- 1The Affiliated Tumor Hospital of Guangxi Medical University, Nanning, China
- 2Department of Biochemistry and Molecular Biology, School of Preclinical Medicine, Guangxi Medical University, Nanning, China
- 3Department of Pharmaceutical Sciences, College of Pharmacy and Health Sciences, St. John’s University, Queens, NY, United States
- 4Department of Pharmacy, The First Affiliated Hospital of Guangxi Medical University, Nanning, China
- 5College of Pharmacy, Guangxi Medical University, Nanning, China
Multidrug resistance is reported to be related to the transmembrane transportation of chemotherapeutic drugs by adenosine triphosphate-binding cassette (ABC) transporters. ABC subfamily G member 2 (ABCG2) is a member of the ABC transporter superfamily proteins, which have been implicated as a key contributor to the development of multidrug resistance in cancers. A new epigallocatechin gallate derivative, Y6 was synthesized in our group. Our previous study revealed that Y6 increased the sensitivity of drug-resistant cells to doxorubicin, which was associated with down-regulation of P-glycoprotein expression. In this study, we further determine whether Y6 could reverse ABCG2-mediated multidrug resistance. Results showed that, at non-toxic concentrations, Y6 significantly sensitized drug-selected non-small cell lung cancer cell line NCI-H460/MX20 to substrate anticancer drugs mitoxantrone, SN-38, and topotecan, and also sensitized ABCG2-transfected cell line HEK293/ABCG2-482-R2 to mitoxantrone and SN-38. Further study demonstrated that Y6 significantly increased the accumulation of [3H]-mitoxantrone in NCI-H460/MX20 cells by inhibiting the transport activity of ABCG2, without altering the expression levels and the subcellular localization of ABCG2. Furthermore, Y6 stimulated the adenosine triphosphatase activity with a concentration-dependent pattern under 20 μM in membranes overexpressing ABCG2. In addition, Y6 exhibited a strong interaction with the human ABCG2 transporter protein. Our findings indicate that Y6 may potentially be a novel reversal agent in ABCG2-positive drug-resistant cancers.
Introduction
Multidrug resistance (MDR) to chemotherapeutic drugs could be found in various types of cancer cells (Fletcher et al., 2010). A wide range of structurally different chemotherapeutic drugs have been shown to be substrates of ATP-binding cassette (ABC) transporter proteins. Powered by the energy from ATP hydrolysis, ABC-transporters have become a significant impediment in cancer therapy. In total, 48 different ABC transporters were identified in the human genome, divided to seven subfamilies (ABCA-ABCG) due to structural similarities. Among all subfamilies, P-glycoprotein (P-gp/ABCB1) and breast cancer resistance protein (BCRP/ABCG2) are major transporters in MDR (Sodani et al., 2012).
ABCG2 is also known as mitoxantrone resistance-associated protein (MXR) or placenta-specific ATP-binding cassette transporter (ABCP) (Miyake et al., 1999; Dean and Allikmets, 2001). The ABCG2 protein has a molecular weight of 72-kDa. It is a half transporter with one nucleotide binding domain (NBD) and one transmembrane domain (TMD) and functions in the form of homodimer or an oligomer (Taylor et al., 2017). ABCG2 transporter is specifically distributed in the plasma membrane. Normally, it is highly expressed in the colon epithelium, the apical surface of small intestines, the canalicular membrane of liver and bile duct, cortical tubules of the kidney and prostate epithelium, and at the luminal surfaces of microvessel endothelium of human brain (Maliepaard et al., 2001; Fetsch et al., 2006). Such distribution results in alteration of the absorption, distribution, metabolism, and elimination of drugs since ABCG2 performs compound transmembrane transport on secretory surfaces of organs. ABCG2 could transport a variety of anti-neoplastic drugs such as mitoxantrone, topotecan, irinotecan, doxorubicin, daunorubicin, 9-aminocamptothecin, and epirubicin as its substrates (Doyle and Ross, 2003).
A mutation at position 482 (Arg or R) of ABCG2 produces a distinct substrate preference (Chen et al., 2003), which results in different drug-binding and drug-efflux capacity of the transporter (Honjo et al., 2001; Pozza et al., 2006; Dai et al., 2009). For example, after Arg at position 482 was replaced by threonine (Thr or T) or glycine (Gly or G), the substrate specificity was changed in both mutant and wild-type variants. Mitoxantrone and major nucleoside inhibitors are common substrates of wild-type and mutant ABCG2, while daunorubicin is the substrate of mutant Gly and Thr variants and is not a substrate of the wild-type ABCG2 (Ejendal and Hrycyna, 2002; Mao and Unadkat, 2005; Ejendal et al., 2006).
Multidrug resistance in acute myeloid leukemia (AML), non-small cell lung cancer (NSCLC), colon carcinoma and breast cancer were also reported to be strongly correlated with the overexpression of ABCG2 (Steinbach et al., 2002; van den Heuvel-Eibrink et al., 2002; Nakanishi and Ross, 2012). Notably, ABCG2 is overexpressed only in subpopulations of AML specimens (Abbott et al., 2002; Suvannasankha et al., 2004). The overexpression of ABCG2 in these subpopulations of stem cells was also reported among other tumors such as neuroblastomas, Ewing sarcomas, breast cancer, small cell lung cancer, and glioblastomas (Hirschmann-Jax et al., 2004). These stem cells with overexpression of ABCG2 may play an important role in resistance to chemotherapeutic drugs.
As one of the MDR reversal modulators, epigallocatechin gallate (EGCG) was revealed to down-regulate P-gp and BCRP in a tamoxifen resistant cancer cell line (Farabegoli et al., 2010). Furthermore, EGCG (Figure 1A) was reported to significantly inhibit proliferation of a drug-resistant cancer cell line, BEL-7404/DOX, in vitro when co-administered with doxorubicin (Liang et al., 2010). However, the application of EGCG was limited due to an unstable chemical profile that could be subjected to rapid oxidation and short duration of action because of multiple phenolic hydroxyl groups (Lee et al., 2002). Inspired by the structure of EGCG, we synthesized Y6 (Figure 1B), an ethylation product of EGCG. Y6 has been evaluated as a reversal agent that specifically reverses ABC transporter-mediated MDR in vitro (Wen et al., 2017). In this study, we determined the potential effect of Y6 as a reversal agent that re-sensitizes ABCG2-mediated MDR in vitro.
Materials and Methods
Reagents
Y6 (purity 96.87%) was synthesized in our research group and was made in a 10 mM stock solution in dimethylsulfoxide (DMSO). Dulbecco’s modified Eagle’s medium (DMEM), penicillin/streptomycin, trypsin 0.25%, fetal bovine serum (FBS) and bovine calf serum were purchased from Hyclone (GE Healthcare Life Science, Pittsburgh, PA, United States). Mitoxantrone, SN-38, topotecan, and cisplatin were products from Sigma-Aldrich (St. Louis, MO, United States). Fumitremorgin C (FTC) was provided by Dr. Susan E. Bates from NIH (Bethesda, MD, United States). [3H]-Mitoxantrone was purchased from Moravek Biochemicals, Inc. (Brea, CA, United States). Mouse monoclonal ABCG2 antibody and mouse monoclonal β-actin antibody were purchased from Gene Tex, Inc. (Irvine, CA, United States). Goat anti-mouse IgG secondary antibody conjugated with Alexa Fluor 488 and 4′,6-diamidino-2-phenylindole (DAPI) were purchased from Thermo Fisher Scientific, Inc. (Rockford, IL, United States). The ATPase assay kit and membrane vesicles were purchased from BD Biosciences (San Jose, CA, United States). PBS and trypsin were purchased from Bosterbio (Pleasanton, CA, United States). RIPA lysis buffer, protein loading buffer, BCA protein assay kit, SDS-PAGE gel preparation kit, protein marker, western transfer buffer, SDS-PAGE running buffer, primary/secondary antibody dilution buffer were purchased from Sigma-Aldrich (St. Louis, MO, United States).
Cell Lines and Cell Culture
Non-small cell lung cancer cell line NCI-H460 and its mitoxantrone-selected ABCG2-overexpressing NCI-H460/MX20 cells were used in the ABCG2 reversal study. NCI-H460/MX20 cells were cultured in a medium containing 20 nM of mitoxantrone. HEK293/pcDNA3.1 and wild-type HEK293/ABCG2-482-R2 were established by transfecting HEK293 cells with either an empty pcDNA3.1 vector or a vector containing full length ABCG2 with Arg at position 482. HEK293/ABCG2-482-R2 cells were cultured in a medium containing 2 mg/mL of G418. All the cell lines were maintained in DMEM containing 10% (v/v) FBS and 1% (v/v) penicillin/streptomycin in a constant temperature incubator containing 5% CO2 at 37°C.
Cytotoxicity by MTT Assay
The viability of NCI-H460 and NCI-H460/MX20 cells or HEK293/pcDNA3.1 and HEK293/ABCG2-482-R2 cells to chemotherapeutic drugs was measured for the ABCG2 reversal study using the MTT assay. Cells (5000 cells per well) were seeded into 96-well microplates with 160 μL per well and cultured overnight. Mitoxantrone, SN-38, topotecan, and cisplatin were diluted with PBS to a series of various concentrations and added to designated wells (20 μL per well) with or without the reversal agents (Y6 and FTC) (20 μL per well). Cells were incubated for 72 h at 37°C. Then, 20 μl of MTT solution (4 mg/mL, in PBS) was added into each well and incubated for 4 h at 37°C in dark. Then the supernatant medium was discarded and 100 μl of DMSO was added to each well for formazan dissolution. Finally, the light absorbance at 490 nm was measured using the OPSYS microplate reader (Dynex Technology, Chantilly, VA, United States). FTC was used as positive reversal agent of ABCG2.
Western Blotting Analysis
NCI-H460/MX20 cells were treated with or without Y6 for 72 h with different drug concentrations (5, 10, 15 μM). In addition, NCI-H460/MX20 cells were also treated with or without 10 μM of Y6 for different time periods (24, 48, 72 h). Then cells were collected and lysed in ice-cold lysis buffer containing 50 mM Tris (pH 7.4), 150 mM NaCl, 1% Triton X-100, 0.1% SDS, 1 mM EDTA, and 1× phosphatase inhibitor cocktail on ice for 20 min. Cell lysates were centrifuged at 4°C at 13,000 rpm/min for 10 min. Subsequently, the supernatant was collected in Eppendorf tubes and protein concentrations were determined by bicinchoninic acid (BCATM)-based protein assay (Thermo Scientific, Rockford, IL, United States). Equal amount of protein was loaded and separated by SDS-polyacrylamide gel electrophoresis and transferred to a polyvinylidene fluoride (PVDF) membrane through electrophoresis. The PVDF membrane was submerged in 5% skim milk for 1 h, and then was incubated with primary monoclonal antibodies (ABCG2 at 1:500 dilution or β-actin at 1:1000 dilution) overnight at 4°C. After the membrane was washed with the TBST buffer, the membrane was incubated with HRP (horseradish peroxidase)-conjugated secondary antibody (1:1000 dilution) at room temperature for 2 h. The washing with TBST buffer, the membrane was exposed to Infrared Imaging System to visualize the bands.
Immunofluorescence of ABCG2
Equal amounts of NCI-H460 and NCI-H460/MX20 cells were seeded in sterile coated 24-well plates and were cultured overnight. NCI-H460/MX20 cells were treated with or without 10 μM of Y6 for different time periods (24, 48, 72 h). Then the cells were fixed in 4% paraformaldehyde for 15 min, permeabilized by 0.25% Triton X-100 for 15 min and then blocked with 6% BSA for 1 h. Cells were further incubated with mouse monoclonal antibody against ABCG2 (1:200) overnight, and then were submerged in Alexa Fluor 488 conjugated secondary antibody (1:2000) solution in the dark for 2 h to localize ABCG2. 2-(4-amidinophenyl)-6-indolecarbamidene dihydrochloride (DAPI) solution was used to counterstain the nuclei in dark. Images were taken with an inverted IX70 microscope (Olympus, Center Valley, PA, United States) following our previous protocol (Guo et al., 2014).
[3H]-Mitoxantrone Accumulation Assay
The accumulation of [3H]-mitoxantrone in NCI-H460 and NCI-H460/MX20 was measured in the presence or absence of inhibitors (FTC or Y6). The cells (5 × 106) in each centrifuge tube were trypsinized, resuspended, and pre-incubated with PBS, Y6 (5 and 10 μM), or FTC (5 μM) for 2 h at 37°C. After the cell suspension was centrifuged at 1500 rpm/min for 5 min, the supernatant was discarded. Subsequently, cells were resuspended in medium containing 0.1 μM [3H]-mitoxantrone at 37°C for 2 h in the presence or absence of inhibitors following above treatment. Cells were washed three times with ice-cold PBS. Then, cells were placed in 5 ml scintillation liquid and their radioactivity was measured in the Packard TRI-CARB 1900CA liquid scintillation analyzer (Packard Instrument, Downers Grove, IL, United States) (Sun et al., 2012; Patel et al., 2013). FTC was used as the positive reversal agent of ABCG2.
[3H]-Mitoxantrone Efflux Assay
Mitoxantrone drug efflux in NCI-H460 or NCI-H460/MX20 was measured. The cells were pretreated with PBS, Y6 (5 and 10 μM) or FTC (5 μM) for 2 h at 37°C. Then radioactive substrate [3H]-mitoxantrone was added and the cells were further incubated for 2 h. Cell suspension was centrifuged at 1500 rpm/min for 5 min and the supernatant was discarded. The cells were re-supplemented with fresh medium with or without a reversal agent. After 0, 30, 60, and 120 min, the aliquots of cells were removed and washed three times with ice-cold PBS immediately. Radioactivity was then measured as described in the accumulation assay above.
ABCG2 ATPase Assay
The vanadate-sensitive ATPase activity of ABCG2 was performed by an ATPase assay kit from BD Biosciences (San Jose, CA, United States) as previously described (Zhang et al., 2016). Briefly, the ABCG2 membrane vesicles were incubated in ATPase buffer with or without vanadate for 5 min at 37°C. Y6 was then added to the assay buffer in concentration gradient (0–40 μM). The mixtures were incubated for 5 min at 37°C. Then Mg-ATP solution was added to assay buffer and was incubated for 20 min at 37°C. The mixture was added with 100 μL of 5% SDS solution and then its light absorption was detected at 880 nm using a spectrophotometer.
Molecular Docking of Y6 With ABCG2 Model
Molecular modeling was performed with Maestro v11.1 (Schroñdinger, LLC, New York, NY, United States 2017) software (Zhang et al., 2017). The protein preparation of wild-type human ABCG2 (PDB ID: 6FFC) (Jackson et al., 2018) was essentially performed and the grid (30 Å) was generated by selecting the same binding pocket as the two substrates (MZ29) in TMD. After that, the structure of Y6 was built and prepared. Compound Y6 with best-scored conformation was obtained through Glide XP docking then was used to generate receptor grid for induced-fit docking (IFD). The IFD protocol with default parameters was performed and the docking score (kcal/mol) was obtained.
Statistical Analysis
The data were analyzed using a t-test method. All values represent the mean ± standard deviation SD of three independent experiments performed in triplicate. The priori p-value for significance was p < 0.05.
Results
Y6 Sensitized ABCG2-Overexpressing Cells to Chemotherapeutic Drugs
In order to investigate the reversal effects of Y6 on drug-resistant cells, we first examined the sensitivity of ABCG2-overexpressing cells to Y6. Based on the results from the cytotoxicity assay, two non-toxic concentrations of Y6 (5.0 and 10.0 μM) were selected for further experimentation (Figures 2A,B).
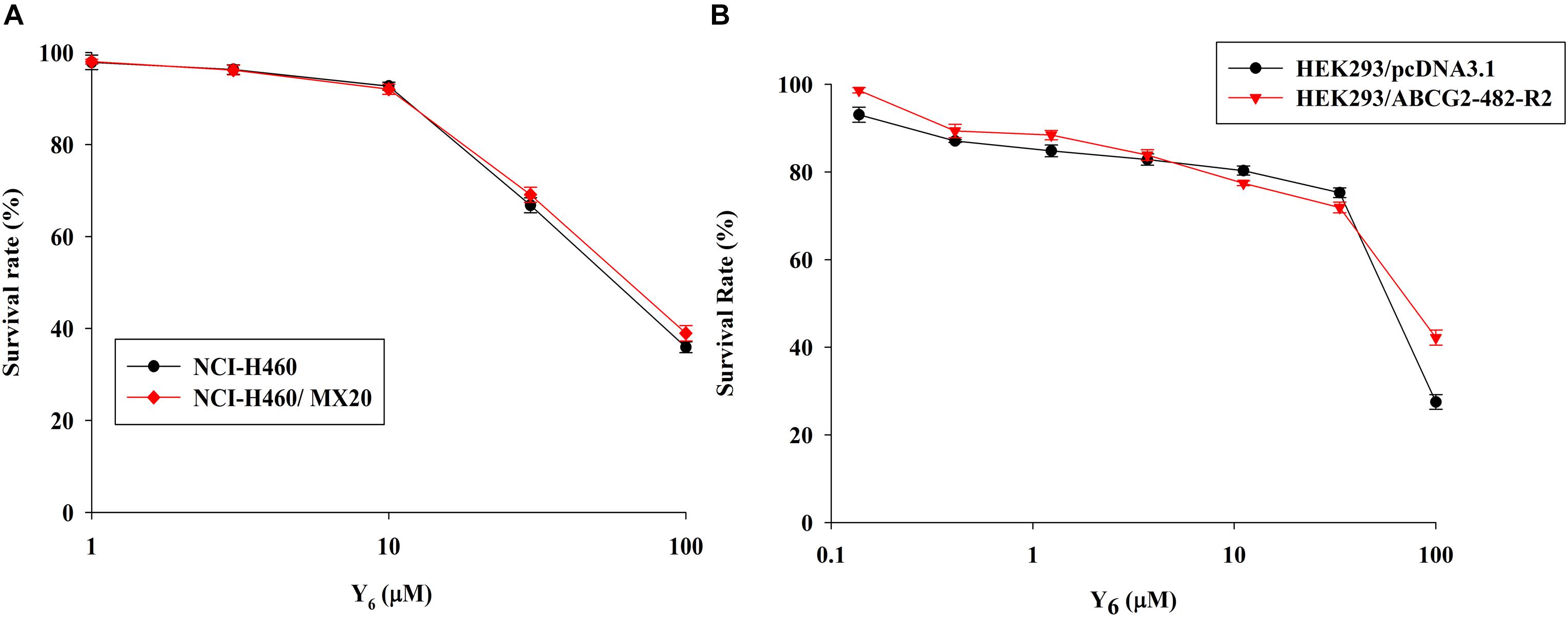
Figure 2. The cytotoxicity of Y6 in parental and ABCG2-overexpressing cells. (A) Cytotoxicity of Y6 was evaluated in parental NCI-H460 and ABCG2-overexpressing NCI-H460/MX20 cells. (B) Cytotoxicity of Y6 was evaluated in transfected HEK293/pcDNA3.1 and ABCG2-overexpressing HEK293/ABCG2-482-R2 cells. Cells were incubated with different concentration of Y6 for 72 h. Survival rate was determined by MTT assay. Representative curves were shown as cell survival rate verses concentration of compounds. Points and error bars display the mean and SD.
In the sensitization experiment, mitoxantrone-selected NCI-H460/MX20 cells showed a much higher IC50 value to ABCG2 substrates (mitoxantrone, SN-38, and topotecan) than that in parental NCI-H460 cells. Y6 at both 5.0 and 10.0 μM were able to significantly increase the sensitivity of NCI-H460/MX20 cells to mitoxantrone, SN-38, and topotecan. A significant reduction in IC50 values was observed with the treatment of Y6 in NCI-H460/MX20 cells as shown in Table 1. Meanwhile, no significant changes in IC50 values were observed in parental NCI-H460 cells. Similarly, Y6 also increased the sensitivity of transfected HEK293/ABCG2-482-R2 cells, which had a much higher IC50 value to ABCG2 substrates than that in parental HEK293/pcDNA3.1 cells. Significant decrease in IC50 values of mitoxantrone and SN-38 was observed in Y6-present treatment of transfected HEK293/ABCG2-482-R2 cells as compared to Y6-absent treatment, and no significant change was observed in HEK293/pcDNA3.1 cells as shown in Table 2. Uniformly, the efficacy of Y6 showed a concentration-dependent pattern. Cisplatin, which is not a substrate of ABCG2, was used as a negative control. FTC at 5.0 μM was used as a positive control to evaluate the effects of Y6.
Based on the above results, it appeared that Y6 could significantly reverse ABCG2-mediated MDR in both NCI-H460/MX20 and HEK293/ABCG2-482-R2 cells.
Y6 Had No Effect on the Protein Expression of ABCG2 in NCI-H460/MX20 Cells
To determine the reversal mechanism of Y6 on ABCG2-mediated MDR in cancer cells, Western blot analysis was performed using the parental NCI-H460 cells and the drug-selective NCI-H460/MX20 cells. NCI-H460/MX20 cells were incubated with either different concentrations of Y6 (0, 5, 10, 15 μM for 72 h) or different incubation time (0, 24, 48, 72 h at 10 μM). No significant changes were observed in the expression level of ABCG2 protein in NCI-H460/MX20 cells treated with Y6 compared to Y6-absent treatment group (Figures 3A,B). The results indicated that the MDR reversal mechanism of Y6 was not relative to the expression level of ABCG2.
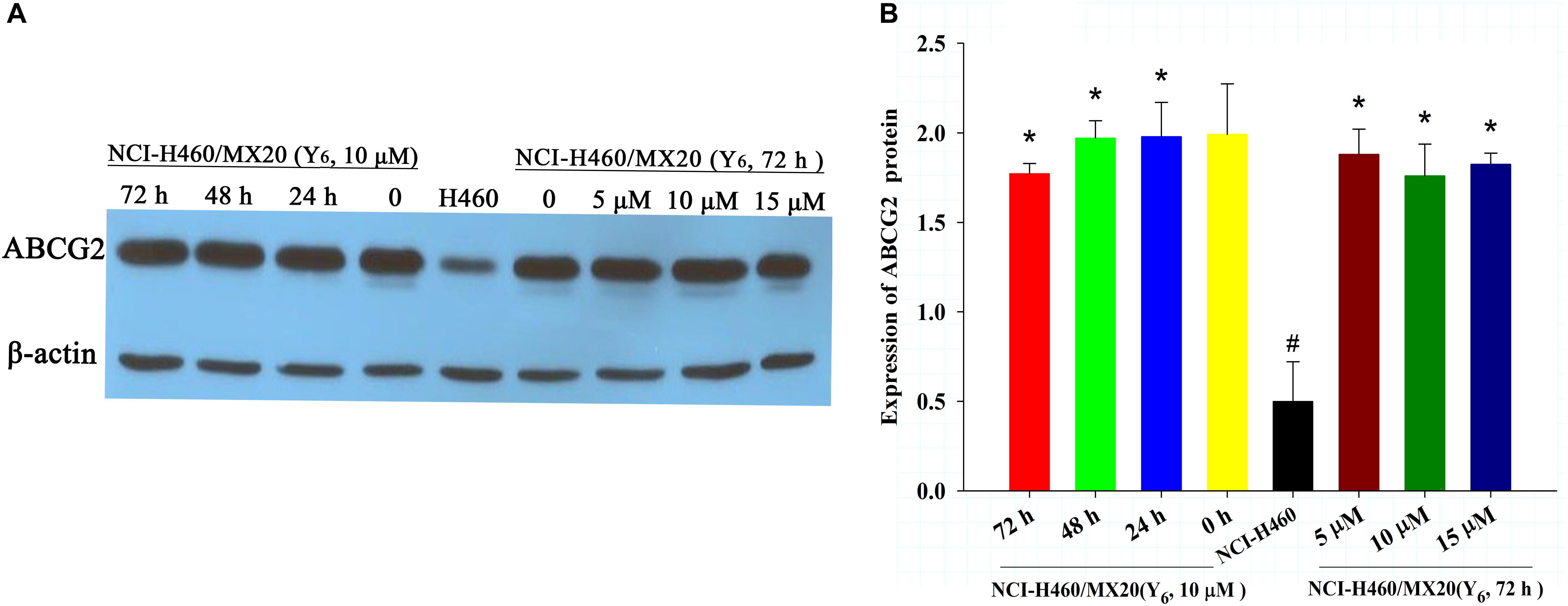
Figure 3. (A) The expression of ABCG2 in NCI-H460 and NCI-H460/MX20 cells with different treatments. Effect of Y6 at 10 μM on expression levels of ABCG2 in NCI-H460 and NCI-H460/MX20 cells at 0, 24, 48, and 72 h; or 0, 5, 10, 15 μM of Y6 after incubation for 72 h. (B) Quantified ABCG2 expression in NCI-H460 and NCI-H460/MX20 cells with different treatments. Values represent means ± SDs of at least three independent experiments performed in triplicate; ∗p > 0.05 vs. the NCI-H460/MX20 cells without treatment on the ABCG2 protein; #p < 0.05 vs. the NCI-H460/MX20 cells without treatment on the ABCG2 protein.
Y6 Did Not Alter the Subcellular Localization of ABCG2 in NCI-H460/MX20 Cells
As a transmembrane protein, ABCG2 could also be affected by protein localization. Thus, effect of Y6 on ABCG2 protein cellular localization was determined with immunofluorescence assay. As is shown in Figure 4, Y6 did not trigger the internalization of ABCG2 in NCI-H460/MX20 cells after incubating with 10 μM of Y6 for 0, 24, 48, and 72 h. The results indicated that the MDR reversal mechanism of Y6 was not induced by altering the localization of ABCG2.
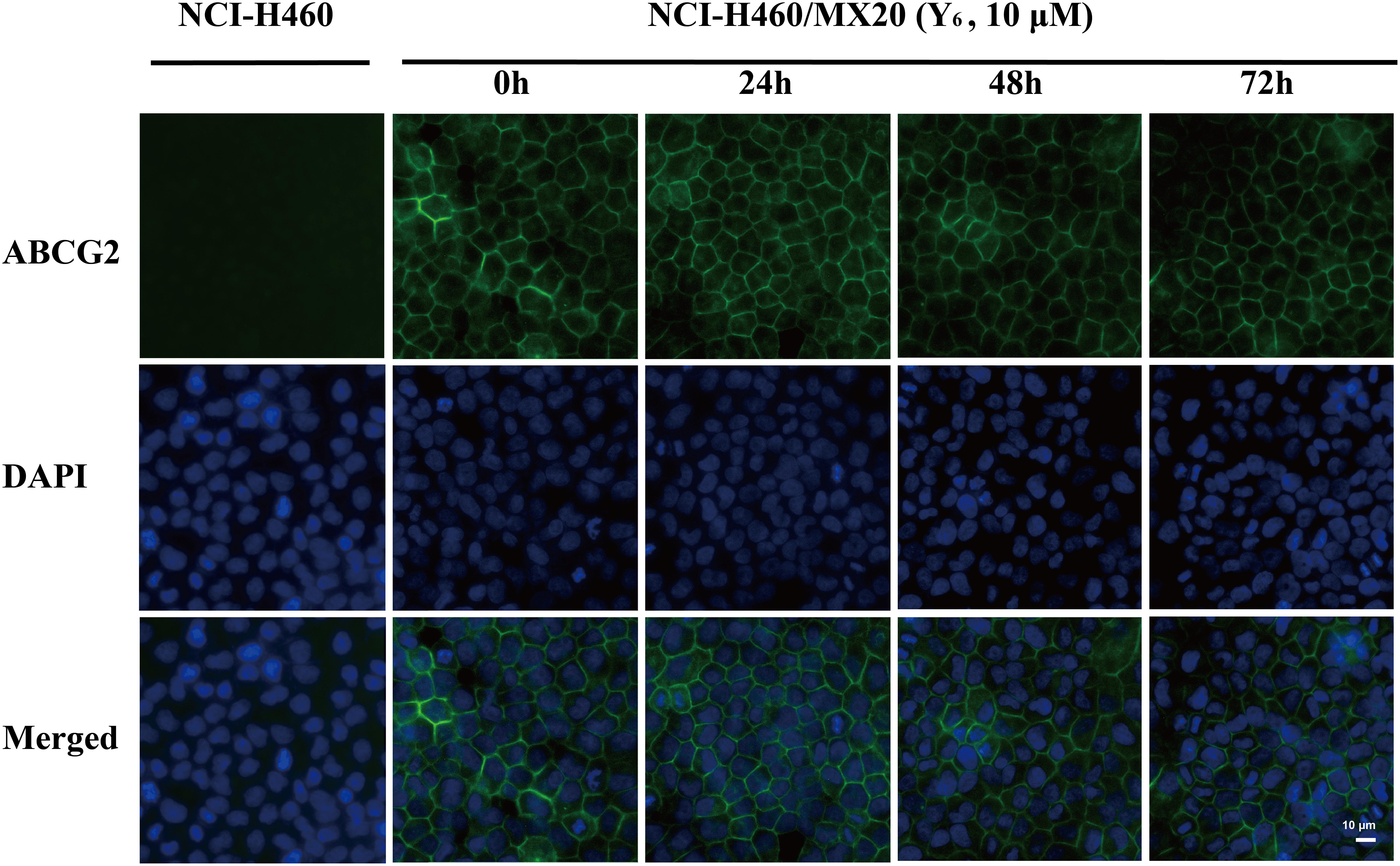
Figure 4. Effect of Y6 at 10 μM and different times on the subcellular localization of ABCG2. Scale bar: 10 μM, DAPI (blue) counterstains the nuclei. ABCG2 staining is shown in green.
Effect of Y6 on the Intracellular Accumulation of [3H]-Mitoxantrone
To further investigate the mechanism of reversal effect, we studied the intracellular accumulation of [3H]-mitoxantrone in NCI-H460/MX20 cells treated by Y6. We found that the intracellular accumulation of [3H]-mitoxantrone was significantly increased in NCI-H460/MX20 cells treated with 5.0 and 10 μM of Y6 (Figure 5A). The accumulation increased with increasing concentrations of Y6. However, Y6 did not show a significant effect on the intracellular accumulation of [3H]-mitoxantrone in parental NCI-H460 cells. The results showed that Y6 has comparable effects to FTC (5 μM). It could be concluded that Y6 significantly increased intracellular concentrations of chemotherapeutic drugs in NCI-H460/MX20 cells and increased cytotoxicity in ABCG2-overexpressing cells.
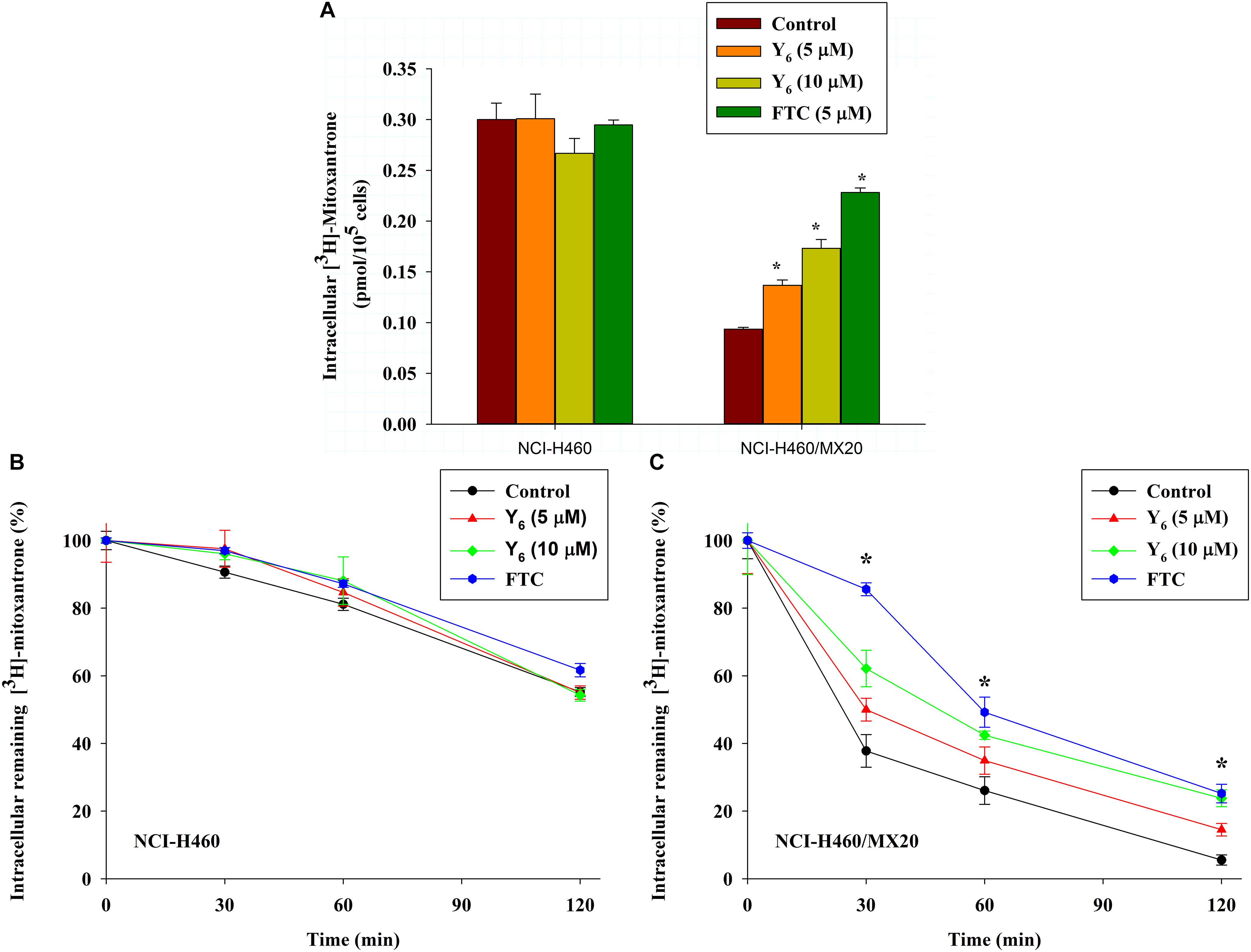
Figure 5. (A) Effect of Y6 on the accumulation of [3H]-mitoxantrone in NCI-H460 and NCI-H460/MX20 cells. ∗p < 0.05 vs. the control group. FTC at 5 μM is used as positive control for ABCG2-overexpressing cells. (B,C) Effect of Y6 on the efflux of [3H]-mitoxantrone in different times. After 0, 30, 60, or 120 min, the same numbers of NCI-H460 (B) and NCI-H460/MX20 (C) were placed in the scintillation fluid to measure the radioactivity. Data points represent the means ± SD of triplicate determinations. Experiments were performed at least three independent times. ∗p < 0.05 vs. control group. FTC at 5 μM was used as a positive control for ABCG2-overexpressing cells.
Effect of Y6 on the [3H]-Mitoxantrone Efflux Time-Course
The ABCG2 transporter is known to induce antitumor drug resistance by pumping out drugs and lowering their intracellular concentration. To further measure if the drug accumulation was relative with Y6 inhibiting ABCG2-mediated drug efflux, we performed a time-course efflux of [3H]-mitoxantrone with or without Y6 at different time points. After removing [3H]-mitoxantrone from the culture medium, efflux occurred in NCI-H460 and NCI-H460/MX20 cells as shown in Figures 5B,C. After NCI-H460/MX20 cells were incubated for 30 min, about 50, 38, and 62% normalized loss of [3H]-mitoxantrone occurred in Y6-treated (5, 10 μM) groups and the control group, respectively. About 65, 58, and 73% normalized loss of [3H]-mitoxantrone, respectively, occurred with or without Y6 after incubating for 60 min; furthermore, NCI-H460/MX20 cells lost about 86, 76, and 94% normalized [3H]-mitoxantrone, respectively, after incubating for 120 min. Meanwhile, 10 μM of Y6 retained more [3H]-mitoxantrone in NCI-H460/MX20 cells than 5 μM of Y6 (Figure 5C). Efflux pattern of NCI-H460 cells were not significantly altered by Y6 (Figure 5B). The results showed that Y6 inhibited the efflux of [3H]-mitoxantrone in NCI-H460/MX20 cells and the retention amount in cells followed a Y6-concentration-dependent pattern.
Effect of Y6 on the ABCG2 ATP Hydrolysis
The ABCG2 transporter could transport substrates across the membrane by utilizing energy derived from ATP hydrolysis. To evaluate the effect of Y6 on the ABCG2 ATP hydrolysis, we measured ATPase activity in the presence of Y6 at a series of concentrations ranging from 0 to 40 μM. Results showed that Y6 stimulated the ATPase activity of ABCG2 at concentrations ranging from 0 to 20 μM. No further increase in ATPase activity occurred when the concentration of Y6 was above 20 μM (Figure 6), which could indicate that Y6 stimulates the ATPase activity of ABCG2 by acting on the drug-substrate-binding site.
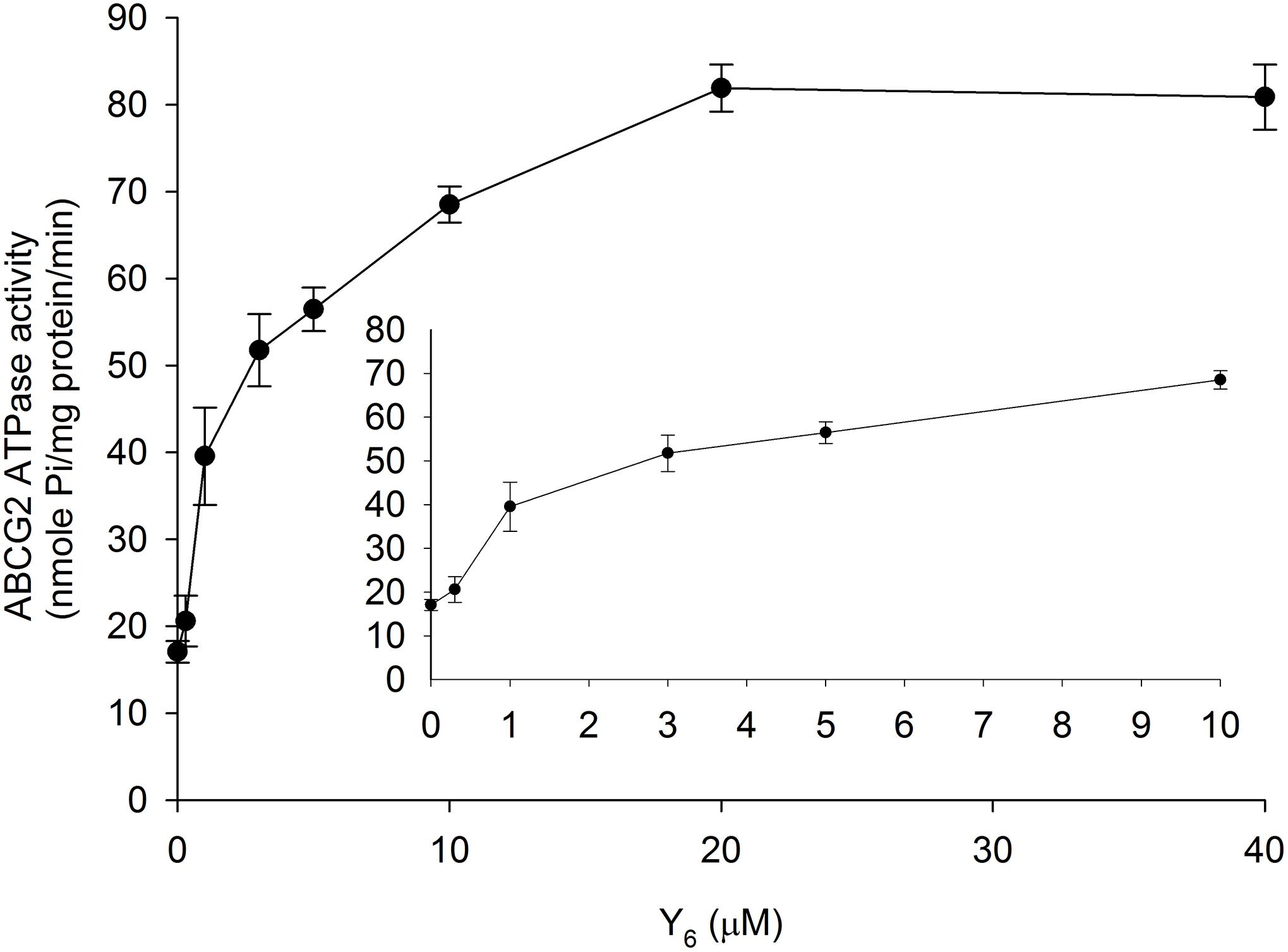
Figure 6. Effect of Y6 on the ABCG2 ATPase activity. The Vi-sensitive ATPase activities of ABCG2 in membrane vesicles were determined with different concentrations of Y6 (0–40 μM). Experiments were repeated three times.
Docking Analysis of Y6 With Human ABCG2 Model
To further learn if Y6 have direct interactions with the ABCG2 transporter, we performed a molecular docking simulation. A two-dimensional ligand-receptor interaction diagram of the best-scored induced-fit docked position of Y6 within the drug-binding cavity of human ABCG2 is shown in Figure 7A, while the three-dimensional ligand-receptor interaction diagram is shown in Figure 7B. The 7-hydroxy group in the chroman ring of compound Y6 forms a hydrogen bonding with Asn436 (-OH…O=C-Asn436), while the hydroxy group in the 3,4-diethoxy-5-hydroxyphenyl group forms a hydrogen bonding with Thr542 (-OH…OH-Thr542). Moreover, compounds Y6 was stabilized into a hydrophilic pocket formed by residues Phe432, Phe439, Val442, Leu539, Ile543, Val546, and Met549 of the ABCG2 protein. From the results, compound Y6 had a strong interaction (-11.448 kcal/mol) with the human ABCG2 transporter protein.
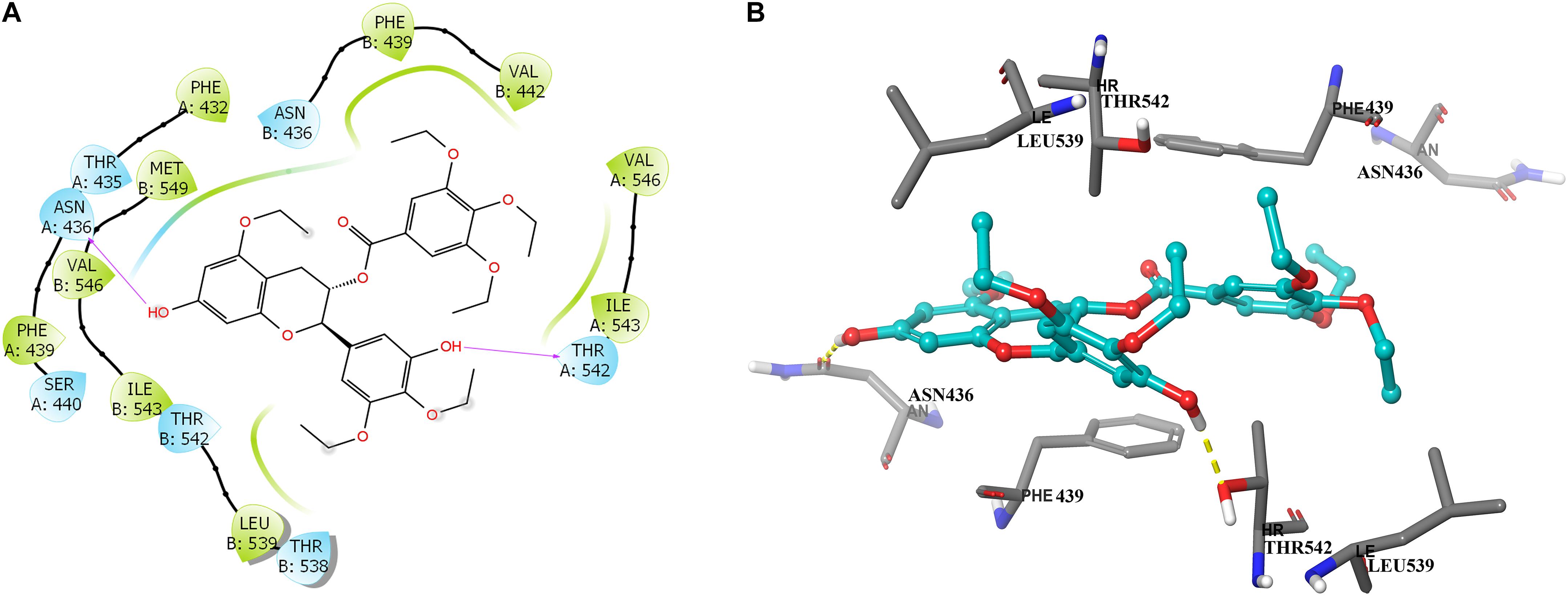
Figure 7. Predicted binding positions of compound Y6 with the human ABCG2 transporter protein. (A) A two-dimensional ligand–receptor interaction diagram shows the important interactions of compound Y6 with the binding site residues of human ABCG2. The amino acids are shown as colored bubbles, cyan indicates polar residues and green indicates hydrophobic residues. Hydrogen bonds are indicated by the purple arrow. (B) Docked position of compound Y6 within the binding site of the human ABCG2 transporter protein. Compound Y6 is shown as a ball and stick model, with the atoms colored as follows: carbon = cyan, hydrogen = white, and oxygen = red. The carbons of the important residues are indicated by the gray color while the other atoms are depicted as sticks with the same color scheme as above and nitrogen is indicated by the blue color. Hydrogen bonds are indicated by dotted yellow lines.
Discussion
In recent years, the studied reversal agents which acted on ABC transporter family mostly targeted ABCB1, and very few targeted ABCG2. FTC was one of the ABCG2 reversal agents that was widely used in ABCG2-mediated MDR research. FTC was a toxin obtained from Aspergillus fumigatus, which could inhibit ABCG2 activity at a low concentration without much effect on the functions of ABCB1 and ABCC1. However, its use was limited in clinical patients because of its central nervous system neurotoxicity, although FTC was a classic ABCG2 reversal agent in experiments (Rabindran et al., 1998). Therefore, to look for more safe and effective ABCG2 reversal agents, we performed research on Y6 and evaluated its reversal effect on ABCG2-overexpressing cells. Our previously published study indicated that Y6 could reverse ABCB1-mediated multidrug resistance by down-regulating ABCB1 protein expression level and changing its function in ABCB1-overexpressing cells (Wen et al., 2017). In this study, we performed the experiments on ABCG2-overexpressing cells and found that Y6 was also effective in inhibiting ABCG2-mediated drug resistance at non-toxic concentrations.
In our experiments, mitoxantrone-selected MDR cells, NCI-H460/MX20, and their parental cells, as well as ABCG2-overexpressing transfected cells, HEK293/ABCG2-482-R2, and their parental cells were used to determine cytotoxicity and reversal effect of Y6. Results showed that Y6 significantly sensitized NCI-H460/MX20 and HEK293/ABCG2-482-R2 to mitoxantrone, SN-38, or topotecan (Tables 1, 2). However, Y6 did not increase the cytotoxic effect of cisplatin, which is not a substrate for the ABCG2 transporter. The results implied that Y6 could reverse ABCG2-mediated drug resistance in ABCG2-overexpressed cells.
ABCG2 played an important role in protecting tumor cells from cytotoxic damage of antineoplastic drugs (Polgar et al., 2008). One of the most prominent MDR mechanisms is ABCG2 overexpression. The overexpression of ABCG2 can produce MDR to antineoplastic drugs as shown in many studies (Sargent et al., 2001; Ho et al., 2008). Susceptibility to drugs could be increased with absence of ABCG2 as shown in the bcrp1 (-/-) knockout mice studies (Kanzaki et al., 2001; Jonker et al., 2002). Increased ABCG2 expression was also correlated with complete remission, overall survival, relapse-free survival and disease-free survival (Suvannasankha et al., 2004; Damiani et al., 2006; van den Heuvel-Eibrink et al., 2007). To find out the possible mechanism of Y6 in reversing ABCG2-mediated drug resistance, we measured its effect on the expression of ABCG2. In our previous study, Y6 could down-regulate the expression of ABCB1 transporter in the reverse resistance experiment (Wen et al., 2017). In this study, the expression of ABCG2 transporter was not changed by Y6 with different concentrations and incubated time (Figure 3). Y6 also did not alter the subcellular localization of ABCG2 in NCI-H460/MX20 cells with different incubated times compared to untreated control (Figure 4). The results indicated that the MDR reversal mechanism of Y6 was not relative with the expression level and subcellular localization of ABCG2.
A decreased concentration of intracellular chemotherapeutic drug was a major cause of MDR in cancer cells. In order to further understand the reversal mechanism of Y6, we conducted a radiolabeled [3H]-mitoxantrone accumulation and efflux study to evaluate the intracellular drug level. ABCG2 is characterized as a part of self-defense systems and function as an efflux pump to transfer the toxic endogenous molecules and xenobiotics, including many cancer chemotherapies, out of the cell (Nakanishi and Ross, 2012). Mitoxantrone is one of ABCG2 substrates as confirmed in studies (Ozvegy et al., 2001; Nakanishi et al., 2003). Cellular resistance to mitoxantrone conferred by ABCG2 could lead to increased efflux and a low level intracellular accumulation of mitoxantrone (Doyle et al., 1998; Miyake et al., 1999; Nakanishi et al., 2003; Robey et al., 2003). Our results showed that NCI-H460/MX20 cells had lower level intracellular accumulation of [3H]-mitoxantrone than parental NCI-H460 cells. Y6 could increase the accumulation of [3H]-mitoxantrone compared to untreated control in the NCI-H460/MX20 cells, and the accumulation followed a Y6-concentration-dependent pattern (Figure 5A). Subsequently, an efflux assay was performed to determine whether the accumulation increase was relative to the inhibition of ABCG2 function by Y6. The results showed that ABCG2-mediated [3H]-mitoxantrone efflux was suppressed by Y6 and the efflux amount showed a negative correlation with the concentration of Y6 (Figures 5B,C). ABCG2 transfer function was inhibited by Y6.
To further understand the interaction of Y6 with ABCG2, an ATPase assay using ABCG2 overexpressed membranes and molecular docking simulation using human ABCG2 model were performed. ABC transporters could use energy from the hydrolysis of ATP to transport their substrates across the membrane (Borths et al., 2002; Locher, 2004; Locher and Borths, 2004). It was observed in the study that Y6 could stimulate ATPase ability of ABCG2 in a dose-dependent pattern at concentrations ranging from 0 to 20 μM, and then leveled off when Y6 concentration was above 20 μM (Figure 6). Moreover, the docking analysis of Y6 to human ABCG2 model further revealed that Y6 had a strong direct interaction with ABCG2 (Figure 7). Thus, we can confer that Y6 stimulates the ATPase ability of ABCG2 by acting on the drug-substrate-binding site.
Conclusion
The study demonstrated that Y6 could reverse ABCG2-mediated MDR in vitro. The mechanisms of Y6 in reversing MDR is related with the inhibition efflux function of ABCG2 by being a typical competitive substrate of ABCG2, while not relative to down-regulating the ABCG2 expression or altering subcellular localization of ABCG2. Thus, Y6 is expected to be a potential ABCG2-mediated resistance reversal agent with multiple targets.
Author Contributions
Y-AX, Z-SC, and GL contributed to experiment design. R-QZ, YW, PG, Z-NL, and C-YC performed the experiments. R-QZ and YW analyzed the data and wrote the initial draft of manuscript. D-HY reviewed the manuscript. Z-SC and YW obtained the funding.
Funding
This work was supported by the Open Project of Guangxi Colleges and Universities Key Laboratory of Biological Molecular Medicine Research (Grant No. GXBMR201602), the Young and Middle-aged Teachers Foundation Ability Enhancement Project of Guangxi Colleges and Universities (Grant No. 2018KY0102), the National Natural Science Foundation of China (Grant No. 81160532), and NIH (Grant No. 1R15CA143701).
Conflict of Interest Statement
The authors declare that the research was conducted in the absence of any commercial or financial relationships that could be construed as a potential conflict of interest.
Acknowledgments
We are thankful to Drs. Susan E. Bates and Robert W. Robey (NIH, Bethesda, MD, United States) for providing us HEK293/pcDNA3.1 (parental), HEK293/ABCG2-482-R2, NCI-H460, and NCI-H460/MX20 cell lines. We thank Dr. Tanaji T. Talele (St. John’s University, New York, NY, United States) for providing the computational resources for molecular modeling, and Dr. Yangmin Chen for reviewing and editing the paper.
References
Abbott, B. L., Colapietro, A. M., Barnes, Y., Marini, F., Andreeff, M., and Sorrentino, B. P. (2002). Low levels of ABCG2 expression in adult AML blast samples. Blood 100, 4594–4601. doi: 10.1182/blood-2002-01-0271
Borths, E. L., Locher, K. P., Lee, A. T., and Rees, D. C. (2002). The structure of Escherichia coli BtuF and binding to its cognate ATP binding cassette transporter. Proc. Natl. Acad. Sci. U.S.A. 99, 16642–16647. doi: 10.1073/pnas.262659699
Chen, Z. S., Robey, R. W., Belinsky, M. G., Shchaveleva, I., Ren, X. Q., Sugimoto, Y., et al. (2003). Transport of methotrexate, methotrexate polyglutamates, and 17beta-estradiol 17-(beta-D-glucuronide) by ABCG2: effects of acquired mutations at R482 on methotrexate transport. Cancer Res. 63, 4048–4054.
Dai, C. L., Liang, Y. J., Wang, Y. S., Tiwari, A. K., Yan, Y. Y., Wang, F., et al. (2009). Sensitization of ABCG2-overexpressing cells to conventional chemotherapeutic agent by sunitinib was associated with inhibiting the function of ABCG2. Cancer Lett. 279, 74–83. doi: 10.1016/j.canlet.2009.01.027
Damiani, D., Tiribelli, M., Calistri, E., Geromin, A., Chiarvesio, A., Michelutti, A., et al. (2006). The prognostic value of P-glycoprotein (ABCB) and breast cancer resistance protein (ABCG2) in adults with de novo acute myeloid leukemia with normal karyotype. Haematologica 91, 825–828.
Dean, M., and Allikmets, R. (2001). Complete characterization of the human ABC gene family. J. Bioenerg. Biomembr. 33, 475–479. doi: 10.1023/A:1012823120935
Doyle, L., and Ross, D. D. (2003). Multidrug resistance mediated by the breast cancer resistance protein BCRP (ABCG2). Oncogene 22, 7340–7358. doi: 10.1038/sj.onc.1206938
Doyle, L. A., Yang, W., Abruzzo, L. V., Krogmann, T., Gao, Y., Rishi, A. K., et al. (1998). A multidrug resistance transporter from human MCF-7 breast cancer cells. Proc. Natl. Acad. Sci. U.S.A. 95, 15665–15670. doi: 10.1073/pnas.95.26.15665
Ejendal, K. F., Diop, N. K., Schweiger, L. C., and Hrycyna, C. A. (2006). The nature of amino acid 482 of human ABCG2 affects substrate transport and ATP hydrolysis but not substrate binding. Protein Sci. 15, 1597–1607. doi: 10.1110/ps.051998406
Ejendal, K. F., and Hrycyna, C. A. (2002). Multidrug resistance and cancer: the role of the human ABC transporter ABCG2. Curr. Protein Pept. Sci. 3, 503–511. doi: 10.2174/1389203023380521
Farabegoli, F., Papi, A., Bartolini, G., Ostan, R., and Orlandi, M. (2010). (-)-Epigallocatechin-3-gallate downregulates Pg-P and BCRP in a tamoxifen resistant MCF-7 cell line. Phytomedicine 17, 356–362. doi: 10.1016/j.phymed.2010.01.001
Fetsch, P. A., Abati, A., Litman, T., Morisaki, K., Honjo, Y., Mittal, K., et al. (2006). Localization of the ABCG2 mitoxantrone resistance-associated protein in normal tissues. Cancer Lett. 235, 84–92. doi: 10.1016/j.canlet.2005.04.024
Fletcher, J. I., Haber, M., Henderson, M. J., and Norris, M. D. (2010). ABC transporters in cancer: more than just drug efflux pumps. Nat. Rev. Cancer 10, 147–156. doi: 10.1038/nrc2789
Guo, H. Q., Zhang, G. N., Wang, Y. J., Zhang, Y. K., Sodani, K., Talele, T. T., et al. (2014). beta-Elemene, a compound derived from Rhizoma zedoariae, reverses multidrug resistance mediated by the ABCB1 transporter. Oncol. Rep. 31, 858–866. doi: 10.3892/or.2013.2870
Hirschmann-Jax, C., Foster, A. E., Wulf, G. G., Nuchtern, J. G., Jax, T. W., Gobel, U., et al. (2004). A distinct “side population” of cells with high drug efflux capacity in human tumor cells. Proc. Natl. Acad. Sci. U.S.A. 101, 14228–14233. doi: 10.1073/pnas.0400067101
Ho, M. M., Hogge, D. E., and Ling, V. (2008). MDR1 and BCRP1 expression in leukemic progenitors correlates with chemotherapy response in acute myeloid leukemia. Exp. Hematol. 36, 433–442. doi: 10.1016/j.exphem.2007.11.014
Honjo, Y., Hrycyna, C. A., Yan, Q. W., Medina-Perez, W. Y., Robey, R. W., van de Laar, A., et al. (2001). Acquired mutations in the MXR/BCRP/ABCP gene alter substrate specificity in MXR/BCRP/ABCP-overexpressing cells. Cancer Res. 61, 6635–6639.
Jackson, S. M., Manolaridis, I., Kowal, J., Zechner, M., Taylor, N., Bause, M., et al. (2018). Structural basis of small-molecule inhibition of human multidrug transporter ABCG2. Nat. Struct. Mol. Biol. 25, 333–340. doi: 10.1038/s41594-018-0049-1
Jonker, J. W., Buitelaar, M., Wagenaar, E., Van Der Valk, M. A., Scheffer, G. L., Scheper, R. J., et al. (2002). The breast cancer resistance protein protects against a major chlorophyll-derived dietary phototoxin and protoporphyria. Proc. Natl. Acad. Sci. U.S.A. 99, 15649–15654. doi: 10.1073/pnas.202607599
Kanzaki, A., Toi, M., Nakayama, K., Bando, H., Mutoh, M., Uchida, T., et al. (2001). Expression of multidrug resistance-related transporters in human breast carcinoma. Jpn. J. Cancer Res. 92, 452–458. doi: 10.1111/j.1349-7006.2001.tb01115.x
Lee, M. J., Maliakal, P., Chen, L., Meng, X., Bondoc, F. Y., Prabhu, S., et al. (2002). Pharmacokinetics of tea catechins after ingestion of green tea and (-)-epigallocatechin-3-gallate by humans: formation of different metabolites and individual variability. Cancer Epidemiol. Biomarkers Prev. 11, 1025–1032.
Liang, G., Tang, A., Lin, X., Li, L., Zhang, S., Huang, Z., et al. (2010). Green tea catechins augment the antitumor activity of doxorubicin in an in vivo mouse model for chemoresistant liver cancer. Int. J. Oncol. 37, 111–123.
Locher, K. P. (2004). Structure and mechanism of ABC transporters. Curr. Opin. Struct. Biol. 14, 426–431. doi: 10.1016/j.sbi.2004.06.005
Locher, K. P., and Borths, E. (2004). ABC transporter architecture and mechanism: implications from the crystal structures of BtuCD and BtuF. FEBS Lett. 564, 264–268. doi: 10.1016/S0014-5793(04)00289-3
Maliepaard, M., Scheffer, G. L., Faneyte, I. F., van Gastelen, M. A., Pijnenborg, A. C., Schinkel, A. H., et al. (2001). Subcellular localization and distribution of the breast cancer resistance protein transporter in normal human tissues. Cancer Res. 61, 3458–3464.
Mao, Q., and Unadkat, J. D. (2005). Role of the breast cancer resistance protein (ABCG2) in drug transport. AAPS J. 7, E118–E133. doi: 10.1208/aapsj070112
Miyake, K., Mickley, L., Litman, T., Zhan, Z., Robey, R., Cristensen, B., et al. (1999). Molecular cloning of cDNAs which are highly overexpressed in mitoxantrone-resistant cells: demonstration of homology to ABC transport genes. Cancer Res. 59, 8–13.
Nakanishi, T., Doyle, L. A., Hassel, B., Wei, Y., Bauer, K. S., Wu, S., et al. (2003). Functional characterization of human breast cancer resistance protein (BCRP, ABCG2) expressed in the oocytes of Xenopus laevis. Mol. Pharmacol. 64, 1452–1462. doi: 10.1124/mol.64.6.1452
Nakanishi, T., and Ross, D. D. (2012). Breast cancer resistance protein (BCRP/ABCG2): its role in multidrug resistance and regulation of its gene expression. Chin. J. Cancer 31, 73–99. doi: 10.5732/cjc.011.10320
Ozvegy, C., Litman, T., Szakacs, G., Nagy, Z., Bates, S., Varadi, A., et al. (2001). Functional characterization of the human multidrug transporter, ABCG2, expressed in insect cells. Biochem. Biophys. Res. Commun. 285, 111–117. doi: 10.1006/bbrc.2001.5130
Patel, A., Tiwari, A. K., Chufan, E. E., Sodani, K., Anreddy, N., Singh, S., et al. (2013). PD173074, a selective FGFR inhibitor, reverses ABCB1-mediated drug resistance in cancer cells. Cancer Chemother. Pharmacol. 72, 189–199. doi: 10.1007/s00280-013-2184-z
Polgar, O., Robey, R. W., and Bates, S. E. (2008). ABCG2: structure, function and role in drug response. Expert Opin. Drug Metab. Toxicol. 4, 1–15. doi: 10.1517/17425255.4.1.1
Pozza, A., Perez-Victoria, J. M., Sardo, A., Ahmed-Belkacem, A., and Di Pietro, A. (2006). Purification of breast cancer resistance protein ABCG2 and role of arginine-482. Cell. Mol. Life Sci. 63, 1912–1922. doi: 10.1007/s00018-006-6159-7
Rabindran, S. K., He, H., Singh, M., Brown, E., Collins, K. I., Annable, T., et al. (1998). Reversal of a novel multidrug resistance mechanism in human colon carcinoma cells by fumitremorgin C. Cancer Res. 58, 5850–5858.
Robey, R. W., Honjo, Y., Morisaki, K., Nadjem, T. A., Runge, S., Risbood, M., et al. (2003). Mutations at amino-acid 482 in the ABCG2 gene affect substrate and antagonist specificity. Br. J. Cancer 89, 1971–1978. doi: 10.1038/sj.bjc.6601370
Sargent, J. M., Williamson, C. J., Maliepaard, M., Elgie, A. W., Scheper, R. J., and Taylor, C. G. (2001). Breast cancer resistance protein expression and resistance to daunorubicin in blast cells from patients with acute myeloid leukaemia. Br. J. Haematol. 115, 257–262. doi: 10.1046/j.1365-2141.2001.03122.x
Sodani, K., Patel, A., Kathawala, R. J., and Chen, Z. S. (2012). Multidrug resistance associated proteins in multidrug resistance. Chin. J. Cancer 31, 58–72. doi: 10.5732/cjc.011.10329
Steinbach, D., Sell, W., Voigt, A., Hermann, J., Zintl, F., and Sauerbrey, A. (2002). BCRP gene expression is associated with a poor response to remission induction therapy in childhood acute myeloid leukemia. Leukemia 16, 1443–1447. doi: 10.1038/sj.leu.2402541
Sun, Y. L., Kathawala, R. J., Singh, S., Zheng, K., Talele, T. T., Jiang, W. Q., et al. (2012). Zafirlukast antagonizes ATP-binding cassette subfamily G member 2-mediated multidrug resistance. Anticancer Drugs 23, 865–873. doi: 10.1097/CAD.0b013e328354a196
Suvannasankha, A., Minderman, H., O’Loughlin, K. L., Nakanishi, T., Ford, L. A., Greco, W. R., et al. (2004). Breast cancer resistance protein (BCRP/MXR/ABCG2) in adult acute lymphoblastic leukaemia: frequent expression and possible correlation with shorter disease-free survival. Br. J. Haematol. 127, 392–398. doi: 10.1111/j.1365-2141.2004.05211.x
Taylor, N., Manolaridis, I., Jackson, S. M., Kowal, J., Stahlberg, H., and Locher, K. P. (2017). Structure of the human multidrug transporter ABCG2. Nature 546, 504–509. doi: 10.1038/nature22345
van den Heuvel-Eibrink, M. M., van der Holt, B., Burnett, A. K., Knauf, W. U., Fey, M. F., Verhoef, G. E., et al. (2007). CD34-related coexpression of MDR1 and BCRP indicates a clinically resistant phenotype in patients with acute myeloid leukemia (AML) of older age. Ann. Hematol. 86, 329–337. doi: 10.1007/s00277-007-0269-7
van den Heuvel-Eibrink, M. M., Wiemer, E. A., Prins, A., Meijerink, J. P., Vossebeld, P. J., van der Holt, B., et al. (2002). Increased expression of the breast cancer resistance protein (BCRP) in relapsed or refractory acute myeloid leukemia (AML). Leukemia 16, 833–839. doi: 10.1038/sj.leu.2402496
Wen, Y., Zhao, R. Q., Zhang, Y. K., Gupta, P., Fu, L. X., Tang, A. Z., et al. (2017). Effect of Y6, an epigallocatechin gallate derivative, on reversing doxorubicin drug resistance in human hepatocellular carcinoma cells. Oncotarget 8, 29760–29770. doi: 10.18632/oncotarget.15964
Zhang, Y. K., Zhang, G. N., Wang, Y. J., Patel, B. A., Talele, T. T., Yang, D. H., et al. (2016). Bafetinib (INNO-406) reverses multidrug resistance by inhibiting the efflux function of ABCB1 and ABCG2 transporters. Sci. Rep. 6:25694. doi: 10.1038/srep25694
Keywords: epigallocatechin gallate (EGCG), 5, 3′, 4′, 3″, 4″, 5″-6-O-ethyl-EGCG (Y6), ABC transporter, ABCG2, multidrug resistance
Citation: Zhao R-Q, Wen Y, Gupta P, Lei Z-N, Cai C-Y, Liang G, Yang D-H, Chen Z-S and Xie Y-A (2019) Y6, an Epigallocatechin Gallate Derivative, Reverses ABCG2-Mediated Mitoxantrone Resistance. Front. Pharmacol. 9:1545. doi: 10.3389/fphar.2018.01545
Received: 05 September 2018; Accepted: 18 December 2018;
Published: 10 January 2019.
Edited by:
Salvatore Salomone, Università degli Studi di Catania, ItalyReviewed by:
Jun Lin, Stony Brook University, United StatesIan Kerr, The University of Nottingham, United Kingdom
Kenneth K. W. To, The Chinese University of Hong Kong, China
Copyright © 2019 Zhao, Wen, Gupta, Lei, Cai, Liang, Yang, Chen and Xie. This is an open-access article distributed under the terms of the Creative Commons Attribution License (CC BY). The use, distribution or reproduction in other forums is permitted, provided the original author(s) and the copyright owner(s) are credited and that the original publication in this journal is cited, in accordance with accepted academic practice. No use, distribution or reproduction is permitted which does not comply with these terms.
*Correspondence: Zhe-Sheng Chen, chenz@stjohns.edu Yu-An Xie, gxxya@aliyun.com
†These authors have contributed equally to this work