- 1Key Laboratory of Anti-Inflammatory and Immune Medicine, Ministry of Education, Collaborative Innovation Center of Anti-Inflammatory and Immune Medicine, Institute of Clinical Pharmacology, Anhui Medical University, Hefei, China
- 2Department of Emergency Medicine, The First Affiliated Hospital, University of Science and Technology of China, Hefei, China
Disorder of the sympathetic nervous system (SNS) is closely related to the pathogenesis of various autoimmune diseases (ADs). Catecholamine triggered beta2-adrenergic receptor (β2-AR) signaling is important in creating a bidirectional response in the progression of ADs due to factors including diverse expression patterns, single nucleotide polymorphisms (SNPs), biased signals, and desensitization of β2-AR, as well as different subtypes of Gα binding to β2-AR. In this review, we summarize the actions of β2-AR signaling in regulating the functions of immunocytes and in the pathogenesis of ADs, and the application of β2-AR agonists or antagonists in treating major types of ADs is also discussed. We suggest that restoring the immune balance via a soft regulation of the expression or activation of β2-AR is one of the promising therapeutic strategies for systematic ADs.
Introduction
Although the current understanding of the pathogenesis of autoimmune diseases (ADs) such as systemic lupus erythematosus (SLE), rheumatoid arthritis (RA), myasthenia gravis (MG), and Grave’s disease (GD) is unsettled; many ADs hold in common the expression of autoantigens, abnormal immunoregulation, and shared genetic factors (Harris et al., 2018). Researchers have found that the nervous system is an important regulator in the function of immune cells and thus affects inflammation important in the pathogenesis of ADs (Weissert, 2017; Bellinger and Lorton, 2018). Elevated pro-inflammatory cytokines signal to brain and influence the activity and reactivity level of sympathetic nerves (Kenney and Ganta, 2014). Chronic inflammation in RA is accompanied by activation of the sympathetic nerves system (SNS) and relative parasympathetic hypofunction (Lowin and Straub, 2015). This imbalanced autonomic nervous system is a consistent feature of RA patients. Both central and peripheral immune organs innervated precisely by sympathetic nervous (Bellinger and Lorton, 2018). Activated sympathetic nervous secrete abundant epinephrine (E) and norepinephrine (NE), which activate α-adrenergic receptors (α-ARs) and β-ARs in immune cells to regulate immune response (Janig and Green, 2014). Literatures reported that β-ARs, which include β1-AR, β2-AR, and β3-AR play important roles in inflammation. Of note, β2-AR is regarded to play a key role in the process of immunological imbalance (Chavan and Tracey, 2017). β-ARs belong to the seven transmembrane G-protein coupled receptors (GPCRs). β1-AR couples with Gαs, while β2-AR is able to couple both Gαs and Gαi. The transition in G-protein coupling from Gαs to Gαi, thus influences cAMP production within the β2-AR microenvironment (Fu et al., 2014). This review will summarize the effects of β2-AR in regulating the function of immunocytes and in the pathogenesis of Ads, with special emphasis on how β2-AR can exert bidirectional function depending on the stage of the disease.
Regulatory Effects of β2-AR on Immune Cells
β2-AR is widely expressed, with distinct densities, on various immune cells, including T cells, B cells, dendritic cells (DCs), and macrophages (Kolmus et al., 2015). Evidence amply confirms its role in immunomodulation. However, during the different processes of immune diseases, β2-AR exerts contradictory effects on immunocytes (Pongratz and Straub, 2013).
T Cells
β2-AR expression is predominant in human and murine T cells when comparing with other immune cell subtypes (Ross et al., 2018). Studies suggest that CD8+ cytotoxic T cells (Tc) express a significantly higher level of β2-AR compared to CD4+ helper T cells (Th). Therefore, stimulation of β2-AR reduced the percentage of interferon-γ (IFN-γ) + Tc to a much higher extent than that of IFN-γ + Th (Zalli et al., 2015). Moreover, the level of β2-AR on memory Tc is further increased than that on naïve Tc, leading to a more sensitive response of memory Tc to catecholamine stimulation manifesting as a decrease in cytokine production (Slota et al., 2015). Thus, treatments that activate β2-AR achieve their immunoregulatory effects primarily through inhibiting the cytokine secretion ability and the cell killing function of Tc, as well as some natural killer (NK) cells which also express CD8. Stimulation of β2-AR reduces the production of interleukin-2 (IL-2), granulocyte-macrophage colony-stimulating factor (GM-CSF), IFN-γ, and IL-3; yet, it does not influence IL-4 level (Grailer et al., 2014) in murine T cells. However, in human T cells, β2-AR signals to IL-2 production facilitates the Th2 cell differentiation and recues the Th1/Th2 balance under the circumstances of inflammation which indicates that the β2-AR function is species dependent (Scanzano and Cosentino, 2015). Even from the same species, the T cells from different tissues are diversely regulated by β2-AR. Chronic β2-AR stimulation differentiates cord blood T cells to Th2 cells, while it cannot promote the differentiation of peripheral blood T cells (Padro and Sanders, 2014). β2-AR agonist terbutaline exacerbates anti-CD3/anti-CD28-induced IL-17A production but reduces IFN-γ-secreting Th1 cells, suggesting that β2-AR plays a reciprocal role in modulating human Th1/Th17 balance (Carvajal Gonczi et al., 2017).
Interestingly, β2-AR attenuates inflammation and immunization by restricting T cells in immune organs (Tracey, 2014). Activation of IL-13+ human memory T cells by β-AR agonist immediately increases the production of cyclic adenosine monophosphate (cAMP) and the activity of protein kinase A (PKA), leading to reduced p38 mitogen-activated protein kinase (MAPK) activation. Subsequently, p38 MAPK inhibits CD3-induced CD25 expression and CD3-mediated IL-13, IFN-γ, and IL-2 production (Lajevic et al., 2011). Calcimycin and phorbol myristate acetate (PMA)-triggered p38 MAPK, ERK, and nuclear factor-κB (NF-κB) activation is biasedly inhibited by β-agonist leading to selective reduction of IFN-γ and IL-2 production but not IL-13 (Loza et al., 2006). Therefore, the impaired function of β2-AR in circulating T cells may induce immunological diseases such as RA by decreasing production of select cytokines (Kenney and Ganta, 2014).
B Cells
Autoantibodies produced by B cells are widely observed in the majority of ADs. When activated, β2-AR initially couples with Gαs to promote physiological production of cAMP and inhibit the proliferation of human peripheral B cells (Faisy et al., 2010). However, the expression of β2-AR is reduced on B cells in RA patients, leading to the abnormal survival of activated B cells and the accelerating progress of disease. Catecholamines including E and NE are reported to have a positive effect on attenuating specific mitogens that mediate B cell proliferation. This function can be abolished by β2-AR antagonists (Hu et al., 2013). Therefore, exciting the autonomic nervous system is regarded as a promising therapeutic strategy for treating RA (Ulloa et al., 2017).β2-AR signaling impairs IL-17 receptor induced maturation and anti-collagen II autoantibody production of B cells in mice with collagen induced arthritis (CIA) (Pongratz et al., 2014). Researchers also reported that activation of β2-AR by NE accelerates antibody responses of B cells upon immune challenge (Simkins et al., 2014). Antigen exposed B cells or β2-AR agonist terbutaline treated B cells express higher level of CD86 than resting B cells, combining with CD86 stimulation, IgG1 and IgE production is obvious increased in IL-4 dependent manner. β2-AR induces CD86 expression through cAMP-PKA pathway (Qiao et al., 2018). Later on, people found that combined stimulation of CD86 and β2-AR increases IgG1 secretion of human B cells through the promotion of transcription of Oct-2 and its coactivator OCA-B (Podojil and Sanders, 2005). When ADAM10 gene transcription and CD23 expression is enhanced by the activation of β2-AR, the upregulated CD23 and ADAM10 can be shuttled to exosomes and stimulate IgE production of recipient primed B cells (Padro et al., 2013). In addition, β2-AR signaling promotes the expression of IgE and its regulator soluble CD23 through downstream PKA and p38 MAPK pathways in B cells (Sergienko et al., 2012). Taken together, these studies portray a picture where a reduction in β2-AR signaling in B cells of individuals with AD is associated with unchecked B cell proliferation, increased autoantibody production, and a reduction in immune response upon challenge. Moreover, the different mechanisms on B cell activation and isotype switching provide a clinical therapeutic target in IgG1 or IgE response-mediated diseases.
Dendritic Cells
Dendritic cells (DCs) express α1-AR, α2-AR, β1-AR and β2-AR. Nevertheless, β2-AR is the primary effecter of DCs for cytokine production and antigen presentation, with β2-AR able to exert either a stimulatory or inhibitory function that is dependent on the differentiation status of DCs and/or the activation time of the receptor (Wu et al., 2016). Activation of β2-AR with the selective agonist clenbuterol restrains human DCs differentiation from monocyte (Giordani et al., 2015). In differentiated DCs, in vitro treatment with isoproterenol (ISO) reduced CD86 and MHC-II expression, enhanced antigen uptake and IL-10 production, and simultaneously prevented T cells activation and pro-inflammatory cytokines secretion such as TNF-α in a β2-AR dependent manner (Wu et al., 2016). In another study, stimulation of β2-AR by NE induced the migration of DCs from antigen sites to immune organs but suppressed the antigen presentation capacity of DCs to activate T cells. In detail, the stimulation of β2-AR on CD11c+CD8a+ DCs impaired DC mediated CD8+ naïve T cell activation (Nijhuis et al., 2014). Meanwhile, β2-AR signaling in DCs reduces IL-12 production via inhibition of the activation of NF-κB pathway. Decreased IL-12 leads to a shift in the balance of IL-12/IL-13 in LPS induced DCs, preventing IFN-γ production and Th1 development while, increasing IL-17 levels, thus affecting adaptive immunity (Takenaka et al., 2016). Stimulation of β2-AR also decreases the level of IL-1, IL-6, and TNF-α through attenuating toll-like receptor (TLR) response (Manni et al., 2011). Therefore, the major effect of β2-AR stimulation in DCs is a reduction in the number of T cells activated which correlates with a reduction in cytokine release from DCs.
Macrophages
Accumulating evidence shows that β2-AR signaling plays a pivotal role in macrophage activation and pro-inflammatory cytokine production. Some researchers reported that stimulation of β2-AR inhibits the activation of RAW264.7 (a mouse macrophage cell line) and THP-1 (a human monocytic cell line) induced by LPS through restraining ERK1/2, JNK and NF-κB pathways (Sharma et al., 2017). Further studies have revealed that β2-AR attenuates ERK activation in a cAMP-dependent manner, and blunts NF-κB pathways via increasing β-arrestin2 expression which is a scaffold protein for IκBα activation (Keranen et al., 2017). The up-regulated cAMP upon β2-AR response triggers cAMP-response element binding protein (CREB), CCAAT/enhancer binding protein beta (C/EBPβ), and activating transcription factor (ATF) signaling. This results in the polarization of macrophages to M2, which is an anti-inflammatory subset (Lamkin et al., 2016). Cyclic-AMP induced expression of mitogen-activated protein kinase phosphatase 1 (MKP-1), an anti-inflammatory factor, also contributes to the negative regulation of macrophages (Levine et al., 1988). Therefore, exciting β2-AR induces the differentiation of microphages into M2 subtype and promotes expression of type 2 cytokines, including IL-6 and TGF-β while simultaneously decreasing the production of pro-inflammatory cytokines such as TNF-α (Grailer et al., 2014). Similarly, β2-AR signaling decreases IL-6, IL-1β and TNF-α in human macrophages derived from monocyte (Ağaç et al., 2018). Nonetheless, β2-AR signaling evokes the secretion of the tumor necrosis factor (ligand) superfamily, member 11 (RANKL) therefore initiating the differentiation of osteoclasts (Jiao et al., 2015).
Other Immune Cells
β2-AR exerts complicated effects on immune cells and influences the function of almost all kinds of immune cells. Stimulation of β2-AR on peripheral blood mononuclear cells (PBMCs) inhibits interferon alpha-1 (IFNA1) production mediated by Toll like receptor 9 (TLR9) (Hilbert et al., 2013). It drives leukocyte migration and tissue infiltration via enhancement of chemokine production (Grisanti et al., 2016). β2-AR signaling is involved in the reduction of NK cells and inhibits their cytotoxicity (De Lorenzo et al., 2015). But conflicting evidence shows that the sympathetic response attracts mature and activated NKs to peripheral blood and primes innate immunity (Bigler et al., 2015). Taken together, the apparent conflicting functions of β2-AR within the immune system lead to the controversial role of β2-AR in the onset and development of ADs.
The Role of β2-AR in the Pathogenesis of ADs
The SNS is activated in ADs and acts on humoral and cellular immunity primarily through β2-AR. Having a better understanding of β2-AR in the course and progression of ADs will help to develop β2-AR targeted drugs for the treatments of ADs (Zhao et al., 2011).
Rheumatoid Arthritis
RA is a long-lasting autoimmune disorder that primarily affects joints. Evidence reveals that the polymorphisms of β2-AR determine the susceptibility of RA. The studies report single nucleotide polymorphisms (SNPs) at codon 16 together with the HLA-DRB1∗04 mutation have a positive correlation with the expression of anti-cyclic citrullinated peptide (CCP) and are strongly associated with RA (Malysheva et al., 2008). Similarly, in northern Sweden, RA susceptibility and activity is higher in patients with variants at Arg16 or Gln27 (Xu et al., 2005). Generally, in RA patients, the expression of β2-AR is decreased on both peripheral blood lymphocytes (PBLs) and synovial fluid lymphocytes (SFLs), especially on SFLs (Wahle et al., 2002). Therefore, β2-AR response is significantly attenuated in patients with RA. Therefore, β2-AR induced B cell death is impaired, which then leads to the elevated production of autoantibodies and the progress of RA (Sanders, 2012). As a further result, T cells fail to shift to the Th2 subtype in response to catecholamines stimulation, causing decreased levels of anti-inflammatory cytokines (Lubahn et al., 2014).
Results indicate that β2-AR desensitization of the β2-AR-AC-cAMP transmembrane signal transduction pathway plays a crucial role in the ongoing inflammation of RA (Zhao et al., 2011). However, β2-AR signaling is observed to exert different functions during the onset of pathogenesis of RA. In the early stages of RA, catecholamines are released from the sympathetic nerve terminal and stimulate β2-AR on T cells, promoting production of IFN-γ, which is a beneficial target for treating RA (Lee et al., 2017). Nevertheless, when catecholamines bind to β2-ARs on other immune cells, they exacerbate the inflammation. For example, β2-AR stimulation promotes B cell autoantibody secretion; drives macrophages to generate various chemokines and pro-inflammatory cytokines including IL-1β and TNF-α; and enhance DC capacity for autoantigen uptake and processing, as well as TNF-α, IL-12, and IL-6 production. At first glance it would appear that β2-AR signaling produced from conflicting roles in regulation of immunoreactions in different cells might cancel each other out, but actually the result is that overall β2-AR signaling accelerates the development of inflammation in early stages of RA. In late stage RA, β2-AR primarily exerts immunosuppressive action by facilitating IFN-γ production from T cells; IL-10 secretion from B cells and macrophages; and IL-10, IL-33 expression from DCs. Moreover, it restrains DC generated inflammatory cytokines such as TNF-α, IL-12, and IL-6 (Pongratz and Straub, 2013) (Figure 1). These studies indicate that the divergent role of β2-AR is cell type and time point dependent.
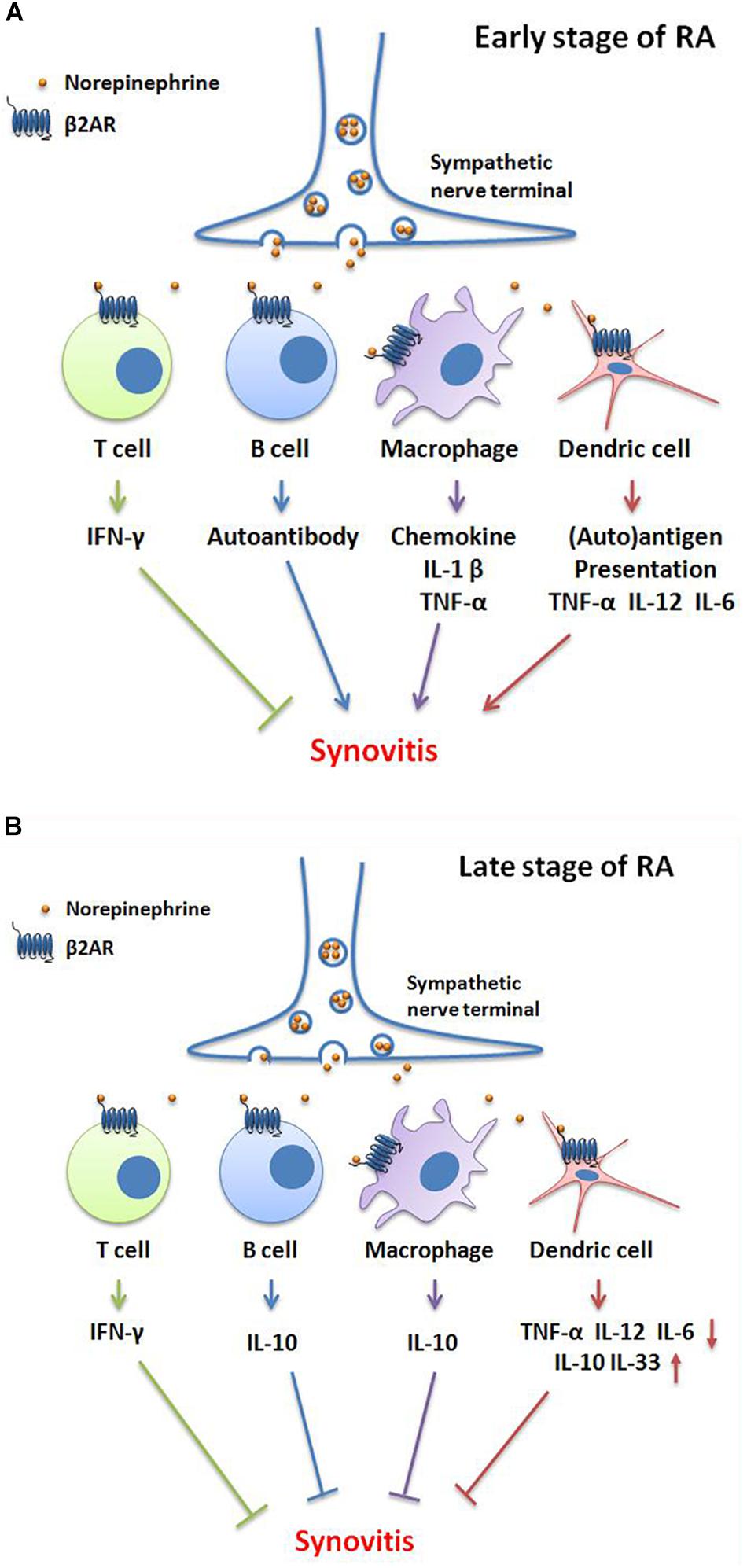
FIGURE 1. Bidirectional functions of β2-AR signaling in different stages of RA. (A) In the early stage of RA, norepinephrine-mediated-β2-AR signaling promotes IFN-γ production of T cells, which is an inhibitory cytokine in RA. However, β2-AR facilitates autoantibody secretion by B cells; chemokine, IL-1β and TNF-α production by macrophages; and auto-antigen presentation, TNF-α, IL-12 and IL-6 generation by dendric cells (DCs), consequently aggravates synovitis and clinical symptoms. (B) In the late stage of RA, the activation of β2-AR induces IFN-γ production by T cells; IL-10 generation by B cells and macrophages; decreases the secretion of TNF-α, IL-12, and IL-6 and, increases the IL-10 and IL-33 levels by DCs. These changes then attenuate synovitis and clinical symptoms.
Systemic Lupus Erythematosus
SLE is a very different disease from RA. Clinical observations indicate that psychological stress, which often stimulates the SNS, aggravates the activity of SLE (Morand, 2018). The up-regulation of β2-AR on PBMC from SLE patients in response to stress is significantly abolished when compared to healthy subjects (Pawlak et al., 1999). As a consequence, the increment of cAMP in B cells upon β2-AR activation is significantly reduced in SLE patients (Wahle et al., 2001). In addition, β2-AR stimulation exacerbates the production of serum anti-DNA antibodies in MRL/1 pr mice (Chedraoui et al., 2008). Therefore, blockage of β2-AR signaling on B cells is able to successfully reduce the level of immunoglobulin, and attenuates SLE (Hudson et al., 2005).
Multiple Sclerosis
Multiple sclerosis (MS) is a potentially disabling disease of the central nervous system (Giovannoni, 2017). The mechanisms of progressive focal inflammatory demyelinating lesions is complex, but basically due to the functional loss of β2-AR, which is important in facilitating glycogenolysis and reducing inducible nitric oxide synthase (NOS2) in astrocytes (Cambron et al., 2012). The impaired β2-AR signaling leads to metabolic disorder in axons, the unbalanced distribution of potassium in astrocytes, and an increase in calcium concentration in astrocytes. The transformation of astrocytes to antigen-presenting cells that ensues triggers inflammation, as well as glutamate excitotoxicity, which results in the progression of damage to the myelin sheath (Durfinova et al., 2014). The expression of β2-AR is found to be upregulated on PBMCs of MS patients, but does not change the immune response of T cells (Giorelli et al., 2004). Stimulation of β2-AR on astrocytes promotes intracellular cAMP production and PKA activation, inhibits the transcription of coactivator class II transactivator (CIITA) and IFN-γ-induced major histocompatibility (MHC) class II molecule, thus suppressing the antigen presenting activity of astrocytes and finally reducing the focal inflammatory demyelinating lesions (De Keyser et al., 2010). Thus, measures that enhancing the expression of β2-AR by IFN-β administration or exercise, or exciting β2-AR response by agonists may be considered as promising strategies in treating MS. A total population-based case-control study performed by Tsai et al. (2014) revealed that the β2-AR agonist, fenoterol significantly improved outcomes associated with MS, and reduced the risk of MS by 51%.
Myasthenia Gravis
MG has the characteristic of formation of autoantibodies to acetylcholine nicotinic receptors at the neuromuscular junction of skeletal muscles (Nacu et al., 2015). The expression of β2-AR on PBMC of MG patients is reduced leading to the attenuated signaling transduction. Thus, stimulation of β2-AR effectively ameliorates many symptoms of MG induced by anti-muscle specific kinase (Ghazanfari et al., 2014). In addition, autoantibodies can also target the second extracellular loop of β2-AR (residues 172–197), which amplifies the β2-AR reactive T and B cells and involves in the patho-genesis of MG. The risk of production of β2-AR autoantibodies in MG patients is increased if SNPs of Arg16Gly on β2-AR are present (Lantsova et al., 2013). Evidence also shows similar polymorphisms of β2-AR affect the incidence of many kinds of ADs such as RA, juvenile idiopathic arthritis (JIA), and GD, the same as MG. The homozygosity for Gly16 can be applied to predict the tolerance of β2-AR agonist treatment, again suggesting that this SNP plays a key role in AD pathology (Lantsova et al., 2013; Wang et al., 2017).
Grave’s Disease
GD is an AD that affects the thyroid. Although the pathogenesis of GD is still unclear, genetic predisposition, especially for SNPs within β2-AR is regarded to be important in the development of GD (Park et al., 2017). Multiple studies have revealed that the SNPs 47A– > G (Arg16Gly) and 79C– > G (Gln27Glu) on chromosomal region 5q31-33 in β2-AR as well as rs1042714 SNPs contribute to the susceptibility of GD in a Caucasian population. However, these polymorphisms have no significant correlation to the risk of GD in Chinese and Korean male populations (Jazdzewski et al., 2007). Differences in how specific SNPs in β2-AR affect the outcomes of GD in different racial groups highlight the probability that GD, as well as other ADs, are polygenic disorders which need more study to identify important genetic factors that can be targeted for treatment.
Other ADs
Genomic variations contribute to the morbidity of various ADs besides those discussed above. The significant prevalence of homozygous Gly16Arg genotype in β2-AR has been found to also have a positive correlation with the predisposition to interstitial cystitis (Sugaya et al., 2002). Conversely, the variation of β2-AR does not influence susceptibility to JIA, even though it has similar symptoms to RA (Pont-Kingdon et al., 2009). In JIA, catecholamine response is still abnormally changed. For instance, β2-AR stimulation reduces cAMP level, probably due to its faster degradation (Kavelaars et al., 1998). The divergent effects of β2-AR perturbation in these different ADs underscore the bidirectional nature of this important receptor.
Targeting β2-AR Treatments of ADs
Catecholamine has both pro- and anti-inflammatory effects in patients with chronic inflammatory disease (Woo et al., 2014). Briefly, by exciting β2-AR, it attenuates type 1 ADs (characterized for cellular immunity) and aggravates the symptoms of type 2 ADs (characterized for humoral immunity) by exciting β2-AR (Namazi, 2004). So far, β2-AR-specific agonists (including salbutamol, metaproterenol, terbutaline, salmetero, ect) or antagonists (such as propranolol, butoxamine, ICI 118551, and so on) have been investigated in treating ADs with inconsistent results probably due to the non-synonymous SNPs of β2-AR, desensitization, or the Gαi/Gαs coupling switch of this receptor in the disease stage (Tandale et al., 2016). The infusion of adrenaline was shown to be a safe and valid method to induce immunological changes in RA patients, with patients presenting an increase in both IL-8- and IL-10-producing monocytes after infusion (Namazi, 2004). In animal models, systemic treatments with a β2-AR agonists like salbutamol or terbutaline is only effective in reducing disease severity when administered starting at disease onset of CIA (Pongratz and Straub, 2014). Correspondingly, the β2-AR agonist fenoterol improves outcomes associated with MS (Tsai et al., 2014).
Conversely, during the initiation phase of adjuvant arthritis (AA), selective β2-AR antagonist butoxamine or ICI 118, 551 induces CD4+ Th0 cells to develop into Th1 cells by blocking the inhibitory effects of endogenous NE on Th1 cell development. β2-AR blockade effectively shifts the immune response to the arthritogenic antigen toward a cell-mediated response, that is beneficial for the processing of the arthritogenic antigen. Consequently, blocking β2-AR function during AA development can reduce pro-inflammatory cytokines production via inhibition of the expression and activation of α1-AR, which is elevated by chronic β2-AR stimulation. Therefore, β-blockers decrease arthritic severity in the active phase of AA (Koopman et al., 2011).
Likewise, the application of salbutamol is able to attenuate the rat model of experimental allergic encephalomyelitis (EAE) by decreasing the average neurologic function score of model rats, reducing calpain activity in brain tissues, and rescue the activities of the BB isoenzymes of creatine kinase and catalase (Zhang et al., 2015). Similarly, the blockage of β2-AR using carvedilol protects rats with experimental autoimmune myocarditis (EAM) by ameliorating systolic and diastolic heart function, decreasing the serum level of IL-1β and TNF-α, and increasing IL-10 expression (Liu et al., 2010). More research investigating the effects of β2-agonists or β2-blockers on ADs are listed in Table 1.
Conclusion
Taken together, β2-AR signaling is involved in the pathogenesis and progress of ADs, and is a potential drug target in treating these diseases. However, the genetic polymorphisms and the expression of the receptor is diverse from individual patients or different disease stages. Meanwhile, the function of β2-AR signaling is bidirectional between different species, different immune cell isotype, and different time point of diseases. In a particular situation, β2-AR aggravates inflammation, but in some cases, it reduces the production of pro-inflammatory cytokines and auto-antibodies, acts as an immunosuppressor. Therefore, there is a need for thorough elucidation of the precise regulation of β2-AR in each specific AD (Kohr et al., 2011). In addition, soft regulation of the expression or activation of β2-AR that restores the immune balance will definitely be beneficial to the systematic immune diseases (Wei, 2016).
Author Contributions
LW wrote the text. YT, MZ, and RW collected references. WZ and JT drew the cartoon. YH wrote the table. QW and WW revised the article. QW designed the topic.
Funding
This work was financially supported through the National Natural Science Foundation of China (Nos. 81330081 and 81202541), the Anhui Provincial Natural Science Foundation (1808085J28 and 1408085MH173), the Key Projects of Natural Science Research of Anhui Colleges and Universities (KJ2017A176), Anhui University Excellent Youth Talent Support Program (gxyqZD2017025), Innovation and Entrepreneurship Support Program for Returnees of Anhui Province (2017), Young Outstanding Doctor Research Program, Anhui Provincial Hospital (2015), and the Key Research and Development Plan of Anhui Province (1804h08020291).
Conflict of Interest Statement
The authors declare that the research was conducted in the absence of any commercial or financial relationships that could be construed as a potential conflict of interest.
References
Ağaç, D., Gill, M. A., and Farrar, J. D. (2018). Adrenergic signaling at the interface of allergic asthma and viral infections. Front. Immunol. 9:736. doi: 10.3389/fimmu.2018.00736
Bellinger, D. L., and Lorton, D. (2018). Sympathetic nerve hyperactivity in the spleen: causal for nonpathogenic-driven chronic immune-mediated inflammatory diseases (IMIDs)? Int. J. Mol. Sci. 19:E1188. doi: 10.3390/ijms19041188
Bigler, M. B., Egli, S. B., Hysek, C. M., Hoenger, G., Schmied, L., Baldin, F. S., et al. (2015). Stress-induced in vivo recruitment of human cytotoxic natural killer cells favors subsets with distinct receptor profiles and associates with increased epinephrine levels. PLoS One 10:e0145635. doi: 10.1371/journal.pone.0145635
Cambron, M., D’haeseleer, M., Laureys, G., Clinckers, R., Debruyne, J., and De Keyser, J. (2012). White-matter astrocytes, axonal energy metabolism, and axonal degeneration in multiple sclerosis. J. Cereb. Blood Flow Metab. 32, 413–424. doi: 10.1038/jcbfm.2011.193
Carvajal Gonczi, C. M., Tabatabaei Shafiei, M., East, A., Martire, E., Maurice-Ventouris, M. H. I., and Darlington, P. J. (2017). Reciprocal modulation of helper Th1 and Th17 cells by the β2-adrenergic receptor agonist drug terbutaline. FEBS J 284, 3018–3028. doi: 10.1111/febs.14166
Chavan, S. S., and Tracey, K. J. (2017). Essential Neuroscience in Immunology. J. Immunol. 198, 3389–3397. doi: 10.4049/jimmunol.1601613
Chedraoui, A., Uthman, I., Abbas, O., and Ghosn, S. (2008). Adrenergic urticaria in a patient with anti-double-stranded DNA antibodies. Acta Derm. Venereol. 88, 263–266. doi: 10.2340/00015555-0435
Cobelens, P. M., Kavelaars, A., Vroon, A., Ringeling, M., van der Zee, R., van Eden, W., et al. (2002). The β2-adrenergic agonist salbutamol potentiates oral induction of tolerance, suppressing adjuvant arthritis and antigen-specific immunity. J. Immunol. 169, 5028–5035. doi: 10.4049/jimmunol.169.9.5028
De Keyser, J., Laureys, G., Demol, F., Wilczak, N., Mostert, J., and Clinckers, R. (2010). Astrocytes as potential targets to suppress inflammatory demyelinating lesions in multiple sclerosis. Neurochem. Int. 57, 446–450. doi: 10.1016/j.neuint.2010.02.012
De Lorenzo, B. H., De Oliveira Marchioro, L., Greco, C. R., and Suchecki, D. (2015). Sleep-deprivation reduces NK cell number and function mediated by beta-adrenergic signalling. Psychoneuroendocrinology 57, 134–143. doi: 10.1016/j.psyneuen.2015.04.006
Durfinova, M., Bartova, R., Prochazkova, L., Balco, M., Liska, B., and Gavurnikova, G. (2014). Role of astrocytes in pathogenesis of multiple sclerosis and their participation in regulation of cerebral circulation. Neuro Endocrinol. Lett. 35, 666–672.
Faisy, C., Pinto, F. M., Blouquit-Laye, S., Danel, C., Naline, E., Buenestado, A., et al. (2010). beta2-Agonist modulates epithelial gene expression involved in the T- and B-cell chemotaxis and induces airway sensitization in human isolated bronchi. Pharmacol. Res. 61, 121–128. doi: 10.1016/j.phrs.2009.08.003
Fu, Q., Xu, B., Liu, Y., Parikh, D., Li, J., Li, Y., et al. (2014). Insulin inhibits cardiac contractility by inducing a Gi-biased beta2-adrenergic signaling in hearts. Diabetes Metab. Res. Rev. 63, 2676–2689. doi: 10.2337/db13-1763
Ghazanfari, N., Morsch, M., Tse, N., Reddel, S. W., and Phillips, W. D. (2014). Effects of the ss2-adrenoceptor agonist, albuterol, in a mouse model of anti-MuSK myasthenia gravis. PLoS One 9:e87840. doi: 10.1371/journal.pone.0087840
Giordani, L., Cuzziol, N., Del Pinto, T., Sanchez, M., Maccari, S., Massimi, A., et al. (2015). beta2-Agonist clenbuterol hinders human monocyte differentiation into dendritic cells. Exp. Cell Res. 339, 163–173. doi: 10.1016/j.yexcr.2015.10.032
Giorelli, M., Livrea, P., and Trojano, M. (2004). Post-receptorial mechanisms underlie functional disregulation of b2-adrenergic receptors in lymphocytes from Multiple Sclerosis patients. J. Neuroimmunol. 155, 143–149. doi: 10.1016/j.jneuroim.2004.05.013
Giovannoni, G. (2017). The neurodegenerative prodrome in multiple sclerosis. Lancet Neurol. 16, 413–414. doi: 10.1016/S1474-4422(17)30127-8
Grailer, J. J., Haggadone, M. D., Sarma, J. V., Zetoune, F. S., and Ward, P. A. (2014). Induction of M2 regulatory macrophages through the beta2-adrenergic receptor with protection during endotoxemia and acute lung injury. J. Innate Immun. 6, 607–618. doi: 10.1159/000358524
Grisanti, L. A., Gumpert, A. M., Traynham, C. J., Gorsky, J. E., Repas, A. A., Gao, E., et al. (2016). Leukocyte-expressed beta2-adrenergic receptors are essential for survival after acute myocardial injury. Circulation 134, 153–167. doi: 10.1161/CIRCULATIONAHA.116.022304
Harris, K. M., Lu, T., Lim, N., and Turka, L. A. (2018). Challenges and opportunities for biomarkers of clinical response to AHSCT in autoimmunity. Front. Immunol. 9:100. doi: 10.3389/fimmu.2018.00100
Hilbert, T., Bongartz, J., Weisheit, C., Knufermann, P., Baumgarten, G., Hoeft, A., et al. (2013). Beta2-adrenoceptor stimulation suppresses TLR9-dependent IFNA1 secretion in human peripheral blood mononuclear cells. PLoS One 8:e65024. doi: 10.1371/journal.pone.0065024
Hu, C., Li, J., Zhu, Y., Bai, C., Zhang, J., Xia, S., et al. (2013). Effects of Al on the splenic immune function and NE in rats. Food Chem. Toxicol. 62, 194–198. doi: 10.1016/j.fct.2013.08.038
Hudson, C. A., Mondal, T. K., Cao, L., Kasten-Jolly, J., Huber, V. C., and Lawrence, D. A. (2005). The dietary supplement ephedrine induces beta-adrenergic mediated exacerbation of systemic lupus erythematosus in NZM391 mice. Lupus 14, 293–307. doi: 10.1191/0961203305lu2078oa
Janig, W., and Green, P. G. (2014). Acute inflammation in the joint: its control by the sympathetic nervous system and by neuroendocrine systems. Auton. Neurosci. 182, 42–54. doi: 10.1016/j.autneu.2014.01.001
Jazdzewski, K., Bednarczuk, T., Stepnowska, M., Liyanarachchi, S., Suchecka-Rachon, K., Limon, J., et al. (2007). beta-2-adrenergic receptor gene polymorphism confers susceptibility to Graves disease. Int. J. Mol. Med. 19, 181–186.
Jiao, K., Niu, L. N., Li, Q. H., Ren, G. T., Zhao, C. M., Liu, Y. D., et al. (2015). beta2-Adrenergic signal transduction plays a detrimental role in subchondral bone loss of temporomandibular joint in osteoarthritis. Sci. Rep. 5:12593. doi: 10.1038/srep12593
Kavelaars, A., De Jong-De Vos Van Steenwijk, T., Kuis, W., and Heijnen, C. J. (1998). The reactivity of the cardiovascular system and immunomodulation by catecholamines in juvenile chronic arthritis. Ann. N. Y. Acad. Sci. 840, 698–704. doi: 10.1111/j.1749-6632.1998.tb09608.x
Kenney, M. J., and Ganta, C. K. (2014). Autonomic nervous system and immune system interactions. Compr. Physiol. 4, 1177–1200. doi: 10.1002/cphy.c130051
Keranen, T., Hommo, T., Moilanen, E., and Korhonen, R. (2017). beta2-receptor agonists salbutamol and terbutaline attenuated cytokine production by suppressing ERK pathway through cAMP in macrophages. Cytokine 94, 1–7. doi: 10.1016/j.cyto.2016.07.016
Khoury, S. J., Healy, B. C., Kivisäkk, P., Viglietta, V., Egorova, S., Guttmann, C. R., et al. (2010). A randomized controlled double-masked trial of albuterol add-on therapy in patients with multiple sclerosis. Arch. Neurol. 67, 1055–1061. doi: 10.1001/archneurol.2010.222
Kneilling, M., Hültner, L., Pichler, B. J., Mailhammer, R., Morawietz, L., Solomon, S., et al. (2007). Targeted mast cell silencing protects against joint destruction and angiogenesis in experimental arthritis in mice. Arthritis Rheum. 56, 1806–1816. doi: 10.1002/art.22602
Kohr, D., Singh, P., Tschernatsch, M., Kaps, M., Pouokam, E., Diener, M., et al. (2011). Autoimmunity against the beta2 adrenergic receptor and muscarinic-2 receptor in complex regional pain syndrome. Pain 152, 2690–2700. doi: 10.1016/j.pain.2011.06.012
Kolmus, K., Tavernier, J., and Gerlo, S. (2015). beta2-Adrenergic receptors in immunity and inflammation: stressing NF-kappaB. Brain Behav. Immun. 45, 297–310. doi: 10.1016/j.bbi.2014.10.007
Koopman, F. A., Stoof, S. P., Straub, R. H., Van Maanen, M. A., Vervoordeldonk, M. J., and Tak, P. P. (2011). Restoring the balance of the autonomic nervous system as an innovative approach to the treatment of rheumatoid arthritis. Mol. Med. 17, 937–948. doi: 10.2119/molmed.2011.00065
Lajevic, M. D., Suleiman, S., Cohen, R. L., and Chambers, D. A. (2011). Activation of p38 mitogen-activated protein kinase by norepinephrine in T-lineage cells. Immunology 132, 197–208. doi: 10.1111/j.1365-2567.2010.03354.x
Lamkin, D. M., Ho, H. Y., Ong, T. H., Kawanishi, C. K., Stoffers, V. L., Ahlawat, N., et al. (2016). beta-Adrenergic-stimulated macrophages: comprehensive localization in the M1-M2 spectrum. Brain Behav. Immun. 57, 338–346. doi: 10.1016/j.bbi.2016.07.162
Lantsova, V. B., Gerasimov, A. S., and Sepp, E. K. (2013). The role of ADRB2 in myasthenia: genetic and immunological factors. Bull. Exp. Biol. Med. 154, 351–353. doi: 10.1007/s10517-013-1948-0
Lee, S. H., Kwon, J. Y., Kim, S. Y., Jung, K., and Cho, M. L. (2017). Interferon-gamma regulates inflammatory cell death by targeting necroptosis in experimental autoimmune arthritis. Sci. Rep. 7:10133. doi: 10.1038/s41598-017-09767-0
Levine, J. D., Coderre, T. J., Helms, C., and Basbaum, A. I. (1988). Beta 2-adrenergic mechanisms in experimental arthritis. Proc. Natl. Acad. Sci. U.S.A. 85, 4553–4556. doi: 10.1073/pnas.85.12.4553
Liu, H., Li, W., Gu, W., Kong, Y., Yang, N., and Chen, L. (2010). Immunoregulatory effects of carvedilol on rat experimental autoimmune myocarditis. Scand. J. Immunol. 71, 38–44. doi: 10.1111/j.1365-3083.2009.02347.x
Lowin, T., and Straub, R. H. (2015). Cannabinoid-based drugs targeting CB1 and TRPV1, the sympathetic nervous system, and arthritis. Arthritis Res. Ther. 17:226. doi: 10.1186/s13075-015-0743-x
Loza, M. J., Foster, S., Peters, S. P., and Penn, R. B. (2006). Beta-agonists modulate T-cell functions via direct actions on type 1 and type 2 cells. Blood 107, 2052–2060. doi: 10.1182/blood-2005-08-3265
Lubahn, C. L., Lorton, D., Schaller, J. A., Sweeney, S. J., and Bellinger, D. L. (2014). Targeting alpha- and beta-adrenergic receptors differentially shifts Th1, Th2, and inflammatory cytokine profiles in immune organs to attenuate adjuvant arthritis. Front. Immunol. 5:346. doi: 10.3389/fimmu.2014.00346
Makhlouf, K., Comabella, M., Imitola, J., Weiner, H. L., and Khoury, S. J. (2001). Oral salbutamol decreases IL-12 in patients with secondary progressive multiple sclerosis. J. Neuroimmunol. 117, 156–165. doi: 10.1016/S0165-5728(01)00322-8
Malysheva, O., Pierer, M., Wagner, U., Wahle, M., Wagner, U., and Baerwald, C. G. (2008). Association between beta2 adrenergic receptor polymorphisms and rheumatoid arthritis in conjunction with human leukocyte antigen (HLA)-DRB1 shared epitope. Ann. Rheum. Dis. 67, 1759–1764. doi: 10.1136/ard.2007.083782
Manni, M., Granstein, R. D., and Maestroni, G. (2011). beta2-Adrenergic agonists bias TLR-2 and NOD2 activated dendritic cells towards inducing an IL-17 immune response. Cytokine 55, 380–386. doi: 10.1016/j.cyto.2011.05.013
Morand, E. F. (2018). Systemic lupus erythematosus: stress and the onset of SLE. Nat. Rev. Rheumatol. 14, 127–128. doi: 10.1038/nrrheum.2018.2
Munoz-Rodriguez, F. J., Font, J., Badia, J. R., Miret, C., Barbera, J. A., Cervera, R., et al. (1997). Shrinking lungs syndrome in systemic lupus erythematosus: improvement with inhaled beta-agonist therapy. Lupus 6, 412–414. doi: 10.1177/096120339700600413
Nacu, A., Andersen, J. B., Lisnic, V., Owe, J. F., and Gilhus, N. E. (2015). Complicating autoimmune diseases in myasthenia gravis: a review. Autoimmunity 48, 362–368. doi: 10.3109/08916934.2015.1030614
Namazi, M. R. (2004). The beneficial effect of beta2-blockers on humoral autoimmune disorders. J. Hypertens. 22, 2397–2398. doi: 10.1097/00004872-200412000-00024
Nijhuis, L. E., Olivier, B. J., Dhawan, S., Hilbers, F. W., Boon, L., Wolkers, M. C., et al. (2014). Adrenergic beta2 receptor activation stimulates anti-inflammatory properties of dendritic cells in vitro. PLoS One 9:e85086. doi: 10.1371/journal.pone.0085086
Nishii, M., Inomata, T., Niwano, H., Takehana, H., Takeuchi, I., Nakano, H., et al. (2006). β2-adrenergic agonists suppress rat autoimmune myocarditis: potential role of β2-adrenergic stimulants as new therapeutic agents for myocarditis. Circulation 114, 936–944. doi: 10.1161/CIRCULATIONAHA.105.607903
Padro, C. J., and Sanders, V. M. (2014). Neuroendocrine regulation of inflammation. Semin. Immunol. 26, 357–368. doi: 10.1016/j.smim.2014.01.003
Padro, C. J., Shawler, T. M., Gormley, M. G., and Sanders, V. M. (2013). Adrenergic regulation of IgE involves modulation of CD23 and ADAM10 expression on exosomes. J. Immunol. 191, 5383–5397. doi: 10.4049/jimmunol.1301019
Park, S., Kim, T. Y., Sim, S., Oh, H. S., Song, E., Kim, M., et al. (2017). Association of KCNJ2 genetic variants with susceptibility to thyrotoxic periodic paralysis in patients with graves’. Dis. Exp. Clin. Endocrinol. Diabetes 125, 75–78. doi: 10.1055/s-0042-119527
Pawlak, C. R., Jacobs, R., Mikeska, E., Ochsmann, S., Lombardi, M. S., Kavelaars, A., et al. (1999). Patients with systemic lupus erythematosus differ from healthy controls in their immunological response to acute psychological stress. Brain Behav. Immun. 13, 287–302. doi: 10.1006/brbi.1999.0553
Podojil, J. R., and Sanders, V. M. (2005). CD86 and beta2-adrenergic receptor stimulation regulate B-cell activity cooperatively. Trends Immunol. 26, 180–185. doi: 10.1016/j.it.2005.02.005
Pongratz, G., Anthofer, J. M., Melzer, M., Anders, S., Grassel, S., and Straub, R. H. (2014). IL-7 receptor alpha expressing B cells act proinflammatory in collagen-induced arthritis and are inhibited by sympathetic neurotransmitters. Ann. Rheum. Dis. 73, 306–312. doi: 10.1136/annrheumdis-2012-202944
Pongratz, G., and Straub, R. H. (2013). Role of peripheral nerve fibres in acute and chronic inflammation in arthritis. Nat. Rev. Rheumatol. 9, 117–126. doi: 10.1038/nrrheum.2012.181
Pongratz, G., and Straub, R. H. (2014). The sympathetic nervous response in inflammation. Arthritis Res. Ther. 16:504. doi: 10.1186/s13075-014-0504-2
Pont-Kingdon, G., Bohnsack, J., Sumner, K., Whiting, A., Clifford, B., Guthery, S. S., et al. (2009). Lack of association between beta 2-adrenergic receptor polymorphisms and juvenile idiopathic arthritis. Scand. J. Rheumatol. 38, 91–95. doi: 10.1080/03009740802541488
Qiao, G., Chen, M., Bucsek, M. J., Repasky, E. A., and Hylander, B. L. (2018). Adrenergic signaling: a targetable checkpoint limiting development of the antitumor immune response. Front. Immunol. 9:164. doi: 10.3389/fimmu.2018.00164
Ross, A. M., Lee, C. S., Lutsep, H., and Clark, W. M. (2018). The Influence of beta-adrenergic receptor kinase-1 on stroke-induced immunodeficiency syndrome. J. Cardiovasc. Nurs. 33, E3–E10. doi: 10.1097/JCN.0000000000000481
Sanders, V. M. (2012). The beta2-adrenergic receptor on T and B lymphocytes: do we understand it yet? Brain Behav. Immun. 26, 195–200. doi: 10.1016/j.bbi.2011.08.001
Scanzano, A., and Cosentino, M. (2015). Adrenergic regulation of innate immunity: a review. Front. Pharmacol. 6:171. doi: 10.3389/fphar.2015.00171
Sergienko, E., Xu, J., Liu, W. H., Dahl, R., Critton, D. A., Su, Y., et al. (2012). Inhibition of hematopoietic protein tyrosine phosphatase augments and prolongs ERK1/2 and p38 activation. ACS Chem. Biol. 7, 367–377. doi: 10.1021/cb2004274
Sharma, M., Patterson, L., Chapman, E., and Flood, P. M. (2017). Salmeterol, a long-acting beta2-adrenergic receptor agonist, inhibits macrophage activation by lipopolysaccharide from Porphyromonas gingivalis. J. Periodontol. 88, 681–692. doi: 10.1902/jop.2017.160464
Simkins, T., Crawford, R. B., Goudreau, J. L., Lookingland, K. J., and Kaplan, B. L. (2014). Enhanced humoral immunity in mice lacking CB1 and CB2 receptors (Cnr1-/-/Cnr2-/- mice) is not due to increased splenic noradrenergic neuronal activity. J. Neuroimmune Pharmacol. 9, 544–557. doi: 10.1007/s11481-014-9549-x
Slota, C., Shi, A., Chen, G., Bevans, M., and Weng, N. P. (2015). Norepinephrine preferentially modulates memory CD8 T cell function inducing inflammatory cytokine production and reducing proliferation in response to activation. Brain Behav. Immun. 46, 168–179. doi: 10.1016/j.bbi.2015.01.015
Soliven, B., Rezania, K., Gundogdu, B., Harding-Clay, B., Oger, J., and Arnason, B. G. (2009). Terbutaline in myasthenia gravis: a pilot study. J. Neurol. Sci. 277, 150–154. doi: 10.1016/j.jns.2008.09.033
Sugaya, K., Nishijima, S., Yamada, T., Miyazato, M., Hatano, T., and Ogawa, Y. (2002). Molecular analysis of adrenergic receptor genes and interleukin-4/interleukin-4 receptor genes in patients with interstitial cystitis. J. Urol. 168, 2668–2671. doi: 10.1016/S0022-5347(05)64241-3
Takenaka, M. C., Araujo, L. P., Maricato, J. T., Nascimento, V. M., Guereschi, M. G., Rezende, R. M., et al. (2016). Norepinephrine controls effector T cell differentiation through beta2-adrenergic receptor-mediated inhibition of Nf-kappab and AP-1 in dendritic cells. J. Immunol. 196, 637–644. doi: 10.4049/jimmunol.1501206
Tandale, A., Joshi, M., and Sengupta, D. (2016). Structural insights and functional implications of inter-individual variability in beta2-adrenergic receptor. Sci. Rep. 6:24379. doi: 10.1038/srep24379
Tracey, K. J. (2014). Lymphocyte called home: beta2-adreneric neurotransmission confines T cells to lymph nodes to suppress inflammation. J. Exp. Med. 211, 2483–2484. doi: 10.1084/jem.21113insight3
Tsai, C. P., Lin, F. C., and Lee, C. T. (2014). Beta2-adrenergic agonist use and the risk of multiple sclerosis: a total population-based case-control study. Mult. Scler. 20, 1593–1601. doi: 10.1177/1352458514528758
Ulloa, L., Quiroz-Gonzalez, S., and Torres-Rosas, R. (2017). Nerve stimulation: immunomodulation and control of inflammation. Trends Mol. Med. 23, 1103–1120. doi: 10.1016/j.molmed.2017.10.006
Wahle, M., Kolker, S., Krause, A., Burmester, G. R., and Baerwald, C. G. (2001). Impaired catecholaminergic signalling of B lymphocytes in patients with chronic rheumatic diseases. Ann. Rheum. Dis. 60, 505–510. doi: 10.1136/ard.60.5.505
Wahle, M., Krause, A., Pierer, M., Hantzschel, H., and Baerwald, C. G. (2002). Immunopathogenesis of rheumatic diseases in the context of neuroendocrine interactions. Ann. N. Y. Acad. Sci. 966, 355–364. doi: 10.1111/j.1749-6632.2002.tb04235.x
Wang, L., Zhang, Y., and He, M. (2017). beta2-Adrenergic receptor gene polymorphisms in the relapse of myasthenia gravis with thymus abnormality. Int. J. Neurosci. 127, 291–298. doi: 10.1080/00207454.2016.1202952
Wei, W. (2016). Soft regulation of inflammatory immune response. Chin. Pharmacol. Bull. 32, 297–303.
Weissert, R. (2017). Adaptive immunity is the key to the understanding of autoimmune and paraneoplastic inflammatory central nervous system disorders. Front. Immunol. 8:336. doi: 10.3389/fimmu.2017.00336
Woo, A. Y., Jozwiak, K., Toll, L., Tanga, M. J., Kozocas, J. A., Jimenez, L., et al. (2014). Tyrosine 308 is necessary for ligand-directed Gs protein-biased signaling of beta2-adrenoceptor. J. Biol. Chem. 289, 19351–19363. doi: 10.1074/jbc.M114.558882
Wu, H., Chen, J., Song, S., Yuan, P., Liu, L., Zhang, Y., et al. (2016). beta2-adrenoceptor signaling reduction in dendritic cells is involved in the inflammatory response in adjuvant-induced arthritic rats. Sci. Rep. 6:24548. doi: 10.1038/srep24548
Xu, B. Y., Arlehag, L., Rantapaa-Dahlquist, S. B., and Lefvert, A. K. (2005). beta2 Adrenoceptor gene single nucleotide polymorphisms are associated with rheumatoid arthritis in northern Sweden. Ann. Rheum. Dis. 64, 773–776. doi: 10.1136/ard.2004.027532
Zalli, A., Bosch, J. A., Goodyear, O., Riddell, N., Mcgettrick, H. M., Moss, P., et al. (2015). Targeting ss2 adrenergic receptors regulate human T cell function directly and indirectly. Brain Behav. Immun. 45, 211–218. doi: 10.1016/j.bbi.2014.12.001
Zhang, Z. W., Qin, X. Y., Che, F. Y., Xie, G., Shen, L., and Bai, Y. Y. (2015). Effects of beta 2 adrenergic agonists on axonal injury and mitochondrial metabolism in experimental autoimmune encephalomyelitis rats. Genet. Mol. Res. 14, 13572–13581. doi: 10.4238/2015.October.28.17
Zhao, W., Tong, T., Wang, L., Li, P. P., Chang, Y., Zhang, L. L., et al. (2011). Chicken type II collagen induced immune tolerance of mesenteric lymph node lymphocytes by enhancing beta2-adrenergic receptor desensitization in rats with collagen-induced arthritis. Int. Immunopharmacol. 11, 12–18. doi: 10.1016/j.intimp.2010.09.018
Keywords: autoimmune diseases, β2-adrenergic receptor, single nucleotide polymorphisms, immune response, soft regulation
Citation: Wu L, Tai Y, Hu S, Zhang M, Wang R, Zhou W, Tao J, Han Y, Wang Q and Wei W (2018) Bidirectional Role of β2-Adrenergic Receptor in Autoimmune Diseases. Front. Pharmacol. 9:1313. doi: 10.3389/fphar.2018.01313
Received: 29 June 2018; Accepted: 29 October 2018;
Published: 27 November 2018.
Edited by:
Stefania Tacconelli, Università degli Studi G. d’Annunzio Chieti e Pescara, ItalyReviewed by:
Astrid Parenti, Università degli Studi di Firenze, ItalySatish Ramalingam, SRM Institute of Science and Technology, India
Jasenka Zubcevic, University of Florida, United States
Copyright © 2018 Wu, Tai, Hu, Zhang, Wang, Zhou, Tao, Han, Wang and Wei. This is an open-access article distributed under the terms of the Creative Commons Attribution License (CC BY). The use, distribution or reproduction in other forums is permitted, provided the original author(s) and the copyright owner(s) are credited and that the original publication in this journal is cited, in accordance with accepted academic practice. No use, distribution or reproduction is permitted which does not comply with these terms.
*Correspondence: Qingtong Wang, aGZ3cXQ3MjdAMTYzLmNvbQ== Wei Wei, d3dlaUBhaG11LmVkdS5jbg==