- 1College of Life Science, Yangtze University, Jingzhou, China
- 2Department of Chemistry, Faculty of Science, University of Hradec Kralove, Hradec Kralove, Czechia
- 3Toxicology and Civil Protection, Faculty of Health and Social Studies, Institute of Radiology, University of South Bohemia České Budějovice, České Budějovice, Czechia
- 4Biomedical Research Centre, University Hospital, Hradec Kralove, Czechia
Beauvericin (BEA) is an emerging Fusarium mycotoxin that contaminates food and feeds globally. BEA biosynthesis is rapidly catalyzed by BEA synthetase through a nonribosomal, thiol-templated mechanism. This mycotoxin has cytotoxicity and is capable of increasing oxidative stress to induce cell apoptosis. Recently, large evidence further shows that this mycotoxin has a variety of biological activities and is being considered a potential candidate for medicinal and pesticide research. It is noteworthy that BEA is a potential anticancer agent since it can increase the intracellular Ca2+ levels and induce the cancer cell death through oxidative stress and apoptosis. BEA has exhibited effective antibacterial activities against both pathogenic Gram-positive and Gram-negative bacteria. Importantly, BEA exhibits an effective capacity to inhibit the human immunodeficiency virus type-1 integrase. Moreover, BEA can simultaneously target drug resistance and morphogenesis which provides a promising strategy to combat life-threatening fungal infections. Thus, in this review, the synthesis and the biological activities of BEA, as well as, the underlying mechanisms, are fully analyzed. The risk assessment of BEA in food and feed are also discussed. We hope this review will help to further understand the biological activities of BEA and cast some new light on drug discovery.
Introduction
Beauvericin (BEA) (Figure 1) is a cyclic hexadepsipeptide that is synthesized by various toxigenic fungi, including several Fusarium species (Wang and Xu, 2012; Tao et al., 2015; Patocka, 2016). BEA can be produced by different Fusarium species in different regions. For example, in the USA and South Africa, F. circinatum is the main BEA producing fungi, whereas, in Europe, F. sambucinum and F. subglutinans are the major ones (more details in Mallebrera et al., 2018). As a mycotoxin, BEA is an important natural contaminant in many bowls of cereal and cereal based products (Shin et al., 2009; Juan et al., 2013a,b). The contamination of BEA is a serious problem in Southern Europe (Santini et al., 2012). Notably, BEA is toxic to human tissues and cells and shows cytotoxicity at a concentration lower than that for aflatoxin B1 (Svingen et al., 2017). BEA induces the generation of reactive oxygen species (ROS) and leads to an increase of oxidative stress, which causes cell apoptosis (Ferrer et al., 2009). The channel forming ability of BEA selectively directs a flux of cations, particularly Ca2+, into the cells. The resulting increased intracellular Ca2+ levels might be, at least in part, responsible for their cytotoxicity (Lemmens-Gruber et al., 2000). BEA activates apoptosis via the internal mitochondrial pathway and influences several cellular signaling pathways and regulators including MAPK, NF-κB, and p53 (Feudjio et al., 2010; Qadri et al., 2011).
Interestingly, this mycotoxin possesses a wide variety of biological properties. It shows promising antibacterial and antifungal activities and potentiates other antifungal agents therapies (Zhang et al., 2007). Importantly, BEA can inhibit cancer cell proliferation and induce cell apoptosis via activating a Ca2+-mediated mechanism (Jow et al., 2004). Moreover, at sub-cytotoxic concentrations, this mycotoxin can also inhibit directional cancer cell motility (haptotaxis) (Zhan et al., 2007). Haptotaxis is important for the development of new blood vessels in tumors (angiogenesis) (Mizukami et al., 2018). The molecular mechanism(s) of the biological action of BEA is related to its ionophoric activity. BEA rapidly increases ion permeability (especially Ca2+) in biological membranes (from extracellular to intracellular) and acts as a cytotoxin. Up to date, BEA has been reported to cause significant cytotoxicity in a variety of cancer cell lines and oxidative stress seems to be one potential mechanism in the induction of cancer cell death at the molecular level (Tonshin et al., 2010). BEA is also genotoxic and induces apoptosis due to the activation of mitochondrial-death pathway (Celik et al., 2010). Recent studies further show that BEA is stable enough to cross the blood-brain barrier, indicating neurotoxicity (Taevernier et al., 2016a).
The in vitro cytotoxicity implies that BEA can be potentially used for cancer therapeutics. This compound inhibits drug efflux pumps, is non-mutagenic and inhibits bone resorption, which suggests it as a potential drug candidate to fight disseminated cancer (Feudjio et al., 2010). Thus, as reported, BEA has many biological activities including antibacterial, antifungal, anticancer, anti-inflammatory, insecticidal, nematicidal, platelet activation, and anti-cholesterol activities (Figure 2). All these characteristics are quite crucial for the development of medicine and pesticides. However, up to date, these reports are scattered, and there is rarely a recent review article available discussing the various biological activities of BEA as well as their potential mechanisms. Thus, in this review, we have updated and discussed the biosynthesis and the major biological activities of BEA.
Chemistry
BEA (CAS 26048-05-5. M.W. 783.957) is a cyclic hexadepsipeptide that contains three D-hydroxy-isovaleryl and three N-methyl-phenylalanyl residues in an alternating sequence (Hamill et al., 1969) (Figure 1). BEA belongs to the enniatins (ENNs) antibiotic family and it is structurally similar to the ENNs, which are also produced by Fusarium species. BEA differs from ENNs in the nature of the N-methylamino acid. Their bioactivities are quite different due to the difference between BEA and ENNs (Shin et al., 2009; Yoo et al., 2017) (Figure 1). The absence of any chargeable groups in cyclic hexapeptide explains the poor water solubility and the low chemical reactivity of BEA, which also is characterized by a three-fold axis of symmetry (Logrieco et al., 2002).
Synthesis
BEA is produced by many fungi including Beaveria bassiana (Hamill et al., 1969; Peczynska-Czoch et al., 1991) and Fusarium spp. (Logrieco et al., 1998). The necessary components in EBA biosynthesis are amino acid L-phenylalanine (L-Phe), the hydroxy acid D-hydroxyisovaleric acid (D-HYIV), ATP/Mg2+, and S-adenosyl-methionine (AdoMet), which is the source of the methyl group for the L-phenylalanyl residues (Wang and Xu, 2012; Zobel et al., 2016). Basically, BEA biosynthesis is rapidly catalyzed by BEA synthetase (BEAS) through a nonribosomal, thiol-templated mechanism (Kopp and Marahiel, 2007; Xu et al., 2008; Steiniger et al., 2017). BEAS consists of a single polypeptide chain (molecular mass 250 kDa) and a calmodulin binding motif (Peeters et al., 1988). B. bassiana BEAS (BbBEAS) can interact with Ca2+ sensor calmodulin (CaM) in a Ca2+-dependent manner (Kim and Sung, 2018). In vitro, CaM-binding assay showed that the His-tagged BbBEAS binds to CaM in a Ca2+-dependent manner. CaM binding to BbBEAS also induces the conformational change of interacted proteins, which will further affect its enzyme activity. Therefore, BbBEAS is a novel CaM-binding protein in B. bassiana. The schematic representation of the BEA biosynthesis is shown in Figure 3.
Risk Assessment of Beauvericin in Food and Feed
There is a high occurrence of BEA in grains and wheat-based products like pasta, infant formulas, breakfast cereals, and biscuits, with incidences between 40 and 90% (Santini et al., 2012; Stanciu et al., 2017). In recent years, BEA has frequently been reported in different countries (South Africa, Norway, China, Croatia, Poland, Spain, Morocco…) as contaminants of, especially wheat, rye, oats, barley, and rice (Decleer et al., 2018). Seed-borne infection of wheat with F. proliferatum leads to contamination of wheat kernels with BEA (15–55 μg/kg) (Guo et al., 2016). In the study of Quiles et al. (2016), around 3% of refrigerated pizza dough were contaminated by BEA with level 22.39 μg/kg. BEA (1.3 ng/L) is also detected in Swiss wastewater treatment plant (Schenzel et al., 2012). Notably, BEA is a common contaminant in Danish cereals and show high hepatoxicity on a high-content imaging platform (Svingen et al., 2017). Because this fungi often contaminate food, feedstock, and animal feed, BEA is a risk factor for environmental health. Although the cereal-based food and feed contamination of Fusaria is huge, and although BEA is regularly found in these products (Hietaniemi et al., 2016; Svingen et al., 2017; Beccari et al., 2018; Carballo et al., 2018), the EFSA Panel on Contaminants in the Food Chain (CONTAM Panel, 2014) concluded that acute exposure to BEA and ENNs do not indicate concern for human health.
Basically, BEA has less occurrence in grains from cooler climates while a higher contamination level of BEA is usually reported in Southern Europe and Morocco (max 59 mg/kg in maize) (Santini et al., 2012). The BEA levels in rice from the local market of Morocco are varied between 3.8 and 26.3 mg/kg (Sifou et al., 2011; Fraeyman et al., 2017). The finding of 10.6 mg/kg of BEA in a rice-based infant cereal sample from Morocco should be highlighted (Mahnine et al., 2011). Mycotoxins contamination in wheat-based products in Romania for direct human consumption were evaluated and 32% of them presented BEA (Stanciu et al., 2017). In corn grits from the Japanese market, BEA was found in 34% of the samples. The maximum concentration of BEA was 26.1 μg/kg (Yoshinari et al., 2016). In China, BEA was detected in 96.9% of the commercial pet food with levels ranging 0.2–153.4 μg/kg (Shao et al., 2018). In Chinese medicinal herbs, BEA is the frequently detectable toxin with a 20% incidence (Hu and Rychlik, 2014). The contamination of BEA in Portuguese cereal-based foods was evaluated by Blesa et al. (2012). The percentage of BEA was 1.6%. For the total samples, the mean contamination of BEA was 0.1 mg/kg BEA. The wheat-based samples showed higher levels and greater prevalence than any other cereals monitored. The occurrence of BEA in analyzed pasta and multi cereal baby food samples from the Campania region (Italy) were below 68% (Juan et al., 2013a). A high incidence (70.3%) and high contamination levels (< 1100 μg/kg) of BEA in multi cereal baby food and its intakes could represent a risk for the infant population.
BEA (6.2–844 μg/kg) is one of the most predominant mycotoxins in food and diet (wheat and maize-based products) from Mediterranean area (Serrano et al., 2012). In the main markets of Abidjan, Bouake, and Korhogo, France, 91% of the rice, maize and peanut samples were contaminated with more than one mycotoxin including BEA (79% of the samples) (Manizan et al., 2018). The peanut paste samples represented the highest risk to consumer health followed by maize and rice samples. BEA (2 μg/kg) were detected in wheat ears randomly collected during year 2014 and 2015 from various localities in the Czech Republic (Sumikova et al., 2017). Besides these countries, BEA was also monitored in different Italian organic cereals and cereal products. Around 80% of analyzed samples contained with BEA (6.7–41 μg/kg) (Juan et al., 2013b).
The levels of BEA were analyzed in feed ingredients and compound feeds that were distributed throughout Korea during 2006 and 2007 (Lee et al., 2010). Twenty seven percentage of feed ingredients were contaminated with BEA at levels of 0.01–1.80 μg/g. The mean concentration of BEA was highest in bran feeds (0.76 μg/g). In compound feeds, 33% of samples were contaminated with BEA at levels of 0.01–4.66 μg/g. The mean BEA concentration was higher in swine (0.74 μg/g) and dairy cattle (0.72 μg/g) feeds than in beef cattle (0.43 μg/g) and chicken (0.37 μg/g) feeds. BEA is also detected in Irish farm silages (21.8 μg/kg) (McElhinney et al., 2015) and corn-dried distiller's grains in Thailand (350 μg/kg) (Tansakul et al., 2013).
Currently, the occurrence data for BEA is still limited and only available from certain parts of the world. Quantitative skin permeability data showed that BEA penetrates through the human skin and cross blood brain barrier to exert toxic effects on human (Taevernier et al., 2016b). However, as an emerging toxin, the toxicokinetic data and the risk assessment of BEA on humans are rarely reported (Bertero et al., 2018). Therefore, it is still difficult to conduct a full risk assessment for BEA. The BEA occurrence in grains and grain-based foodstuffs in recent years are summarized in Table 1.
Bioactivity
BEA shows cytotoxic, apoptotic, anticancer, anti-inflammatory, antimicrobial, insecticidal, and nematicidal activities. BEA is also an ionophoric cyclodepsipeptide which forms complexes with cations and increases the permeability of biological membranes (Massini and Näf, 1980; Toman et al., 2011; Wätjen et al., 2014; Lu et al., 2016). Because BEA has very efficient effects in the anticancer, antimicrobial, and insecticidal activities, this mycotoxin is considered to have the potential to be developed as a medicine or a pesticide. The various kinds of bioactivities of BEA are due to some unique active mechanisms, including the ions transport, oxidative stress, and autophagy (Wang and Xu, 2012).
Cytotoxic Activity
The cytotoxic effects of BEA have been studied by several authors. BEA (10 μM) can induce significant toxicity in TM-Luc 102 and Caco-2 cells (Fernández-Blanco et al., 2016). BEA shows toxicity in oocytes and cumulus cells at concentrations exceeding 0.5 μM (Schoevers et al., 2016). BEA significantly inhibits bovine granulosa cell proliferation at 3, 6, and 10 μM (Albonico et al., 2017). At 30 μM, BEA shows inhibitory effects on IGF1-induced CYP1 and CYP1 mRNA abundance (Albonico et al., 2017).
The mechanism of the BEA cytotoxicity is not fully understood. Researchers showed that BEA reduces cell viability correlating with the ROS generation and malondialdehyde formation (Ferrer et al., 2009). Similarly, in the study of Jow et al. (2004), BEA induces human leukemia cell death and this process has underwent an apoptotic pathway. In their study, CCRF-CEM cells were treated with BEA (1–10 μM) for 24 h, BEA-induced cell death exhibited a dose and time-dependent manner. This incidence of nuclear fragmentation and apoptotic body formation were significantly increased. Cytosolic caspase-3 activity and the release of Cyt c from mitochondria were also observed. The cellular toxicity targets of BEA are the mitochondrion and the homeostasis of potassium ions (Tonshin et al., 2010). In isolated rat liver mitochondria, exposure to BEA depleted the mitochondrial transmembrane potential, uncoupled oxidative phosphorylation, induced mitochondrial swelling and decreased Ca2+ retention capacity of the mitochondria (Tonshin et al., 2010). BEA can alter the mitochondrial membrane potential and produces DNA strand breakage (Mallebrera et al., 2016). Moreover, Mallebrera et al. (2016) showed that, BEA exposure for 24 h arrested the G0/G1 phase of CHO-K1 cell cycle and produces apoptosis. It seems that BEA-induced apoptosis is controlled by a balanced expression between apoptotic (Bax, Bad) and antipoptotic (Bcl-2) proteins (Mallebrera et al., 2018).
Importantly, a pre-treatment of the Ca2+ chelator can significantly increase the survival rate of the cells. Thus, intracellular Ca2+ plays an important function, perhaps as a mediator in the induced cell death signaling (Jow et al., 2004). A very recent study (Manyes et al., 2018) further showed that the cytotoxicity of BEA involves mitochondrial alterations, apoptosis, and cell cycle disturbances, since they observed a much higher percentage of apoptotic rate. Caspase-3 and 7 were highly activated and BEA arrested the S phase of the cell proliferation. Moreover, we further suspect that the BEA-induced cytoxicity has a relationship with autophagy, although the study of autophagy in BEA has rarely been reported. Since autophagy has a complex function in cell proliferation (Wu et al., 2018), therefore, it is quite interesting if some studies of autophagy in the cytotoxicity of BEA are conducted in the future.
On the other hand, BEA can increase ion permeability in biological membranes by forming a complex with essential cations (Ca2+, Na+, K+), which may affect the ionic homeostasis (Jow et al., 2004). Zhan et al. (2007) further showed that BEA is capable of inhibiting the metastatic prostate cancer and breast cancer cells and has antiangiogenic activity in HUVEC-2 cells.
The results of the studies suggest that BEA could be neurotoxic, but almost nothing is known about the mechanism of its neurotoxic effect (Zuzek et al., 2016). In the paper of Zuzek et al. (2016), BEA was shown to disrupt neurotransmission at the motor endplate. BEA reduced the release of acetylcholine from the presynaptic terminal. BEA formed cation-selective channels thus could depolarize and inactivate voltage-dependent sodium Nav1.4 type channels (Kouri et al., 2003).
Anticancer Activity
Currently, the anticancer potential of BEA is a hot topic (Zhan et al., 2007; Lu et al., 2016). BEA inhibits migration of the metastatic prostate cancer ((PC-3M)), breast cancer (MDA-MB-231) cells and exhibits antiangiogenic activity in HUVEC-2 cells (Zhan et al., 2007). BEA was also reported to induce the apoptosis of human non-small cell lung cancer (NSCLC) A549 cells (Lin et al., 2005), KB, and KBv200 cells (Tao et al., 2015). The anticancer activities of BEA are mainly studied by the working group of Jow [see Jow et al., 2004; Lin et al., 2005; Tang et al., 2005; Chen et al., 2006)]. BEA induced apoptosis through mitochondrial pathways, including decrease of ROS generation, loss of mitochondrial membrane potential, release of cytochrome c, and activation of Caspase-9 and−3 (Tao et al., 2015).
As discussed, the mechanism of BEA-induced cancer cell apoptosis involves multiple cellular/molecular pathways and pro- and anti-apoptotic Bcl-2 family proteins (Lu et al., 2016). Moreover, the BEA-induced cell death is mainly due to the increase of intracellular Ca2+ concentration (Lu et al., 2016). However, it should be further to identify whether the increase of intracellular Ca2+ (from extracellular or intracellular stores) is an important factor with the apoptotic pathway to conduct EA-induced cancer cell death. In a subsequent study (Chen et al., 2006), the effect of BEA on Ca2+ concentration [[Ca2+]i] and the underlying mechanisms responsible for the changes of [Ca2+]i in CCRF-CEM cells were further investigated. Indeed, BEA caused a rapid and sustained [Ca2+]i rise. Excess extracellular Ca2+ facilitated a BEA-induced [Ca2+]i rise in the bathing medium. It is noteworthy that the voltage-dependent Ca2+ channel blocker or intracellular Ca2+ depletion does not affect the BEA-induced [Ca2+]i rise. Thus, BEA should act as a potent Ca2+ mobilizer by stimulating an extracellular Ca2+ influx and inducing the cancer cell apoptosis (Figure 4). Similarly, BEA can activate Ca2+-activated Cl− currents and induces cell death in Xenopus oocytes via the influx of extracellular Ca2+ (Tang et al., 2005).
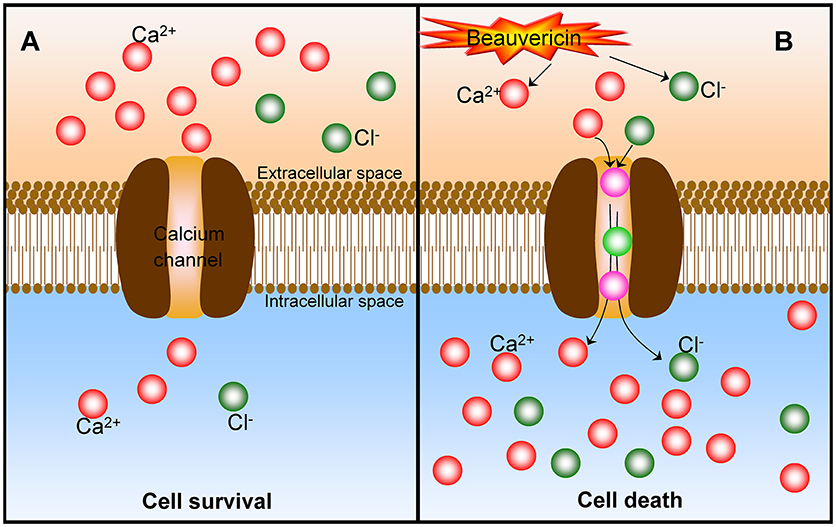
Figure 4. The mechanism of Ca2+ in the beauvericin-induced cancer cell death. (A) At normal status, there is a much higher extracellular Ca2+ content, and cancer cells keep surviving. (B) After the exposure of beauvericin, the extracellular Ca2+ are motivated to transfer into the intracellular through the calcium channel and induce the cancer cell apoptosis and death. Beauvericin can activate Ca2+-activated Cl− currents and induce cell death as well.
As mentioned, BEA induces apoptosis in a variety of cancer cell lines, but the underlying mechanism(s) is still not fully understood. Lin et al. (2005) showed that BEA-induced human NSCLC A549 cell apoptosis through the up-regulation of cytokines Bax and p-Bad and down-regulation of p-Bcl-2. Also, the reduction of mitochondrial membrane potential, the activation of caspase 3 and cytochrome c release were observed and should be involved in the mechanism of BEA-induced cancer cell death (Jow et al., 2004; Lin et al., 2005). However, in the study of Tao et al. (2015), the decrease of protein level Bcl-2 and Bax after treatment with BEA (3–12 μM) for 48 h was not observed in KB cells and KBv200 cells. This different effect on pro and anti-apoptotic Bcl-2 family proteins induced by BEA might be relevant to various characteristics of the cell lines.
Oxidative stress is one important effect involved in BEA anticancer activity (Prosperini et al., 2013). BEA can increase the ROS level at an early stage. BEA induced cancer cell death via an apoptotic process with reduced G0/G1 phase and with an arrest in G2/M. Moreover, BEA caused DNA damage after 12.0 μM concentration. Also, BEA exposure produces DNA strand breakage and induces CHO-K1 cell apoptosis (Mallebrera et al., 2016). In a very recent study (Escrivá et al., 2018), BEA was further shown to induce mitochondrial damage to affect the respiratory chain through the caspase cascade in Jurkat cells. However, on the contrary, one research group Dornetshuber et al. (2009) demonstrated that the oxidative stress and DNA interactions were not involved in BEA-mediated apoptosis. The reasons are not clear but are possibly due to the different cell lines, BEA concentrations, and functioning times used in their specific studies.
Some signaling pathways are involved in the anticancer mechanisms of BEA (Wätjen et al., 2014; Lu et al., 2016). For example, in cancer cell lines HepG2 and H4IIE, BEA reduced the ERK and NF-κB protein expression and promoted JNK phosphorylation (Wätjen et al., 2014). As known, deregulated NF-κB activity contributes to many human diseases, including tumors. Since NF-κB is a transcription factor which is responsible for cell survival, this inhibition may also contribute to the toxic effects of BEA. BEA induces an increased JNK phosphorylation, which is generally associated with cell death. Additionally, numerous protein kinase in the signaling transduction pathway showed a selective inhibition of Src kinase by BEA (Wätjen et al., 2014). MEK1/2-ERK42/44-90RSK pathway plays an important role in the mechanism of BEA-induced NSCLC A549 cancer cell apoptosis (Lu et al., 2016). Further, BEA can decrease the ERK and NF-κB phosphorylation but increase JNK phosphorylation in H4IIE cells. During a screening of 21 protein kinases, BEA shows selective inhibition of Src kinase in signal transduction pathways (IC50 = 9.8 μg/ml) (Wätjen et al., 2014). Recently, Lu et al. (2016) reported that BEA induces NSCLC A549 cancer cell apoptosis through the mitogen-activated protein kinase pathway (MAPK), BEA can also activate MEK1/2-ERK42/44-90RSK crosstalk signaling pathway which can induce A549 cell cycle arrest in the S phase and apoptosis (Lu et al., 2016). In vivo, BEA reduces the levels of TNF-α and IFN-γ in mice serum. BEA suppresses IFN-γ-STAT1-T-bet signaling and leads to T cell apoptosis (Massini and Näf, 1980). Compared with other mycotxins, for example, trichothecenes (Wu et al., 2017), the study of the signaling pathway of BEA in anicancer mechanisms is relatively limited. More studies on the signaling pathways in the BEA cytotoxicity are warranted.
It should be noted that most of the above studies are performed in vitro using cancer cell lines and the in vivo studies are urgently needed to identify the anticancer capacity of BEA. Recently, Heilos et al. (2017) have tested the in vivo anticancer effects of BEA by treating mice bearing murine CT-26 or human KB-3-1-grafted tumors, respectively. Decreased tumor volumes and weights in BEA-treated mice without any adverse effects were observed. BEA accumulation was also detected in tumor tissues. Moreover, a significant increase of necrotic areas within whole tumor sections of BEA-treated mice was observed, confirming its promising role as a novel natural compound for anticancer therapy.
Thus, BEA shows promising anticancer potential through investigation of different cancer cell lines. Normally, BEA induces extracellular Ca2+ translocated into the cytosol, which leads to an increase intracellular Ca2+ level. The increased Ca2+ may activate a series of signaling pathways, for example, MAPK, NF-κB, and decreases the mitochondrial transmembrane potential, release of Cyt c, and activates caspase, therefore further promotes the cancer cell apoptosis. However, up to date, we have rarely seen the in vivo data of anticancer of BEA. The in vivo data is very important since we need to know that whether BEA is stable and active in the body. Moreover, the toxicity of this product is an important issue for consider. Thus, the information of the in vivo anticancer activity of BEA are urgently needed. In addition, in the future, the study of the BEA-monoclonal antibodies (mAb) should be a promising strategy in anticancer therapies.
Anti-inflammatory Activity
BEA has anti-inflammatory activities and it inhibits inflammatory responses by inhibiting the NF-κB pathway. In a recent study by Yoo et al. (2017), BEA blocked the production of nitric oxide (NO) in lipopolysaccharide-treated RAW264.7 cells without inducing cell cytotoxicity. Moreover, BEA inhibited the nuclear translocation of the NF-κB subunits p65 and p50. Luciferase reporter gene assays demonstrated that BEA suppressed MyD88-dependent NF-κB activation. By analyzing upstream signaling events for NF-κB activation, overexpression of Src and Syk were observed and these two enzymes were the potential targets of BEA. Thus, BEA is a strong anti-inflammatory agent that attenuates NF-κB-dependent inflammatory responses by suppressing enzymes Src and Syk (Figure 5) (Yoo et al., 2017).
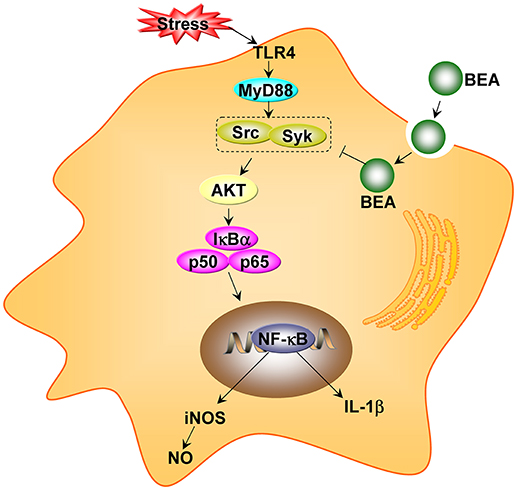
Figure 5. The proposed anti-inflammatory pathway of beauvericin (BEA) in macrophages (adapted from Yoo et al., 2017).
The current therapeutic goals of colitis are aimed at reducing the occurrence of the main symptoms and preventing further development of the disease. BEA has a therapeutic role in the colitis. Inflammatory bowel disease is usually accompanied by abnormal secretion of inflammatory cytokines. These abnormally secreted cytokines also promote inflammation and play an important role in the occurrence and development of inflammatory bowel disease. BEA can inhibit the expression of L-12, IL-1β, and IFN-γ in enteritis colon tissue with enteritis (Wu et al., 2013). Because of the anti-inflammatory effect, BEA shows a promising capacity for the treatment of colonic inflammation by targeting the PI3K/Akt pathway (Wu et al., 2013). BEA decreased serum levels of TNF-α and interferon IFN-γ and suppressed T-cell proliferation. BEA further reduced the activation of IFN-γ-STAT1-T-bet signaling and led to T cell apoptosis by suppressing Bcl-2 and increasing cleavage of caspases and PARP (Wu et al., 2013). Therefore, targeting PI3K/Akt in the activated T cells by BEA maybe a novel therapy for Crohn's disease.
Antimicrobial Activity
BEA has a strong antibacterial activity against Gram-positive and Gram-negative pathogenic bacteria (Nilanonta et al., 2000, 2002; Meca et al., 2010). Also, numerous bacterial strains without distinction between Gram-positive and Gram-negative bacteria, are inhibited by BEA as well, for example, Bacillus spp., Bifidobacterium adolescentis, Clostridium perfringens, Paenibacillus spp., and Peptostreptococcus spp. (Castlebury et al., 1999; Xu et al., 2010). BEA exhibits minimum inhibitory concentration (MIC) values of 0.8–1.6 mg/mL against M. tuberculosis, and IC50 values of 1.3–2.4 mg/mL against P. falciparum (Nilanonta et al., 2002). BEA showed remarkable activity against two Gram-negative strains (B. cereus and S. typhimurium) with respective MIC values of 3.12 and 6.25 μg/ml (Dzoyema et al., 2017). As described by Xu et al. (2010), the median effective inhibitory concentration values of BEA against 6 test bacteria (B. subtilis, S. haemolyticus, P. lachrymans, A. tumefaciens, E. coli, and X. vesicatoria) were between 18.4 and 70.7 μg/mL. In another study (Zhang et al., 2016), BEA showed an inhibitory effect on three human pathogenic microbes, C. albicans, E. coli, and S. aureus. In particular, BEA exhibited the strongest antimicrobial activity against S. aureus with MIC values of 3.91 μM.
Regarding its antibacterial mechanism of BEA, the antibacterial effect of BEA is different from other antibiotics. Unlike most antibiotics, the bacterial cell wall is not the antibacterial mode of BEA activation. Cell organelles or enzyme systems are the targets of BEA (Prince et al., 1974; Wang and Xu, 2012). Based on the antibacterial activity against plant pathogens (Xu et al., 2010), BEA could be utilized in the control of non-food crop diseases and to solve the problems of drug resistance (Tong et al., 2016).
BEA also has a very effective antifungal activity. An administration of BEA with ketoconazole shows an antifungal effect with more than 100-fold higher than that by a single application (Zhang et al., 2007). The mechanism of antifungal activity of BEA has been studies by numerous studies (Mei et al., 2009; Shekhar-Guturja et al., 2016a; Tong et al., 2016). The synergetic effect is not due to their pharmacokinetic interactions (Mei et al., 2009). Tong et al. (2016) further reported that BEA can counteract multidrug resistant Candida albicans by blocking ABC transporters. As a drug efflux pump modulator, BEA reverses the multi-drug resistant phenotype of C. albicans by blocking the ATP-binding cassette transporters. BEA shows fungicidal activity by elevating intracellular Ca2+ and ROS (Tong et al., 2016). Recently, a powerful strategy to enhance antifungal efficacy against human fungal pathogens was investigated by using BEA (Shekhar-Guturja et al., 2016a,b). BEA potentiated the activity of azole antifungals against azole-resistant Candida isolates via blocking multidrug efflux and inhibition of global regulator TORC1 kinase; thereby activating protein kinase CK2 and inhibiting the molecular chaperone Hsp90. Pdr5 substitutions enable BEA efflux (Shekhar-Guturja et al., 2016a). BEA itself was effluxed via Yor1. Zcf29 bound to and regulated the expression of multidrug transporter genes (Shekhar-Guturja et al., 2016b). Beyond drug resistance, BEA blocked the C. albicans morphogenetic transition from yeast to filamentous growth in response to diverse cues. BEA repressed the expression of many filament-specific genes, including the transcription factor BRG1 (Shekhar-Guturja et al., 2016b). Thus, BEA simultaneously targets drug resistance and morphogenesis provides a promising strategy to combat life-threatening fungal infections. The antifungal activity of BEA alone is very weak but can be greatly increased in combination with ketoconazole or miconazole (Fukuda et al., 2004; Zhang et al., 2007). The structure-activity relationship (SAR) of BEA should be further studied to explore its antimicrobial activity.
Insecticidal and Nematicidal Activity
BEA shows a very promising insecticidal potential. In 1969, the working group of Hamill et al., firstly discovered the insecticidal activity of BEA against a model organism Artina salina (Hamill et al., 1969). Similarly, other authors investigated the insecticidal effect of BEA on Aedes aegypti (Grove and Pople, 1980), Calliphora erythrocephala (Daniel et al., 2017), Spodoptera frugiperda (Fornelli et al., 2004), and Lygus spp. (Leland et al., 2005). Although BEA was claimed to have insecticidal properties (Hamill et al., 1969), it is a pity that little detailed information has been published. Currently, BEA was confirmed against model organism to study insecticidal activity, Artemia salina (Hamill et al., 1969), against C. erythrocephala (Grove and Pople, 1980), A. aegypti (Wang and Xu, 2012), Lygus spp. (Leland et al., 2005), S. frugiperda (Fornelli et al., 2004), and Schizaphis graminum (Ganessi et al., 2002).
Up to date, there are very few reports about the insecticidal mechanism of BEA. Although BEA has similar chemical structures with other cyclic hexadepsipeptide mycotoxins, this mycotoxin is more effective against A. aegypti and suggesting a unique mechanism of action exists (Grove and Pople, 1980). The methanolic and ethyl acetate-methanolic extracts of B. bassiana showed larvicidal activity against 3rd instar of A. aegypti (Daniel et al., 2017). Cyclodepsipeptides are the active principles for the larvicidal action. BEA is a potential insecticidal component in the formulations for the Dengue and Zika vector.
BEA shows a promising nematicidal activity. Culture filtrates of B. bassiana were evaluated for nematicidal activity against the northern root-knot nematode (Meloidogyne hapla) (Liu et al., 2008). The nematode population densities and subsequent gall formation and egg-mass production by M. hapla were thoroughly decreased by the filtrates (Liu et al., 2008). Zhao et al. (2013) further demonstrated that the culture filtrate of different isolates of B. bassiana had different levels of activities against the same nematode, and the same culture filtrate had selective toxicity against different nematodes (Kepenekci et al., 2017). In addition, BEA shows nematicidal activities against the pine wood nematode B. xylophilus and the free-living nematode C. elegans (Shimada et al., 2010). Very recently, a new-to-nature octa-BEA showed up to very effective antiparasitic activity against Leishmania donovani and Trypanosoma cruzi (Steiniger et al., 2017). However, its antiparasitic mechanism is poorly understood and needs more investigations.
Concluding Remarks
BEA as a mycotoxin has different kinds of biological activities. It has a therapeutic potential for cancer, and viral or bacterial infections, as well as, other deadly diseases. Regarding these appealing pharmacological properties, BEA and its metabolites fulfill the requirements to be considered for further pre-clinical development as the treatment option for cancers. However, this mycotoxin is a common part of food and food ingredients as the contaminant, and there is still no clear answer of the question on whether long-term consumption of the low-dose of mycotoxin BEA can cause harm to humans and animal health. Detailed studies on the consequences of chronic and bolus BEA exposure are eagerly needed. Furthermore, occurrence data for BEA is still quite limited and only available from certain parts of the world. Therefore, currently, it is not possible to carry out a full risk assessment for BEA. In the future, it is necessary to evaluate the effectiveness of BEA as a substance that can be used in agriculture or medicine. The investigation on the BEA-mAb in anticancer therapies should be encouraged.
Author Contributions
QW, EN, and JP wrote the manuscript. KK reviewed and edited the manuscript. All the authors critically reviewed the literature and contributed to drafting the manuscript.
Conflict of Interest Statement
The authors declare that the research was conducted in the absence of any commercial or financial relationships that could be construed as a potential conflict of interest.
Acknowledgments
This work was supported by the National Natural Science Foundation of China (grant no. 31602114), the long-term organization development plan (University Hospital, Hradec Kralove, Czech Republic) as well as the project of Long-term development plan UHK and the excellence project PrF UHK.
References
Albonico, M., Schutz, L. F., Caloni, F., Cortinovis, C., and Spicer, L. J. (2017). In vitro effects of the Fusarium mycotoxins fumonisin B1 and beauvericin on bovine granulosa cell proliferation and steroid production. Toxicon. 128, 38–45. doi: 10.1016/j.toxicon.2017.01.019
Beccari, G., Senatore, M. T., Tini, F., Sulyok, M., and Covarelli, L. (2018). Fungal community, Fusarium head blight complex and secondary metabolites associated with malting barley grains harvested in Umbria, central Italy. Int. J. Food Microbiol. 273, 33–42. doi: 10.1016/j.ijfoodmicro.2018.03.005
Bertero, A., Moretti, A., Spicer, L. J., and Caloni, F. (2018). Fusarium molds and mycotoxins: potential species-specific effects. Toxins 10:E244. doi: 10.3390/toxins10060244
Blesa, J., Marín, R., Lino, C. M., and Mañes, J. (2012). Evaluation of enniatins A, A1, B, B1 and beauvericin in Portuguese cereal-based foods. Food Addit. Contam. A 29, 1727–1735. doi: 10.1080/19440049.2012.702929
Carballo, D., Font, G., Ferrer, E., and Berrada, H. (2018). Evaluation of mycotoxin residues on ready-to-eat food by chromatographic methods coupled to mass spectrometry in tandem. Toxins 10:E243. doi: 10.3390/toxins10060243
Castlebury, L. A., Sutherland, J. B., Tanner, L. A., Henderson, A. L., and Cerniglia, C. E. (1999). Use of a bioassay to evaluate the toxicity of beauvericin to bacteria. World J. Microb. Biot. 15, 119–121. doi: 10.1023/A:1008895421989
Celik, M., Aksoy, H., and Yilmaz, S. (2010). Evaluation of beauvericin genotoxicity with the chromosomal aberrations, sister-chromatid exchanges and micronucleus assays. Ecotoxicol. Environ. Saf. 73, 1553–1557. doi: 10.1016/j.ecoenv.2010.07.036
Chen, B. F., Tsai, M. C., and Jow, G. M. (2006). Induction of calcium influx from extracellular fluid by beauvericin in human leukemia cells. Biochem. Biophys. Res. Commun. 340, 134–139. doi: 10.1016/j.bbrc.2005.11.166
CONTAM Panel. (2014). (EFSA Panel on Contaminants in the Food Chain). Scientific Opinion on the risks to human and animal health related to the presence of beauvericin and enniatins in food and feed. EFSA J, 12:3916. doi: 10.2903/j.efsa.2014.3916
Covarelli, L., Beccari, G., Prodi, A., Generotti, S., Etruschi, F., Meca, G., et al. (2015). Biosynthesis of beauvericin and enniatins in vitro by wheat Fusarium species and natural grain contamination in an area of central Italy. Food Microbiol. 46, 618–626. doi: 10.1016/j.fm.2014.09.009
Daniel, J. F. S., Silva, A. A., Nakagawa, D. H., de Medeiros, L. S., and Carvalho, M. G. (2017). Larvicidal activity of Beauveria bassiana extracts against Aedes aegypti and Identification of Beauvericins. J. Braz. Chem. Soc. 28, 1003–1013. doi: 10.21577/0103-5053.20160253
Decleer, M., Landschoot, S., De Saeger, S., Rajkovic, A., and Audenaert, K. (2018). Impact of fungicides and weather on cyclodepsipeptide-producing Fusarium spp. and beauvericin and enniatin levels in wheat grains. J. Sci. Food Agric. doi: 10.1002/jsfa.9167. [Epub ahead of print].
Dornetshuber, R., Heffeter, P., Lemmens-Gruber, R., Elbling, L., Marko, D., Micksche, M., et al. (2009). Oxidative stress and DNA interactions are not involved in Enniatin- and Beauvericin-mediated apoptosis induction. Mol. Nutr. Food Res. 53, 1112–1122. doi: 10.1002/mnfr.200800571
Dzoyema, J. P., Melong, R., Tsamoc, A. T., Maffoc, T., Kapched, D. G., Ngadjuic, B. T., et al. (2017). Cytotoxicity, antioxidant and antibacterial activity of four compounds produced by an endophytic fungus Epicoccum nigrum associated with Entada abyssinica. Revista Brasileira de Farmacognosia. 27, 251–253. doi: 10.1016/j.bjp.2016.08.011
Escrivá, L., Jennen, D., Caiment, F., and Manyes, L. (2018). Transcriptomic study of the toxic mechanism triggered by beauvericin in Jurkat cells. Toxicol. Lett. 284, 213–221. doi: 10.1016/j.toxlet.2017.11.035
Fernández-Blanco, C., Frizzell, C., Shannon, M., Ruiz, M. J., and Connolly, L. (2016). An in vitro investigation on the cytotoxic and nuclear receptor transcriptional activity of the mycotoxins fumonisin B1 and beauvericin. Toxicol. Lett. 57, 1–10. doi: 10.1016/j.toxlet.2016.05.021
Ferrer, E., Juan-García, A., Font, G., and Ruiz, M. J. (2009). Reactive oxygen species induced by beauvericin, patulin and zearalenone in CHO-K1 cells. Toxicol. In Vitro 23, 1504–1509. doi: 10.1016/j.tiv.2009.07.009
Feudjio, F. T., Dornetshuber, R., Lemmens, M., Hoffmann, O., Lemmens-Gruber, R., and Berger, W. (2010). Beauvericin and enniatin: emerging toxins and/or remedies? World Mycotoxin J. 3, 415–430. doi: 10.3920/WMJ2010.1245
Fornelli, F., Minervini, F., and Logrieco, A. (2004). Cytotoxicity of fungal metabolites to lepidopteran (Spodoptera frugiperda) cell line (SF-9). J. Invertebrate Pathol. 85, 74–79. doi: 10.1016/j.jip.2004.01.002
Fraeyman, S., Croubels, S., Devreese, M., and Antonissen, G. (2017). Emerging Fusarium and alternaria mycotoxins: occurrence, toxicity and toxicokinetics. Toxins 9:E228. doi: 10.3390/toxins9070228
Fukuda, K., Arai, M., Yamaguchi, Y., and Masuma, R. (2004). New beauvericins, potentiators of antifungal miconazole activity produced by Beauveria sp. FKI-1366. J. Antibiot. 57, 110–116. doi: 10.7164/antibiotics.57.110
Ganessi, S., Moretti, A., Bonvicini Pagliai, A. M., Logrieco, A., and Sabatini, A. (2002). Effects of beauvericin on Schizaphis graminium (Aphididae). J. Invert. Pathol. 80, 90–96. doi: 10.1016/S0022-2011(02)00125-8
Grove, J. F., and Pople, M. (1980). The insecticidal activity of beauvericin and the enniatin complex. Mycopathologia 70, 103–105. doi: 10.1007/BF00443075
Guo, Z. Q., Pfohl, K., Karlovsky, P., Dehne, H. W., and Altincicek, B. (2016). Fumonisin B-1 and beauvericin accumulation in wheat kernels after seed-borne infection with Fusarium proliferatum. Agric. Food Sci. 25, 138–145. doi: 10.23986/afsci.55539
Hamill, R. L., Higgens, C. E., Boaz, H. E., and Gorman, M. (1969). The structure op beauvericin, a new depsipeptide antibiotic toxic to Artemia salina. Tetrahedron. Lett. 10, 4255–4258. doi: 10.1016/S0040-4039(01)88668-8
Heilos, D., Rodríguez-Carrasco, Y., Englinger, B., Timelthaler, G., van Schoonhoven, S., Sulyok, M., et al. (2017). The natural fungal metabolite beauvericin exerts anticancer activity in vivo: a pre-clinical pilot study. Toxins 9:E258. doi: 10.3390/toxins9090258
Hietaniemi, V., Rämö, S., Yli-Mattila, T., Jestoi, M., Peltonen, S., Kartio, M., et al. (2016). Updated survey of Fusarium species and toxins in Finnish cereal grains. Food Addit. Contam. A 33, 831–848. doi: 10.1080/19440049.2016.1162112
Hu, L., and Rychlik, M. (2014). Occurrence of enniatins and beauvericin in 60 Chinese medicinal herbs. Food Addit. Contam. A 31, 1240–1245. doi: 10.1080/19440049.2014.913813
Jestoi, M., Rokka, M., Yli-Marrila, T., Parikka, P., Rizzo, A., and Peltonen, K. (2004). Presence and concentrations of the Fusarium-related mycotoxins beauvericin, enniatins and moniliformin in Finnish grain samples. Food Add. Contam. 21, 794–802. doi: 10.1080/02652030410001713906
Jow, G. M., Chou, C. J., Chen, B. F., and Tsai, J. H. (2004). Beauvericin induces cytotoxic effects in human acute lymphoblastic leukemia cells through cytochrome c release, caspase 3 activation: the causative role of calcium. Cancer Lett. 216, 165–173. doi: 10.1016/j.canlet.2004.06.005
Juan, C., Mañes, J., Raiola, A., and Ritieni, A. (2013a). Evaluation of beauvericin and enniatins in Italian cereal products and multicereal food by liquid chromatography coupled to triple quadrupole mass spectrometry. Food Chem. 140, 755–762. doi: 10.1016/j.foodchem.2012.08.021
Juan, C., Raiola, A., Manes, J., and Ritieni, A. (2014). Presence of mycotoxin in commercial infant formulas and baby foods from Italian market. Food Cont. 39, 227–236. doi: 10.1016/j.foodcont.2013.10.036
Juan, C., Ritieni, A., and Mañes, J. (2013b). Occurrence of Fusarium mycotoxins in Italian cereal and cereal products from organic farming. Food Chem. 141, 1747–1755. doi: 10.1016/j.foodchem.2013.04.061
Jurjevic, Z., Solfrizzo, M., Cvjetkovic, B., De Girolamo, A., and Visconti, A. (2002). Occurrence of beauvericin in corn from Croatia. Food Technol. Biotechnol. 40, 91–94.
Kepenekci, I., Saglam, H. D., Oksal, E., Yanar, D., and Yanar, Y. (2017). Nematicidal activity of Beauveria bassiana (Bals.-Criv.) Vuill. against root-knot nematodes on tomato grown under natural conditions. Egyptian J. Biol. Pest Control 27:117.
Kim, J., and Sung, G. H. (2018). Beauvericin synthetase contains a calmodulin binding motif in the entomopathogenic fungus Beauveria bassiana. J. Gen. Appl. Microbiol. 64, 145–147. doi: 10.2323/jgam.2017.09.003
Kopp, F., and Marahiel, M. A. (2007). Macrocyclization strategies in polyketide and nonribosomal peptide biosynthesis. Nat. Prod. Rep. 24, 735–749. doi: 10.1039/b613652b
Kostecki, M., Szczesna, J., Chelkowski, J., and Wisniewska, H. (1995). Beauvericin and moniliformin production by Polish isolates of Fusarium subglutinans and natural co-occurrence of both mycotoxins in maize samples. Microbiol. Aliments Nutr. 13, 67–70.
Kouri, K., Lemmens, M., and Lemmens-Gruber, R. (2003). Beauvericin-induced channels in ventricular myocytes and liposomes. Biochim. Biophys. Acta 1609, 203–210. doi: 10.1016/S0005-2736(02)00689-2
Lee, K. E., Kim, B. H., and Lee, C. (2010). Occurrence of Fusarium mycotoxin beauvericin in animal feeds in Korea. Anim. Feed Sci. Tech. 157, 190–194. doi: 10.1016/j.anifeedsci.2010.03.003
Leland, J. E., McGuire, M. R., Grace, J. A., Jaronski, S. T., Ulloa, M., Park, Y. H., et al. (2005). Strain selection of a fungal entomopathogen, Beauveria bassiana, for control of plant bugs (Lygus spp.)(Heteroptera: Miridae). Biol. Control 35, 104–114. doi: 10.1016/j.biocontrol.2005.06.005
Lemmens-Gruber, R., Rachoy, B., Steininger, E., Kouri, K., Saleh, P., Krska, P., et al. (2000). The effect of the Fusarium metabolite beauvericin on electromechanical and -physiological properties in isolated smooth and heart muscle preparations of guinea pigs. Mycopathologia 149, 5–12. doi: 10.1023/A:1007293812007
Lin, H. I., Lee, Y. J., Chen, B. F., Tsai, M. C., Lu, J. L., Chou, C. J., et al. (2005). Involvement of Bcl-2 family, cytochrom c and caspase 3 in induction of apoptosis by beauvericin in human non-small cell lung cancer cells. Cancer Lett. 230, 248–259. doi: 10.1016/j.canlet.2004.12.044
Lindblad, M., Gidlund, A., Sulyok, M., Borjesson, T., Krska, R., Olsen, M., et al. (2013). Deoxynivalenol and other selected Fusarium toxins in Swedish wheat—occurrence and correlation to specific Fusarium species. Int. J. Food Microbiol. 167, 284–291. doi: 10.1016/j.ijfoodmicro.2013.07.002
Liu, T., Wang, L., Duan, Y. X., and Wang, X. (2008). Nematicidal activity of culture filtrate of Beauveria bassiana against Meloidogyne hapla. World J. Microbiol. Biotechnol. 24, 113–118. doi: 10.1007/s11274-007-9446-z
Logrieco, A., Moretti, A., Castella, G., Kostecki, M., Golinski, P., Ritieni, A., et al. (1998). Beauvericin production by Fusarium species. Appl. Environ. Microbiol. 64, 3084–3088.
Logrieco, A., Moretti, A., Ritieni, A., Caiaffa, M. F., and Macchia, L. (2002). “Beauvericin: chemistry, biology and significance,” in Advances in Microbial Toxin Research and Its Biotechnological Exploitation, eds Upadhyay and K. Rajeev (Boston, MA: Springer Verlag), 23–30. doi: 10.1007/978-1-4757-4439-2_2
Lu, C. L., Lin, H. I., Chen, B. F., and Jow, G. M. (2016). Beauvericin-induced cell apoptosis through the mitogen-activated protein kinase pathway in human nonsmall cell lung cancer A549 cells. J. Toxicol. Sci. 41, 429–437. doi: 10.2131/jts.41.429
Mahnine, N., Meca, G., Elabidi, A., Fekhaoui, M., Saoiabi, A., Font, G., et al. (2011). Further data on the levels of emerging Fusarium mycotoxins enniatins (A, A1, B, B1), beauvericin and fusaproliferin in breakfast and infant cereals from Morocco. Food Chem. 124, 481–485. doi: 10.1016/j.foodchem.2010.06.058
Mallebrera, B., Juan-Garcia, A., Font, G., and Ruiz, M. J. (2016). Mechanisms of beauvericin toxicity and antioxidant cellular defense. Toxicol. Lett. 246, 28–34. doi: 10.1016/j.toxlet.2016.01.013
Mallebrera, B., Prosperini, A., Font, G., and Ruiz, M. J. (2018). In vitro mechanisms of Beauvericin toxicity: a review. Food Chem. Toxicol. 111, 537–545. doi: 10.1016/j.fct.2017.11.019
Manizan, A. L., Oplatowska-Stachowiak, M., Piro-Metayer, I., Campbell, K., Koffi-Nevry, R., Elliott, C., et al. (2018). Multi-mycotoxin determination in rice, maize and peanut products most consumed in Cote d'Ivoire by UHPLC-MS/MS. Food Control 87, 22–30. doi: 10.1016/j.foodcont.2017.11.032
Manyes, L., Escrivá, L., Ruiz, M. J., and Juan-García, A. (2018). Beauvericin and enniatin B effects on a human lymphoblastoid Jurkat T-cell model. Food Chem. Toxicol. 115, 127–135. doi: 10.1016/j.fct.2018.03.008
Massini, P., and Näf, U. (1980). Ca2+ ionophores and the activation of human blood platelets. The effects of ionomycin, beauvericin, lysocellin, virginiamycin S, lasalocid-derivatives and McN 4308. Biochim. Biophys. Acta 598, 575–587. doi: 10.1016/0005-2736(80)90037-1
McElhinney, C., Danaher, M., Elliott, C. T., and O'Kiely, P. (2015). Mycotoxins in farm silages – a 2-year Irish national survey. Grass Forage Sci. 71, 339–352. doi: 10.1111/gfs.12191
Meca, G., Sospedra, I., Soriano, J. M., Ritieni, A., Moretti, A., and Manes, J. (2010). Antibacterial effect of the bioactive compound beauvericin produced by Fusarium proliferatum on solid medium of wheat. Toxicon 56, 349–354. doi: 10.1016/j.toxicon.2010.03.022
Mei, L., Zhang, L., and Dai, R. (2009). An inhibition study of beauvericin on human and rat cytochrome P450 enzymes and its pharmacokinetics in rats. J. Enzyme Inhib. Med. Chem. 24, 753–762. doi: 10.1080/14756360802362041
Mizukami, M., Otsuka, H., and Yokota, T. (2018). Global existence and boundedness in a chemotaxis-haptotaxis system with signal-dependent sensitivity. J. Math. Anal. Appl. 464, 354–369. doi: 10.1016/j.jmaa.2018.04.002
Nilanonta, C., Isaka, M., Kittakoop, P., Palittapongarnpim, P., Kamchonwongpaisan, S., Pittayakhajonwut, D., et al. (2000). Antimycobacterial and antiplasmodial cyclodepsipeptides from the insect pathogenic fungus Paecilomyces tenuipes BCC 1614. Planta Med. 66, 756–758. doi: 10.1055/s-2000-9776
Nilanonta, C., Isaka, M., Kittakoop, P., and Trakulnaleamsai, S. (2002). Precursordirected biosynthesis of beauvericin analogs by the insect pathogenic fungus Paecilomyces tenuipes BCC 1614. Tetrahedron 58, 3355–3360. doi: 10.1016/S0040-4020(02)00294-6
Patocka, J. (2016). Bioactive metabolites of entomopathogenic fungi Beauveria bassiana. Mil. Med. Sci. Lett. 85, 80–88. doi: 10.31482/mmsl.2016.015
Peczynska-Czoch, W., Urbanczyk, M. J., and Bałazy, S. (1991). Formation of beauvericin by selected strains of Beauveria bassiana. Arch. Immunol. Ther. Exp. 139, 175–179.
Peeters, H., Zocher, R., and Kleinkauf, H. (1988). Synthesis of beauvericin by a multifunctional enzyme. J Antibiotics 41, 352–359. doi: 10.7164/antibiotics.41.352
Prince, R. C., Crofts, A. R., and Steinrauf, L. K. (1974). A comparison of beauvericin, enniatin and valinomycin as calcium transporting agents in liposomes and chromatophores. Biochem. Biophys. Res. Commun. 59, 697–703. doi: 10.1016/S0006-291X(74)80036-7
Prosperini, A., Juan-García, A., Font, G., and Ruiz, M. J. (2013). Beauvericin-induced cytotoxicity via ROS production and mitochondrial damage in Caco-2 cells. Toxicol. Lett. 22, 204–211. doi: 10.1016/j.toxlet.2013.07.005
Qadri, S. M., Kucherenko, Y., and Lang, F. (2011). Beauvericin induced erythrocyte cell membrane scrambling. Toxicology 283, 24–31. doi: 10.1016/j.tox.2011.01.023
Quiles, J. M., Saladino, F., Mañes, J., Fernández-Franzón, M., and Meca, G. (2016). Occurrence of mycotoxins in refrigerated pizza dough and risk assessment of exposure for the Spanish population. Food Chem. Toxicol. 94, 19–24. doi: 10.1016/j.fct.2016.05.011
Santini, A., Meca, G., Uhlig, S., and Ritieni, A. (2012). Fusaproliferin, beauvericin and enniatins: occurrence in food-a review. World Mycotoxin J. 5, 71–81. doi: 10.3920/WMJ2011.1331
Schenzel, J., Hungerbühler, K., and Bucheli, T. D. (2012). Mycotoxins in the environment: II. Occurrence and origin in Swiss river waters. Environ. Sci. Technol. 46, 13076–13084. doi: 10.1021/es301558v
Schoevers, E. J., Santos, R. R., Fink-Gremmels, J., and Roelen, B. A. (2016). Toxicity of beauvericin on porcine oocyte maturation and preimplantation embryo development. Reprod. Toxicol. 65, 159–169. doi: 10.1016/j.reprotox.2016.07.017
Serrano, A. B., Font, G., Mañes, J., and Ferrer, E. (2013). Emerging Fusarium mycotoxins in organic and conventional pasta collected in Spain. Food Chem. Toxicol. 51, 259–266. doi: 10.1016/j.fct.2012.09.034
Serrano, A. B., Font, G., Ruiz, M. J., and Ferrer, E. (2012). Co-occurrence and risk assessment of mycotoxins in food and diet from Mediterranean area. Food Chem. 135, 423–429. doi: 10.1016/j.foodchem.2012.03.064
Sewram, V., Nieuwoudt, T. W., Marasas, W. F., Shephard, G. S., and Ritieni, A. (1999). Determination of Fusarium mycotoxins, fusaproliferin and beauvericin by high-perfomance liquid chromatography-electrospray ionization mass spectrometry. J. Chromatogr. A. 858, 175–185. doi: 10.1016/S0021-9673(99)00814-6
Shao, M., Li, L., Gu, Z., Yao, M., Xu, D., Fan, W., et al. (2018). Mycotoxins in commercial dry pet food in China. Food Addit. Contam. Part B Surveill. 11, 237–245. doi: 10.1080/19393210.2018.1475425
Shekhar-Guturja, T., Gunaherath, G. M., Wijeratne, E. M., Lambert, J. P., Averette, A. F., Lee, S. C., et al. (2016a). Dual action antifungal small molecule modulates multidrug efflux and TOR signaling. Nat. Chem. Biol. 12, 867–875. doi: 10.1038/nchembio.2165
Shekhar-Guturja, T., Tebung, W. A., Mount, H., Liu, N., Köhler, J. R., Whiteway, M., et al. (2016b). Beauvericin potentiates azole activity via inhibition of multidrug efflux, blocks Candida albicans morphogenesis, and is effluxed via Yor1 and circuitry controlled by Zcf29. Antimicrob. Agents Chemother. 60, 7468–7480. doi: 10.1128/AAC.01959-16
Shimada, A., Fujioka, S., Koshino, H., and Kimura, Y. (2010). Nematicidal activity of beauvericin produced by the fungus Fusarium bulbicola. Z. Naturforsch. C. J Biosci. 65, 207–210. doi: 10.1515/znc-2010-3-407
Shin, C. G., An, D. G., Song, H. H., and Lee, C. (2009). Beauvericin and enniatins H, I and MK1688 are new potent inhibitors of human immunodeficiency virus type-1 integrase. J. Antibiot. 62, 687–690. doi: 10.1038/ja.2009.102
Sifou, A., Meca, G., Serrano, A. B., Mahnine, N., El Abidi, A., Mañes, J., et al. (2011). First report on the presence of emerging Fusarium mycotoxins enniatins (A, A1, B, B1), beauvericin and fusaproliferin in rice on the Moroccan retail markets. Food Control 22, 1826–1830. doi: 10.1016/j.foodcont.2011.04.019
Srobarova, A., Moretti, A., Ferracane, R., Ritieni, A., and Logrieco, A. (2002). Toxigenic Fusarium species of liseola section in pre-harvest maize ear rot and associated mycotoxins in Slovakia. Eur. J. Plant Pathol. 108, 299–306. doi: 10.1023/A:1015645813231
Stanciu, O., Juan, C., Miere, D., Loghin, F., and Mañes, J. (2017). Presence of enniatins and beauvericin in romanian wheat samples: from raw material to products for direct human consumption. Toxins 9:E189. doi: 10.3390/toxins9060189
Steiniger, C., Hoffmann, S., Mainz, A., Kaiser, M., Voigt, K., Meyer, V., et al. (2017). Harnessing fungal nonribosomal cyclodepsipeptide synthetases for mechanistic insights and tailored engineering. Chem. Sci. 8, 7834–7843. doi: 10.1039/C7SC03093B
Sumikova, T., Chrpova, J., Dzuman, Z., Salava, J., Sterbova, L., Palicova, J., et al. (2017). Mycotoxins content and its association with changing patterns of Fusarium pathogens in wheat in the Czech Republic. World Mycotoxin J. 10, 143–151. doi: 10.3920/WMJ2016.2133
Svingen, T., Lund Hansen, N., Taxvig, C., Vinggaard, A. M., Jensen, U., and Have Rasmussen, P. (2017). Enniatin B and beauvericin are common in Danish cereals and show high hepatotoxicity on a high-content imaging platform. Environ. Toxicol. 32, 1658–1664. doi: 10.1002/tox.22367
Taevernier, L., Bracke, N., Veryser, L., Wynendaele, E., Gevaert, B., Peremans, K., et al. (2016a). Blood-brain barrier transport kinetics of the cyclic depsipeptide mycotoxins beauvericin and enniatins. Toxicol. Lett. 258, 175–184. doi: 10.1016/j.toxlet.2016.06.1741
Taevernier, L., Veryser, L., Roche, N., Peremans, K., Burvenich, C., Delesalle, C., et al. (2016b). Human skin permeation of emerging mycotoxins (beauvericin and enniatins). J. Expo. Sci. Environ. Epidemiol. 26, 277–287. doi: 10.1038/jes.2015.10
Tang, C. Y., Chen, Y. W., Jow, G. M., Chou, C. J., and Jeng, C. J. (2005). Beauvericin activates Ca2+-activated Cl- currents and induces cell deaths in Xenopus oocytes via influx of extracellular Ca2+. Chem. Res. Toxicol. 18, 825–833. doi: 10.1021/tx049733d
Tansakul, N., Jala, P., Laopiem, S., Tangmunkhong, P., and Limsuwan, S. (2013). Co-occurrence of five Fusarium toxins in corn-dried distiller's grains with solubles in Thailand and comparison of ELISA and LC-MS/MS for fumonisin analysis. Mycotoxin Res. 29, 255–260. doi: 10.1007/s12550-013-0173-z
Tao, Y. W., Lin, Y. C., She, Z. G., Lin, M. T., Chen, P. X., and Zhang, J. Y. (2015). Anticancer activity and mechanism investigation of beauvericin isolated from secondary metabolites of the mangrove endophytic fungi. Anticancer Agents Med. Chem. 15, 258–266. doi: 10.2174/1871520614666140825112255
Toman, E., Makrlík, E., and Vanura, P. (2011). On the complexation of the sodium cation with beauvericin: experimental and theoretical study. Monatsh. Chem. 142, 779–782. doi: 10.1007/s00706-011-0499-1
Tong, Y., Liu, M., Zhang, Y., Liu, X., Huang, R., Song, F., et al. (2016). Beauvericin counteracted multi-drug resistant Candida albicans by blocking ABC transporters. Synth. Syst. Biotechnol. 1, 158–168. doi: 10.1016/j.synbio.2016.10.001
Tonshin, A. A., Teplova, V. V., Andersson, M. A., and Salkinoja-Salonen, M. S. (2010). The Fusarium mycotoxins enniatins and beauvericin cause mitochondrial dysfunction by affecting the mitochondrial volume regulation, oxidative phosphorylation and ion homeostasis. Toxicology 276, 49–57. doi: 10.1016/j.tox.2010.07.001
Uhlig, S., Jestoi, M., and Parikka, P. (2007). Fusarium avenaceum—the North European situation. Int. J. Food Microbiol. 119, 17–24. doi: 10.1016/j.ijfoodmicro.2007.07.021
Uhlig, S., Torp, M., and Heier, B. T. (2006). Beauvericin and enniatins A, A1, B and B1 in Norwegian grain: a survey. Food Chem. 94, 193–201. doi: 10.1016/j.foodchem.2004.11.004
Van der Fels-Klerx, H. J., Kandhai, M. C., Brynestad, S., Dreyer, M., Börjesson, T., Martins, H. M., et al. (2009). Development of a European system for identification of emerging mycotoxins in wheat supply chains. World Mycotoxin J. 2, 119–127. doi: 10.3920/WMJ2008.1122
Wang, Q., and Xu, L. (2012). Beauvericin, a bioactive compound produced by fungi: a short review. Molecules 17, 2367–2377. doi: 10.3390/molecules17032367
Wätjen, W., Debbab, A., Hohlfeld, A., Chovolou, Y., and Proksch, P. (2014). The mycotoxin beauvericin induces apoptotic cell death in H4IIE hepatoma cells accompanied by an inhibition of NF-κB-activity and modulation of MAP-kinases. Toxicol. Lett. 231, 9–16. doi: 10.1016/j.toxlet.2014.08.021
Wu, Q., Wang, X., Nepovimova, E., Miron, A., Liu, Q., Wang, Y., et al. (2017). Trichothecenes: immunomodulatory effects, mechanisms, and anti-cancer potential. Arch. Toxicol. 91, 3737–3785. doi: 10.1007/s00204-017-2118-3
Wu, Q., Wang, X., Nepovimova, E., Wang, Y., Yang, H., and Kuca, K. (2018). Mechanism of cyclosporine A nephrotoxicity: oxidative stress, autophagy, and signalings. Food Chem. Toxicol. 118, 889–907. doi: 10.1016/j.fct.2018.06.054
Wu, X., and Smith, J. S. (2007). A gas chromatography-flame ionization detection method for detection of fusaproliferin in corn. J. Agric. Food Chem. 55, 3211–3216. doi: 10.1021/jf063489+
Wu, X. F., Xu, R., Ouyang, Z. J., Qian, C., Shen, Y., Wu, X. D., et al. (2013). Beauvericin ameliorates experimental colitis by inhibiting activated T cells via downregulation of the PI3K/Akt signaling pathway. PLoS ONE 8:e83013. doi: 10.1371/journal.pone.0083013
Xu, L., Wang, J., Zhao, J., Li, P., Shan, T., Wang, J., et al. (2010). Beauvericin from the endophytic fungus, Fusarium redolens, isolated from Dioscorea zingiberensis and its antibacterial activity. Nat. Prod. Commun. 5, 811–814. doi: 10.1016/j.jbiotec.2008.07.304
Xu, Y., Orozco, R., Wijeratne, E. M., Gunatilaka, A. A., Stock, S. P., and Molnár, I. (2008). Biosynthesis of the cyclooligomer depsipeptide beauvericin, a virulence factor of the entomopathogenic fungus Beauveria bassiana. Chem. Biol. 15, 898–907. doi: 10.1016/j.chembiol.2008.07.011
Yoo, S., Kim, M. Y., and Cho, J. Y. (2017). Beauvericin, a cyclic peptide, inhibits inflammatory responses in macrophages by inhibiting the NF-κB pathway. Korean J. Physiol. Pharmacol. 21, 449–456 doi: 10.4196/kjpp.2017.21.4.449
Yoshinari, T., Suzuki, Y., Sugita-Konishi, Y., Ohnishi, T., and Terajima, J. (2016). Occurrence of beauvericin and enniatins in wheat flour and corn grits on the Japanese market, and their co-contamination with type B trichothecene mycotoxins. Food Addit. Contam. Part A 33, 1620–1626. doi: 10.1080/19440049.2016.1228126
Zhan, J., Burns, A. M., Liu, M. X., Faeth, S. H., and Gunatilaka, A. A. (2007). Search for cell motility and angiogenesis inhibitors with potential anticancer activity: Beauvericin and other constituents of two endophytic strains of Fusarium oxysporum. J. Nat. Prod. 70, 227–232. doi: 10.1021/np060394t
Zhang, H., Ruan, C., Bai, X., Zhang, M., Zhu, S., and Jiang, Y. (2016). Isolation and identification of the antimicrobial agent beauvericin from the endophytic Fusarium oxysporum 5-19 with NMR and ESI-MS/MS. Biomed. Res. Int. 2016:1084670. doi: 10.1155/2016/1084670
Zhang, L., Yan, K., Zhang, Y., Huang, R., Bian, J., Zheng, C., et al. (2007). High-throughput synergy screening identifies microbial metabolites as combination agents for the treatment of fungal infections. Proc. Nat. Acad. Sci. U. S. A. 104, 4606–4611. doi: 10.1073/pnas.0609370104
Zhao, D., Liu, B., Wang, Y., Zhu, X., Duan, Y., and Chen, L. (2013). Screening for nematicidal activities of Beauveria bassiana and associated fungus using culture filtrate. African J. Microbiol. Res. 7, 974–978. doi: 10.5897/AJMR12.2340
Zinedine, A., Meca, G., Mañes, J., and Font, G. (2011). Further data on the occurrence of Fusarium emerging mycotoxins enniatins (A, A1, B, B1), fusaproliferin and beauvericin in raw cereals commercialized in Morocco. Food Control 22, 1–5. doi: 10.1016/j.foodcont.2010.05.002
Zobel, S., Boecker, S., Kulke, D., Heimbach, D., Meyer, V., and Süssmuth, R. D. (2016). Reprogramming the biosynthesis of cyclodepsipeptide synthetases to obtain new enniatins and beauvericins. Chembiochem 17, 283–287. doi: 10.1002/cbic.201500649
Keywords: beauvericin, biosynthesis, bioactivity, anticancer, Fusarium
Citation: Wu Q, Patocka J, Nepovimova E and Kuca K (2018) A Review on the Synthesis and Bioactivity Aspects of Beauvericin, a Fusarium Mycotoxin. Front. Pharmacol. 9:1338. doi: 10.3389/fphar.2018.01338
Received: 11 August 2018; Accepted: 30 October 2018;
Published: 20 November 2018.
Edited by:
Yiqun Deng, South China Agricultural University, ChinaReviewed by:
Daniela Gwiazdowska, Poznan University of Economics, PolandAgnieszka Waśkiewicz, Poznan University of Life Sciences, Poland
Copyright © 2018 Wu, Patocka, Nepovimova and Kuca. This is an open-access article distributed under the terms of the Creative Commons Attribution License (CC BY). The use, distribution or reproduction in other forums is permitted, provided the original author(s) and the copyright owner(s) are credited and that the original publication in this journal is cited, in accordance with accepted academic practice. No use, distribution or reproduction is permitted which does not comply with these terms.
*Correspondence: Kamil Kuca, a2FtaWwua3VjYUB1aGsuY3o=