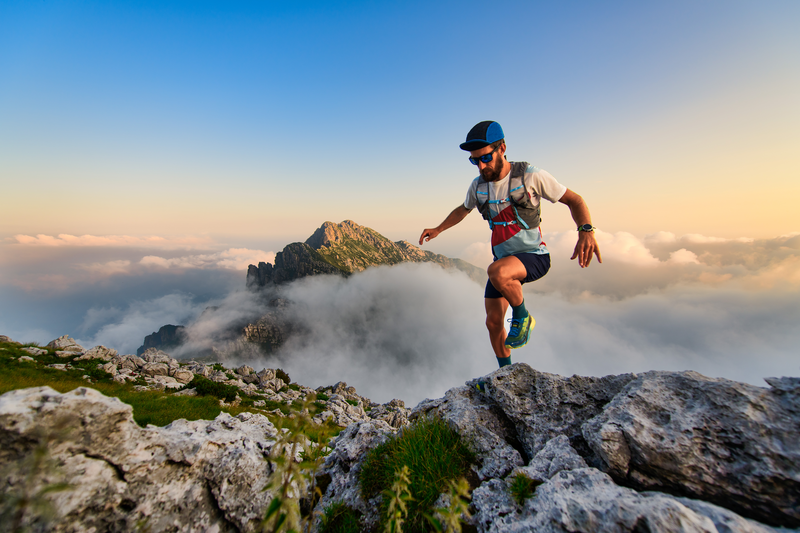
94% of researchers rate our articles as excellent or good
Learn more about the work of our research integrity team to safeguard the quality of each article we publish.
Find out more
ORIGINAL RESEARCH article
Front. Pharmacol. , 26 November 2018
Sec. Drug Metabolism and Transport
Volume 9 - 2018 | https://doi.org/10.3389/fphar.2018.01365
Background: Cannabidiol is being pursued as a therapeutic treatment for multiple conditions, usually by oral delivery. Animal studies suggest oral bioavailability is low, but literature in humans is not sufficient. The aim of this review was to collate published data in this area.
Methods: A systematic search of PubMed and EMBASE (including MEDLINE) was conducted to retrieve all articles reporting pharmacokinetic data of CBD in humans.
Results: Of 792 articles retireved, 24 included pharmacokinetic parameters in humans. The half-life of cannabidiol was reported between 1.4 and 10.9 h after oromucosal spray, 2–5 days after chronic oral administration, 24 h after i.v., and 31 h after smoking. Bioavailability following smoking was 31% however no other studies attempted to report the absolute bioavailability of CBD following other routes in humans, despite i.v formulations being available. The area-under-the-curve and Cmax increase in dose-dependent manners and are reached quicker following smoking/inhalation compared to oral/oromucosal routes. Cmax is increased during fed states and in lipid formulations. Tmax is reached between 0 and 4 h.
Conclusions: This review highlights the paucity in data and some discrepancy in the pharmacokinetics of cannabidiol, despite its widespread use in humans. Analysis and understanding of properties such as bioavailability and half-life is critical to future therapeutic success, and robust data from a variety of formulations is required.
The Cannabis sativa plant contains more than a hundred phytocannabinoid compounds, including the non-psychotomimetic compound cannabidiol (CBD) (Izzo et al., 2009). CBD has attracted significant interest due to its anti-inflammatory, anti-oxidative and anti-necrotic protective effects, as well as displaying a favorable safety and tolerability profile in humans (Bergamaschi et al., 2011), making it a promising candidate in many therapeutic avenues including epilepsy, Alzheimer's disease, Parkinson's disease, and multiple sclerosis. GW pharmaceuticals have developed an oral solution of pure CBD (Epidiolex®) for the treatment of severe, orphan, early-onset, treatment-resistant epilepsy syndromes, showing significant reductions in seizure frequency compared to placebo in several trials (Devinsky et al., 2017, 2018a; Thiele et al., 2018). Epidiolex® has recently received US Food and Drug Administration (FDA) approval (GW Pharmaceuticals, 2018). CBD is also being pursued in clinical trials in Parkinson's disease, Crohn's disease, society anxiety disorder, and schizophrenia (Crippa et al., 2011; Leweke et al., 2012; Chagas et al., 2014; Naftali et al., 2017), showing promise in these areas. Additionally, CBD is widely used as a popular food supplement in a variety of formats for a range of complaints. It is estimated that the CBD market will grow to $2.1 billion in the US market in consumer sales by 2020 (Hemp Business, 2017).
From previous investigations including animal studies, the oral bioavailability of CBD has been shown to be very low (13–19%) (Mechoulam et al., 2002). It undergoes extensive first pass metabolism and its metabolites are mostly excreted via the kidneys (Huestis, 2007). Plasma and brain concentrations are dose-dependent in animals, and bioavailability is increased with various lipid formulations (Zgair et al., 2016). However, despite the breadth of use of CBD in humans, there is little data on its pharmacokinetics (PK). Analysis and understanding of the PK properties of CBD is critical to its future use as a therapeutic compound in a wide range of clinical settings, particularly regarding dosing regimens and routes of administration. Therefore, the aim of this systematic review was to collate and analyse all available CBD PK data recorded in humans and to highlight gaps in the literature.
The systematic review was carried out in accordance with PRISMA (Preferred Reporting Items for Systematic Reviews and Meta-Analyses) guidelines (Moher et al., 2009). A systematic search of PubMed and EMBASE (including MEDLINE) was conducted to retrieve all articles reporting pharmacokinetic data of CBD in humans. Search terms included: CBD, cannabidiol, Epidiolex, pharmacokinetics, Cmax, plasma concentrations, plasma levels, half-life, peak concentrations, absorption, bioavailability, AUC, Tmax, Cmin, and apparent volume of distribution. No restrictions were applied to type of study, publication year, or language. The searches were carried out by 14 March 2018 by two independent researchers.
The titles and abstracts of retrieved studies were examined by two independent researchers, and inappropriate articles were rejected. Inclusion criteria were as follows: an original, peer-reviewed paper that involved administration of CBD to humans, and included at least one pharmacokinetic measurement as listed in the search strategy.
The included articles were analyzed, and the following data extracted: sample size, gender, administration route of CBD, source of CBD, dose of CBD, and any pharmacokinetic details. Where available, plasma mean or median Cmax (ng/mL) were plotted against CBD dose (mg). Similarly, mean or median Tmax and range, and mean or median area under the curve (AUC0−t) and SD were plotted against CBD dose (mg). The source/supplier of the CBD was also recorded. No further statistical analysis was possible due to sparsity of data and heterogeneity of populations used. All studies were assessed for quality using an amended version of the National Institute for Health (NIH), National Heart, Lung and Blood Institute, Quality Assessment Tool for Before-After (Pre-Post) Studies with No Control Group (National Institute for Health, 2014). A sample size of ≤ 10 was considered poor, between 11 and 19 was considered fair, and ≥20 was considered good (Ogungbenro et al., 2006).
Tmax: Time to the maximum measured plasma concentration.
Cmax: Maximum measured plasma concentration over the time span specified.
t1/2: Final time taken for the plasma concentration to be reduced by half.
AUC0−t: The area under the plasma concentration vs. time curve, from time zero to “t.”
AUC0−inf: The area under the plasma concentration vs. time curve from zero to t calculated as AUC0−t plus the extrapolated amount from time t to infinity.
Kel: The first-order final elimination rate constant.
In total, 792 records were retrieved from the database searching, 24 of which met the eligibility criteria (Figure 1). Table 1 summarizes each included study. Routes of administration included intravenous (i.v.) (n = 1), oromucosal spray (n = 21), oral capsules (n = 13), oral drops (n = 2), oral solutions (n = 1), nebuliser (n = 1), aerosol (n = 1), vaporization (n = 1), and smoking (n = 8). CBD was administered on its own in 9 publications, and in combination with THC or within a cannabis extract in the remainder. One study was conducted in children with Dravet syndrome, while the remainder were conducted in healthy adult volunteers (Devinsky et al., 2018b). Overall, the included studies were of good quality (Supplementary Table 1). However, many studies had small sample sizes. Additionally, not all studies included both males and females, and frequent cannabis smokers were included in a number of studies. Thus, interpretation and extrapolation of these results should be done with caution.
Within the 25 included studies, Cmax was reported on 58 occasions (for example within different volunteer groups or doses in a single study), Tmax on 56 occasions and area under the curve (AUC0−t) on 45 occasions. These data from plasma/blood are presented in Figures 2A–C. The AUC0−t and Cmax of CBD is dose-dependent, and Tmax occurs between 0 and 5 h, but does not appear to be dose-dependent.
Figure 2. (A) Mean or median Tmax (h) and range against CBD dose (mg) (B) mean or median area under the curve (AUC0-t) (h × ng/mL) and SD against CBD dose (mg) and (C) plasma mean or median concentration max (Cmax; ng/mL) against CBD dose (mg). It was not possible to present error bars for Cmax as SD and SEM were both reported in the data. IV, intravenous; SD, standard deviation; SEM, standard error of the mean.
A number of trials in humans were conducted by Guy and colleagues to explore administration route efficiency of sprays, an aerosol, and a nebuliser containing CBD or CBD and THC (CBD dose 10 or 20 mg) (Guy and Flint, 2004; Guy and Robson, 2004a,b). Oromucosal spray, either buccal, sublingual, or oropharyngeal administration, resulted in mean Cmax between 2.5 and 3.3 ng/mL and mean Tmax between 1.64 and 4.2 h. Sublingual drops resulted in similar Cmax of 2.05 and 2.58 ng/mL and Tmax of 2.17 and 1.67 h, respectively. Other oromucosal single dose studies reported Cmax and Tmax values within similar ranges (Karschner et al., 2011; Atsmon et al., 2017b).
Minimal evidence of plasma accumulation has been reported by chronic dosing studies over 5–9 days (Sellers et al., 2013; Stott et al., 2013a). Cmax appears to be dose-dependent. A dose of 20 mg/day resulted in a mean Cmax of 1.5 ng/mL and mean AUC0−t of 6.1 h × ng/mL while 60 mg/day equated to a mean Cmax of 4.8 ng/mL and AUC0−t was 38.9 h × ng/mL (Sellers et al., 2013). In another study, Cmax increased dose-dependently from 0.4 to 1.2 and 2.2 ng/mL following 5, 10, and 20 mg single doses, respectively, and from 0.5 to 1.1 and 3.2 ng/mL, respectively following chronic dosing over 9 consecutive days (Stott et al., 2013a). There was a significant increase in time-dependent exposure during the chronic treatment. Mean AUC0−t for the single doses were 0.8, 4.5, 9.9, and 2.5, 6.7, and 20.3 for the chronic dosing schedule, respectively. Tmax does not appear to be dose-dependent, nor affected by acute or chronic dosing schedules.
Stott et al. reported an increase in CBD bioavailability under fed vs. fasted states in 12 men after a single 10 mg dose of CBD administered through an oromucosal spray which also contained THC (Stott et al., 2013a,b). Mean AUC and Cmax were 5- and 3-fold higher during fed conditions compared to fasted (AUC0−t 23.1 vs. 4.5; Cmax 3.7 vs. 1.2 ng/mL). Tmax was also delayed under the fed state (4.0 vs. 1.4 h).
In children, Devinsky et al. reported mean AUC as 70, 241, 722, and 963 h × ng/mL in groups receiving 2.5, 5, 10, and 20 mg/Kg/day of CBD in oral solution (Devinsky et al., 2018b).
Cmax and AUC following oral administration also appears to be dose dependent. A dose of 10 mg CBD resulted in mean Cmax of 2.47 ng/mL at 1.27 h, and a dose of 400 or 800 mg co-administered with i.v. fentanyl (a highly potent opioid) to examine its safety resulted in a mean Cmax of 181 ng/mL (at 3.0 h) and 114 ng/mL (at 1.5 h) for 400 mg, and 221 ng/mL (at 3.0 h) and 157 ng/mL (at 4.0 h) for 800 mg, in 2 sessions, respectively (Guy and Robson, 2004b; Manini et al., 2015). A dose of 800 mg oral CBD in a study involving 8 male and female cannabis smokers, reported a mean Cmax of 77.9 ng/mL and mean Tmax of 3.0 h (Haney et al., 2016). Although, an increase in dose corresponds with an increase in Cmax, the Cmax between the higher doses of CBD does not greatly differ, suggesting a saturation effect (e.g., between 400 and 800 mg).
One hour after oral capsule administration containing 5.4 mg CBD in males and females, mean Cmax was reported as 0.93 ng/mL (higher for female participants than male) (Nadulski et al., 2005a). A subset (n = 12) consumed a standard breakfast meal 1 h after the capsules, which slightly increased mean Cmax to 1.13 ng/mL. CBD remained detectable for 3–4 h after administration (Nadulski et al., 2005b).
Cherniakov et al. examined the pharmacokinetic differences between an oromucosal spray and an oral capsule with piperine pro-nanolipospheres (PNL) (both 10 mg CBD) in 9 men. The piperine-PNL oral formulation had a 4-fold increase in Cmax (2.1 ng/mL vs. 0.5 ng/mL), and a 2.2-fold increase in AUC0−t (6.9 vs. 3.1 h × ng/mL), while Tmax was decreased (1.0 vs. 3.0 h) compared to the oromucosal spray (Cherniakov et al., 2017a). This group further developed self-emulsifying formulations and reported again an increased bioavailability and increased Cmax within a shorter time compared to a reference spray (Atsmon et al., 2017a,b).
The highest plasma concentrations of CBD were reported by Ohlsson et al. following i.v. administration of 20 mg of deuterium-labeled CBD. Mean plasma CBD concentrations were reported at 686 ng/mL (3 min post-administration), which dropped to 48 ng/mL at 1 h.
After smoking a cigarette containing 19.2 mg of deuterium-labeled CBD, highest plasma concentrations were reported as 110 ng/mL, 3 min post dose, which dropped to 10.2 ng/ml 1 h later (Ohlsson et al., 1986). Average bioavailability by the smoked route was 31% (Ohlsson et al., 1986). A nebuliser resulted in a Cmax of 9.49 ng/mL which occurred at 0.6 h, whereas aerosol administration produced Cmax (2.6 ng/mL) at 2.35 h (Guy and Flint, 2004). In 10 male and female usual, infrequent cannabis smokers, Cmax was 2.0 ng/mL at 0.25 h after smoking a cigarette containing 2 mg of CBD (Schwope et al., 2011). CBD was detected in 60% of whole blood samples and in 80% of plasma samples at observed Cmax, and no longer detected after 1.0 h. A study in 14 male and female cannabis smokers reported 15.4% detection in frequent smokers with no CBD detected in occasional smokers in whole blood analysis (Desrosiers et al., 2014). In plasma however, there was a 53.8 and 9.1% detection in the frequent and occasional groups, with corresponding Cmax of 1.1 ng/mL in the frequent group, and below limits of detection in the occasional group.
The mean half-life (t1/2) of CBD was reported as 1.1 and 2.4 h following nebuliser and aerosol administration (20 mg) (Guy and Flint, 2004), 1.09 and 1.97 h following single oral administration (10 and 20 mg) (Guy and Flint, 2004; Guy and Robson, 2004b), 2.95 and 3.21 h following 10 mg oral lipid capsules (Atsmon et al., 2017a,b), between 1.44 and 10.86 h after oromucosal spray administration (5–20 mg) (Guy and Robson, 2004b; Sellers et al., 2013; Stott et al., 2013a,b; Atsmon et al., 2017b), 24 h after i.v. infusion, 31 h after smoking (Ohlsson et al., 1986), and 2–5 days after chronic oral administration (Consroe et al., 1991).
Mean elimination rate constant (Kel [1/h]) has been reported as 0.148 in fasted state, and 0.155 in fed state after 10 mg CBD was administered in an oromucosal spray also containing THC (Stott et al., 2013a,b). After single doses of 5 and 20 mg CBD, mean Kel (1/h) was reported as 0.173 and 0.123 (Stott et al., 2013a). Following 20 mg CBD administration through a nebuliser and pressurized aerosol, mean Kel was reported as 0.98 and 0.43, respectively, while 20 mg CBD administered as sublingual drops was reported as 0.37 (Guy and Flint, 2004).
Plasma apparent clearance, CL/F (L/h) has been reported to range from 2,546 to 4,741 in a fasted stated following 10 mg CBD administered via oromucosal spray (Stott et al., 2013a,c). This value decreases to 533 following the same concentration in a fed state (Stott et al., 2013b). A plasma apparent clearance of 3,252 and 3,783 was reported following 5 and 20 mg single doses of CBD via oromucosal spray (Stott et al., 2013a). Ohlsson et al. reported plasma apparent clearance as 74.4 L/h following i.v. injection (Ohlsson et al., 1986).
Mean apparent volume of distribution (V/F [L]) was reported as 2,520 L following i.v. administration (Ohlsson et al., 1986). Following single acute doses through oromucosal spray administration, apparent volume of distribution was reported as 26,298, 31,994, and 28,312 L (Stott et al., 2013a).
The aim of this study was to review and analyse all available PK data on CBD in humans. Only 8 publications reported PK parameters after administering CBD on its own, and the others were in combination with THC/cannabis. Only 1 study reported the bioavailability of CBD in humans (31% following smoking). From the analysis of these papers, the following observations were made; peak plasma concentrations and area under the curve (AUC) are dose-dependent and show minimal accumulation; Cmax is increased and reached faster following i.v., smoking or inhalation; Cmax is increased and reached faster after oral administration in a fed state or in a pro-nanoliposphere formulation; Tmax does not appear to be dose-dependent; and half-life depends on dose and route of administration. Overall, considerable variation was observed between studies, although they were very heterogeneous, and further work is warranted.
Human studies administering CBD showed that the AUC0−t and Cmax are dose-dependent, and Tmax mostly occurred between 1 and 4 h. Animal studies in piglets, mice, and rats also all demonstrate a dose-dependent relationship between CBD and both plasma and brain concentrations (Long et al., 2012; Hammell et al., 2016; Garberg et al., 2017), suggesting that human brain concentrations will also be dose-dependent. Ten publications in this review reported the half-life of CBD which ranged from 1 h to 5 days and varies depending on the dose and route of administration. Very limited data was available for detailed analysis on the elimination rate, apparent clearance or distribution of CBD in humans.
Plasma levels of CBD were increased when CBD was administered with food or in a fed state, or when a meal is consumed post-administration. Oral capsules with piperine pro-nanolipospheres also increased AUC and Cmax. This is also demonstrated in animal studies; co-administration of lipids with oral CBD increased systemic availability by almost 3-fold in rats (Zgair et al., 2016) and a pro-nanoliposphere formulation increased oral bioavailability by about 6-fold (Cherniakov et al., 2017b). As CBD is a highly lipophilic molecule, it is logical that CBD may dissolve in the fat content of food, increasing its solubility, and absorption and therefore bioavailability as demonstrated by numerous pharmacological drugs (Winter et al., 2013). Thus, it may be advisable to administer CBD orally in a fed state to allow for optimal absorption.
Only one study used intravenous administration of CBD and reported PK details, which could be a beneficial route of administration in some acute indications. Results from other routes such as rectal, transdermal, or intraperitoneal have also not been published in humans, although transdermal CBD gel and topical creams have been demonstrated to be successful in animal studies (Giacoppo et al., 2015; Hammell et al., 2016). Interestingly, intraperitoneal (i.p.) injection of CBD corresponded to higher plasma and brain concentrations than oral administration in mice, however in rats, similar concentrations were observed for both administration routes, and brain concentrations were in fact higher following oral compared to i.p. route (Deiana et al., 2012). No published data exists on the tissue distribution of CBD in humans. Although plasma levels of CBD do not show accumulation with repeated dosing, it is possible that there may be tissue accumulation.
Only one study in this review was conducted in children (n = 34) (Devinsky et al., 2018b). Children (4–10 years) with Dravet syndrome were administered an oral solution of CBD and AUC was reported to increase dose-dependently. It is important to emphasize the statement that children are not small adults, and there are many differences in their pharmacokinetic and pharmacodynamic profiles. Absorption, excretion, metabolism, and plasma protein binding are generally reduced in children compared to adults, and apparent volume of distribution is generally increased (Fernandez et al., 2011). These parameters need to be explored fully for CBD in order to understand and advise dose adjustments.
Within the adult studies, inter- and intra-subject variability was observed in studies, and it remains to be seen whether i.v. and other routes of administration that by-pass initial metabolism will alleviate this issue. Interestingly, although each of the subject's weight was taken into account, none of the studies addressed subject fat content as a factor in their exclusion criteria; as muscle can weigh more than the same proportion of fat. It is well-known that cannabinoids are highly lipophilic compounds and accumulate in fatty tissue which can then be released gradually (Gunasekaran et al., 2009). It may be of benefit in future study to either put in place more stringent exclusion criteria and measure subject fat content or assess the possible accumulation of CBD in fatty tissue. Differences in metabolism, distribution and accumulation in fat, and in biliary and renal elimination may be responsible for prolonged elimination half-life and variable pharmacokinetic outcomes. CBD use is widespread and has been recommended for use by the FDA in childhood-onset epilepsy. CBD also displays therapeutic promise in other disorders such as schizophrenia and post-traumatic stress disorder. If we are to understand the actions of CBD in those disorders and increase the success rate for treatment, these groups of patients and their distinct characteristics must be assessed as they may not be comparable to a healthy volunteer population.
A systematic review in 2014 concluded that CBD generally has a low risk of clinically significant drug-interactions (Stout and Cimino, 2014). A few studies in the current review included examination of drug-drug interactions with CBD. GW Pharmaceuticals performed a clinical trial investigating the pharmacokinetic interaction between CBD/THC spray (sativex) and rifampicin (cytochrome P450 inducer), ketoconazole, and omeprazole (cytochrome P450 inhibitors) (Stott et al., 2013c). Authors concluded overall that CBD in combination with the drugs were well-tolerated, but consideration should be noted when co-administering with other drugs using the CYP3A4 pathway. Caution is also advised with concomitant use of CBD and substrates of UDP-glucuronosyltransferases UGT1A9 and UGT2B7, and other drugs metabolized by the CYP2C19 enzyme (Al Saabi et al., 2013; Jiang et al., 2013). Manini et al. co-administered CBD with i.v. fentanyl (a high potency opioid) which was reported as safe and well-tolerated (Manini et al., 2015). In a number of trials with CBD in children with severe epilepsy, clobazam concentrations increased when CBD was co-administered and dosage of clobazam had to be reduced in some patients in one study (Geffrey et al., 2015; Devinsky et al., 2018b). Gaston and colleagues performed a safety study in adults and children in which CBD was administered with commonly-used anti-epileptic drugs (AEDs) (Gaston et al., 2017). Most changes in AED concentrations were within acceptable ranges but abnormal liver function tests were reported in those taking valproate and authors emphasized the importance of continued monitoring of AED concentrations and liver function during treatment with CBD.
Limitations of this review should be acknowledged. Different population types including healthy and patient populations and cannabis naïve or not were all grouped together which may impede generalizability. The proportions of men and women in each study were also not uniform, and it is still being elucidated whether men and women have distinct pharmacokinetic profiles with regards to cannabinoids (Fattore and Fratta, 2010). One study suggested that the PK of CBD was different in their female volunteers (Nadulski et al., 2005a). It should also be mentioned that CBD is currently not an approved product with a pharmacopeia entry so using different sources of CBD that are subject to different polymeric forms, different particle sizes, and different purities may also affect the PK profiles observed. It is important for future work that researchers record the source of the CBD material used so that results have the highest chance of being replicated. Despite a thorough search of the two databases chosen, the addition of more databases may have widened the search to increase the number of results and hence improve the reliability and validity of the findings. However, the review was carried out by two independent reviewers, and searches generated were analyzed separately and then compared.
In conclusion, this review demonstrates the lack of research in this area, particularly in routes of administration other than oral. An absence of studies has led to failure in addressing the bioavailability of CBD despite intravenous formulations being available. This is of critical importance due to the popularity of CBD products and will help interpret other PK values. Standardized and robust formulations of CBD and their PK data are required for both genders, with consideration of other factors such as adiposity, genetic factors that might influence absorption and metabolism, and the effects of disease states.
SM, SO, and AY: substantial contributions to the conception or design of the work. SM: writing of the manuscript. SM and NS: database searching and data extraction. All authors: the analysis and interpretation of data for the work; drafting the work or revising it critically for important intellectual content; final approval of the version to be published; agreement to be accountable for all aspects of the work in ensuring that questions related to the accuracy or integrity of any part of the work are appropriately investigated and resolved.
This work was supported by the Biotechnology and Biological Sciences Research Council [Grant number BB/M008770/1].
AY was employed by company Artelo Biosciences.
The remaining authors declare that the research was conducted in the absence of any commercial or financial relationships that could be construed as a potential conflict of interest.
The Supplementary Material for this article can be found online at: https://www.frontiersin.org/articles/10.3389/fphar.2018.01365/full#supplementary-material
Al Saabi, A., Allorge, D., Sauvage, F. L., Tournel, G., Gaulier, J. M., Marquet, P., et al. (2013). Involvement of UDP-glucuronosyltransferases UGT1A9 and UGT2B7 in ethanol glucuronidation, and interactions with common drugs of abuse. Drug Metab. Dispos. 41, 568–574. doi: 10.1124/dmd.112.047878
Atsmon, J., Cherniakov, I., Izgelov, D., Hoffman, A., Domb, A. J., Deutsch, L., et al. (2017a). PTL401, a new formulation based on pro-nano dispersion technology, improves oral cannabinoids bioavailability in healthy volunteers. J. Pharm. Sci. 107, 1423–1429. doi: 10.1016/j.xphs.2017.12.020
Atsmon, J., Heffetz, D., Deutsch, L., Deutsch, F., and Sacks, H. (2017b). Single-Dose pharmacokinetics of oral cannabidiol following administration of PTL101: a new formulation based on gelatin matrix pellets technology. Clin. Pharmacol. Drug Dev. 7:751–758. doi: 10.1002/cpdd.408
Bergamaschi, M. M., Queiroz, R. H., Zuardi, A. W., and Crippa, J. A. (2011). Safety and side effects of cannabidiol, a Cannabis sativa constituent. Curr. Drug Saf. 6, 237–249. doi: 10.2174/157488611798280924
Chagas, M. H., Zuardi, A. W., Tumas, V., Pena-Pereira, M. A., Sobreira, E. T., Bergamaschi, M. M., et al. (2014). Effects of cannabidiol in the treatment of patients with Parkinson's disease: an exploratory double-blind trial. J. Psychopharmacol. 28, 1088–1098. doi: 10.1177/0269881114550355
Cherniakov, I., Izgelov, D., Barasch, D., Davidson, E., Domb, A. J., and Hoffman, A. (2017a). Piperine-pro-nanolipospheres as a novel oral delivery system of cannabinoids: pharmacokinetic evaluation in healthy volunteers in comparison to buccal spray administration. J. Control. Release 266, 1–7. doi: 10.1016/j.jconrel.2017.09.011
Cherniakov, I., Izgelov, D., Domb, A. J., and Hoffman, A. (2017b). The effect of Pro NanoLipospheres (PNL) formulation containing natural absorption enhancers on the oral bioavailability of delta-9-tetrahydrocannabinol (THC) and cannabidiol (CBD) in a rat model. Eur. J. Pharm. Sci. 109, 21–30. doi: 10.1016/j.ejps.2017.07.003
Consroe, P., Kennedy, K., and Schram, K. (1991). Assay of plasma cannabidiol by capillary gas chromatography/ion trap mass spectroscopy following high-dose repeated daily oral administration in humans. Pharmacol. Biochem. Behav. 40, 517–522. doi: 10.1016/0091-3057(91)90357-8
Crippa, J. A., Derenusson, G. N., Ferrari, T. B., Wichert-Ana, L., Duran, F. L., Martin-Santos, R., et al. (2011). Neural basis of anxiolytic effects of cannabidiol (CBD) in generalized social anxiety disorder: a preliminary report. J. Psychopharmacol. 25, 121–130. doi: 10.1177/0269881110379283
Deiana, S., Watanabe, A., Yamasaki, Y., Amada, N., Arthur, M., Fleming, S., et al. (2012)Plasma brain pharmacokinetic profile of cannabidiol (CBD), cannabidivarine (CBDV), Delta(9)-tetrahydrocannabivarin (THCV) and cannabigerol (CBG) in rats and mice following oral and intraperitoneal administration CBD action on obsessive-compulsive behaviour. Psychopharmacology 219, 859–873. doi: 10.1007/s00213-011-2415-0
Desrosiers, N. A., Himes, S. K., Scheidweiler, K. B., Concheiro-Guisan, M., Gorelick, D. A., and Huestis, M. A. (2014). Phase I and II cannabinoid disposition in blood and plasma of occasional and frequent smokers following controlled smoked cannabis. Clin. Chem. 60, 631–643. doi: 10.1373/clinchem.2013.216507
Devinsky, O., Cross, J. H., and Wright, S. (2017). Trial of Cannabidiol for drug-resistant seizures in the Dravet Syndrome. N. Engl. J. Med. 377, 699–700. doi: 10.1056/NEJMc1708349
Devinsky, O., Patel, A. D., Cross, J. H., Villanueva, V., Wirrell, E. C., Privitera, M., et al. (2018a). Effect of Cannabidiol on drop seizures in the Lennox-Gastaut Syndrome. N. Engl. J. Med. 378, 1888–1897. doi: 10.1056/NEJMoa1714631
Devinsky, O., Patel, A. D., Thiele, E. A., Wong, M. H., Appleton, R., Harden, C. L., et al. (2018b). Randomized, dose-ranging safety trial of cannabidiol in Dravet syndrome. Neurology 90, e1204–e1211. doi: 10.1212/WNL.0000000000005254
Eichler, M., Spinedi, L., Unfer-Grauwiler, S., Bodmer, M., Surber, C., Luedi, M., et al. (2012). Heat exposure of Cannabis sativa extracts affects the pharmacokinetic and metabolic profile in healthy male subjects. Planta Med. 78, 686–691. doi: 10.1055/s-0031-1298334
Fattore, L., and Fratta, W. (2010). How important are sex differences in cannabinoid action? Br. J. Pharmacol. 160, 544–548. doi: 10.1111/j.1476-5381.2010.00776.x
Fernandez, E., Perez, R., Hernandez, A., Tejada, P., Arteta, M., and Ramos, J. T. (2011). Factors and mechanisms for pharmacokinetic differences between pediatric population and adults. Pharmaceutics 3, 53–72. doi: 10.3390/pharmaceutics3010053
Garberg, H. T., Solberg, R., Barlinn, J., Martinez-Orgado, J., Loberg, E. M., and Saugstad, O. D. (2017). High-dose cannabidiol induced hypotension after global hypoxia-ischemia in piglets. Neonatology 112, 143–149. doi: 10.1159/000471786
Gaston, T. E., Bebin, E. M., Cutter, G. R., Liu, Y., and Szaflarski, J. P. (2017). Interactions between cannabidiol and commonly used antiepileptic drugs. Epilepsia 58, 1586–1592. doi: 10.1111/epi.13852
Geffrey, A. L., Pollack, S. F., Bruno, P. L., and Thiele, E. A. (2015). Drug-drug interaction between clobazam and cannabidiol in children with refractory epilepsy. Epilepsia 56, 1246–1251. doi: 10.1111/epi.13060
Giacoppo, S., Galuppo, M., Pollastro, F., Grassi, G., Bramanti, P., and Mazzon, E. (2015). A new formulation of cannabidiol in cream shows therapeutic effects in a mouse model of experimental autoimmune encephalomyelitis. Daru 23:48. doi: 10.1186/s40199-015-0131-8
Gunasekaran, N., Long, L., Dawson, B., Hansen, G., Richardson, D., Li, K., et al. (2009). Reintoxication: the release of fat-stored Δ9-tetrahydrocannabinol (THC) into blood is enhanced by food deprivation or ACTH exposure. Br. J. Pharmacol. 158, 1330–1337. doi: 10.1111/j.1476-5381.2009.00399.x
Guy, G. W., and Flint, M. E. (2004). A single centre, placebo-controlled, four period, crossover, tolerability study assessing, pharmacodynamic effects, pharmacokinetic characteristics and cognitive profiles of a single dose of three formulations of Cannabis Based Medicine Extracts (CBMEs) (GWPD9901), plus a two period tolerability study comparing pharmacodynamic effects and pharmacokinetic characteristics of a single dose of a cannabis based medicine extract given via two administration routes (GWPD9901 EXT). J. Cannabis Ther. 3, 35–77. doi: 10.1300/J175v03n03_03
Guy, G. W., and Robson, P. J. (2004a). A phase I, double blind, three-way crossover study to assess the pharmacokinetic profile of Cannabis Based Medicine Extract (CBME) administered sublingually in variant cannabinoid ratios in normal healthy male volunteers. J. Cannabis Ther. 3, 121–152. doi: 10.1300/J175v03n04_02
Guy, G. W., and Robson, P. J. (2004b). A phase I, open label, four-way crossover study to compare the pharmacokinetic profiles of a single dose of 20 mg of a Cannabis Based Medicine Extract (CBME) Administered on 3 different areas of the buccal mucosa and to investigate the pharmacokinetics of CBME per Oral in Healthy Male and Female Volunteers (GWPK0112). J. Cannabis Ther. 3, 79–120. doi: 10.1300/J175v03n04_01
GW Pharmaceuticals (2018). Subsidiary Greenwich Biosciences Announce FDA Approval of EPIDIOLEX® (Cannabidiol) Oral Solution–The First Plant-Derived Cannabinoid Prescription Medicine.
Hammell, D. C., Zhang, L. P., Ma, F., Abshire, S. M., McIlwrath, S. L., Stinchcomb, A. L., et al. (2016). Transdermal cannabidiol reduces inflammation and pain-related behaviours in a rat model of arthritis. Eur. J. Pain 20, 936–948. doi: 10.1002/ejp.818
Haney, M., Malcolm, R. J., Babalonis, S., Nuzzo, P. A., Cooper, Z. D., Bedi, G., et al. (2016). Oral cannabidiol does not alter the subjective, reinforcing or cardiovascular effects of smoked cannabis. Neuropsychopharmacology 41, 1974–1982. doi: 10.1038/npp.2015.367
Huestis, M. A. (2007). Human cannabinoid pharmacokinetics. Chem. Biodivers. 4, 1770–1804. doi: 10.1002/cbdv.200790152
Izzo, A. A., Borrelli, F., Capasso, R., Di Marzo, V., and Mechoulam, R. (2009). Non-psychotropic plant cannabinoids: new therapeutic opportunities from an ancient herb. Trends Pharmacol. Sci. 30, 515–527. doi: 10.1016/j.tips.2009.07.006
Jiang, R., Yamaori, S., Okamoto, Y., Yamamoto, I., and Watanabe, K. (2013). Cannabidiol is a potent inhibitor of the catalytic activity of cytochrome P450 2C19. Drug Metab. Pharmacokinet. 28, 332–338. doi: 10.2133/dmpk.DMPK-12-RG-129
Karschner, E. L., Darwin, W. D., Goodwin, R. S., Wright, S., and Huestis, M. A. (2011). Plasma cannabinoid pharmacokinetics following controlled oral Δ9-tetrahydrocannabinol and oromucosal cannabis extract administration. Clin. Chem. 57, 66–75. doi: 10.1373/clinchem.2010.152439
Lee, D., Schwope, D. M., Milman, G., Barnes, A. J., Gorelick, D. A., and Huestis, M. A. (2012). Cannabinoid disposition in oral fluid after controlled smoked cannabis. Clin. Chem. 58, 748–756. doi: 10.1373/clinchem.2011.177881
Leweke, F. M., Piomelli, D., Pahlisch, F., Muhl, D., Gerth, C. W., Hoyer, C., et al. (2012). Cannabidiol enhances anandamide signaling and alleviates psychotic symptoms of schizophrenia. Transl. Psychiatry 2:e94. doi: 10.1038/tp.2012.15
Long, L. E., Chesworth, R., Huang, X. F., Wong, A., Spiro, A., McGregor, I. S., et al. (2012). Distinct neurobehavioural effects of cannabidiol in transmembrane domain neuregulin 1 mutant mice. PLoS ONE 7:e34129. doi: 10.1371/journal.pone.0034129
Manini, A. F., Yiannoulos, G., Bergamaschi, M. M., Hernandez, S., Olmedo, R., Barnes, A. J., et al. (2015). Safety and pharmacokinetics of oral cannabidiol when administered concomitantly with intravenous fentanyl in humans. J. Addict. Med. 9, 204–210. doi: 10.1097/ADM.0000000000000118
Mechoulam, R., Parker, L. A., and Gallily, R. (2002). Cannabidiol: an overview of some pharmacological aspects. J. Clin. Pharmacol. 42, 11S−19S. doi: 10.1002/j.1552-4604.2002.tb05998.x
Moher, D., Liberati, A., Tetzlaff, J., and Altman, D. G. (2009). Preferred reporting items for systematic reviews and meta-analyses: the PRISMA statement. Ann. Int. Med. 151, 264–269, w64. doi: 10.7326/0003-4819-151-4-200908180-00135
Nadulski, T., Pragst, F., Weinberg, G., Roser, P., Schnelle, M., Schnelle, M., Fronk, E. M., et al. (2005a). Randomized, double-blind, placebo-controlled study about the effects of Cannabidiol (CBD) on the pharmacokinetics of D9-Tetrahydrocannabinol (THC) after oral application of THC verses standardized cannabis extract. Ther. Drug Monit. 27, 799–810. doi: 10.1097/01.ftd.0000177223.19294.5c
Nadulski, T., Sporkert, F., Schnelle, M., Stadelmann, A. M., Roser, P., Schefter, T., et al. (2005b). Simultaneous and sensitive analysis of THC, 11-OH-THC, THC-COOH, CBD, and CBN by GC-MS in plasma after oral application of small doses of THC and cannabis extract. J. Anal. Toxicol. 29, 782–789. doi: 10.1093/jat/29.8.782
Naftali, T., Mechulam, R., Marii, A., Gabay, G., Stein, A., Bronshtain, M., et al. (2017). Low-dose cannabidiol is safe but not effective in the treatment for crohn's disease, a randomized controlled trial. Dig. Dis. Sci. 62, 1615–1620. doi: 10.1007/s10620-017-4540-z
National Institute for Health (2014). National Lung and Blood Institute Quality Assessment Tool for Before-After (Pre-Post) Studies with No Control Group. Available online at: https://www.nhlbi.nih.gov/health-topics/study-quality-assessment-tools
Newmeyer, M. N., Desrosiers, N. A., Lee, D., Mendu, D. R., Barnes, A. J., Gorelick, D. A., et al. (2014). Cannabinoid disposition in oral fluid after controlled cannabis smoking in frequent and occasional smokers. Drug Test. Anal. 6, 1002–1010. doi: 10.1002/dta.1632
Ogungbenro, K., Aarons, L., and Graham, G. (2006). Sample size calculations based on generalized estimating equations for population pharmacokinetic experiments. J. Biopharm. Stat. 16, 135–150. doi: 10.1080/10543400500508705
Ohlsson, A., Lindgren, J. E., Andersson, S., Agurell, S., Gillespie, H., and Hollister, L. E. (1986). Single-dose kinetics of deuterium-labelled cannabidiol in man after smoking and intravenous administration. Biomed. Environ. Mass Spectrom. 13, 77–83. doi: 10.1002/bms.1200130206
Schwope, D. M., Karschner, E. L., Gorelick, D. A., and Huestis, M. A. (2011). Identification of recent cannabis use: whole-blood and plasma free and glucuronidated cannabinoid pharmacokinetics following controlled smoked cannabis administration. Clin. Chem. 57, 1406–1414. doi: 10.1373/clinchem.2011.171777
Sellers, E. M., Schoedel, K., Bartlett, C., Romach, M., Russo, E. B., Stott, C. G., et al. (2013). A multiple-dose, randomized, double-blind, placebo-controlled, parallel-group QT/QTc study to evaluate the electrophysiologic effects of THC/CBD spray. Clin. Pharmacol. Drug Dev.2, 285–294. doi: 10.1002/cpdd.36
Stott, C., White, L., Wright, S., Wilbraham, D., and Guy, G. (2013c). A Phase I, open-label, randomized, crossover study in three parallel groups to evaluate the effect of Rifampicin, Ketoconazole, and Omeprazole on the pharmacokinetics of THC/CBD oromucosal spray in healthy volunteers. Springerplus 2:236. doi: 10.1186/2193-1801-2-236
Stott, C. G., White, L., Wright, S., Wilbraham, D., and Guy, G. W. (2013a). A phase I study to assess the single and multiple dose pharmacokinetics of THC/CBD oromucosal spray. Eur. J. Clin. Pharmacol. 69, 1135–1147. doi: 10.1007/s00228-012-1441-0
Stott, C. G., White, L., Wright, S., Wilbraham, D., and Guy, G. W. (2013b). A phase I study to assess the effect of food on the single dose bioavailability of the THC/CBD oromucosal spray. Eur. J. Clin. Pharmacol. 69, 825–834. doi: 10.1007/s00228-012-1393-4
Stout, S. M., and Cimino, N. M. (2014). Exogenous cannabinoids as substrates, inhibitors, and inducers of human drug metabolizing enzymes: a systematic review. Drug Metab. Rev. 46, 86–95. doi: 10.3109/03602532.2013.849268
Swortwood, M. J., Newmeyer, M. N., Andersson, M., Abulseoud, O. A., Scheidweiler, K. B., and Huestis, M. A. (2017). Cannabinoid disposition in oral fluid after controlled smoked, vaporized, and oral cannabis administration. Drug Test. Anal. 9, 905–915. doi: 10.1002/dta.2092
Thiele, E. A., Marsh, E. D., French, J. A., Mazurkiewicz-Beldzinska, M., Benbadis, S. R., Joshi, C., et al. (2018). Cannabidiol in patients with seizures associated with Lennox-Gastaut syndrome (GWPCARE4): a randomised, double-blind, placebo-controlled phase 3 trial. Lancet 391, 1085–1096. doi: 10.1016/S0140-6736(18)30136-3
Winter, H., Ginsberg, A., Egizi, E., Erondu, N., Whitney, K., Pauli, E., et al. (2013). Effect of a high-calorie, high-fat meal on the bioavailability and pharmacokinetics of PA-824 in healthy adult subjects. Antimicrob. Agents Chemother. 57, 5516–5520. doi: 10.1128/AAC.00798-13
Keywords: pharmacokinetics, endocannabinoid system, bioavailability, CMAX, TMAX, half life, plasma clearance, volume of distribution
Citation: Millar SA, Stone NL, Yates AS and O'Sullivan SE (2018) A Systematic Review on the Pharmacokinetics of Cannabidiol in Humans. Front. Pharmacol. 9:1365. doi: 10.3389/fphar.2018.01365
Received: 19 September 2018; Accepted: 07 November 2018;
Published: 26 November 2018.
Edited by:
Thomas Dorlo, The Netherlands Cancer Institute (NKI), NetherlandsReviewed by:
Constantin Mircioiu, Carol Davila University of Medicine and Pharmacy, RomaniaCopyright © 2018 Millar, Stone, Yates and O'Sullivan. This is an open-access article distributed under the terms of the Creative Commons Attribution License (CC BY). The use, distribution or reproduction in other forums is permitted, provided the original author(s) and the copyright owner(s) are credited and that the original publication in this journal is cited, in accordance with accepted academic practice. No use, distribution or reproduction is permitted which does not comply with these terms.
*Correspondence: Sophie A. Millar, c3R4c2FtaWxAbm90dGluZ2hhbS5hYy51aw==
Disclaimer: All claims expressed in this article are solely those of the authors and do not necessarily represent those of their affiliated organizations, or those of the publisher, the editors and the reviewers. Any product that may be evaluated in this article or claim that may be made by its manufacturer is not guaranteed or endorsed by the publisher.
Research integrity at Frontiers
Learn more about the work of our research integrity team to safeguard the quality of each article we publish.