, Beijing, China
Salvia miltiorrhiza Bunge, a member of the Lamiaceae family, is valued in traditional Chinese Medicine. Its dried root (named Danshen) has been used for hundreds of years, primarily for the treatment of cardiovascular and cerebrovascular diseases. Tanshinones are the main active ingredients in S. miltiorrhiza and exhibit significant pharmacological activities, such as antioxidant activity, anti-inflammatory activity, cardiovascular effects, and antitumor activity. Danshen dripping pill of Tianshili is an effective drug widely used in the clinical treatment of cardiovascular diseases. With the increasing demand for clinical drugs, the traditional method for extracting and separating tanshinones from medicinal plants is insufficient. Therefore, in combination with synthetic biological methods and strategies, it is necessary to analyze the biosynthetic pathway of tanshinones and construct high-yield functional bacteria to obtain tanshinones. Moreover, the biosynthesis of tanshinones has been studied for more than two decades but remains to be completely elucidated. This review will systematically present the composition, extraction and separation, pharmacological activities and biosynthesis of tanshinones from S. miltiorrhiza, with the intent to provide references for studies on other terpenoid bioactive components of traditional Chinese medicines and to provide new research strategies for the sustainable development of traditional Chinese medicine resources.
Introduction
Salvia miltiorrhiza Bunge, an important Salvia species with terrific economic, social and medicinal benefits (Su et al., 2015), was first recorded in the Shenlong Bencao Jing (200–300 AD, Han Dynasty), the oldest medicine monograph in China. Salviae miltiorrhizae Radix et Rhizoma, dry roots of Danshen, has been widely applied in the clinical treatment of cardiovascular diseases (Chen and Chen, 2017), dysmenorrhea, amenorrhea, and hypertension, hepatocirrhosis, chronic renal failure and other diseases (Su et al., 2015). With the development of science and technology, the dosage forms containing Danshen have been gradually diversified. Tablet, injection solution, dripping pill, oral liquid, capsule, slow-release formulation, and soft gel are all dosage forms that have been prepared into medicines. Among these diverse preparations, composite Danshen droplet pills, used to treat angina pectoris and coronary heart disease, represent a star drug and, moreover, a demonstration of traditional Chinese medicine entering the international market. Currently, advanced clinical tests on this drug are ongoing in the United States. The active ingredients in S. miltiorrhiza (Table 1) can be mainly divided into two categories: fat-soluble tanshinone compounds and water-soluble salvianolic acids. Both ingredients have significant pharmacological activities and are also representative active ingredients in traditional Chinese medicine (Ma et al., 2015), especially tanshinones.
As secondary metabolites, tanshinones mainly accumulate in the roots, but the yield is very low. Currently, tanshinones are obtained from roots by chemical separation and purification. However, because traditional methods have low efficiency, high energy consumption and unfriendliness to the environment and plant resources, it is meaningful and necessary to find new methods. With the successful applications of synthetic biology technology in the study of taxol, ginsenoside, and artemisinin (Huang et al., 2014), this approach could also provide new developments and approaches for the modern study of tanshinones (Shi et al., 2018). Based on research on tanshinones at home and abroad, this paper will systematically review the research results of extraction, purification, pharmacological activity and biosynthesis of the original plant S. miltiorrhiza.
The Plant S. miltiorrhiza
Salvia miltiorrhiza Bunge belongs to the genus Salvia of the Lamiaceae family, which is a perennial erect herb. Its dry root is used as Danshen and has the effects of promoting blood circulation, relieving pain, regulating heat, and calming the nerves.
Wild S. miltiorrhiza generally grows in central and northeastern China. Environmental and soil conditions affect the quality of Danshen and the content of active ingredients (Peng et al., 2014). The Chinese Flora reports that there are two varieties in the S. miltiorrhiza species, namely, the original variety (S. miltiorrhiza var. miltiorrhiza) and S. miltiorrhiza Bunge var. charbonnelii. The original variety can be divided into two forms, namely, the original form (S. miltiorrhiza var. miltiorrhiza f. miltiorrhiza) and S. miltiorrhiza var. miltiorrhiza f. alba. These forms mainly differ in plant morphology and geographical distribution. For example, the flower color of S. miltiorrhiza var. miltiorrhiza f. alba is white, and it originates from Shandong, China.
In recent years, due to the excessive harvesting of wild S. miltiorrhiza, the resources are already on the verge of extinction. With the reduction in wild resources, artificial domestication cultivation of S. miltiorrhiza has been carried out since the 1970s. The main producing areas of the current cultivated S. miltiorrhiza are Linyi Shandong Province, Jiaozuo Henan Province, Wanrong Shanxi Province, Shangluo Shaanxi Province, Zhongjiang Sichuan Province and other places. To date, S. miltiorrhiza from Sichuan Province is still considered to have the best quality. Due to the low yield of secondary metabolites and the long growth cycle of cultivated plants, the production of tanshinones from cultivated S. miltiorrhiza can no longer meet the rapidly growing market demand. It is essential to use modern biotechnology methods to increase the yield. Therefore, various in vitro culture systems of S. miltiorrhiza, including suspension cell (Zhao et al., 2010), callus (Wu et al., 2003), adventitious root and hairy root, as well as new techniques such as using endophytic fungi (Li et al., 2016; Zhou et al., 2018) and transgenic plants (Liu et al., 2017; Wei et al., 2018), can be used to accumulate tanshinone production.
Tanshinones of S. miltiorrhiza
In the 1930s, domestic and foreign scholars have begun to study the chemical constituents of S. miltiorrhiza (Xu et al., 2018). Japanese scholar Nakao first isolated tanshinone IIA from S. miltiorrhiza and identified its chemical structure in 1934. After the 1970s, with the development of clinical application of S. miltiorrhiza and the advancement of Chinese medicine extraction and separation technology, its chemical composition research has developed rapidly (Kakisawa et al., 1968, 1969). Tanshinones, also known as total tanshinone, are a general term for a class of lipophilic phenanthrene compounds that are rich in the roots of S. miltiorrhiza (Ma et al., 2015). What these compounds have in common is that they possess an ortho- or fluorene structure and can be reduced to a diphenol derivative, which is converted to hydrazine after oxidation. In this process, these molecules facilitate electron transfer, and can promote or interfere with various reactions of the body; accordingly, these compounds exhibit significant pharmacological properties such as anti-cancer, antibacterial and anti-viral effects. To date, more than 40 tanshinones (Pang et al., 2016) have been isolated from S. miltiorrhiza, including ortho-quinones (i.e., tanshinone I, tanshinone IIA, dihydrotanshinone, and cryptotanshinone) and para-quinones (i.e., isotanshinone I, isotanshinone IIA, and isocryptotanshinone). In addition, other diterpenoids and triterpenoids have been isolated from Danshen (Wu et al., 2016), such as danshenxinkun A/B/C/D and neo-tanshinlactone (Gao S. et al., 2012). With the increasing clinical demand for tanshinones, more research is currently being conducted to explore how to efficiently obtain tanshinones and enhance their pharmacological effects by exploring structure activity relationships (Pickens et al., 2011; Krivoruchko and Nielsen, 2015; Luo et al., 2015; Figure 1).
Extraction and Separation Methods of Tanshinones
Due to the clinical application of tanshinones, the extraction, and purification processes of these compounds were developed early in tanshinone research, and the research on associated extraction and separation process technologies is relatively extensive. The main extraction methods include alcohol extraction, ultrasonic extraction, supercritical CO2 fluid extraction (Wang L. et al., 2015), pressurized liquid extraction (PLE) (Ong and Len, 2004; Li et al., 2007), microwave-assisted extraction (Pan et al., 2001), high-speed countercurrent chromatography, column chromatography, and vacuum liquid phase extraction.
Because terpenoids generally have low polarity, they are often extracted with chloroform, ethyl acetate or petroleum ether. For some slightly more polar compounds, the plant roots are usually first degreased and then extracted with a polar solvent such as acetone (Wang et al., 2012) and n-butanol (Pang et al., 2016). Although the alcohol extraction method is a relatively traditional extraction process, it is still widely used in the market for its simple and easy operation, and modern ideas have been incorporated to optimize the method. For example, by using orthogonal design, the best extraction and purification processes for total tanshinones were identified. The content of total tanshinones in crude extract increased from 18.23 to 57.29% by purification.
The ultrasonic extraction method does not require heating due to the short production cycle, thus avoiding the thermal degradation reaction of tanshinone IIA. Compared with traditional methods, such as methanol reflux extraction and organic solvents macerating at room temperature, ultrasonic-assisted extraction and microwave-assisted extraction of tanshinones have advantages such as extraction speed and high efficiency (Pan et al., 2002). Similarly, supercritical fluid extraction technology (SFE) (Wang L. et al., 2015) has a short production cycle and does not require heating, which avoids the degradation reaction of tanshinone IIA. Compared with the traditional alcohol extraction method, SFE can retain more tanshinone IIA, but due to the expensive equipment and high production cost, it still needs further promotion to enter the market.
In addition, the extraction of tanshinones has many new methods and new technologies, such as non-ionic surfactant-assisted extraction (Bi et al., 2011), infrared-assisted extraction (Chen et al., 2010), ionic liquid-based ultrahigh pressure extraction (Liu et al., 2013), and selectively modified microfluidic chips (Xuan et al., 2010). The combined application of methods can make extraction more efficient and productive, for example, ultrasound-assisted extraction combined with an amino-modified monolithic cartridge used as a solid-phase extraction sorbent to improve extraction efficiency (Tao et al., 2013); on-line continuous sampling combined with ionic liquid-based dynamic microwave-assisted extraction (Gao S. et al., 2015); a dynamic continuous ultrasound-assisted extraction with a high-intensity ultrasonic probe (CUAE-HIUP) combined with solid-phase extraction (SPE) (Qiao et al., 2007); and ionic liquid surfactant-mediated ultrasonic-assisted extraction combined with high performance liquid chromatography (HPLC) (Wu et al., 2015).
Chromatography is commonly used for the separation and purification of tanshinone monomers. The semipreparative high-speed countercurrent chromatography (HSCCC) technique (Sun et al., 2011) could successfully separate and purify the four major components of Danshen at high purities of over 95% (Tian et al., 2002). Furthermore, the high-efficiency separation of tanshinones could be achieved by the macroporous resin adsorption method and the countercurrent chromatography analog gradient elution method (Wu S. et al., 2012; Figure 2).
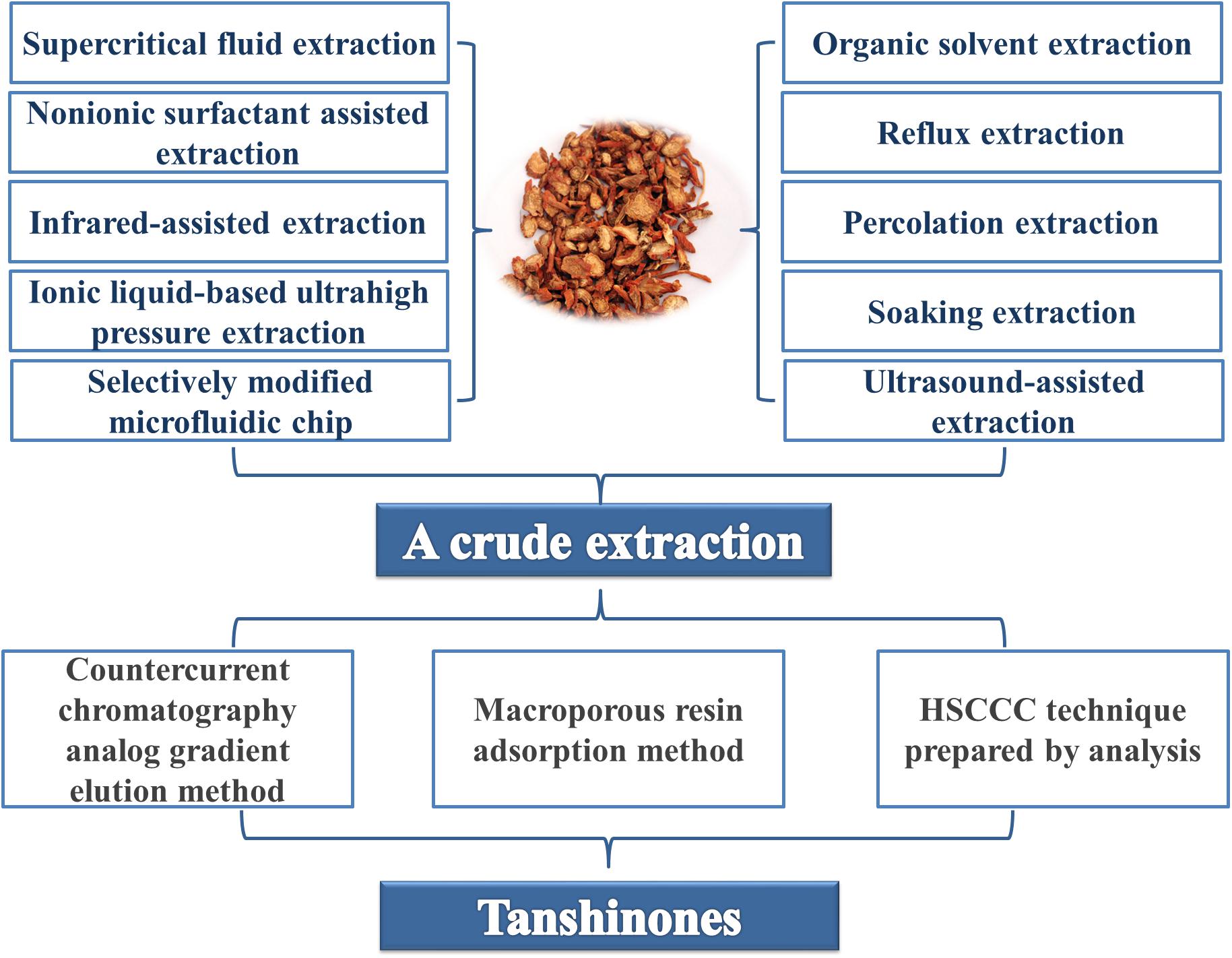
Figure 2. A brief summary of the extraction and separation of tanshinones from the original plants of S. miltiorrhiza.
Pharmacological Activities of Tanshinones
Tanshinones have received extensive attention due to their remarkable activities in the clinical treatment of cardiovascular diseases. With deepening research, these compounds have been found to possess a wide range of pharmacological activities, such as antibacterial, antioxidant, anti-inflammatory, and anti-tumor properties. A brief introduction to the pharmacological activity of tanshinones will follow (Figure 3).
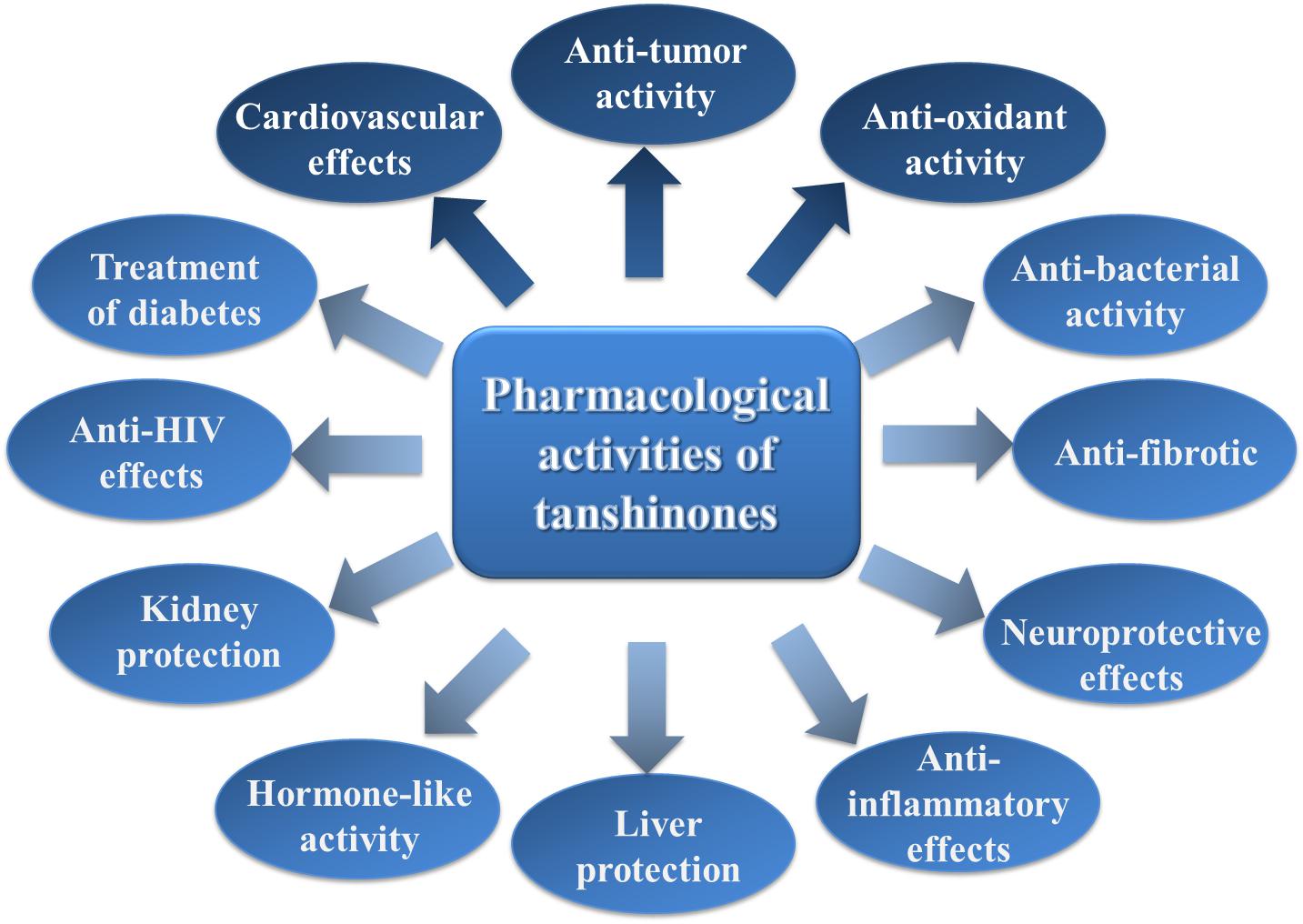
Figure 3. The brief summary of the pharmacological activities of tanshinones. The boxes filled with dark blue indicate that the pharmacological activities are stronger, and the boxes filled with light blue indicate that the pharmacological activities are general.
Anti-tumor Activity
There are many kinds of anti-tumor drugs, and their mechanisms of action are also different. For example, some drugs can interfere with the anabolism of nucleic acids, inhibit mitosis, and inhibit protein synthesis. Currently, most of the applied chemotherapy drugs are cytotoxic and cannot only kill cancer cells, but also cause side effects (Yuan et al., 2003). The phenanthrene-quinone structure widely present in tanshinone compounds is a major cause of the cytotoxicity (Wu et al., 1991; Shi et al., 1997; Wang et al., 2004). During the antitumor reaction, the DNA molecule binds to the phenanthrene ring structure of the tanshinone, and the furan ring and steroid structure generate free radicals to hinder the synthesis of DNA in the tumor cells. Tanshinone IIA can regulate oxidative stress and reduce the mitochondrial membrane potential to induce apoptosis in cancer cells such as human lung cancer A549 cells (Chiu and Su, 2010); tanshinones inhibit the growth of human prostate cancer cell lines by arresting the cell cycle and inducing apoptosis in vitro. Among them, tanshinone I has the most significant activity with IC50 approximately 3–6 μM and its side effects on normal prostate epithelial cells are relatively small (Gong et al., 2011); cryptotanshinone induces tumor cell apoptosis by triggering cell cycle arrest, altering mitochondrial signaling pathways, and by other apoptosis-regulating proteins or death receptor pathways; the methanol extract of S. miltiorrhiza induced apoptosis in non-small cell lung cancer through phosphatase and tensin homolog (PTEN)-mediated inhibition of the PI3K/Alct pathway (Ye et al., 2017); S. miltiorrhiza exerted clear cytotoxic effects, and strongly inhibited the proliferation of HepG2 cells (Liu et al., 2000); and tanshinone I inhibits tumor angiogenesis by the phosphorylation of reducing signal transducers and activators of transcription (STAT3) at TYR705 and hypoxia-induced HIF-1a accumulation in both endothelial and tumor cells (Wang Y. et al., 2015). In summary, tanshinones are expected to become a new type of anti-tumor drug with high efficiency and low toxicity.
Effects on the Cardiovascular System
Cardiovascular diseases refer to ischemic or hemorrhagic diseases in the heart, brain and whole body tissues caused by hyperlipidemia, blood viscous, atherosclerosis, and hypertension. S. miltiorrhiza and its compound preparations, such as composite Danshen droplet pills (Zhang et al., 2008) and Guanxin Danshen drop pills (Yao et al., 2018), have achieved good curative effects in the clinical treatment of cardiovascular diseases. Modern pharmacological research has found that S. miltiorrhiza has been widely used in the treatment of coronary artery, myocardial ischemia and myocardial infarction, improvement of microcirculation, and reduction of myocardial oxygen consumption.
Tanshinone IIA is a representative monomeric compound in S. miltiorrhiza. However, due to the poor water solubility, its water-soluble derivative, sodium tanshinone IIA silate (STS), is more widely used in the clinical treatment. Experimental results show that STS restrains the proliferation and migration of high glucose-induced vascular smooth muscle cells (VSMC), possibly through adenosine 5′-monophosphate (AMP)-activated protein kinase (AMPK) activation (Chen, 1984; Kyoko et al., 2002; Yang et al., 2007). In addition, STS has protective effects on myocardial apoptosis induced by acute myocardial infarction (MI) in adult rats (Yang et al., 2008) and inhibits cardiac fibrosis after pathological stimuli to the cardiovascular system (Le et al., 2009). The clinical efficacy results also show that tanshinone IIA sodium sulfonate injection is effective in the treatment of acute cerebral infarction, and can also be used as an additional treatment for patients with unstable angina pectoris (Zhang et al., 2014).
As the main lipophilic component of S. miltiorrhiza, tanshinone IIA also has cardiovascular protective effects. Its mechanisms of action mainly include the following: significantly reducing intimal thickening and restraining the proliferation and migration of VSMC, which is a significant event in the development of atherosclerosis (AS); by reducing the content of macrophages, reducing the uptake of oxidized low-density lipoprotein (ox-LDL) (Gong et al., 2009; Liu et al., 2014), promoting cholesterol excretion, and reducing the formation of foam cells, thereby regulating blood lipids and reducing body weight; inhibiting the development of left ventricular hypertrophy and reducing the level of apoptosis; significantly reducing blood viscosity, inhibiting thrombin activation, promoting fibrin degradation and inhibiting the formation of thrombosis (Wu L. et al., 2012). In summary, tanshinone IIA has broad application prospects in the prevention and treatment of cardiovascular diseases, but its specific mechanism remains to be further studied. In addition to tanshinone IIA, cryptotanshinone (Li et al., 2018), tanshinone I, dihydrotanshinone I (Wei Y. et al., 2016), etc., have proved to be effective coronary dilators, which can improve microcirculation and enhance vascular blood flow.
Antioxidant Activity
Oxidative stress can cause DNA oxidative damage and abnormal expression of proteins puts the body in a vulnerable state, and is intently connected with the occurrence and development of various diseases. Previous studies have shown that tanshinone I, tanshinone IIB, cryptotanshinone, dihydrotanshinone I, methylenetanshinquinone, and miltiradiene (Houlihan et al., 1985) were all found to act as antioxidants (Ke et al., 1990; Weng and Gordon, 1992). The specific antioxidant activity of tanshinone IIA is that it can effectively restrain the interaction between DNA and the intracellular lipid peroxidation products (Cao et al., 1996; Niu et al., 2000; Zhou et al., 2013) and protect DNA by eliminating lipid free radicals and blocking the chain reaction of lipid peroxidation. In addition, tanshinone IIA inhibits atherosclerosis calcification in rats by inhibiting oxidative stress (Tang et al., 2007). Latter researches have indicated that it can also inhibit the activity and gene expression of nicotinamide adenine dinucleotide phosphate (NADPH) oxidase, as well as the production of reactive oxygen species (ROS) (Yang et al., 2010; Wang et al., 2011; Huang et al., 2018).
Anti-bacterial Activity
The pharmacological effects of S. miltiorrhiza are very extensive, and in addition to the anti-tumor activity, which has been explored deeply, it has antibacterial and anti-inflammatory effects. The main lipophilic components (Lee et al., 1999) that play the antibacterial roles in S. miltiorrhiza are cryptotanshinone, dihydrotanshinone I (Honda et al., 1988), hydroxyl tanshinone and tanshinone IIB. These compounds could significantly inhibit gram-positive bacteria, especially cryptotanshinone and dihydrotanshinone I exhibited strong antimicrobial activity. They could bring forth superoxide radicals, which are of great significance in the antibacterial action. In comparison, tanshinone IIA and tanshinone I were confirmed to have medium antimicrobial activity (Zhao et al., 2011).
Effects on Neurodegenerative Disorders
Neurodegenerative diseases are caused by the loss of neurons or their myelin, which over time, in succession causes degeneration of the structure and function of the central nervous system (Javed et al., 2016). Neurodegenerative diseases could be divided into two categories according to their phenotype: one that affects body movement, like Parkinson’s disease, and one that affects memory and related dementia, like Alzheimer’s disease (AD) (Prince et al., 2013; Ahmed et al., 2015; Orhan et al., 2015). Due to the good lipophilicity and small molecular weight, tanshinones are able to penetrate the blood-brain barrier (BBB). For example, tanshinone I (Jing et al., 2016), tanshinone IIA (Bi et al., 2008), and cryptotanshinone (Zhang F. et al., 2009; Park et al., 2012) have been reported to exhibit significant neuroprotective effects. Tanshinone I selectively suppressed pro-inflammatory gene expression in activated microglia and prevented nigrostriatal dopaminergic neurodegeneration in a mouse model of Parkinson’s disease (Wang S. et al., 2015). Tanshinone IIA protects neurons from the neurotoxicity of amyloid beta (Aβ) 25–35, increases neuronal viability and downregulates the expression of phosphorylated tau in AD. In addition, tanshinone IIA inhibits the transcription and expression of genes encoding inducible nitric oxide synthase (iNOS), metalloproteinase (MMP)-2, and human nuclear factor (NF-κBp65) pathway, thereby inhibiting the occurrence of neuroinflammation and reducing AD risk (Jiang P. et al., 2014).
Other Bioactivities
In addition to the above mentioned pharmacological activities, tanshinone compounds exhibit anti-inflammatory effects (Fan et al., 2009), liver protection, protection against kidney damage, hormone-like activity, and so on. Methyl tanshinonate, hydroxytanshinone, tanshinone IIA, and tanshinone IIB show anti-platelet aggregation activity. Tanshinone IIA has the potential to ameliorate bone resorption diseases in vivo by reducing both the number and activity of osteoclasts (Han et al., 2007). Cryptotanshinone and dihydrotanshinone I have been reported to have anti-cholinesterase activity (Ren et al., 2004). Due to their wide range of pharmacological activities, tanshinones are expected to contribute to the development of new drugs for clinical treatment.
Biosynthesis of Tanshinones in S. miltiorrhiza
Terpenoids are derived from methylpentahydroxy acid and have a molecular skeleton of an isoprene unit (C5 unit) as a basic structure unit. Terpenoids are widely found in nature and are also important class of compounds in Chinese herbal medicine (Cheng et al., 2007). According to the number of C5 units, terpenoids can be divided into monoterpenes, sesquiterpenes, diterpenes, and triterpenes, in addition to the more specific structure of iridoids. The biosynthesis pathway of terpenoids has certain rules to follow. The common precursor iospentenyl diphosphate (IPP) and its isomer dimethylallyl diphosphate (DMAPP) are generated by the 2-C-methyl-D-erythritol 4-phosphate (MEP) pathway and/or the mevalonate (MVA) pathway. Under the action of prenyltransferase, IPP and DMAPP condense to form precursors of monoterpenes, sesquiterpenes, diterpenes, and similar compounds. These precursors form the basic skeleton of various terpenoids under the action of a quinone synthase, and then form a final product under the action of a modifying enzyme such as cytochrome P450 hydroxylase or glycosyltransferase (Hasan et al., 2014; Zi et al., 2014). Tanshinones are a group of diterpenoids, and the biosynthesis pathway in S. miltiorrhiza also follows the above rules. Interference experiments have shown that the MVA pathway plays a more critical role in the cell growth of S. miltiorrhiza hairy roots, while the MEP pathway is more committed to the production of tanshinones (Yang et al., 2012). Of course, the interrelationship and crosstalk between the two pathways together promote cell growth and metabolite production (Figure 4).
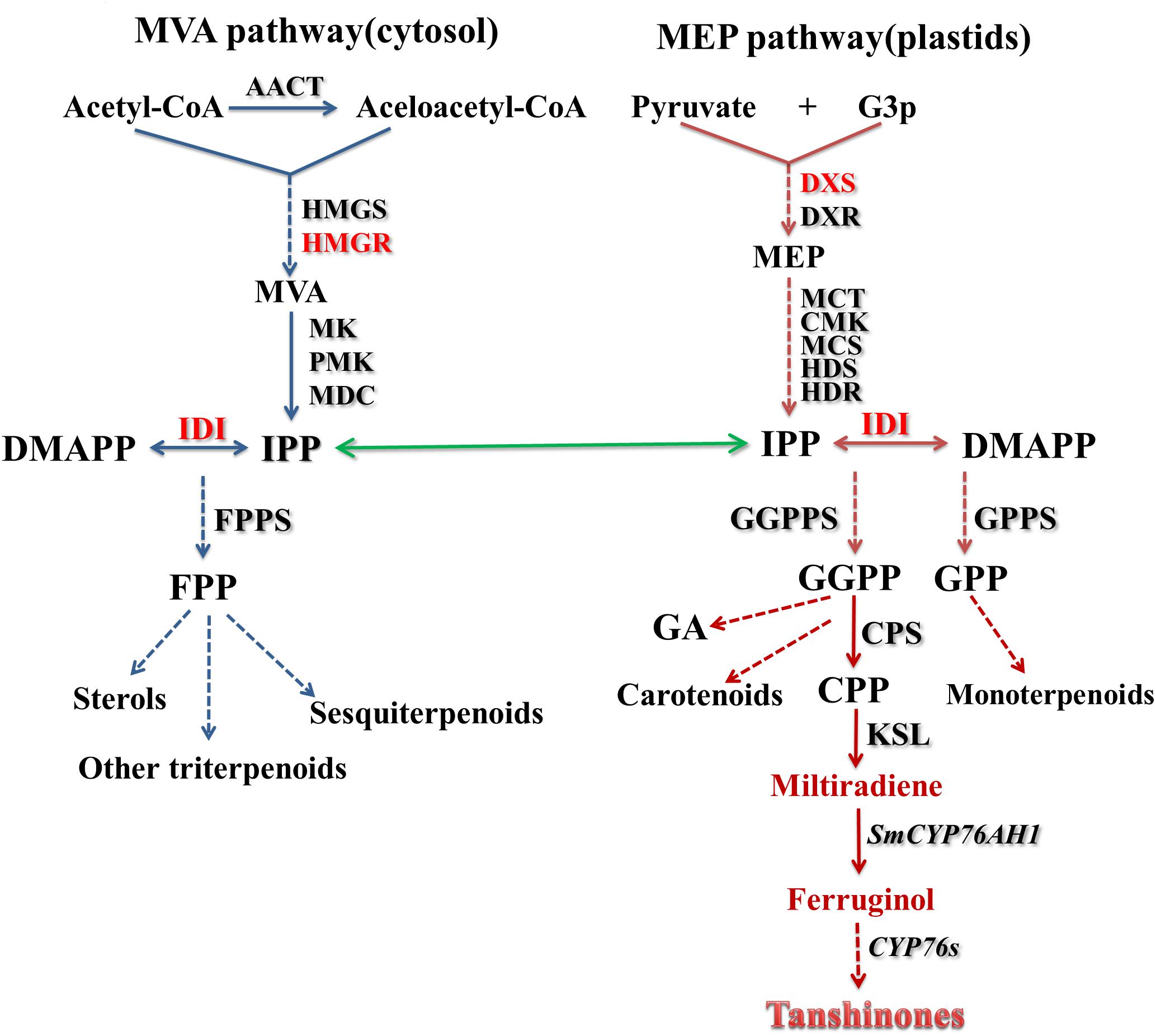
Figure 4. Outline of the biosynthesis pathway of tanshinones in S. miltiorrhiza. The common precursors IPP and DMAPP of the universal isoprene precursors are generated by MEP and MVA pathways. TPSs catalyze the production of GGPP by IPP and DMAPP, and GGPP further produces tanshinones through a series of cyclization and hydroxylation reactions. The abbreviations used are listed in Table 1.
The MVA Pathway
Since the late 1950s, the MVA pathway has received extensive attention due to its involvement in the biosynthesis pathway of plant steroids. The MVA pathway begins with acetyl-CoA and ends with the production of IPP and DMAPP. 3-Hydroxy-3-methylglutaryl-CoA reductase (HMGR) (Dai et al., 2011), a rate-limiting enzyme in isoprenoid biosynthesis, catalyzes the conversion of HMG-CoA to MVA. There are four SmHMGRs (Cui et al., 2011) in the draft genome of S. miltiorrhiza.
The analysis of the tissue expression of the four genes is as follows: SmHMGR1 has the highest tissue expression in flowers and root steles and the lowest expression in leaves; SmHMGR2 is expressed in higher amounts in roots and stems; in addition to the low expression level in flowers, SmHMGR3 expressed the highest of the four homologous genes in other tissues (Zhang et al., 2012); in contrast, SmHMGR4 has the highest tissue expression in flowers, but has the lowest expression in other tissues among the four homologous genes. Induced expression experiments show that SmHMGR1, SmHMGR2 and SmHMGR3 are sensitive to methyl jasmonate (MeJA) (Pan et al., 2009; Dai et al., 2011; Ma et al., 2012). Overexpression of the above three genes can significantly increase the content of tanshinones in hairy root culture.
In addition to SmHMGRs, the genes involved in the MVA pathway include SmAACT, SmHMGS, SmMK, SmPMK, and SmMDC (Cui et al., 2010; Lin et al., 2011; Ma et al., 2012). The proteins encoded by these genes each play a specific function, catalyzing the corresponding reactions and performing their duties; thus, IPP and DMAPP are finally obtained from the MVA pathway.
The MEP Pathway
The MEP pathway, also appearing the non-mevalonate pathway, uses pyruvate (Pyr) and glyceraldehyde 3-phosphate (G3P) as precursors to produce the two products (IPP and DMAPP) under the action of a series of enzymes. In plants, the MEP pathway is localized in the plastid and provides precursors of the synthesis of terpenoids, as well as side chains of chlorophylls and tocopherols.
Many genes in the MEP pathway have been cloned and identified (Wang et al., 2008; Gao W. et al., 2012; Ma et al., 2012; Cheng Q.Q. et al., 2013; Hao et al., 2013; Jiang D. et al., 2014). 1-Deoxy-D-xylulose-5-phosphate synthase (DXS) is the first enzyme in the MEP pathway and a key regulatory site for the pathway (Wei Z. et al., 2016). There are five DXSs (Ma et al., 2012) in the draft genome of S. miltiorrhiza, and SmDXS2 expression was predominantly detected in roots than the other four (Kai et al., 2011; Wu et al., 2011), which suggested that SmDXS2 is more likely to be involved in the biosynthetic pathway of tanshinones. Phylogenetic analysis showed that SmDXS2 and SmDXS3 were clustered into one branch, belonging to the DXS2 clade. SmDXS3 also has high tissue expression in roots and root steles and is highly sensitive to MeJA, indicating that it may be involved in plant defense mechanisms.
SmDXR catalyzes 1-deoxy-D-xylulose 5- phosphate reductoisomerase (DXP) to generate MEP. SmDXR is expressed in various tissues and has the highest expression in roots. Under high-osmotic stress and yeast elicitors, the content of tanshinones in S. miltiorrhiza hairy roots increased significantly and RT-PCR results showed that the transcription level of SmDXR gene was also upregulated. The DXR genes may contribute to the control of tanshinone metabolic route and be a regulatory site for efficient heterogeneous production of tanshinones (Yan et al., 2009; Kai et al., 2011; Wu et al., 2011).
Genes Involved in the Miltiradiene Branch Pathway
Isopentenyl diphosphate isomerase (IDI), also known as isopentenyl pyrophosphate isomerase, catalyzes the conversion of the relatively unreactive IPP to the more-reactive electrophile DMAPP. Two IDI genes (SmIDI1 and SmIDI2) have been found and cloned from S. miltiorrhiza. SmIDI1 has been confirmed as a candidate gene involved in the biosynthesis of tanshinone (Cui et al., 2011; Yang et al., 2011). Tissue expression analysis revealed that SmIDI1 has the higher expression in leaves than in the roots and stems. The relative expression of SmIDI1 increased under the induction of Ag+ and MeJA. Color complementation experiments showed that SmIDI1 promoted the accumulation of lycopene. Compared with SmIDI1, there are few studies about SmIDI2 (Ma et al., 2012), which has a chloroplast-localized transit peptide, whereas SmIDI1 does not have one. The tissue expression level of SmIDI2 was lower than that of SmIDI1 in each analyzed tissue. In general, SmIDI1 may play a more important role in the biosynthesis process of tanshinones.
As universal precursors for the biosynthesis of terpeniods, both IPP and DMAPP condense to form precursors for monoterpene, diterpene, sesquiterpene and triterpenes, and so forth under the action of specific prenyltransferases. For example, one molecule of IPP and one molecule of DMAPP generate farnesyl diphosphate (FPP) under the catalysis of farnesyl diphosphate synthase (FPPS). FPPS belongs to family of short-chain prenyltransferases which also includes geranyl diphosphate synthase (GPPS), geranylgeranyl diphosphate synthase (GGPPS).
Analysis of the S. miltiorrhiza genome shows there are five GPPS genes (SmGPPS encoding the homomeric GPPS subunit; SmGPPS.LSU, SmGPPS.SSUI, SmGPPS.SSUII.1, and SmGPPS.SSUII.2 encoding heteromeric GPPS subunits), one FPPS gene (SmFPPS), and three GGPPS genes (SmGGPPS1, SmGGPPS2, and SmGGPPS3) (Cui et al., 2011; Kai et al., 2011; Ma et al., 2012, 2015).
GPPS is generally considered to be regarded as the backbone of monoterpene constituents, but recent studies have found that it is also necessary in the biosynthesis pathway of some diterpenoids, such as gibberellins (van Schie et al., 2010). To date, there are still few studies on the five GPPS genes in S. miltiorrhiza. The differences in homology and tissue specificity also make the research more difficult. The monoterpenes and sesquiterpenoids contained in many Salvia species are mainly enriched in the trichomes of the aerial parts. They also have a wide range of pharmacological activities, such as antimicrobial and antioxidant activities. There is still a need to further identify and characterize the SmFPPS and SmGPPS genes to explore the roles they played in the biosynthesis of essential oils in S. miltiorrhiza (Ma et al., 2012).
GGPPS catalyzes the condensation reaction between FPP and IPP to form GGPP. GGPP is a key precursor of biosynthesis of diterpenoids, chlorophylls, and carotenoids. S. miltiorrhiza contains three GGPPS genes. The full-length cDNA of SmGGPPS1 has been isolated by rapid amplification of cDNA ends (RACE). Tissue expression pattern analysis showed that SmGGPPS1 expression was higher in leaves and roots than stems. Functional identification experiments showed that SmGGPPS1 can catalyze the production of carotenoids, and the introduction of SmGGPPS1 gene into the transgenic hairy roots could significantly increase the content of tanshinones. So far, there are few studies on SmGGPPS2 and SmGGPPS3, the functions of the two genes remain to be elucidated (Zhang L. et al., 2009; Kai et al., 2010; Hua et al., 2012; Ma et al., 2012; Yan et al., 2014).
Comparing the structures of GGPP and tanshinone compounds, the differences can be clearly observed and GGPP needs to undergo a cyclization to form tanshinones. The key enzymes involved in the process from GGPP to the production of miltiradiene are labdadienyl/copalyl diphosphate synthases (CPS) and kaurene synthase-like (KSL), which are class II diterpene cyclases. There are twelve SmCPS and nine SmKSL homologs reported in S. miltiorrhiza and based on the genome sequences (Ma et al., 2012), seven cDNA sequences encoding TPSs (including SmCPS1 to SmCPS5, SmKSL1, and SmKSL2) have been cloned. Previous work has indicated that SmCPS1 and SmKSL1 participate in the tanshinone biosynthesis (Gao et al., 2009) and using the modular path engineering (MOPE) strategy, SmCPS, SmKSL and other related genes construction fusion proteins were expressed in the optimized yeast strain and finally the yield of miltiradiene in the 15 L bioreactor reached 365 mg/L (Zhou et al., 2012). Combining SmCPS2 and SmKSL1 expressed proteins and feeding GGPP as substrate also lead to production of miltiradiene. Phylogenetic analysis indicates that SmCPS1 to SmCPS3 are closely related to CPSs involved in the biosynthesis of CPP, while SmCPS4 and SmCPS5 are closely related to the CPSs generally for gibberellin biosynthesis (Ma et al., 2012; Cheng Q. et al., 2013; Cui et al., 2015). Compared with SmKSL2, SmKSL1 has experienced a loss of the N-terminal g-domain, which indicates that SmKSL1 involved in a more specific diterpenoid metabolic process. Phylogenetic analysis also indicated SmKSL2 clusters with other dicotyledonous KSL genes, many of which have been identified to be involved in GA biosynthesis (Gao et al., 2009; Ma et al., 2012; Cui et al., 2015).
Under the action of TPS, GGPP undergoes cyclization reactions to form miltiradiene, which needs to be further modified to produce tanshinones. These modification reactions include decarboxylation, oxidation and reductions, and each step can be catalyzed by the corresponding specific enzyme.
Cytochromes P450 is an important mixed-function heme oxidoreductase in animals, plants and microorganisms, and is a gene superfamily (Ghosh, 2017). It can catalyze a variety of chemical reactions and plays an important role in protecting organisms from external aggression and in plant secondary metabolism (David and Danièle, 2011). CYP450s often form multi-enzyme complexes with other enzymes on the endoplasmic reticulum to act on the biosynthetic pathway of phenylpropanoid. With the development of modern sequencing technology and the analysis of plant genomes, more and more P450 genes involved in plant secondary metabolic activities have been discovered (Chen et al., 2014). The transcripts of the induction process of S. miltiorrhiza hair roots were analyzed to reveal 125 CYPs expressed therein (Gao et al., 2014). Guo et al. used a next-generation sequencing approach to identify six candidate CYP genes being related with the biosynthesis of tanshinones in both the rhizome and Danshen hairy roots. Among these six genes, they identified SmCYP76AH1 could catalyze the conversion of miltiradiene to ferruginol in vivo and in vitro, making the biosynthesis pathway of tanshinone a critical step. And the ferruginol content reached 10.5 mg/L by heterologous expression of SmCYP76AH1 and phyto-CYP reductase genes in yeast (Guo et al., 2013). With further research, they found other two SmCYPs, respectively, named CYP76AH3 and CYP76AK1, exhibiting similar transcription profiles as SmCYP76AH1 in elicited S. miltiorrhiza hairy roots (Guo et al., 2016). SmCYP76AH3 oxidizes ferruginol at two different carbon centers to obtain 11-hydroxy ferruginol and 11-hydroxy sugiol. And then SmCYP76AK1 could hydroxylate C-20 of two of the resulting intermediates. Together, sequential cooperation between two CYPs convert ferruginol into 11, 20-dihydroxy ferruginol and 11, 20-dihydroxy sugiol which may be a bifurcating pathway for the biosynthesis of tanshinones. After analyzing the structure of ferruginol and tanshinones, a putative biosynthetic pathway can be inferred, that is, ferruginol can be dehydrogenated to form cryptotanshinone, and then cryptotanshinone can be reduced by reductases to form tanshinone IIA (Cai et al., 2013; Guo et al., 2016).
Two full length cytochrome P450 reductase (CPR) genes have also been cloned from S. miltiorrhiza named SmCPR1 and SmCPR2. Although there are relatively few studies on these two genes, they have a great role in supporting the CYP450 genes to play the catalytic functions (Schenkman and Ingela, 2003). The experiment shows that when importing SmCYP76AH1 alone into the miltiradiene-producing yeast strain failed to produce ferruginol, in contrast yeast strains with plant CPRs after introducing SmCYP76AH1 could produce ferruginol (Zhou et al., 2015).
The biosynthetic pathway from ferruginol to tanshinones remains to be further resolved. The discovery and comparison of structural features of intermediates that may occur in the tanshinone biosynthesis pathway represent a more effective way to find related functional enzymes, excavate functional genes, and analyze the complete pathway. Currently, the field of gene sequencing is full of unknowns and expectations. The development and application of fourth-generation gene sequencing technology will definitely bring brighter prospects to the fields of scientific research and medicine.
Synthetic Biology of Tanshinones
In recent years, the discovery of key genes for the biosynthesis of medicinal active ingredients and the use of synthetic biology strategies to design and engineer microbial strains to produce natural products have been considered a promising method for resource acquisition (Gao W. et al., 2015). Miltiradiene is a key precursor for the biosynthesis of tanshinones. At present, Saccharomyces cerevisiae is used as the chassis cell, the engineered strains with a high yield of miltiradiene were constructed by designing functional modules; the yield could reach 488 mg/L (Gao et al., 2009; Zhou et al., 2012). The cloning of the first modified enzyme gene CYP76AH1 downstream of the tanshinone biosynthesis pathway successfully transformed miltiradiene into ferruginol, which is stable and biologically active. The highest yield of ferruginol reached 10.5 mg/L under shake flask fermentation conditions (Guo et al., 2016).
Increasingly more scholars have focused on not only functional genes in the biosynthetic pathway but also the effects of elicitors and regulatory genes on secondary metabolites in plants. Elicitors can directly or indirectly prompt the accumulation of plant secondary metabolites in plant tissues, cell culture and hairy root culture. According to their sources, these elicitors can be divided into biological inducers and abiotic inducers. Biological elicitors such as Trichoderma atroviride (Ming et al., 2013), Chaetomium globosum D38 (Zhai et al., 2017), Alternaria sp. A13 (Zhou et al., 2018) and other endophytic fungi, as well as some non-biological inducers such as MeJA, salicylic acid, yeast extract, Ag+ (Xing et al., 2014), and ultraviolet-B (Wang et al., 2016), could induce the accumulation of active ingredients in S. miltiorrhiza.
Conclusion and Prospects
As a perennial cross-pollinating plant species, S. miltiorrhiza is strongly adaptive and widely distributed with generations having overlapping features and significant genetic diversity. Since ancient times, the single-component Danshen or its combination with other drugs has been used in the clinical treatment of various diseases exhibiting good effects. S. miltiorrhiza contains a variety of active ingredients, among which tanshinones have exhibited significant pharmacological properties, such as antibacterial, antioxidant, anti-inflammatory, and anti-tumor activities. In this paper, the extraction and purification methods of tanshinones are briefly introduced. Many new methods and new technologies have been applied, and it is worth mentioning that the combination of different methods can achieve high efficiency and low consumption and may have promising development prospects.
The traditional method of obtaining tanshinones has been unable to meet the clinical drug needs. In recent years by continuously investigating the functional genes in the tanshinone biosynthesis pathway, analyzing the complete pathway and reconstituting the biosynthetic pathway in heterologous hosts, the method of efficiently and heterologously producing tanshinones has become the focus of attention. To date, studies have shown that miltiradiene is an intermediate for the biosynthesis of tanshinones, and that it can be converted to tanshinones under the action of various modified enzymes. Therefore, more functional genes must be further discovered. Synthetic biology strategies and methods not only provide ideas for more efficient and convenient access to tanshinones, but also lay the foundation for the sustainable use of traditional Chinese medicine resources.
Author Contributions
ZJ, WG, and LH contributed the conception and design of the study. ZJ organized the database and wrote the first draft of the manuscript. WG revised parts of the manuscript. All authors contributed to manuscript revision, read and approved the submitted version.
Funding
This work was supported by Support Project of High-level Teachers in Beijing Municipal Universities in the Period of 13th Five-year Plan (CIT&TCD20170324), the Key Project at central government level: The ability establishment of sustainable use for valuable Chinese medicine resources (No. 2060302) and National Program for Special Support of Eminent Professionals.
Conflict of Interest Statement
The authors declare that the research was conducted in the absence of any commercial or financial relationships that could be construed as a potential conflict of interest.
References
Ahmed, T., Gilani, A. U. H., Abdollahi, M., Daglia, M., Nabavi, S. F., and Nabavi, S. M. (2015). Berberine and neurodegeneration: a review of literature. Pharmacol. Rep. 67, 970–979. doi: 10.1016/j.pharep.2015.03.002
Bi, H. C., Zuo, Z., Chen, X., Xu, C. S., Wen, Y. Y., Sun, H. Y., et al. (2008). Preclinical factors affecting the pharmacokinetic behaviour of tanshinone IIA, an investigational new drug isolated from Salvia miltiorrhiza for the treatment of ischaemic heart diseases. Xenobiotica 38, 185–222. doi: 10.1080/00498250701767675
Bi, W., Tian, M., and Row, K. H. (2011). Extraction and concentration of tanshinones in Salvia miltiorrhiza Bunge by task-specific non-ionic surfactant assistance. Food Chem. 126, 1985–1990. doi: 10.1016/j.foodchem.2010.12.059
Cai, Y., Guo, J., Zhou, Y., Zhu, Z., Wu, W., Huang, L., et al. (2013). Construction of Saccharomyces cerevisiae whole-cell biocatalyst system for conversion miltiradiene. Acta Pharm. Sin. 48, 1618–1623.
Cao, E. H., Liu, X. Q., Wang, J. J., and Xu, N. F. (1996). Effect of natural antioxidant tanshinone II-A on DNA damage by lipid peroxidation in liver cells. Free Radic. Biol. Med. 20, 801–806. doi: 10.1016/0891-5849(95)02211-2
Chen, H., Wu, B., Nelson, D. R., Wu, K., and Liu, C. (2014). Computational identification and systematic classification of novel cytochrome P450 genes in Salvia miltiorrhiza. PLoS One 9:e115149. doi: 10.1371/journal.pone.0115149
Chen, W., and Chen, G. (2017). Danshen (Salvia miltiorrhiza Bunge): a prospective healing sage for cardiovascular diseases. Curr. Pharm. Des. 23, 5125–5135. doi: 10.2174/1381612823666170822101112
Chen, Y. L., Duan, G. L., Xie, M. F., Chen, B., and Li, Y. (2010). Infrared-assisted extraction coupled with high-performance liquid chromatography for simultaneous determination of eight active compounds in Radix Salviae miltiorrhizae. J. Sep. Sci. 33, 2888–2897. doi: 10.1002/jssc.201000234
Cheng, A. X., Lou, Y. G., Mao, Y. B., Lu, S., Wang, L. J., and Chen, X. Y. (2007). Plant terpenoids: biosynthesis and ecological functions. J. Integr. Plant Biol. 49, 179–186. doi: 10.1111/j.1744-7909.2007.00395.x
Cheng, Q., He, Y., Li, G., Liu, Y., Gao, W., and Huang, L. (2013). Effects of combined elicitors on tanshinone metabolic profiling and SmCPS expression in Salvia miltiorrhiza hairy root cultures. Molecules 18, 7473–7485. doi: 10.3390/molecules18077473
Cheng, Q. Q., He, Y. F., Li, G., Jiang, C., Yuan, Y., Gao, W., et al. (2013). Cloning and induced expression analysis of 4-hydroxy-3-methyl-but-2-enyl diphosphate reductase gene (SmHDR) of Salvia miltiorrhiza. Acta Pharm. Sin. 48, 236–242.
Chiu, T. L., and Su, C. C. (2010). Tanshinone IIA induces apoptosis in human lung cancer A549 cells through the induction of reactive oxygen species and decreasing the mitochondrial membrane potential. Int. J. Mol. Med. 25,231–236.
Cui, G., Duan, L., Jin, B., Qian, J., Xue, Z., Shen, G., et al. (2015). Functional divergence of diterpene syntheses in the medicinal plant Salvia miltiorrhiza. Plant Physiol. 169, 1607–1618. doi: 10.1104/pp.15.00695
Cui, G., Huang, L., Tang, X., and Zhao, J. (2011). Candidate genes involved in tanshinone biosynthesis in hairy roots of Salvia miltiorrhiza revealed by cDNA microarray. Mol. Biol. Rep. 38, 2471–2478. doi: 10.1007/s11033-010-0383-9
Cui, G. H., Wang, X. Y., Feng, H., Zhao, J. X., and Huang, L. Q. (2010). Molecular cloning and SNP analysis of a acetyl-CoA C-acetyltransferase gene (SmAACT) from Salvia miltiorrhiza. Acta Pharm. Sin. 45, 785–790.
Dai, Z., Cui, G., Zhou, S. F., Zhang, X., and Huang, L. (2011). Cloning and characterization of a novel 3-hydroxy-3-methylglutaryl coenzyme A reductase gene from Salvia miltiorrhiza involved in diterpenoid tanshinone accumulation. J. Plant Physiol. 168, 148–157. doi: 10.1016/j.jplph.2010.06.008
David, N., and Danièle, W. R. (2011). A P450-centric view of plant evolution. Plant J. 66, 194–211. doi: 10.1111/j.1365-313X.2011.04529.x
Fan, G. W., Gao, X. M., Wang, H., Zhu, Y., Zhang, J., Hu, L. M., et al. (2009). The anti-inflammatory activities of Tanshinone IIA, an active component of TCM, are mediated by estrogen receptor activation and inhibition of iNOS. J. Steroid Biochem. 113, 275–280. doi: 10.1016/j.jsbmb.2009.01.011
Gao, S., Liu, Z., Li, H., Little, P. J., Liu, P., and Xu, S. (2012). Cardiovascular actions and therapeutic potential of tanshinone IIA. Atherosclerosis 220, 3–10. doi: 10.1016/j.atherosclerosis.2011.06.041
Gao, W., Cheng, Q. Q., Ma, X. H., He, Y. F., Jiang, C., Yuan, Y., et al. (2012). Cloning and bioinformatics analysis of 2-c-methyl-D-erythritol 2,4-cyclodiphosphate synthase gene in Salvia miltiorrhiza. China J. Chin. Mater. Med. 37, 3365–3370.
Gao, S., Yu, W., Yang, X., Liu, Z., Jia, Y., and Zhang, H. (2015). On-line ionic liquid-based dynamic microwave-assisted extraction-high performance liquid chromatography for the determination of lipophilic constituents in root of Salvia miltiorrhiza Bunge. J. Sep. Sci. 35, 2813–2821. doi: 10.1002/jssc.201200267
Gao, W., Hu, T. Y., Guo, J., Lv, D. M., Dai, Z. B., Zhou, Y. J., et al. (2015). Research progress of synthetic biology for tanshinones. China J. Chin. Mater. Med. 40, 2486–2491.
Gao, W., Hillwig, M. L., Huang, L., Cui, G., Wang, X., Kong, J., et al. (2009). A functional genomics approach to tanshinone biosynthesis provides stereochemical insights. Org. Lett. 11, 5170–5173. doi: 10.1021/ol902051v
Gao, W., Sun, H. X., Xiao, H., Cui, G., Hillwig, M. L., Jackson, A., et al. (2014). Combining metabolomics and transcriptomics to characterize tanshinone biosynthesis in Salvia miltiorrhiza. BMC Genomics 15:73. doi: 10.1186/1471-2164-15-73
Ghosh, S. (2017). Triterpene structural diversification by plant cytochrome P450 enzymes. Front. Plant Sci. 8:1886. doi: 10.3389/fpls.2017.01886
Gong, Y., Li, Y., Lu, Y., Li, L., Abdolmaleky, H., Blackburn, G. L., et al. (2011). Bioactive tanshinones in Salvia miltiorrhiza inhibit the growth of prostate cancer cells in vitro and in mice. Int. J. Cancer 129, 1042–1052. doi: 10.1002/ijc.25678
Gong, Z., Huang, C., Sheng, X., Zhang, Y., Li, Q., Wang, M. W., et al. (2009). The role of tanshinone IIA in the treatment of obesity through peroxisome proliferator-activated receptor gamma antagonism. Endocrinology 150, 104–113. doi: 10.1210/en.2008-0322
Guo, J., Ma, X., Cai, Y., Ma, Y., Zhan, Z., Zhou, Y. J., et al. (2016). Cytochrome P450 promiscuity leads to a bifurcating biosynthetic pathway for tanshinones. New Phytol. 210, 525–534. doi: 10.1111/nph.13790
Guo, J., Zhou, Y. J., Hillwig, M. L., Shen, Y., Yang, L., Wang, Y., et al. (2013). CYP76AH1 catalyzes turnover of miltiradiene in tanshinones biosynthesis and enables heterologous production of ferruginol in yeasts. Proc. Natl. Acad. Sci. U.S.A. 110, 12108–12113. doi: 10.1073/pnas.1218061110
Han, K. Y., Yang, D., Chang, E. J., Lee, Y., Huang, H., Sang, H. S., et al. (2007). Inhibition of osteoclast differentiation and bone resorption by sauchinone. Biochem. Pharmacol. 74, 911–923. doi: 10.1016/j.bcp.2007.06.044
Hao, G., Shi, R., Tao, R., Fang, Q., Jiang, X., Ji, H., et al. (2013). Cloning, molecular characterization and functional analysis of 1-hydroxy-2-methyl-2-(E)-butenyl-4-diphosphate reductase (HDR) gene for diterpenoid tanshinone biosynthesis in Salvia miltiorrhiza Bge. f. alba. Plant Physiol. Biochem. 70, 21–32. doi: 10.1016/j.plaphy.2013.05.010
Hasan, M. M., Kim, H. S., Jeon, J. H., Kim, S. H., Moon, B. K., Song, J. Y., et al. (2014). Metabolic engineering of Nicotiana benthamiana for the increased production of taxadiene. Plant Cell Rep. 33, 895–904. doi: 10.1007/s00299-014-1568-9
Honda, G., Koezuka, Y., and Tabata, M. (1988). Isolation of an antidermatophytic substance from the root of Salvia miltiorrhiza. Chem. Pharm. Bull. 36, 408–411. doi: 10.1248/cpb.36.408
Houlihan, C. M., Ho, C. T., and Chang, S. S. (1985). The structure of rosmariquinone — a new antioxidant isolated from Rosmarinus officinalis L. J. Am. Oil Chem. Soc. 62, 96–98. doi: 10.1007/BF02541500
Hua, W., Song, J., Li, C., and Wang, Z. (2012). Molecular cloning and characterization of the promoter of SmGGPPs and its expression pattern in Salvia miltiorrhiza. Mol. Biol. Rep. 39, 5775–5783. doi: 10.1007/s11033-011-1388-8
Huang, L., Zhu, J., Zheng, M., Zou, R., Zhou, Y., and Zhu, M. (2018). Tanshinone IIA protects against subclinical lipopolysaccharide induced cardiac fibrosis in mice through inhibition of NADPH oxidase. Int. Immunopharmacol. 60, 59–63. doi: 10.1016/j.intimp.2018.04.036
Huang, L. Q., Gao, W., and Zhou, Y. J. (2014). Application of synthetic biology to sustainable utilization of Chinese materia medica resources. Acta Pharm. Sin. 49, 37–43.
Javed, S., Tariq, A., Ahmed, T., Budzyńska, B., Tejada, S., Daglia, M., et al. (2016). Tanshinones and mental diseases: from chemistry to medicine. Rev. Neurosci. 27, 777–791. doi: 10.1515/revneuro-2016-0012
Jiang, D., Rong, Q. X., Yuan, Q. J., Zhang, W. J., Zhang, Y. Q., and Huang, L. Q. (2014). Cloning and prokaryotic expression analysis of HDS from Salvia miltiorrhiza bge.f.alba. Acta Pharm. Sin. 49, 1614–1620.
Jiang, P., Li, C., Xiang, Z., and Jiao, B. (2014). Tanshinone IIA reduces the risk of Alzheimer’s disease by inhibiting iNOS, MMP-2 and NF-κBp65 transcription and translation in the temporal lobes of rat models of Alzheimer’s disease. Mol. Med. Rep. 10, 689–694. doi: 10.3892/mmr.2014.2254
Jing, X., Wei, X., Ren, M., Wang, L., Zhang, X., and Lou, H. (2016). Neuroprotective effects of tanshinone I against 6-OHDA-induced oxidative stress in cellular and mouse model of Parkinson’s disease through upregulating Nrf2. Neurochem. Res. 41, 779–786. doi: 10.1007/s11064-015-1751-6
Kai, G., Liao, P., Zhang, T., Zhou, W., Wang, J., Xu, H., et al. (2010). Characterization, expression profiling, and functional identification of a gene encoding geranylgeranyl diphosphate synthase from Salvia miltiorrhiza. Biotechnol. Bioprocess Eng. 15, 236–245. doi: 10.1007/s12257-009-0123-y
Kai, G., Xu, H., Zhou, C., Liao, P., Xiao, J., Luo, X., et al. (2011). Metabolic engineering tanshinone biosynthetic pathway in Salvia miltiorrhiza hairy root cultures. Metab. Eng. 13, 319–327. doi: 10.1016/j.ymben.2011.02.003
Kakisawa, H., Hayashi, T., Okazaki, I., and Ohashi, M. (1968). Isolation and structures of new tanshinones. Tetrahedron Lett. 9, 3231–3234. doi: 10.1016/S0040-4039(00)89532-5
Kakisawa, H., Hayashi, T., and Yamazaki, T. (1969). Structures of isotanshinones. Tetrahedron Lett. 10, 301–304. doi: 10.1016/S0040-4039(01)87676-0
Ke, Q. Z., Bao, Y., Ping, W., Rosen, R. T., and Chi, T. H. (1990). Antioxidative components of tanshen (Salvia miltiorhiza Bung). J. Agric. Food Chem. 38, 1194–1197. doi: 10.1021/jf00095a007
Krivoruchko, A., and Nielsen, J. (2015). Production of natural products through metabolic engineering of Saccharomyces cerevisiae. Curr. Opin. Biotechnol. 35, 7–15. doi: 10.1016/j.copbio.2014.12.004
Kyoko, T., Xinshou, O., Katsuko, K., Norio, N., Masao, H., Akemichi, B., et al. (2002). Sodium tanshinone IIA sulfonate derived from Danshen (Salvia miltiorrhiza) attenuates hypertrophy induced by angiotensin II in cultured neonatal rat cardiac cells. Biochem. Pharmacol. 64, 745–750. doi: 10.1016/S0006-2952(02)01250-9
Le, Y., Zou, X., Gao, X., Chen, H., Luo, J., Wang, Z., et al. (2009). Sodium tanshinone IIA sulfonate attenuates angiotensin II-induced collagen type I expression in cardiac fibroblasts in vitro. Exp. Mol. Med. 41, 508–516. doi: 10.3858/emm.2009.41.7.056
Lee, D. S., Lee, S. H., Noh, J. G., and Hong, S. D. (1999). Antibacterial activities of cryptotanshinone and dihydrotanshinone I from a medicinal herb, Salvia miltiorrhiza Bunge. Biosci. Biotechnol. Biochem. 63, 2236–2239. doi: 10.1271/bbb.63.2236
Li, P., Li, S. P., Yang, F. Q., and Wang, Y. T. (2007). Simultaneous determination of four tanshinones in Salvia miltiorrhiza by pressurized liquid extraction and capillary electrochromatography. J. Sep. Sci. 30, 900–905. doi: 10.1002/jssc.200600368
Li, X., Zhai, X., Shu, Z., Dong, R., Ming, Q., Qin, L., et al. (2016). Phoma glomerata D14: an endophytic fungus from Salvia miltiorrhiza that produces Salvianolic acid C. Curr. Microbiol. 73, 31–37. doi: 10.1007/s00284-016-1023-y
Li, Z., Zhang, S., Yan, H., and Liu, J. (2018). Development of cryptotanshinone-loaded pellets for angina chronotherapy: in virto/in vivo prediction and evaluation. Asian J. Pharm. Sci. 13, 310–316. doi: 10.1016/j.ajps.2018.02.009
Lin, Z., Yan, X., Jing, W., Li, S., Pan, L., and Kai, G. (2011). Molecular cloning and expression analysis of a new putative gene encoding 3-hydroxy-3-methylglutaryl-CoA synthase from Salvia miltiorrhiza. Acta Physiol. Plant. 33, 953–961. doi: 10.1007/s11738-010-0627-2
Liu, F., Wang, D., Liu, W., Wang, X., Bai, A., and Huang, L. (2013). Ionic liquid-based ultrahigh pressure extraction of five tanshinones from Salvia miltiorrhiza Bunge. Sep. Purif. Technol. 110, 86–92. doi: 10.1016/j.seppur.2013.03.012
Liu, J., Shen, H. M., and Ong, C. N. (2000). Salvia miltiorrhiza inhibits cell growth and induces apoptosis in human hepatoma HepG 2 cells. Cancer Lett. 153, 85–93. doi: 10.1016/S0304-3835(00)00391-8
Liu, Y., Sun, G., Zhong, Z., Ji, L., Zhang, Y., Zhou, J., et al. (2017). Overexpression of AtEDT1 promotes root elongation and affects medicinal secondary metabolite biosynthesis in roots of transgenic Salvia miltiorrhiza. Protoplasma 254, 1–9. doi: 10.1007/s00709-016-1045-0
Liu, Z., Wang, J., Huang, E., Gao, S., Li, H., Lu, J., et al. (2014). Tanshinone IIA suppresses cholesterol accumulation in human macrophages: role of heme oxygenase-1. J. Lipid Res. 55, 201–213. doi: 10.1194/jlr.M040394
Luo, Y., Li, B. Z., Liu, D., Zhang, L., Chen, Y., Jia, B., et al. (2015). Engineered biosynthesis of natural products in heterologous hosts. Chem. Soc. Rev. 44, 5265–5290. doi: 10.1039/c5cs00025d
Ma, X. H., Ma, Y., Tang, J. F., He, Y. L., Liu, Y. C., Ma, X. J., et al. (2015). The biosynthetic pathways of tanshinones and phenolic acids in Salvia miltiorrhiza. Molecules 20, 16235–16254. doi: 10.3390/molecules200916235
Ma, Y., Yuan, L., Wu, B., Li, X., Chen, S., and Lu, S. (2012). Genome-wide identification and characterization of novel genes involved in terpenoid biosynthesis in Salvia miltiorrhiza. J. Exp. Bot. 63, 2809–2823. doi: 10.1093/jxb/err466
Ming, Q., Su, C., Zheng, C., Jia, M., Zhang, Q., Zhang, H., et al. (2013). Elicitors from the endophytic fungus Trichoderma atroviride promote Salvia miltiorrhiza hairy root growth and tanshinone biosynthesis. J. Exp. Bot. 64, 5687–5694. doi: 10.1093/jxb/ert342
Niu, X. L., Ichimori, K., Yang, X., Hirota, Y., Hoshiai, K., Li, M., et al. (2000). Tanshinone II-A inhibits low density lipoprotein oxidation in vitro. Free Radic. Res. 33, 305–312. doi: 10.1080/10715760000301471
Ong, E., and Len, S. (2004). Evaluation of pressurized liquid extraction and pressurized hot water extraction for tanshinone I and IIA in Salvia miltiorrhiza using LC and LC-ESI-MS. J. Chromatogr. Sci. 42, 211–216. doi: 10.1093/chromsci/42.4.211
Orhan, I. E., Daglia, M., Nabavi, S. F., Loizzo, M. R., Sobarzo-Sánchez, E., and Nabavi, S. M. (2015). Flavonoids and dementia: an update. Curr. Med. Chem. 22, 1004–1015. doi: 10.2174/0929867322666141212122352
Pan, L., Zhou, W., Zhang, L., Wang, J., Yan, X., Zhang, Y., et al. (2009). Molecular cloning, characterization and expression analysis of a new gene encoding 3-hydroxy-3-methylglutaryl coenzyme A reductase from Salvia miltiorrhiza. Acta Physiol. Plant. 31, 565–572. doi: 10.1016/j.jplph.2010.06.008
Pan, X., Niu, G., and Liu, H. (2001). Microwave-assisted extraction of tanshinones from Salvia miltiorrhiza Bunge with analysis by high-performance liquid chromatography. J. Chromatogr. A 922, 371–375. doi: 10.1016/S0021-9673(01)00949-9
Pan, X., Niu, G., and Liu, H. (2002). Comparison of microwave-assisted extraction and conventional extraction techniques for the extraction of tanshinones from Salvia miltiorrhiza Bunge. Biochem. Eng. J. 12, 71–77. doi: 10.1016/S1369-703X(02)00039-6
Pang, H., Wu, L., Tang, Y., Zhou, G., Qu, C., and Duan, J. A. (2016). Chemical analysis of the herbal medicine Salviae miltiorrhizae radix et Rhizoma (Danshen). Molecules 21:51. doi: 10.3390/molecules21010051
Park, O. K., Choi, J. H., Park, J. H., Kim, I. H., Yan, B. C., Ahn, J. H., et al. (2012). Comparison of neuroprotective effects of five major lipophilic diterpenoids from Danshen extract against experimentally induced transient cerebral ischemic damage. Fitoterapia 83, 1666–1674. doi: 10.1016/j.fitote.2012.09.020
Peng, L., Ru, M., Wang, K., Li, B., Wang, Y., Xia, P., et al. (2014). Spaceflight environment-induced variation in root yield and active constituents of Salvia miltiorrhiza. Planta Med. 80, 1029–1035. doi: 10.1055/s-0034-1382908
Pickens, L. B., Tang, Y., and Chooi, Y. H. (2011). Metabolic engineering for the production of natural products. Annu. Rev. Chem. Biomol. 2, 211–236. doi: 10.1146/annurev-chembioeng-061010-114209
Prince, M., Bryce, R., Albanese, E., Wimo, A., Ribeiro, W., and Ferri, C. P. (2013). The global prevalence of dementia: a systematic review and meta analysis. Alzheimers Dement. 9, 63–75. doi: 10.1016/j.jalz.2012.11.007
Qiao, Y., Zhang, X. L., Li, X. Y., Tang, W. K., Zhang, J. X., Fang, C. X., et al. (2007). Coupling continuous ultrasound-assisted extraction with ultrasonic probe, solid-phase extraction and high-performance liquid chromatography for the determination of sodium Danshensu and four tanshinones in Salvia miltiorrhiza Bunge. Anal. Chim. Acta 589, 231–238. doi: 10.1016/j.aca.2007.03.005
Ren, Y., Houghton, P. J., Hider, R. C., and Howes, M. J. (2004). Novel diterpenoid acetylcholinesterase inhibitors from Salvia miltiorhiza. Planta Med. 70, 201–204. doi: 10.1055/s-2004-815535
Schenkman, J. B., and Ingela, J. (2003). The many roles of cytochrome b5. Pharmacol. Therapeut. 97, 139–152. doi: 10.1016/S0163-7258(02)00327-3
Shi, M., Huang, F., Deng, C., Wang, Y., and Kai, G. (2018). Bioactivities, biosynthesis and biotechnological production of phenolic acids in Salvia miltiorrhiza. Crit. Rev. Food Sci. doi: 10.1080/10408398.2018.1474170 [Epub ahead of print].
Shi, Y. R., Chong, O. L., and Sang, U. C. (1997). In vitro cytotoxicity of tanshinones from Salvia miltiorrhiza. Planta Med. 63, 339–342. doi: 10.1055/s-2006-957696
Su, C., Ming, Q. L., Rahman, K., Han, T., and Qin, L. (2015). Salvia miltiorrhiza: traditional medicinal uses, chemistry, and pharmacology. Chin. J. Nat. Med. 13, 163–182. doi: 10.1016/S1875-5364(15)30002-9
Sun, A., Zhang, Y., Li, A., Meng, Z., and Liu, R. (2011). Extraction and preparative purification of tanshinones from Salvia miltiorrhiza Bunge by high-speed counter-current chromatography. J. Chromatogr. B 879, 1899–1904. doi: 10.1016/j.jchromb.2011.05.014
Tang, F., Wu, X., Wang, T., Wang, P., Li, R., Zhang, H., et al. (2007). Tanshinone II A attenuates atherosclerotic calcification in rat model by inhibition of oxidative stress. Vasc. Pharmacol. 46, 427–438. doi: 10.1016/j.vph.2007.01.001
Tao, Z., Eun, P. H., and Ho, R. K. (2013). Ultrasonic-assisted extraction of tanshinones from Korean red ginseng by using amino-modified monolithic cartridge. Asian J. Chem. 25, 7765–7768. doi: 10.14233/ajchem.2013.14597
Tian, G., Zhang, T., Zhang, Y., and Ito, Y. (2002). Separation of tanshinones from Salvia miltiorrhiza Bunge by multidimensional counter-current chromatography. J. Chromatogr. A 945, 281–285. doi: 10.1016/S0021-9673(01)01495-9
van Schie, C. C. N., Ament, K., Axel, S., Theo, L., Haring, M. A., and Schuurink, R. C. (2010). Geranyl diphosphate synthase is required for biosynthesis of gibberellins. Plant J. 52, 752–762. doi: 10.1111/j.1365-313X.2007.03273.x
Wang, B., Wang, H., Wang, E., Yan, W., Ye, W., and Li, P. (2012). Isolation and structure characterization of related impurities in Sodium Tanshinone IIA Sulfonate by LC/ESI-MS(n) and NMR. J. Pharmaceut. Biomed. 67-68, 36–41. doi: 10.1016/j.jpba.2012.04.029
Wang, C. H., Zheng, L. P., Tian, H., and Wang, J. W. (2016). Synergistic effects of ultraviolet-B and methyl jasmonate on tanshinone biosynthesis in Salvia miltiorrhiza hairy roots. J. Photochem. Photobiol. B 159, 93–100. doi: 10.1016/j.jphotobiol.2016.01.012
Wang, L., Song, Y., Cheng, Y., and Liu, X. (2015). Orthogonal array design for the optimization of supercritical fluid extraction of tanshinones from Danshen. J. Sep. Sci. 31, 321–328. doi: 10.1002/jssc.200700386
Wang, P., Wu, X., Bao, Y., Fang, J., Zhou, S., Gao, J., et al. (2011). Tanshinone IIA prevents cardiac remodeling through attenuating NAD (P)H oxidase-derived reactive oxygen species production in hypertensive rats. Pharmazie 66, 517–524.
Wang, S., Jing, H., Yang, H., Liu, Z., Guo, H., Chai, L., et al. (2015). Tanshinone I selectively suppresses pro-inflammatory genes expression in activated microglia and prevents nigrostriatal dopaminergic neurodegeneration in a mouse model of Parkinson×s disease. J. Ethnopharmacol. 164, 247–255. doi: 10.1016/j.jep.2015.01.042
Wang, Y., Li, J. X., Wang, Y. Q., and Miao, Z. H. (2015). Tanshinone I inhibits tumor angiogenesis by reducing STAT3 phosphorylation at TYR705 and hypoxia-induced HIF-1α accumulation in both endothelial and tumor cells. Oncotarget 6, 16031–16042. doi: 10.18632/oncotarget.3648
Wang, X., Bastow, K. F., Sun, C. M., Lin, Y. L., Yu, H. J., Don, M. J., et al. (2004). Antitumor agents. 239. isolation, structure elucidation, total synthesis, and anti-breast cancer activity of neo-tanshinlactone from Salvia miltiorrhiza. J. Med. Chem. 47, 5816–5819. doi: 10.1021/jm040112r
Wang, X. Y., Cui, G. H., Huang, L. Q., Gao, W., and Yuan, Y. (2008). A full length cDNA of 4-(cytidine 5’-diphospho)-2-C-methyl-D-erythritol kinase cloning and analysis of introduced gene expression in Salvia miltiorrhiza. Acta Pharm. Sin. 43, 1251–1257.
Wei, T., Deng, K., Wang, H., Zhang, L., Wang, C., Song, W., et al. (2018). Comparative transcriptome analyses reveal potential mechanisms of enhanced drought tolerance in transgenic Salvia Miltiorrhiza plants expressing AtDREB1A from Arabidopsis. Int. J. Mol. Sci. 19, 827–827. doi: 10.3390/ijms19030827
Wei, Y., Xu, M., Ren, Y., Lu, G., Xu, Y., Song, Y., et al. (2016). The cardioprotection of dihydrotanshinone I against myocardial ischemia-reperfusion injury via inhibition of arachidonic acid omega-hydroxylase. Can. J. Physiol. Pharm. 94, 1267–1275. doi: 10.1139/cjpp-2016-0036
Wei, Z., Huang, F., Shen, L., Yang, W., Zhou, C., Min, S., et al. (2016). Molecular cloning and characterization of two 1-deoxy- d -xylulose-5-phosphate synthase genes involved in tanshinone biosynthesis in Salvia miltiorrhiza. Mol. Breed. 36, 1–12.
Weng, X. C., and Gordon, M. H. (1992). Antioxidant activity of quinones extracted from tanshen (Salvia miltiorrhiza Bunge). J. Agric. Food Chem. 40, 1331–1336. doi: 10.1021/jf00020a007
Wu, C., Mulabagal, V., Nalawade, S. M., Chen, C., Yang, T., and Tsay, H. (2003). Isolation and quantitative analysis of cryptotanshinone, an active quinoid diterpene formed in callus of Salvia miltiorrhiza BUNGE. Biol. Pharm. Bull. 26, 845–848. doi: 10.1248/bpb.26.845
Wu, K., Zhang, Q., Liu, Q., Tang, F., Long, Y., and Yao, S. (2015). Ionic liquid surfactant-mediated ultrasonic-assisted extraction coupled to HPLC: application to analysis of tanshinones in Salvia miltiorrhiza bunge. J. Sep. Sci. 32, 4220–4226. doi: 10.1002/jssc.200900398
Wu, L., Lin, X., and Sun, H. (2012). Tanshinone IIA protects rabbits against LPS-induced disseminated intravascular coagulation (DIC). Acta Pharmacol. Sin. 33, 1254–1259. doi: 10.1038/aps.2012.84
Wu, S., Wu, D., Liang, J., and Berthod, A. (2012). Modeling gradient elution in countercurrent chromatography: efficient separation of tanshinones from Salvia miltiorrhiza Bunge. J. Sep. Sci. 35, 964–976. doi: 10.1002/jssc.201100993
Wu, S. J., Shi, M., and Wu, J. Y. (2011). Cloning and characterization of the 1-deoxy-D-xylulose 5-phosphate reductoisomerase gene for diterpenoid tanshinone biosynthesis in Salvia miltiorrhiza (Chinese sage) hairy roots. Biotechnol. Appl. Biochem. 52, 89–95. doi: 10.1042/BA20080004
Wu, W., Chang, W., and Chen, C. (1991). Cytotoxic activities of tanshinones against human carcinoma cell lines. Am. J. Chin. Med. 19, 207–216. doi: 10.1142/S0192415X91000284
Wu, Y., Ni, Z.-Y., Shi, Q.-W., Dong, M., Kiyota, H., Gu, Y.-C., et al. (2016). Constituents from Salvia species and their biological activities. Chem. Rev. 112, 5967–6026. doi: 10.1021/cr200058f
Xing, B., Yang, D., Guo, W., Liang, Z., Yan, X., Zhu, Y., et al. (2014). Ag+ as a more effective elicitor for production of tanshinones than phenolic acids in Salvia miltiorrhiza hairy roots. Molecules 20, 309–324. doi: 10.3390/molecules20010309
Xu, J., Wei, K., Zhang, G., Lei, L., Yang, D., Wang, W., et al. (2018). Ethnopharmacology, phytochemistry, and pharmacology of Chinese Salvia species: a review. J. Ethnopharmacol. 225, 18–30. doi: 10.1016/j.jep.2018.06.029
Xuan, M., Liang, Q., Hu, P., Ren, K., Wang, Y., and Luo, G. (2010). Selectively modified microfluidic chip for solvent extraction of Radix Salvia Miltiorrhiza using three-phase laminar flow to provide double liquid–liquid interface area. Microfluid. Nanofluid. 9, 365–373. doi: 10.1007/s10404-009-0554-y
Yan, X., Lin, Z., Jing, W., Pan, L., Yan, Z., Ran, Z., et al. (2009). Molecular characterization and expression of 1-deoxy- d -xylulose 5-phosphate reductoisomerase (DXR) gene from Salvia miltiorrhiza. Acta Physiol. Plant. 31, 1015–1022. doi: 10.1007/s11738-009-0320-5
Yan, Y., Zhang, S., Yang, D., Zhang, J., and Liang, Z. (2014). Effects of Streptomyces pactum Act12 on Salvia miltiorrhiza hairy root growth and tanshinone synthesis and its mechanisms. Appl. Biochem. Biotechnol. 173, 883–893. doi: 10.1007/s12010-014-0876-4
Yang, D., Du, X., Liang, X., Han, R., Liang, Z., Liu, Y., et al. (2012). Different roles of the mevalonate and methylerythritol phosphate pathways in cell growth and tanshinone production of Salvia miltiorrhiza hairy roots. PLoS One 7:e46797. doi: 10.1371/journal.pone.0046797
Yang, L., Zhou, X., Liang, Q., Chen, H., Feng, J., Yan, L., et al. (2007). Sodium tanshinone IIA sulfonate depresses angiotensin II-induced cardiomyocyte hypertrophy through MEK/ERK pathway. Exp. Mol. Med. 39, 65–73. doi: 10.1038/emm.2007.8
Yang, P., Jia, Y., Li, J., Zhou, F., and Li, L. (2010). Anti-oxidation of Tanshinone II A and Prohibitin on cardiomyocytes. Chin. Herb. Med. 2, 204–210.
Yang, R., Liu, A., Ma, X., Li, L., Su, D., and Liu, J. (2008). Sodium tanshinone IIA sulfonate protects cardiomyocytes against oxidative stress-mediated apoptosis through inhibiting JNK activation. J. Cardiovasc. Pharm. 51, 396–401. doi: 10.1097/FJC.0b013e3181671439
Yang, Y., Zhou, L., Hua, W. P., and Wang, Z. Z. (2011). Bioinformatics and expression analysis of isopentenyl diphosphate isomerase (SmIPI) from Salvia miltiorrhiza Bunge. Plant Physiol. J. 47, 1086–1090.
Yao, H., Huang, X., Xie, Y., Huang, X., Ruan, Y., Lin, X., et al. (2018). Identification of pharmacokinetic markers for Guanxin Danshen drop pills in rats by combination of pharmacokinetics, systems pharmacology, and pharmacodynamic assays. Front. Pharmacol. 9:1493. doi: 10.3389/fphar.2018.01493
Ye, Y. T., Zhong, W., Sun, P., Wang, D., Wang, C., Hu, L. M., et al. (2017). Apoptosis induced by the methanol extract of Salvia miltiorrhiza Bunge in non–small cell lung cancer through PTEN-mediated inhibition of PI3K/Akt pathway. J. Ethnopharmacol. 200, 107–116. doi: 10.1016/j.jep.2016.12.051
Yuan, S., Wang, X., and Wei, Y. (2003). Anticancer effect of tanshinone and its mechanisms. Chin. J. Cancer 22, 1363–1366.
Zhai, X., Luo, D., Li, X., Han, T., Jia, M., Kong, Z., et al. (2017). Endophyte Chaetomium globosum D38 promotes bioactive constituents accumulation and root production in Salvia miltiorrhiza. Front. Microbiol. 8:2694. doi: 10.3389/fmicb.2017.02694
Zhang, F., Zheng, W., Pi, R., Mei, Z., Bao, Y., Gao, J., et al. (2009). Cryptotanshinone protects primary rat cortical neurons from glutamate-induced neurotoxicity via the activation of the phosphatidylinositol 3-kinase/Akt signaling pathway. Exp. Brain Res. 193, 109–118. doi: 10.1007/s00221-008-1600-9
Zhang, L., Dai, Z., Cui, G., Cheng, Y., Qi, X., and Gao, Z. (2009). Cloning and characterization of geranylgeranyl diphosphate synthase gene of Salvia miltiorrhia. China J. Chin. Mater. Med. 34, 2704–2708.
Zhang, H., Long, M., Wu, Z., Han, X., and Yu, Y. (2014). Sodium tanshinone IIA silate as an add-on therapy in patients with unstable angina pectoris. J. Thorac. Dis. 6, 1794–1799. doi: 10.3978/j.issn.2072-1439.2014.12.37
Zhang, J., Shang, H., Gao, X., Zhang, B., Xiang, Y., Cao, H., et al. (2008). Compound Salvia droplet pill, a traditional Chinese medicine, for the treatment of unstable angina pectoris: a systematic review. Med. Sci. Monit. 14, RA1–RA7.
Zhang, X., Guo, J., Shen, Y., and Huang, L. (2012). Cloning and expression analysis of a new 3-hydroxy-3-methylglutaryl coenzyme A reductase gene from Salvia miltiorrhiza (SmHMGR3). China J. Chin. Mater. Med. 37, 2378–2382.
Zhao, J., Lou, J., Mou, Y., Li, P., Wu, J., and Zhou, L. (2011). Diterpenoid tanshinones and phenolic acids from cultured hairy roots of Salvia miltiorrhiza Bunge and their antimicrobial activities. Molecules 16, 2259–2267. doi: 10.3390/molecules16032259
Zhao, J. L., Zhou, L. G., and Wu, J. Y. (2010). Effects of biotic and abiotic elicitors on cell growth and tanshinone accumulation in Salvia miltiorrhiza cell cultures. Appl. Microbiol. Biot. 87, 137–144. doi: 10.1007/s00253-010-2443-4
Zhou, K., Qiao, K., Edgar, S., and Stephanopoulos, G. (2015). Distributing a metabolic pathway among a microbial consortium enhances production of natural products. Nat. Biotechnol. 33, 377–383. doi: 10.1038/nbt.3095
Zhou, L., Tang, K., and Guo, S. (2018). The plant growth-promoting fungus (PGPF) Alternaria sp A13 markedly enhances Salvia miltiorrhiza root growth and active ingredient accumulation under greenhouse and field conditions. Int. J. Mol. Sci. 19, 270–284. doi: 10.3390/ijms19010270
Zhou, S., Chen, W., Su, H., and Zheng, X. (2013). Protective properties of tanshinone I against oxidative DNA damage and cytotoxicity. Food Chem. Toxicol. 62, 407–412. doi: 10.1016/j.fct.2013.08.084
Zhou, Y. J., Gao, W., Rong, Q., Jin, G., Chu, H., Liu, W., et al. (2012). Modular pathway engineering of diterpenoid synthases and the mevalonic acid pathway for miltiradiene production. J. Am. Chem. Soc. 134, 3234–3241. doi: 10.1021/ja2114486
Keywords: tanshinones, Salvia miltiorrhiza, extraction and separation, pharmacological activities, biosynthesis
Citation: Jiang Z, Gao W and Huang L (2019) Tanshinones, Critical Pharmacological Components in Salvia miltiorrhiza. Front. Pharmacol. 10:202. doi: 10.3389/fphar.2019.00202
Received: 07 December 2018; Accepted: 18 February 2019;
Published: 14 March 2019.
Edited by:
Rong-Rong He, Jinan University, ChinaReviewed by:
Jian-Qiang Kong, Institute of Materia Medica (IMM), ChinaDan Hu, Jinan University, China
Lei Zhang, The Second Military Medical University, China
Copyright © 2019 Jiang, Gao and Huang. This is an open-access article distributed under the terms of the Creative Commons Attribution License (CC BY). The use, distribution or reproduction in other forums is permitted, provided the original author(s) and the copyright owner(s) are credited and that the original publication in this journal is cited, in accordance with accepted academic practice. No use, distribution or reproduction is permitted which does not comply with these terms.
*Correspondence: Wei Gao, weigao@ccmu.edu.cn