- 1Beijing University of Chinese Medicine, Beijing, China
- 2Dalian Municipal Central Hospital Affiliated of Dalian Medical University, Dalian, China
- 3Dongfang Hospital, Beijing University of Chinese Medicine, Beijing, China
- 4Chaoyang Hospital, Capital Medical University, Beijing, China
A substantial knowledge on the pathogenesis of diabetes mellitus (DM) by oxidative stress and inflammation is available. Berberine is a biologically active botanical that can combat oxidative stress and inflammation and thus ameliorate DM, especially type 2 DM. This article describes the potential of berberine against oxidative stress and inflammation with special emphasis on its mechanistic aspects. In diabetic animal studies, the modified levels of proinflammatory cytokines and oxidative stress markers were observed after administering berberine. In renal, fat, hepatic, pancreatic and several others tissues, berberine-mediated suppression of oxidative stress and inflammation was noted. Berberine acted against oxidative stress and inflammation through a very complex mechanism consisting of several kinases and signaling pathways involving various factors, including NF-κB (nuclear factor-κB) and AMPK (AMP-activated protein kinases). Moreover, MAPKs (mitogen-activated protein kinases) and Nrf2 (nuclear factor erythroid-2 related factor 2) also have mechanistic involvement in oxidative stress and inflammation. In spite of above advancements, the mechanistic aspects of the inhibitory role of berberine against oxidative stress and inflammation in diabetes mellitus still necessitate additional molecular studies. These studies will be useful to examine the new prospects of natural moieties against DM.
Background
Diabetes mellitus, especially type 2 diabetes mellitus (T2DM), is a very distressing pathology throughout the world. In spite of the extensive research, the exact mode of the pathogenesis of T2DM is still unclear. Therefore, the investigators are actively attempting to explore the pathogenesis of T2DM, particularly development of T2DM through oxidative stress and inflammation (Evans et al., 2005; Donath and Shoelson, 2011; Mazidi et al., 2017).
The metabolic disorders could lead to oxidative stress, which harmfully affects the insulin activity (Bonnefont-Rousselot, 2002; Furukawa et al., 2004) through several interacting pathways (Alberici et al., 2011) and generating the reactive oxygen species (ROS) such as hydrogen peroxide and superoxide anions (Rosen et al., 2001). These species could deteriorate the islets β-cells of the pancreas resulting in the reduced release of insulin (Evans et al., 2003). Besides, several signaling pathways in cells, for instance, NF-κB (nuclear factor-κB) and PKC (protein kinase C), could also be activated by ROS. It could lead to interference with the insulin signaling pathways resulting in the development of insulin resistance (IR) (Scivittaro et al., 2000; Kaneto et al., 2002; Goldin et al., 2006).
One of the crucial risk factors of DM is inflammation (Donath and Shoelson, 2011; Xie and Du, 2011). The inflammatory condition triggers the development of IR and DM through a very complex mechanism consisting of several kinases and signaling pathways (Crook, 2004; Donath, 2013; Mahmoud and Al-Ozairi, 2013; Patel et al., 2013; Gratas-Delamarche et al., 2014). Mechanistically, the adipocytes and immunocytes produce various proinflammatory cytokines including IL-6 (interleukin-6) and TNF-α (tumor necrosis factor-α) that are involved in the pathogenesis of DM (Crook, 2004; Donath, 2013; Mahmoud and Al-Ozairi, 2013; Patel et al., 2013; Gratas-Delamarche et al., 2014). These cytokines are involved in the activation of the NF-κB pathway leading to serine phosphorylation of IRS (insulin receptor substrate) resulting in the IR (Mahmoud and Al-Ozairi, 2013; Patel et al., 2013). Additionally, DM is also induced by the islets β-cells dysfunctioning, caused by excessive IL-6 and TNF-α (Donath, 2013).
Many therapeutic moieties, both chemical and natural, are available for the management of T2DM (Yin et al., 2008; Hung et al., 2012; Patti et al., 2018). Berberine (Figure 1) is a bioactive botanical originated from Hydrastis canadensis and Coptis chinensis. It is an alkaloidal compound having a wide range of pharmacological activities (Yao et al., 2015; Zhang et al., 2015; Caliceti et al., 2016; Cicero and Baggioni, 2016; Imenshahidi and Hosseinzadeh, 2016; Cicero et al., 2017), due to its interaction with multiple proteins in the body (Figure 2). The animal and clinical studies have suggested the potential role of berberine in altering lipometabolism (Dong et al., 2012; Liu et al., 2013) and glycometabolism (Banach et al., 2018). Its major metabolites are berberrubine, thalifendine, demethyleneberberine, and jatrorrhizine (Dong et al., 2012, 2016; Yin et al., 2012; Zhang and Chen, 2012; Liu et al., 2013; Chang et al., 2016).
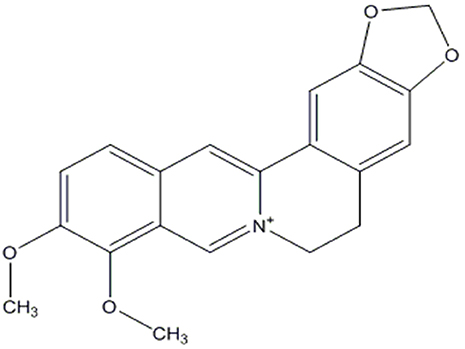
Figure 1. Chemical structure of berberine (Dong et al., 2012).
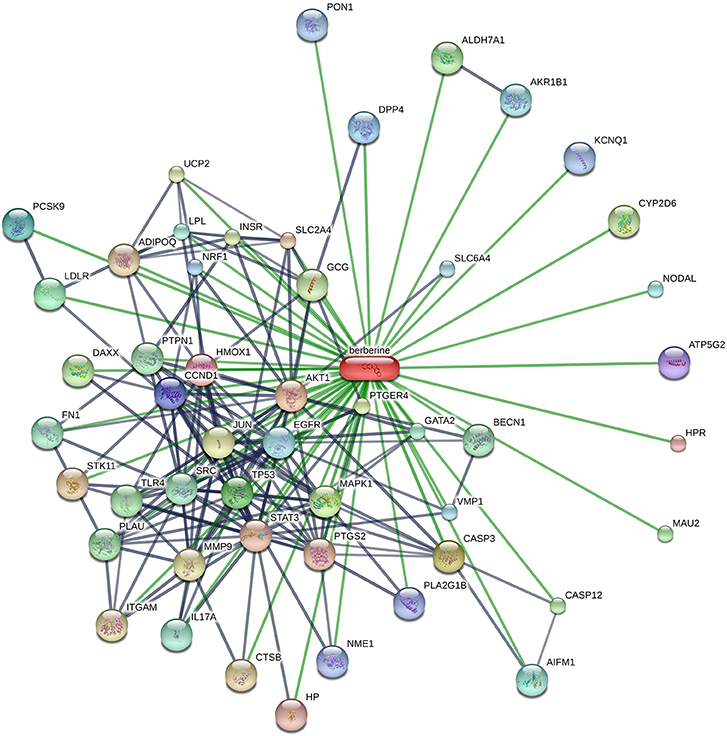
Figure 2. Schematic illustration of the protein networks of berberine and its interacting entities, acquired from STITCH database (accessed in March, 2017). Thicker lines represent the stronger linkages. Gray and green lines show the protein-protein interaction. [AKT1, v-akt murine thymoma viral oncogene homolog 1; CASP3, caspase 3; MAPK1, mitogen-activated protein kinase 1; TP53, tumor protein p53; LDLR, low density lipoprotein receptor; PCSK9, proprotein convertase subtilisin/kexin type 9; DPP4, dipeptidyl-peptidase 4; CCND1, cyclin D1; ATP5G2, ATP synthase; HMOX1, heme oxygenase (decycling) 1; HPR, haptoglobin-related protein; HP, Haptoglobin; STAT3, signal transducer and activator of transcription 3; PTGS2, prostaglandin-endoperoxide synthase 2; SLC2A4, solute carrier family 2 (facilitated glucose transporter), member 4; STK11, serine/threonine kinase 11; ADIPOQ, Adiponectin; CTSB, cathepsin B; ITGAM, integrin, alpha M; MMP9, matrix metallopeptidase 9; JUN, jun proto-oncogene; PTGER4, prostaglandin E receptor 4; UCP2, uncoupling protein 2; INSR, insulin receptor; CYP2D6, cytochrome P450 enzyme; GCG, glucagon; DAXX, death-domain associated protein; CASP12, caspase 12 (gene/pseudogene); ALDH7A1, aldehyde dehydrogenase 7 family, member A1; MAU2, MAU2 chromatid cohesion factor homolog; TLR4, toll-like receptor 4; PLAU, plasminogen activator, urokinase; PTPN1, protein tyrosine phosphatase, non-receptor type 1; BECN1, beclin 1, autophagy related; SRC, v-src sarcoma (Schmidt-Ruppin A-2) viral oncogene homolog (avian); FN1, fibronectin 1; GATA2, GATA binding protein 2; IL17A, interleukin 17A; LPL, lipoprotein lipase; AIFM1, apoptosis-inducing factor, mitochondrion-associated, 1; NODAL, nodal homolog; AKR1B1, aldo-keto reductase family 1, member B1 (aldose reductase); EGFR, epidermal growth factor receptor; VMP1, vacuole membrane protein 1; SLC6A4, solute carrier family 6 (neurotransmitter transporter, serotonin), member 4; NRF1, nuclear respiratory factor 1; PON1, paraoxonase 1; KCNQ1, potassium voltage-gated channel, KQT-like subfamily, member 1; and NME1, NME/NM23 nucleoside diphosphate kinase 1].
Owing to the excellent antidiabetic features (Pirillo and Catapano, 2015), the treatment efficacy of berberine has been found comparable with the reference antidiabetic drugs such as metformin (Zhang et al., 2010a, 2014; Liu et al., 2014, 2015; Xu et al., 2014; Goguet-Rubio et al., 2016). Berberine is also effective in combating diabetes-related pathologies (Lee et al., 2010) such as endothelial dysfunction, retinopathy (Tasdelen et al., 2013; Chang et al., 2015), nephropathy (Fu et al., 2016; Tang et al., 2016), and neuropathy (Ni et al., 2015). Based on the low toxicity and excellent efficacy of berberine (Zhang et al., 2010b; Ma et al., 2016), it has been suggested to be prescribed in the hepatic patients (Liu et al., 2013).
The ameliorated insulin sensitivity and the decrease in blood glucose level after administering berberine are attributed to the gut-microbiota modulation, islets β-cell regulation, activated AMPK (AMP-activated protein kinase), suppressed mitochondrial functions, and the upregulated insulin receptor expression (Hasan et al., 2015; Jiang et al., 2015; Lan et al., 2015; Suman et al., 2016). Current studies have reported the significance of berberine against oxidative stress and inflammation in cells, elaborating its vital role in DM. This review article summarizes the promising activities of berberine against oxidative stress and inflammation with special emphasis on its mechanistic aspects in the treatment of DM and IR.
Antioxidant Potential of Berberine and the Underlying Mechanisms in DM Treatment
Effect of Berberine on Oxidative Stress
Several studies (Table 1) have been conducted on animal models (Tang et al., 2006; Liu et al., 2008a, 2015; Zhou et al., 2009; Wang et al., 2011a; Zhou and Zhou, 2011; Lao-Ong et al., 2012; Wu et al., 2012; Chatuphonprasert et al., 2013; Xie et al., 2013a; Pang et al., 2015) and the cultured cells grown on high glucose-containing media to explore the antidiabetic effect pf berberine (Bhutada et al., 2011). These studies reveal that berberine possesses antioxidant feature since it promisingly inhibits oxidative stress, as evident from the altered levels of antioxidant enzymes and oxidative stress markers such as GSH (glutathione, a lipid oxidation product that is reduced in oxidative stress) and MDA (malondialdehyde that is increased in oxidative stress). Oxidative stress is characterized by lower levels of GSH (Moghaddam et al., 2013) but higher levels of MDA (Liu et al., 2008a). GSH possesses the antioxidant characteristics since it acts as a substrate of superoxide dismutase (SOD) and GSH-Px (glutathione peroxidase) enzymes and is involved in the process of peroxides scavenging (Moghaddam et al., 2013). First-line defense includes the antioxidant system that is involved in the maintenance of redox potential in the body. The performance of these antioxidants could be damaged in DM (Ceballos-Picot et al., 1996).
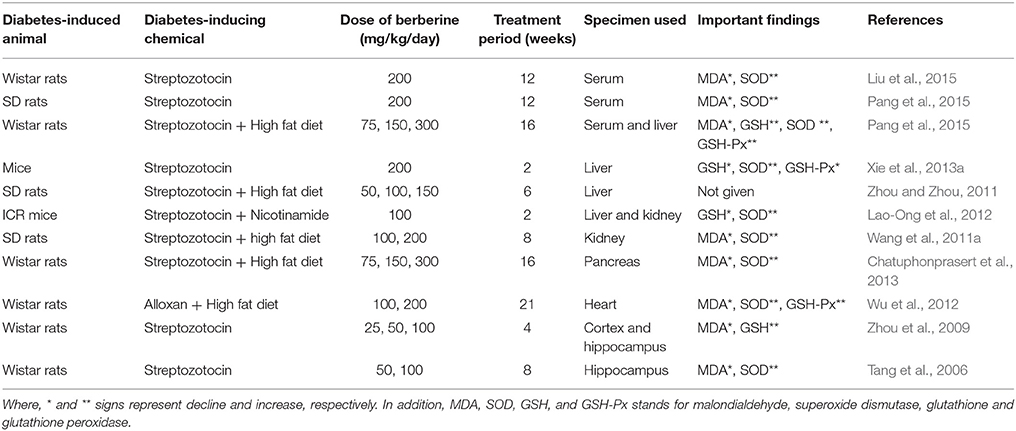
Table 1. The influence of orally administered berberine on the antioxidant parameters in diabetic rat/mice.
A large number of studies (Tang et al., 2006; Liu et al., 2008b, 2015; Zhou et al., 2009; Wang et al., 2011b; Zhou and Zhou, 2011; Lao-Ong et al., 2012; Wu et al., 2012; Chatuphonprasert et al., 2013; Xie et al., 2013b; Pang et al., 2015) have supported the antioxidant activity of berberine in the model animals with alloxan- or streptozotocin-triggered hyperglycemia (Table 1). In these studies, the modified levels of antioxidant enzymes and oxidative stress markers were used as the indicators of antioxidant potential of berberine. Overall, an elevated level of GSH, GSHPx, and SOD while the suppressed level of MDA has been observed in hyperglycemic animals fed on berberine. It combats oxidative stress via scavenging the needless free radicals (Ceballos-Picot et al., 1996; Liu et al., 2008b; Moghaddam et al., 2013). One of the studies (Xie et al., 2013b) reported that mice with streptozotocin-triggered hyperglycemia showed higher levels of GSH and GSHPx and reduced contents of SOD, likely owing to the development of oxidative stress (Maritim et al., 2003; Del Rio et al., 2005; Majithiya and Balaraman, 2005). On treating these diabetic mice with berberine, there was suppressed level of GSH and GSHPx and, on the other hand, upregulation of mRNA content of SOD in different tissues including serum, liver, kidney, pancreas, heart, cortex, and hippocampus. Conclusively, it can be stated that berberine is involved in the regulation of GSH/GSHPx antioxidant system in diabetic patients. Moreover, these studies depict an association between the antioxidant potential of berberine and its suppressive influence on developing DM and IR.
Antioxidant Mechanisms of Berberine Against Oxidative Stress
The available data on berberine has revealed the useful relationships between oxidative stress and different cellular pathways, as illustrated in Figure 3. In literature, an in vitro study conducted in alkaline DMSO (dimethyl sulfoxide) has revealed the scavenging of superoxide free radicals by berberine (Hill et al., 2000). In addition, berberine-mediated upregulation of mRNA content of SOD in diabetic mice also suppresses oxidative stress (Lao-Ong et al., 2012; Xie et al., 2013b). Sirtuin 1 (SIRT1) is a deacetylase that exhibits excellent antioxidant property. While, SIRT1 triggers deacetylation of FOXO (forkhead box O) factors as well as provokes transcription of FOXO target genes including SOD in oxidative stress (Hill et al., 2000). On the other hand, the expression level of SIRT1 is reported to be augmented under the effect of berberine (Mulder et al., 1996). Thus it can be extracted from this knowledge that SIRT1/FOXO pathway is involved in the berberine-mediated increase in SOD expression.
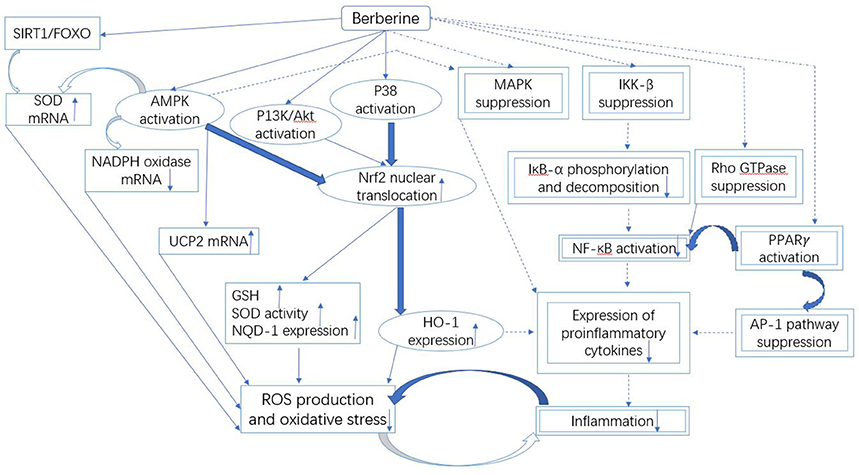
Figure 3. Mechanistic aspects of the antioxidant and anti-inflammatory action of berberine. It can be divided into three parts: Firstly, the downregulated NADPH oxidase expression and upregulated UCP and SOD could be involved in the berberine-induced suppression of oxidative stress that is likely controlled by the SIRT1/FOXO or AMPK pathways. Secondly, the antioxidant and anti-inflammatory action of berberine involves the activation of Nrf2 pathway, which further depends on the activated P38, AMPK and P13K/Akt signaling pathways. Finally, the inflammation is inhibited by berberine through the suppressed MAPK, Rho GTPase, NF-κB, and AP-1 pathways. The molecules and the pathways involved in the antioxidant activity of berberine are shown by the squared boxes/lines, while double squared boxes/dotted lines represent molecular species and pathways engaged in the anti-inflammatory activity. Additionally, the molecules and pathways shared by both antioxidant and anti-inflammatory activities of berberine are indicated by the encircled boxes. The pathways and the mechanisms that necessitate further investigations are shown by the curved bold lines. Berberine could terminate the malicious association between oxidative stress and inflammation.
Oxidative stress is reduced by berberine through the inhibition of expression of nicotinamide adenine dinucleotide phosphate (NADPH) oxidase also, which is a key origin of ROS (Shirwaikar et al., 2006; Zhu et al., 2013). The reason of this excessive generation of ROS could be NADPH oxidase-induced upregulation of high contents of various glycation products, fatty acids and glucose (Bonnefont-Rousselot, 2002; Furukawa et al., 2004; Xie et al., 2011). NADPH oxidase exists in different isoforms. NADPH oxidase 2/4 is the only isoform that is suppressed by berberine leading to the reduced generation of ROS (Shirwaikar et al., 2006; Zhu et al., 2013).
The onset of diabetes is related to activation of NADPH oxidase (Bonnefont-Rousselot, 2002; Furukawa et al., 2004; Xie et al., 2011), which is, therefore, a promising target for treating diabetes and comorbidities (Booth et al., 2016) such as nephropathy and neuropathy (van der Horst et al., 2004; Salminen et al., 2013). The inhibition of NADPH oxidase by berberine could suppress ROS production leading to the ameliorated effect on the diabetic condition (Zhu et al., 2013; Chang et al., 2016). On the other hand, the regulation of NADPH oxidase is negatively affected by activation of AMPK (AMP-activated protein kinase) (Sarna et al., 2010; Cheng et al., 2013), thus AMPK could be involved in the mechanism of berberine action against diabetes (Dong et al., 2016). However, it is not clear whether downregulation of NADPH oxidase by berberine occur through the activation of AMPK or some direct evidence is involved.
It is obvious from the literature that AMPK is involved in the berberine-mediated antioxidant activity.
The investigators who administered berberine to diabetic mice reported that the activation of AMPK was not only associated with downregulation of NADPH oxidase (Zhou and Zhou, 2011; Lao-Ong et al., 2012), but also related to the upregulation of SOD expression (Eid et al., 2010; Gray et al., 2013). Additionally, the expression of UCP2 (uncoupling protein 2) in arteries could be increased by berberine that, on the other hand, could suppress the arterial oxidative stress through AMPK (Eid et al., 2010). UCP2 exists in the mitochondrial membrane and is involved in the negative regulation of ROS generation and oxidative stress (Kukidome et al., 2006; Wang et al., 2010a). The contradicting information on the role of berberine in UCP2 expression, i.e. the expression of UCP2 in liver cells could be decreased by berberine has been also documented (Xie et al., 2008). Until now, it is not clear whether the regulation of UCP2 by berberine is affected by the nature of tissue or not.
It has been elaborated that the upregulation of UCP2 is dually associated with DM, i.e. the upregulated UCP2 could suppress the production of ROS in renal or adipose tissues resulting in the ameliorated diabetic condition, while in islets β cells, the upregulated UCP2 could inhibit the secretion of insulin (Wang et al., 2011b). Thus, the mechanism of berberine in the regulation of UCP2 in islets β cells needs to be revealed.
Berberine-induced suppression of oxidative stress is also mediated through Nrf2 (nuclear factor erythroid-2 related factor 2) pathway (Negre-Salvayre et al., 1997; Arsenijevic et al., 2000; de Souza et al., 2011; Yang et al., 2011). Nrf2 is a transcription factor that exhibits excellent antioxidant property via expression of HO-1 (heme oxygenase-1) and NQO-1 (NADPH quinine oxidoreductase 1) (Mo et al., 2013). Nrf2 is involved in the energy metabolism and maintenance of redox potential in cells (Mo et al., 2013). Few studies have revealed that antioxidant activity of berberine could be eliminated through the blockage of Nrf2 in neurons and macrophages (Negre-Salvayre et al., 1997; Arsenijevic et al., 2000; de Souza et al., 2011; Yang et al., 2011), thus the reduction in oxidative stress by berberine could be associated with Nrf2. Nrf2-mediated activity of berberine depends on the activation of P38, AMPK, and PI3K (phosphatidylinositol 3 kinase)/Akt pathways (Figure 3), since the blockage of these pathways could suppress the stimulating effect of berberine on Nrf2 (Negre-Salvayre et al., 1997; Arsenijevic et al., 2000; de Souza et al., 2011; Yang et al., 2011). These pathways could be activated by berberine, it leads to translocation of Nrf2 in the nucleus resulting in the activation of expression of antioxidant enzymes. It causes an increase in the cellular level of GSH and SOD that eventually suppresses the generation of ROS leading to reduced oxidative stress (Figure 3).
The Anti-Inflammatory Potential of Berberine and the Underlying Mechanisms in DM Treatment
Effect of Berberine on Inflammation
Multiple studies (Table 2) have been conducted in vitro and in vivo to explore the anti-inflammatory effect of berberine using acute phase proteins and proinflammatory cytokines as markers (Choi et al., 2006; Jeong et al., 2009; Shang et al., 2010; Chen et al., 2011; Lin and Lin, 2011; Lou et al., 2011; Hsu et al., 2012, 2013; Bae et al., 2013; Wang, 2013; Pang et al., 2015). It has been reported that there is suppressed generation of MMP9 (matrix metalloprotease 9), TNF-α, COX2 (cyclooxygenase-2), iNOS (inducible nitric oxide synthase), MCP1 (monocyte chemoattractant protein 1), IL-6, IL-1β, CRP (C-reactive protein), and HP (hepatoglobin) in berberine-treated immune cells, hepatocytes, adipose tissues, islets β-cells or spleen cells (Choi et al., 2006; Lou et al., 2011; Hsu et al., 2012, 2013; Bae et al., 2013). One of these studies (Hsu et al., 2013) conducted on insulin resistant HepG2 cells reported the relationship between the insulin sensitizing effect of berberine and its anti-inflammatory effect. After treatment with berberine, there was a significant decline in cytokine generation and serine phosphorylation, while an increase in tyrosine phosphorylation of IRS mediated through insulin was observed in palmitate-treated HepG2 cells (Hsu et al., 2013).
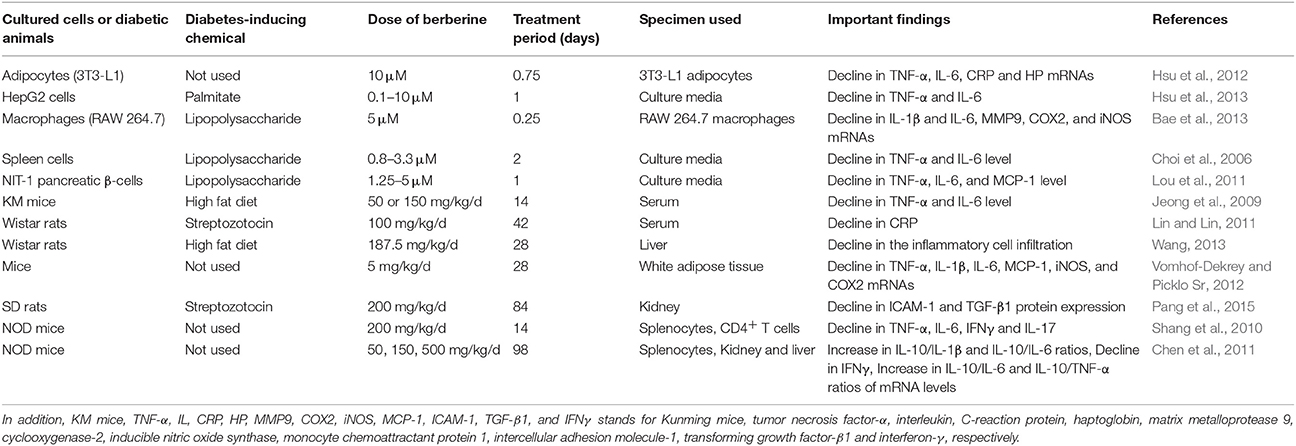
Table 2. The influence of orally administered berberine on the proinflammatory parameters in the cultured cells or diabetic animals.
In other studies (Choi et al., 2006; Lou et al., 2011; Bae et al., 2013; Pang et al., 2015), after injecting various chemicals such as HFD, alloxan or streptozotocin to animals for inducing DM or IR, the reduction in the level of proinflammatory cytokines and acute phase proteins in renal, hepatic, adipose and other tissues of berberine-treated animals (Choi et al., 2006; Lou et al., 2011; Bae et al., 2013; Pang et al., 2015) was observed (Table 2). Conclusively, this decrease in inflammation was attributed to improvement in diabetic condition and its complications.
A couple of other studies (Cui et al., 2009; Xing et al., 2011) reported the decrease in inflammation, leading to the improvement in type I diabetic condition in berberine-treated NOD mice (Table 2) that exhibits the suppressed levels of various pro-inflammatory cytokines such as IL-6, IL-17, TNF-α, and IFN-γ (interferon-γ) (Cui et al., 2009; Tian et al., 2016). Besides, the investigators computed the ratio of antiinflammatory factor (IL-10) to each of the following pro-inflammatory factors such as TNF-α, IL-6, and IL-1β and found that all the ratios were increased in berberine-fed NOD mice (Xing et al., 2011). Moreover, berberine activity was observed in hepatic, renal, spleen, and other tissues (Xing et al., 2011; Tian et al., 2016).
A clinical study (Chueh and Lin, 2012a) to assess the effect of berberine in diabetic patients against inflammation was conducted. There was a significant reduction in the IL-6 level of serum after administering a dose of one gram of berberine per day for 3 months.
Anti-inflammatory Mechanisms of Berberine
The suppression of inflammation by berberine is a complex phenomenon. It involves multiple pathways that are partially shared with antioxidant pathways (Figure 3).
AMPK is not only involved in the antioxidant effect, but also in the process of inflammation inhibition by berberine (Vomhof-Dekrey and Picklo Sr, 2012). In case of AMPK blockage, berberine could not inhibit the generation of pro-inflammatory cytokines such as COX2 and iNOS (Zhang et al., 2008). The elevated level of iNOS leads to the excessive release of nitric oxide resulting in the development of IR (Perreault and Marette, 2001). COX2 is involved in the prostaglandin synthesis (DuBois et al., 1998), while the prostaglandins mediate the pathogenesis of DM and its complications (Mima, 2013).
The inflammation activates MAPK (mitogen-activated protein kinase) pathway, which could be partially suppressed by berberine via activation of AMPK (Vomhof-Dekrey and Picklo Sr, 2012); it reduces the inflammation (Jeong et al., 2009; Jia et al., 2012; Vomhof-Dekrey and Picklo Sr, 2012; Wang et al., 2012). On the other hand, berberine activates P38 that plays a crucial role in combating oxidative stress and inflammation by berberine (de Souza et al., 2011; Lee et al., 2013). Thus, berberine possesses dual properties of MAPK signaling.
Similar conflicting findings were also observed in berberine-induced glucose metabolism mediated through P38. For instance, P38 is activated by berberine, which instead, enhances glucose uptake by L6 cells. Thus, P38 inhibitor could be used to terminate the berberine-mediated glucose metabolism (Cheng et al., 2006). Conversely, a study on the adipose cells did not show any involvement of P38 in glucose uptake under the influence of berberine (Zhou et al., 2007). It indicates that berberine could regulate MAPK. However, further studies are required to assess the interaction of MAPK with other signaling pathways and the resulting pharmacological effect of berberine.
Nrf2 is not only involved in antioxidant activity but also in anti-inflammatory activity of berberine (Figure 3) (Vomhof-Dekrey and Picklo Sr, 2012; Lee et al., 2013). In case of Nrf2 blockage, berberine could not suppress the production of pro-inflammatory cytokines in macrophages (Vomhof-Dekrey and Picklo Sr, 2012). The Nrf2-mediated activity of berberine activates P38 and AMPK pathways, which lead to translocation of Nrf2 in the nucleus, resulting in the suppression of pro-inflammatory cytokines (Lee et al., 2013).
Nrf2 drives the expression of an antioxidant (Mo et al., 2013) and anti-inflammatory enzyme, HO-1 (Lee et al., 2013), which is inducible by berberine (DuBois et al., 1998; Perreault and Marette, 2001; Zhang et al., 2008; Vomhof-Dekrey and Picklo Sr, 2012; Lee et al., 2013). In case of HO-1 blockage, berberine could not suppress the production of pro-inflammatory cytokines in macrophages (Lee et al., 2013). At present, HO-1 is known to be useful against DM and IR (Vomhof-Dekrey and Picklo Sr, 2012). Thus, the future studies could be focused on HO-1 as a valuable target to develop new promising drugs against DM.
The inflammation is also mediated via NF-κB pathway (Gratas-Delamarche et al., 2014), which could be targeted by berberine to induce the anti-inflammatory activity (Figure 3). In addition to food substances such as fatty acids and glucose (Goldin et al., 2006), the inflammatory stimuli including TNF-α (Gratas-Delamarche et al., 2014) could also activate IκB kinase-β (IKK-β) through serine phosphorylation (ser181) (Mercurio et al., 1997; Karin, 1999; Son et al., 2013) in NF-κB pathway. After feeding berberine, a decrease in activation of IKK-β and phosphorylation of ser181 in the adipose cells of the obese HFD-fed mice was noted (Yi et al., 2008). Moreover, IKK-β having a cysteine residue at position 179 is useful for berberine to exert inhibitory effect (Pandey et al., 2008).
IKK-β is involved in the phosphorylation and then degradation of IκB-α (inhibitory κB-α) (Mercurio et al., 1997; Karin, 1999; Son et al., 2013). Berberine could inhibit IKK-β leading to the stabilized IκB-α (Lee et al., 2007; Jia et al., 2012; Li et al., 2016). IκB-α turn restricts the nuclear transfer of NF-κB transcription factor (Lee et al., 2007; Jiang et al., 2011; Jia et al., 2012; Li et al., 2016), which induces the expression of pro-inflammatory cytokines including IL-6, iNOS, COX2, and TNF-α (Goldin et al., 2006; Wan et al., 2013; Gratas-Delamarche et al., 2014). These factors could be inhibited by berberine leading to negative regulation of the NF-κB pathway by berberine.
The Rho GTPase signaling pathway could be inhibited by berberine, mediating the suppression of kidney inflammation (Pang et al., 2015). Rho GTPase is a multifunctional protein that belongs to a big family of enzymes, small GTP binding proteins (Shi and Wei, 2013). Rho GTPase is involved in the positive regulation of NF-κB pathway (Xie et al., 2013b). Here we find a discrepancy, i.e., NF-κB pathway is regulated by berberine; on the other hand, berberine could suppress it by inhibiting Rho GTPase (Remppis et al., 2010; Pang et al., 2015). This activity of berberine was similar to its antioxidant property.
Activator protein 1 (AP-1) is another transcription factor that is involved in the anti-inflammatory activity of berberine (Ricote et al., 1998; Kuo et al., 2004; Schonthaler et al., 2011). Mechanistically, the berberine-mediated inhibition of AP-1 binding with DNA suppresses the production of pro-inflammatory cytokines such as COX2 and MCP1.
Berberine-mediated activation of PPARγ (peroxisome proliferator-activated receptor γ) is found to inhibit NF-κB and AP-1 (Delerive et al., 1999; Pasceri et al., 2000; Huang et al., 2006; Chen et al., 2008). It results in the reduced production of pro-inflammatory cytokines in the intestinal cells and macrophages (Zhou and Zhou, 2010; Feng et al., 2012) leading to the suppression of inflammation (Chen and Xie, 1986; Li et al., 2011).
Clinical Use of Berberine
Various clinical studies have described the safety and effectiveness of berberine (at a dose of 0.2–10 g/day) in treating T2DM patients. Generally, a decrease in blood glucose level by 20–40% is reported in fasting patients treated with berberine alone, this effect resembles to that of rosiglitazone and metformin (Zhang et al., 2010a; Dong et al., 2012). Moreover, additive hypoglycemic effect was observed in the Italian T2DM patients when treated with berberine in combination with sulfonylureas or metformin, the standard hypoglycemic drugs (di Pierro et al., 2012). However, the safer nature of this botanical is its important feature in comparison with the synthetic drugs including rosiglitazone or metformin. For instance, the synthetic drugs are not recommended for comorbid patients having T2DM and chronic hepatitis, because it could result in further destruction of hepatic functions. In contrast, berberine is safe and effective, exerting an ameliorative effect on the hepatic function and blood glucose level (Zhang et al., 2010a). The likely modes of action of berberine are ameliorated insulin sensitivity, enhanced insulin release, PPARs-modulated regulation of glucose- and lipid-metabolism, suppressed uptake of glucose via enterocytes, modulated effect on gut microbiota, and the inhibitory effect on oxidation and inflammation (Kim et al., 2007; Kong et al., 2009; Vuddanda et al., 2010; Chueh and Lin, 2011, 2012b; Zhang et al., 2011a,b; Derosa et al., 2012; Yang and Yin, 2012; Singh and Mahajan, 2013; Wu et al., 2016). However, the above-mentioned conclusions are extracted from various short-term studies, which must be supported by the large-scale, high-quality, and long-term randomized clinical trials to validate the effect of berberine on DM and the diabetic complications and recommend its routine clinical use as an effective moiety against DM.
Additionally, several studies have revealed therapeutic effects of berberine on diabetic complications, including diabetic cardiovascular diseases, neuropathy, and nephropathy (Dorr et al., 2012; Yao et al., 2015). A course of treating T2DM and its associated cardiovascular diseases in human with berberine resulted in an improvement in the endothelial function, likely via suppressing oxidative stress on the vascular endothelium mediated by CD31+/CD42- microphages (Gu et al., 2011). From results, it was obvious that berberine enhanced the expression of Nox4 proteins, suppressed synthesis of NO, and increased the production of ROS in human umbilical vein endothelial cells. In addition, diabetic cardiomyopathy is another cardiovascular diseases that could likely be treated using berberine since berberine influences cardiomyopathy-inducing factors, such as oxidative stress, the homeostasis of glucose and lipids, and endothelial dysfunction (Zhang et al., 2011a). Moreover, nephropathy is another diabetic complication that is promisingly treated by berberine. Berberine suppressed the excretion of albumin through urine, ameliorating the ratio of kidney to body weight and reducing the fasting blood glucose level, blood creatinine, glomerular area, and blood urea nitrogen in diabetic nephropathy in rats (Li and Shah, 2003; Tang et al., 2011). Additionally, berberine could significantly ameliorate nerve conduction velocity in diabetic neuropathy in rats (Hua et al., 2001). However, there are no direct scientific evidences at present to prove the role of berberine in above-stated diabetic associated complications, necessitating the additional mechanistic studies.
Discussion
After conducting the first study in 1986 on the antidiabetic activity of berberine in animals (Chen and Xie, 1986), this phytochemical moiety emerged as an excellent antidiabetic molecule (Ni, 1988). Later on, in 1988, the first clinical study in diabetic patients verified the blood glucose lowering potential of berberine (Lugrin et al., 2013). Hitherto, a large number of studies have been conducted to explore the molecular basis of berberine activity against diabetes and its complications. In this review article, the antioxidant and anti-inflammatory effects of berberine against DM are summarized.
Metabolically, there is a strong association between oxidative stress and inflammation (Soskic et al., 2011; Munoz and Costa, 2013; Gratas-Delamarche et al., 2014), owing to their regulation by the shared regulators such as NF-κB (Goldin et al., 2006; Soskic et al., 2011; Munoz and Costa, 2013; Gratas-Delamarche et al., 2014). Oxidative stress-induced generation of pro-inflammatory cytokines including IL-6 and TNF-α mediates the production of ROS, which enhances the oxidative stress (Zhang et al., 2012; Donath, 2013). Obviously, the malicious association between oxidative stress and inflammation could damage IR (Xie and Du, 2011; Gratas-Delamarche et al., 2014). Berberine could exert the inhibitory effect on oxidative stress and inflammation via multiple cellular pathways, for instance, AMPK signaling pathway (Vomhof-Dekrey and Picklo Sr, 2012; Lee et al., 2013). Future study can be executed to examine the restrictive effect of berberine on oxidative-inflammatory cycle.
Several mechanisms relating to berberine-mediated inhibition of oxidative stress and inflammation have been already proposed. Further to the above described modes of berberine, its activity against inflammation and gut-associated valuable effects are associated with each other (Xie and Du, 2011; Liu et al., 2016; Jia et al., 2017). Since berberine is poorly bioavailable (Peng et al., 2009; Han et al., 2011; Shan et al., 2013), the most part of its orally administered quantity could be involved in modulating colonic flora that leads to a buildup of short-chain fatty acids. These fatty acids are not only involved in the enhanced production of gut bacteria (Xie and Du, 2011), but it also plays a crucial role in the amelioration of selective permeability of intestine and suppression of inflammation by preventing entry of toxic materials into the systemic circulation (Zhang et al., 2011b). This concept has been proven by the ameliorative role of berberine in TNF-α–induced mucosal damage in the intestine (Amasheh et al., 2010; Tan et al., 2013). In spite of low oral bioavailability of berberine (Wang et al., 2012), berberine and its metabolites have been found in excess in several organs such as liver and kidney, explaining its excellent biological effects even with its low systemic contents (Peng et al., 2009; Wang et al., 2012). The metabolites of berberine have also been found to be involved in activating AMPK (Wu et al., 2013). Until now, no study has been conducted to explain the inhibitory role of berberine metabolites against oxidative stress and inflammation mediating DM and IR.
There are still several concerns about the antioxidant and anti-inflammatory role of berberine that necessitate further studies. For instance, the conflicting findings of the berberine-mediated regulation of PPARγ, UCP2, and MAPKs signaling pathways are not clarified yet. Based on the inhibitory potential of berberine against oxidative stress and inflammation, treatment of DM by berberine needs to be studied in the clinical setting. Moreover, berberine has been found to effectively combat endoplasmic reticulum stress in islets β-cell impairment and IR (Xue et al., 2005; Hanada et al., 2007; Wang et al., 2010b). Endoplasmic reticulum stress has grown into an excellent therapeutic target (Evans-Molina et al., 2013) owing to its involvement in the pathogenesis of DM via ROS and inflammation (Evans-Molina et al., 2013). Future studies should be focused on studying the effect of berberine on the endoplasmic reticulum stress and its association with oxidative stress and inflammation, explaining the mechanism of berberine against DM. These studies will be valuable to explore new horizons of natural moieties against DM.
Conclusion
The naturally existing phytochemical berberine exhibits an excellent activity against oxidative stress and inflammation via several signaling pathways including NF-κB, AMPK, Nrf2/HO, and MAPKs pathways and various kinases in cells, and likely contribute to treating DM and IR (Figure 3). Owing to the growing attention of clinicians in the usage of berberine during last two decades, the mechanistic aspects of the inhibitory role of berberine against oxidative stress and inflammation necessitate the advance studies at the molecular level.
Author Contributions
XM, ZC, LW, and ZZ designed and wrote this review, and GW, ZW, XD, and BW collected the information.
Funding
This work was supported by the National Natural Science Foundation of China (no. 81503382) and Special research project of State Chinese Medicine Administration Bureau (JDZX2015288).
Conflict of Interest Statement
The authors declare that the research was conducted in the absence of any commercial or financial relationships that could be construed as a potential conflict of interest.
Abbreviations
DM, diabetes mellitus; NF-κB, nuclear factor-κB; AMPK, AMP-activated protein kinases; MAPKs, mitogen-activated protein kinases; Nrf2, nuclear factor erythroid-2 related factor 2; ROS, reactive oxygen species; PKC, protein kinase C; IR, insulin resistance; IL-6, interleukin-6; TNF-α, tumor necrosis factor-α; IRS, insulin receptor substrate; AKT1, v-akt murine thymoma viral oncogene homolog 1; CASP3, caspase 3; MAPK1, mitogen-activated protein kinase 1; TP53, tumor protein p53; LDLR, low density lipoprotein receptor; PCSK9, proprotein convertase subtilisin/kexin type 9; DPP4, dipeptidyl-peptidase 4; CCND1, cyclin D1; ATP5G2, ATP synthase; HMOX1, heme oxygenase (decycling) 1; HPR, haptoglobin-related protein; HP, Haptoglobin; STAT3, signal transducer and activator of transcription 3; PTGS2, prostaglandin-endoperoxide synthase 2; SLC2A4, solute carrier family 2 (facilitated glucose transporter), member 4; STK11, serine/threonine kinase 11; ADIPOQ, Adiponectin; CTSB, cathepsin B; ITGAM, integrin, alpha M; MMP9, matrix metallopeptidase 9; JUN, jun proto-oncogene; PTGER4, prostaglandin E receptor 4; UCP2, uncoupling protein 2; INSR, insulin receptor; CYP2D6, cytochrome P450 enzyme; GCG, glucagon; DAXX, death-domain associated protein; CASP12, caspase 12 (gene/pseudogene); ALDH7A1, aldehyde dehydrogenase 7 family, member A1; MAU2, MAU2 chromatid cohesion factor homolog; TLR4, toll-like receptor 4; PLAU, plasminogen activator, urokinase; PTPN1, protein tyrosine phosphatase, non-receptor type 1; BECN1, beclin 1, autophagy related; SRC, v-src sarcoma (Schmidt-Ruppin A-2) viral oncogene homolog (avian); FN1, fibronectin 1; GATA2, GATA binding protein 2; IL17A, interleukin 17A; LPL, lipoprotein lipase; AIFM1, apoptosis-inducing factor, mitochondrion-associated, 1; NODAL, nodal homolog; AKR1B1, aldo-keto reductase family 1, member B1 (aldose reductase); EGFR, epidermal growth factor receptor; VMP1, vacuole membrane protein 1; SLC6A4, solute carrier family 6 (neurotransmitter transporter, serotonin), member 4; NRF1, nuclear respiratory factor 1; PON1, paraoxonase 1; KCNQ1, potassium voltage-gated channel, KQT-like subfamily, member 1; NME1, NME/NM23 nucleoside diphosphate kinase 1; GSH, glutathione; MDA, malondialdehyde; SOD, superoxide dismutase; GSH-Px, glutathione peroxidase; DMSO, dimethyl sulfoxide; SIRT1, Sirtuin 1; FOXO, forkhead box O; NADPH, nicotinamide adenine dinucleotide phosphate; HO-1, heme oxygenase-1; NQO-1, NADPH quinine oxidoreductase 1; PI3K, phosphatidylinositol 3 kinase; MMP9, matrix metalloprotease 9; COX2, cyclooxygenase-2; iNOS, inducible nitric oxide synthase; MCP1, monocyte chemoattractant protein 1; CRP, C-reactive protein; HP, hepatoglobin; IFN-α, interferon-β; IL-10, interleukin-10; IKK-β, IκB kinase-β; HFD, high fat diet; IκB-α, inhibitory κB-α; AP-1, activator protein 1; PPARγ, peroxisome proliferator-activated receptor γ.
References
Alberici, L. C., Vercesi, A. E., and Oliveira, H. C. (2011). Mitochondrial energy metabolism and redox responses to hypertriglyceridemia. J. Bioenerg. 43, 19–23. doi: 10.1007/s10863-011-9326-y
Amasheh, M., Fromm, A., and Krug, S. M. (2010). TNFα-induced and berberine-antagonized tight junction barrier impairment via tyrosine kinase, Akt and NFκB signaling. J. Cell Sci. 123, 4145–4155. doi: 10.1242/jcs.070896
Arsenijevic, D., Onuma, H., and Pecqueur, C. (2000). Disruption of the uncoupling protein-2 gene in mice reveals a role in immunity and reactive oxygen species production. Nat. Genet. 26, 435–439. doi: 10.1038/82565
Bae, J., Lee, D., Kim, Y. K., Gil, M., Lee, J. Y., and Lee, K. J. (2013). Berberine protects 6-hydroxydopamine-induced human dopaminergic neuronal cell death through the induction of heme oxygenase-1. Mol. Cells 35, 151–157. doi: 10.1007/s10059-013-2298-5
Banach, M., Patti, A. M., Giglio, R. V., Cicero, A. F. G., Atanasov, A. G., Bajraktari, G., et al. (2018). The role of nutraceuticals in statin intolerant patients: position paper from an international lipid expert panel. J. Am. Coll. Cardiol. 72, 96–118. doi: 10.1016/j.jacc.2018.04.040
Bhutada, P., Mundhada, Y., and Bansod, K. (2011). Protection of cholinergic and antioxidant system contributes to the effect of berberine ameliorating memory dysfunction in rat model of streptozotocin-induced diabetes. Behav. Brain Res. 220, 30–41. doi: 10.1016/j.bbr.2011.01.022
Bonnefont-Rousselot, D. (2002). Glucose and reactive oxygen species. Curr. Opin. Clin. Nutr. Metab. Care 5, 561–568. doi: 10.1097/00075197-200209000-00016
Booth, J. N., Colantonio, L. D., Howard, G., Safford, M. M., Banach, M., Reynolds, K., et al. (2016). Healthy lifestyle factors and incident heart disease and mortality in candidates for primary prevention with statin therapy. Int. J. Cardiol. 207, 196–202. doi: 10.1016/j.ijcard.2016.01.001
Caliceti, C., Franco, P., Spinozzi, S., Roda, A., and Cicero, A. F. (2016). Berberine: new insights from pharmacological aspects to clinical evidences in the management of metabolic disorders. Curr. Med. Chem. 23, 1460–1476. doi: 10.2174/0929867323666160411143314
Ceballos-Picot, I., Witko-Sarsat, V., and Merad-Boudia, M. (1996). Glutathione antioxidant system as a marker of oxidative stress in chronic renal failure. Free Radic. Biol. Med. 21, 845–853. doi: 10.1016/0891-5849(96)00233-X
Chang, W., Chen, L., and Hatch, G. M. (2015). Berberine as a therapy for type 2 diabetes and its complications: from mechanism of action to clinical studies. Biochem. Cell Biol. 93, 479–486. doi: 10.1139/bcb-2014-0107
Chang, W., Chen, L., and Hatch, G. M. (2016). Berberine treatment attenuates the palmitate-mediated inhibition of glucose uptake and consumption through increased 1,2,3-triacyl-sn-glycerol synthesis and accumulation in H9c2 cardiomyocytes. Biochim. Biophys. Acta 1861, 352–362. doi: 10.1016/j.bbalip.2015.12.017
Chatuphonprasert, W., Lao-Ong, T., and Jarukamjorn, K. (2013). Improvement of superoxide dismutase and catalase in streptozotocin-nicotinamide-induced type 2-diabetes in mice by berberine and glibenclamide. Pharm. Biol. 52, 419–427. doi: 10.3109/13880209.2013.839714
Chen, F. L., Yang, Z. H., and Liu, Y. (2008). Berberine inhibits the expression of TNFalpha, MCP-1, and IL-6 in AcLDL-stimulated macrophages through PPARgamma pathway. Endocrine 33, 331–337. doi: 10.1007/s12020-008-9089-3
Chen, Q. M., and Xie, M. Z. (1986). Studies on the hypoglycemic effect of Coptis chinensis and berberine. Aata Pharm. Sin. 21, 401–406.
Chen, Y., Wang, Y., Zhang, J., Sun, C., and Lopez, A. (2011). Berberine improves glucose homeostasis in streptozotocin-induced diabetic rats in association with multiple factors of insulin resistance. ISRN Endocrinol. 2011:519371. doi: 10.5402/2011/519371
Cheng, F., Wang, Y., and Li, J. (2013). Berberine improves endothelial function by reducing endothelial microparticles-mediated oxidative stress in humans. Int. J. Cardiol. 167, 936–942. doi: 10.1016/j.ijcard.2012.03.090
Cheng, Z., Pang, T., and Gu, M. (2006). Berberine-stimulated glucose uptake in L6 myotubes involves both AMPK and p38 MAPK. Biochim. Biophys. Acta 1760, 1682–1689. doi: 10.1016/j.bbagen.2006.09.007
Choi, B. H., Ahn, I. S., Kim, Y. H., Park, J. W, Lee, S. Y, and Hyun, C. K (2006). Berberine reduces the expression of adipogenic enzymes and inflammatory molecules of3T3-L1adipocyte. Exp. Mol. Med. 38, 599–605. doi: 10.1038/emm.2006.71
Chueh, W. H., and Lin, J. Y. (2012a). Protective effect of berberine on serum glucose levels in non-obese diabetic mice. Int. Immunopharmacol. 12, 534–538. doi: 10.1016/j.intimp.2012.01.003
Chueh, W. H., and Lin, J.-Y. (2012b). Protective effect of isoquinoline alkaloid berberine on spontaneous inflammation in the spleen, liver and kidney of non-obese diabetic mice through downregulating gene expression ratios of pro-/anti-inflammatory and Th1/Th2 cytokines. Food Chem. 131, 1263–1271. doi: 10.1016/j.foodchem.2011.09.116
Chueh, W. H., and Lin, J. Y. (2011). Berberine, an isoquinoline alkaloid in herbal plants, protects pancreatic islets and serum lipids in nonobese diabetic mice. J. Agric. Food Chem. 59, 8021–8027. doi: 10.1021/jf201627w
Cicero, A. F. G., Colletti, A., Bajraktari, G., Descamps, O., Djuric, D. M., Ezhov, M., et al. (2017). Lipid lowering nutraceuticals in clinical practice: position paper from an international lipid expert panel. Arch. Med. Sci. 13, 965–1005. doi: 10.5114/aoms.2017.69326
Cicero, A. F., and Baggioni, A. (2016). Berberine and its role in chronic disease. Adv. Exp. Med. Biol. 928, 27–45. doi: 10.1007/978-3-319-41334-1_2
Crook, M. (2004). Type 2 diabetes mellitus: a disease of the innate immune system? An update. Diabetic Med. 21, 203–207. doi: 10.1046/j.1464-5491.2003.01030.x
Cui, G., Qin, X., Zhang, Y., Gong, Z., Ge, B., and Zang, Y. Q. (2009). Berberine differentially modulates the activities of ERK, p38 MAPK, and JNK to suppress Th17 and Th1 T cell differentiation in type 1 diabetic mice. J. Biol. Chem. 284, 28420–28429. doi: 10.1074/jbc.M109.012674
Souza, B. M., Assmann, T. S., Kliemann, L. M., Gross, J. L., Canani, L. H., and Crispim, D. (2011). The role of uncoupling protein 2 (UCP2) on the development of type 2 diabetes mellitus and its chronic complications. Arquivos Brasil. Endocrinol. Metabol. 55, 239–248. doi: 10.1590/S0004-27302011000400001
Del Rio, D., Stewart, A. J., and Pellegrini, N. (2005). A review of recent studies on malondialdehyde as toxic molecule and biological marker of oxidative stress. Nutr. Metab. Cardiovasc. Dis. 15, 316–328. doi: 10.1016/j.numecd.2005.05.003
Delerive, P., Martin-Nizard, F., and Chinetti, G. (1999). Peroxisome proliferator-activated receptor activators inhibit thrombin-induced endothelin-1 production in human vascular endothelial cells by inhibiting the activator protein-1 signaling pathway. Circ. Res. 85, 394–402. doi: 10.1161/01.RES.85.5.394
Derosa, G., Maffioli, P., and Cicero, A. F. G. (2012). Berberine on metabolic and cardiovascular risk factors: an analysis from preclinical evidences to clinical trials. Expert Opin. Biol. Ther. 12, 1113–1124. doi: 10.1517/14712598.2012.704014
Di Pierro, F., Villanova, N., Agostini, F., Marzocchi, R., Soverini, V., and Marchesini, G. (2012). Pilot study on the additive effects of berberine and oral type 2 diabetes agents for patients with suboptimal glycemic control. Diabetes Metab. Syndr. Obes. 5, 213–217. doi: 10.2147/DMSO.S33718
Donath, M. Y. (2013). Targeting inflammation in the treatment of type 2 diabetes. Diabetes Obes. Metab. 15(Suppl. 3), 193–196. doi: 10.1111/dom.12172
Donath, M. Y., and Shoelson, S. E. (2011). Type 2 diabetes as an inflammatory disease. Nat. Rev. Immunol. 11, 98–107. doi: 10.1038/nri2925
Dong, H., Wang, N., Zhao, L., and Lu, F. (2012). Berberine in the treatment of type 2 diabetes mellitus: a systemic review and metaanalysis. Evid. Based Compl. Alternat. Med. 2012:591654. doi: 10.1155/2012/591654.
Dong, Y., Chen, Y. T., Yang, Y. X., Zhou, X. J., Dai, S. J., Tong, J. F., et al. (2016). Metabolomics study of type 2 diabetes mellitus and the antidiabetic effect of berberine in Zucker diabetic fatty rats using Uplc-ESI-Hdms. Phytother Res. 30, 823–828. doi: 10.1002/ptr.5587
Dörr, R., Stumpf, J., Spitzer, S. G., Krosse, B., Tschöpe, D., and Lohmann, T. (2012). Prävalenz unentdeckter Glukosestoffwechselstörungen in der invasiven und interventionellen Kardiologie. Herz 37, 244–251. doi: 10.1007/s00059-012-3607-z
Dubois, R. N., Abramson, S. B., Crofford, L. Gupta R. A., Simon, L. S, and Van De Putte, L. B (1998). Cyclooxygenase in biology and disease. FASEB J. 12, 1063–1073. doi: 10.1096/fasebj.12.12.1063
Eid, A. A., Ford, B. M., and Block, K. (2010). AMP-activated Protein kinase (AMPK) negatively regulates Nox4-dependent activation of p53 and epithelial cell apoptosis in diabetes. J. Biol. Chem. 285, 37503–37512. doi: 10.1074/jbc.M110.136796
Evans, J. L., Goldfine, I. D., Maddux, B. A., and Grodsky, G. M. (2003). Are oxidative stress—activated signaling pathways mediators of insulin resistance and β-cell dysfunction? Diabetes 52, 1–8. doi: 10.2337/diabetes.52.1.1
Evans, J. L., Maddux, B. A., and Goldfine, I. D. (2005). The molecular basis for oxidative stress-induced insulin resistance. Antioxidants Redox Signal. 7, 1040–1052. doi: 10.1089/ars.2005.7.1040
Evans-Molina, C., Hatanaka, M., and Mirmira, R. G. (2013). Lost in translation: endoplasmic reticulum stress and the decline of β-cell health in diabetes mellitus. Diabetes Obes. Metab. 15 (Suppl. 3), 159–169. doi: 10.1111/dom.12163
Feng, A. W., Gao, W., Zhou, G. R., Yu, R., Li, N., and Huang, X. L (2012). Berberine ameliorates COX-2 expression in rat small intestinal mucosa partially through PPARγ pathway during acute endotoxemia. Int. Immunopharmacol. 12, 182–188. doi: 10.1016/j.intimp.2011.11.009
Fu, D., Yu, J. Y., Connell, A. R., Yang, S., Hookham, M. B., McLeese, R., et al. (2016). Beneficial effects of berberine on oxidized LDL-induced cytotoxicity to human retinal Müller cells. Invest. Ophthalmol. Vis. Sci. 57, 3369–3379. doi: 10.1167/iovs.16-19291
Furukawa, S., Fujita, T., and Shimabukuro, M. (2004). Increased oxidative stress in obesity and its impact on metabolic syndrome. J. Clin. Investig. 114, 1752–1761. doi: 10.1172/JCI21625
Goguet-Rubio, P., Seyran, B., Gayte, L., Bernex, F., Sutter, A., and Delpech, H. (2016). E4F1-mediated control of pyruvate dehydrogenase activity is essential for skin homeostasis. Proc. Natl. Acad. Sci. U.S.A. 11, 11004–11009. doi: 10.1073/pnas.1602751113
Goldin, A., Beckman, J. A., Schmidt, A. M., and Creager, M. A. (2006). Advanced glycation end products: sparking the development of diabetic vascular injury. Circulation 114, 597–605. doi: 10.1161/CIRCULATIONAHA.106.621854
Gratas-Delamarche, A., Derbré, F., Vincent, S., and Cillard, J. (2014). Physical inactivity, insulin resistance, and the oxidativeinflammatory loop. Free Radical Res. 48, 93–108. doi: 10.3109/10715762.2013.847528
Gray, S. P., Di Marco, E., and Okabe, J. (2013). NADPH oxidase 1 plays a key role in diabetes mellitus-accelerated atherosclerosis. Circulation 127, 1888–1902. doi: 10.1161/CIRCULATIONAHA.112.132159
Gu, L., Li, N., Gong, J., Li, Q., Zhu, W., and Li, J. (2011). Berberine ameliorates intestinal epithelial tight-junction damage and down-regulates myosin light chain kinase pathways in a mouse model of endotoxinemia. J. Infect. Dis. 203, 1602–1612. doi: 10.1093/infdis/jir147
Han, J., Lin, H., and Huang, W. (2011). Modulating gut microbiota as an anti-diabetic mechanism of berberine. Med. Sci. Monitor 17, RA164–RA167. doi: 10.12659/MSM.881842
Hanada, S., Harada, M., and Kumemura, H. (2007). Oxidative stress induces the endoplasmic reticulum stress and facilitates inclusion formation in cultured cells. J. Hepatol. 47, 93–102. doi: 10.1016/j.jhep.2007.01.039
Hasan, N. M., Longacre, M. J., Stoker, S. W., Kendrick, M. A., and MacDonald, M. J. (2015). Mitochondrial malic enzyme 3 is important for insulin secretion in pancreatic β-cells. Mol. Endocrinol. 29, 396–410. doi: 10.1210/me.2014-1249
Hill, C., Flyvbjerg, A., and Grønbæk, H. (2000). The renal expression of transforming growth factor-β isoforms and their receptors in acute and chronic experimental diabetes in rats. Endocrinology 141, 1196–1208. doi: 10.1210/endo.141.3.7359
Hsu, Y. Y., Chen, C. S, Wu, S. N., Jong, Y. J., and Lo, Y. C. (2012). Berberine activates Nrf2 nuclear translocation and protects against oxidative damage via a phosphatidylinositol 3-kinase/ Akt-dependent mechanism in NSC34 motor neuron-like cells. Eur. J. Pharmaceut. Sci. 46, 415–425. doi: 10.1016/j.ejps.2012.03.004
Hsu, Y. Y., Tseng, Y. T., and Lo, Y. C. (2013). Berberine, a natural antidiabetes drug, attenuates glucose neurotoxicity and promotes Nrf2-related neurite outgrowth. Toxicol. Appl. Pharmacol. 272, 787–796. doi: 10.1016/j.taap.2013.08.008
Hua, W. G., Song, J. M., and Liao, H. (2001). Effect of Huang Lian Su on nerve conduction velocity and hormone level to diabetic neuropathy in rats. labeled immunoassay. Clin. Med. 8, 212–214. doi: 10.3969/j.issn.1006-1703.2001.04.009
Huang, C., Zhang, Y., and Gong, Z. (2006). Berberine inhibits 3T3L1 adipocyte differentiation through the PPARγ pathway. Biochem. Biophys. Res. Commun. 348, 571–578. doi: 10.1016/j.bbrc.2006.07.095
Hung, H. Y., Qian, K., Morris-Natschke, S. L., Hsu, C. S., and Lee, K. H. (2012). Recent discovery of plant-derived anti-diabetic natural products. Nat. Prod. Rep. 29, 580–606. doi: 10.1039/c2np00074a
Imenshahidi, M., and Hosseinzadeh, H. (2016). Berberis vulgaris and berberine: an update review. Phytother. Res. 30, 1745–1764. doi: 10.1002/ptr.5693
Jeong, H. W., Hsu, K. C., Lee, J. W., Ham, M., Huh, J. Y., and Shin, H. J (2009). Berberine suppresses proinflammatory responses through AMPK activation in macrophages. Am. J. Physiol. Endocrinol. Metab. 296, E955–E964. doi: 10.1152/ajpendo.90599.2008
Jia, L., Liu, J., and Song, Z. (2012). Berberine suppresses amyloidbeta-induced inflammatory response in microglia by inhibiting nuclear factor-kappaB and mitogen-activated protein kinase signalling pathways. J. Pharm. Pharmacol. 64, 1510–1521. doi: 10.1111/j.2042-7158.2012.01529.x
Jia, Y., Xu, B., and Xu, J. (2017). Effects of type 2 diabetes mellitus on the pharmacokinetics of berberine in rats. Pharm Biol. 55, 510–515. doi: 10.1080/13880209.2016.1255649
Jiang, Q., Liu, P., and Wu, X. (2011). Berberine attenuates lipopolysaccharide-induced extracelluar matrix accumulation and inflammation in rat mesangial cells: involvement of NF-κB signaling pathway. Mol. Cell. Endocrinol. 331, 34–40. doi: 10.1016/j.mce.2010.07.023
Jiang, S. J., Dong, H., Li, J. B., Xu, L. J., Zou, X., Wang, K. F., et al. (2015). Berberine inhibits hepatic gluconeogenesis via the LKB1-AMPK-TORC2 signaling pathway in streptozotocin-induced diabetic rats. World J. Gastroenterol. 21, 7777–7785. doi: 10.3748/wjg.v21.i25.7777
Kaneto, H., Xu, G., Fujii, N., Kim, S., Bonner-Weir, S., and GWeir, C. (2002). Involvement of c-Jun N-terminal kinase in oxidative stress-mediated suppression of insulin gene expression. J. Biol. Chem. 277, 30010–30018. doi: 10.1074/jbc.M202066200
Karin, M. (1999). Positive and negative regulation of IκB kinase activity through IKKβ subunit phosphorylation. Science 284, 309–313.
Kim, S. H., Shin, E. J., Kim, E. D., Bayaraa, T., Frost, S. C., and Hyun, C. K. (2007). Berberine activates GLUT1-mediated glucose uptake in 3T3-L1 adipocytes. Biol. Pharm. Bull. 30, 2120–2125. doi: 10.1248/bpb.30.2120
Kong, W.-J., Zhang, H., and Song, D.-Q. (2009). Berberine reduces insulin resistance through protein kinase C-dependent upregulation of insulin receptor expression. Metab. Clin. Exp. 58, 109–119. doi: 10.1016/j.metabol.2008.08.013
Kukidome, D., Nishikawa, T., and Sonoda, K. (2006). Activation of AMP-activated protein kinase reduces hyperglycemia-induced mitochondrial reactive oxygen species production and promotes mitochondrial biogenesis in human umbilical vein endothelial cells. Diabetes 55, 120–127. doi: 10.2337/diabetes.55.01.06.db05-0943
Kuo, C. L., Chi, C. W., and Liu, T. Y. (2004). The anti-inflammatory potential of berberine in vitro and in vivo. Cancer Lett. 203, 127–137. doi: 10.1016/j.canlet.2003.09.002
Lan, J., Zhao, Y., Dong, F., Yan, Z., Zheng, W., Fan, J., et al. (2015). Meta-analysis of the effect and safety of berberine in the treatment of type 2 diabetes mellitus, hyperlipemia and hypertension. J. Ethnopharmacol. 161, 69–81. doi: 10.1016/j.jep.2014.09.049
Lao-Ong, T., Chatuphonprasert, W., Nemoto, N., and Jarukamjorn, K. (2012). Alteration of hepatic glutathione peroxidase and superoxide dismutase expression in streptozotocin-induced diabetic mice by berberine. Pharmaceut. Biol. 50, 1007–1012. doi: 10.3109/13880209.2012.655377
Lee, C.-H., Chen, J.-C., Hsiang, C.-Y., Wu, S.-L., Wu, H.-C., and Ho, T.-Y. (2007). Berberine suppresses inflammatory agents-induced interleukin-1β and tumor necrosis factor-α productions via the inhibition of IκB degradation in human lung cells. Pharmacol. Res. 56, 193–201. doi: 10.1016/j.phrs.2007.06.003
Lee, D., Bae, J., and Kim, K. Y. (2013). Inhibitory effects of berberine on lipopolysaccharide-induced inducible nitric oxide synthase and the high-mobility group box 1 release in macrophages. Biochem. Biophys. Res. Commun. 431, 506–511. doi: 10.1016/j.bbrc.2012.12.143
Lee, M. H., Jeon, Y. J., Lee, S. M., Park, M. H., Jung, S. C., and Kim, Y. J. (2010). Placental gene expression is related to glucose metabolism and fetal cord blood levels of insulin and insulin-like growth factors in intrauterine growth restriction. Early Hum. Dev. 86, 45–50. doi: 10.1016/j.earlhumdev.2010.01.001
Li, F., Zhao, Y. B., Wang, D. K., Zou, X., Fang, K., and Wang, K. F. (2016). Berberine relieves insulin resistance via the cholinergic anti-inflammatory pathway in HepG2 cells. J. Huazhong Univ. Sci. Technolog. Med. Sci. 36, 64–69. doi: 10.1007/s11596-016-1543-5
Li, G. S., Liu, X.H., Zhu, H., Huang, L, Liu, Y. L., and Ma, C. M (2011). Berberine-improved visceral white adipose tissue insulin resistance associated with altered sterol regulatory element-binding proteins, liver X receptors, and peroxisome proliferator-activated receptors transcriptional programs in diabetic hamsters. Biol. Pharmaceut. Bull. 34, 644–654. doi: 10.1248/bpb.34.644
Li, J.-M., and Shah, A. M. (2003). ROS generation by nonphagocytic NADPH oxidase: potential relevance in diabetic nephropathy. J. Am. Soc. Nephrol. 14(Suppl. 3), S221–S226. doi: 10.1097/01.ASN.0000077406.67663.E7
Lin, W. C., and Lin, J. Y. (2011). Five bitter compounds display different anti-inflammatory effects through modulating cytokine secretion using mouse primary splenocytes in vitro. J. Agric. Food Chem. 59, 184–192. doi: 10.1021/jf103581r
Liu, C. S., Zheng, Y. R., Zhang, Y. F., and Long, X. Y. (2016). Research progress on berberine with a special focus on its oral bioavailability. Fitoterapia 109, 274–282. doi: 10.1016/j.fitote.2016.02.001
Liu, C., Wang, Z., Song, Y., Wu, D., Zheng, X., and Li, P. (2015). Effects of berberine on amelioration of hyperglycemia and oxidative stress in high glucose and high fat diet-induced diabetic hamsters in vivo. BioMed Res. Int. 2015:313808. doi: 10.1155/2015/313808
Liu, L., Liu, J., Gao, Y., Yu, X., Xu, G., and Huang, Y. (2014). Uncoupling protein-2 mediates the protective action of berberine against oxidative stress in rat insulinoma INS-1E cells and in diabetic mouse islets. Br. J. Pharmacol. 171, 3246–3254. doi: 10.1111/bph.12666
Liu, W. H., Hei, Z. Q., Nie, H., Tang, F. T., Huang, H. Q., and Li, X. J. (2008b). Berberine ameliorates renal injury in streptopzotocin-induced diabetic rats by suppression of both oxidative stress and aldose reductase. Chin. Med. J. 121, 706–712. doi: 10.3969/j.issn.1006-0588.2015.03.002
Liu, W., Liu, P., and Tao, S. (2008a). Berberine inhibits aldose reductase and oxidative stress in rat mesangial cells cultured under high glucose. Arch. Biochem. Biophys. 475, 128–134. doi: 10.1016/j.abb.2008.04.022
Liu, Y., Zhang, L., Song, H., and Ji, G. (2013). Update on berberine in nonalcoholic fatty liver disease. Evid. Based Compl. Altern. Med. 2013:308134. doi: 10.1155/2013/308134
Lou, T., Zhang, Z., and Xi, Z. (2011). Berberine inhibits inflammatory response and ameliorates insulin resistance in hepatocytes. Inflammation 34, 659–667. doi: 10.1007/s10753-010-9276-2
Lugrin, J., Rosenblatt-Velin, N., Parapanov, R., and Liaudet, L. (2013). The role of oxidative stress during inflammatory processes. Biol. Chem. 395, 203–230. doi: 10.1515/hsz-2013-0241
Ma, Z. J., Hu, S. L., Wang, S. S., Guo, X., Zhang, X. N., Sun, B., et al. (2016). Effects and underlying mechanism of berberine on renal tubulointerstitial injury in diabetic rats. Zhonghua Yi Xue Za Zhi 96, 3072–3077. doi: 10.3760/cma.j.issn.0376-2491.2016.38.008
Mahmoud, F., and Al-Ozairi, E. (2013). Inflammatory cytokines and the risk of cardiovascular complications in type 2 diabetes. Dis. Markers 35, 235–241. doi: 10.1155/2013/931915
Majithiya, J. B., and Balaraman, R. (2005). Time-dependent changes in antioxidant enzymes and vascular reactivity of aorta in streptozotocin-induced diabetic rats treated with curcumin. J. Cardiovasc. Pharmacol. 46, 697–705. doi: 10.1097/01.fjc.0000183720.85014.24
Maritim, A. C., Sanders, R. A., and Watkins, J. B. (2003). Diabetes, oxidative stress, and antioxidants: a review. J. Biochem. Mol. Toxicol. 17, 24–38. doi: 10.1002/jbt.10058
Mazidi, M., Kengne, A. P., Mikhailidis, D. P., Toth, P. P., Ray, K. K., and Banach, M. (2017). Dietary food patterns and glucose/insulin homeostasis: a cross-sectional study involving 24,182 adult Americans. Lipids Health Dis. 16, 192–197. doi: 10.1186/s12944-017-0571-x
Mercurio, F., Zhu, H., and Murray, B. W. (1997). IKK-1 and IKK-2: cytokine-activated IκB kinases essential for NF-κB activation. Science 278, 860–866.
Mima, A. (2013). Inflammation and oxidative stress in diabetic nephropathy: new insights on its inhibition as new therapeutic targets. J. Diabetes Res. 2013:248563. doi: 10.1155/2013/248563
Mo, C., Wang, L., and Zhang, J. (2013). The crosstalk between Nrf2 and AMPK signal pathways is important for the anti-inflammatory effect of berberine in LPS-stimulated macrophages and endotoxin-shocked mice. Antioxidants Redox Signal. 20, 574–588. doi: 10.1089/ars.2012.5116
Moghaddam, H. K., Baluchnejadmojarad, T., and Roghani, M. (2013). Berberine ameliorate oxidative stress and astrogliosis in the hippocampus of STZ-induced diabetic rats. Mol. Neurobiol. 49, 820–826. doi: 10.1007/s12035-013-8559-7
Mulder, H., Ahrén, B., and Sundler, F. (1996). Islet amyloid polypeptide' (amylin) and insulin are differentially expressed in chronic diabetes induced by streptozotocin in rats. Diabetologia 39, 649–657. doi: 10.1007/BF00418536
Muñoz, A., and Costa, M. (2013). Nutritionally mediated oxidative~ stress and inflammation. Oxid. Med. Cell. Longevity 2013:610950. doi: 10.1155/2013/610950
Negre-Salvayre, A., Hirtz, C., and Carrera, G. (1997). A role for‘ uncoupling protein-2 as a regulator of mitochondrial hydrogen peroxide generation. FASEB J. 11, 809–815. doi: 10.1096/fasebj.11.10.9271366
Ni, W. J., Ding, H. H., and Tang, L. Q. (2015). Berberine as a promising anti-diabetic nephropathy drug: an analysis of its effects and mechanisms. Eur. J. Pharmacol. 760, 103–112. doi: 10.1016/j.ejphar.2015.04.017
Ni, Y. X. (1988). Therapeutic effect of berberine on 60 patients with type II diabetes mellitus and experimental research. Zhong Xi Yi Jie He Za Zhi 8, 711–713.
Pandey, M. K., Sung, B., Kunnumakkara, A. B., Sethi, G., Chaturvedi, M. M., and Aggarwal, B. B. (2008). Berberine modifies cysteine 179 of IκBα kinase, suppresses nuclear factor-κB-regulated antiapoptotic gene products, and potentiates apoptosis. Cancer Res. 68, 5370–5379. doi: 10.1158/0008-5472.CAN-08-0511
Pang, B., Zhao, L. H., Zhou, Q., Zhao, T. Y., Wang, H., and Gu, C. J. (2015). Application of berberine on treating type 2 diabetes mellitus. Int. J. Endocrinol. 2015:905749. doi: 10.1155/2015/905749
Pasceri, V., Wu, H. D., Willerson, J. T., and Yeh, E. T. H. (2000). Modulation of vascular inflammation in vitro and in vivo by peroxisome proliferator-activated receptor-γ activators. Circulation 101, 235–238. doi: 10.1161/01.CIR.101.3.235
Patel, P. S., Buras, E. D., and Balasubramanyam, A. (2013). The role of the immune system in obesity and insulin resistance. J. Obes. 2013:616193. doi: 10.1155/2013/616193
Patti, A. M., Al-Rasadi, K., Giglio, R. V., Nikolic, D., Mannina, C., Castellino, G., et al. (2018). Natural approaches in metabolic syndrome management. Arch. Med. Sci. 14, 422–441. doi: 10.5114/aoms.2017.68717
Peng, L., Li, Z. R., Green, R. S., Holzman, I. R., and Lin, J. (2009). Butyrate enhances the intestinal barrier by facilitating tight junction assembly via activation of AMP-activated protein kinase in Caco-2 cell monolayers. J. Nutr. 139, 1619–1625. doi: 10.3945/jn.109.104638
Perreault, M., and Marette, A. (2001). Targeted disruption of inducible nitric oxide synthase protects against obesity-linked insulin resistance in muscle. Nat. Med. 7, 1138–1143. doi: 10.1038/nm1001-1138
Pirillo, A., and Catapano, A. L. (2015). Berberine, a plant alkaloid with lipid- and glucose-lowering properties: from in vitro evidence to clinical studies. Atherosclerosis 243, 449–461. doi: 10.1016/j.atherosclerosis.2015.09.032
Remppis, A., Bea, F., and Greten, H. J. (2010). Rhizoma coptidis inhibits LPS-induced MCP-1/CCL2 production in murine macrophages via an AP-1 and NF B-dependent pathway. Mediators Inflam. 2010:194896. doi: 10.1155/2010/194896
Ricote, M., Li, A. C., Willson, T. M., Kelly, C. J., and Glass, C.K (1998). The peroxisome proliferator-activated receptor-γ is a negative regulator of macrophage activation. Nature 391, 79–82.
Rosen, P., Nawroth, L., King, G., Moller, W., Tritschler, H. J., and Packer, L. (2001). The role of oxidative stress in the onset and progression of diabetes and its complications: a summary of a congress series sponsored by UNESCO-MCBN, the American diabetes association and the German diabetes society. Diabetes Metab. Res. Rev. 17, 189–212. doi: 10.1002/dmrr.196
Salminen, A., Kaarniranta, K., and Kauppinen, A. (2013). Crosstalk between oxidative stress and SIRT1: impact on the aging process. Int. J. Mol. Sci. 14, 3834–3859. doi: 10.3390/ijms14023834
Sarna, L. K., Wu, N., S., -Y., Hwang, Y., Siow, Y. L., and Karmin, O. (2010). Berberine inhibits NADPH oxidase mediated superoxide anion production in macrophages. Can. J. Physiol. Pharmacol. 88, 369–378. doi: 10.1139/Y09-136
Schonthaler, H. B., Guinea-Viniegra, J., and Wagner, E. F. (2011). Targeting inflammation by modulating the Jun/AP-1 pathway. Ann. Rheum. Dis. 70, i109–i112. doi: 10.1136/ard.2010.140533
Scivittaro, V., Ganz, M. B., and Weiss, F. M. (2000). AGEs induce oxidative stress and activate protein kinase C-(II) in neonatal mesangial cells. Am. J. Physiol. 278, F676–F683. doi: 10.1152/ajprenal.2000.278.4.F676
Shan, Y. Q., Zhu, Y. P., and Pang, J. (2013). Tetrandrine potentiates the hypoglycemic efficacy of berberine by inhibiting P-glycoprotein function. Biol. Pharmaceut. Bull. 36, 1562–1569. doi: 10.1248/bpb.b13-00272
Shang, W., Liu, J., Yu, X., and Zhao, J. (2010). Effects of berberine on serum levels of inflammatory factors and inflammatory signaling pathway in obese mice induced by high fat diet. Zhongguo Zhongyao Zazhi 35, 1474–1477.
Shi, J., and Wei, L. (2013). Rho kinases in cardiovascular physiology and pathophysiology: the effect of fasudil. J. Cardiovasc. Pharmacol. 62, 341–354. doi: 10.1097/FJC.0b013e3182a3718f
Shirwaikar, A., Shirwaikar, A., Rajendran, K., and Punitha, I. S. R. (2006). In vitro antioxidant studies on the benzyl tetra isoquinoline alkaloid berberine. Biol. Pharmaceut. Bull. 29, 1906–1910. doi: 10.1248/bpb.29.1906
Singh, I. P., and Mahajan, S. (2013). Berberine and its derivatives: a patent review (2009–2012). Expert Opin. Ther. Patents 23, 215–231. doi: 10.1517/13543776.2013.746314
Son, Y., Lee, J. H., Chung, H. T., and Pae, H. O. (2013). Therapeutic roles of heme oxygenase-1 in metabolic diseases: curcumin and resveratrol analogues as possible Inducers of heme oxygenase-1. Oxid. Med. Cell. Longev. 2013:639541. doi: 10.1155/2013/639541.
Soskic, S. S., Dobutovic, B. D., and Sudar, E. M. (2011). Regulation' of inducible Nitric Oxide synthase (iNOS) and its potential role in insulin resistance, diabetes and heart failure. Open Cardiovasc. Med. J. 5, 153–163. doi: 10.2174/1874192401105010153
Suman, R. K., Borde, M. K., Mohanty, I. R., Maheshwari, U., and Deshmukh, Y. A. (2016). Myocardial salvaging effects of berberine in experimental diabetes co-existing with myocardial infarction. J. Clin. Diagn. Res. 10, 13–18. doi: 10.7860/JCDR/2016/15794.7459
Tan, X. S., Ma, J. Y., and Feng, R. (2013). Tissue distribution of berberine and its metabolites after oral administration in rats. PLoS ONE 8:e77969. doi: 10.1371/journal.pone.0077969
Tang, L. Q., Wei, W., Chen, L. M., and Liu, S. (2006). Effects of berberine on diabetes induced by alloxan and a high-fat/high-cholesterol diet in rats. J. Ethnopharmacol. 108, 109–115. doi: 10.1016/j.jep.2006.04.019
Tang, L. Q., Ni, W. J., Cai, M., Ding, H. H., Liu, S., and Zhang, S. T. (2016). Renoprotective effects of berberine and its potential effect on the expression of β-arrestins and intercellular adhesion molecule-1 and vascular cell adhesion molecule-1 in streptozocin-diabetic nephropathy rats. J. Diabetes 8, 693–700. doi: 10.1111/1753-0407.12349
Tang, L., Lv, F., Liu, S., and Zhang, S. (2011). Effect of berberine on expression of transforming growth factor-beta1 and type IV collagen proteins in mesangial cells of diabetic rats with nephropathy. Chin. Pharm. J. 36, 3494–3497.
Tasdelen, I., van Beekum, O., Gorbenko, O., Fleskens, V., van den Broek, N. J., and Koppen, A. (2013). The serine/threonine phosphatase PPM1B (PP2Cβ) selectively modulates PPARγ activity. Biochem. J. 451, 45–53. doi: 10.1042/BJ20121113
Tian, C. M., Jiang, X., Ouyang, X. X., Zhang, Y. O., and Xie, W. D. (2016). Berberine enhances antidiabetic effects and attenuates untoward effects of canagliflozin in streptozotocin-induced diabetic mice. Chin. J. Nat. Med.14, 518–526. doi: 10.1016/S1875-5364(16)30061-9
van der Horst, A., Tertoolen, L. G. J., De Vries-Smits, L. M. M., Frye, R. A., Medema, R. H., and Burgering, B. M. T. (2004). FOXO4 is acetylated upon peroxide stress and deacetylated by the longevity protein hSirSIRT1. J. Biol. Chem. 279, 28873–28879. doi: 10.1074/jbc.M401138200
Vomhof-Dekrey, E. E., and Picklo Sr, M. J. (2012). The Nrf2-antioxidant response element pathway: a target for regulating energy metabolism. J. Nutr. Biochem. 23, 1201–1206. doi: 10.1016/j.jnutbio.2012.03.005
Vuddanda, P. R., Chakraborty, S., and Singh, S. (2010). Berberine: a potential phytochemical with multispectrum therapeutic activities. Expert Opin. Invest. Drugs 19, 1297–1307. doi: 10.1517/13543784.2010.517745
Wan, X., Chen, X., and Liu, L. (2013). Berberine ameliorates chronic kidney injury caused by atherosclerotic renovascular disease through the suppression of NFκB signaling pathway in rats. PLoS ONE 8:e59794. doi: 10.1371/journal.pone.0059794
Wang, Q., Qi, J., Hu, R., Chen, Y., Kijlstra, A., and Yang, P. (2012). Effect of berberine on proinflammatory cytokine production by ARPE19 cells following stimulation with tumor necrosis factor-α. Invest. Ophthalmol. Vis. Sci. 53, 2395–2402. doi: 10.1167/iovs.11-8982
Wang, Q., Zhang, M., Liang, B., Shirwany, N., Zhu, Y., and Zou, M.-H. (2011a). Activation of AMP-activated protein kinase is required for berberine-induced reduction of atherosclerosis in mice: the role of uncoupling protein 2. PLoS ONE 6:e25436. doi: 10.1371/journal.pone.0025436
Wang, S., Zhang, M., and Liang, B. (2010a). AMPKα2 deletion causes aberrant expression and activation of NAD(P)H oxidase and consequent endothelial dysfunction in vivo: role of 26S proteasomes. Circ. Res. 106, 1117–1128. doi: 10.1161/CIRCRESAHA.109.212530
Wang, Y. (2013). Attenuation of berberine on lipopolysaccharide-induced inflammatory and apoptosis responses in β-cells via TLR4-independent JNK/NF-κB pathway. Pharmaceut. Biol. 52, 532–538. doi: 10.3109/13880209.2013.840851
Wang, Y., Campbell, T., Perry, B., Beaurepaire, C., and Qin, L. (2011b). Hypoglycemic and insulin-sensitizing effects of berberine in high-fat diet- and streptozotocin-induced diabetic rats. Metabolism 60, 298–305. doi: 10.1016/j.metabol.2010.02.005
Wang, Z.-S., Lu, F. E., Xu, L. J., and Dong, H. (2010b). Berberine reduces endoplasmic reticulum stress and improves insulin signal transduction in Hep G2 cells. Acta Pharmacol. Sinica 31, 578–584. doi: 10.1038/aps.2010.30
Wu, D., Wen, W., and Qi, C. L. (2012). Ameliorative effect of berberine on renal damage in rats with diabetes induced by high-fat diet and streptozotocin. Phytomedicine 19, 712–718. doi: 10.1016/j.phymed.2012.03.003
Wu, S., Lu, F. E., and Dong, H. (2013). Effect of berberine on the endoplasmic reticulum stress-related apoptosis in pancreatic β cells. Chin. J. Diabetes 21, 544–547. doi: 10.3969/j.issn.1006-6187.2013.06.021
Wu, Y. S., Chen, Y.-T., Bao, Y.-T., Li, Z.-M., Zhou, X.-J., He, J.-N., et al. (2016). Identification and verification of potential therapeutic target genes in berberine-treated zucker diabetic fatty rats through bioinformatics analysis. PLoS ONE 11:e0166378. doi: 10.1371/journal.pone.0166378
Xie, W., and Du, L. (2011). Diabetes is an inflammatory disease: evidence from traditional Chinese medicines. Diabetes Obes. Metab. 13, 289–301. doi: 10.1111/j.1463-1326.2010.01336.x
Xie, W., Gu, D., Li, J., Cui, K., and Zhang, Y. (2011). Effects and action mechanisms of berberine and rhizoma coptidis on gut microbes and obesity in high-fat diet-fed C57BL/6J mice. PLoS ONE 6:e24520. doi: 10.1371/journal.pone.0024520
Xie, X., Chang, X., and Chen, L. (2013b). Berberine ameliorates experimental diabetes-induced renal inflammation and fibronectin by inhibiting the activation of RhoA/ROCK signaling. Mol. Cell. Endocrinol. 381:56–65. doi: 10.1016/j.mce.2013.07.019
Xie, X., Peng, J., and Chang, X. (2013a). Activation of RhoA/ROCK regulates NF-κB signaling pathway in experimental diabetic nephropathy. Mol. Cell. Endocrinol. 369, 86–97. doi: 10.1016/j.mce.2013.01.007
Xie, Z., Zhang, J., Wu, J., Viollet, B., and Zou, M. H. (2008). Upregulation of mitochondrial uncoupling protein-2 by the AMP-Activated protein kinase in endothelial cells attenuates oxidative stress in diabetes. Diabetes 57, 3222–3230. doi: 10.2337/db08-0610
Xing, L. J., Zhang, L., Liu, T., Hua, Y. Q., Zheng, P. Y., and Ji, G. (2011). Berberine reducing insulin resistance by up-regulating IRS-2 mRNA expression in nonalcoholic fatty liver disease (NAFLD) rat liver. Eur. J. Pharmacol. 668, 467–471. doi: 10.1016/j.ejphar.2011.07.036
Xu, M., Xiao, Y., Yin, J., Hou, W., Yu, X., Shen, L., et al. (2014). Berberine promotes glucose consumption independently of AMP-activated protein kinase activation. PLoS ONE 9:e103702. doi: 10.1371/journal.pone.0103702
Xue, X. J., Piao, H., and Nakajima, A. (2005). Tumor necrosis factor α (TNFα) induces the unfolded protein response (UPR) in a reactive oxygen species (ROS)-dependent fashion, and the UPR counteracts ROS accumulation by TNFα. J. Biol. Chem. 280, 33917–33925. doi: 10.1074/jbc.M505818200
Yang, J., and Yin, J. H. (2012). Berberine improves insulin sensitivity by inhibiting fat store and adjusting adipokines profile in human preadipocytes and metabolic syndrome patients. Evid. Based Compl. Alternat. Med. 2012:363845. doi: 10.1155/2012/363845
Yang, Q. M., Hu, S.-P., and Zhang, Y.-P. (2011). Effect of berberine on expressions of uncoupling protein-2 mRNA and protein in hepatic tissue of non-alcoholic fatty liver disease in rats. Chin. J. Integr. Med. 17, 205–211. doi: 10.1007/s11655-011-0668-4
Yao, J., Kong, W., and Jiang, J. (2015). Learning from berberine: treating chronic diseases through multiple targets. Sci. China 58, 854–859. doi: 10.1007/s11427-013-4568-z
Yi, P., Lu, F. E., Xu, L. J., Chen, G., Dong, H., and Wang, K. F. (2008). Berberine reverses free-fatty-acid-induced insulin resistance in 3T3-L1 adipocytes through targeting IKKβ. World J. Gastroenterol. 14, 876–883. doi: 10.3748/wjg.14.876
Yin, J., Ye, J., and Jia, W. (2012). Effects and mechanisms of berberine in diabetes treatment. Acta Pharmaceut. Sinica B 2, 327–334. doi: 10.1016/j.apsb.2012.06.003
Yin, J., Zhang, H., and Ye, J. (2008). Traditional Chinese medicine in treatment of metabolic syndrome. Endocr. Metab. Immune Disord. 8, 99–111. doi: 10.2174/187153008784534330
Zhang, H., Kong, W. J., and Shan, Y. Q. (2010b). Protein kinase D activation stimulates the transcription of the insulin receptor gene. Mol. Cell. Endocrin. 330, 25–32. doi: 10.1016/j.mce.2010.07.022
Zhang, H., Wei, J., Xue, R., Wu, J. D., Zhao, W., Wang, Z. Z., et al. (2010a). Berberine lowers blood glucose in type 2 diabetes mellitus patients through increasing insulin receptor expression. Metabolism 59, 285–292. doi: 10.1016/j.metabol.2009.07.029
Zhang, M., and Chen, L. (2012). Berberine in type 2 diabetes therapy: a new perspective for an old antidiarrheal drug. Acta Pharmaceut. Sinica B 2, 379–386. doi: 10.1016/j.apsb.2012.06.004
Zhang, M., Wang, C. M., Lv, X. Y., Wang, Y. J., Liu, J., and Chen, L. (2011a). Protective effects of berberine on aortic endothelial function in type 2diabeticrats. Chin. J. Gerontol. 4, 1160–1163. doi: 10.3969/j.issn.1005-9202.2011.07.029
Zhang, Q., Xiao, X., and Feng, K. (2011b). Berberine moderates glucose and lipid metabolism through multipathway mechanism. Evid. Based Complement. Alternat. Med. 2011:924851. doi: 10.1155/2011/924851
Zhang, Q., Xiao, X., Li, M., Li, W., Yu, M., Zhang, H., et al. (2014). Berberine moderates glucose metabolism through the GnRH-GLP-1 and MAPK pathways in the intestine. BMC Complement. Alternat. Med. 14:188.A doi: 10.1186/1472-6882-14-188
Zhang, Q., Li, Y., and Chen, L. (2015). Effect of berberine in treating type 2 diabetes mellitus and complications and its relevant mechanisms. Zhongguo Zhong Yao Za Zhi 40, 1660–1665.
Zhang, X., Zhao, Y., and Zhang, M. (2012). Structural changes of gut microbiota during berberine-mediated prevention of obesity and insulin resistance in high-fat diet-fed rats. PLoS ONE 7:e42529. doi: 10.1371/journal.pone.0042529
Zhang, Y., Li, X., and Zou, D. (2008). Treatment of type 2 diabetes and dyslipidemia with the natural plant alkaloid berberine. J. Clin. Endocrin. Metab. 93, 2559–2565. doi: 10.1210/jc.2007-2404
Zhou, J., Zhou, S., and Tang, J. (2009). Protective effect of berberine on beta cells in streptozotocin- and high-carbohydrate/high-fat diet-induced diabetic rats. Eur. J. Pharmacol. 606, 262–268. doi: 10.1016/j.ejphar.2008.12.056
Zhou, J. Y., and Zhou, S. W. (2011). Protective effect of berberine on antioxidant enzymes and positive transcription elongation factor b expression in diabetic rat liver. Fitoterapia 82, 184–189. doi: 10.1016/j.fitote.2010.08.019
Zhou, J., and Zhou, S. (2010). Berberine regulates peroxisome proliferator-activated receptors and positive transcription elongation factor b expression in diabetic adipocytes. Eur. J. Pharmacol. 649, 390–397. doi: 10.1016/j.ejphar.2010.09.030
Zhou, L., Yang, Y., and Wang, X. (2007). Berberine stimulates glucose transport through a mechanism distinct from insulin. Metabolism 56, 405–412. doi: 10.1016/j.metabol.2006.10.025
Keywords: pathogenesis, diabetes mellitus, oxidative stress, inflammation, cytokines, signaling pathways
Citation: Ma X, Chen Z, Wang L, Wang G, Wang Z, Dong X, Wen B and Zhang Z (2018) The Pathogenesis of Diabetes Mellitus by Oxidative Stress and Inflammation: Its Inhibition by Berberine. Front. Pharmacol. 9:782. doi: 10.3389/fphar.2018.00782
Received: 08 May 2018; Accepted: 27 June 2018;
Published: 27 July 2018.
Edited by:
Issy Laher, University of British Columbia, CanadaReviewed by:
Suowen Xu, University of Rochester, United StatesMaciej Banach, Medical University of Lodz, Poland
Copyright © 2018 Ma, Chen, Wang, Wang, Wang, Dong, Wen and Zhang. This is an open-access article distributed under the terms of the Creative Commons Attribution License (CC BY). The use, distribution or reproduction in other forums is permitted, provided the original author(s) and the copyright owner(s) are credited and that the original publication in this journal is cited, in accordance with accepted academic practice. No use, distribution or reproduction is permitted which does not comply with these terms.
*Correspondence: Zhichen Zhang, fhg4560@126.com
†These authors have contributed equally to this work.