- 1Department of Internal Medicine III, Hematology and Oncology, University Hospital Regensburg, Regensburg, Germany
- 2Department of Surgery, University Hospital Regensburg, Regensburg, Germany
- 3Department Biology, Universita' di Roma Tor Vergata, Rome, Italy
- 4Faculty Chemistry, Institut for Analytical Chemistry, University Vienna, Vienna, Austria
Classic tumor therapy, consisting of cytotoxic agents and/or targeted therapy, has not overcome therapeutic limitations like poor risk genetic parameters, genetic heterogeneity at different metastatic sites or the problem of undruggable targets. Here we summarize data and trials principally following a completely different treatment concept tackling systems biologic processes: the principle of communicative reprogramming of tumor tissues, i.e., anakoinosis (ancient greek for communication), aims at establishing novel communicative behavior of tumor tissue, the hosting organ and organism via re-modeling gene expression, thus recovering differentiation, and apoptosis competence leading to cancer control – in contrast to an immediate, “poisoning” with maximal tolerable doses of targeted or cytotoxic therapies. Therefore, we introduce the term “Master modulators” for drugs or drug combinations promoting evolutionary processes or regulating homeostatic pathways. These “master modulators” comprise a broad diversity of drugs, characterized by the capacity for reprogramming tumor tissues, i.e., transcriptional modulators, metronomic low-dose chemotherapy, epigenetically modifying agents, protein binding pro-anakoinotic drugs, such as COX-2 inhibitors, IMiDs etc., or for example differentiation inducing therapies. Data on 97 anakoinosis inducing schedules indicate a favorable toxicity profile: The combined administration of master modulators, frequently (with poor or no monoactivity) may even induce continuous complete remission in refractory metastatic neoplasia, irrespectively of the tumor type. That means recessive components of the tumor, successively developing during tumor ontogenesis, are accessible by regulatory active drug combinations in a therapeutically meaningful way. Drug selection is now dependent on situative systems characteristics, to less extent histology dependent. To sum up, anakoinosis represents a new substantive therapy principle besides novel targeted therapies.
Introduction
Each cancer treatment strategy is based on simplifying methodological assumptions (Reichle, 2010). One basic consideration relies on the suggestion that essential tumor-promoting pathways are ubiquitously distributed at metastatic tumor sites and should be shut off, or single cell types within a tumor disease should be knocked out via specific targets. Molecular tumor boards and corresponding “intelligent” treatment algorithms are the logic consequence of this assumption.
Therapeutic limitations of classic targeted tumor therapies are (molecular-) genetic heterogeneity at metastatic sites, poor risk genetic parameters as well as context-dependent validity and denotation of tumor-promoting aberrations and targets (dependent of the genetic background) (Box 1). This fact may be exemplified by context-dependent efficacy of B-RAF inhibitors in different tumor histologies (Flaherty et al., 2010; Kopetz et al., 2015).
Box 1. Explanation of communication terms.
Here we summarize trials principally following a completely different treatment concept. All the mentioned schedules are tackling systems biologic processes, such as dysregulated homeostatic pathways in tumors or are recalling or perfectioning patterns of evolutionary processes (“metabolism of evolution”) provided by single cell types and cell systems in a tumor (Box 1). Thus, also drugs, particularly drug combinations, may be introduced with biomodulatory activity, so called master modulators of tissues, promoting evolutionary processes or regulating homeostatic pathways for treating metastatic and refractory metastatic disease or hematologic neoplasia (Hart et al., 2015).
Master modulators of tumor tissues, such as transcriptional modulators, hormones, cytokines, vitamins, epigenetically active drugs, metronomic low-dose chemotherapy and protein-binding drugs cyclooxygenase-2 (COX-2) inhibitors, IMiDs, arsenic trioxide etc. are aiming at reconditioning tumor tissue into a controlled phenotype, thereby diversifying palliative care, or even inducing continuous complete remission (Table 1; Box 1; Hart et al., 2015). Master modulators may therapeutically cope with different, but iterative patterns and physical constitutions of hallmarks of cancer supported by quite heterogeneous tumor genotypes. Those different patterns of acquired chromosomal aberrations may support a unique hallmark, exemplified in acute leukemias by the rapidly displacing growth in the bone marrow.
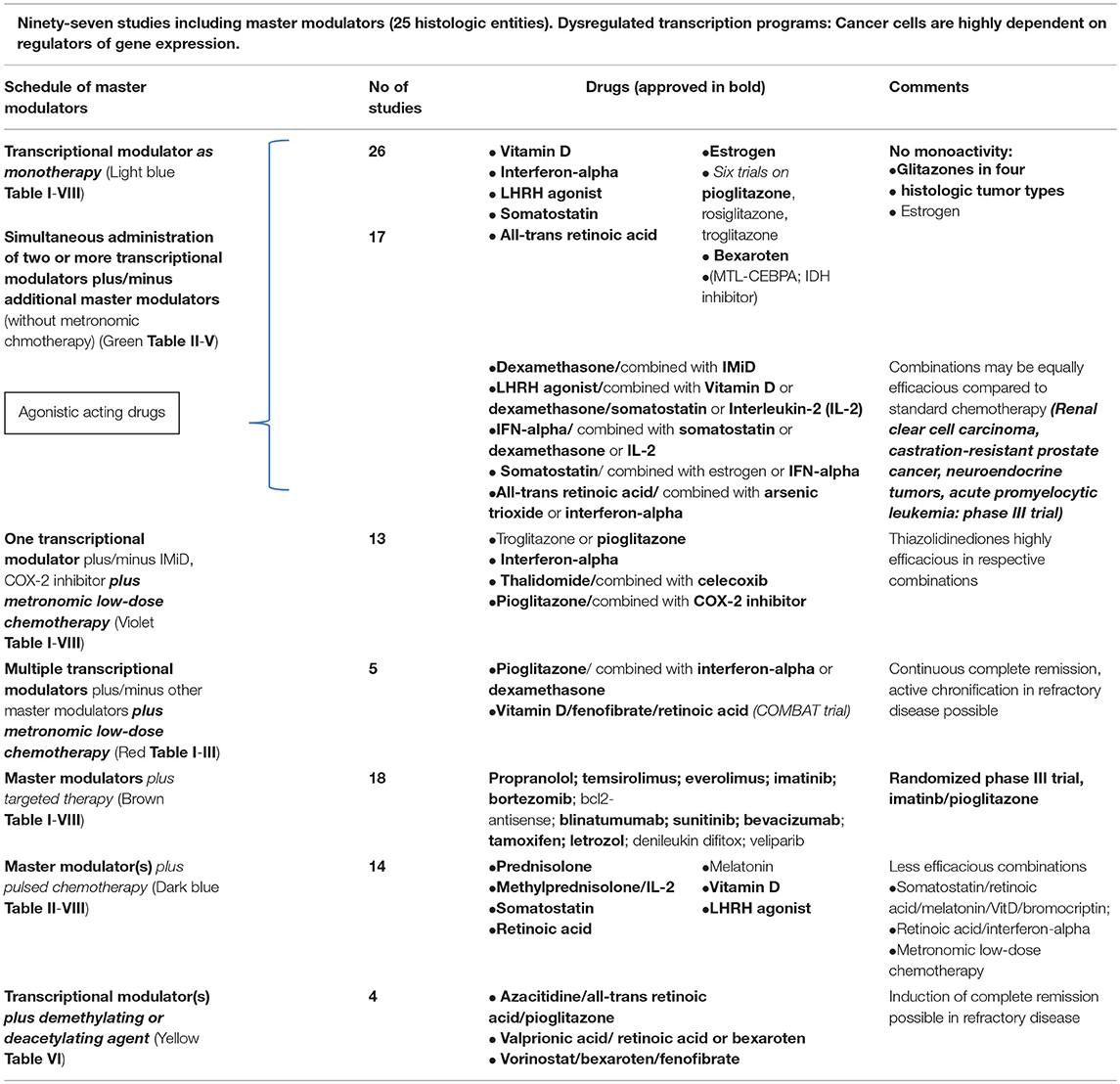
Table 1. Master modulators including transcriptional modulators in 97 clinical trials: Master modulators are transcriptional modulators (hormones, cytokines, vitamins etc.), metronomic low-dose chemotherapy, protein-binding drugs (arsenic trioxide, COX-2 inhibitors, IMiDs etc.), metabolic active drugs, such as PPAR gamma/α agonists, statins, and metformin (interventional statin and metformin trials are not included in the review; also, not included nuclear receptor antagonists).
Basis for the concerted regulatory activity profile of master modulators are during tumor ontogenesis developing dysregulated transcription programs, networks of pathways and interlaced communication routes among cancer cells, adjacent stroma cells, tumor bearing organ and organism.
Communicative reprogramming of tumor tissues, i.e., anakoinosis, aims at establishing novel communicative behavior of tumor tissue, the hosting organ and organism via re-modeling gene expression, thus recovering differentiation, and apoptosis competence leading to cancer control (Box 1; Hart et al., 2015).
The presented tool of clinical observations on anakoinosis inducing therapy approaches reveals that tumor tissue provides an extensive design space, including the interaction of tumor and tumor bearing organ and organism (Hart et al., 2015). The biological necessity of tumor site to respond with clinically relevant changes in tumor behavior following exposure to master modulators that means anakoinosis-inducing drugs, is predefined by not necessarily histologically determined prerequisites guiding communication.
Master Modulators, the Backbone of Anakoinosis Inducing Therapies
Broad Repertoire of Possible Approaches for Inducing Anakoinosis: Diversity of Master Modulators of Tumor Tissues
The instruments for inducing anakoinosis are multifaceted and still insufficiently explored. An important distinguishing characteristic, in contrast to classic targeted therapy, is the generally observed minor monoactivity, but frequently “concerted” activity profile of single pro-anakoinotic drugs (Tables I–VIII), the possibility for successfully administering agonistic, immunomodulatory and anti-inflammatory drugs and the modest toxicity profile.
Starting point for the current review are a series of systematically developed clinical trials on refractory metastatic tumor diseases including at least one nuclear receptor agonist and metronomic low-dose chemotherapy or epigenetically active drugs as pro-anakoinotic therapy approaches (Hart et al., 2015, 2016; Walter et al., 2017).
Pro-anakoinotic schedules include epigenetically and transcriptionally active drugs, such as agonists of nuclear transcription factors (glitazones, all-trans retinoic acid, bexarotene, glucocorticoids, vitamin D etc.), but also cytokines (e.g., interferon-α, Interleukin-2 etc.) and vitamins. Transcriptional modulators have in common that they may up-regulate tumor suppressor genes (Berger et al., 2011). Thus, also differentiation inducing liposomal encapsulated small oligonucleotide encoding small activating RNAs (MTL-CEBPA) are pro-anakoinotic drugs (Reebye et al., 2018; Sarker et al., 2018). Table V exemplarily summarizes first clinical results on MTL-CEBPA or ivosidenib, an IDH (Isocitrat-Dehydrogenase) inhibitor as differentiation inducing drugs.
In addition, metabolic modulators have been introduced, like metformin (Attia et al., 2008; Chae et al., 2016; Lecarpentier et al., 2017), which may also act transcriptionally (Coyle et al., 2016) and statins. IMiDs, COX-2 inhibitors and arsenic trioxide represent protein-binding pro-anakoinotic drugs.
Anakoinosis drugs include epigenetically acting agents, e.g., azacitidine, decitabine, valproic acid etc. acing broadly on chromatin, but also, (here, only mentioned) small molecules targeting specific epigenetic mechanisms, e.g., by inhibiting BET bromodomain transcriptional regulators; EZH2 (Enhancer of zeste homolog 2); DOT1L (DOT1-like, histone H3 methyl-transferase); IDH (Dawson et al., 2011; McCabe et al., 2012; Kim et al., 2015; Dang et al., 2016; Tögel et al., 2016; DiNardo et al., 2018).
The pro-anakoinotic activity of metronomic low-dose chemotherapies with their pleiotropic angiostatic, immunomodulatory, anti-inflammatory and drug specific effects, may be particularly exploited in combination with further master modulators (Hart et al., 2015). Clarithromycin, metronomically administered, shows similar activity profiles (Hart et al., 2015; van Nuffel et al., 2015; Romiti et al., 2017). Some pro-anakoinotic therapeutics on protein-binding level are arsenic trioxide, IMiDs, and COX-2 inhibitors are approved. Still in pre-clinical evaluation is a novel technology aiming at the targeted shut off transcriptional modulators with small molecules (Bradner et al., 2017; Tables 1, 2).
Frequently “old drugs” are used within pro-anakoinotic schedules in quite new functions, what is called “drug repurposing” (Bertolini et al., 2015). For the main part, the present review compiles drugs with poor monoactivity, particularly, also with respect to the scheduled dose reductions of single drugs for long-term administration (Hart et al., 2015).
The metronomic scheduling of drugs is an important component of pro-anakoinotic therapy approaches (André et al., 2017). Although in some tumor diseases rapid responses may be achieved within a 3–4-week cycle, other responses occur delayed, showing that a continuous systems therapeutic approach is necessary (Hart et al., 2015).
Clinical results of the reviewed therapeutic concepts integrating anakoinosis-inducing drug combinations indicate that features of palliative tumor care may diversified in a therapeutically meaningful way and that pro-anakoinotic schedules even have the capacity for inducing (continuous) complete remission (Hart et al., 2015; Thomas et al., 2015; Mayer et al., 2017).
Reviewed Pro-anakoinotic Schedules for Explicating the Novel Treatment Approach
For explicating the novel methodological approach, we summarized data on the clinical administration of master modulators, i.e., transcriptional modulators in monotherapy or in various combinations (Table 1), (1) combined with metronomic low-dose chemotherapy, (2) pulsed chemotherapy, (3) demethylating agents, (4) classic targeted therapies or (5) protein-binding pro-anakoinotic drugs (arsenic trioxide, IMiDs, COX-2 inhibitors) (Tables I–VIII). Agonists and antagonists of transcriptional modulators, metronomic low-dose chemotherapy, epigenetically active agents, protein- binding pro-anakoinotic drugs, but also classic targeted therapies inducing for example differentiation (Table V) are considered as master modulators exploiting the tumors design space.
Available study data are unsuitable for presentation in a Cochrane or PRISMA analysis due to the diversity of schedules and the respective low patient numbers treated in each trial. Likewise, it would go beyond the scope of this study to give a comprehensive review on single master modulators.
Just the diversity of response patterns following anakoinosis-inducing schedules, their successful administration independent of tumor histology, the possibility for classifying responses according to operated communication tools, or for elaborating mechanisms of action, may highlight the sum of reported pro-anakoinotic treatment approaches as unique therapeutic pillar (Tables 1, 2).
Concerning transcriptional modulators, the explication of the novel methodological approach is restricted to selected transcriptional modulators in mono- or combination therapy, i.e., glitazones, glucocorticoids, vitamin D, somatostatin analogs, melatonin, interferon-alpha, estrogen, luteinizing hormone-releasing hormone (LHRH) agonist, retinoic acid and bexarotene.
For clarity, we do not consider (nuclear) receptor antagonists as clinical data on these drugs well established their clinical benefit, for example in breast and prostate cancer. In addition, not included are studies using COX-2 inhibitors plus pulsed chemotherapy, metformin or statins, also for reasons of comprehensibility.
For a better assessment of the clinical results on combinations of master modulators, particularly transcriptional modulators, additional data from randomized clinical trials are given comparing metronomic chemotherapy ± targeted therapies or metronomic with pulsed chemotherapy in Table VIII.
Monoactivity of Anakoinosis Inducing Drugs
Twenty-four reviewed studies included one transcriptional modulator, as monotherapy for cancer treatment, either a glitazone, a hormone or cytokine. Table 1 indicates the administered master modulators.
Monoactivity of glitazones or estrogen in cancer patients is very modest, whereas strong activity is well established in single tumor histologies for dexamethasone, LHRH agonist, somatostatin, and bexarotene (Tables IA/B, II, IV–VII; Querfeld et al., 2006; Sharma et al., 2008; Sidéris et al., 2012). The administration of interferon-α is superseded for melanoma or multiple myeloma (Browman et al., 1995; Di Trolio et al., 2015).
Oncological praxis does still not integrate metronomic low-dose chemotherapy, as routine therapeutic concept: Even combination therapies with classic targeted approaches or pulsed chemotherapy often show poor results in randomized comparison (Table VIII). Even more so, there is a growing number of combinatory schedules (Kerbel and Shaked, 2017), which shall bring to the fore the metronomic idea, meanwhile advanced in years, on the background of novel pharmacokinetic data (Bocci and Kerbel, 2016; Ciccolini et al., 2017).
Like metronomic chemotherapy, clarithromycin has a multi-functional activity profile and is currently being used in anakoinosis inducing schedules (ClinicalTrials.gov Identifier: NCT02852083) (Table 1; van Nuffel et al., 2015).
Demethylating and deacetylating agents show monoactivity in the range of commonly approved dose levels (Nervi et al., 2015). However, in anakoinosis inducing schedules much lower doses are going to be established (ClinicalTrials.gov Identifier: NCT02942758) (Thomas et al., 2015).
Agonists of “adopted” orphan receptors commonly have poor monoactivity in interventional cancer trials (Smith et al., 2004; Di Masi et al., 2015), in contrast to hormones and cytokines (McDermott et al., 2005; Mitchell et al., 2005). Particularly, dexamethasone plays a decisive role in the induction treatment for acute lymphocytic leukemia or multiple myeloma (Mitchell et al., 2005).
Metabolically active drugs, such as metformin or PPARγ/α agonists, are considered as chemopreventive agents (Fröhlich and Wahl, 2015; Higurashi et al., 2016). Metformin may prolong survival in cancer patients following surgery, but only in distinct histologic tumor types, as retrospective studies are indicating (Coyle et al., 2016).
Among the protein-binding drugs, arsenic trioxide and immunomodulatory imide drugs (IMiDs) have known monoactivity in hematologic diseases (Quach et al., 2010; Iland and Seymour, 2013) but both drugs are commonly administered combined with transcriptional modulators, all-trans-retinoic acid and dexamethasone, respectively (Lo-Coco et al., 2013; Benboubker et al., 2014).
Simultaneous Administration of Two or More Transcriptional Modulators Plus/Minus Additional Master Modulators (Without Metronomic Low-Dose Chemotherapy)
Synergistic activity of dual transcriptional modulation has been well established in pre-clinical studies, for example for pioglitazone and all-trans retinoic acid in tumor cell lines of different histology (Papi et al., 2009, 2010, 2012, 2013), but also for glitazones in combination with chemotherapy (Elrod and Sun, 2008). Clinical trial designs translated these pre-clinical results hesitantly. Predominantly drugs, coming from immunomodulatory approaches (Il-2, interferon-α) found their way into combinatorial use (McDermott et al., 2005). Somatostatin analogs are administered besides their original application field, e.g., neuroendocrine tumors, also in castration-resistant prostate cancer, here in combination with estrogen or dexamethasone and LHRH analogs (Sciarra et al., 2004; Koutsilieris et al., 2006). In castration-resistant prostate cancer, the combination of transcriptional modulators alone may induce durable response (Table V; Koutsilieris et al., 2006). Combinations of estrogen with gestagen failed to show activity in endometrial carcinoma (Chlebowski et al., 2016).
Interestingly, interferon-α is active in renal cell carcinoma, both in combination with retinoids or pioglitazone (Buer et al., 1995; Walter et al., 2012; Aviles et al., 2015). Topical application of interferon-α and retinoids is helpful in corneal epithelial neoplasia (Krilis et al., 2012).
Standard schedules for the treatment of multiple myeloma include dexamethasone and IMiDs, also for maintenance therapy (Roussel et al., 2014).
Transcriptional Modulators Combined With Metronomic Low-Dose Chemotherapy
Adding transcriptional modulators to metronomic low-dose chemotherapy schedules even led to complete remissions in a series of phase II trials for histologically quite different refractory tumor entities (Hart et al., 2015). Schedules included metronomic low-dose chemotherapy, COX-2 inhibitor and transcriptional modulators. In single patients with Langerhans cell histiocytosis, renal clear cell carcinoma, in epithelioid, less differentiated angiosarcoma, these schedules induced continuous complete remission (Vogt et al., 2003; Coras et al., 2004; Reichle et al., 2005; Heudobler et al., 2016).
It was only after the addition of interferon-α to metronomic chemotherapy plus pioglitazone and COX-2 inhibitor that continuous complete remissions occurred in metastatic renal clear cell carcinoma (Walter et al., 2012; Hart et al., 2015). This example clinically shows the pro-anakoinotic synergy effects of transcriptional modulators. Induction of continuous complete remission with dual transcriptional modulation only, supports current experimental data, showing that PPARγ plays neither a tumor-suppressive nor an oncogenic role in advanced renal clear cell carcinoma, and that single-agent PPARγ agonists are unlikely to be effective for the treatment of this disease (Sanchez et al., 2018).
In a series of advanced and refractory hematologic diseases and solid tumors, combination therapies of pioglitazone with dexamethasone or interferon-α or all-trans-retinoic acid could be successfully used in addition to metronomic low-dose chemotherapy or azacitidine. Figure 1 indicates diversified outcomes in a summary of selected published studies on seven different histologic tumor entities (Walter B. et al., 2010; Walter et al., 2012; Hart et al., 2015, 2016; Thomas et al., 2015; Ugocsai et al., 2016).
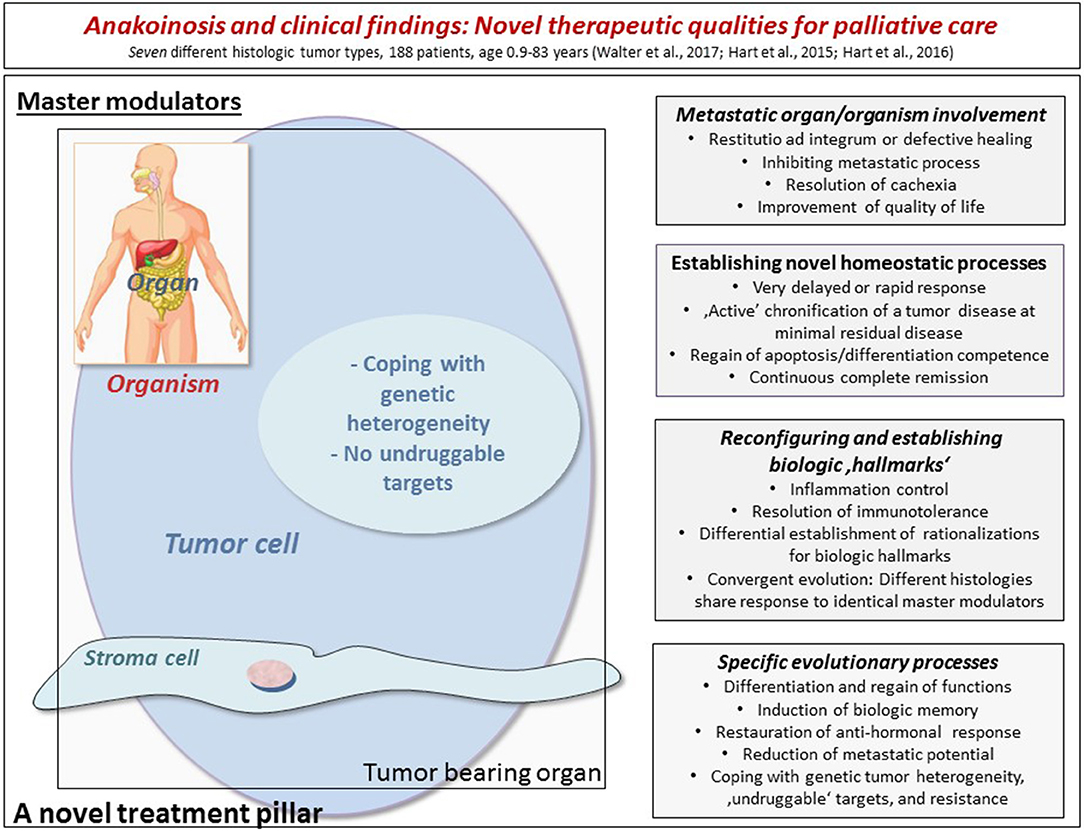
Figure 1. The figure indicates diversified outcomes in a summary of selected published studies on seven different histologic tumor entities (altogether 188 refractory and metastatic patients with age, ranging from 0.9 to 83 years). By modulating therapeutically accessible communication tools (left side), homeostasis mechanisms may be “normalized” in the tumor tissue, in the tumor-bearing organ and organism as indicated by a broad diversification of palliative tumor care or even continuous complete remission. The right side lists the multifaceted clinically observed phenomena during anakoinosis inducing therapies. The diversified clinical outcomes highlight clinical advantages compared to classic targeted therapies.
Fenofibrate, retinoic acid, and vitamin D combined with metronomic chemotherapy induced in pediatric patients with refractory tumors of quite different histology complete remissions (15%) (Zapletalova et al., 2012).
Long-term responses at PSA levels <0.1 ng/ml occurred in rapidly progressive prostate cancer with PSA doubling times <3 months using combined transcriptional modulation, dexamethasone and pioglitazone in addition to metronomic low-dose chemotherapy. Pioglitazone may activate PPARγ, which is suggested to be a tumor suppressor in prostate cancer (Olokpa et al., 2017). The addition of imatinib in this schedule did not add any benefit. Interestingly, after discontinuing the study therapy due to non-tumor related surgery, patients remained at the status of minimal residual disease for up to 1.5 years. Such clinical observations are indicating anakoinosis as basis for the development of “biologic memory” in the anakoinotically modulated tumor tissue (Hart et al., 2015; Vogelhuber et al., 2015).
Besides nuclear receptor antagonists, LHRH agonists are standard therapies in breast and prostate cancer, mostly concomitantly integrated in therapy schedules (Graham and Schweizer, 2016; Nourmoussavi et al., 2017; Table VIII).
The worldwide alarming incidence of advanced liver cell carcinoma represents a great challenge for tumor therapy. An anakoinosis inducing therapy approach, including pioglitazone, COX-2 inhibitor and metronomic low-dose chemotherapy, has shown a comparably favorable influence on overall survival in advanced liver cell carcinoma, in comparison to more sophisticated systems therapies, including sorafenib, lenvatinib or anti-PD-1 antibodies (Walter et al., 2017). At that, an anakoinosis inducing therapy is cost-effective, and shows a lower toxicity rate. Therefore, a randomized comparison or a combination with targeted therapies seems to be a logical next step.
Demethylation Plus All-Trans Retinoic Acid and Pioglitazone or Deacetylation Plus Bexarotene
The combination of azacitidine plus all-trans retinoic acid and pioglitazone may induce ex vivo granulocytic differentiation in more of 50% of blasts from acute myelocytic leukemia (Thomas et al., 2015). Moreover, these granulocytes regain phagocytic activity, when exposed to E. coli (Klobuch et al., 2018). Clinically, it is possible to induce continuous complete remission in acute myelocytic leukemia with the triple combination, while using only about 50% of the recommended dose of azacitidine.
Bexarotene, fenofibrate plus vorinostat may induce complete remission in tumor-stage mycosis fungoides (Steinhoff et al., 2008).
Classic Targeted Therapies Combined With Anakoinosis Inducing Drugs
Among the combination of pro-anakoinotic substances combined with classic targeted therapies are several approved therapy schedules, interferon-α plus bevacuzimab (Escudier et al., 2007), proteasome inhibitors combined with dexamethasone, sandostatin plus mTor inhibitor, LHRH agonist plus tamoxifen (Tables I–VIII, brown). Classic targeted therapies may be successfully combined with anakoinosis inducing drugs, e.g., mTor-inhibitors in melanoma, or in 5th-line in refractory Hodgkin lymphoma, or imatinib in chronic myelocytic leukemia (CML), not achieving molecular complete remission, or bexarotene combined with denileukin difitox in cutaneous T-cell lymphomas (Foss et al., 2005; Hart et al., 2015; Prost et al., 2015; Ugocsai et al., 2016).
Dual metronomic chemotherapy combined with bevacizumab was efficacious in breast cancer: Higher baseline circulating endothelial cells correlate with significantly improved overall response and progression-free survival (Dellapasqua et al., 2008).
Although, representing a specifically targeted approach, CAR-T-cells act anakoinotic by infiltration, proliferation and cytokine storm in the tumor tissue (Chmielewski et al., 2014). Demethylating agents can efficiently modulate the immunophenotype of melanoma cells (Fratta et al., 2013).
Randomized Trials
Rosiglitazone, given as monotherapy, did not delay PSA progression in a placebo-controlled trial for prostate cancer (Smith et al., 2004).
In a randomized trial in T-cell lymphomas, dual transcriptional modulation with interferon-α plus retinoid is as potent as a chemotherapy containing regimen combined with interferon-α, but less toxic (Aviles et al., 2015). An analogous combination, interferon-α, pioglitazone plus metronomic low-dose chemotherapy, also shows synergistic effects in renal cell carcinoma in a historic comparison (Reichle et al., 2007a; Walter et al., 2012). Interferon-α has activity as maintenance therapy in lymphomas (Herold et al., 2015). The combination of interferon-α with lanreotide did not show any synergistic effects in neuroendocrine tumors (Faiss et al., 2003). Monoactivity of interferon-α is commonly weak among quite different tumor types (Eggermont et al., 2008). Therefore, the drug did not find its way in routine use or novel drugs are meanwhile more active, for example in melanoma (Agha and Tarhini, 2017).
Interestingly, the anakoinosis inducing combination of LHRH agonist, somatostatin analog plus dexamethasone is as efficacious as pulsed chemotherapy including estramustine and etoposide for treatment of castration-resistant prostate cancer (Dimopoulos et al., 2004).
A randomization in metastatic melanoma highlighted the addition of pioglitazone and COX-2 inhibitor to metronomic chemotherapy: The triple combination resulted in a significantly improved progression-free survival (Reichle et al., 2007b).
Although gastric cancer is commonly expressing PPARγ, the addition of pioglitazone and COX-2 inhibitor to metronomic chemotherapy did not improve outcome in a randomized comparison (Reichle and Hildebrandt, 2009).
Low-dose metronomic chemotherapy was not superior compared to pulsed chemotherapy in glioblastoma patients (Clarke et al., 2009). The addition of pioglitazone and COX-2 inhibitor to metronomic chemotherapy led to disease stabilization in heavily pre-treated patients with glioblastoma or astrocytoma (Hau et al., 2007).
In T-cell lymphoma, interferon-α plus retinoid may substitute low-dose MTX plus interferon-α (Aviles et al., 2015).
The combination arsenic trioxide and all-trans retinoic acid outcompeted ATRA plus chemotherapy for standard risk promyelocytic leukemia (APL) (Efficace et al., 2014).
Anakoinosis Inducing Therapy Concomitantly or Sequentially to Pulsed Chemotherapy
Administered as adjuvant therapy, metronomic chemotherapy failed to show superiority, or patients only modestly benefit despite the addition of bevacizumab in osteosarcoma or colorectal cancer, respectively (Simkens et al., 2015; Senerchia et al., 2017; Table VIII).
Probably, raising vitamin D levels in serum from low/sub-normal to normal/high may contribute to a prolonged survival following chemo-immunotherapy in lymphomas (Bittenbring et al., 2014) whereas no advantage could be observed by the addition of vitamin D to docetaxel in prostate cancer (Attia et al., 2008).
The possible importance of normal or high vitamin D levels in serum on overall survival does not seem to be restricted to lymphoma patients treated with chemo-immune therapy, but there are also hints that patients following allogeneic blood stem cell transplantation may benefit from vitamin D substitution by a lower incidence of chronic graft-vs.-host reactions (Caballero-Velázquez et al., 2016).
Treatment of metastatic NSCLC with pulsed chemotherapy plus anakoinotically acting approaches including transcriptional modulators was less successful (Mantovani et al., 2000; Norsa and Martino, 2007). From available data one cannot conclude, whether the combination with pulsed chemotherapy may “destroy” anakoinotic activity profiles or the anakoinosis inducing “cocktail” was not chosen adequately adapted to the respective tissues' systems pathophysiology. The addition of a COX-2 inhibitor to pulsed chemotherapy did not significantly improve progression-free survival in first-line therapy for non-small-cell lung cancer (Edelman et al., 2017).
In contrast, metronomic chemotherapy plus combined transcriptional modulation may even induce complete remission in refractory tumors. Dual transcriptional modulation seems to be more efficacious as indicated by the response rate, long-term tumor control and continuous complete remissions (Reichle et al., 2007b; Hart et al., 2015).
In lymphoma patients, differential chemotherapy schedules may be associated with significantly different overall survival rates, irrespective of the fact that patients received identical prednisolone doses in each treatment arm (Bastion et al., 1997).
Interferon-α does not enhance activity of 5-fluorouracil (5-FU) in colon cancer (Hill et al., 1995; Thirion et al., 2001). Interferon-α plus retinoid adds no benefit to pulsed cisplatin in cervical cancer (Basu et al., 2016).
Some Combinations of Transcriptional Modulators Are “Supportive”
LHRH agonists are used for preserving fertility during pulsed chemotherapy (Taylan and Oktay, 2017), like osteoprotective regimens, such as LHRH agonist plus vitamin D (Scharla et al., 1990).
Specific Methodological Aspects of Anakoinosis Inducing Therapies
What Is the Appropriate Dosage of Pro-Anakoinotic Therapy?
The question for the appropriate dose of each drug in an anakoinosis inducing schedule can be answered only pragmatically, based on clinical results and scheduled dose reductions, but currently, not yet pharmacokinetically (Hart et al., 2015; Bocci and Kerbel, 2016; Ciccolini et al., 2017; Walter et al., 2017).
Striking clinical results of anakoinosis inducing schedules are at first glance surprising on the background that the single drugs, particularly glitazones, have poor or no monoactivity. This comes true also for metronomic low-dose chemotherapy, as scheduled dose reductions have been performed within trials up to a dose, which would correspond to less than a quarter of the respective 3 weekly-administered cumulative dose (Hart et al., 2015; Walter et al., 2017).
Concertedly, pro-anakoinotic drug combinations may induce remissions, even continuous complete remissions, and may result in diversified palliative care strategies, as shown by multifold beneficial palliative effects in refractory neoplasia, as summarized in Figure 1. This way, the broad spectrum of available drugs, such as low-dose metronomic chemotherapy and transcriptional modulators come out from the corner as therapies validated as modestly efficacious: Once combined, these pro-anakoinotic treatment modalities (Tables 1, 2) have the capability for controlling refractory tumor disease. Thus, the drug combinations obviously act concertedly as regulators of the tumor cell systems, thereby, re-establishing apoptosis and differentiation competence in refractory tumor disease. Importantly, master modulators do not necessarily compromise non-diseased, homeostatically balanced organ systems, but may even improve organ functions (Hart et al., 2015; Walter et al., 2017). Possible side effects, allow an early scheduled dose reduction for avoiding further toxicity, and importantly, without significant loss of efficacy (Walter et al., 2017). Therapeutic efficacy despite dose reduction, particularly in case of metronomic low-dose chemotherapy, and the fact that, glitazones show no monoactivity in all available trials, are indicating the pro-anakoinotic, regulatory activity profile of the schedules (Table 1). We included metronomic low-dose chemotherapy also in the category “master modulators”: No evidence could be found in any reviewed clinical trial that scheduled dose reduction would have any impact on outcome–as far as transcriptional modulators have been additionally administered in the study schedule (Hart et al., 2015; Walter et al., 2017). Striking examples are the COMBAT trial for children with refractory tumors (Combined Oral Metronomic Biodifferentiating Antiangiogenic Treatment), and prospectively evaluated data on scheduled dose reduction of capecitabine in hepatocellular carcinoma; the schedule combined capecitabine etoricoxib and pioglitazone (Zapletalova et al., 2012; Walter et al., 2017). Reasons for a still available biomodulatory activity at very low-doses of metronomic chemotherapy could be synergisms between transcriptional modulators and metronomic chemotherapy: Pioglitazone, for example, generally up-regulates PTEN (Teresi and Waite, 2008; Berger et al., 2011), thereby, sensitizes hepatocellular carcinoma cell lines to 5-fluorouracil (5-FU), which is the active metabolite of capecitabine (Cao et al., 2007).
For monitoring anakoinosis inducing therapies it is meanwhile realistic to establish appropriate serum analytics (Mayer et al., 2017; Muqaku et al., 2017) to appreciate the functional status of cell systems and their changes (Pitteri et al., 2011). This way, we can approach the questions, which is the lowest, still regulative active dose of a single drug, and which are the most prominent players promoting tumor growth in the tumor tissue and the tumor bearing organ?
Transcriptional Modulation in Cancer
Dysregulated transcription programs are an invariable consequence of oncogenic events, and represent the backbone of cancer (Berger et al., 2011). Dysregulation of transcriptional networks are the reason, why cancer cells are highly dependent on regulators of gene expression (Bradner et al., 2017). Gene regulatory network features reveal key regulatory networks and epigenetic changes that underpin tumor disease (Jin et al., 2018). Clusters of enhancers facilitate precise control of gene expression across normal cellular hierarchies, and are potential targets as central hubs in tumor disease (Bahr et al., 2018). The use of master modulators for treatment of metastatic refractory tumor disease, and the observed multifaceted clinically meaningful outcomes support the suggestion of hubs for regulation of transcriptional webs (Reichle and Hildebrandt, 2009; Hart et al., 2015; Huang et al., 2018).
Dysregulated transcriptional programs provide pivotal opportunities for a series of novel therapeutic interventions in metastatic refractory cancer (Giovannelli et al., 2012; Hart et al., 2015; Winter et al., 2015). Despite of currently expanding data, it is still difficult to broadly implement the current knowledge about transcriptional addiction for patients' benefit by targeting oncogenic transcription factors (e.g., PMLRARA), such as in case of acute promyelocytic leukemia (APL), resulting in substantial clinical benefit (Cicconi et al., 2016).
The targeted blockade of tissue-specifying nuclear transcription factors in cancer is well-established (estrogen, progesterone, androgen receptor) (Giovannelli et al., 2012). However, our knowledge of tissue-specifying transcription factors remains limited, and drugs with pro-anakoinotic activity, such as agonists of nuclear transcription factors regularly have an activity profile far above the capacity of hermeneutic comprehension (Reichle and Vogt, 2008).
Therapeutics, disrupting oncogenic transcription factors by targeted protein degradation, are still clinically not approved, but successfully studied in pre-clinical settings (Winter et al., 2015). In pre-clinical trials, blocking of super-enhancers of transcriptional networks is possible (Mack et al., 2018).
Master modulators in pro-anakoinotic therapy schedules evolve tumor systems in a therapeutically meaningful way by promoting communicative reprogramming, anakoinosis, implicating changes of validity and denotation of cell elements, and therefore also of cellular identity (Klobuch et al., 2018). Vice versa, neoplasia endogenously evolves, based on anakoinotic mechanisms. For example, hepatocellular carcinoma develops on basis of liver cirrhosis, or aging processes in normal B-cells precede B-cell chronic lymphatic leukemia. In case of cachexia, the tumor even affects the whole organism via reprogramming platelets, for example in metastatic melanoma (Mayer et al., 2017; Muqaku et al., 2017).
Transcriptional modulation with agonistic drugs, particularly dual transcriptional modulators, as discussed in the current review, showed clinically meaningful efficacy in a series of refractory metastatic neoplasia by communicatively reprogramming transcriptional networks maintained by tumor and adjacent stroma cells: Anakoinosis inducing approaches may re-adapt or “normalize” homeostatic pathways (Hart et al., 2015) by modulating functionally defined subsystems. Consecutively, subsystems may take over novel validity and denotation for constituting diversified rationalizations of biologic hallmarks (Box 1; Reichle and Hildebrandt, 2009).
Therapeutically intended transcriptional networking may have decisive regulatory impact on tumor promotion, for instance, on the angiogenic switch or on tumor stem cell behavior (Trosko, 2006). Targeting functionally defined subsystems with modulators of transcription factors seems to become of increasing interest, as subsystems within tumors may be exclusively functionally defined in a systems context but simultaneously linked to alternating structural systems (Pahler et al., 2008; Reichle and Hildebrandt, 2009).
Characteristics of Master Modulators of Tumor Tissues
Contrary to the genetic, molecular genetic and phenotypic heterogeneity of metastatic tumor cells (Allgayer, 2018; Gerner et al., 2018); tumor growth-promoting sub-systems supporting hallmarks of cancer promise a high grade of similarities in constituting hallmarks of cancer or respective tumor-specific patterns of hallmarks. The successful administration of similar pro-anakoinotic schedules in refractory Hodgkin lymphomas and malignant melanomas, in castration-resistant prostate cancer and multiple myeloma, or in refractory angiosarcomas and Langerhans cell histiocytosis (Hart et al., 2015) underpins that tumors draw on a distinct repertoire of rationalizations supporting biologic hallmarks for constituting tumor phenotype (Box 1; Gerner et al., 2018). Thus, similar repertoires of drug combinations with pro-anakoinotic activity profile might be available, which target and regulate corresponding tumor-associated communicative subsystems mirrored for example by inflammation-related biomarkers etc. (Reichle et al., 2007a).
How can we integrate the finding that different histologic tumor types may share tumor response to distinct combinations of master modulators (Hart et al., 2015).
Recurring oncogenic events, such as alterations of NF-κB, TGFβ, Ras, p53, Myc, E2F/Rb/CDKN2, are associated with multifold tumor phenotypes (Ahmadiyeh et al., 2010): This observation explains that the tissue of origin predominantly characterizes tumor phenotypes as indicated by histology. The tumor phenotype depicts the recessive and thus, therapeutically accessible communicative interactions occurring during tumor evolution according to the restrictions given by the tissue-specific “metabolism” of evolution.
The tissue-specific “metabolism” of evolution makes different cancer types sharing similar tissue disruptions, alterations in homeostatic pathways and dysregulation of transcription factors, as tumor evolution deeply interweaves ubiquitously available wound healing mechanisms, inflammation, immunity, angiogenesis and metabolic processes (Virchow, 1859; Dvorak, 2015).
So-called super-enhancers spatially and temporally coordinate transcriptional webs for maintaining cell identity. Eqipollent, the neighboring environment communicatively mediates cellular identities (Reichle, 2013). Accordingly, synchronized “super enhancers” are responsible for higher order spatial re-organization of chromatin clusters that finally define cell identity (Hnisz et al., 2013). Oncogenic events must draw on such super-enhancers for establishing tumor-associated conditions described by the observation that tumors behave like never healing wounds (Virchow, 1859; Dvorak, 2015). Transcriptional super-enhancers may also explain convergence of developmental and oncogenic signaling pathways and their unique therapeutic accessibility (Hnisz et al., 2015).
Clinically observable changes in cell identities as indicated for example by differentiation of blasts from acute myelocytic leukemias and regain of granulocytic functions, suggest therapeutically important modifications of super-enhancers by pro-anakoinotic, transcriptionally active drugs (Hnisz et al., 2013; Hart et al., 2015; Sim et al., 2017; Klobuch et al., 2018). Figure 1 summarizes that cellular identity compromising tumor biologic changes are as expected, highly diversified, with strong impact on outcome. Interestingly, pre-clinical activities of inhibitors of super-enhancers among different tumor types are as multifaceted as clinical results presented in Figure 1 (Sengupta and George, 2017).
“Biologic memory” or long-term response in castration-resistant prostate cancer exemplifies the possibility for “active” chronifying tumor disease. Induction of molecular complete remission via differentiation induction could be observed in acute myelocytic leukemia or delayed objective response in renal clear cell carcinoma with consecutive continuous complete remission. Interestingly, combinatorial use of transcriptional modulators seems to be even more efficacious concerning outcome of refractory metastatic tumor disease (Koutsilieris et al., 2006; Hart et al., 2015).
An anakoinosis inducing schedule in hepatocellular carcinomas clinically demonstrated that the discrimination of local or metastatic disease has no significant impact on overall survival (Walter et al., 2017). By using the appropriate pro-anakoinotic therapy approach the suggested genetic heterogeneity at metastatic sites plays a minor role for outcome. These results highlight the novel pathophysiologic concept as basis for pro-anakoinotic therapies (Reichle and Hildebrandt, 2009).
Glitazones are the most frequently used transcriptionally active master modulators for anakoinosis induction besides glucocorticoids (Heudobler et al., 2018b). For example, pioglitazone, a peroxisome-proliferator-activated receptor (PPAR) γ/α agonist, seems to be an important many-sided applicable master modulator for communicative processes in neoplasia. Despite the missing monoactivity of glitazones in cancer (Table 1), pioglitazone is highly efficacious in combination with metronomic low-dose chemotherapy, or epigenetically active drugs (azacitidine), plus/minus further transcriptional regulators (interferon-α, dexamethasone or all-trans-retinoic acid (ATRA) (Tables I–VIII): After 3 years, the median overall survival has not been reached in a study on castration-resistant prostate cancer (Vogelhuber et al., 2015). In refractory Langerhans cell histiocytosis (Reichle et al., 2005; Heudobler et al., 2016), and (hem-) angiosarcomas continuous complete remissions have been observed (Vogt et al., 2003). Also in refractory acute myelocytic leukemia (Thomas et al., 2015) molecular complete remissions occurred, indeed by differentiation of blasts in phagocytic active neutrophils (Figure 1; Klobuch et al., 2018), but also by rescuing healthy hematopoietic maturation while repressing leukemic growth (Boyd et al., 2017). The addition of pioglitazone to imatinib in chronic myelocytic leukemias, not responding with molecular complete remission (MCR) may induce in a high percentage of patients MCR (Rousselot et al., 2017). In hepatocellular carcinoma, clinical data give hints that pioglitazone combined with metronomic low-dose chemotherapy and COX-2 inhibitor may communicatively reprogram in a clinically meaningful way the cirrhotic liver, tumor microenvironment and carcinoma cells (Walter et al., 2017).
Master modulators might interfere with master transcription factors and mediators establishing super-enhancers at key cell identity genes (Whyte et al., 2013). For example, PPARγ binds to the promoter of Dlc1 gene, a super-enhancer to regulate its expression during both white and brown adipocyte differentiation (Sim et al., 2017).
Performing studies on PPARγ in tumor cell cultures only, without co-culturing heterologous stroma derived cells leads to the conclusion that PPARγ may also function as a tumor promoter (Yun et al., 2018). Co-culturing tumor cells, however, with tumor-associated fibroblasts and dual stimulation with nuclear transcription factor agonists consistently results in tumor response within quite different histologic tumor types (Papi et al., 2009, 2010, 2012, 2013). In vivo, PPARγ agonists promote cell cycle arrest, cell differentiation (Klobuch et al., 2018; Ryu et al., 2018), and apoptosis and reduce inflammation (Hart et al., 2015), angiogenesis, oxidative stress, cell proliferation, invasion (Reichle and Vogt, 2008), and cell migration (Vallée and Lecarpentier, 2018).
Many pre-clinical data indicate that pioglitazone up-regulates non-mutated tumor suppressor genes and consecutively modulates homeostatic pathways in tumor tissues (Mulholland et al., 2005; Teresi and Waite, 2008; Berger et al., 2011; Rosner et al., 2017; Walter et al., 2017; Vallée et al., 2018). Mutations in the PPARγ gene are rare in neoplasia. Follicular thyroid cancer harbors a fusion gene (Eberhardt et al., 2010): A trial with pioglitazone is ongoing (ClinicalTrials.gov Identifier: NCT01655719).
Follow-up studies of the ProActive trial do not support any more the possibility that pioglitazone may favor the development of urothelial carcinoma in patients with diabetes mellitus type II (Erdmann et al., 2016).
Pro-Anakoinotic Therapy Schedules: Indications and Diagnostics
Transcriptional modulators are master modulators of tissue communication and are important pro-anakoinotic drugs, with obvious combinatorial activity among themselves, combined activity with metronomic low-dose chemotherapy, demethylating agents, pulsed chemotherapy or classic targeted therapy. It remains striking that the combinatorial use of the defined anakoinotic active drugs (Table 1) may induce (continuous) complete remission in refractory tumor disease.
All these observations prompt the assumption that irrespectively of the therapeutic technique for achieving apoptosis competence, either classically targeted, or with a cytotoxic approach, or by communicatively reprogramming a tumor disease, anakoinotic mechanisms are essentially necessary for finally initiating mechanisms, paving the way for continuous complete remission.
Assuming differential sequential biologic steps, necessary for achieving long-term tumor control in case of metastatic tumor disease (Beyar-Katz et al., 2016; Was et al., 2018), induction of anakoinosis opens the window for an active guidance and specified therapeutic support for re-establishing apoptosis or differentiation competence. Anakoinosis focuses on therapeutically guiding dynamic communication processes linked to tumor evolution or tumor response (Beyar-Katz et al., 2016; Was et al., 2018). More static evaluations of tumor characteristics, such as histology, immunohistology, molecular genetics and genetics serve as starting point for classic targeted therapies. Classic pathology-associated tumor evaluation includes parameters, which are mostly heterogeneous at metastatic sites (Figure 1).
In contrast, similarities of cellular immune response at primary site and metastatic sites of renal cell carcinoma or colorectal cancer (Remark et al., 2013) underline that tumor heterogeneity may be principally therapeutically overcome by pro-anakoinotic therapy approaches. Thus, evaluation of the respective “evolution-adjusted” tumor pathophysiology, such as homeostatic mechanisms, down-regulated tumor suppressor genes, transcriptional dysregulation etc., may in future diagnostically guide the selection of pro-anakoinotic schedules (Figure 1; Reichle, 2013).
Besides their function as rescue therapies for refractory neoplasia, anakoinosis inducing therapy schedules might supplement or substitute known consolidation therapies, such as continuous long-term maintenance therapy (Rousselot et al., 2017), adjuvant chemotherapies, or high-dose chemotherapy combined with autologous stem cell transplantation: Preliminarily results from clinical trials already indicate that anakoinosis inducing schedules may decisively influence long-term outcome (Tables I–VIII). For example, in chronic myelocytic leukemia without molecular remission (Rousselot et al., 2017) the addition of pioglitazone to imatinib may induce continuous complete remission: Even a discontinuation of imatinib and pioglitazone is possible. In patients with lymphoma serum vitamin D levels (Kelly et al., 2015; Prost et al., 2015) are predictive for overall survival. Lenalidomide is well established as maintenance therapy in multiple myeloma, all-trans retinoic acid in promyelocytic leukemia (Cicconi et al., 2016; McCarthy et al., 2017). Unfortunately, many chosen anakoinotic acting maintenance therapies showed modest improvement of progression-free survival, failed to demonstrate any important clinical effects or not used any more due to the availability of novel targeted therapies (Browman et al., 1995; Di Trolio et al., 2015; Simkens et al., 2015; Rochlitz et al., 2016).
Pro-anakoinotic therapy schedules might be the ideal adjuvants for classic targeted therapies efficaciously controlling, even eradicating disseminated neoplasia (Hart et al., 2015; Prost et al., 2015; Neelapu et al., 2017).
Generally, anakoinosis inducing therapies are characterized by a favorable toxicity profile (Dimopoulos et al., 2004; Zapletalova et al., 2012; Hart et al., 2015; Cicconi et al., 2016; Rousselot et al., 2017; Figure 1).
Acknowledgment of a Novel Therapy Concept
The review shows that even refractory metastatic tumor disease may respond to induction of anakoinosis: Moreover, independent of the histologic tumor type, anakoinosis inducing therapies are available for the successful treatment of refractory metastatic tumor stage (Figure 1).
Four communication-derived targets are accessible for anakoinosis-inducing therapy approaches: Figure 1 summarizes clinically observed events induced by anakoinosis in metastatic (refractory) neoplasia (Hart et al., 2015; Walter et al., 2017).
Clinical results from anakoinotic therapy approaches may topographically map four communication levels: Pro-anakoinotic combination therapies simultaneously modulate the single cell phenotype (Klobuch et al., 2018), but also tumor-stroma-cell, tumor-organ (Hart et al., 2016) and tumor-organism interactions (Muqaku et al., 2017), as particularly indicated in metastatic hepatocellular carcinoma or (uveal) melanoma (Hart et al., 2016; Walter et al., 2017). The clinical results depict also four communication tools, available for targeting: The holistic communicative system, i.e., specific tumor-stroma-organ-organism interactions, modularity, rationalizations, i.e., the physical constitution of biologic hallmarks‘, and the specific metabolism of evolution, given by a distinct cellular context at an organ site (Box 1).
Known prognostic parameters, such as therapy refractoriness in neoplasia, the specific mutations in Philadelphia chromosome in chronic myelocytic leukemia (Rousselot et al., 2017), FLT3 positivity in acute promyelocytic leukemia treated with all-trans retinoic acid plus arsenic trioxide (Cicconi et al., 2016), may ultimately lose their exclusive unfavorable prognostic significance by induction of anoikis, programmed cell death (Hart et al., 2015). Particularly, metronomic low-dose chemotherapy plus pioglitazone and COX-2 inhibitor separated PPARγ expression as late-stage prognostic parameter (Meyer et al., 2009).
The evidences are so strong as to indicate that anakoinosis represents a substantive therapy principle besides novel targeted therapies. Routine pathophysiological studies including homeostatic pathways, down-regulated tumor suppressor genes etc. are now in the center of diagnostic interest for guiding pro-anakoinotic therapy approaches. Here we still find a big diagnostic gap (Figure 1).
Perspectives
Do tumor systems' complexity and the myriad of reductionist therapeutic approaches targeting separately tumor or stroma cells precede the simplicity of anakoinosis inducing treatment strategies (Reichle and Hildebrandt, 2009)?
Anakoinosis may induce a broad spectrum of palliative effects, moreover, continuous complete remission in refractory neoplasia (Hart et al., 2015). Indeed, anakoinosis inducing therapies may circumvent the problem of “undruggable targets” and cope with the general therapeutic problem of (molecular-) genetic tumor heterogeneity (Walter et al., 2017). In addition, pro-anakoinotic schedules may directly target tumor stem cells (Katoh and Katoh, 2007; Prost et al., 2015; Zhang et al., 2016; André et al., 2017).
The basic concept looms that fixed combinations of pro-anakoinotically acting drugs are available for treatment of quite different tumor histologies. Nevertheless, the respective therapy “cocktails” may be adopted according to specific, convergent organized systems rationalizations of cancer hallmarks, probably shared by different tumor histologies (Box 1; Hart et al., 2015). Pro-anakoinotic therapies may be specified according to the tumors' ontology (e.g., liver cirrhosis, myelodysplasia etc.), but also directed to the individual response patterns of the organism in response to the tumor disease, for example in case of cachexia (Hart et al., 2015; Muqaku et al., 2017). These multi-level communication tools are becoming accessible now by anakoinosis inducing therapy approaches, and spotlight again the impact of multi-dimensional communication levels on tumor promotion. We are just in the beginnings for appropriate diagnostic and therapeutic steps in this direction.
Anakoinosis inducing therapies successfully integrate many supplementary classic targeted approaches, as shown by initial results with mTor inhibitors, imatinib and bevacizumab, respectively (Table 1).
Anakoinotic processes may principally cope with fundamental obstacles of classic targeted therapies, with tumor heterogeneity and poor risk parameters, with context-dependent validity and denotation of tumor-promoting aberrations and targets, with drug resistance or undruggable targets by targeting dynamic evolutionary processes, for example multifaceted biologic steps necessary for establishing “active” long-term tumor control or continuous complete remission due to drug and tumor specific host responses (Box 1; Hart et al., 2015). Pro-anakoinotic therapies may inhibit further metastatic progression in case of metastatic disease (Figure 1; Reichle and Vogt, 2008).
A series of multicenter randomized phase II trials with anakoinosis inducing therapies, initiated by the University Hospital Regensburg, currently enrolling, include refractory acute myelocytic leukemia, castration-refractory prostate cancer, metastatic melanoma and non-small cell lung cancer. Large European trials on promyelocytic leukemia or chronic myelocytic leukemia are on the way.
Anakoinosis provides a novel therapy strategy for controlling even therapy-resistant metastatic tumor disease. The novel therapy principle draws on “old” drugs, and promotes drug repurposing in a rational way, oriented at recessive tumor associated events (Hart et al., 2015; Boyd et al., 2017; Walter et al., 2017).
Tissue engineering procedures may also implement anakoinosis for integrating multiple cell systems.
By introducing pro-anakoinotic schedules, innovation must not be adapted to local budget constraints to meet an area's need, e.g., in low-budget countries (Bouche, 2017), but may be universally applicable as novel therapeutic principle.
Author Contributions
DH, AR, LG, and CG conceived the meta-analysis. DH, LG, CG, and AR wrote the manuscript. LG and CG supported through interpretation of data for the work. All the authors revised the manuscript critically, approved the final manuscript, and agreed to be accountable for all aspects of the manuscript.
Conflict of Interest Statement
The authors declare that the research was conducted in the absence of any commercial or financial relationships that could be construed as a potential conflict of interest.
References
(1999). Interferon-a and survival in metastatic renal carcinoma: early results of a randomised controlled trial. Medical Research Council Renal Cancer Collaborators. Lancet 353, 14–17.
Agha, A., and Tarhini, A. A. (2017). Adjuvant therapy for melanoma. Curr. Oncol. Rep. 19:36. doi: 10.1007/s11912-017-0594-5
Ahmadiyeh, N., Pomerantz, M. M., Grisanzio, C., Herman, P., Jia, L., Almendro, V., et al. (2010). 8q24 prostate, breast, and colon cancer risk loci show tissue-specific long-range interaction with MYC. Proc. Natl. Acad. Sci. U. S. A. 107, 9742–9746. doi: 10.1073/pnas.0910668107
Allgayer, H. (2018). The genomic landscape characterizing metastasis. Cancer Microenviron. 2018 (Suppl. 1), 59–60.
Altucci, L., Leibowitz, M. D., Ogilvie, K. M., Lera, A. R., de, and Gronemeyer, H. (2007). RAR and RXR modulation in cancer and metabolic disease. Nat. Rev. Drug Discov. 6, 793–810. doi: 10.1038/nrd2397
André, N., Tsai, K., Carré, M., and Pasquier, E. (2017). Metronomic chemotherapy: direct targeting of cancer cells after all? Trends Cancer 3, 319–325. doi: 10.1016/j.trecan.2017.03.011
Ansell, S. M. (2015). Hodgkin lymphoma: diagnosis and treatment. Mayo Clin. Proc. 90, 1574–1583. doi: 10.1016/j.mayocp.2015.07.005
Attia, S., Eickhoff, J., Wilding, G., McNeel, D., Blank, J., Ahuja, H., et al. (2008). Randomized, double-blinded phase II evaluation of docetaxel with or without doxercalciferol in patients with metastatic, androgen-independent prostate cancer. Clin. Cancer Res. 14, 2437–2443. doi: 10.1158/1078-0432.CCR-07-4274
Aviles, A., Neri, N., Fernandez-Diez, J., Silva, L., and Nambo, M.-J. (2015). Interferon and low doses of methotrexate versus interferon and retinoids in the treatment of refractory/relapsed cutaneous T-cell lymphoma. Hematology 20, 538–542. doi: 10.1179/1607845415Y.0000000002
Bahr, C., Paleske, L., von, Uslu, V. V., Remeseiro, S., Takayama, N., Ng, S. W., et al. (2018). A Myc enhancer cluster regulates normal and leukaemic haematopoietic stem cell hierarchies. Nature 553, 515–520. doi: 10.1038/nature25193
Bastion, Y., Blay, J. Y., Divine, M., Brice, P., Bordessoule, D., Sebban, C., et al. (1997). Elderly patients with aggressive non-Hodgkin's lymphoma: disease presentation, response to treatment, and survival–a Groupe d'Etude des Lymphomes de l'Adulte study on 453 patients older than 69 years. J. Clin. Oncol. 15, 2945–2953. doi: 10.1200/JCO.1997.15.8.2945
Basu, P., Jenson, A. B., Majhi, T., Choudhury, P., Mandal, R., Banerjee, D., et al. (2016). Phase 2 randomized controlled trial of radiation therapy plus concurrent interferon-alpha and retinoic acid versus cisplatin for stage III cervical carcinoma. Int. J. Radiat. Oncol. Biol. Phys. 94, 102–110. doi: 10.1016/j.ijrobp.2015.09.040
Benboubker, L., Dimopoulos, M. A., Dispenzieri, A., Catalano, J., Belch, A. R., Cavo, M., et al. (2014). Lenalidomide and dexamethasone in transplant-ineligible patients with myeloma. N. Engl. J. Med. 371, 906–917. doi: 10.1056/NEJMoa1402551
Berger, A. H., Knudson, A. G., and Pandolfi, P. P. (2011). A continuum model for tumour suppression. Nature 476, 163–169. doi: 10.1038/nature10275
Bertolini, F., Sukhatme, V. P., and Bouche, G. (2015). Drug repurposing in oncology–patient and health systems opportunities. Nat. Revi. Clin. Oncol. 12, 732–742. doi: 10.1038/nrclinonc.2015.169
Beyar-Katz, O., Magidey, K., Ben-Tsedek, N., Alishekevitz, D., Timaner, M., Miller, V., et al. (2016). Bortezomib-induced pro-inflammatory macrophages as a potential factor limiting anti-tumour efficacy. J. Pathol. 239, 262–273. doi: 10.1002/path.4723
Bittenbring, J. T., Neumann, F., Altmann, B., Achenbach, M., Reichrath, J., Ziepert, M., et al. (2014). Vitamin D deficiency impairs rituximab-mediated cellular cytotoxicity and outcome of patients with diffuse large B-cell lymphoma treated with but not without rituximab. J. Clin. Oncol. 32, 3242–3248. doi: 10.1200/JCO.2013.53.4537
Bocci, G., and Kerbel, R. S. (2016). Pharmacokinetics of metronomic chemotherapy: a neglected but crucial aspect. Nat. Rev. Clin. Oncol. 13, 659–673. doi: 10.1038/nrclinonc.2016.64
Bottini, A., Generali, D., Brizzi, M. P., Fox, S. B., Bersiga, A., and Bonardi, S. (2006). Randomized phase II trial of letrozole and letrozole plus low-dose metronomic oral cyclophosphamide as primary systemic treatment in elderly breast cancer patients. J. Clin. Oncol. 24, 3623–3628. doi: 10.1200/JCO.2005.04.5773
Bouche, G. (2017). Cancer care: Tap latent source of frugal cancer ideas. Nature 550:188. doi: 10.1038/550188a
Bousquet, C., Lasfargues, C., Chalabi, M., Billah, S. M., Susini, C., Vezzosi, D., et al. (2012). Clinical review: current scientific rationale for the use of somatostatin analogs and mTOR inhibitors in neuroendocrine tumor therapy. J. Clin. Endocrinol. Metab. 97, 727–737. doi: 10.1210/jc.2011-2088
Boyd, A. L., Reid, J. C., Salci, K. R., Aslostovar, L., Benoit, Y. D., Shapovalova, Z., et al. (2017). Acute myeloid leukaemia disrupts endogenous myelo-erythropoiesis by compromising the adipocyte bone marrow niche. Nat. Cell Biol. 19, 1336–1347. doi: 10.1038/ncb3625
Bradner, J. E., Hnisz, D., and Young, R. A. (2017). Transcriptional Addiction in Cancer. Cell 168, 629–643. doi: 10.1016/j.cell.2016.12.013
Braud, A.-C., Gonzague, L., Bertucci, F., Genre, D., Camerlo, J., Gravis, G., et al. (2002). Retinoids, cisplatin and interferon-α in recurrent or metastatic cervical squamous cell carcinoma: clinical results of 2 phase II trials. European Cytokine Netw. 13, 115–120.
Browman, G. P., Bergsagel, D., Sicheri, D., O'Reilly, S., Wilson, K. S., Rubin, S., et al. (1995). Randomized trial of interferon maintenance in multiple myeloma: a study of the National Cancer Institute of Canada Clinical Trials Group. J. Clin. Oncol. 13, 2354–2360. doi: 10.1200/JCO.1995.13.9.2354
Buer, J., Probst, M., Ganser, A., and Atzpodien, J. (1995). Response to 13-cis-retinoic acid plus interferon alfa-2a in two patients with therapy-refractory advanced renal cell carcinoma. J. Clin. Oncol. 13, 2679–2680. doi: 10.1200/JCO.1995.13.10.2679
Burstein, H. J., Demetri, G. D., Mueller, E., Sarraf, P., Spiegelman, B. M., and Winer, E. P. (2003). Use of the peroxisome proliferator-activated receptor (PPAR) gamma ligand troglitazone as treatment for refractory breast cancer: a phase II study. Breast Cancer Res. Treat. 79, 391–397. doi: 10.1023/A:1024038127156
Caballero-Velázquez, T., Montero, I., Sánchez-Guijo, F., Parody, R., Saldaña, R., Valcarcel, D., et al. (2016). Immunomodulatory effect of vitamin D after allogeneic stem cell transplantation: results of a prospective multicenter clinical trial. Clin. Cancer Res. 22, 5673–5681. doi: 10.1158/1078-0432.CCR-16-0238
Cao, L.-Q., Chen, X.-L., Wang, Q., Huang, X.-H., Zhen, M.-C., Zhang, L.-J., et al. (2007). Upregulation of PTEN involved in rosiglitazone-induced apoptosis in human hepatocellular carcinoma cells. Acta Pharmacol. Sin. 28, 879–887. doi: 10.1111/j.1745-7254.2007.00571.x
Chae, Y. K., Arya, A., Malecek, M.-K., Shin, D. S., Carneiro, B., Chandra, S., et al. (2016). Repurposing metformin for cancer treatment: current clinical studies. Oncotarget 7, 40767–40780. doi: 10.18632/oncotarget.8194
Chanan-Khan, A. A., Niesvizky, R., Hohl, R. J., Zimmerman, T. M., Christiansen, N. P., Schiller, G. J., et al. (2009). Phase III randomised study of dexamethasone with or without oblimersen sodium for patients with advanced multiple myeloma. Leuk. Lymphoma 50, 559–565. doi: 10.1080/10428190902748971
Chlebowski, R. T., Anderson, G. L., Sarto, G. E., Haque, R., Runowicz, C. D., Aragaki, A. K., et al. (2016). Continuous combined estrogen plus progestin and endometrial cancer: the women's health initiative randomized trial. J. Natl. Cancer Inst. 108:djv350. doi: 10.1093/jnci/djv350
Chmielewski, M., Hombach, A. A., and Abken, H. (2014). Of CARs and TRUCKs: chimeric antigen receptor (CAR) T cells engineered with an inducible cytokine to modulate the tumor stroma. Immunol. Rev. 257, 83–90. doi: 10.1111/imr.12125
Ciccolini, J., Barbolosi, D., Meille, C., Lombard, A., Serdjebi, C., Giacometti, S., et al. (2017). Pharmacokinetics and pharmacodynamics-based mathematical modeling identifies an optimal protocol for metronomic chemotherapy. Cancer Res. 77, 4723–4733. doi: 10.1158/0008-5472.CAN-16-3130
Cicconi, L., Divona, M., Ciardi, C., Ottone, T., Ferrantini, A., Lavorgna, S., et al. (2016). PML-RARα kinetics and impact of FLT3-ITD mutations in newly diagnosed acute promyelocytic leukaemia treated with ATRA and ATO or ATRA and chemotherapy. Leukemia 30, 1987–1992. doi: 10.1038/leu.2016.122
Cimino, G., Lo-Coco, F., Fenu, S., Travaglini, L., Finolezzi, E., Mancini, M., et al. (2006). Sequential valproic acid/all-trans retinoic acid treatment reprograms differentiation in refractory and high-risk acute myeloid leukemia. Cancer Res. 66, 8903–8911.
Clarke, J. L., Iwamoto, F. M., Sul, J., Panageas, K., Lassman, A. B., DeAngelis, L. M., et al. (2009). Randomized phase II trial of chemoradiotherapy followed by either dose-dense or metronomic temozolomide for newly diagnosed glioblastoma. J. Clin. Oncol. 27, 3861–3867. doi: 10.1200/JCO.2008.20.7944
Coras, B., Hafner, C., Reichle, A., Hohenleutner, U., Szeimies, R.-M., Landthaler, M., et al. (2004). Antiangiogenic therapy with pioglitazone, rofecoxib, and trofosfamide in a patient with endemic kaposi sarcoma. Arch. Dermatol. 140, 1504–1507. doi: 10.1001/archderm.140.12.1504
Coyle, C., Cafferty, F. H., Vale, C., and Langley, R. E. (2016). Metformin as an adjuvant treatment for cancer: a systematic review and meta-analysis. Ann. Oncol. 27, 2184–2195. doi: 10.1093/annonc/mdw410
Dang, L., Yen, K., and Attar, E. C. (2016). IDH mutations in cancer and progress toward development of targeted therapeutics. Ann. Oncol. 27, 599–608. doi: 10.1093/annonc/mdw013
Dawson, M. A., Prinjha, R. K., Dittmann, A., Giotopoulos, G., Bantscheff, M., Chan, W.-I., et al. (2011). Inhibition of BET recruitment to chromatin as an effective treatment for MLL-fusion leukaemia. Nature 478, 529–533. doi: 10.1038/nature10509
Debrock, G., Vanhentenrijk, V., Sciot, R., Debiec-Rychter, M., Oyen, R., and van Oosterom, A. (2003). A phase II trial with rosiglitazone in liposarcoma patients. Br. J. Cancer 89, 1409–1412. doi: 10.1038/sj.bjc.6601306
Dellapasqua, S., Bertolini, F., Bagnardi, V., Campagnoli, E., Scarano, E., Torrisi, R., et al. (2008). Metronomic cyclophosphamide and capecitabine combined with bevacizumab in advanced breast cancer. J. Clin. Oncol. 26, 4899–4905. doi: 10.1200/JCO.2008.17.4789
Demetri, G. D., Fletcher, C. D., Mueller, E., Sarraf, P., Naujoks, R., Campbell, N., et al. (1999). Induction of solid tumor differentiation by the peroxisome proliferator-activated receptor-γ ligand troglitazone in patients with liposarcoma. Proc. Natl. Acad. Sci. U.S.A. 96, 3951–3956. doi: 10.1073/pnas.96.7.3951
Di Masi, A., Leboffe, L., Marinis, E., Pagano, F., Cicconi, L., Rochette-Egly, C., et al. (2015). Retinoic acid receptors: from molecular mechanisms to cancer therapy. Mol. Aspects Med. 41, 1–115. doi: 10.1016/j.mam.2014.12.003
Di Trolio, R., Simeone, E., Di Lorenzo, G., Buonerba, C., and Ascierto, P. A. (2015). The use of interferon in melanoma patients: a systematic review. Cytokine Growth Factor Rev. 26, 203–212. doi: 10.1016/j.cytogfr.2014.11.008
Dimopoulos, M., Spencer, A., Attal, M., Prince, H. M., Harousseau, J.-L., Dmoszynska, A., et al. (2007). Lenalidomide plus dexamethasone for relapsed or refractory multiple myeloma. New Engl. J. Med. 357, 2123–2132. doi: 10.1056/NEJMoa070594
Dimopoulos, M. A., Kiamouris, C., Gika, D., Deliveliotis, C., Giannopoulos, A., Zervas, A., et al. (2004). Combination of LHRH analog with somatostatin analog and dexamethasone versus chemotherapy in hormone-refractory prostate cancer: a randomized phase II study. Urology 63, 120–125. doi: 10.1016/j.urology.2003.08.041
DiNardo, C. D., Stein, E. M., Botton, S., de, Roboz, G. J., Altman, J. K., Mims, A. S., et al. (2018). Durable Remissions with Ivosidenib in IDH1-Mutated Relapsed or Refractory AML. N. Engl. J. Med. 378, 2386–2398. doi: 10.1056/NEJMoa1716984
Dummer, R., Beyer, M., Hymes, K., Epping, M. T., Bernards, R., Steinhoff, M., et al. (2012). Vorinostat combined with bexarotene for treatment of cutaneous T-cell lymphoma: in vitro and phase I clinical evidence supporting augmentation of retinoic acid receptor/retinoid X receptor activation by histone deacetylase inhibition. Leuk. Lymphoma 53, 1501–1508. doi: 10.3109/10428194.2012.656625
Dvorak, H. F. (2015). Tumors: wounds that do not heal-redux. Cancer Immunol. Res. 3, 1–11. doi: 10.1158/2326-6066.CIR-14-0209
Eberhardt, N. L., Grebe, S. K. G., McIver, B., and Reddi, H. V. (2010). The role of the PAX8/PPARgamma fusion oncogene in the pathogenesis of follicular thyroid cancer. Mol. Cell. Endocrinol. 321, 50–56. doi: 10.1016/j.mce.2009.10.013
Edelman, M. J., Wang, X., Hodgson, L., Cheney, R. T., Baggstrom, M. Q., Thomas, S. P., et al. (2017). Phase III randomized, placebo-controlled, double-blind trial of celecoxib in addition to standard chemotherapy for advanced non-small-cell lung cancer with cyclooxygenase-2 overexpression: CALGB 30801 (Alliance). J. Clin. Oncol. 35, 2184–2192. doi: 10.1200/JCO.2016.71.3743
Efficace, F., Mandelli, F., Avvisati, G., Cottone, F., Ferrara, F., Di Bona, E., et al. (2014). Randomized phase III trial of retinoic acid and arsenic trioxide versus retinoic acid and chemotherapy in patients with acute promyelocytic leukemia: health-related quality-of-life outcomes. J. Clin. Oncol. 32, 3406–3412. doi: 10.1200/JCO.2014.55.3453
Eggermont, A. M. M., Suciu, S., Santinami, M., Testori, A., Kruit, W. H. J., Marsden, J., et al. (2008). Adjuvant therapy with pegylated interferon alfa-2b versus observation alone in resected stage III melanoma: final results of EORTC 18991, a randomised phase III trial. Lancet 372, 117–126. doi: 10.1016/S0140-6736(08)61033-8
Elrod, H. A., and Sun, S.-Y. (2008). PPARgamma and apoptosis in cancer. PPAR Res. 2008:704165. doi: 10.1155/2008/704165
Erdmann, E., Harding, S., Lam, H., and Perez, A. (2016). Ten-year observational follow-up of PROactive: a randomized cardiovascular outcomes trial evaluating pioglitazone in type 2 diabetes. Diabetes Obes. Metab. 18, 266–273. doi: 10.1111/dom.12608
Escudier, B., Pluzanska, A., Koralewski, P., Ravaud, A., Bracarda, S., Szczylik, C., et al. (2007). Bevacizumab plus interferon alfa-2a for treatment of metastatic renal cell carcinoma: a randomised, double-blind phase III trial. Lancet 370, 2103–2111. doi: 10.1016/S0140-6736(07)61904-7
Faiss, S., Pape, U.-F., Böhmig, M., Dörffel, Y., Mansmann, U., Golder, W., et al. (2003). Prospective, randomized, multicenter trial on the antiproliferative effect of lanreotide, interferon alfa, and their combination for therapy of metastatic neuroendocrine gastroenteropancreatic tumors–the International Lanreotide and Interferon Alfa Study Group. J. Clin. Oncol. 21, 2689–2696. doi: 10.1200/JCO.2003.12.142
Fazio, N., Braud, F. d., Delle Fave, G., and Oberg, K. (2007). Interferon-α and somatostatin analog in patients with gastroenteropancreatic neuroendocrine carcinoma: single agent or combination? Ann. Oncol. 18, 13–19. doi: 10.1093/annonc/mdl144
Feldman, D., Krishnan, A. V., Swami, S., Giovannucci, E., and Feldman, B. J. (2014). The role of vitamin D in reducing cancer risk and progression. Nat. Revi. Cancer 14, 342-357. doi: 10.1038/nrc3691
Flaherty, K. T., Puzanov, I., Kim, K. B., Ribas, A., McArthur, G. A., Sosman, J. A., et al. (2010). Inhibition of mutated, activated BRAF in metastatic melanoma. N. Engl. J. Med. 363, 809–819. doi: 10.1056/NEJMoa1002011
Foss, F., Demierre, M. F., and DiVenuti, G. (2005). A phase-1 trial of bexarotene and denileukin diftitox in patients with relapsed or refractory cutaneous T-cell lymphoma. Blood 106, 454–457. doi: 10.1182/blood-2004-11-4570
Fratta, E., Sigalotti, L., Covre, A., Parisi, G., Coral, S., and Maio, M. (2013). Epigenetics of melanoma: implications for immune-based therapies. Immunotherapy 5, 1103–1116. doi: 10.2217/imt.13.108
Fröhlich, E., and Wahl, R. (2015). Chemotherapy and chemoprevention by thiazolidinediones. Biomed Res. Int. 2015:845340. doi: 10.1155/2015/845340
Gerner, C., Neuditschko, B., Del Favero, G., Janker, L., Marko, D., and Keppler, B. (2018). From cutaneous metastasis tob rain metastasis: proteome signatures and functional features of melanoma cells. Cancer Microenviron. (Suppl.1), 29–30.
Giovannelli, P., Di Donato, M., Giraldi, T., Migliaccio, A., Castoria, G., and Auricchio, F. (2012). Targeting rapid action of sex-steroid receptors in breast and prostate cancers. Front. Biosci. 4, 453–461. doi: 10.2741/3849
Goldberg, J. S., Vargas, M., Rosmarin, A. S., Milowsky, M. I., Papanicoloau, N., Gudas, L. J., et al. (2002). Phase I trial of interferon alpha2b and liposome-encapsulated all-trans retinoic acid in the treatment of patients with advanced renal cell carcinoma. Cancer 95, 1220–1227. doi: 10.1002/cncr.10809
Graham, L., and Schweizer, M. T. (2016). Targeting persistent androgen receptor signaling in castration-resistant prostate cancer. Med. Oncol. 33, 44. doi: 10.1007/s12032-016-0759-3
Hart, C., Vogelhuber, M., Hafner, C., Landthaler, M., Berneburg, M., Haferkamp, S., et al. (2016). Biomodulatory metronomic therapy in stage IV melanoma is well-tolerated and may induce prolonged progression-free survival, a phase I trial. J. Eur. Acad. Dermatol. Venereol. 30, e119–e121. doi: 10.1111/jdv.13391
Hart, C., Vogelhuber, M., Wolff, D., Klobuch, S., Ghibelli, L., Foell, J., et al. (2015). Anakoinosis: Communicative reprogramming of tumor systems - for rescuing from chemorefractory neoplasia. Cancer Microenviron. 8, 75–92. doi: 10.1007/s12307-015-0170-1
Hau, P., Kunz-Schughart, L., Bogdahn, U., Baumgart, U., Hirschmann, B., Weimann, E., et al. (2007). Low-dose chemotherapy in combination with COX-2 inhibitors and PPAR-gamma agonists in recurrent high-grade gliomas-a phase II study. Oncology 73, 21–25. doi: 10.1159/000120028
Herold, M., Scholz, C. W., Rothmann, F., Hirt, C., Lakner, V., and Naumann, R. (2015). Long-term follow-up of rituximab plus first-line mitoxantrone, chlorambucil, prednisolone and interferon-alpha as maintenance therapy in follicular lymphoma. J. Cancer Res. Clin. Oncol. 141, 1689–1695. doi: 10.1007/s00432-015-1963-9
Heudobler, D., Klobuch, S., Thomas, S., Hahn, J., Herr, W., and Reichle, A. (2018a). Cutaneous leukemic infiltrates successfully treated with biomodulatory therapy in a rare case of therapy-related high risk MDS/AML. Front. Pharmacol. 9:1279. doi: 10.3389/fphar.2018.01279
Heudobler, D., Rechenmacher, M., Lüke, F., Vogelhuber, M., Pukrop, T., Herr, W., et al. (2018b). Peroxisome proliferator-activated receptors (PPAR)γ agonists as master modulators of tumor tissue. Int. J. Mol. Sci. 19:E3540. doi: 10.3390/ijms19113540
Heudobler, D., Rehe, K., Foell, J., Corbacioglu, S., Hildebrandt, G., Herr, W., et al. (2016). Biomodulatory metronomic therapy shows remarkable activity in chemorefractory multi-system langerhans cell histiocytosis. Blood 128:4254.
Higurashi, T., Hosono, K., Takahashi, H., Komiya, Y., Umezawa, S., Sakai, E., et al. (2016). Metformin for chemoprevention of metachronous colorectal adenoma or polyps in post-polypectomy patients without diabetes: a multicentre double-blind, placebo-controlled, randomised phase 3 trial. Lancet Oncol. 17, 475–483. doi: 10.1016/S1470-2045(15)00565-3
Hill, M., Norman, A., Cunningham, D., Findlay, M., Nicolson, V., Hill, A., et al. (1995). Royal Marsden phase III trial of fluorouracil with or without interferon alfa-2b in advanced colorectal cancer. J. Clin. Oncol. 13, 1297–1302. doi: 10.1200/JCO.1995.13.6.1297
Hnisz, D., Abraham, B. J., Lee, T. I., Lau, A., Saint-André, V., Sigova, A. A., et al. (2013). Super-enhancers in the control of cell identity and disease. Cell 155, 934–947. doi: 10.1016/j.cell.2013.09.053
Hnisz, D., Schuijers, J., Lin, C. Y., Weintraub, A. S., Abraham, B. J., Lee, T. I., et al. (2015). Convergence of developmental and oncogenic signaling pathways at transcriptional super-enhancers. Mol. Cell 58, 362–370. doi: 10.1016/j.molcel.2015.02.014
Huang, J., Li, K., Cai, W., Liu, X., Zhang, Y., Orkin, S. H., et al. (2018). Dissecting super-enhancer hierarchy based on chromatin interactions. Nat. Commun. 9:943. doi: 10.1038/s41467-018-03279-9
Iland, H. J., and Seymour, J. F. (2013). Role of arsenic trioxide in acute promyelocytic leukemia. Curr. Treat. Options Oncol. 14, 170–184. doi: 10.1007/s11864-012-0223-3
Jin, Y., Chen, K., Paepe, A., de, Hellqvist, E., Krstic, A. D., Metang, L., et al. (2018). Active enhancer and chromatin accessibility landscapes chart the regulatory network of primary multiple myeloma. Blood 131, 2138–2150. doi: 10.1182/blood-2017-09-808063
Katoh, M., and Katoh, M. (2007). WNT signaling pathway and stem cell signaling network. Clin. Cancer Res. 13, 4042–4045. doi: 10.1158/1078-0432.CCR-06-2316
Kelly, J. L., Salles, G., Goldman, B., Fisher, R. I., Brice, P., Press, O., et al. (2015). Low serum vitamin D levels are associated with inferior survival in follicular lymphoma: a prospective evaluation in SWOG and LYSA studies. J. Clin. Oncol. 33, 1482–1490. doi: 10.1200/JCO.2014.57.5092
Kerbel, R. S., and Shaked, Y. (2017). The potential clinical promise of ‘multimodality’ metronomic chemotherapy revealed by preclinical studies of metastatic disease. Cancer Lett. 400, 293–304. doi: 10.1016/j.canlet.2017.02.005
Kim, T. K., Gore, S. D., and Zeidan, A. M. (2015). Epigenetic therapy in acute myeloid leukemia: current and future directions. Semin. Hematol. 52, 172–183. doi: 10.1053/j.seminhematol.2015.04.003
Klobuch, S., Steinberg, T., Bruni, E., Mirbeth, C., Heilmeier, B., Ghibelli, L., et al. (2018). Biomodulatory treatment with azacitidine, all-trans retinoic acid and pioglitazone induces differentiation of primary AML blasts into neutrophil like cells capable of ROS production and phagocytosis. Front. Pharmacol. doi: 10.3389/fphar.2018.01380
Kopetz, S., Desai, J., Chan, E., Hecht, J. R., O'Dwyer, P. J., Maru, D., et al. (2015). Phase II pilot study of vemurafenib in patients with metastatic BRAF-mutated colorectal cancer. J. Clin. Oncol. 33, 4032–4038. doi: 10.1200/JCO.2015.63.2497
Koutsilieris, M., Bogdanos, J., Milathianakis, C., Dimopoulos, P., Dimopoulos, T., Karamanolakis, D., et al. (2006). Combination therapy using LHRH and somatostatin analogues plus dexamethasone in androgen ablation refractory prostate cancer patients with bone involvement: a bench to bedside approach. Expert Opin. Investig. Drugs 15, 795–804. doi: 10.1517/13543784.15.7.795
Krilis, M., Tsang, H., and Coroneo, M. (2012). Treatment of conjunctival and corneal epithelial neoplasia with retinoic acid and topical interferon alfa-2b: long-term follow-up. Ophthalmology 119, 1969–1973. doi: 10.1016/j.ophtha.2012.03.045
Kulke, M. H., Demetri, G. D., Sharpless, N. E., Ryan, D. P., Shivdasani, R., Clark, J. S., et al. (2002). A phase II study of troglitazone, an activator of the PPARgamma receptor, in patients with chemotherapy-resistant metastatic colorectal cancer. Cancer J. 8, 395–399. doi: 10.1097/00130404-200209000-00010
Kummar, S., Wade, J. L., Oza, A. M., Sullivan, D., Chen, A. P., Gandara, D. R., et al. (2016). Randomized phase II trial of cyclophosphamide and the oral poly (ADP-ribose) polymerase inhibitor veliparib in patients with recurrent, advanced triple-negative breast cancer. Invest. New Drugs 34, 355–363. doi: 10.1007/s10637-016-0335-x
Lecarpentier, Y., Claes, V., Vallée, A., and Hébert, J.-L. (2017). Interactions between PPAR gamma and the canonical Wnt/Beta-catenin pathway in Type 2 diabetes and colon cancer. PPAR Res. 2017:5879090. doi: 10.1155/2017/5879090
Lissoni, P., Barni, S., Tancini, G., Mainini, E., Piglia, F., Maestroni, G. J., et al. (1995). Immunoendocrine therapy with low-dose subcutaneous interleukin-2 plus melatonin of locally advanced or metastatic endocrine tumors. Oncology 52, 163–166. doi: 10.1159/000227450
Lo-Coco, F., Avvisati, G., Vignetti, M., Thiede, C., Orlando, S. M., Iacobelli, S., et al. (2013). Retinoic acid and arsenic trioxide for acute promyelocytic leukemia. N. Engl. J. Med. 369, 111–121. doi: 10.1056/NEJMoa1300874
Lønning, P. E., Taylor, P. D., Anker, G., Iddon, J., Wie, L., Jørgensen, L. M., et al. (2001). High-dose estrogen treatment in postmenopausal breast cancer patients heavily exposed to endocrine therapy. Breast Cancer Res. Treat. 67, 111–116. doi: 10.1023/A:1010619225209
Mack, S. C., Pajtler, K. W., Chavez, L., Okonechnikov, K., Bertrand, K. C., Wang, X., et al. (2018). Therapeutic targeting of ependymoma as informed by oncogenic enhancer profiling. Nature 553, 101–105. doi: 10.1038/nature25169
Mantovani, G., Macciò, A., Lai, P., Massa, E., Massa, D., Mulas, C., et al. (2000). Results of a dose-intense phase 1 study of a combination chemotherapy regimen with cisplatin and epidoxorubicin including medroxyprogesterone acetate and recombinant interleukin-2 in patients with inoperable primary lung cancer. J. Immunother. 23, 267–274.
Masood, R., Nagpal, S., Zheng, T., Cai, J., Tulpule, A., Smith, D. L., et al. (2000). Kaposi sarcoma is a therapeutic target for vitamin D(3) receptor agonist. Blood 96, 3188–3194.
Mayer, R. L., Schwarzmeier, J. D., Gerner, M. C., Bileck, A., Mader, J. C., Meier-Menches, S. M., et al. (2017). Proteomics and metabolomics identify molecular mechanisms of aging potentially predisposing for chronic lymphocytic leukemia. Mol. Cell. Proteomics 17, 290–303. doi: 10.1074/mcp.RA117.000425
McCabe, M. T., Ott, H. M., Ganji, G., Korenchuk, S., Thompson, C., van Aller, G. S., et al. (2012). EZH2 inhibition as a therapeutic strategy for lymphoma with EZH2-activating mutations. Nature 492, 108–112. doi: 10.1038/nature11606
McCarthy, P. L., Holstein, S. A., Petrucci, M. T., Richardson, P. G., Hulin, C., Tosi, P., et al. (2017). Lenalidomide maintenance after autologous stem-cell transplantation in newly diagnosed multiple myeloma: a meta-analysis. J. Clin. Oncol. 35, 3279–3289. doi: 10.1200/JCO.2017.72.6679
McDermott, D. F., Regan, M. M., Clark, J. I., Flaherty, L. E., Weiss, G. R., Logan, T. F., et al. (2005). Randomized phase III trial of high-dose interleukin-2 versus subcutaneous interleukin-2 and interferon in patients with metastatic renal cell carcinoma. J. Clin. Oncol. 23, 133–141. doi: 10.1200/JCO.2005.03.206
Meyer, S., Vogt, T., Landthaler, M., Berand, A., Reichle, A., Bataille, F., et al. (2009). Cyclooxygenase 2 (COX2) and peroxisome proliferator-activated receptor gamma (PPARG) are stage-dependent prognostic markers of malignant melanoma. PPAR Res. 2009:848645. doi: 10.1155/2010/848645
Miller, V. A., Benedetti, F. M., Rigas, J. R., Verret, A. L., Pfister, D. G., Straus, D., et al., (1997). Initial clinical trial of a selective retinoid X receptor ligand, LGD1069. J. Clin. Oncol. 15, 790–795. doi: 10.1200/JCO.1997.15.2.790
Miller, V. A., Rigas, J. R., Benedetti, F. M., Verret, A. L., Tong, W. P., Kris, M. G., et al. (1996). Initial clinical trial of the retinoid receptor pan agonist 9-cis retinoic acid. Clin. Cancer Res. 2, 471–475.
Mitchell, C. D., Richards, S. M., Kinsey, S. E., Lilleyman, J., Vora, A., and Eden, T. O. B. (2005). Benefit of dexamethasone compared with prednisolone for childhood acute lymphoblastic leukaemia: results of the UK Medical Research Council ALL97 randomized trial. Br. J. Haematol. 129, 734–745. doi: 10.1111/j.1365-2141.2005.05509.x
Motzer, R. J., Hutson, T. E., Tomczak, P., Michaelson, M. D., Bukowski, R. M., Rixe, O., et al. (2007). Sunitinib versus interferon alfa in metastatic renal-cell carcinoma. New Engl. J. Med. 356, 115–124. doi: 10.1056/NEJMoa065044
Mueller, E., Smith, M., Sarraf, P., Kroll, T., Aiyer, A., Kaufman, D. S., et al. (2000). Effects of ligand activation of peroxisome proliferator-activated receptor gamma in human prostate cancer. Proc. Natl. Acad. Sci. U.S.A. 97, 10990–10995. doi: 10.1073/pnas.180329197
Mulholland, D. J., Dedhar, S., Coetzee, G. A., and Nelson, C. C. (2005). Interaction of nuclear receptors with the Wnt/beta-catenin/Tcf signaling axis: Wnt you like to know? Endocr. Rev. 26, 898–915. doi: 10.1210/er.2003-0034
Muqaku, B., Eisinger, M., Meier, S. M., Tahir, A., Pukrop, T., Haferkamp, S., et al. (2017). Multi-omics analysis of serum samples demonstrates reprogramming of organ functions via systemic calcium mobilization and platelet activation in metastatic melanoma. Mol. Cell. Proteomics 16, 86–99. doi: 10.1074/mcp.M116.063313
Neelapu, S. S., Locke, F. L., Bartlett, N. L., Lekakis, L. J., Miklos, D. B., Jacobson, C. A., et al. (2017). Axicabtagene Ciloleucel CAR T-Cell therapy in refractory large B-Cell lymphoma. N. Engl. J. Med. 377, 2531–2544. doi: 10.1056/NEJMoa1707447
Nervi, C., Marinis, E., and Codacci-Pisanelli, G. (2015). Epigenetic treatment of solid tumours: a review of clinical trials. Clin. Epigenetics 7, 127. doi: 10.1186/s13148-015-0157-2
Nicolini, A., Carpi, A., Ferrari, P., Biava, P. M., and Rossi, G., (2016). Immunotherapy and hormone-therapy in metastatic breast cancer: a review and an update. Curr. Drug Targets 17, 1127–1139. doi: 10.2174/1389450117666160201114752
Norsa, A., and Martino, V. (2007). Somatostatin, retinoids, melatonin, vitamin D, bromocriptine, and cyclophosphamide in chemotherapy-pretreated patients with advanced lung adenocarcinoma and low performance status. Cancer Biother. Radiopharm. 22, 50–55. doi: 10.1089/cbr.2006.365
Nourmoussavi, M., Pansegrau, G., Popesku, J., Hammond, G. L., Kwon, J. S., and Carey, M. S. (2017). Ovarian ablation for premenopausal breast cancer: a review of treatment considerations and the impact of premature menopause. Cancer Treat. Rev. 55, 26–35. doi: 10.1016/j.ctrv.2017.02.005
Olokpa, E., Moss, P. E., and Stewart, L. V. (2017). Crosstalk between the Androgen receptor and PPAR gamma signaling pathways in the prostate. PPAR Res. 2017:9456020. doi: 10.1155/2017/9456020
Pahler, J. C., Tazzyman, S., Erez, N., Chen, Y.-Y., Murdoch, C., Nozawa, H., et al. (2008). Plasticity in tumor-promoting inflammation: impairment of macrophage recruitment evokes a compensatory neutrophil response. Neoplasia 10, 329–340.
Papi, A., Guarnieri, T., Storci, G., Santini, D., Ceccarelli, C., Taffurelli, M., et al. (2012). Nuclear receptors agonists exert opposing effects on the inflammation dependent survival of breast cancer stem cells. Cell Death Differ. 19, 1208–1219. doi: 10.1038/cdd.2011.207
Papi, A., Rocchi, P., Ferreri, A. M., and Orlandi, M. (2010). RXRgamma and PPARgamma ligands in combination to inhibit proliferation and invasiveness in colon cancer cells. Cancer Lett. 297, 65–74. doi: 10.1016/j.canlet.2010.04.026
Papi, A., Storci, G., Guarnieri, T., Carolis, S., Bertoni, S., Avenia, N., et al. (2013). Peroxisome proliferator activated receptor-α/hypoxia inducible factor-1α interplay sustains carbonic anhydrase IX and apoliprotein E expression in breast cancer stem cells. PLoS ONE 8:e54968. doi: 10.1371/journal.pone.0054968
Papi, A., Tatenhorst, L., Terwel, D., Hermes, M., Kummer, M. P., Orlandi, M., et al. (2009). PPARgamma and RXRgamma ligands act synergistically as potent antineoplastic agents in vitro and in vivo glioma models. J. Neurochem. 109, 1779–1790. doi: 10.1111/j.1471-4159.2009.06111.x
Pasquier, E., André, N., Street, J., Chougule, A., Rekhi, B., Ghosh, J., et al. (2016). Effective management of advanced angiosarcoma by the synergistic combination of propranolol and vinblastine-based metronomic chemotherapy: a bench to bedside study. EBioMedicine 6, 87-95. doi: 10.1016/j.ebiom.2016.02.026
Pitteri, S. J., Kelly-Spratt, K. S., Gurley, K. E., Kennedy, J., Buson, T. B., Chin, A., et al. (2011). Tumor microenvironment-derived proteins dominate the plasma proteome response during breast cancer induction and progression. Cancer Res. 71, 5090–5100. doi: 10.1158/0008-5472.CAN-11-0568
Pomerantz, M., Manola, J., Taplin, M.-E., Bubley, G., Inman, M., Lowell, J., et al. (2007). Phase II study of low dose and high dose conjugated estrogen for androgen independent prostate cancer. J. Urol. 177, 2146–2150. doi: 10.1016/j.juro.2007.01.119
Pramanik, R., Agarwala, S., Gupta, Y. K., Thulkar, S., Vishnubhatla, S., Batra, A., et al. (2017). Metronomic chemotherapy vs best supportive care in progressive pediatric solid malignant tumors: a randomized clinical trial. JAMA Oncol. 3, 1222–1227. doi: 10.1001/jamaoncol.2017.0324
Prost, S., Relouzat, F., Spentchian, M., Ouzegdouh, Y., Saliba, J., Massonnet, G., et al. (2015). Erosion of the chronic myeloid leukaemia stem cell pool by PPARγ agonists. Nature 525, 380–383. doi: 10.1038/nature15248
Quach, H., Ritchie, D., Stewart, A. K., Neeson, P., Harrison, S., Smyth, M. J., et al. (2010). Mechanism of action of immunomodulatory drugs (IMiDS) in multiple myeloma. Leukemia 24, 22–32. doi: 10.1038/leu.2009.236
Querfeld, C., Nagelli, L. V., Rosen, S. T., Kuzel, T. M., and Guitart, J. (2006). Bexarotene in the treatment of cutaneous T-cell lymphoma. Expert Opin. Pharmacother. 7, 907–915. doi: 10.1517/14656566.7.7.907
Querfeld, C., Rosen, S. T., Guitart, J., Rademaker, A., Fung, B. B., Posten, W., et al. (2004). Comparison of selective retinoic acid receptor- and retinoic X receptor-mediated efficacy, tolerance, and survival in cutaneous t-cell lymphoma. J. Am. Acad. Dermatol. 51, 25–32. doi: 10.1016/j.jaad.2003.11.058
Reebye, V., Huang, K.-W., Lin, V., Jarvis, S., Cutilas, P., Dorman, S., et al. (2018). Gene activation of CEBPA using saRNA: preclinical studies of the first in human saRNA drug candidate for liver cancer. Oncogene 37, 3216–3228. doi: 10.1038/s41388-018-0126-2
Reichle, A. (2010). From Molecular to Modular Tumor Therapy: Tumors are Reconstructible Communicatively Evolving Systems. Dordrecht: Springer Science+Business Media B.V.
Reichle, A., Grassinger, J., Bross, K., Wilke, J., Suedhoff, T., Walter, B., et al. (2007a). C-reactive protein in patients with metastatic clear cell renal carcinoma: an important biomarker for tumor-associated inflammation. Biomark. Insights 1, 87–98.
Reichle, A., Hart, C., Grube, M., and Andreesen, R. (2012). Anti-Inflammatory, immuno-modulatory and angiostatic treatment as third-line therapy for multiple myeloma (MM) - a combined treatment setting of lenalidomide with pioglitazone, dexamethasone and low-dose treosulfan (phase I/II). Blood 120:5029.
Reichle, A., and Hildebrandt, G. C. (2009). Principles of modular tumor therapy. Cancer Microenviron. 2(Suppl. 1), 227–237. doi: 10.1007/s12307-009-0023-x
Reichle, A., Lugner, A., Ott, C., Klebl, F., Vogelhuber, M., Berand, A., and Andreesen, R. (2009). Control of cancer-associated inflammation and survival: Results from a prospective randomized phase II trial in gastric cancer. J. Clin. Oncol. 27:15S. doi: 10.1200/jco.2009.27.15s.e15584
Reichle, A., and Vogt, T. (2008). Systems biology: a therapeutic target for tumor therapy. Cancer Microenviron. 1, 159–170. doi: 10.1007/s12307-008-0012-5
Reichle, A., Vogt, T., Coras, B., Terheyden, P., Neuber, K., Trefzer, U., et al. (2007b). Targeted combined anti-inflammatory and angiostatic therapy in advanced melanoma: a randomized phase II trial. Melanoma Res. 17, 360–364. doi: 10.1097/CMR.0b013e3282f1d2c8
Reichle, A., Vogt, T., Kunz-Schughart, L., Bretschneider, T., Bachthaler, M., Bross, K., et al. (2005). Anti-inflammatory and angiostatic therapy in chemorefractory multisystem Langerhans' cell histiocytosis of adults. Br. J. Haematol. 128, 730–732. doi: 10.1111/j.1365-2141.2004.05359.x
Remark, R., Alifano, M., Cremer, I., Lupo, A., Dieu-Nosjean, M.-C., Riquet, M., et al. (2013). Characteristics and clinical impacts of the immune environments in colorectal and renal cell carcinoma lung metastases: influence of tumor origin. Clin. Cancer Res. 19, 4079–4091. doi: 10.1158/1078-0432.CCR-12-3847
Richardson, P. G., Sonneveld, P., Schuster, M. W., Irwin, D., Stadtmauer, E. A., Facon, T., et al. (2005). Bortezomib or high-dose dexamethasone for relapsed multiple myeloma. New Engl. J. Med. 352, 2487–2498. doi: 10.1056/NEJMoa043445
Rochlitz, C., Bigler, M., Moos, R., Bernhard, J., Matter-Walstra, K., Wicki, A., et al. (2016). SAKK 24/09: safety and tolerability of bevacizumab plus paclitaxel vs. bevacizumab plus metronomic cyclophosphamide and capecitabine as first-line therapy in patients with HER2-negative advanced stage breast cancer - a multicenter, randomized phase III trial. BMC Cancer 16:780. doi: 10.1186/s12885-016-2823-y
Romiti, A., Falcone, R., Roberto, M., and Marchetti, P. (2017). Current achievements and future perspectives of metronomic chemotherapy. Invest. New Drugs 35, 359–374. doi: 10.1007/s10637-016-0408-x
Rosner, M., Pham, H. T. T., Moriggl, R., and Hengstschläger, M. (2017). Human stem cells alter the invasive properties of somatic cells via paracrine activation of mTORC1. Nat. Commun. 8:595. doi: 10.1038/s41467-017-00661-x
Roussel, M., Lauwers-Cances, V., Robillard, N., Hulin, C., Leleu, X., Benboubker, L., et al. (2014). Front-line transplantation program with lenalidomide, bortezomib, and dexamethasone combination as induction and consolidation followed by lenalidomide maintenance in patients with multiple myeloma: a phase II study by the Intergroupe Francophone du Myélome. J. Clin. Oncol. 32, 2712–2717. doi: 10.1200/JCO.2013.54.8164
Rousselot, P., Prost, S., Guilhot, J., Roy, L., Etienne, G., Legros, L., et al. (2017). Pioglitazone together with imatinib in chronic myeloid leukemia: a proof of concept study. Cancer 123, 1791–1799. doi: 10.1002/cncr.30490
Ryu, S., Kim, D. S., Lee, M. W., Lee, J. W., Sung, K. W., Koo, H. H., et al. (2018). Anti-leukemic effects of PPARγ ligands. Cancer Lett. 418, 10–19. doi: 10.1016/j.canlet.2018.01.020
San Miguel, J., Weisel, K., Moreau, P., Lacy, M., Song, K., Delforge, M., et al. (2013). Pomalidomide plus low-dose dexamethasone versus high-dose dexamethasone alone for patients with relapsed and refractory multiple myeloma (MM-003): a randomised, open-label, phase 3 trial. Lancet Oncol. 14, 1055–1066. doi: 10.1016/S1470-2045(13)70380-2
Sanchez, D. J., Steger, D. J., Skuli, N., Bansal, A., and Simon, M. C. (2018). PPARγ is dispensable for clear cell renal cell carcinoma progression. Mol. Metab. 14, 139–149. doi: 10.1016/j.molmet.2018.05.013
Sarker, D., Plummer, E.R., Basu, B., Meyer, T., Huang, K.-W., and Evans, T. R. J. (2018). Preliminary results of a first-in-human, first-in-class phase I study of MTL-CEBPA, a small activating RNA (saRNA) targeting the transcription factor C/EBP-α in patients with advanced liver cancer. J. Clin. Oncol. 36(15 Suppl.):2509–2509. doi: 10.1200/JCO.2018.36.15_suppl.2509
Scharla, S. H., Minne, H. W., Waibel-Treber, S., Schaible, A., Lempert, U. G., Wüster, C., et al. (1990). Bone mass reduction after estrogen deprivation by long-acting gonadotropin-releasing hormone agonists and its relation to pretreatment serum concentrations of 1,25-dihydroxyvitamin D3. J. Clin. Endocrinol. Metab. 70, 1055–1061. doi: 10.1210/jcem-70-4-1055
Sciarra, A., Bosman, C., Monti, G., Gentile, V., Autran Gomez, A. M., Ciccariello, M., et al. (2004). Somatostatin analogues and estrogens in the treatment of androgen ablation refractory prostate adenocarcinoma. J. Urol. 172, 1775–1783. doi: 10.1097/01.ju.0000140875.07255.f5
Senerchia, A. A., Macedo, C. R., Ferman, S., Scopinaro, M., Cacciavillano, W., Boldrini, E., et al. (2017). Results of a randomized, prospective clinical trial evaluating metronomic chemotherapy in nonmetastatic patients with high-grade, operable osteosarcomas of the extremities: A report from the Latin American Group of Osteosarcoma Treatment. Cancer 123, 1003–1010. doi: 10.1002/cncr.30411
Sengupta, S., and George, R. E. (2017). Super-enhancer-driven transcriptional dependencies in cancer. Trends Cancer 3, 269–281. doi: 10.1016/j.trecan.2017.03.006
Sharma, R., Hamilton, A., and Beith, J. (2008). LHRH agonists for adjuvant therapy of early breast cancer in premenopausal women. Cochrane Database Syst. Rev. CD004562. doi: 10.1002/14651858.CD004562.pub3
Sidéris, L., Dubé, P., and Rinke, A. (2012). Antitumor effects of somatostatin analogs in neuroendocrine tumors. Oncologist 17, 747–755. doi: 10.1634/theoncologist.2011-0458
Sim, C. K., Kim, S.-Y., Brunmeir, R., Zhang, Q., Li, H., Dharmasegaran, D., et al. (2017). Regulation of white and brown adipocyte differentiation by RhoGAP DLC1. PLoS ONE 12:e0174761. doi: 10.1371/journal.pone.0174761
Simkens, L. H. J., van Tinteren, H., May, A., Tije, A. J., Creemers, G.-J. M., Loosveld, O. J. L., et al. (2015). Maintenance treatment with capecitabine and bevacizumab in metastatic colorectal cancer (CAIRO3): a phase 3 randomised controlled trial of the Dutch Colorectal Cancer Group. Lancet 385, 1843–1852. doi: 10.1016/S0140-6736(14)62004-3
Simonsson, B., Gedde-Dahl, T., Markevärn, B., Remes, K., Stentoft, J., Almqvist, A., et al. (2011). Combination of pegylated IFN-α2b with imatinib increases molecular response rates in patients with low- or intermediate-risk chronic myeloid leukemia. Blood 118, 3228–3235. doi: 10.1182/blood-2011-02-336685
Smith, M. R., Manola, J., Kaufman, D. S., George, D., Oh, W. K., Mueller, E., et al. (2004). Rosiglitazone versus placebo for men with prostate carcinoma and a rising serum prostate-specific antigen level after radical prostatectomy and/or radiation therapy. Cancer 101, 1569–1574. doi: 10.1002/cncr.20493
Steinhoff, M., Beyer, M., Roewert-Huber, J., Lukowsky, A., Assaf, C., and Sterry, W. (2008). Complete clinical remission of tumor-stage mycosis fungoides after acute extensive skin necroses, granulomatous reaction, and fever under treatment with bexarotene, vorinostat, and high-dose fenofibrate. J. Am. Acad. Dermatol. 58, S88–91. doi: 10.1016/j.jaad.2007.07.012
Sverrisdottir, A., Johansson, H., Johansson, U., Bergh, J., Rotstein, S., Rutqist, L., et al. (2011). Interaction between goserelin and tamoxifen in a prospective randomised clinical trial of adjuvant endocrine therapy in premenopausal breast cancer. Breast Cancer Res. Treat. 128, 755–763. doi: 10.1007/s10549-011-1593-0
Taylan, E., and Oktay, K. H. (2017). Current state and controversies in fertility preservation in women with breast cancer. World J. Clin. Oncol. 8, 241–248. doi: 10.5306/wjco.v8.i3.241
Teresi, R. E., and Waite, K. A. (2008). PPARgamma, PTEN, and the fight against cancer. PPAR Res. 2008:932632. doi: 10.1155/2008/932632
Thirion, P., Piedbois, P., Buyse, M., O'Dwyer, P. J., Cunningham, D., Man, A., et al. (2001). Alpha-interferon does not increase the efficacy of 5-fluorouracil in advanced colorectal cancer. Br. J. Cancer 84, 611–620. doi: 10.1054/bjoc.2000.1669
Thomas, S., Schelker, R., Klobuch, S., Zaiss, S., Troppmann, M., Rehli, M., et al. (2015). Biomodulatory therapy induces complete molecular remission in chemorefractory acute myeloid leukemia. Haematologica 100, e4–6. doi: 10.3324/haematol.2014.115055
Thornton, P. D., Hamblin, M., Treleaven, J. G., Matutes, E., Lakhani, A. K., and Catovsky, D. (1999). High dose methyl prednisolone in refractory chronic lymphocytic leukaemia. Leuk. Lymphoma 34, 167–170. doi: 10.3109/10428199909083393
Tögel, L., Nightingale, R., Chueh, A. C., Jayachandran, A., Tran, H., Phesse, T., et al. (2016). Dual targeting of bromodomain and extraterminal domain proteins, and WNT or MAPK signaling, inhibits c-MYC expression and proliferation of colorectal cancer cells. Mol. Cancer Ther. 15, 1217–1226. doi: 10.1158/1535-7163.MCT-15-0724
Tontonoz, P., Singer, S., Forman, B. M., Sarraf, P., Fletcher, J. A., Fletcher, C. D., et al. (1997). Terminal differentiation of human liposarcoma cells induced by ligands for peroxisome proliferator-activated receptor gamma and the retinoid X receptor. Proc. Natl. Acad. Sci. U.S.A. 94, 237–241. doi: 10.1073/pnas.94.1.237
Trosko, J. E. (2006). From adult stem cells to cancer stem cells: Oct-4 Gene, cell-cell communication, and hormones during tumor promotion. Ann. N. Y. Acad. Sci. 1089, 36–58. doi: 10.1196/annals.1386.018
Tsai, D. E., Luger, S. M., Andreadis, C., Vogl, Dan, T., Kemner, A., Potuzak, M., et al. (2008). A phase I study of bexarotene, a retinoic X receptor agonist, in non-M acute myeloid leukemia. Clin. Cancer Res. 14, 5619–5625. doi: 10.1158/1078-0432.CCR-07-5185
Ugocsai, P., Wolff, D., Menhart, K., Hellwig, D., Holler, E., Herr, W., et al. (2016). Biomodulatory metronomic therapy induces PET-negative remission in chemo- and brentuximab-refractory Hodgkin lymphoma. Br. J. Haematol. 172, 290–293. doi: 10.1111/bjh.13480
Vallée, A., and Lecarpentier, Y. (2018). Crosstalk between peroxisome proliferator-activated receptor gamma and the canonical WNT/β-catenin pathway in chronic inflammation and oxidative stress during carcinogenesis. Front. Immunol. 9:745. doi: 10.3389/fimmu.2018.00745
Vallée, A., Lecarpentier, Y., Guillevin, R., and Vallée, J.-N. (2018). Opposite interplay between the canonical WNT/β-catenin pathway and PPAR gamma: a potential therapeutic target in gliomas. Neurosci. Bull. 34, 573–588. doi: 10.1007/s12264-018-0219-5
van Beurden-Tan, C. H. Y., Franken, M. G., Blommestein, H. M., Uyl-de, G., Carin, A., and Sonneveld, P. (2017). Systematic literature review and network meta-analysis of treatment outcomes in relapsed and/or refractory multiple myeloma. J. Clin. Oncol. 35, 1312–1319. doi: 10.1200/JCO.2016.71.1663
van Nuffel, A. M., Sukhatme, V., Pantziarka, P., Meheus, L., Sukhatme, V. P., and Bouche, G. (2015). Repurposing Drugs in Oncology (ReDO)-clarithromycin as an anti-cancer agent. Ecancermedicalscience 9:513. doi: 10.3332/ecancer.2015.513
Viardot, A., Goebeler, M.-E., Hess, G., Neumann, S., Pfreundschuh, M., Adrian, N., et al. (2016). Phase 2 study of the bispecific T-cell engager (BiTE) antibody blinatumomab in relapsed/refractory diffuse large B-cell lymphoma. Blood 127, 1410–1416. doi: 10.1182/blood-2015-06-651380
Virchow, R. (1859). Die Cellularpathologie in Ihrer Begründung auf Physiologische und Pathologische Gewebelehre. Berlin: Verlag von August Hirschwald.
Vogelhuber, M., Feyerabend, S., Stenzl, A., Suedhoff, T., Schulze, M., Huebner, J., et al. (2015). Biomodulatory treatment of patients with castration-resistant prostate cancer: a phase ii study of imatinib with pioglitazone, etoricoxib, dexamethasone and low-dose treosulfan. Cancer Microenviron. 8, 33–41. doi: 10.1007/s12307-014-0161-7
Vogt, T., Coras, B., Hafner, C., Landthaler, M., and Reichle, A. (2006). Antiangiogenic therapy in metastatic prostate carcinoma complicated by cutaneous lupus erythematodes. Lancet Oncol. 7, 695–697. doi: 10.1016/S1470-2045(06)70798-7
Vogt, T., Hafner, C., Bross, K., Bataille, F., Jauch, K.-W., Berand, A., et al. (2003). Antiangiogenetic therapy with pioglitazone, rofecoxib, and metronomic trofosfamide in patients with advanced malignant vascular tumors. Cancer 98, 2251–2256. doi: 10.1002/cncr.11775
Walter, B., Rogenhofer, S., Vogelhuber, M., Berand, A., Wieland, W. F., Andreesen, R., et al. (2010). Modular therapy approach in metastatic castration-refractory prostate cancer. World J. Urol. 28, 745–750. doi: 10.1007/s00345-010-0567-x
Walter, B., Schrettenbrunner, I., Vogelhuber, M., Grassinger, J., Bross, K., Wilke, J., et al. (2012). Pioglitazone, etoricoxib, interferon-α, and metronomic capecitabine for metastatic renal cell carcinoma: final results of a prospective phase II trial. Med. Oncol. 29, 799–805. doi: 10.1007/s12032-011-9982-0
Walter, I., Schulz, U., Vogelhuber, M., Wiedmann, K., Endlicher, E., Klebl, F., et al. (2017). Communicative reprogramming non-curative hepatocellular carcinoma with low-dose metronomic chemotherapy, COX-2 inhibitor and PPAR-gamma agonist: a phase II trial. Med. Oncol. 34:192. doi: 10.1007/s12032-017-1040-0
Wang, Y. M., Jin, B. Z., Ai, F., Duan, C. H., Lu, Y. Z., Dong, T. F., Fu, Q. L. (2012). The efficacy and safety of melatonin in concurrent chemotherapy or radiotherapy for solid tumors: a meta-analysis of randomized controlled trials. Cancer Chemother. Pharmacol. 69, 1213–1220. doi: 10.1007/s00280-012-1828-8
Was, H., Czarnecka, J., Kominek, A., Barszcz, K., Bernas, T., Piwocka, K., et al. (2018). Some chemotherapeutics-treated colon cancer cells display a specific phenotype being a combination of stem-like and senescent cell features. Cancer Biol. Ther. 19, 63–75. doi: 10.1080/15384047.2017.1385675
Weber, D. M., Chen, C., Niesvizky, R., Wang, M., Belch, A., Stadtmauer, E. A., et al. (2007). Lenalidomide plus dexamethasone for relapsed multiple myeloma in North America. New Engl. J. Med. 357, 2133–2142. doi: 10.1056/NEJMoa070596
Whyte, W. A., Orlando, D. A., Hnisz, D., Abraham, B. J., Lin, C. Y., Kagey, M. H., et al. (2013). Master transcription factors and mediator establish super-enhancers at key cell identity genes. Cell 153, 307–319. doi: 10.1016/j.cell.2013.03.035
Winter, G. E., Buckley, D. L., Paulk, J., Roberts, J. M., Souza, A., Dhe-Paganon, S., et al. (2015). DRUG DEVELOPMENT. Phthalimide conjugation as a strategy for in vivo target protein degradation. Science 348, 1376–1381. doi: 10.1126/science.aab1433
Yun, S.-H., Han, S.-H., and Park, J.-I. (2018). Peroxisome proliferator-activated receptor γ and PGC-1α in cancer: dual actions as tumor promoter and suppressor. PPAR Res. 2018:6727421. doi: 10.1155/2018/6727421
Zapletalova, D., André, N., Deak, L., Kyr, M., Bajciova, V., Mudry, P., et al. (2012). Metronomic chemotherapy with the COMBAT regimen in advanced pediatric malignancies: a multicenter experience. Oncology 82, 249–260. doi: 10.1159/000336483
Keywords: Anakoinosis, communicative reprogramming, transcriptional modulators, metronomic low-dose chemotherapy, glitazones, all-trans retinoic acid, COX-2 inhibitor, master modulators
Citation: Heudobler D, Rechenmacher M, Lüke F, Vogelhuber M, Klobuch S, Thomas S, Pukrop T, Hackl C, Herr W, Ghibelli L, Gerner C and Reichle A (2018) Clinical Efficacy of a Novel Therapeutic Principle, Anakoinosis. Front. Pharmacol. 9:1357. doi: 10.3389/fphar.2018.01357
Received: 19 June 2018; Accepted: 05 November 2018;
Published: 28 November 2018.
Edited by:
Salvatore Salomone, Università degli Studi di Catania, ItalyReviewed by:
Eumorphia Remboutsika, National and Kapodistrian University of Athens Medical School, GreeceAlfonso Pompella, Università degli Studi di Pisa, Italy
Copyright © 2018 Heudobler, Rechenmacher, Lüke, Vogelhuber, Klobuch, Thomas, Pukrop, Hackl, Herr, Ghibelli, Gerner and Reichle. This is an open-access article distributed under the terms of the Creative Commons Attribution License (CC BY). The use, distribution or reproduction in other forums is permitted, provided the original author(s) and the copyright owner(s) are credited and that the original publication in this journal is cited, in accordance with accepted academic practice. No use, distribution or reproduction is permitted which does not comply with these terms.
*Correspondence: Albrecht Reichle, YWxicmVjaHQucmVpY2hsZUB1a3IuZGU=