- 1School of Pharmaceutical Sciences, University of São Paulo, São Paulo, Brazil
- 2Bioinformatics and Pharmacogenetics Laboratory, METOSMOD Research Group, School of Pharmacy and Biochemistry, Universidad Nacional Mayor de San Marcos, Lima, Peru
- 3Nephrology Division, Hospital do Rim, Federal University of São Paulo, São Paulo, Brazil
- 4Department of Basic Sciences, Center of Excellence in Translational Medicine, BIOREN, Universidad de La Frontera, Temuco, Chile
Monitoring of immunosuppressive drugs, such as calcineurin and mTOR inhibitors, is essential to avoid undesirable kidney transplant outcomes. Polymorphisms in pharmacokinetics-related genes have been associated with variability in blood levels of immunosuppressive drugs and adverse effects, but influence of pharmacodynamics-related genes remains to be elucidated. The influence of polymorphisms in genes of the mTOR and calcineurin signaling pathways on long-term clinical outcomes was investigated in Brazilian kidney transplant recipients within the 1-year post-transplant. Two-hundred and sixty-nine kidney transplant recipients were enrolled at a kidney transplant center in São Paulo city, Brazil, and treated with tacrolimus plus everolimus or mycophenolate sodium (clinical trial NCT01354301). Clinical and laboratory data, including renal function parameters and drug blood levels were recorded. Genomic DNA was extracted from blood samples. Polymorphisms in MTOR rs1057079 (c.4731G>A), rs1135172 (c.1437T>C), and rs1064261 (c.2997C>T); PPP3CA rs3730251 (c.249G>A); FKBP1A rs6033557 (n.259+24936T>C); FKBP2 rs2159370 (c.-2110G>T); and FOXP3 rs3761548 (c.-23+2882A>C) and rs2232365 (c.-22-902A>G) were analyzed by real-time PCR. Frequencies of gene polymorphisms did not differ among the treatment groups. Analysis of primary outcomes showed that patients carrying MTOR c.1437CC and FOXP3 c.-23+2882CC genotypes had higher serum creatinine than non-carriers (p < 0.05) at 1-year post-transplant. MTOR c.4731G allele (AG+GG genotype) was associated with increased risk for acute rejection (OR = 3.53, 95% CI = 1.09–11.48, p = 0.037). Moreover, 1-year cumulative incidence of rejection was higher in MTOR c.4731G allele carriers compared to AA genotype carriers (p = 0.027). Individually, analysis of secondary outcomes revealed that FKBP2 c.-2110GG genotype carriers had higher risk of leukopenia, FKBP1A n.259+24936C allele carriers had increased risk of constipation, and FOXP3 c.-22-902A or c.-23+2882A allele had higher risk of gastrointestinal disorders (p < 0.05). However, these results were not maintained in the multivariable analysis after p-value adjustment. In conclusion, variants in genes of mTOR and calcineurin pathways are associated with long-term impaired renal function, increased risk of acute rejection, and, individually, with adverse events in Brazilian kidney transplant recipients.
Introduction
The calcineurin inhibitor (CNI), such as tacrolimus (TAC) and mTOR inhibitor (mTORi), such as everolimus (EVR) are immunosuppressive drugs helpful to prevent allograft rejection and simultaneously improve graft and patient survival in kidney transplantation (Lim et al., 2017).
TAC and EVR binding to their cytoplasmic protein receptor, the FK506-binding protein 12 (FKBP12). The TAC-FKBP12 complex interacts with calcineurin while the EVR-FKBP12 targets mTOR. These complexes lead to inhibition of T cell activation and proliferation, besides other important implications in alloimmune responses (Tong and Jiang, 2015; Bergan et al., 2016).
The therapeutic monitoring of these immunosuppressants is widely accepted because there is high between-patient variability in their pharmacokinetics and concentration–effect relationship (van Gelder, 2014). In this way, in addition to biodemographic and clinical factors, genetics play an important role. The key genetic variants involved in the metabolism and distribution of the immunosuppressive drugs have been largely investigated (Picard et al., 2016; Zhang et al., 2018). On the contrary, those involved with drug targets and molecular signaling pathway remain poorly known in solid organ transplantation.
In kidney transplant recipients on CNI-based immunosuppression, some polymorphisms in three calcineurin subunit genes (PPP3CA, PPP3CB, and PPP3R1) were not associated with renal function and incidence of rejection or adverse events (Moes et al., 2016; Pouché et al., 2016a; Salgado et al., 2017).
Five variants in MTOR (rs1770345, rs2300095, rs2076655, rs1883965, and rs12732063) were investigated in kidney transplant recipients switched from CNI to mTORi. The AGAAA haplotype was associated with a slightly decreasing in hemoglobin levels (Woillard et al., 2012), but it seems that it was due to the physiological mechanism rather than pharmacogenetic (Pouché et al., 2016b).
This study investigated the influence of polymorphisms in MTOR, PPP3CA, FKBP1A (FKBP12), FKBP2, and FOXP3 on long-term clinical outcomes (renal function, acute rejection and adverse events) of kidney recipients treated with TAC and EVR-based immunosuppressive therapy. FKBP2 encodes the FKBP13 that is closely related to FKBP12 (Tong and Jiang, 2015). FOXP3 is a key transcriptional factor of the regulatory T cells (Tregs), which are important in suppressing alloimmune responses and maintenance of transplantation tolerance (Dummer et al., 2012).
Materials and Methods
Study Population
This pharmacogenetic study was carried out in a sample of kidney transplant recipients previously enrolled in the clinical trial registered as NCT01354301 at the US Clinical Trials database (Tedesco-Silva et al., 2015). The study was approved by the Ethics Committee of the UNIFESP (Protocol # 0339/11) and performed according to the international regulations in Good Clinical Practices and to the ethical principles of the Declaration of Helsinki. All patients provided written informed consent prior to enrollment.
The aforementioned trial included low/moderate-immunological risk adult recipients indicated for first ABO-compatible kidney transplantation, from either alive or deceased donor, of which, the 269 patients who completed the study were selected. Exclusion criteria considered kidneys from HLA identical or expanded criteria deceased donors, positive cytotoxic cross match or panel reactive antibody equal to or above 50%, either class I or class II. Use of contraceptives methods during the trial were requested for women of childbearing potential (Tedesco-Silva et al., 2015).
Immunosuppressive Regimens
The patients were randomized in three study groups of immunossupressive treatment, as follows: (i) Group TAC5/EVR: single dose of anti-thymocyte globulin at first day post-transplant, TAC 0.05 mg/kg b.i.d. and EVR 1.5 mg daily b.i.d.; (ii) TAC10/EVR: basiliximab induction on days 0 and 4. On day 1, TAC 0.1 mg/kg b.i.d. and EVR 1.5 mg b.i.d.; (iii) TAC10/MPS: basiliximab induction on days 0 and 4. On day 1, TAC 0.1 mg/kg b.i.d. and MPS 720-mg b.i.d. Before transplantation, all patients were treated with 1 g of methylprednisolone, and began receiving on day 1 post-transplant 0.5 mg/kg/day oral prednisone (not exceeding a 30 mg dose) tapered to 5 mg/day dose by day 45.
Clinical Characteristics and Laboratory Data
Clinical characteristics and laboratory data of the kidney recipients were recorded pre- and post-transplant). Clinical characteristics included cause of CKD, dialysis procedure, maintenance hemodialysis, cold ischemia time, graft loss and DGF. Laboratory data consisted of serum creatinine, eGFR, glucose, insulin, total cholesterol, HDL and LDL cholesterol, triglycerides, hemoglobin, erythrocytes and leukocyte counts, urinary proteins, immunodiagnostic test for CMV, kidney graft biopsies. eGFR was estimated by MDRD formula (Levey et al., 2009).
Immunosuppressive Drug Monitoring
TAC and EVR blood concentration, the prescribed doses and adjusted concentration for dose administered (Co/D) were recorded. Blood concentrations were analyzed by chemoluminiscent microparticle immunoassay (Abbott Diagnostics, Lake Forest, IL, United States) and liquid chromatography-tandem mass spectrometry, respectively.
Clinical Outcomes
Primary clinical outcomes were considered serum creatinine, eGFR, cellular and humoral acute kidney rejection, and CMV events, including asymptomatic (infection) or symptomatic (disease) viremia (Kotton et al., 2013).
Secondary clinical outcomes were considered the adverse events that were classified according to the Medical Dictionary for Regulatory activities (Medical Dictionary for Regulatory Activities [MedDRA], 2016), as: blood and lymphatic system disorders, gastrointestinal disorders, general disorders and administration site conditions, metabolism and nutrition disorders, and renal and urinary disorders. Recurrence of adverse event was not considered in the analysis of the secondary outcomes.
Genomic DNA Extraction
Genomic DNA was isolated from peripheral blood sample using the QIAamp® DNA Blood Mini Kit (Qiagen Inc., Valencia, CA, United States) on the QIAcube system (Qiagen Sciences Inc., Germantown, MD, United States). DNA quantification and purity were evaluated by 260/280 nm spectrophotometry using NanoDrop ND-1000 (NanoDrop Technologies Inc., Wilmington, NC, United States) and DNA integrity was evaluated by 1.0% agarose gel electrophoresis. Samples were stored at −20°C.
Gene Polymorphisms Selection and Analysis
Gene polymorphisms [MTOR rs1057079 (c.4731G>A, p.Ala1577=), rs1135172 (c.1437T>C, p.Asp479=) and rs1064261 (c.2997C>T, p.Asn999=); PPP3CA rs3730251 (c.249G>A, p.Ala83=); FKBP1A rs6033557 (n. 259+24936T>C); FKBP2 rs2159370 (c.-2110G>T); and FOXP3 rs2232365 (c.-22-902A>G), and rs3761548 (c.-23+2882A>C)] were selected using a two-step approach based on pathway-gene method, as described elsewhere (Kooloos et al., 2009).
Genotyping was performed using the following pre-designed TaqMan SNP genotyping assays (Thermo Fisher Scientific Inc., Foster City, CA, United States): C_8862305_1 (rs1057079), C_8862346_10 (rs1135172), C_28027381_10 (rs1064261), C_25618069_10 (rs3730251), C__30149598_20 (rs6033557), C___2981262_20 (rs2159370), C__15942641_10 (rs2232365), C__27476877_10 (rs3761548). The PCR mixture contained 1X TaqMan genotyping master mix, 1X TaqMan SNP genotyping assay and 40 ng of template DNA. The real-time PCR assays were carried out according to the manufacturer’s instructions using an ABI 7500 FAST system (Applied Biosystems, Foster City, CA, United States). Genotype calling was performed using 7500 SDS software (Thermo Fisher Scientific Inc., Foster City, CA, United States). To assess the accuracy of the genotype calling, 20% of the DNA samples were randomly genotyped twice.
Statistical Analysis
Continuous variables are expressed as median and interquartile range and compared by Mann–Whitney or Kruskal–Wallis test and Bonferroni’s correction test for multiple comparisons. Categorical variables are expressed as percentage and number of individuals in parenthesis, and compared by chi-square test or likelihood ratio test (for number of individuals less than 5).
Allele and genotype frequencies were estimated by counting. Hardy–Weinberg equilibrium (HWE) was estimated using Haploview program (Barrett et al., 2005). For polymorphisms located at X chromosome HWE was estimated considering only female individuals in order to avoid hemizygous male genotypes.
The influence of gene polymorphisms on clinical outcomes was studied using dominant, co-dominant and recessive inheritance models. The dominant model, in which the presence of at least one minor allele has an effect on the phenotype or clinical outcome, provides the highest power values of the statistical tests (Hong and Park, 2012). Therefore, homozygous and heterozygous patients carrying the minor allele were analyzed together.
Cumulative incidence of acute rejection episodes (time-dependent variable) during the follow-up was analyzed by Kaplan–Meier survival analysis, and comparisons between major and minor allele carriers were performed using the log-rank test.
Univariable logistic regression analysis was used to assess the relationship between gene variants and primary and secondary clinical outcomes. Continuous variables such as eGFR and serum creatinine were divided by tertiles to be used as categorical variables in the logistic regression analysis. The combined effect of covariates and SNP was investigated using multivariable logistic regression analysis. The covariates considered in the models were age, weight, gender, donor type, time on hemodialysis, DGF time, cold ischemia time, acute rejection, proteinuria, immunosuppressive therapy group and CMV. Only covariates with p-value lower than 0.15 in univariable logistic regression analysis were included in the multivariable logistic regression (Heinze and Dunkler, 2017). To account for multiple hypothesis testing in model with more than two covariates, adjustment of p-values was performed using Bonferroni–Holm sequential correction method.
Association analysis of MTOR polymorphisms with primary and secondary outcomes was carried out with data from groups treated with EVR (TAC5/EVR and TAC10/EVR), which is the mTORi in the immunosuppressive therapy.
The statistical analyses were performed using the SPSS for windows (SPSS Inc., Chicago, IL, United States), GraphPad Prism (GraphPad Software Inc., La Jolla, CA, United States) and SigmaStat (Systat Software Inc., San Jose, CA, United States). The p-value threshold for significance was 0.05.
Results
Characteristics of the Study Population
Biodemographic and clinical characteristics of the kidney transplant recipients are shown in Table 1. Median age and weight of the kidney recipients were 44.0 [34.0–56.0] years and 68.3 [59.0–77.9] kg, respectively. The studied group had mainly men (66.2%) and Caucasians (51.7%). Other self-reported ethnicities were African (14.9%) and intermediate/mixed (33.4%). Causes of CKD were glomerulonephritis (12.3%), hypertension (10.0%) and diabetes (10.0%). Hemodialysis was the most frequent dialysis procedure (93.3%) lasting a median time of 33.0 [16.0–54.0] months. Grafts were exposed to cold ischemia for 20.3 [17.6–23.0] h. DGF was observed in 33.8% of the kidney recipients, and it lasted 10.0 [6.0–15.0] days. Kidney grafts were mainly obtained from deceased donors (69.1%). The proportion of these variables did not differ among the therapy groups, except for graft loss which was higher in the TAC10/MPS group (7.5%) compared to TAC5/EVR (1.3%) and TAC10/EVR (1.0%) (p = 0.026).
Drug monitoring data of the kidney recipients at 1-year post-transplant is shown in Supplementary Table 1. TAC concentration was higher in TAC10/MPS group compared to TAC5/EVR and TAC10/EVR groups (<0.05), while TAC Co/Do were similar among the therapy groups. EVR monitoring data did not differ between the TAC5/EVR and TAC10/EVR groups (p > 0.05).
Clinical Outcomes
Primary and secondary kidney outcomes at 1-year post-transplant are shown in Table 2. In the total group, serum creatinine and eGFR were 1.3 [1.1–1.6] mg/dL and 63.5 [49.7–78.2] ml/min/1.73 m2, respectively. TAC10/EVR group had higher serum creatinine and lower eGFR compared to TAC10/MPS group (p < 0.05). Acute rejection occurred in 14.9% of the patients, mainly T cell-mediated episodes (13.4%), but it did not differ among the treatment groups (p = 0.198).
Analysis of the secondary outcomes showed that the most common blood and lymphatic system disorders were anemia (8.2%) and leucopenia (5.2%). Leukopenia was more frequent in the TAC10/MPS group (9.7%) than the TAC5/EVR (5.0%) and TAC10/EVR (1.0%) groups (p = 0.018) (Table 2). Gastrointestinal disorders were mainly constipation (12.6%), diarrhea (25.7%), dyspeptic disorder (8.6%), epigastric pain (12.3%) and nausea and/or vomiting (9.7%), but frequencies of these events were similar among the therapy groups (p. > 0.05). Edema was the most common general disorder (41.3%) with the higher proportion in TAC5/EVR (45.0%) and TAC10/EVR (47.9%) groups than in the TAC10/MPS (31.2%) (p = 0.047). CMV infection and/or disease was detected in 18.2% of the total group, but it was more frequent in TAC10/MPS group (37.6%) compared with the other groups (p < 0.001). Hyperglycemia (17.8%) and post-transplant diabetes (10.4%) were the common metabolism and nutritional disorders. Proteinuria, a renal and urinary disorder, was detected in 8.9% of the total kidney recipients.
Gene Polymorphisms and Primary Clinical Outcomes
Frequencies of gene polymorphisms in the sample population are summarized in Table 3. Genotypes and minor allele frequencies of the SNP were similar among the study groups (p > 0.05). Further, no deviation from HWE was observed (data not shown). These results are suggestive that patients were randomly distributed in the therapy groups.
Median values of serum creatinine at 1-year post-transplant were higher in subjects carrying MTOR c.1437T>C CC genotype and FOXP3 c.-23+2882A>C CC genotype (Figure 1). The association of gene polymorphisms with the pre-transplant levels of serum creatinine was further investigated, and no differences were found between carriers of the different genotypes of MTOR (Supplementary Figure 1). However, individuals carrying the FOXP3 c.-23+2882CC genotype had higher values of pre-transplant serum creatinine than A allele (genotype CA+AA) carriers (Supplementary Figure 2). The studied polymorphisms did not influence eGFR values (data not shown). We also investigated the influence of MTOR c.1437T>C and FOXP3 c.-23+2882A>C variants on TAC blood concentrations at month 12 but no significant differences were found between genotype carriers (p > 0.05) (data not shown). This result is suggestive that immunosuppressant concentration did not influence in the association between genetic variants and serum creatinine.
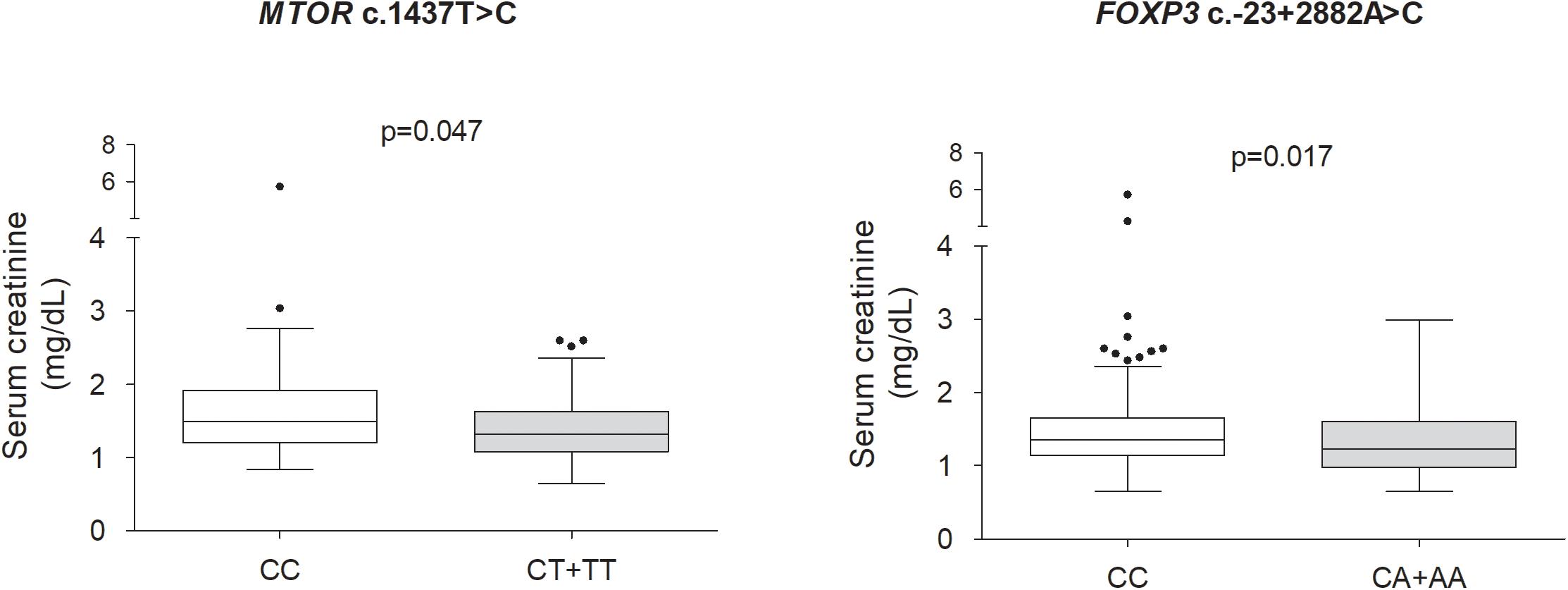
FIGURE 1. Influence of MTOR and FOXP3 variants on serum creatinine in kidney recipients at 1-year post-transplant. Data are shown as median and interquartile range, and were compared by Mann–Whitney test. Comparisons between MTOR c.1437T>C genotypes were carried with data from TAC5/EVR and TAC10/EVR groups.
Univariable logistic regression showed that FOXP3 c.-23+2882CC genotype was associated with increased serum creatinine at 1-year post-transplant (OR = 1.75, 95% CI = 1.07–2.86, p = 0.025) (Supplementary Table 2). Patient weight and gender and acute rejection were also variables associated with increased risk for high serum creatinine (p < 0.05), independently of the groups therapy tested (models 1 and 2) (Supplementary Table 3).
Multivariable regression analysis revealed that male gender remained a risk factor for high serum creatinine at 1-year post-transplant using both model 1 (TAC5/EVR and TAC10/EVR) and model 2 (all therapy groups) (adjusted p < 0.05), while acute rejection was confirmed only using the model 2 (adjusted p = 0.024) (Table 4).
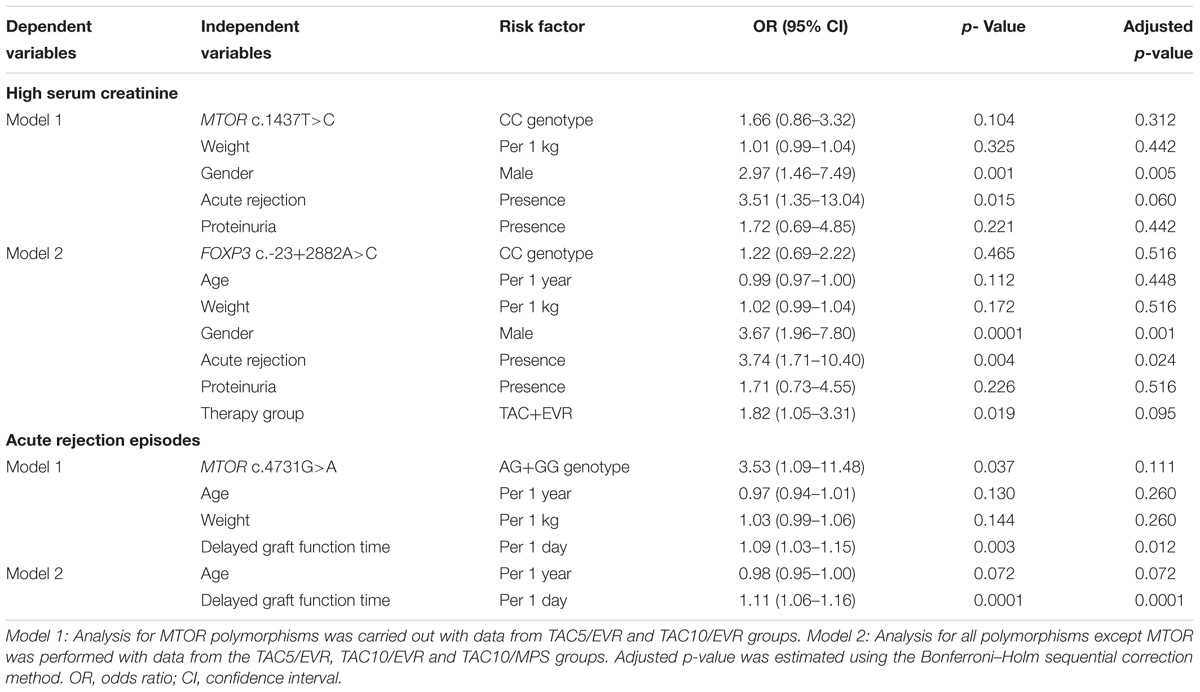
TABLE 4. Variables associated with primary outcomes in kidney recipients at 1-year post-transplant: Multivariable logistic regression analysis.
Association of genetic and non-genetic variables associated with acute rejection within 1-year post-transplant was also tested by univariate regression analysis (Supplementary Table 4). MTOR c.4731G allele (AG+GG genotype) carriers had increased risk for acute rejection (OR = 3.37, 95% CI = 1.10–10.30, p = 0.037). Moreover, patients with increased DGF time have higher risk of acute rejection using both model 1 (p = 0.001) and model 2 (p = 0.0001).
Multivariable regression analysis showed that MTOR c.4731G allele remained associated with acute rejection (p = 0.037), but not after Bonferroni–Holm correction (adjusted p = 0.111), whereas increased DGF time was confirmed as a risk factor for rejection (adjusted p < 0.05) (Table 4).
The influence of MTOR c.4731G>A on 1-year cumulative incidence of acute rejection was further investigated using the Kaplan–Meier survival analysis. Patients carrying the G allele (AG+GG genotype) had higher cumulative incidence of acute rejection (18.6%) compared to AA genotype carriers (6.3%; log-rank p < 0.027) (Figure 2).
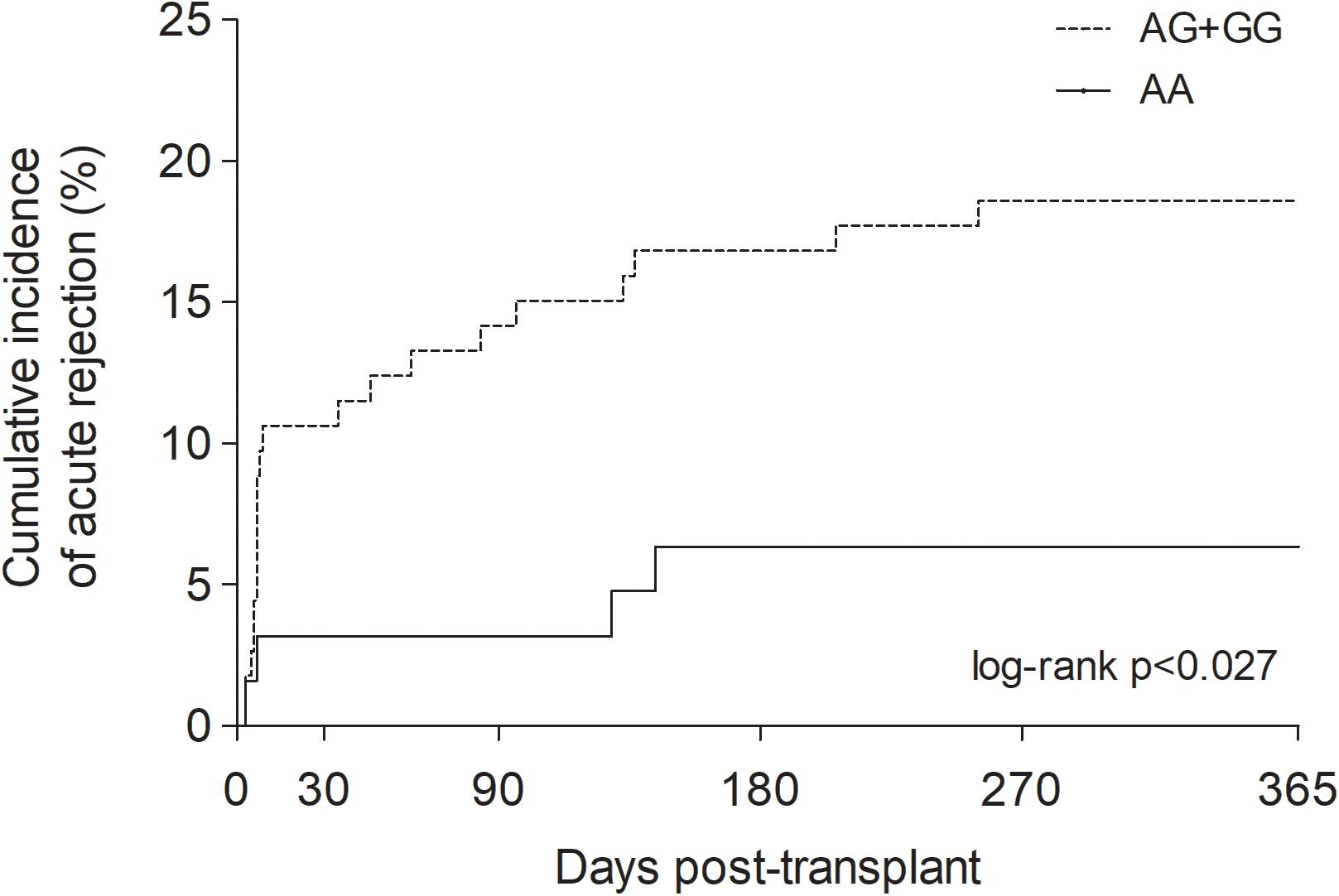
FIGURE 2. One-year cumulative incidence of acute rejection in kidney recipients stratified by MTOR c.4731G>A genotypes. Log-rank p-value was estimated by Kaplan–Meier survival analysis.
Gene Polymorphisms and Secondary Clinical Outcomes
The results of the univariable regression analysis for gene polymorphism and other variables associated with secondary clinical outcomes within 1-year post-transplant of kidney recipients are shown in Supplementary Table 5. FKBP2 c.-2110GG genotype (OR = 3.87, 95% CI = 1.26–11.91, p = 0.018), therapy group (TAC+MPS; OR = 3.66, 95% CI = 1.19–11.28, p = 0.024) and CMV (OR = 9.68, 95% CI = 3.08–30.38, p = 0.0001) were associated with leukopenia (p < 0.05). FKBP1A n.259+24936C allele (TC+CC genotype) was associated with constipation (OR = 2.40, 95% CI = 1.08–5.37, p = 0.033). FOXP3 c.-22-902A allele (GA+AA genotype) was associated with epigastric pain (OR = 2.15, 95% CI = 1.01–4.56, p = 0.047) and FOXP3 c.-23+2882A allele (CA+AA genotype) was associated with nausea and/or vomiting episodes (OR = 2.38, 95% CI = 1.05–5.38, p = 0.038).
Multivariable regression analysis showed that FKBP2 c.-2110GG genotype did not remained associated with leukopenia using the model 1 (adjusted p = 0.085) (Table 5). The FKBP1A and FOXP3 variants were associated, respectively, with constipation and epigastric pain using the model 2 (p < 0.05), but these results were not confirmed after Bonferroni–Holm correction (adjusted p > 0.05).
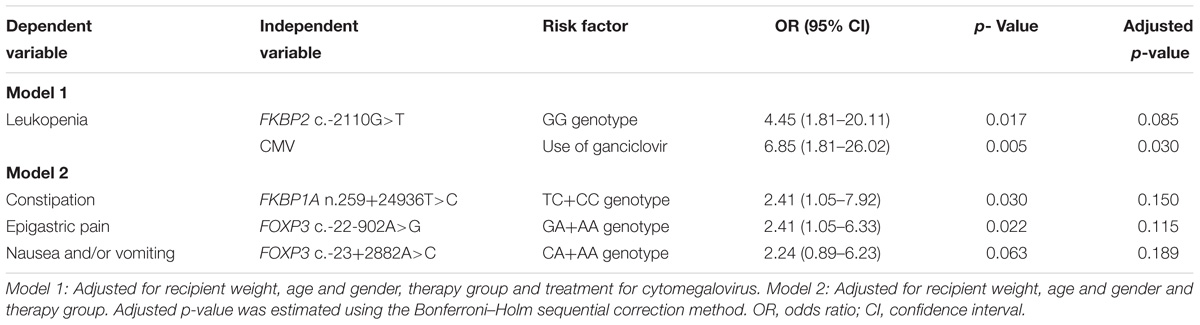
TABLE 5. Variables associated with secondary outcomes in kidney recipients at 1-year post-transplant: Multivariable logistic regression analysis.
Discussion
This study investigated the influence of polymorphisms in genes of the mTOR and calcineurin signaling pathway on long-term clinical outcomes in kidney recipients treated with TAC and EVR-based immunosuppressive therapy. Factors related to long-term acute rejection and adverse events were also investigated. Pre-transplant clinical and laboratory characteristics of the kidney recipients were similar among the immunosuppressive therapy groups, as it was previously reported (Tedesco-Silva et al., 2015). Interestingly the TAC10/EVR group had lower eGFR and higher serum creatinine than TAC10/MPS at 1-year post-transplant.
A meta-analysis examined long-term renal outcomes comparing different immunosuppressant regimens. The authors observed that cohorts using combination of non-reduced doses of CNI and mycophenolic acid, as compared with mTORi group, showed higher creatinine clearance and lower serum creatinine values (Xie et al., 2015). Therefore, in agreement to our results, the combination of traditional CNI regimens and MPS might lead to better renal function. In a large Brazilian single-center study, after 1 year, no differences in eGFR values were found between de novo use of mTORi or antimetabolites, such as mycophenolic acid, in combination with CNIs (de Paula et al., 2016), but a higher proportion of living donors could have influenced this result.
In this study, MTOR c.1437T>C (CC genotype) was associated with higher serum creatinine, but this variant did not influence eGFR at 1-year post-transplant. No previous study evaluated the relationship between MTOR variants and renal outcomes in kidney recipients. MTOR c.1437T>C (p.Asp479=) does not cause a functional disruption in the mTOR protein, which could be related to a worst renal outcome. It is conceivable that this variant is in linkage disequilibrium with a pathogenic variant within MTOR.
MTOR c.1437T>C was reported to be linked to the MTOR rs2295080 (c.-141C>A), a promoter variant, of which GT genotype was previously associated with less risk for prostate cancer (Liu et al., 2017). Further in vitro experiments with luciferase reporter in normal or cancer gastric cell lines (GES-1, BGC823, MGC803, and SGC-7901) revealed a higher transcription activity in the presence of the T allele of rs2295080 (Xu et al., 2013).
It is likely that the C allele of MTOR c.1437T>C is correlated with the T allele of MTOR rs2295080 (c.-141C>A), and the higher mTOR transcriptional levels might lead to a worst renal outcome due to insufficient immunosuppressive activity of EVR.
Carriers of the G allele of MTOR c.4731G>A (genotype AG+GG) were found to present higher incidence of acute rejection episodes during the first-year post-transplant. Regression analysis showed that G allele of the MTOR c.4731G>A polymorphism was associated with increased risk for long-term acute rejection in kidney transplants. This variant, which is in linkage disequilibrium with MTOR c.1787-116A>G, was previously reported to be associated with high risk for colon cancer in a sample from United States (Slattery et al., 2010). Also, the MTOR c.1787-116AA genotype was associated with reduced MTOR mRNA expression in healthy colon tissue (Slattery et al., 2014).
These evidences are suggestive that the subjects carrying the MTOR c.4731G allele may have lower MTOR transcript levels, which could be associated with increased incidence of acute rejection. Besides, the MTOR variants, mainly MTOR c.1787-116A>G (rs2024627), were recommended as genetic markers of pharmacogenetics of kidney transplant (Pouché et al., 2016b). Finally, patient variability within the efficacy kidney outcomes may rely on the MTOR variants yet to be studied.
Alloantigens are immunomodulatory molecules that stimulate T cell receptor (Ingulli, 2010); upon its activation, mTOR is activated, which in turn regulates T cell fate (Chi, 2012). Immunosuppressant agents targeting mTOR as EVR inhibit the transduction of interleukin-2 stimulus in T cells causing a cell-cycle arrest (Ma et al., 2018). On those alloactivated T cells, mTOR inhibitors might reduce the immune response toward the graft, avoiding acute rejection episodes through the reduction of mTOR levels.
mTOR signaling pathway also exerts a role regulating the differentiation of regulatory and effectors T cells (Zeng and Chi, 2017), and its activation led to the proliferation and suppressive activity of Tregs. In this way, inactivation of the mTOR pathway could also led to a lower activity of Tregs, and subsequent autoimmunity problems (Zeng et al., 2013). In agreement to our hypothesis, the lower expression of mTOR could turn the graft more vulnerable to immune responses, and subsequent acute rejection episodes. Therefore, in spite of mechanistic explanations for acute rejection and MTOR variants, the relevance of polymorphisms in the mTOR signaling pathway is pointed out by our study.
MTOR variants were not associated with long-term adverse events in this work, in spite of a previous study reported a relationship of other MTOR polymorphisms with low hemoglobin levels in kidney recipients treated with sirolimus-based immunosuppressive therapy (Woillard et al., 2012).
In this study, the CC genotype of the FOXP3 c.-23+2882A>C was associated with higher serum creatinine pre- and post-transplant in kidney recipients. It is likely that FOXP3 c.-23+2882CC genotype may account for a worse renal function. On the other hand, a previous study showed that individuals carrying the CC genotype had increased eGFR values and TAC blood levels in kidney recipients at 21 days post-transplant (Ge et al., 2016). These results are suggestive that the influence of this FOXP3 variant on renal function may depend on the time of exposure to TAC.
Additional analysis used an additive model, which grouped male hemizygous genotypes with each one of the corresponding female homozygous genotypes. Our results indicated that females carrying the CA genotype, but not CC/C, of the FOXP3 c.-23+2882A>C had a higher risk of serum creatinine compared to the individuals carrying the AA/A genotypes/alleles (data not shown). Subgroup analysis in small samples could lower statistical power. Besides, disparity in the frequency distribution of the female heterozygotes could also have influenced this result.
One study explored the impact of FOXP3 polymorphisms on the long-term renal outcomes in patients receiving a cyclosporine-based treatment. FOXP3 c.-22-2361C>T but not c.-23+2882A>C was associated with lower eGFR values. To our knowledge, this is the only study which explored FOXP3 polymorphisms considering hemizygous males (Xu et al., 2018), and even though did not report any association for FOXP3 c.-23+2882A>C polymorphism, it drew the attention to these group of genetic variants.
FOXP3 c.-22-902A>G and c.-23+2882A>C polymorphisms were not related to long term acute rejection in kidney recipients, even when the analysis was carried out considering male as hemizygous and grouping with correspondent homozygous genotypes (data not shown). A previous study also did not find association of both FOXP3 variant with acute or chronic rejection, even in a 10-years follow-up period (Park et al., 2017). On the other hand, other studies reported association of the FOXP3 c.-23+2882A>C (A allele) polymorphism with long-term (2 and 5 years) acute rejection or nephrotoxicity in kidney recipients from different populations treated with cyclosporine- or TAC- based immunosuppressive therapy (Qiu et al., 2012; Misra et al., 2016; Wu et al., 2017). The c.-23+2882A allele was also associated with worst graft survival and higher rate of recurrence of the original glomerular disease in kidney recipients at 3-year post-transplant (Adamek et al., 2017).
FOXP3 variants are associated with susceptibility to autoimmune diseases and infections (Fodor et al., 2011; Kwon et al., 2017), but the underlying mechanisms are poorly understood. The FOXP3 c.-23+2882A>C variant can cause loss of binding of transcriptional factors to the “GGGCGG” sequence located in the core of the promoter region, altering the transcription of the gene (Oda et al., 2013). Functional analysis demonstrated the A allele of FOXP3 c.-23+2882A>C decreased the promoter activity (Shen et al., 2010), however, this finding is not reliable since the article was retracted. Therefore, more studies are needed in order to clarify whether the FOXP3 polymorphism behaves as a risk or protective factor for renal function.
Interestingly both FOXP3 c.-22-902A>G and c.-23+2882A>C were associated with long-term gastrointestinal adverse effects, but this association was not maintained in the multivariable analysis after p-value adjustment, even when the patients were analyzed with the additive model (data not shown). To our knowledge, there is no report investigating the effect of FOXP3 variants in the occurrence of adverse events.
Likewise, an association between the C allele of FKBP1A n.259+24936T>C and constipation was also found but this relationship was not sustained after Holm’s p-value adjustment. Moreover, FKBP2 c.-2110G>T was associated with adverse event (leukopenia) but not with renal dysfunction or acute rejection in this sample population. However, this relationship did not remain after p-value adjustment in the multivariable logistic regression model.
Both MPS-based immunosuppressive therapy and ganciclovir were also individually associated with leukopenia, but only the latter remained significant after adjustment. It is likely that carriers of the GG genotype of FKBP2 c.-2111G>T are more responsive to immunosuppressive drugs. Additional analysis showed that the GG genotype and the use of ganciclovir were not associated (data not shown). This might imply that CMV treatment is a strong predictor for leukopenia events rather than the FKBP2 c.-2110G>T variant.
It has been described that up to 35% of patients under treatment with MPS develops episodes of leukopenia (Yang et al., 2015). Another factor causing this drug adverse effect is the use of ganciclovir (Matsumoto et al., 2015). Thus, individuals with susceptible genotypes could be exposed to a higher risk of leucopenia, which is favorable to the effect of immunosuppressive agents.
In our work, among the non-genetic factors influencing kidney outcomes, the time of DGF was strongly associated with acute rejection, even in the presence of other confounders such as recipient and donor factors or cold ischemia time. Likewise, in a cohort of kidney recipients treated with TAC-based therapy, the presence of DGF was associated with increased risk of acute rejection (Wu et al., 2015). These findings emphasize careful surveillance of patients with DGF in order to mitigate possible episodes of acute rejection.
To best of our knowledge, this is the first study to investigate the association of MTOR, FKBP1A, FKBP2 and FOXP3 polymorphisms with renal function, acute rejection and drug-related adverse events in Brazilian kidney recipients.
The small sample size and the population substructure are limitations of this study, and in particular, the low number of female patients for the analysis of polymorphisms located in the X chromosome. The low number of cases with acute rejection and graft failure also limited the study of associations between these outcomes and polymorphisms. Also a heterogeneity in the therapeutic regimens of calcineurin and mTOR inhibitors for every study group is observed. The analyses of this work are exploratory considering that the main study was not designed to evaluate pharmacogenetic aspects.
Conclusion
In conclusion, this work shows that MTOR variants are associated with long-term kidney outcomes, in particular, with acute rejection and renal function. Furthermore, the variants in FKBP2, FKBP1A, and FOXP3 were individually associated with the occurrence of drug-related adverse events.
Author Contributions
AC-S, FG, AC, and RH wrote the article. AC-S, CF, HT-S, JM-P, MH, and RH designed the research. AC-S, GM, and RB performed the research. AC-S, FG, AC, and RH analyzed the data.
Funding
This work was supported by grants from CNPq (# 306061/2013-9) and FAPESP (2016/13118-8), Brazil. AC-S, GM, and RB had fellowships from CNPq and USP, Brazil. MH, RH, and FG are recipients of fellowships from CNPq and FAPESP, Brazil.
Conflict of Interest Statement
The authors declare that the research was conducted in the absence of any commercial or financial relationships that could be construed as a potential conflict of interest.
Acknowledgments
The authors thank Cristina M. Fajardo, Nagilla Oliveira, Vivian Bonezi, Alvaro M. Nishikawa, Patricia Salgado, and Andreia Dietzius for the technical support and for helping with the patient selection.
Supplementary Material
The Supplementary Material for this article can be found online at: https://www.frontiersin.org/articles/10.3389/fphar.2018.01296/full#supplementary-material
Abbreviations
Co/D, drug concentration for dose administered; CKD, chronic kidney disease; CMV, cytomegalovirus; CNI, calcineurin inhibitor; DGF, delayed graft function; eGFR, estimated glomerular filtration rate; EVR, everolimus; MPS, mycophenolate sodium; mTOR, mechanistic target of rapamycin; mTORi, mTOR inhibitor; TAC, tacrolimus; Tregs, regulatory T cells.
References
Adamek, M., Döhler, B., Hasan, K. K., Fiedler, G., Scherer, S., Opelz, G., et al. (2017). Assessing the impact of FoxP3 and Vav1 gene polymorphisms on kidney allograft survival. HLA 90, 102–105. doi: 10.1111/tan.13049
Barrett, J. C., Fry, B., Maller, J., and Daly, M. J. (2005). Haploview: analysis and visualization of LD and haplotype maps. Bioinformatics 21, 263–265. doi: 10.1093/bioinformatics/bth457
Bergan, S., Bremer, S., and Vethe, N. T. (2016). Drug target molecules to guide immunosuppression. Clin. Biochem. 49, 411–418. doi: 10.1016/j.clinbiochem.2015.10.001
Chi, H. (2012). Regulation and function of mTOR signalling in T cell fate decisions. Nat. Rev. Immunol. 12, 325–338. doi: 10.1038/nri3198
de Paula, M. I., Medina Pestana, J. O., Nicolau Ferreira, A., Pontello Cristelli, M., Fabiano Franco, M., Aguiar, W. F., et al. (2016). Long-term follow-up of de novo use of mTOR and calcineurin inhibitors after kidney transplantation. Ther. Drug Monit. 38, 22–31. doi: 10.1097/FTD.0000000000000227
Dummer, C. D., Carpio, V. N., Gonçalves, L. F., Manfro, R. C., and Veronese, F. V. (2012). FOXP3 + regulatory T cells: from suppression of rejection to induction of renal allograft tolerance. Transpl Immunol. 26, 1–10. doi: 10.1016/j.trim.2011.08.009
Fodor, E., Garaczi, E., Polyánka, H., Koreck, A., Kemény, L., and Széll, M. (2011). The rs3761548 polymorphism of FOXP3 is a protective genetic factor against allergic rhinitis in the Hungarian female population. Hum. Immunol. 72, 926–929. doi: 10.1016/j.humimm.2011.06.011
Ge, J., Wang, J., Zhao, H., Li, K., Jing, Y., and Li, G. (2016). Impact of FOXP3 polymorphisms on the blood level of tacrolimus in renal transplant recipients. Transpl. Proc. 48, 1962–1967. doi: 10.1016/j.transproceed.2016.04.016
Heinze, G., and Dunkler, D. (2017). Five myths about variable selection. Transpl. Int. 30, 6–10. doi: 10.1111/tri.12895
Hong, E. P., and Park, J. W. (2012). Sample size and statistical power calculation in genetic association studies. Genomics Inform. 10, 117–122. doi: 10.5808/GI.2012.10.2.117
Ingulli, E. (2010). Mechanism of cellular rejection in transplantation. Pediatr. Nephrol. 25, 61–74. doi: 10.1007/s00467-008-1020-x
Kooloos, W. M., Wessels, J. A., van der Straaten, T., Huizinga, T. W., and Guchelaar, H. J. (2009). Criteria for the selection of single nucleotide polymorphisms in pathway pharmacogenetics: TNF inhibitors as a case study. Drug Discov. Today 14, 837–844. doi: 10.1016/j.drudis.2009.05.017
Kotton, C. N., Kumar, D., Caliendo, A. M., Asberg, A., Chou, S., Danziger-Isakov, L., et al. (2013). Updated international consensus guidelines on the management of cytomegalovirus in solid-organ transplantation. Transplantation 96, 333–360. doi: 10.1097/TP.0b013e31829df29d
Kwon, T. K., Chung, E. J., Lee, N., Roh, E. Y., and Song, E. Y. (2017). Associations of FoxP3 gene polymorphisms with severe recurrent respiratory papillomatosis in Korean patients. J. Otolaryngol. Head. Neck Surg. 46, 21. doi: 10.1186/s40463-017-0197-z
Levey, A. S., Stevens, L. A., Schmid, C. H., Zhang, Y. L., Castro, A. F. III, Feldman, H. I., et al. (2009). A new equation to estimate glomerular filtration rate. Ann. Intern. Med. 150, 604–612. doi: 10.7326/0003-4819-150-9-200905050-00006
Lim, M. A., Kohli, J., and Bloom, R. D. (2017). Immunosuppression for kidney transplantation: where are we now and where are we going? Transpl. Rev. 31, 10–17. doi: 10.1016/j.trre.2016.10.006
Liu, T., Gulinaer, A., Shi, X., Wang, F., An, H., Cui, W., et al. (2017). Gene polymorphisms in the PI3K/AKT/mTOR signaling pathway contribute to prostate cancer susceptibility in Chinese men. Oncotarget 8, 61305–61317. doi: 10.18632/oncotarget.18064
Ma, M. K. M., Yung, S., and Chan, T. M. (2018). mTOR inhibition and kidney diseases. Transplantation 102(Suppl. 1), S32–S40. doi: 10.1097/TP.0000000000001729
Matsumoto, K., Shigemi, A., Ikawa, K., Kanazawa, N., Fujisaki, Y., Morikawa, N., et al. (2015). Risk factors for ganciclovir-induced thrombocytopenia and leukopenia. Biol. Pharm. Bull. 38, 235–238. doi: 10.1248/bpb.b14-00588
Medical Dictionary for Regulatory Activities [MedDRA] (2016). Medical Dictionary for Regulatory Activities. Available at: http://www.meddra.org [accessed January 4, 2016].
Misra, M. K., Mishra, A., Pandey, S. K., Kapoor, R., Sharma, R. K., and Agrawal, S. (2016). Association of functional genetic variants of transcription factor Forkhead Box P3 and nuclear factor-κB with end-stage renal disease and renal allograft outcome. Gene 581, 57–65. doi: 10.1016/j.gene.2016.01.028
Moes, D. J., Press, R. R., Ackaert, O., Ploeger, B. A., Bemelman, F. J., Diack, C., et al. (2016). Exploring genetic and non-genetic risk factors for delayed graft function, acute and subclinical rejection in renal transplant recipients. Br. J. Clin. Pharmacol. 82, 227–237. doi: 10.1111/bcp.12946
Oda, J. M., Hirata, B. K., Guembarovski, R. L., and Watanabe, M. A. (2013). Genetic polymorphism in FOXP3 gene: imbalance in regulatory T-cell role and development of human diseases. J. Genet. 92, 163–171. doi: 10.1007/s12041-013-0213-7
Park, H., Lee, N., In, J. W., Roh, E. Y., Park, K. U., Shin, S., et al. (2017). Association of Foxp3 polymorphism with allograft outcome in kidney transplantation. Ann. Lab. Med. 37, 420–425. doi: 10.3343/alm.2017.37.5.420
Picard, N., Bergan, S., Marquet, P., van Gelder, T., Wallemacq, P., Hesselink, D. A., et al. (2016). Pharmacogenetic biomarkers predictive of the pharmacokinetics and pharmacodynamics of immunosuppressive drugs. Ther. Drug Monit. 38(Suppl. 1), S57–S69. doi: 10.1097/FTD.0000000000000255
Pouché, L., Koitka, M., Stojanova, J., Woillard, J. B., Monchaud, C., Villeneuve, C., et al. (2016a). A candidate gene approach of the calcineurin pathway to identify variants associated with clinical outcomes in renal transplantation. Pharmacogenomics 17, 375–391. doi: 10.2217/pgs.15.181
Pouché, L., Stojanova, J., Marquet, P., and Picard, N. (2016b). New challenges and promises in solid organ transplantation pharmacogenetics: the genetic variability of proteins involved in the pharmacodynamics of immunosuppressive drugs. Pharmacogenomics 17, 277–296. doi: 10.2217/pgs.15.169
Qiu, X. Y., Jiao, Z., Zhang, M., Chen, J. P., Shi, X. J., and Zhong, M. K. (2012). Genetic association of FOXP3 gene polymorphisms with allograft rejection in renal transplant patients. Nephrology 17, 423–430. doi: 10.1111/j.1440-1797.2012.01561.x
Salgado, P. C., Genvigir, F. D., Felipe, C. R., Tedesco-Silva, H. Jr., Medina-Pestana, J. O., Doi, S. Q., et al. (2017). Association of the PPP3CA c.249G > A variant with clinical outcomes of tacrolimus-based therapy in kidney transplant recipients. Pharmgenomics Pers. Med. 10, 101–106. doi: 10.2147/PGPM.S131390
Shen, Z., Chen, L., Hao, F., Wang, G., and Liu, Y. (2010). Intron-1 rs3761548 is related to the defective transcription of Foxp3 in psoriasis through abrogating E47/c-Myb binding. J. Cell. Mol. Med. 14, 226–241. doi: 10.1111/j.1582-4934.2008.00370.x
Slattery, M. L., Herrick, J. S., Lundgreen, A., Fitzpatrick, F. A., Curtin, K., and Wolff, R. K. (2010). Genetic variation in a metabolic signaling pathway and colon and rectal cancer risk: mTOR, PTEN, STK11, RPKAA1, PRKAG2, TSC1, TSC2, PI3K and Akt1. Carcinogenesis 31, 1604–1611. doi: 10.1093/carcin/bgq142
Slattery, M. L., Lundgreen, A., Mullany, L. E., Penney, R. B., and Wolff, R. K. (2014). Influence of CHIEF pathway genes on gene expression: a pathway approach to functionality. Int. J. Mol. Epidemiol. Genet. 5, 100–111.
Tedesco-Silva, H., Felipe, C., Ferreira, A., Cristelli, M., Oliveira, N., Sandes-Freitas, T., et al. (2015). Reduced incidence of cytomegalovirus infection in kidney transplant recipients receiving everolimus and reduced tacrolimus doses. Am. J. Transpl. 15, 2655–2664. doi: 10.1111/ajt.13327
Tong, M., and Jiang, Y. (2015). FK506-binding proteins and their diverse functions. Curr. Mol. Pharmacol. 9, 48–65. doi: 10.2174/1874467208666150519113541
van Gelder, T. (2014). Within-patient variability in immunosuppressive drug exposure as a predictor for poor outcome after transplantation. Kidney Int. 85, 1267–1268. doi: 10.1038/ki.2013.484
Woillard, J. B., Kamar, N., Rousseau, A., Rostaing, L., Marquet, P., and Picard, N. (2012). Association of sirolimus adverse effects with m-TOR, p70S6K or raptor polymorphisms in kidney transplant recipients. Pharmacogenet. Genomics 22, 725–732. doi: 10.1097/FPC.0b013e328357359d
Wu, W. K., Famure, O., Li, Y., and Kim, S. J. (2015). Delayed graft function and the risk of acute rejection in the modern era of kidney transplantation. Kidney Int. 88, 851–858. doi: 10.1038/ki.2015.190
Wu, Z., Xu, Q., Qiu, X., Jiao, Z., Zhang, M., and Zhong, M. (2017). FOXP3 rs3761548 polymorphism is associated with tacrolimus-induced acute nephrotoxicity in renal transplant patients. Eur. J. Clin. Pharmacol. 73, 39–47. doi: 10.1007/s00228-016-2140-z
Xie, X., Jiang, Y., Lai, X., Xiang, S., Shou, Z., and Chen, J. (2015). mTOR inhibitor versus mycophenolic acid as the primary immunosuppression regime combined with calcineurin inhibitor for kidney transplant recipients: a meta-analysis. BMC Nephrol. 16:91. doi: 10.1186/s12882-015-0078-5
Xu, M., Tao, G., Kang, M., Gao, Y., Zhu, H., Gong, W., et al. (2013). A polymorphism (rs2295080) in mTOR promoter region and its association with gastric cancer in a Chinese population. PLoS One 8:e60080. doi: 10.1371/journal.pone.0060080
Xu, Q. X., Qiu, X. Y., Jiao, Z., Zhang, M., and Zhong, M. K. (2018). FOXP3 rs3761549 polymorphism predicts long-term renal allograft function in patients receiving cyclosporine-based immunosuppressive regimen. Gene 644, 93–100. doi: 10.1016/j.gene.2017.10.081
Yang, Y., Yu, B., and Chen, Y. (2015). Blood disorders typically associated with renal transplantation. Front. Cell. Dev. Biol. 3:18. doi: 10.3389/fcell.2015.00018
Zeng, H., and Chi, H. (2017). mTOR signaling in the differentiation and function of regulatory and effector T cells. Curr. Opin. Immunol. 46, 103–111. doi: 10.1016/j.coi.2017.04.005
Zeng, H., Yang, K., Cloer, C., Neale, G., Vogel, P., and Chi, H. (2013). mTORC1 couples immune signals and metabolic programming to establish T(reg)-cell function. Nature 499, 485–490. doi: 10.1038/nature12297
Keywords: kidney transplant, immunosuppressive drugs, mTOR, calcineurin, FOXP3, pharmacogenetics
Citation: Campos-Salazar AB, Genvigir FDV, Felipe CR, Tedesco-Silva H, Medina-Pestana J, Monteiro GV, Basso RG, Cerda A, Hirata MH and Hirata RDC (2018) Polymorphisms in mTOR and Calcineurin Signaling Pathways Are Associated With Long-Term Clinical Outcomes in Kidney Transplant Recipients. Front. Pharmacol. 9:1296. doi: 10.3389/fphar.2018.01296
Received: 10 July 2018; Accepted: 22 October 2018;
Published: 14 November 2018.
Edited by:
Ulrich M. Zanger, Dr. Margarete Fischer-Bosch-Institut für Klinische Pharmakologie (IKP), GermanyReviewed by:
Julio Benitez, Universidad de Extremadura, SpainWenndy Hernandez, University of Chicago, United States
Copyright © 2018 Campos-Salazar, Genvigir, Felipe, Tedesco-Silva, Medina-Pestana, Monteiro, Basso, Cerda, Hirata and Hirata. This is an open-access article distributed under the terms of the Creative Commons Attribution License (CC BY). The use, distribution or reproduction in other forums is permitted, provided the original author(s) and the copyright owner(s) are credited and that the original publication in this journal is cited, in accordance with accepted academic practice. No use, distribution or reproduction is permitted which does not comply with these terms.
*Correspondence: Rosario Dominguez Crespo Hirata, cm9zYXJpb2hpcmF0YUB1c3AuYnI=