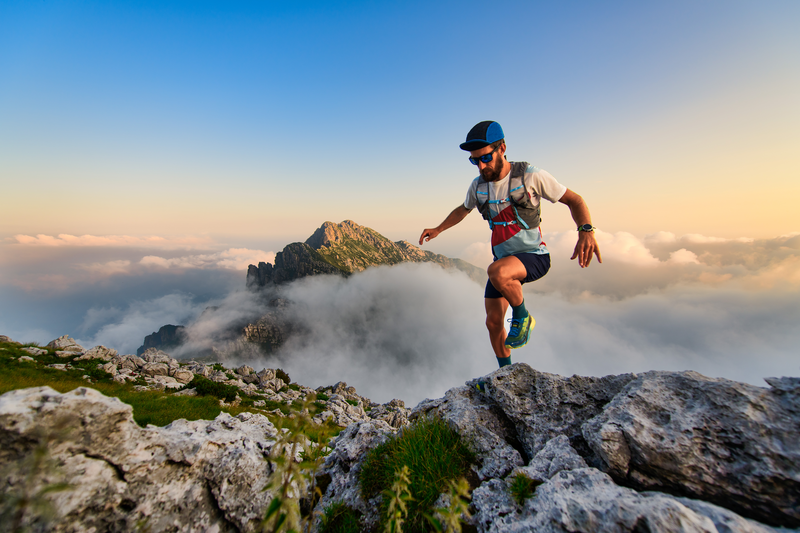
95% of researchers rate our articles as excellent or good
Learn more about the work of our research integrity team to safeguard the quality of each article we publish.
Find out more
ORIGINAL RESEARCH article
Front. Pharmacol. , 19 September 2018
Sec. Pharmacogenetics and Pharmacogenomics
Volume 9 - 2018 | https://doi.org/10.3389/fphar.2018.01008
This article is part of the Research Topic Next-Generation Sequencing in Pharmacogenetics/genomics View all 12 articles
Background: Many gene variants modulate the individual perception of pain and possibly also its persistence. The limited selection of single functional variants is increasingly being replaced by analyses of the full coding and regulatory sequences of pain-relevant genes accessible by means of next generation sequencing (NGS).
Methods: An NGS panel was created for a set of 77 human genes selected following different lines of evidence supporting their role in persisting pain. To address the role of these candidate genes, we established a sequencing assay based on a custom AmpliSeqTM panel to assess the exomic sequences in 72 subjects of Caucasian ethnicity. To identify the systems biology of the genes, the biological functions associated with these genes were assessed by means of a computational over-representation analysis.
Results: Sequencing generated a median of 2.85 ⋅ 106 reads per run with a mean depth close to 200 reads, mean read length of 205 called bases and an average chip loading of 71%. A total of 3,185 genetic variants were called. A computational functional genomics analysis indicated that the proposed NGS gene panel covers biological processes identified previously as characterizing the functional genomics of persisting pain.
Conclusion: Results of the NGS assay suggested that the produced nucleotide sequences are comparable to those earned with the classical Sanger sequencing technique. The assay is applicable for small to large-scale experimental setups to target the accessing of information about any nucleotide within the addressed genes in a study cohort.
Persisting pain has been proposed to result from a gene environment interaction where nerve injuries or inflammatory processes act as triggers while the clinical symptoms develop only in a minority of subjects (Lee and Tracey, 2013). A role of the genetic background in pain is supported by evidence of many variants modulating the individual perception of pain and the development of its persistence (Diatchenko et al., 2005; Lötsch et al., 2009b; Mogil, 2012). Genetic variants have been reported to confer protection against pain such as the rs1799971 variant in the μ-opioid receptor gene (OPRM1) (Lötsch et al., 2006), or to increase the risk for persisting pain such as the rs12584920 variant of the 5-hydroxytryptamine receptor 2A gene (HTR2A) (Nicholl et al., 2011) or the rs734784 polymorphism in the voltage-gated potassium ion channel modifier, subfamily S member 1, gene (KCNS1) (Costigan et al., 2010). Nevertheless, the genetic background of persisting pain is still incompletely understood (Mogil, 2009; Lötsch and Geisslinger, 2010) and under intense discussion.
Until recently, research focused on the role of selected functional genetic variants as protective or risk factors of persisting pain. This has changed with the broader availability of next generation sequencing (NGS) (Metzker, 2010). To make use of these technical advancements, we developed a custom AmpliSeqTM library and sequencing assay for efficient detection of genetic variants possibly associated with persisting pain. We propose an assay of a set of 77 genes supported by evidence of an involvement in pain and its development toward persistence. The set size fully uses the technical specifications of the AmpliSeqTM gene sequencing library technique.
A set of candidate genes with shown or biologically plausible relevance to persisting pain was created by applying a combination of criteria, which provided three different genetic subsets. Subset 1 was chosen exclusively on the basis of computational functional genomics based on a recently published analysis of persisting pain regarded as displaying systemic features of learning and neuronal plasticity (Mansour et al., 2014). As discussed previously (Ultsch et al., 2016), the view of chronic pain as a dysregulation in biological processes of learning and neuronal plasticity (Alvarado et al., 2013) seems to be captured by the controlled vocabulary (Camon et al., 2004) of the Gene Ontology (GO) knowledge base by the GO terms “learning or memory” (GO:0007611)1 and “nervous system development” (GO:0007399)2. An intersection of the genes annotated to these GO terms with a set of 539 “pain genes” identified empirically as relevant to pain provided the first subset of 34 genes described in detail previously (Ultsch et al., 2016). Briefly, the intersecting set of so-called “pain genes” consists of a combination of (i) genes listed in the PainGenes database (Lacroix-Fralish et al., 2007)3, (ii) genes causally involved in human hereditary diseases associated with extreme pain phenotypes, (iii) genes found to be associated with chronic pain in at least three human studies, and (iv) genes coding for targets of novel analgesics under clinical development (Lötsch et al., 2013).
Subset 2 consisted of genes that were reported to carry variants modulating the risk or the phenotypic symptoms in at least two different clinical settings of persisting pain. They were obtained using (i) a PubMed database search for the string “(chronic OR persisting OR neuropathic OR back OR inflammatory OR musculoskeletal OR visceral OR widespread OR idiopathic OR fibromyalgia) AND pain AND (polymorphism OR variant) NOT review,” to which genes highlighted in overviews on pain genetics (e.g., Edwards, 2006) were added. The intersection of the queried genes with the set of 539 “pain genes” (see above) provided a subset of 13 genes (Table 1).
TABLE 1. Genes included in the proposed NGS panel of persisting pain, combined from three subsets included on different bases.
Finally, subset 3 comprised genes that have consistently been included in human pain research projects over the last several years. One of them is the OPRM1 gene that codes for the human μ-opioid receptor and which has been shown to modulate the time course of persisting cancer pain by delaying the necessity of opioid treatment (Lötsch et al., 2010). However, further genes were added such as the GDNF gene coding for the glial cell derived neurotrophic factor, which has been shown to be involved in a glia-dependent mechanism of neuropathic pain (Wang et al., 2014) although no modulating human genetic variants have been reported so far. Following expert counseling within the EU-funded “glial-opioid interface in chronic pain, GLORIA” research consortium (Kringel and Lötsch, 2015)4, a subset of 30 genes (Table 1) was identified. Thus, the complete set as the union of the three subsets comprised 43 + 13 + 30 = 77 genes that are proposed to be included in an NGS panel of human persisting pain.
Due to the costs of assay development (for details, see second paragraph of the Discussion), the AmpliseqTM panel was established in a limited number of n = 72 DNA samples. This corresponds to the number of samples used in comparable recent studies for NGS assay establishment and validation (Bruera et al., 2018; De Luca et al., 2018; Mustafa et al., 2018; Shah et al., 2018). To further limit the project costs, the AmpliseqTM panel was established in a subset of samples originating from a clinical cohort of 1,000 women who had undergone breast cancer surgery (Kaunisto et al., 2013; Lötsch et al., 2018). The study followed the Declaration of Helsinki and was approved by the Coordinating Ethics Committee of the Helsinki University Hospital. Each participating subject had provided a written informed consent including genetic studies.
Specifically, for the presently reported method establishment, a subsample of 72 women (age 58.4 ± 8 years, mean ± standard deviation, weight 69.3 ± 11 kg), was drawn from the clinical subgroup not having developed persisting pain during the observation period. This was believed to come closer to a random sample than a mixture of patients with persisting and without persisting pain. This limitation of the sample selection has probably affected which and how many variants were identified. However, it is unlikely to have jeopardized the general applicability of the gene selection heuristics, assay establishment and validation, and of the functional analysis of the selected subset of genes.
A multiplex PCR amplification strategy for the coding gene sequences was accomplished online (Ion AmpliseqTM Designer)5 to amplify the target region specified above (for primer sequences, see Supplementary Table 1) with 25 base pair exon padding. After a comparison of several primer design options, the design providing the maximum target sequence coverage was chosen. The ordered 1,953 amplicons covered approximately 97.5% of the target sequence (Supplementary Table 2). A total of 10 ng DNA per sample was used for the target enrichment by a multiplex PCR and each DNA pool was amplified with the Ion AmpliseqTM Library Kit in conjunction with the Ion AmpliseqTM “custom Primer Pool”-protocols according to the manufacturer’s procedures (Life Technologies, Darmstadt, Germany).
After each pool had undergone 18 PCR cycles, the PCR primers were removed with FuPa Reagent and the amplicons were ligated to the sequencing adaptors with short stretches of index sequences (barcodes) that enabled sample multiplexing for subsequent steps (Ion XpressTM Barcode Adapters Kit; Life Technologies). After purification with AMPure XP beads (Beckman Coulter, Krefeld, Germany), the barcoded libraries were quantified with a Qubit® 2.0 Fluorimeter (Life Technologies, Darmstadt, Germany) and normalized for DNA concentration to a final concentration of 20 pmol/l using the Ion Library EqualizerTM Kit (Life Technologies, Darmstadt, Germany). Equalized barcoded libraries from seven to eight samples at a time were pooled. To clonally amplify the library DNA onto the Ion Sphere Particles (ISPs; Life Technologies, Darmstadt, Germany), the library pool was subjected to emulsion PCR by using an Ion PGM HI-Q View Template Kit on an PGM OneTouch system (Life Technologies, Darmstadt, Germany) following the manufacturer’s protocol.
Enriched ISPs which carried many copies of the same DNA fragment were subjected to sequencing on an Ion 318 Chip to sequence pooled libraries with seven to eight samples. During this process, bases are inferred from light intensity signals, a process commonly referred to as base-calling (Ledergerber and Dessimoz, 2011). The number of combined libraries that can be accommodated in a single sequencing run depends on the size of the chip, the balance of barcoded library concentration, and the coverage required. The high-capacity 318 chip was chosen (instead of the low-capacity 314 or the medium-capacity 316 chip) to obtain a high sequencing depth of coverage for a genomic DNA library with >95% of bases at 30x. Sequencing was performed using the sequencing kit (Ion PGM Hi-Q Sequencing Kit; Life Technologies, Darmstadt, Germany) as per the manufacturer’s instructions with the 200 bp single-end run configuration. This kit contained the most advanced sequencing chemistry available to users of the Ion PGM System (Life Technologies, Darmstadt, Germany).
The raw data (unmapped BAM-files) from the sequencing runs were processed using Torrent Suite Software (Version 5.2.2, Life Technologies, Darmstadt, Germany) to generate read alignments which were filtered by the software into mapped BAM-files using the reference genomic sequence (hg19) of target genes. Variant calling was performed with the Torrent Variant Caller Plugin using as key parameters: minimum allele frequency = 0.15, minimum quality = 10, minimum coverage = 20 and minimum coverage on either strand = 3.
The annotation of called variants was done using the Ion Reporter Software (Version 4.4; Life Technologies, Darmstadt, Germany) for the VCF files that contained the nucleotide reads and the GenomeBrowse® software (Version 2.0.4, Golden Helix, Bozeman, MT, United States) to map the sequences to the reference sequences GRCh37 hg19 (dated February 2009). The SNP and Variation Suite software (Version 8.4.4; Golden Helix, Bozeman, MT, United States) was used for the analysis of sequence quality, coverage and for variant identification.
Based on the observed allelic frequency, the expected number of homozygous and heterozygous carriers of the respective SNP (single nucleotide polymorphism) was calculated using the Hardy-Weinberg equation. Only variants within the Hardy-Weinberg equilibrium as assessed using Fisher’s exact test (Emigh, 1980) were retained. The SNP and Variation Suite software (Version 8.4.4; Golden Helix, Bozeman, MT, United States) was used for the analysis of sequence quality, coverage and for variant identification.
Method validation was accomplished by means of Sanger sequencing (Sanger and Coulson, 1975; Sanger et al., 1977) in an independent external laboratory (Eurofins Genomics, Ebersberg, Germany). As performed previously with different AmpliSeqTM panels (Kringel et al., 2017) and other genotyping assays (Skarke et al., 2004, 2005), four DNA samples have been chosen randomly from an independent cohort of healthy subjects and sequenced with the current NGS panel. For the detected variant type, single nucleotide polymorphisms from five different genomic regions for which clinical associations have been reported (Table 2), i.e., rs324420 (FAAH), rs333970 (CSF1), rs4986790 (TLR4), rs4633 (COMT), and rs17151558 (RELN) were chosen for external sequencing. Amplification of the respective DNA segments was done using PCR primer pairs (forward, reverse) of (i) 5′-TTTCTTAAAAAGGCCAGCCTCCT-3′ and 5′-AATGACCCAAGATGCAGAGCA-3′ (ii) 5′-GCCTTCAACCCCGGGATGG-3′ and 5′-CTCCGATCCCTGGTGCTCCTC-3′ (iii) 5′-TTTATTGCACAGACTTGCGGGTTC-3′ and 5′-AGCCTTTTGAGAGATTTGAGTTTCA-3′ (iv) 5′-CCTTATCGGCTGGAACGAGTT-3′ and 5′-GTAAGGGCTTTGATGCCTGGT-3′ (v) 5′-GTTATTCCTCTGTAAGCAGCTGCCT-3′ and 5′-TGTTTGTTTTAGATTGTGGTGGGTT-3′. Results of Sanger sequencing were aligned with the genomic sequence and analyzed using Chromas Lite® (Version 2.1.1, Technelysium Pty Ltd, South Brisbane, QLD, Australia) and the GenomeBrowse® (Version 2.0.4, Golden Helix, Bozeman, MT, United States) was used to compare the sequences obtained with NGS or Sanger techniques.
TABLE 2. A list of coding human variants in the 77 putative chronic pain genes, found in the present random sample of 72 subjects of Caucasian ethnicity, for which clinical associations have been reported.
The NGS assay of the proposed set of 77 human genes relevant to persisting pain was established in 72 genomic DNA samples. As applied previously (Kringel et al., 2017), only exons including 25 bases of padding around all targeted coding regions for which the realized read-depths for each nucleotide was higher than 20 were contemplated as successfully analyzed. With this acceptance criterion the whole or almost whole coverage of the relevant sequences was obtained (Table 1; for details on missing variants, see Supplementary Table 3). The NGS sequencing process of the whole patient cohort required ten separate runs, each with samples of n = 7 or n = 8 patients. Coverage statistics were analogous between all runs and matched the scope of accepted quality levels [20–22]. A median of 2.85 ⋅ 106 reads per run was produced. The mean depth was close to 200 reads, the mean read length of called bases resulted in 205 bases and average chip loading was 71% (Figure 1A). To establish a sequencing output with a high density of ISPs on a sequencing chip, the chip loading value should exceed 60% (Life Technologies, Carlsbad, United States). The generated results of all NGS runs matched with the results obtained with Sanger sequencing of random samples (Figure 1B), meaning the accordance of nucleotide sequences between NGS and Sanger sequencing was 100% in all validated samples.
FIGURE 1. Assay establishment and validation. (A) Pseudo-color image of the Ion 318TM v2 Chip plate showing percent loading across the physical surface. This sequencing run had a 76% loading, which ensures a high Ion Sphere Particles (ISP) density. Every 318 chip contains 11 million wells and the color scale on the right side conduces as a loading indicator. Deep red coloration stays for a 100% loading, which means that every well in this area contains an ISP (templated and non-templated) whereas deep blue coloration implies that the wells in this area are empty. (B) Alignment of a segment of the ion torrent sequence of the COMT gene as a Golden Helix Genome Browse® readout versus the same sequence according to an externally predicted Sanger electropherogram. Highlighted is the COMT variant rs4633 (COMT c.186C>T → p.His62 =) as a heterozygous mutation and a non-mutated wild type. The SNP is part of the functional COMT haplotype comprising rs4633, rs4818 and rs4680, which showed >11-fold difference in expressed enzyme activity and was reported to be associated with different phenotypes of pain sensitivity (Diatchenko et al., 2005).
Following elimination of nucleotides agreeing with the standard human genome sequence GRCh37 g1k (dated February 2009), the result of the NGS consisted of a vector of nucleotide information about the d = 77 genes for each individual DNA sample (Figure 2). This vector had a length equaling the set union of the number of chromosomal positions in which a non-reference nucleotide had been found in any probe of the actual cohort. Specifically, a total of 3,185 genetic variants was found, of which 659 were located in coding parts of the genes, 1,241 were located in introns and 1,285 in the 3′-UTR, 5′-UTR, upstream or downstream regions. The coding variants for which a clinical or phenotypic association have been reported are listed in Table 2 together with an example of each variant. Most of the observed variants were single nucleotide polymorphisms (d = 571) whereas mixed polymorphisms (d = 26), nucleotide insertions (d = 18) or nucleotide deletions (d = 44) were more rarely found.
FIGURE 2. Mosaic plot representing a contingency table of the types of genetic variants detected by means of the present AmpliSeqTM panel versus the genes included in the assay. The vertical size of the cells is proportional to the number of variants of a particular type; the horizontal size of the cells is proportional to the number of variants found in the respective gene. The location of the variants is indicated at the left of the mosaic plot in letters colored similarly to the respective bars in the mosaic plot. Variants were not found at all possible locations of each gene, which causes the reduction of several bars to dashed lines drawn as placeholders and indicating that at the particular location no variant has been found in the respective gene. The figure has been created using the R software package (version 3.4.2 for Linux; http://CRAN.R-project.org/, R Development Core Team, 2008). UTR: untranslated region. NCExonic: Non-coding exonic.
In this report, development and validation of a novel AmpliseqTM NGS assay for the coding regions and boundary parts of d = 77 genes qualifying as candidate modulators of persisting pain is described. The NGS assay produced nucleotide sequences that corresponded, with respect to the selected validation probes, to the results of classical Sanger sequencing. However, the NGS assay substantially reduced the laboratory effort to obtain the genetic information and provides the perquisites to be used in high throughput environments. In particular, the presented NGS assay is convenient for small up to large-scale setups. As mentioned in the methods section, a limitation of the present results applies to the identified genetic variants as only samples from Caucasian women were included. By contrast, the validity of gene selection and assay establishment is unlikely to be reduced by this selection chosen to remain within the financial limits of the present project.
Specifically, as observed previously (Kringel et al., 2017), the comprehensive genetic information and the high throughput are reflected in the assay costs. Specifically, sequencing of the 77 genes in 72 DNA samples required approximately € 18,000 for the AmpliSeqTM custom panel, € 5,500 for library preparation, € 700 for template preparation and € 700 for sequencing. Ten 318 sequencing chips cost around € 7,000 and in addition and basic consumables and laboratory supplies issued approximately € 800. With 7–8 barcoded samples loaded on ten chips, the expense to analyses the gene sequence for a single patient were around € 325. While NGS costs are likely to decrease in the near future (Lohmann and Klein, 2014), present assay establishment was therefore applied in DNA samples planned for future genotype versus phenotype association analysis, which required using DNA from patients of a pain-relevant cohort instead from a true random sample of healthy subjects.
As a result of the present assay development, a set of d = 77 genes was chosen as potentially relevant to persisting pain. The chosen set of genes differs from alternative proposals aiming at similar phenotypes (Mogil, 2012; Zorina-Lichtenwalter et al., 2016). However, when analyzing these alternatives for mutual agreement, only limited overlap could be observed (Figure 3). This emphasizes that the genetic architecture of persisting pain is incompletely understood, and several independent lines of research can be pursued. Of note, the present set showed the largest agreement with a set of d = 539 genes identified empirically as relevant to pain and listed in the PainGenes database (Lacroix-Fralish et al., 2007)6 or recognized as causing human hereditary diseases associated with extreme pain phenotypes (Lötsch et al., 2013; Ultsch et al., 2016). Combining all proposals into a large panel was not an option due to the technical limitations of the IonTorrent restricting the panel size to 500 kb (pipeline version 5.6.2); therefore, further genes would need to be addressed in separate panels.
FIGURE 3. Venn diagram (Venn, 1880) visualizing the intersections between the presently proposed set of human genes involved in modulating the risk or the clinical course of persisting pain (“Current set,” green frame), and two alternative proposals [“Mogil” (Mogil, 2012), blue frame and “Zorina-Lichtenwalter” (Zorina-Lichtenwalter et al., 2016), violet frame]. In addition, a set of d = 539 genes identified empirically as relevant to pain and either listed in the PainGenes database (http://www.jbldesign.com/jmogil/enter.html, Lacroix-Fralish et al., 2007) or added because recognized as causing human hereditary diseases associated with extreme pain phenotypes, found to be regulated in chronic pain in at least three studies including human association studies, or being targets of novel analgesics. The number of shared genes between data sets is numerically shown in the respective intersections of the Venn diagram. The figure has been created using the R software package (version 3.4.2 for Linux; http://CRAN.R-project.org/, R Development Core Team, 2008) with the particular package “Vennerable” (Swinton J., https://r-forge.r-project.org/R/?group_id=474).
In the present study sample, selected with a certain bias by using, as explained above for cost saving, clinical samples from only women and only Caucasians, a total of 659 genetic coding variants were found. Regardless of the sample preselection, 105 clinical associations (Table 2) could be queried for the observed variants from openly obtainable data sources comprising (i) the Online Mendelian Inheritance in Man (OMIM®) database7, (ii) the NCBI gene index database8, the GeneCards database9 [27] and the “1000 Genomes Browser”10 (all accessed in December 2017). The observation of functional variants in the present cohort preselected for the absence of pain persistence is plausible as (i) variants can exert protective effects against chronic pain and (ii) most genetic variants identified so far exert only small effects on pain and the individual result of their functional modulations depends on their combined effects or from the sum of positive and negative effects on pain perception (Lötsch et al., 2009a).
The selection of genes (Table 1) relied on empirical evidence of their involvement in pain. For subset #1 (d = 34), this had been shown for 33 genes in the original paper (Ultsch et al., 2016). As the hypothesis that persisting pain displays systemic features of learning and of neuronal plasticity (Mansour et al., 2014) could be substantiated at a computational functional genomics level, the further gene (PTPRZ1, protein tyrosine phosphatase Z 1) can also be regarded as supported by prior knowledge to be included in the present set. The subset comprised, for example, genes associated with the mesolimbic dopaminergic system, i.e., DRD1, DRD2, DRD3, which code for dopamine receptors, and TH, which is the coding gene for the tyrosine hydroxylase, a metabolic restricting enzyme in dopaminergic pathways, which have been implicated in promoting chronic back pain (Hagelberg et al., 2003, 2004; Jaaskelainen et al., 2014; Martikainen et al., 2015). Further 14 genes were involved in the circadian rhythm recognized as a modulatory factor in various pain conditions such as arthritis (Haus et al., 2012; Gibbs and Ray, 2013) and neuropathic pain (Gilron and Ghasemlou, 2014). The subset further included three NMDA receptor genes (GRIN1, GRIN2A, and GRIN2B) known to be major players in a number of essential physiological functions including neuroplasticity (Coyle and Tsai, 2004). In addition, metabotropic glutamate receptors (mGluR) have been implemented in several chronic pain conditions. One subtype, mGluR5, coded by GRM5, is of particular interest in the context of pain conditions as recent studies showed a pro-nociceptive role of mGluR5 in models of chronic pain (Walker et al., 2001; Crock et al., 2012). Furthermore, genes associated with histaminergic signaling such as HRH3 have been implicated in pain transmission (Hough and Rice, 2011) and analgesia (Huang et al., 2007).
The second subset of genes relied on a new PubMed search rather than on a previously published and hypothesis-based selection of candidate genes. A computational functional genomics analysis of this subset (details not shown) suggested its involvement in (i) immune processes and (ii) nitric oxide signaling. The genes annotated to the GO term “immune system process” included interleukin (IL1B, IL4, IL6, IL10) (Dinarello, 1994; Choi and Reiser, 1998; Mocellin et al., 2004; Nemeth et al., 2004) and histocompatibility complex related (HLA-B) genes (Dupont and Ceppellini, 1989), which have been shown to be involved in immunological mechanisms of pain (Sato et al., 2002; de Rooij et al., 2009). This is also supported by published evidence for the further genes in this list, such as, TNF (Vassalli, 1992; Franchimont et al., 1999), GCH1 (Schott et al., 1993) and P2RX7 (Chen and Brosnan, 2006). The second major process group emerging from the functional genomics analysis of the key evidence for genetic modulation of clinical chronic pain was nitric oxide signaling, in particular metabolic processes, summarized in this context under the GO term “reactive oxygen species metabolic process” which includes the genes IL6 (Deakin et al., 1995), TNF (Deakin et al., 1995; Katusic et al., 1998), ESR1 (Clapauch et al., 2014), IL10 (Cattaruzza et al., 2003), GCH1 (Katusic et al., 1998; Zhang et al., 2007), IL1B (Katusic et al., 1998), IL4 (Coccia et al., 2000), P2RX7 (Gendron et al., 2003), SOD2 (Fridovich, 1978). Furthermore, catecholamines including noradrenaline, adrenaline and dopamine have multiple functions in the brain and spinal cord including pain perception and processing (D’Mello and Dickenson, 2008). Catechol-O-methyltransferase, encoded by the COMT gene, is one of several enzymes that degrade dopamine, noradrenaline and adrenaline and has become one of the most frequently addressed genes in pain research (Nackley et al., 2006).
Finally, subset #3 (d = 30) consists of genes repeatedly shown to play a role in the genetic modulation of persisting pain in humans or, by contrast, included a few novel items only recently published in the context of pain. This included members of the transient receptor potential (TRP) family (TRPA1, TRPM8, TRPV4) that are expressed at nociceptors and which are well established players in the perception of pain via their excitation by chemical, thermal or mechanical stimuli (Clapham, 2003). This similarly applies to the opioidergic system represented by the inclusion of the genes coding for the major opioid receptors (OPRM1, OPRK1 OPRD1), which have been associated with variations in pain or opioid response in various settings (Lötsch and Geisslinger, 2005). The most important of this group, the μ-opioid receptor encoded by the OPRM1 gene, carriers several variants of which the 118 A>G (rs1799971) has been studied most extensively since the early description of its association with a functional phenotype in humans (Lötsch et al., 2002).
Almost half of the present sets of genes were chosen based on a computational functional genomics analysis that attributed persisting pain to GO processes of “learning or memory” and “nervous system development” (Ultsch et al., 2016) as likely to reflect systemic features of persisting pain. This implied a functional bias and therefore, the present set of d = 77 genes (Figure 4) was analyzed whether this bias prevailed when comparing it with the alternative sets of human genes proposed to modulate persisting pain (Mogil, 2012; Zorina-Lichtenwalter et al., 2016). As applied previously (Lippmann et al., 2018), the biological roles of the set of d = 77 genes were queried from the Gene Ontology knowledgebase (GO)11 (Ashburner et al., 2000) where the knowledge about the biological processes, the molecular functions and the cellular components of genes is formulated using a controlled and clearly defined vocabulary of GO terms. Particular biological roles of the set of d = 77 genes, among all human genes, were analyzed by means of over-representation analysis (ORA). This compared the occurrence of the particular GO terms associated with the present set of genes with their expected occurrence by chance (Backes et al., 2007). In contrast to enrichment analysis, any quantitative criteria such as gene expression values are disregarded (Backes et al., 2007). The analyses were performed using our R library “dbtORA” (Lippmann et al., 2018)12 on the R software environment (version 3.4.2 for Linux; R Development Core Team, 2008)13.
FIGURE 4. Top–down representation of the annotations (GO terms) representing the taxonomy of the functional differences between the set of d = 77 genes included in the proposed NGS panel of persisting pain and two alternative proposals of genes modulating persisting pain in humans (Mogil, 2012; Zorina-Lichtenwalter et al., 2016). The figure represents the results of an over-representation analysis of the present set of d = 77 genes against the reference comprising the set intersection of the alternative gene lists. A p-value threshold of 0.01 and Bonferroni α-correction were applied. Significant terms are shown as colored circles with the number of member genes, the number of expected genes by change and the significance of the deviation of the observed from the expected number of genes indicated (yellow = headline, red = significant term, blue = significant term located as a leave at the end of a taxonomy in the polyhierarchy). The graphical representation follows the standard of the GO knowledgebase, where GO terms are related to each other by “is-a,” “part-of,” and “regulates” relationships forming a polyhierarchy organized in a directed acyclic graph (DAG, Thulasiraman and Swamy, 1992). The figure has been created using our R library “dbtORA” (https://github.com/IME-TMP-FFM/dbtORA, Lippmann et al., 2018) on the R software package (version 3.4.2 for Linux; http://CRAN.R-project.org/, R Development Core Team, 2008) and the freely available graph visualization software GraphViz (http://www.graphviz.org, Gansner and North, 2000).
Surprisingly, the results of this analysis indicated that the functional bias of the present gene set toward “learning or memory” (GO:0007611) and “nervous system development” (GO:0007399) was not maintained against the alternative gene sets. Instead, a few more general GO terms such as “behavior” (“single organism behavior,” GO:0044708), or “response to organic cyclic compound” (GO:0014070) and response to alkaloid (GO:0043279), which could be identified as morphine and cocaine when repeating the analysis with a less conservative α-correction (further details not shown), were overrepresented, as well as the pain specific term “sensory perception of pain” (GO:0019233). A possible explanation that the selection bias of the present gene set was not maintained when comparing it with alternative proposals is that the two biological processes, “learning or memory” and “nervous system development,” reflect indeed an important biological function of persisting pain and even when choosing candidate genes without having these processes in mind as for the alternative gene sets, they are nevertheless included. This may be regarded as support for the present gene set as suitable candidates for future association studies with persisting pain phenotypes.
Although the present gene set has been assembled with a focus of a relevance to pain, many of its members have pharmacological implications. Specifically, 58 of the 77 genes (75%) have been chosen as targets of analgesics, approved or under current clinical development (Table 3). Moreover, several of the genes in the present NGS panel have been implicated in pharmacogenetic modulations of drug effects (Table 4). Possibly the most widely studied gene in analgesic research is OPRM1 because coding for the primary target of opioids (Peiro et al., 2016). Several polymorphisms have been described in OPRM1, among which the best characterized may be rs1799971 (OPRM1 118A>G) that leads to an asparagine to aspartate substitution at the extracellular terminal of the receptor protein (Bond et al., 1998). May studies have addressed this variant (for reviews, see Walter et al., 2013; Somogyi et al., 2015). Summarizing its effects, the variant is associated with decreased receptor expression and signaling efficiency (Oertel et al., 2012) which leads to reproducibly reduced pharmacodynamic effects in human experimental settings while the effect size seems insufficient to be a major factor of opioid response in clinical settings, despite several reports of modulations of opioid demands or side effects. For example, subjects carrying the 118A>G variant were found to have a reduced response to morphine treatment (Hwang et al., 2014), reduced analgesic response to alfentanil (Oertel et al., 2006) and demanded higher doses of morphine for pain relief (Klepstad et al., 2004; Hwang et al., 2014). However, the importance of this variant seems to be comparatively high in patients with an Asian ethnic background, which might be related to the higher allelic frequency as compared to other ethnicities. COMT is a key modulator of dopaminergic neurotransmission and in the signaling response to opioids The Val158Met polymorphism (rs4680) causes an amino acid substitution in the enzyme, which reduced the enzyme active to a forth (Peiro et al., 2016). Carriers of the homozygous Met/Met variant had lower morphine requirements than those with a the wild type COMT (Rakvag et al., 2005). Furthermore, a modulation of the effects of TRPV1 targeting analgesics is supported by observations that intronic TRPV1 variants were associated with insensitivity to capsaicin (Park et al., 2007) while the coding TRPV1 variant rs8065080 was associated with altered responses to experimentally induced pain(Kim et al., 2004). Moreover, gain-of-function mutations in TRPV1 have been associated with increased pain sensitivity (Boukalova et al., 2014), for which TRPV1 antagonists would enable a specific pharmacogenetics-based personalized cure.
TABLE 3. Current targeting of the genes included in the proposed NGS panel of persisting pain by novel drugs that are currently under active clinical development and include analgesia as the main clinical target or at least as one of the intended clinical indication.
TABLE 4. Summary of variants in genes included in the proposed NGS panel of persisting pain, that have been implicated in a pharmacogenetic context to modulate the effects of drugs administered for the treatment of pain or as disease modifying therapeutics in painful disease.
The breakthrough in mapping the whole human genome (Lander et al., 2001; Venter et al., 2001) along with genome wide association studies (GWAS) has led to rapid advances in the knowledge of the genetic bases of human diseases (Wellcome Trust Case Control and Consortium, 2007). Genetic research in pain medicine has directed to the recognition of genes in which variants influence pain behavior, post-operative drug requirements, and the temporal developments of pain toward persistence (James, 2013). While many candidate gene association studies have identified multiple genes relevant for pain phenotypes (Fillingim et al., 2008), pain related genetic studies have so far been owned by investigations of a limited number of genes. Roughly ten genes or gene complexes account for over half of the extant findings and several of these candidate gene associations have held up in replication (Mogil, 2012). The selection of variants has been limited and they have been addressed in most studies repeatedly, leading to the perception that genetic research in pain produces often unsatisfactory results (Mogil, 2009). However, this may soon change with the arise of new technologies. In this manuscript, we present a validated NGS assay for a set of 77 genes supported by empirical evidence and computational functional genomics analyses as relevant factors modulating the risk for persisting pain or its clinical picture.
JL, DK, and EK conceived and designed the experiments. DK performed the experiments. JL and DK analyzed the data and wrote the paper. CL provided methodological expertise and bioinformatical tools. DK and JL interpreted the results. EK and MK provided DNA samples.
This work has been funded by the European Union Seventh Framework Programme (FP7/2007 – 2013) under grant agreement no. 602919 (“GLORIA”, EK and JL) and the LandesOffensive zur Entwicklung Wissenschaftlich-ökonomischer Exzellenz (LOEWE), LOEWE-Zentrum für Translationale Medizin und Pharmakologie (JL). These public funders had no role in study design, data collection and analysis, decision to publish, or preparation of the manuscript.
The authors declare that the research was conducted in the absence of any commercial or financial relationships that could be construed as a potential conflict of interest.
The Supplementary Material for this article can be found online at: https://www.frontiersin.org/articles/10.3389/fphar.2018.01008/full#supplementary-material
Abbadie, C., Besson, J. M., and Calvino, B. (1994). c-Fos expression in the spinal cord and pain-related symptoms induced by chronic arthritis in the rat are prevented by pretreatment with Freund adjuvant. J. Neurosci. 14, 5865–5871. doi: 10.1523/JNEUROSCI.14-10-05865.1994
Achkar, J.-P., Klei, L., De Bakker, P. I. W., Bellone, G., Rebert, N., Scott, R., et al. (2012). Amino acid position 11 of HLA-DRβ1 is a major determinant of chromosome 6p association with ulcerative colitis. Genes Immun. 13, 245–252. doi: 10.1038/gene.2011.79
Al-Eitan, L. N., Jaradat, S. A., Su, S. Y., Tay, G. K., and Hulse, G. K. (2012). Mu opioid receptor (OPRM1) as a predictor of treatment outcome in opiate-dependent individuals of Arab descent. Pharmgen. Pers. Med. 5, 99–111. doi: 10.2147/PGPM.S33351
Alvarado, S., Tajerian, M., Millecamps, M., Suderman, M., Stone, L. S., and Szyf, M. (2013). Peripheral nerve injury is accompanied by chronic transcriptome-wide changes in the mouse prefrontal cortex. Mol. Pain 9:21. doi: 10.1186/1744-8069-9-21
Andreoli, V., De Marco, E. V., Trecroci, F., Cittadella, R., Di Palma, G., and Gambardella, A. (2014). Potential involvement of GRIN2B encoding the NMDA receptor subunit NR2B in the spectrum of Alzheimer’s disease. J. Neural Transm. (Vienna) 121, 533–542.
Ashburner, M., Ball, C. A., Blake, J. A., Botstein, D., Butler, H., Cherry, J. M., et al. (2000). Gene ontology: tool for the unification of biology. Gene Ontol. Consortium. Nat. Genet. 25, 25–29. doi: 10.1038/75556
Backes, C., Keller, A., Kuentzer, J., Kneissl, B., Comtesse, N., Elnakady, Y. A., et al. (2007). GeneTrail–advanced gene set enrichment analysis. Nucleic Acids Res. 35, W186–W192. doi: 10.1093/nar/gkm323
Biesiada, J., Chidambaran, V., Wagner, M., Zhang, X., Martin, L. J., Meller, J., et al. (2014). Genetic risk signatures of opioid-induced respiratory depression following pediatric tonsillectomy. Pharmacogenomics 15, 1749–1762. doi: 10.2217/pgs.14.137
Bond, C., Laforge, K. S., Tian, M., Melia, D., Zhang, S., and Borg, L. (1998). Single-nucleotide polymorphism in the human mu opioid receptor gene alters beta-endorphin binding and activity: possible implications for opiate addiction. Proc. Natl. Acad. Sci. U.S.A. 95, 9608–9613. doi: 10.1073/pnas.95.16.9608
Boukalova, S., Touska, F., Marsakova, L., Hynkova, A., Sura, L., Chvojka, S., et al. (2014). Gain-of-function mutations in the transient receptor potential channels TRPV1 and TRPA1: how painful? Physiol. Res. 63(Suppl. 1), S205–S213.
Bourinet, E., Altier, C., Hildebrand, M. E., Trang, T., Salter, M. W., and Zamponi, G. W. (2014). Calcium-permeable ion channels in pain signaling. Physiol. Rev. 94, 81–140. doi: 10.1152/physrev.00023.2013
Bravo, L., Torres-Sanchez, S., Alba-Delgado, C., Mico, J. A., and Berrocoso, E. (2014). Pain exacerbates chronic mild stress-induced changes in noradrenergic transmission in rats. Eur. Neuropsychopharmacol. 24, 996–1003. doi: 10.1016/j.euroneuro.2014.01.011
Bravo-Hernández, M., Corleto, J. A., Barragán-Iglesias, P., González-Ramírez, R., Pineda-Farias, J. B., Felix, R., et al. (2016). The α5 subunit containing GABAA receptors contribute to chronic pain. Pain 157, 613–626. doi: 10.1097/j.pain.0000000000000410
Bruera, G., Pepe, F., Malapelle, U., Pisapia, P., Mas, A. D., Di Giacomo, D., et al. (2018). KRAS, NRAS and BRAF mutations detected by next generation sequencing, and differential clinical outcome in metastatic colorectal cancer (MCRC) patients treated with first line FIr-B/FOx adding bevacizumab (BEV) to triplet chemotherapy. Oncotarget 9, 26279–26290. doi: 10.18632/oncotarget.25180
Buchheit, T., Van De Ven, T., and Shaw, A. (2012). Epigenetics and the transition from acute to chronic pain. Pain. Med. 13, 1474–1490. doi: 10.1111/j.1526-4637.2012.01488.x
Buskila, D., Cohen, H., Neumann, L., and Ebstein, R. P. (2004). An association between fibromyalgia and the dopamine D4 receptor exon III repeat polymorphism and relationship to novelty seeking personality traits. Mol. Psychiatry 9, 730–731. doi: 10.1038/sj.mp.4001568
Cajanus, K., Holmström, E. J., Wessman, M., Anttila, V., Kaunisto, M. A., and Kalso, E. (2016). Effect of endocannabinoid degradation on pain: role of FAAH polymorphisms in experimental and postoperative pain in women treated for breast cancer. Pain 157, 361–369. doi: 10.1097/j.pain.0000000000000398
Camilleri, M., Klee, E. W., Shin, A., Carlson, P., Li, Y., Grover, M., et al. (2014). Irritable bowel syndrome-diarrhea: characterization of genotype by exome sequencing, and phenotypes of bile acid synthesis and colonic transit. Am. J. Physiol. Gastrointest. Liver Physiol. 306, G13–G26. doi: 10.1152/ajpgi.00294.2013
Camon, E., Magrane, M., Barrell, D., Lee, V., Dimmer, E., Maslen, J., et al. (2004). The gene ontology annotation (GOA) database: sharing knowledge in uniprot with Gene Ontology. Nucleic Acids Res. 32, D262–D266. doi: 10.1093/nar/gkh021
Cargnin, S., Viana, M., Sances, G., Bianchi, M., Ghiotto, N., Tassorelli, C., et al. (2014). Combined effect of common gene variants on response to drug withdrawal therapy in medication overuse headache. Eur. J. Clin. Pharmacol. 70, 1195–1202. doi: 10.1007/s00228-014-1726-6
Cattaruzza, M., Slodowski, W., Stojakovic, M., Krzesz, R., and Hecker, M. (2003). Interleukin-10 induction of nitric-oxide synthase expression attenuates CD40-mediated interleukin-12 synthesis in human endothelial cells. J. Biol. Chem. 278, 37874–37880. doi: 10.1074/jbc.M301670200
Ceolin, L., Siqueira, D. R., Romitti, M., Ferreira, C. V., and Maia, A. L. (2012). Molecular basis of medullary thyroid carcinoma: the role of RET polymorphisms. Int. J. Mol. Sci. 13, 221–239. doi: 10.3390/ijms13010221
Chatti, I., Woillard, J. B., Mili, A., Creveaux, I., Ben Charfeddine, I., Feki, J., et al. (2017). Genetic analysis of mu and kappa opioid receptor and COMT enzyme in cancer pain tunisian patients under opioid treatment. Iran J. Public Health 46, 1704–1711.
Chen, C. L., Broom, D. C., Liu, Y., De Nooij, J. C., Li, Z., Cen, C., et al. (2006). Runx1 determines nociceptive sensory neuron phenotype and is required for thermal and neuropathic pain. Neuron 49, 365–377. doi: 10.1016/j.neuron.2005.10.036
Chen, D., Zhang, T.-L., and Wang, L.-M. (2014). The association of CSF-1 gene polymorphism with chronic periodontitis in the Han Chinese population. J. Periodontol. 85, e304–e312. doi: 10.1902/jop.2014.130688
Chen, L., and Brosnan, C. F. (2006). Regulation of immune response by P2X7 receptor. Crit. Rev. Immunol. 26, 499–513. doi: 10.1615/CritRevImmunol.v26.i6.30
Chen, X., Williamson, V. S., An, S.-S., Hettema, J. M., Aggen, S. H., Neale, M. C., et al. (2008). Cannabinoid receptor 1 gene association with nicotine dependence. Arch. Gen. Psychiatry 65, 816–824. doi: 10.1001/archpsyc.65.7.816
Choi, P., and Reiser, H. (1998). IL-4: role in disease and regulation of production. Clin. Exp. Immunol. 113, 317–319. doi: 10.1046/j.1365-2249.1998.00690.x
Clapauch, R., Mourao, A. F., Mecenas, A. S., Maranhao, P. A., Rossini, A., and Bouskela, E. (2014). Endothelial function and insulin resistance in early postmenopausal women with cardiovascular risk factors: importance of ESR1 and NOS3 polymorphisms. PLoS One 9:e103444. doi: 10.1371/journal.pone.0103444
Clapham, D. E. (2003). TRP channels as cellular sensors. Nature 426, 517–524. doi: 10.1038/nature02196
Coccia, E. M., Stellacci, E., Marziali, G., Weiss, G., and Battistini, A. (2000). IFN-gamma and IL-4 differently regulate inducible NO synthase gene expression through IRF-1 modulation. Int. Immunol. 12, 977–985. doi: 10.1093/intimm/12.7.977
Corominas, R., Ribases, M., Camiña, M., Cuenca-León, E., Pardo, J., Boronat, S., et al. (2009). Two-stage case-control association study of dopamine-related genes and migraine. BMC Med. Genet. 10:95. doi: 10.1186/1471-2350-10-95
Coskun, S., Varol, S., Ozdemir, H. H., Agacayak, E., Aydın, B., Kapan, O., et al. (2016). Association of brain-derived neurotrophic factor and nerve growth factor gene polymorphisms with susceptibility to migraine. Neuropsychiatr. Dis. Treat. 12, 1779–1785. doi: 10.2147/NDT.S108814
Costigan, M., Belfer, I., Griffin, R. S., Dai, F., Barrett, L. B., Coppola, G., et al. (2010). Multiple chronic pain states are associated with a common amino acid-changing allele in KCNS1. Brain 133, 2519–2527. doi: 10.1093/brain/awq195
Coyle, J. T., and Tsai, G. (2004). NMDA receptor function, neuroplasticity, and the pathophysiology of schizophrenia. Int. Rev. Neurobiol. 59, 491–515. doi: 10.1016/S0074-7742(04)59019-0
Crock, L. W., Stemler, K. M., Song, D. G., Abbosh, P., Vogt, S. K., Qiu, C. S., et al. (2012). Metabotropic glutamate receptor 5 (mGluR5) regulates bladder nociception. Mol. Pain 8:20. doi: 10.1186/1744-8069-8-20
Davila-Fajardo, C. L., Marquez, A., Pascual-Salcedo, D., Moreno Ramos, M. J., Garcia-Portales, R., Magro, C., et al. (2014). Confirmation of -174G/C interleukin-6 gene promoter polymorphism as a genetic marker predicting antitumor necrosis factor treatment outcome. Pharmacogenet. Genom. 24, 1–5. doi: 10.1097/FPC.0000000000000013
De Luca, C., Rappa, A. G., Gragnano, G., Malapelle, U., Troncone, G., and Barberis, M. (2018). Idylla assay and next generation sequencing: an integrated EGFR mutational testing algorithm. J. Clin. Pathol. 71, 745–750. doi: 10.1136/jclinpath-2018-205197
de Rooij, A. M., Florencia Gosso, M., Haasnoot, G. W., Marinus, J., Verduijn, W., Claas, F. H., et al. (2009). HLA-B62 and HLA-DQ8 are associated with complex regional pain syndrome with fixed dystonia. Pain 145, 82–85. doi: 10.1016/j.pain.2009.05.015
Deakin, A. M., Payne, A. N., Whittle, B. J., and Moncada, S. (1995). The modulation of IL-6 and TNF-alpha release by nitric oxide following stimulation of J774 cells with LPS and IFN-gamma. Cytokine 7, 408–416. doi: 10.1006/cyto.1995.0056
Diatchenko, L., Anderson, A. D., Slade, G. D., Fillingim, R. B., Shabalina, S. A., Higgins, T. J., et al. (2006). Three major haplotypes of the beta2 adrenergic receptor define psychological profile, blood pressure, and the risk for development of a common musculoskeletal pain disorder. Am. J. Med. Genet. B Neuropsychiatr. Genet. 141B, 449–462. doi: 10.1002/ajmg.b.30324
Diatchenko, L., Slade, G. D., Nackley, A. G., Bhalang, K., Sigurdsson, A., Belfer, I., et al. (2005). Genetic basis for individual variations in pain perception and the development of a chronic pain condition. Hum. Mol. Genet. 14, 135–143. doi: 10.1093/hmg/ddi013
Dinarello, C. A. (1994). The biological properties of interleukin-1. Eur. Cytokine Netw. 5, 517–531.
Dineley, K. T., Pandya, A. A., and Yakel, J. L. (2015). Nicotinic ACh receptors as therapeutic targets in CNS disorders. Trends Pharmacol. Sci. 36, 96–108. doi: 10.1016/j.tips.2014.12.002
D’Mello, R., and Dickenson, A. H. (2008). Spinal cord mechanisms of pain. Br. J. Anaesth. 101, 8–16. doi: 10.1093/bja/aen088
Doehring, A., Kirchhof, A., and Lötsch, J. (2009). Genetic diagnostics of functional variants of the human dopamine D2 receptor gene. Psychiatr. Genet. 19, 259–268. doi: 10.1097/YPG.0b013e32832d0941
Doehring, A., Küsener, N., Flühr, K., Neddermeyer, T. J., Schneider, G., and Lötsch, J. (2011). Effect sizes in experimental pain produced by gender, genetic variants and sensitization procedures. PLoS One 6:e17724. doi: 10.1371/journal.pone.0017724
Dominguez, C. A., Kalliomaki, M., Gunnarsson, U., Moen, A., Sandblom, G., Kockum, I., et al. (2013). The DQB1 ∗03:02 HLA haplotype is associated with increased risk of chronic pain after inguinal hernia surgery and lumbar disc herniation. Pain 154, 427–433. doi: 10.1016/j.pain.2012.12.003
Duan, G., Guo, S., Zhang, Y., Ying, Y., Huang, P., Wang, Q., et al. (2015). The effect of SCN9A variation on basal pain sensitivity in the general population: an experimental study in young women. J. Pain 16, 971–980. doi: 10.1016/j.jpain.2015.06.011
Dutta, S., Gangopadhyay, P. K., Sinha, S., Chatterjee, A., Ghosh, S., and Rajamma, U. (2011). An association analysis of reelin gene (RELN) polymorphisms with childhood epilepsy in eastern Indian population from West Bengal. Cell. Mol. Neurobiol. 31, 45–56. doi: 10.1007/s10571-010-9551-7
Dziedziejko, V., Kurzawski, M., Safranow, K., Chlubek, D., and Pawlik, A. (2011). The effect of ESR1 and ESR2 gene polymorphisms on the outcome of rheumatoid arthritis treatment with leflunomide. Pharmacogenomics 12, 41–47. doi: 10.2217/pgs.10.164
Edwards, R. R. (2006). Genetic predictors of acute and chronic pain. Curr. Rheumatol. Rep. 8, 411–417. doi: 10.1007/s11926-006-0034-2
Emigh, T. H. (1980). A comparison of tests for hardy-weinberg equilibrium. Biometrics 36, 627–642. doi: 10.2307/2556115
Ersig, A. L., Schutte, D. L., Standley, J., Leslie, E., Zimmerman, B., Kleiber, C., et al. (2017). Relationship of genetic variants with procedural pain, anxiety, and distress in children. Biol. Res. Nurs. 19, 339–349. doi: 10.1177/1099800417692878
Eskola, P. J., Kjaer, P., Daavittila, I. M., Solovieva, S., Okuloff, A., Sorensen, J. S., et al. (2010). Genetic risk factors of disc degeneration among 12-14-year-old danish children: a population study. Int. J. Mol. Epidemiol. Genet. 1, 158–165.
Feng, Y., Egan, B., and Wang, J. (2016). Genetic factors in intervertebral disc degeneration. Genes Dis. 3, 178–185. doi: 10.1016/j.gendis.2016.04.005
Feng, Y., Zhao, X., Zhou, C., Yang, L., Liu, Y., Bian, C., et al. (2013). The associations between the Val158Met in the catechol-O-methyltransferase (COMT) gene and the risk of uterine leiomyoma (ULM). Gene 529, 296–299. doi: 10.1016/j.gene.2013.07.019
Fillingim, R. B., Wallace, M. R., Herbstman, D. M., Ribeiro-Dasilva, M., and Staud, R. (2008). Genetic contributions to pain: a review of findings in humans. Oral Dis. 14, 673–682. doi: 10.1111/j.1601-0825.2008.01458.x
Florio, S. K., Loh, C., Huang, S. M., Iwamaye, A. E., Kitto, K. F., Fowler, K. W., et al. (2009). Disruption of nNOS-PSD95 protein-protein interaction inhibits acute thermal hyperalgesia and chronic mechanical allodynia in rodents. Br. J. Pharmacol. 158, 494–506. doi: 10.1111/j.1476-5381.2009.00300.x
Franchimont, D., Martens, H., Hagelstein, M. T., Louis, E., Dewe, W., Chrousos, G. P., et al. (1999). Tumor necrosis factor alpha decreases, and interleukin-10 increases, the sensitivity of human monocytes to dexamethasone: potential regulation of the glucocorticoid receptor. J. Clin. Endocrinol. Metab. 84, 2834–2839.
Fridovich, I. (1978). The biology of oxygen radicals. Science 201, 875–880. doi: 10.1126/science.210504
Fujii, T., Ota, M., Hori, H., Hattori, K., Teraishi, T., Matsuo, J., et al. (2014). The common functional FKBP5 variant rs1360780 is associated with altered cognitive function in aged individuals. Sci. Rep. 4:6696. doi: 10.1038/srep06696
Gadotti, V. M., and Zamponi, G. W. (2011). Cellular prion protein protects from inflammatory and neuropathic pain. Mol. Pain 7:59. doi: 10.1186/1744-8069-7-59
Gansner, E. R., and North, S. C. (2000). An open graph visualization system and its applications to software engineering. Softw. Pract. Exp. 30, 1203–1233. doi: 10.1002/1097-024X(200009)30:11<1203::AID-SPE338>3.0.CO;2-N
Garassino, M. C., Piva, S., La Verde, N., Spagnoletti, I., Iorno, V., Carbone, C., et al. (2013). Randomised phase II trial (NCT00637975) evaluating activity and toxicity of two different escalating strategies for pregabalin and oxycodone combination therapy for neuropathic pain in cancer patients. PLoS One 8:e59981. doi: 10.1371/journal.pone.0059981
Garriock, H. A., Tanowitz, M., Kraft, J. B., Dang, V. C., Peters, E. J., Jenkins, G. D., et al. (2010). Association of mu-opioid receptor variants and response to citalopram treatment in major depressive disorder. Am. J. Psychiatry 167, 565–573. doi: 10.1176/appi.ajp.2009.08081167
Gębura, K., Świerkot, J., Wysoczańska, B., Korman, L., Nowak, B., Wiland, P., et al. (2017). Polymorphisms within genes involved in regulation of the NF-κB pathway in patients with rheumatoid arthritis. Int. J. Mol. Sci. 18, E1432. doi: 10.3390/ijms18071432
Gendron, F. P., Chalimoniuk, M., Strosznajder, J., Shen, S., Gonzalez, F. A., Weisman, G. A., et al. (2003). P2X7 nucleotide receptor activation enhances IFN gamma-induced type II nitric oxide synthase activity in BV-2 microglial cells. J. Neurochem. 87, 344–352. doi: 10.1046/j.1471-4159.2003.01995.x
Georgi, A., Jamra, R. A., Klein, K., Villela, A. W., Schumacher, J., Becker, T., et al. (2007). Possible association between genetic variants at the GRIN1 gene and schizophrenia with lifetime history of depressive symptoms in a German sample. Psychiatr. Genet. 17, 308–310. doi: 10.1097/YPG.0b013e3280c1e5fb
Gibbs, J. E., and Ray, D. W. (2013). The role of the circadian clock in rheumatoid arthritis. Arthritis Res. Ther. 15:205. doi: 10.1186/ar4146
Gilron, I., and Ghasemlou, N. (2014). Chronobiology of chronic pain: focus on diurnal rhythmicity of neuropathic pain. Curr. Opin. Support. Palliat. Care 8, 429–436. doi: 10.1097/SPC.0000000000000085
Goodin, B. R., Ness, T. J., and Robbins, M. T. (2015). Oxytocin – A multifunctional analgesic for chronic deep tissue pain. Curr. Pharm. Des. 21, 906–913. doi: 10.2174/1381612820666141027111843
Goodyer, I. M., Croudace, T., Dunn, V., Herbert, J., and Jones, P. B. (2010). Cohort profile: risk patterns and processes for psychopathology emerging during adolescence: the ROOTS project. Int. J. Epidemiol. 39, 361–369. doi: 10.1093/ije/dyp173
Gramage, E., and Herradon, G. (2010). Genetic deletion of pleiotrophin leads to disruption of spinal nociceptive transmission: evidence for pleiotrophin modulation of morphine-induced analgesia. Eur. J. Pharmacol. 647, 97–102. doi: 10.1016/j.ejphar.2010.08.029
Greenbaum, L., Tegeder, I., Barhum, Y., Melamed, E., Roditi, Y., and Djaldetti, R. (2012). Contribution of genetic variants to pain susceptibility in Parkinson disease. Eur. J. Pain 16, 1243–1250. doi: 10.1002/j.1532-2149.2012.00134.x
Guerrero, M., Urbano, M., Brown, S. J., Cayanan, C., Ferguson, J., Cameron, M., et al. (2010). “Optimization and characterization of an opioid kappa receptor (OPRK1) antagonist,” in Probe Reports from the NIH Molecular Libraries Program. Bethesda (MD): National Center for Biotechnology Information (US).
Hack, L. M., Kalsi, G., Aliev, F., Kuo, P.-H., Prescott, C. A., Patterson, D. G., et al. (2011). Limited associations of dopamine system genes with alcohol dependence and related traits in the Irish Affected Sib Pair Study of Alcohol Dependence (IASPSAD). Alcohol. Clin. Exp. Res. 35, 376–385. doi: 10.1111/j.1530-0277.2010.01353.x
Hagelberg, N., Forssell, H., Aalto, S., Rinne, J. O., Scheinin, H., Taiminen, T., et al. (2003). Altered dopamine D2 receptor binding in atypical facial pain. Pain 106, 43–48. doi: 10.1016/S0304-3959(03)00275-6
Hagelberg, N., Jaaskelainen, S. K., Martikainen, I. K., Mansikka, H., Forssell, H., Scheinin, H., et al. (2004). Striatal dopamine D2 receptors in modulation of pain in humans: a review. Eur. J. Pharmacol. 500, 187–192. doi: 10.1016/j.ejphar.2004.07.024
Hahn, W.-H., Suh, J.-S., and Cho, B.-S. (2011). Linkage and association study of neurotrophins and their receptors as novel susceptibility genes for childhood IgA nephropathy. Pediatr. Res. 69, 299–305. doi: 10.1203/PDR.0b013e31820b9365
Haus, E., Sackett-Lundeen, L., and Smolensky, M. H. (2012). Rheumatoid arthritis: circadian rhythms in disease activity, signs and symptoms, and rationale for chronotherapy with corticosteroids and other medications. Bull. NYU Hosp. Jt. Dis. 70(Suppl. 1), 3–10.
He, G.-H., Cai, W.-K., Zhang, J.-B., Ma, C.-Y., Yan, F., Lu, J., et al. (2016). Associations of polymorphisms in HRH2, HRH3, DAO, and HNMT genes with risk of chronic heart failure. Biomed. Res. Int. 2016:1208476. doi: 10.1155/2016/1208476
Herman, A. I., and Balogh, K. N. (2012). Polymorphisms of the serotonin transporter and receptor genes: susceptibility to substance abuse. Subst. Abuse Rehabil. 3, 49–57. doi: 10.2147/SAR.S25864
Hillard, C. J., Weinlander, K. M., and Stuhr, K. L. (2012). Contributions of endocannabinoid signaling to psychiatric disorders in humans: genetic and biochemical evidence. Neuroscience 204, 207–229. doi: 10.1016/j.neuroscience.2011.11.020
Ho, A. M., Tang, N. L., Cheung, B. K., and Stadlin, A. (2008). Dopamine receptor D4 gene -521C/T polymorphism is associated with opioid dependence through cold-pain responses. Ann. N. Y. Acad. Sci. 1139, 20–26. doi: 10.1196/annals.1432.054
Hocking, L. J., Smith, B. H., Jones, G. T., Reid, D. M., Strachan, D. P., and Macfarlane, G. J. (2010). Genetic variation in the beta2-adrenergic receptor but not catecholamine-O-methyltransferase predisposes to chronic pain: results from the 1958 British Birth Cohort study. Pain 149, 143–151. doi: 10.1016/j.pain.2010.01.023
Hogart, A., Nagarajan, R. P., Patzel, K. A., Yasui, D. H., and Lasalle, J. M. (2007). 15q11-13 GABAA receptor genes are normally biallelically expressed in brain yet are subject to epigenetic dysregulation in autism-spectrum disorders. Hum. Mol. Genet. 16, 691–703. doi: 10.1093/hmg/ddm014
Hohmann, S. W., Angioni, C., Tunaru, S., Lee, S., Woolf, C. J., Offermanns, S., et al. (2017). The G2A receptor (GPR132) contributes to oxaliplatin-induced mechanical pain hypersensitivity. Sci. Rep. 7:446. doi: 10.1038/s41598-017-00591-0
Hough, L. B., and Rice, F. L. (2011). H3 receptors and pain modulation: peripheral, spinal, and brain interactions. J. Pharmacol. Exp. Ther. 336, 30–37. doi: 10.1124/jpet.110.171264
Hu, Y., Tang, W., Liu, R., Dong, Z., Chen, X., Pan, M., et al. (2014). Higher prevalence of migraine in essential tremor: a case-control study. Cephalalgia 34, 1142–1149. doi: 10.1177/0333102414531153
Huang, L., Adachi, N., Nagaro, T., Liu, K., and Arai, T. (2007). Histaminergic involvement in neuropathic pain produced by partial ligation of the sciatic nerve in rats. Reg. Anesth Pain. Med. 32, 124–129. doi: 10.1097/00115550-200703000-00006
Hulse, R. P., Donaldson, L. F., and Wynick, D. (2012). Peripheral galanin receptor 2 as a target for the modulation of pain. Pain Res. Treat. 2012, 545386. doi: 10.1155/2012/545386
Hutchinson, M. R., Zhang, Y., Shridhar, M., Evans, J. H., Buchanan, M. M., Zhao, T. X., et al. (2010). Evidence that opioids may have toll-like receptor 4 and MD-2 effects. Brain Behav. Immun. 24, 83–95. doi: 10.1016/j.bbi.2009.08.004
Hwang, I. C., Park, J. Y., Myung, S. K., Ahn, H. Y., Fukuda, K., and Liao, Q. (2014). OPRM1 A118G gene variant and postoperative opioid requirement: a systematic review and meta-analysis. Anesthesiology 121, 825–834. doi: 10.1097/ALN.0000000000000405
Ide, S., Nishizawa, D., Fukuda, K.-I., Kasai, S., Hasegawa, J., Hayashida, M., et al. (2014). Haplotypes of P2RX7 gene polymorphisms are associated with both cold pain sensitivity and analgesic effect of fentanyl. Mol. Pain 10:75. doi: 10.1186/1744-8069-10-75
Jaaskelainen, S. K., Lindholm, P., Valmunen, T., Pesonen, U., Taiminen, T., Virtanen, A., et al. (2014). Variation in the dopamine D2 receptor gene plays a key role in human pain and its modulation by transcranial magnetic stimulation. Pain 155, 2180–2187. doi: 10.1016/j.pain.2014.08.029
James, S. (2013). Human pain and genetics: some basics. Br. J. Pain 7, 171–178. doi: 10.1177/2049463713506408
Jayamanne, A., Greenwood, R., Mitchell, V. A., Aslan, S., Piomelli, D., and Vaughan, C. W. (2006). Actions of the FAAH inhibitor URB597 in neuropathic and inflammatory chronic pain models. Br. J. Pharmacol. 147, 281–288. doi: 10.1038/sj.bjp.0706510
Jhun, E., He, Y., Yao, Y., Molokie, R. E., Wilkie, D. J., and Wang, Z. J. (2014). Dopamine D3 receptor Ser9Gly and catechol-o-methyltransferase Val158Met polymorphisms and acute pain in sickle cell disease. Anesth. Analg. 119, 1201–1207. doi: 10.1213/ANE.0000000000000382
Jhun, E. H., Yao, Y., He, Y., Mack, A. K., Wilkie, D. J., Molokie, R. E., et al. (2015). Prevalence of pain-related single nucleotide polymorphisms in patients of African origin with sickle cell disease. Pharmacogenomics 16, 1795–1806. doi: 10.2217/pgs.15.126
Jurečeková, J., Babušíková, E., Kmet’ová, M., Kliment, J., and Dobrota, D. (2015). Estrogen receptor alpha polymorphisms and the risk of prostate cancer development. J. Cancer Res. Clin. Oncol. 141, 1963–1971. doi: 10.1007/s00432-015-1966-6
Katusic, Z. S., Stelter, A., and Milstien, S. (1998). Cytokines stimulate GTP cyclohydrolase I gene expression in cultured human umbilical vein endothelial cells. Arterioscler. Thromb. Vasc. Biol. 18, 27–32. doi: 10.1161/01.ATV.18.1.27
Kaunisto, M. A., Jokela, R., Tallgren, M., Kambur, O., Tikkanen, E., Tasmuth, T., et al. (2013). Pain in 1000 women treated for breast cancer: a prospective study of pain sensitivity and postoperative pain. Anesthesiology 119, 1410–1421. doi: 10.1097/ALN.0000000000000012
Khalil, H., Sereika, S. M., Dai, F., Alexander, S., Conley, Y., Gruen, G., et al. (2017). OPRM1 and COMT gene-gene interaction is associated with postoperative pain and opioid consumption after orthopedic trauma. Biol. Res. Nurs. 19, 170–179. doi: 10.1177/1099800416680474
Kim, H., Lee, H., Rowan, J., Brahim, J., and Dionne, R. A. (2006a). Genetic polymorphisms in monoamine neurotransmitter systems show only weak association with acute post-surgical pain in humans. Mol. Pain 2:24. doi: 10.1186/1744-8069-2-24
Kim, H., Mittal, D. P., Iadarola, M. J., and Dionne, R. A. (2006b). Genetic predictors for acute experimental cold and heat pain sensitivity in humans. J. Med. Genet. 43:e40. doi: 10.1136/jmg.2005.036079
Kim, H., Neubert, J. K., San Miguel, A., Xu, K., Krishnaraju, R. K., Iadarola, M. J., et al. (2004). Genetic influence on variability in human acute experimental pain sensitivity associated with gender, ethnicity and psychological temperament. Pain 109, 488–496. doi: 10.1016/j.pain.2004.02.027
Kim, H. Y. (2015). Phospholipids: a neuroinflammation emerging target. Nat. Chem. Biol. 11, 99–100. doi: 10.1038/nchembio.1740
Kim, J. I., Kim, J.-W., Park, J.-E., Park, S., Hong, S.-B., Han, D. H., et al. (2017). Association of the GRIN2B rs2284411 polymorphism with methylphenidate response in attention-deficit/hyperactivity disorder. J. Psychopharmacol. 31, 1070–1077. doi: 10.1177/0269881116667707
Klein, C. J., Wu, Y., Kilfoyle, D. H., Sandroni, P., Davis, M. D., Gavrilova, R. H., et al. (2013). Infrequent SCN9A mutations in congenital insensitivity to pain and erythromelalgia. J. Neurol. Neurosurg. Psychiatry 84, 386–391. doi: 10.1136/jnnp-2012-303719
Klepstad, P., Rakvag, T. T., Kaasa, S., Holthe, M., Dale, O., Borchgrevink, P. C., et al. (2004). The 118 A > G polymorphism in the human micro-opioid receptor gene may increase morphine requirements in patients with pain caused by malignant disease. Acta Anaesthesiol. Scand. 48, 1232–1239. doi: 10.1111/j.1399-6576.2004.00517.x
Ko, S. W., Vadakkan, K. I., Ao, H., Gallitano-Mendel, A., Wei, F., Milbrandt, J., et al. (2005). Selective contribution of Egr1 (zif/268) to persistent inflammatory pain. J. Pain 6, 12–20. doi: 10.1016/j.jpain.2004.10.001
Kooloos, W. M., Wessels, J. A., Van Der Straaten, T., Allaart, C. F., Huizinga, T. W., and Guchelaar, H. J. (2010). Functional polymorphisms and methotrexate treatment outcome in recent-onset rheumatoid arthritis. Pharmacogenomics 11, 163–175. doi: 10.2217/pgs.09.139
Kringel, D., and Lötsch, J. (2015). Pain research funding by the european union seventh framework programme. Eur. J. Pain 19, 595–600. doi: 10.1002/ejp.690
Kringel, D., Sisignano, M., Zinn, S., and Lötsch, J. (2017). Next-generation sequencing of the human TRPV1 gene and the regulating co-players LTB4R and LTB4R2 based on a custom AmpliSeq panel. PLoS One 12:e0180116. doi: 10.1371/journal.pone.0180116
Kumar, D., Chakraborty, J., and Das, S. (2012). Epistatic effects between variants of kappa-opioid receptor gene and A118G of mu-opioid receptor gene increase susceptibility to addiction in Indian population. Prog. Neuropsychopharmacol. Biol. Psychiatry 36, 225–230. doi: 10.1016/j.pnpbp.2011.10.018
Kumar, V., and Mahal, B. A. (2012). NGF – The TrkA to successful pain treatment. J. Pain Res. 5, 279–287. doi: 10.2147/JPR.S33408
Kurita, G. P., Ekholm, O., Kaasa, S., Klepstad, P., Skorpen, F., and Sjøgren, P. (2016). Genetic variation and cognitive dysfunction in opioid-treated patients with cancer. Brain Behav. 6:e00471. doi: 10.1002/brb3.471
Kwon, H. J., Jang, W.-C., and Lim, M. H. (2016). Association between RELN gene polymorphisms and attention deficit hyperactivity disorder in korean children. Psychiatry Investig. 13, 210–216. doi: 10.4306/pi.2016.13.2.210
Lacroix-Fralish, M. L., Ledoux, J. B., and Mogil, J. S. (2007). The pain genes database: an interactive web browser of pain-related transgenic knockout studies. Pain 131, e1–e4. doi: 10.1016/j.pain.2007.04.041
Lander, E. S., Linton, L. M., Birren, B., Nusbaum, C., Zody, M. C., Baldwin, J., et al. (2001). Initial sequencing and analysis of the human genome. Nature 409, 860–921. doi: 10.1038/35057062
Law, P. Y., Reggio, P. H., and Loh, H. H. (2013). Opioid receptors: toward separation of analgesic from undesirable effects. Trends Biochem. Sci. 38, 275–282. doi: 10.1016/j.tibs.2013.03.003
Ledergerber, C., and Dessimoz, C. (2011). Base-calling for next-generation sequencing platforms. Brief. Bioinform. 12, 489–497. doi: 10.1093/bib/bbq077
Lee, M., and Tracey, I. (2013). Neuro-genetics of persistent pain. Curr. Opin. Neurobiol. 23, 127–132. doi: 10.1016/j.conb.2012.11.007
Leung, L., and Cahill, C. M. (2010). TNF-alpha and neuropathic pain–a review. J. Neuroinflamm. 7:27. doi: 10.1186/1742-2094-7-27
Li, J. Y., Tao, F., Wu, X. X., Tan, Y. Z., He, L., and Lu, H. (2015). Polymorphic variations in manganese superoxide dismutase (MnSOD) and endothelial nitric oxide synthase (eNOS) genes contribute to the development of type 2 diabetes mellitus in the Chinese Han population. Genet. Mol. Res. 14, 12993–13002. doi: 10.4238/2015.October.21.20
Li, T., Zhao, J., Yang, J., Ma, X., Dai, Q., Huang, H., et al. (2016). A Meta-Analysis of the association between ESR1 genetic variants and the risk of breast cancer. PLoS One 11:e0153314. doi: 10.1371/journal.pone.0153314
Lin, C.-H., Chaudhuri, K. R., Fan, J.-Y., Ko, C.-I., Rizos, A., Chang, C.-W., et al. (2017). Depression and Catechol-O-methyltransferase (COMT) genetic variants are associated with pain in Parkinson’s disease. Sci. Rep. 7:6306. doi: 10.1038/s41598-017-06782-z
Lindstedt, F., Karshikoff, B., Schalling, M., Olgart Hoglund, C., Ingvar, M., Lekander, M., et al. (2012). Serotonin-1A receptor polymorphism (rs6295) associated with thermal pain perception. PLoS One 7:e43221. doi: 10.1371/journal.pone.0043221
Lippmann, C., Kringel, D., Ultsch, A., and Lötsch, J. (2018). Computational functional genomics-based approaches in analgesic drug discovery and repurposing. Pharmacogenomics 19, 783–797. doi: 10.2217/pgs-2018-0036
Liu, J., Shi, Y., Tang, J., Guo, T., Li, X., Yang, Y., et al. (2005). SNPs and haplotypes in the S100B gene reveal association with schizophrenia. Biochem. Biophys. Res. Commun. 328, 335–341. doi: 10.1016/j.bbrc.2004.12.175
Liu, Y. N., Yang, X., Suo, Z. W., Xu, Y. M., and Hu, X. D. (2014). Fyn kinase-regulated NMDA receptor- and AMPA receptor-dependent pain sensitization in spinal dorsal horn of mice. Eur. J. Pain 18, 1120–1128. doi: 10.1002/j.1532-2149.2014.00455.x
Loggia, M. L., Chonde, D. B., Akeju, O., Arabasz, G., Catana, C., Edwards, R. R., et al. (2015). Evidence for brain glial activation in chronic pain patients. Brain 138, 604–615. doi: 10.1093/brain/awu377
Lohmann, K., and Klein, C. (2014). Next generation sequencing and the future of genetic diagnosis. Neurotherapeutics 11, 699–707. doi: 10.1007/s13311-014-0288-8
Loncar, Z., Curic, G., Mestrovic, A. H., Mickovic, V., and Bilic, M. (2013). Do IL-1B and IL-1RN modulate chronic low back pain in patients with post-traumatic stress disorder? Collegium Antropol. 37, 1237–1244.
Lötsch, J., Doehring, A., Mogil, J. S., Arndt, T., Geisslinger, G., and Ultsch, A. (2013). Functional genomics of pain in analgesic drug development and therapy. Pharmacol. Ther. 139, 60–70. doi: 10.1016/j.pharmthera.2013.04.004
Lötsch, J., Fluhr, K., Neddermayer, T., Doehring, A., and Geisslinger, G. (2009a). The consequence of concomitantly present functional genetic variants for the identification of functional genotype-phenotype associations in pain. Clin. Pharmacol. Ther. 85, 25–30. doi: 10.1038/clpt.2008.103
Lötsch, J., Geisslinger, G., and Tegeder, I. (2009b). Genetic modulation of the pharmacological treatment of pain. Pharmacol. Ther. 124, 168–184. doi: 10.1016/j.pharmthera.2009.06.010
Lötsch, J., Von Hentig, N., Freynhagen, R., Griessinger, N., Zimmermann, M., Doehring, A., et al. (2009c). Cross-sectional analysis of the influence of currently known pharmacogenetic modulators on opioid therapy in outpatient pain centers. Pharmacogenet. Genom. 19, 429–436. doi: 10.1097/FPC.0b013e32832b89da
Lötsch, J., and Geisslinger, G. (2005). Are mu-opioid receptor polymorphisms important for clinical opioid therapy? Trends Mol. Med. 11, 82–89. doi: 10.1016/j.molmed.2004.12.006
Lötsch, J., and Geisslinger, G. (2010). A critical appraisal of human genotyping for pain therapy. Trends Pharmacol. Sci. 31, 312–317. doi: 10.1016/j.tips.2010.04.002
Lötsch, J., Klepstad, P., Doehring, A., and Dale, O. (2010). A GTP cyclohydrolase 1 genetic variant delays cancer pain. Pain 148, 103–106. doi: 10.1016/j.pain.2009.10.021
Lötsch, J., Sipilä, R., Tasmuth, T., Kringel, D., Estlander, A. M., Meretoja, T., et al. (2018). Machine-learning-derived classifier predicts absence of persistent pain after breast cancer surgery with high accuracy. Breast Cancer Res./Treatment. [Epub ahead of print]. doi: 10.1007/s10549-018-4841-8
Lötsch, J., Skarke, C., Grosch, S., Darimont, J., Schmidt, H., and Geisslinger, G. (2002). The polymorphism A118G of the human mu-opioid receptor gene decreases the pupil constrictory effect of morphine-6-glucuronide but not that of morphine. Pharmacogenetics 12, 3–9. doi: 10.1097/00008571-200201000-00002
Lötsch, J., Stuck, B., and Hummel, T. (2006). The human mu-opioid receptor gene polymorphism 118A > G decreases cortical activation in response to specific nociceptive stimulation. Behav. Neurosci. 120, 1218–1224. doi: 10.1037/0735-7044.120.6.1218
Lu, H., Zhu, L., Lian, L., Chen, M., Shi, D., and Wang, K. (2015). Genetic variations in the PRKCG gene and osteosarcoma risk in a Chinese population: a case-control study. Tumour Biol. 36, 5241–5247. doi: 10.1007/s13277-015-3182-z
Maertens, O., De Schepper, S., Vandesompele, J., Brems, H., Heyns, I., Janssens, S., et al. (2007). Molecular dissection of isolated disease features in mosaic neurofibromatosis type 1. Am. J. Hum. Genet. 81, 243–251. doi: 10.1086/519562
Mansour, A. R., Farmer, M. A., Baliki, M. N., and Apkarian, A. V. (2014). Chronic pain: the role of learning and brain plasticity. Restor. Neurol. Neurosci. 32, 129–139.
Marcos, M., Pastor, I., De La Calle, C., Barrio-Real, L., Laso, F.-J., and González-Sarmiento, R. (2012). Cannabinoid receptor 1 gene is associated with alcohol dependence. Alcohol. Clin. Exp. Res. 36, 267–271. doi: 10.1111/j.1530-0277.2011.01623.x
Martikainen, I. K., Nuechterlein, E. B., Pecina, M., Love, T. M., Cummiford, C. M., Green, C. R., et al. (2015). Chronic back pain is associated with alterations in dopamine neurotransmission in the ventral striatum. J. Neurosci. 35, 9957–9965. doi: 10.1523/JNEUROSCI.4605-14.2015
Maxwell, J. R., Potter, C., Hyrich, K. L., Barton, A., Worthington, J., Isaacs, J. D., et al. (2008). Association of the tumour necrosis factor-308 variant with differential response to anti-TNF agents in the treatment of rheumatoid arthritis. Hum. Mol. Genet. 17, 3532–3538. doi: 10.1093/hmg/ddn245
McIntosh, A. M., Simen, A. A., Evans, K. L., Hall, J., Macintyre, D. J., Blackwood, D., et al. (2012). Genetic variation in hyperpolarization-activated cyclic nucleotide-gated channels and its relationship with neuroticism, cognition and risk of depression. Front. Genet. 3:116. doi: 10.3389/fgene.2012.00116
Mead, S., Poulter, M., Uphill, J., Beck, J., Whitfield, J., Webb, T. E. F., et al. (2009). Genetic risk factors for variant Creutzfeldt-Jakob disease: a genome-wide association study. Lancet Neurol. 8, 57–66. doi: 10.1016/S1474-4422(08)70265-5
Metzker, M. L. (2010). Sequencing technologies – The next generation. Nat. Rev. Genet. 11, 31–46. doi: 10.1038/nrg2626
Mill, J., Kiss, E., Baji, I., Kapornai, K., Daróczy, G., Vetró, A., et al. (2008). Association study of the estrogen receptor alpha gene (ESR1) and childhood-onset mood disorders. Am. J. Med. Genet. B Neuropsychiatr. Genet. 147B, 1323–1326. doi: 10.1002/ajmg.b.30751
Milne, R. L., Burwinkel, B., Michailidou, K., Arias-Perez, J.-I., Zamora, M. P., Menéndez-Rodríguez, P., et al. (2014). Common non-synonymous SNPs associated with breast cancer susceptibility: findings from the Breast Cancer association consortium. Hum. Mol. Genet. 23, 6096–6111. doi: 10.1093/hmg/ddu311
Mitra, A. K., Crews, K., Pounds, S., Cao, X., Downing, J. R., Raimondi, S., et al. (2011). Impact of genetic variation in FKBP5 on clinical response in pediatric acute myeloid leukemia patients: a pilot study. Leukemia 25, 1354–1356. doi: 10.1038/leu.2011.74
Mocellin, S., Marincola, F., Rossi, C. R., Nitti, D., and Lise, M. (2004). The multifaceted relationship between IL-10 and adaptive immunity: putting together the pieces of a puzzle. Cytokine Growth. Factor. Rev. 15, 61–76. doi: 10.1016/j.cytogfr.2003.11.001
Mogil, J. S. (2009). Are we getting anywhere in human pain genetics? Pain 146, 231–232. doi: 10.1016/j.pain.2009.07.023
Mogil, J. S. (2012). Pain genetics: past, present and future. Trends Genet. 28, 258–266. doi: 10.1016/j.tig.2012.02.004
Mustafa, A. E., Faquih, T., Baz, B., Kattan, R., Al-Issa, A., Tahir, A. I., et al. (2018). Validation of ion torrent(TM) inherited disease panel with the PGM(TM) sequencing platform for rapid and comprehensive mutation detection. Genes (Basel.) 9, 267. doi: 10.3390/genes9050267
Nackley, A. G., Shabalina, S. A., Tchivileva, I. E., Satterfield, K., Korchynskyi, O., Makarov, S. S., et al. (2006). Human catechol-O-methyltransferase haplotypes modulate protein expression by altering mRNA secondary structure. Science 314, 1930–1933. doi: 10.1126/science.1131262
Naumov, D. E., Perelman, J. M., Kolosov, V. P., Potapova, T. A., Maksimov, V. N., and Zhou, X. (2015). Transient receptor potential melastatin 8 gene polymorphism is associated with cold-induced airway hyperresponsiveness in bronchial asthma. Respirology 20, 1192–1197. doi: 10.1111/resp.12605
Nemeth, E., Rivera, S., Gabayan, V., Keller, C., Taudorf, S., Pedersen, B. K., et al. (2004). IL-6 mediates hypoferremia of inflammation by inducing the synthesis of the iron regulatory hormone hepcidin. J. Clin. Invest. 113, 1271–1276. doi: 10.1172/JCI200420945
Nicholl, B. I., Holliday, K. L., Macfarlane, G. J., Thomson, W., Davies, K. A., O’neill, T. W., et al. (2011). Association of HTR2A polymorphisms with chronic widespread pain and the extent of musculoskeletal pain: results from two population-based cohorts. Arthritis Rheum. 63, 810–818. doi: 10.1002/art.30185
Nissenbaum, J., Devor, M., Seltzer, Z., Gebauer, M., Michaelis, M., Tal, M., et al. (2010). Susceptibility to chronic pain following nerve injury is genetically affected by CACNG2. Genome Res. 20, 1180–1190. doi: 10.1101/gr.104976.110
Nöthen, M. M., Cichon, S., Hemmer, S., Hebebrand, J., Remschmidt, H., Lehmkuhl, G., et al. (1994). Human dopamine D4 receptor gene: frequent occurrence of a null allele and observation of homozygosity. Hum. Mol. Genet. 3, 2207–2212. doi: 10.1093/hmg/3.12.2207
Obata, K., and Noguchi, K. (2006). BDNF in sensory neurons and chronic pain. Neurosci. Res. 55, 1–10. doi: 10.1016/j.neures.2006.01.005
Oertel, B. G., Doehring, A., Roskam, B., Kettner, M., Hackmann, N., Ferreiros, N., et al. (2012). Genetic-epigenetic interaction modulates mu-opioid receptor regulation. Hum. Mol. Genet. 21, 4751–4760. doi: 10.1093/hmg/dds314
Oertel, B. G., Schmidt, R., Schneider, A., Geisslinger, G., and Lötsch, J. (2006). The mu-opioid receptor gene polymorphism 118A>G depletes alfentanil-induced analgesia and protects against respiratory depression in homozygous carriers. Pharmacogenet. Genom. 16, 625–636. doi: 10.1097/01.fpc.0000220566.90466.a2
Offenbaecher, M., Bondy, B., De Jonge, S., Glatzeder, K., Kruger, M., Schoeps, P., et al. (1999). Possible association of fibromyalgia with a polymorphism in the serotonin transporter gene regulatory region. Arthritis Rheum. 42, 2482–2488. doi: 10.1002/1529-0131(199911)42:11<2482::AID-ANR27>3.0.CO;2-B
Onaya, T., Ishii, M., Katoh, H., Shimizu, S., Kasai, H., Kawamura, M., et al. (2013). Predictive index for the onset of medication overuse headache in migraine patients. Neurol. Sci. 34, 85–92. doi: 10.1007/s10072-012-0955-7
Onojjighofia, T., Meshkin, B., Nguyen, S. V., Schwartz, D., and Akindele, B. (2014). Perception of analgesia in narcotic users with chronic pain: a multi-center cross-sectional study comparing genotype to pain VAS (P.A.I.N. Study). Neurology 82:E39.
Oyanguren-Desez, O., Rodríguez-Antigüedad, A., Villoslada, P., Domercq, M., Alberdi, E., and Matute, C. (2011). Gain-of-function of P2X7 receptor gene variants in multiple sclerosis. Cell Calcium 50, 468–472. doi: 10.1016/j.ceca.2011.08.002
Palmirotta, R., Barbanti, P., De Marchis, M. L., Egeo, G., Aurilia, C., Fofi, L., et al. (2015). Is SOD2 Ala16Val polymorphism associated with migraine with aura phenotype? Antioxid. Redox Signal. 22, 275–279. doi: 10.1089/ars.2014.6069
Park, J. J., Lee, J., Kim, M. A., Back, S. K., Hong, S. K., and Na, H. S. (2007). Induction of total insensitivity to capsaicin and hypersensitivity to garlic extract in human by decreased expression of TRPV1. Neurosci. Lett. 411, 87–91. doi: 10.1016/j.neulet.2006.10.046
Peiro, A. M., Planelles, B., Juhasz, G., Bagdy, G., Libert, F., Eschalier, A., et al. (2016). Pharmacogenomics in pain treatment. Drug Metab. Pers Ther. 31, 131–142. doi: 10.1515/dmpt-2016-0005
Pelletier, C., and Weidhaas, J. B. (2010). MicroRNA binding site polymorphisms as biomarkers of cancer risk. Expert. Rev. Mol. Diagn. 10, 817–829. doi: 10.1586/erm.10.59
Petrenko, A. B., Yamakura, T., Baba, H., and Shimoji, K. (2003). The role of N-methyl-D-aspartate (NMDA) receptors in pain: a review. Anesth. Analg. 97, 1108–1116. doi: 10.1213/01.ANE.0000081061.12235.55
Potapova, T. A., Babenko, V. N., Kobzev, V. F., Romashchenko, A. G., Maksimov, V. N., and Voevoda, M. I. (2014). Associations of cold receptor TRPM8 gene single nucleotide polymorphism with blood lipids and anthropometric parameters in Russian population. Bull. Exp. Biol. Med. 157, 757–761. doi: 10.1007/s10517-014-2660-4
Potvin, S., Larouche, A., Normand, E., De Souza, J. B., Gaumond, I., Grignon, S., et al. (2009). DRD3 Ser9Gly polymorphism is related to thermal pain perception and modulation in chronic widespread pain patients and healthy controls. J. Pain 10, 969–975. doi: 10.1016/j.jpain.2009.03.013
Prasad, H. C., Steiner, J. A., Sutcliffe, J. S., and Blakely, R. D. (2009). Enhanced activity of human serotonin transporter variants associated with autism. Philos. Trans. R. Soc. Lond. B Biol. Sci. 364, 163–173. doi: 10.1098/rstb.2008.0143
R Development Core Team (2008). R: A Language and Environment for Statistical Computing. Vienna: R Development Core Team.
Rakvag, T. T., Klepstad, P., Baar, C., Kvam, T. M., Dale, O., Kaasa, S., et al. (2005). The Val158Met polymorphism of the human catechol-O-methyltransferase (COMT) gene may influence morphine requirements in cancer pain patients. Pain 116, 73–78. doi: 10.1016/j.pain.2005.03.032
Reimann, F., Cox, J. J., Belfer, I., Diatchenko, L., Zaykin, D. V., Mchale, D. P., et al. (2010). Pain perception is altered by a nucleotide polymorphism in SCN9A. Proc. Natl. Acad. Sci. U.S.A. 107, 5148–5153. doi: 10.1073/pnas.0913181107
Reyes-Gibby, C. C., Shete, S., Rakvag, T., Bhat, S. V., Skorpen, F., Bruera, E., et al. (2007). Exploring joint effects of genes and the clinical efficacy of morphine for cancer pain: OPRM1 and COMT gene. Pain 130, 25–30. doi: 10.1016/j.pain.2006.10.023
Ribeiro-Dasilva, M. C., Peres Line, S. R., Leme Godoy, Dos Santos, M. C., Arthuri, M. T., Hou, W., et al. (2009). Estrogen receptor-alpha polymorphisms and predisposition to TMJ disorder. J. Pain 10, 527–533. doi: 10.1016/j.jpain.2008.11.012
Rodrigues, P., De Marco, G., Furriol, J., Mansego, M. L., Pineda-Alonso, M., Gonzalez-Neira, A., et al. (2014). Oxidative stress in susceptibility to breast cancer: study in Spanish population. BMC Cancer 14:861. doi: 10.1186/1471-2407-14-861
Root, T. L., Szatkiewicz, J. P., Jonassaint, C. R., Thornton, L. M., Pinheiro, A. P., Strober, M., et al. (2011). Association of candidate genes with phenotypic traits relevant to anorexia nervosa. Eur. Eat Disord. Rev. 19, 487–493. doi: 10.1002/erv.1138
Sah, D. W., Ossipov, M. H., Rossomando, A., Silvian, L., and Porreca, F. (2005). New approaches for the treatment of pain: the GDNF family of neurotrophic growth factors. Curr. Top. Med. Chem. 5, 577–583. doi: 10.2174/1568026054367593
Sanger, F., and Coulson, A. R. (1975). A rapid method for determining sequences in DNA by primed synthesis with DNA polymerase. J. Mol. Biol. 94, 441–448. doi: 10.1016/0022-2836(75)90213-2
Sanger, F., Nicklen, S., and Coulson, A. R. (1977). DNA sequencing with chain-terminating inhibitors. Proc. Natl. Acad. Sci. U.S.A. 74, 5463–5467. doi: 10.1073/pnas.74.12.5463
Sato, M., Ohashi, J., Tsuchiya, N., Kashiwase, K., Ishikawa, Y., Arita, H., et al. (2002). Association of HLA-A∗3303-B∗4403-DRB1∗1302 haplotype, but not of TNFA promoter and NKp30 polymorphism, with postherpetic neuralgia (PHN) in the Japanese population. Genes Immun. 3, 477–481. doi: 10.1038/sj.gene.6363890
Savas, S., Schmidt, S., Jarjanazi, H., and Ozcelik, H. (2006). Functional nsSNPs from carcinogenesis-related genes expressed in breast tissue: potential breast cancer risk alleles and their distribution across human populations. Hum. Genomics 2, 287–296. doi: 10.1186/1479-7364-2-5-287
Schleinitz, D., Carmienke, S., Böttcher, Y., Tönjes, A., Berndt, J., Klöting, N., et al. (2010). Role of genetic variation in the cannabinoid type 1 receptor gene (CNR1) in the pathophysiology of human obesity. Pharmacogenomics 11, 693–702. doi: 10.2217/pgs.10.42
Schmidt, S., Hauser, M. A., Scott, W. K., Postel, E. A., Agarwal, A., Gallins, P., et al. (2006). Cigarette smoking strongly modifies the association of LOC387715 and age-related macular degeneration. Am. J. Hum. Genet. 78, 852–864. doi: 10.1086/503822
Schott, K., Gutlich, M., and Ziegler, I. (1993). Induction of GTP-cyclohydrolase I mRNA expression by lectin activation and interferon-gamma treatment in human cells associated with the immune response. J. Cell. Physiol. 156, 12–16. doi: 10.1002/jcp.1041560103
Schurks, M., Kurth, T., Buring, J. E., and Zee, R. Y. (2009). A candidate gene association study of 77 polymorphisms in migraine. J. Pain 10, 759–766. doi: 10.1016/j.jpain.2009.01.326
Schürks, M., Rist, P. M., and Kurth, T. (2010). Sex hormone receptor gene polymorphisms and migraine: a systematic review and meta-analysis. Cephalalgia 30, 1306–1328. doi: 10.1177/0333102410364155
Schütz, M., Oertel, B. G., Heimann, D., Doehring, A., Walter, C., Dimova, V., et al. (2014). Consequences of a human TRPA1 genetic variant on the perception of nociceptive and olfactory stimuli. PLoS One 9:e95592. doi: 10.1371/journal.pone.0095592
Schwartz, E. S., Kim, H. Y., Wang, J., Lee, I., Klann, E., Chung, J. M., et al. (2009). Persistent pain is dependent on spinal mitochondrial antioxidant levels. J. Neurosci. 29, 159–168. doi: 10.1523/JNEUROSCI.3792-08.2009
Schweinhardt, P., Sauro, K. M., and Bushnell, M. C. (2008). Fibromyalgia: a disorder of the brain? Neuroscientist 14, 415–421. doi: 10.1177/1073858407312521
Serý, O., Prikryl, R., Castulík, L., and St’astný, F. (2010). A118G polymorphism of OPRM1 gene is associated with schizophrenia. J. Mol. Neurosci. 41, 219–222. doi: 10.1007/s12031-010-9327-z
Shah, N. D., Shah, P. S., Panchal, Y. Y., Katudia, K. H., Khatri, N. B., Ray, H. S. P., et al. (2018). Mutation analysis of BRCA1/2 mutations with special reference to polymorphic SNPs in Indian breast cancer patients. Appl. Clin. Genet. 11, 59–67. doi: 10.2147/TACG.S155955
Sherry, S. T., Ward, M. H., Kholodov, M., Baker, J., Phan, L., Smigielski, E. M., et al. (2001). dbSNP: the NCBI database of genetic variation. Nucleic Acids Res. 29, 308–311. doi: 10.1093/nar/29.1.308
Shi, J., Badner, J. A., Hattori, E., Potash, J. B., Willour, V. L., Mcmahon, F. J., et al. (2008). Neurotransmission and bipolar disorder: a systematic family-based association study. Am. J. Med. Genet. B Neuropsychiatr. Genet. 147B, 1270–1277. doi: 10.1002/ajmg.b.30769
Shi, T.-J. S., Liu, S.-X. L., Hammarberg, H., Watanabe, M., Xu, Z.-Q. D., and Hökfelt, T. (2008). Phospholipase C{beta}3 in mouse and human dorsal root ganglia and spinal cord is a possible target for treatment of neuropathic pain. Proc. Natl. Acad. Sci. U.S.A. 105, 20004–20008. doi: 10.1073/pnas.0810899105
Shoskes, D. A., Albakri, Q., Thomas, K., and Cook, D. (2002). Cytokine polymorphisms in men with chronic prostatitis/chronic pelvic pain syndrome: association with diagnosis and treatment response. J. Urol. 168, 331–335. doi: 10.1016/S0022-5347(05)64916-6
Siqueira, E. C. D., De Sousa, S. F., França, J. A., Diniz, M. G., Pereira, T. D. S. F., Moreira, R. G., et al. (2017). Targeted next-generation sequencing of glandular odontogenic cyst: a preliminary study. Oral. Surg. Oral Med. Oral. Pathol. Oral Radiol. 124, 490–494. doi: 10.1016/j.oooo.2017.07.001
Skarke, C., Kirchhof, A., Geisslinger, G., and Lötsch, J. (2004). Comprehensive mu-opioid-receptor genotyping by pyrosequencing. Clin. Chem. 50, 640–644. doi: 10.1373/clinchem.2003.027607
Skarke, C., Kirchhof, A., Geisslinger, G., and Lötsch, J. (2005). Rapid genotyping for relevant CYP1A2 alleles by pyrosequencing. Eur. J. Clin. Pharmacol. 61, 887–892. doi: 10.1007/s00228-005-0029-3
Sluka, K. A., and Audette, K. M. (2006). Activation of protein kinase C in the spinal cord produces mechanical hyperalgesia by activating glutamate receptors, but does not mediate chronic muscle-induced hyperalgesia. Mol. Pain 2:13. doi: 10.1186/1744-8069-2-13
Smith, F. L., Fujimori, K., Lowe, J., and Welch, S. P. (1998). Characterization of delta9-tetrahydrocannabinol and anandamide antinociception in nonarthritic and arthritic rats. Pharmacol. Biochem. Behav. 60, 183–191. doi: 10.1016/S0091-3057(97)00583-2
Snider, W. D., and McMahon, S. B. (1998). Tackling pain at the source: new ideas about nociceptors. Neuron 20, 629–632. doi: 10.1016/S0896-6273(00)81003-X
Solus, J. F., Chung, C. P., Oeser, A., Li, C., Rho, Y. H., Bradley, K. M., et al. (2015). Genetics of serum concentration of IL-6 and TNFα in systemic lupus erythematosus and rheumatoid arthritis: a candidate gene analysis. Clin. Rheumatol. 34, 1375–1382. doi: 10.1007/s10067-015-2881-6
Somogyi, A. A., Coller, J. K., and Barratt, D. T. (2015). Pharmacogenetics of opioid response. Clin. Pharmacol. Ther. 97, 125–127. doi: 10.1002/cpt.23
Somogyi, A. A., Sia, A. T., Tan, E.-C., Coller, J. K., Hutchinson, M. R., and Barratt, D. T. (2016). Ethnicity-dependent influence of innate immune genetic markers on morphine PCA requirements and adverse effects in postoperative pain. Pain 157, 2458–2466. doi: 10.1097/j.pain.0000000000000661
Sonoda, T., Takada, J., Iba, K., Asakura, S., Yamashita, T., and Mori, M. (2012). Interaction between ESRα polymorphisms and environmental factors in osteoporosis. J. Orthop. Res. 30, 1529–1534. doi: 10.1002/jor.22083
Sorge, R. E., Trang, T., Dorfman, R., Smith, S. B., Beggs, S., Ritchie, J., et al. (2012). Genetically determined P2X7 receptor pore formation regulates variability in chronic pain sensitivity. Nat. Med. 18, 595–599. doi: 10.1038/nm.2710
Stephens, K., Cooper, B. A., West, C., Paul, S. M., Baggott, C. R., Merriman, J. D., et al. (2014). Associations between cytokine gene variations and severe persistent breast pain in women following breast cancer surgery. J. Pain 15, 169–180. doi: 10.1016/j.jpain.2013.09.015
Sugaya, K., Nishijima, S., Yamada, T., Miyazato, M., Hatano, T., and Ogawa, Y. (2002). Molecular analysis of adrenergic receptor genes and interleukin-4/interleukin-4 receptor genes in patients with interstitial cystitis. J. Urol. 168, 2668–2671. doi: 10.1016/S0022-5347(05)64241-3
Sun, Y.-G., Gracias, N. G., Drobish, J. K., Vasko, M. R., Gereau, R. W., and Chen, Z.-F. (2009). The c-kit signaling pathway is involved in the development of persistent pain. Pain 144, 178–186. doi: 10.1016/j.pain.2009.04.011
Tegeder, I., Costigan, M., Griffin, R. S., Abele, A., Belfer, I., Schmidt, H., et al. (2006). GTP cyclohydrolase and tetrahydrobiopterin regulate pain sensitivity and persistence. Nat. Med. 12, 1269–1277. doi: 10.1038/nm1490
Thuault, S. (2016). A peripheral messenger for chronic pain. Nat. Neurosci. 19:9. doi: 10.1038/nn.4217
Thulasiraman, K., and Swamy, M. N. S. (1992). Graphs : Theory and Algorithms. New York, NY: Wiley. doi: 10.1002/9781118033104
Tian, W., Fu, Y., Garcia-Elias, A., Fernández-Fernández, J. M., Vicente, R., Kramer, P. L., et al. (2009). A loss-of-function nonsynonymous polymorphism in the osmoregulatory TRPV4 gene is associated with human hyponatremia. Proc. Natl. Acad. Sci. U.S.A. 106, 14034–14039. doi: 10.1073/pnas.0904084106
Tsai, S.-J., Hong, C.-J., Cheng, C.-Y., Liao, D.-L., and Liou, Y.-J. (2007). Association study of polymorphisms in post-synaptic density protein 95 (PSD-95) with schizophrenia. J. Neural Transm. (Vienna) 114, 423–426. doi: 10.1007/s00702-006-0587-2
Tsantoulas, C., Mooney, E. R., and Mcnaughton, P. A. (2016). HCN2 ion channels: basic science opens up possibilities for therapeutic intervention in neuropathic pain. Biochem. J. 473, 2717–2736. doi: 10.1042/BCJ20160287
Tulah, A. S., Beghe, B., Barton, S. J., Holloway, J. W., and Sayers, I. (2012). Leukotriene B4 receptor locus gene characterisation and association studies in asthma. BMC Med. Genet. 13:110. doi: 10.1186/1471-2350-13-110
Ultsch, A., Kringel, D., Kalso, E., Mogil, J. S., and Lötsch, J. (2016). A data science approach to candidate gene selection of pain regarded as a process of learning and neural plasticity. Pain 157, 2747–2757. doi: 10.1097/j.pain.0000000000000694
Ursu, D., Ebert, P., Langron, E., Ruble, C., Munsie, L., Zou, W., et al. (2014). Gain and loss of function of P2X7 receptors: mechanisms, pharmacology and relevance to diabetic neuropathic pain. Mol Pain 10:37. doi: 10.1186/1744-8069-10-37
Vaclavikova, E., Dvorakova, S., Skaba, R., Pos, L., Sykorova, V., Halkova, T., et al. (2014). RET variants and haplotype analysis in a cohort of Czech patients with Hirschsprung disease. PLoS One 9:e98957. doi: 10.1371/journal.pone.0098957
Vadakkan, K. I., Wang, H., Ko, S. W., Zastepa, E., Petrovic, M. J., Sluka, K. A., et al. (2006). Genetic reduction of chronic muscle pain in mice lacking calcium/calmodulin-stimulated adenylyl yclases. Mol. Pain 2:7. doi: 10.1186/1744-8069-2-7
Vassalli, P. (1992). The pathophysiology of tumor necrosis factors. Annu. Rev. Immunol. 10, 411–452. doi: 10.1146/annurev.iy.10.040192.002211
Venn, J. (1880). On the diagrammatic and mechanical representation of propositions and reasonings. Dublin Philos. Mag. J. Sci. 9, 1–18. doi: 10.1080/14786448008626877
Venter, J. C., Adams, M. D., Myers, E. W., Li, P. W., Mural, R. J., Sutton, G. G., et al. (2001). The sequence of the human genome. Science 291, 1304–1351. doi: 10.1126/science.1058040
Walker, K., Bowes, M., Panesar, M., Davis, A., Gentry, C., Kesingland, A., et al. (2001). Metabotropic glutamate receptor subtype 5 (mGlu5) and nociceptive function. I. Selective blockade of mGlu5 receptors in models of acute, persistent and chronic pain. Neuropharmacology 40, 1–9. doi: 10.1016/S0028-3908(00)00113-1
Walter, C., Doehring, A., Oertel, B. G., and Lötsch, J. (2013). μ-opioid receptor gene variant OPRM1 118 A>G: a summary of its molecular and clinical consequences for pain. Pharmacogenomics 14, 1915–1925. doi: 10.2217/pgs.13.187
Wang, C., Wang, H., Pang, J., Li, L., Zhang, S., Song, G., et al. (2014). Glial cell-derived neurotrophic factor attenuates neuropathic pain in a mouse model of chronic constriction injury: possible involvement of E-cadherin/p120ctn signaling. J. Mol. Neurosci. 54, 156–163. doi: 10.1007/s12031-014-0266-y
Wang, X.-S., Song, H.-B., Chen, S., Zhang, W., Liu, J.-Q., Huang, C., et al. (2015). Association of single nucleotide polymorphisms of ABCB1, OPRM1 and COMT with pain perception in cancer patients. J. Huazhong Univ. Sci. Technolog. Med. Sci. 35, 752–758. doi: 10.1007/s11596-015-1502-6
Wedrén, S., Lovmar, L., Humphreys, K., Magnusson, C., Melhus, H., Syvänen, A.-C., et al. (2008). Estrogen receptor alpha gene polymorphism and endometrial cancer risk–a case-control study. BMC Cancer 8:322. doi: 10.1186/1471-2407-8-322
Wellcome Trust, Case Control, and Consortium. (2007). Genome-wide association study of 14,000 cases of seven common diseases and 3,000 shared controls. Nature 447, 661–678. doi: 10.1038/nature05911
Wise, B. L., Demissie, S., Cupples, L. A., Felson, D. T., Yang, M., Shearman, A. M., et al. (2009). The relationship of estrogen receptor-alpha and -beta genes with osteoarthritis of the hand. J. Rheumatol. 36, 2772–2779. doi: 10.3899/jrheum.081208
Wolters, P. L., Burns, K. M., Martin, S., Baldwin, A., Dombi, E., Toledo-Tamula, M. A., et al. (2015). Pain interference in youth with neurofibromatosis type 1 and plexiform neurofibromas and relation to disease severity, social-emotional functioning, and quality of life. Am. J. Med. Genet. A 167A, 2103–2113. doi: 10.1002/ajmg.a.37123
Woo, J. H., Kim, H., Kim, J. H., and Kim, J. G. (2015). Cannabinoid receptor gene polymorphisms and bone mineral density in Korean postmenopausal women. Menopause 22, 512–519. doi: 10.1097/GME.0000000000000339
Yamamoto, M., Ito, Y., Mitsuma, N., Hattori, N., and Sobue, G. (2003). Pain-related differential expression of NGF, GDNF, IL-6, and their receptors in human vasculitic neuropathies. Intern. Med. 42, 1100–1103. doi: 10.2169/internalmedicine.42.1100
Yang, L., Gu, X., Zhang, W., Zhang, J., and Ma, Z. (2014). Cdk5 inhibitor roscovitine alleviates neuropathic pain in the dorsal root ganglia by downregulating N-methyl-D-aspartate receptor subunit 2A. Neurol. Sci. 35, 1365–1371. doi: 10.1007/s10072-014-1713-9
Yücel, Y., Coşkun, S., Cengiz, B., Özdemir, H. H., Uzar, E., Çim, A., et al. (2016). Association of polymorphisms within the serotonin receptor genes 5-HTR1A, 5-HTR1B, 5-HTR2A and 5-HTR2C and migraine susceptibility in a turkish population. Clin. Psychopharmacol. Neurosci. 14, 250–255. doi: 10.9758/cpn.2016.14.3.250
Yucesoy, B., Johnson, V. J., Lummus, Z. L., Kissling, G. E., Fluharty, K., Gautrin, D., et al. (2012). Genetic variants in antioxidant genes are associated with diisocyanate-induced asthma. Toxicol. Sci. 129, 166–173. doi: 10.1093/toxsci/kfs183
Zanette, S. A., Dussan-Sarria, J. A., Souza, A., Deitos, A., Torres, I. L. S., and Caumo, W. (2014). Higher serum S100B and BDNF levels are correlated with a lower pressure-pain threshold in fibromyalgia. Mol. Pain 10:46. doi: 10.1186/1744-8069-10-46
Zhang, L., Rao, F., Zhang, K., Khandrika, S., Das, M., Vaingankar, S. M., et al. (2007). Discovery of common human genetic variants of GTP cyclohydrolase 1 (GCH1) governing nitric oxide, autonomic activity, and cardiovascular risk. J. Clin. Invest. 117, 2658–2671. doi: 10.1172/JCI31093
Zinn S., Sisignano M., Kern K., Pierre S., Tunaru S., Jordan H., et al. (2017). The leukotriene B4 receptors BLT1 and BLT2 form an antagonistic sensitizing system in peripheral sensory neurons. J. Biol. Chem. 292, 6123–6134. doi: 10.1074/jbc.M116.769125
Keywords: pain, data science, knowledge discovery, functional genomics, next generation sequencing (NGS)
Citation: Kringel D, Kaunisto MA, Lippmann C, Kalso E and Lötsch J (2018) Development of an AmpliSeqTM Panel for Next-Generation Sequencing of a Set of Genetic Predictors of Persisting Pain. Front. Pharmacol. 9:1008. doi: 10.3389/fphar.2018.01008
Received: 24 May 2018; Accepted: 17 August 2018;
Published: 19 September 2018.
Edited by:
Ulrich M. Zanger, Dr. Margarete Fischer-Bosch-Institut für Klinische Pharmakologie (IKP), GermanyReviewed by:
Theodora Katsila, University of Patras, GreeceCopyright © 2018 Kringel, Kaunisto, Lippmann, Kalso and Lötsch. This is an open-access article distributed under the terms of the Creative Commons Attribution License (CC BY). The use, distribution or reproduction in other forums is permitted, provided the original author(s) and the copyright owner(s) are credited and that the original publication in this journal is cited, in accordance with accepted academic practice. No use, distribution or reproduction is permitted which does not comply with these terms.
*Correspondence: Jörn Lötsch, ai5sb2V0c2NoQGVtLnVuaS1mcmFua2Z1cnQuZGU=
Disclaimer: All claims expressed in this article are solely those of the authors and do not necessarily represent those of their affiliated organizations, or those of the publisher, the editors and the reviewers. Any product that may be evaluated in this article or claim that may be made by its manufacturer is not guaranteed or endorsed by the publisher.
Research integrity at Frontiers
Learn more about the work of our research integrity team to safeguard the quality of each article we publish.