- 1State Key Laboratory of Special Economic Animal Molecular Biology, Institute of Special Animal and Plant Sciences, Chinese Academy of Agricultural Sciences, Changchun, China
- 2College of Animal Science and Technology, Hebei Normal University of Science and Technology, Qinhuangdao, China
- 3College of Animal Science and Technology, Jilin Agricultural University, Changchun, China
- 4High-Tech Zone Laboratory of Public Test and Analysis Service, Shenyang, China
Background: Hair follicles play an essential role in the growth of hair. Epigallocatechin-3-gallate (EGCG), a catechin polyphenol in green tea, has various bioactivities. The present study aims to evaluate the effect of EGCG on the growth of mink hair follicles and investigate the possible molecular mechanisms.
Methods: The length of hair follicles was recorded up to 6 days in presence of 0.1–5 μM EGCG. Primary dermal papilla cells (DPCs) and outer root sheath cells (ORSCs) were treated with 0.25–4 μM EGCG, and their growth was evaluated by MTT assay and cell cycle detection. The levels of key molecules in sonic hedgehog (Shh) and protein kinase B (AKT) signaling pathways were further assessed by quantitative real-time PCR, western blot and immunofluorescence. To determine the involvement of Shh and AKT pathways in EGCG-mediated growth-promotion of ORSCs and DPCs, Shh pathway inhibitors cyclopamine and GANT61 or AKT pathway inhibitor LY294002 were employed, and then cell proliferation and cell cycle were analyzed.
Results: Data from ex vivo culture showed that, in presence of 0.5–2.5 μM EGCG, the growth of mink hair follicles was promoted. In vitro, the proliferation of DPCs and ORSCs was enhanced by 0.5–4 μM EGCG treatment. More cells entered S phase upon treatment of EGCG, accompanied with upregulation of cyclin D1 and cyclin E1. Furthermore, when exposed to EGCG, the Shh and AKT signaling pathways were activated in both hair follicles and primary DPCs and ORSCs. Inhibiting either of these two pathways partly reversed the effect of EGCG on proliferation and cell cycle of DPCs and ORSCs.
Conclusion: EGCG promotes the growth of mink hair follicles at concentrations of 0.5–2.5 μM. This growth-promoting effect of EGCG may be associated with the increased proliferation of DPCs and ORSCs through activating Shh and AKT signaling pathways.
Introduction
Hair follicles, which produce and moor hair shafts, are mini-organs with self-regeneration capability (Schneider et al., 2009). In postnatal life, hair follicles undergo regular cycles of anagen, catagen, and telogen. Anagen is a prolonged phase of rapid growth, with elongated and pigmented hair shafts. Then hair follicles cycle back to the relative quiescence (telogen) via an apoptosis-mediated regression (catagen) (Langan et al., 2015). Hair follicles can maintain some of the key characteristics even cultured ex vivo (Schneider et al., 2009). Hair follicles consist of several types of cells surrounding hair shafts, including outer root sheath cells (ORSCs), inner root sheath cells and dermal papilla cells (DPCs). Outer root sheaths, which are composed of a group of non-keratinized epithelial cells, are the connecting component between hair follicles and epidermis. ORSCs contribute to the development of hair follicles and represent a cell population with high proliferation capability during anagen phase (Vidal et al., 2005). DPCs are a group of specialized mesenchymal cells in hair follicle bulbs. In anagen, the number of DPCs is increased. They undergo asymmetric differentiation to generate progenies which are compositions of surrounding neighbors (Legue and Nicolas, 2005). DPCs also activate stem cells and lead to downward growth of follicles (Driskell et al., 2011). DPCs originating from various sources are able to induce de novo hair follicle formation (Jahoda et al., 1984). Thus, DPCs also play an important role in hair follicle development and contribute to hair growth (Driskell et al., 2011; Chi et al., 2013).
Epigallocatechin-3-gallate (EGCG) (Figure 3F) is the most abundant polyphenol in green tea, accounting for more than 50% of total polyphenols (Oliveira et al., 2016). As a major bioactive molecule in green tea, EGCG exhibits anti-inflammatory and anti-oxidative activities (Ahmed et al., 2002; Singh et al., 2002; Cavet et al., 2011; Gao et al., 2016; Shen et al., 2017), and performs protective effects in various diseases (Singh et al., 2003; Stangl et al., 2006; Wolfram et al., 2006). In addition, EGCG has been found to induce keratinocyte proliferation in human skin in vivo and in vitro (Chung et al., 2003). Importantly, Kwon et al. (2007) has reported that EGCG stimulates human hair growth via enhancing proliferation of DPCs. However, the detailed molecular mechanism of the pro-proliferative effect of EGCG has not been revealed.
The growth of hair follicles is a complex process regulated by a series of signals. The sonic hedgehog (Shh) and protein kinase B (AKT) signaling pathways are essential for the development and growth of hair follicles and transition between anagen and telogen (St-Jacques et al., 1998; Wang et al., 2000; Sohn et al., 2015). Activation of the Shh or AKT signals can enhance the proliferation of hair follicle cells and promote hair growth (Li et al., 2015; Lin et al., 2015; Sohn et al., 2015; Woo et al., 2017). EGCG has been demonstrated to trigger Shh signaling pathway in hippocampus of adult mice and enhance the hippocampal neurogenesis (Wang et al., 2012). In addition, EGCG can also activate AKT signaling pathway in various cells (Ding et al., 2017; Mi et al., 2017).
Based on these findings, we propose that these two signaling pathways may be involved in the growth-promoting effect of EGCG in hair follicles. The present study evaluated the effect of EGCG on mink hair follicles ex vivo and primary DPCs and ORSCs in vitro, and investigated the possible mechanism by focusing on the Shh and AKT signaling pathways.
Materials and Methods
Isolation and Culture of Hair Follicles
Anagen hair follicles were obtained from 4-month-old, male neovison visons (American minks). Small pieces of dorsal skin (∼1 cm2) were harvested and the hair shafts and subcutaneous fat were removed. The skin pieces were rinsed in PBS containing 100 U/ml penicillin and 0.1 mg/ml streptomycin, disinfected with iodine, discolored with 75% ethanol and then digested with Collagenase D (0.2 mg/ml; Sigma, St. Louis, MO, United States) at 4°C overnight. Subsequently, the hair follicles were isolated under a dissecting microscope and cultured in Williams E medium (Gibco, Grand Island, NY, United States) supplemented with insulin (10 μg/ml; Sigma), transferring (10 μg/ml; Sigma), hydrocortisone (10 ng/ml; Sigma), sodium selenite (10 ng/ml; Sigma), L-glutamine (2 mM; Sigma), penicillin (100 U/ml; Sigma) and streptomycin (0.1 mg/ml; Sigma) in a humid atmosphere at 37°C with 5% CO2 (Supplementary Figure S8). The hair follicles were treated with indicated concentrations of EGCG (0.1, 0.5, 1, 2.5, and 5 μM, Melonepharma, Dalian, China) for 6 days. The medium was changed every 2 days. Images of hair follicles were captured. The length of hair shafts extending from hair follicles was recorded with CellSens Standard 1.7. The daily growth of hair follicles was calculated. Daily growth of hair follicle = the length of hair follicle at one time point – the length of hair follicle at the day before. This study was performed in accordance with the Guidelines for the care and use of laboratory animals and approved by the Experimental Animal Management and Ethics Committee of the Institute of Special Animal and Plant Sciences in the Chinese Academy of Agricultural Sciences (Changchun, China).
Isolation and Culture of DPCs and ORSCs
Dermal papilla cells were isolated according to the report of Wen et al. (2018), with some modification. Dorsal skin pieces were digested with Dispase II (0.5 mg/ml; Sigma) at 4°C overnight and incubated at 37°C for additional 30 min. Then the tissue pieces were minced and digested with Collagenase D (0.2 mg/ml) at 37°C for 6 h until the dissociation of DPCs from liquid fats can be observed under a microscope. Thereafter, the DPCs were rinsed with PBS until the supernatant was clear, filtered through a 75-μm filter and digested with trypsin. The isolated DPCs were cultured in Dulbecco’s Modified Eagle’s Medium (DMEM; Gibco) supplemented with 10% fetal bovine serum (FBS; Gibco) in a humid atmosphere at 37°C with 5% CO2.
Outer root sheath cells were isolated according to previously reports (Cui et al., 2012; Li et al., 2012). The hair follicles obtained as described above were seeded in a culture dish, cultured in DMEM supplemented with 10% FBS, and maintained in a humid atmosphere at 37°C with 5% CO2. The medium was changed every 3 days. When the ORSCs gradually attached to the dishes, the hair follicles were picked up, the ORSCs were digested with trypsin and cultured in another dish in DMEM supplemented with 10% FBS.
Immunofluorescence
The isolated DPCs and ORSCs were characterized by α-smooth muscle actin (α-SMA) and cytokeratin 19 (CK19), respectively. Briefly, the cells were seeded onto glass slices. Then the cells were fixed in 4% paraformaldehyde for 30 min and permeabilized in 1% TritonX-100 for 30 min. Following blockade with 5% bovine serum albumin, the cells were incubated with anti-α-SMA antibody (Boster, Wuhan, China) or anti-CK19 antibody (Boster) respectively, overnight at 4°C. Then the cells were rinsed in PBS and incubated with FITC-labeled secondary antibodies (Beyotime, Haimen, China) for 60 min in the dark. Thereafter, the cells were counterstained with DAPI (Gene operation, Ann Arbor, MI, United States) and observed under a fluorescence microscope.
The isolated hair follicles were embedded with optimal cutting temperature compound (SAKURA, Torrance, CA, United States) and cut into 10 μm-sections. The sections were kept in antigen-retrieval buffer for 10 min and blocked with goat serum. Thereafter, the sections were rinsed in PBS and incubated with anti-Shh antibody (Proteintech, Wuhan, China) or anti-p-AKT antibody (Boster) overnight at 4°C. After rinsing in PBS, the sections were incubated with Cy3-labeled secondary antibodies (Beyotime) for 60 min at room temperature and counterstained with DAPI. The sections were observed under a fluorescence microscope.
EGCG and Inhibitors Treatments
To evaluate the effect of EGCG on cell growth of DPCs and ORSCs, DPCs and ORSCs were treated with different concentrations of EGCG (0.25, 0.5, 1, 2, and 4 μM) for 12, 24, or 48 h. To investigate whether the Shh and AKT signaling pathways are involved in the effect of EGCG on growth of DPCs and ORSCs, the cells were divided into the following groups, treated accordingly and incubated for 48 h: (1) Control; (2) EGCG (0.5 μM); (3) Cyclopamine (5 μM, Medchem Express, Monmouth Junction, NJ, United States); (4) EGCG+Cyclopamine; (5) GANT61 (10 μM; Medchem Express); (6) EGCG+GANT61; (7) LY2940002 (10 μM; Beyotime); (8) EGCG+LY2940002.
MTT Assay
Cells were seeded into 96-well plates (4 × 103 cells/well). After treatments as described above, MTT (0.5 mg/ml; Sigma) was added into each well and incubated at 37°C for 4 h. Thereafter, the supernatants were removed and 200 μl dimethyl sulfoxide (Sigma) was added into each well. The optical density at 490 nm was measured with a microplate reader (BIOTEK, Winooski, VT, United States).
Cell Cycle Detection
Cells were seeded in 6-well plates (1 × 105 cells/well) and treated with EGCG or signaling pathway inhibitors as described above. Thereafter, the cells were fixed with 70% ice-cold ethanol at 4°C for 2 h. After rinsing with PBS and resuspending in 500 μl binding buffer, 25 μl propidium iodide and 10 μl RNase A were added into cells for further incubation at 37°C for 30 min in darkness. The cell cycle was then analyzed through a flow cytometer (BD, Franklin Lakes, NJ, United States).
Quantitative Real-Time PCR (qRT-PCR)
Total RNA was extracted from hair follicles in each group using a RNA extraction kit (BioTeke, Beijing, China). The RNA was reverse transcribed into cDNA using Super M-MLV reverse transcriptase (BioTeke) and oligo(dT)15 according to the manufacturer’s protocol. The mRNA levels of Shh, patched (PTCH), smoothened (Smo), glioma-associated oncogene homolog 1 (Gli1) were measured by qRT-PCR (SYBR Green method) with cDNA as template and primers in Table 1. Relative mRNA levels were normalized to β-actin and calculated using 2-ΔΔCt method.
Western Blot
Proteins were extracted using radio immunoprecipitation assay lysis buffer with 1% phenylmethanesulfonyl fluoride (Beyotime). After measuring protein concentrations with a BCA protein assay kit (Beyotime), 30 μg proteins from each group were separated by SDS-PAGE and then transferred onto polyvinylidene fluoride membranes (Millipore, Bedford, MA, United States). Following blockade with 5% skim milk or 1% bovine serum albumin, the membranes were incubated with anti-cyclinB1, anti-cyclinD1 (1: 400; Boster), anti-PTCH (1: 500; Novus Biologicals, Littleton, CO, United States), anti-Smo (1: 100; Santa Cruz, Dallas, TX, United States), anti-Gli1 (1: 200; Santa Cruz), anti-AKT, anti-p-AKT (1: 500; Bioss, Beijing, China), anti-Shh (1: 1000; Abcam, Cambridge, United Kingdom) or anti-β-actin (1: 1000; Santa Cruz) antibodies at 4°C overnight. The membranes were rinsed in tris buffered saline with Tween (TBST) and incubated with corresponding horseradish peroxidase (HRP)-conjugated secondary antibodies (1: 5000; Beyotime) at 37°C for 45 min. Thereafter, the membranes were rinsed in TBST and visualized with an enhanced chemiluminescence detection kit (Beyotime). Relative protein levels were calculated using β-actin as the internal reference.
Statistical Analysis
All experiments were performed three times and the results are presented as mean ± SD. Differences among groups were analyzed using One-way Analysis of Variance followed by Bonferroni’s mutiple comparison test as post hoc. P < 0.05 was considered significant.
Results
EGCG Promotes Hair Follicle Growth
In the present study, the effect of EGCG on hair follicle growth was investigated. The length of hair shafts extending from hair follicles was significantly increased after treatment with 0.5–2.5 μM EGCG, but a little bit of inhibition after treatment with 5 μM EGCG (Table 2 and Supplementary Figure S1). The morphology of hair follicles was shown in Supplementary Figure S9. These results demonstrated that EGCG promoted the growth of hair follicles at a concentration of 0.5–2.5 μM.
EGCG Promotes the Growth of DPCs and ORSCs
As the growth of DPCs and ORSCs is closely related to hair follicle growth, we also evaluated the effect of EGCG on the growth of DPCs and ORSCs. The isolated DPCs and ORSCs were characterized by α-SMA and CK19 respectively (Supplementary Figure S2). EGCG at 0.25 μM did not affect the growth of DPCs or ORSCs. While, EGCG at 0.5–4 μM significantly increased the growth of these cells compared with the control cells (Figures 1A,B). These results demonstrated that EGCG promoted the growth of DPCs and ORSCs at a concentration of 0.5–4 μM.
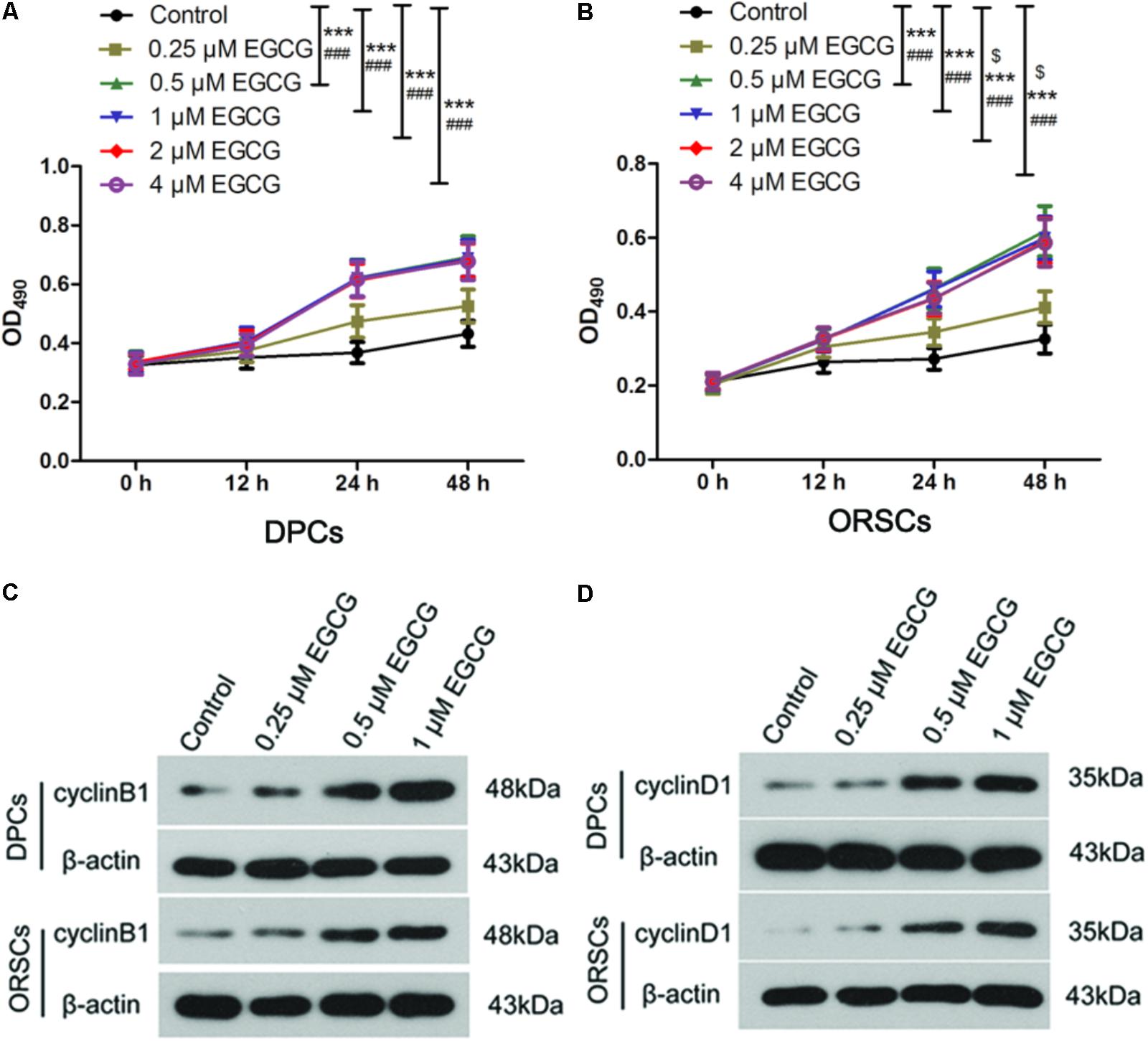
FIGURE 1. Epigallocatechin-3-gallate (EGCG) accelerates the growth of DPCs and ORSCs. (A,B) After treatment with 0.25, 0.5, 1, 2, and 4 μM EGCG for 12, 24, and 48 h, the cell viability of DPCs and ORSCs was assessed by MTT assay. $p < 0.05 for 12 h, ∗∗∗p < 0.001 for 24 h, ###p < 0.001 for 48 h, all compared with the control group. (C) After treatment with different concentrations of EGCG, the protein level of cyclinB1 in DPCs and ORSCs was detected by western blot. β-actin served as the internal reference. (D) Western blot was performed to detect the protein level of cyclinD1 in DPCs and ORSCs after treatment with EGCG. All experiments were repeated three times. The results are presented as mean ± SD.
As EGCG at concentrations of 0.5, 1, 2, and 4 μM resulted in similar results, 0.25, 0.5, and 1 μM EGCG were selected for the subsequent experiments. Cell cycle is an important event that impacts cell growth. In this study, we detected the effect of EGCG on cell cycle in DPCs and ORSCs by flow cytometry. Compared with the control cells, treatment with 0.5 and 1 μM EGCG increased the proportion of cells those entered into S phase (Table 3 and Supplementary Figure S3A), which indicates that EGCG accelerates the cell cycle process. Cyclins are important regulators of cell cycle. The results of western blot showed that, after treatment with 0.5 and 1 μM EGCG, the protein levels of cyclinB1 and cyclinD1 were increased significantly compared with the control cells (Figures 1C,D and Supplementary Figures S3B,C). These results provided further evidence for the growth-promoting effect of EGCG.
EGCG Activates the Shh and AKT Signaling Pathways
The Shh and AKT signaling pathways play important roles in hair follicle growth. In our study, the results of qRT-PCR showed that the mRNA levels of Shh, PTCH, Smo and Gli1 in hair follicles were increased to 4.48 ± 0.92-, 4.18 ± 0.85-, 4.71 ± 0.98-, and 1.67 ± 0.41-fold, respectively, after treatment with 0.5 μM EGCG, and to 2.34 ± 0.51-, 2.46 ± 0.57-, 2.18 ± 0.49-, and 1.58 ± 0.36-fold, respectively, after treatment with 1 μM EGCG (Figures 2A–D). The results of Western blot analysis also showed that the protein levels of Shh, PTCH, Smo and Gli1 in hair follicles were increased significantly after EGCG treatment (Figures 2E–H and Supplementary Figures S4A–D). The Shh level in hair follicles was also evaluated by immunofluorescence. As shown in Figure 2I, the Shh level was increased after treatment with EGCG, which was consistent with the results of western blot. These results indicated that the Shh signaling pathway was activated after treatment with EGCG. In addition, the phosphorylation level of AKT was also markedly increased to 4.91 ± 1.19-fold after treatment with 0.5 μM EGCG and to 2.30 ± 0.6-fold after treatment with 1 μM EGCG (Figure 2J and Supplementary Figure S4E). Similar results were gotten through immunofluorescence (Figure 2K), indicating that the AKT signaling pathway was activated.
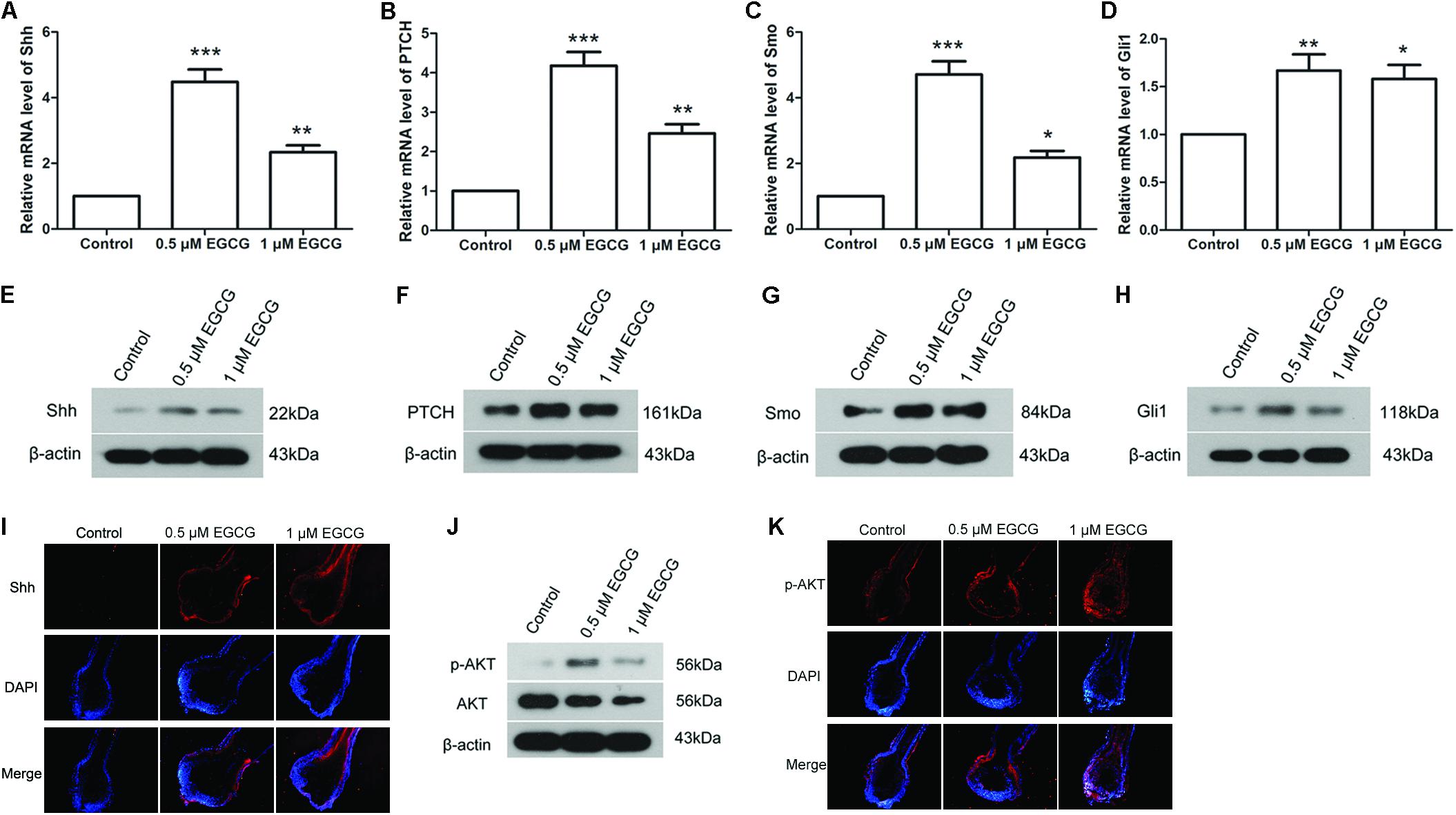
FIGURE 2. Epigallocatechin-3-gallate activates the Shh and AKT signaling pathways in hair follicles. After treatment with 0.5 and 1 μM EGCG, the mRNA levels of Shh (A), PTCH (B), Smo (C), and Gli1 (D) in hair follicles were detected by qRT-PCR. The relative mRNA levels were calculated using 2-ΔΔCt method. Western blot was also performed to detect the protein levels of Shh (E), PTCH (F), Smo (G), and Gli1 (H) in hair follicles. β-actin served as the internal reference. (I) Level of Shh in hair follicles was assessed by immunofluorescence. Red fluorescence: Shh; blue fluorescence: DAPI. (J) The phosphorylation level of AKT was assessed by western blot. (K) Level of p-AKT in hair follicles was assessed by immunofluorescence. Red fluorescence: p-AKT; blue fluorescence: DAPI. Each experiment was repeated three times and the results are presented as mean ± SD. ∗p < 0.05, ∗∗p < 0.01, ∗∗∗p < 0.001 compared with the control group.
The effect of EGCG on the Shh and AKT signaling pathways was also investigated in DPCs and ORSCs. After treatment with 0.5 μM or 1 μM EGCG, the protein levels of Shh, PTCH, Smo and Gli1 were significantly increased in both DPCs and ORSCs compared with the control cells (Figures 3A–D and Supplementary Figures S5A–D). Additionally, phosphorylated AKT level was also elevated in EGCG-treated DPCs and ORSCs (Figure 3E and Supplementary Figures S5E,F). These results suggested that the Shh and AKT signaling pathways might be involved in the effect of EGCG on hair follicle growth.
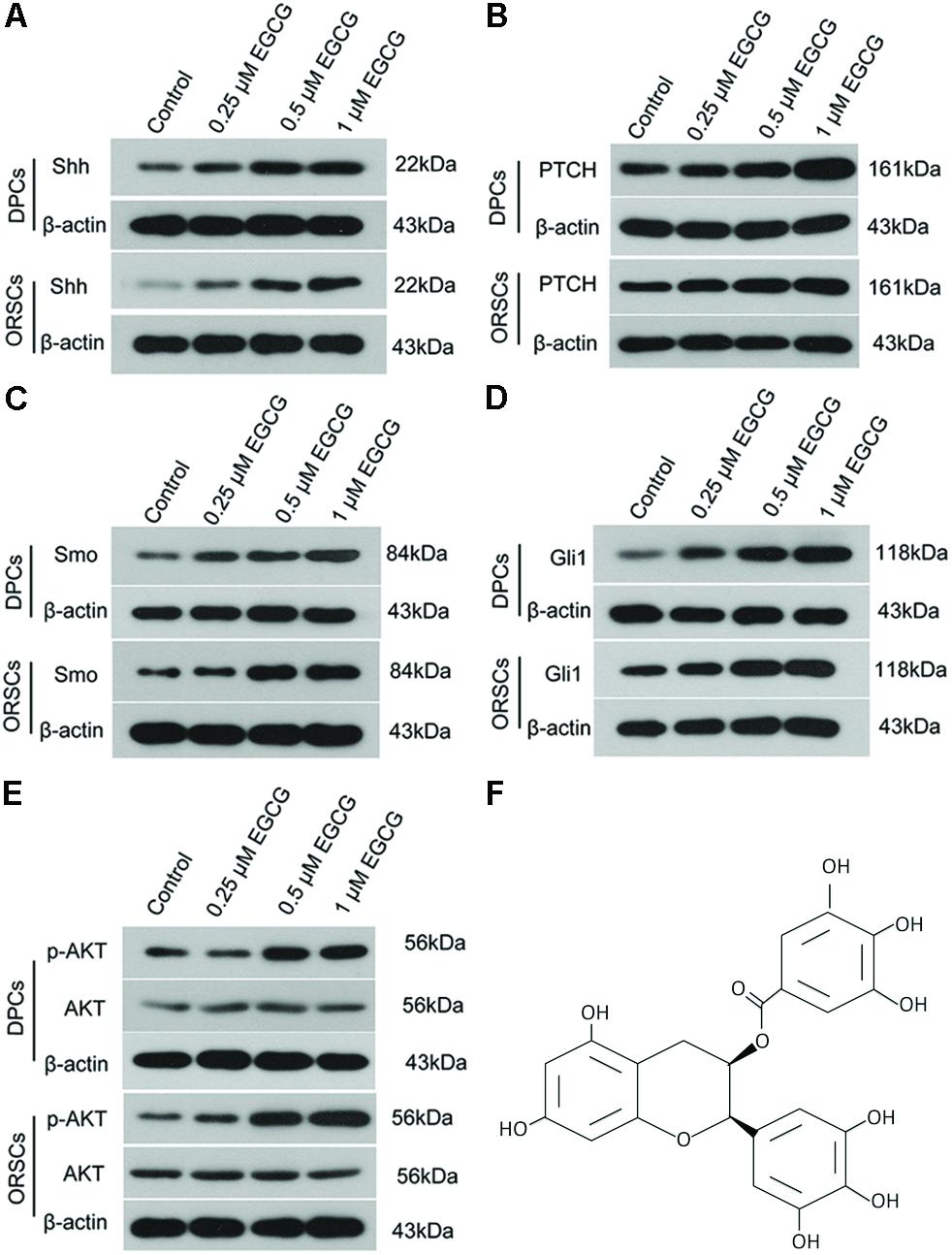
FIGURE 3. Epigallocatechin-3-gallate activates the Shh and AKT signaling pathways in DPCS and ORSCs. Upon treatment with EGCG, the protein levels of Shh (A), PTCH (B), Smo (C), and Gli1 (D) in DPCs and ORSCs were detected by western blot with β-actin as the internal reference. (E) Western blot was performed to assess the levels of AKT and p-AKT in each group with β-actin as the internal reference. (F) Chemical structure of EGCG. Each experiment was repeated three times.
EGCG Modulates the Growth of DPCs and ORSCs Through Shh and AKT Signaling Pathways
To investigate the role of Shh and AKT signaling pathways in the growth-promoting effect of EGCG, inhibitors of Shh signaling pathway (Cyclopamine and GANT61) and AKT signaling pathway (LY2940002) were employed in this study. The effects of these inhibitors on their corresponding signaling pathways were verified (Supplementary Figure S6). The results of the MTT assay demonstrated that the growth-promoting effect of EGCG on DPCs and ORSCs was abolished by Cyclopamine, GANT61 or LY2940002 (Figures 4A,B). Meanwhile, when co-treated with Cyclopamine, GANT61 or LY2940002, the effect of EGCG on cell cycle was also blocked, as evidenced by markedly increased cell population in G0/G1 phase compared with the cells treated with EGCG alone (Table 4 and Supplementary Figure S7A). Furthermore, the EGCG-induced upregulation of cyclinB1 and cyclinD1 expressions in DPCs and ORSCs were also markedly weakened by Cyclopamine, GANT61 or LY2940002 (Figures 4C,D and Supplementary Figures S7B,C). These results indicated that EGCG might modulate the growth of DPCs and ORSCs through Shh and AKT signaling pathways.
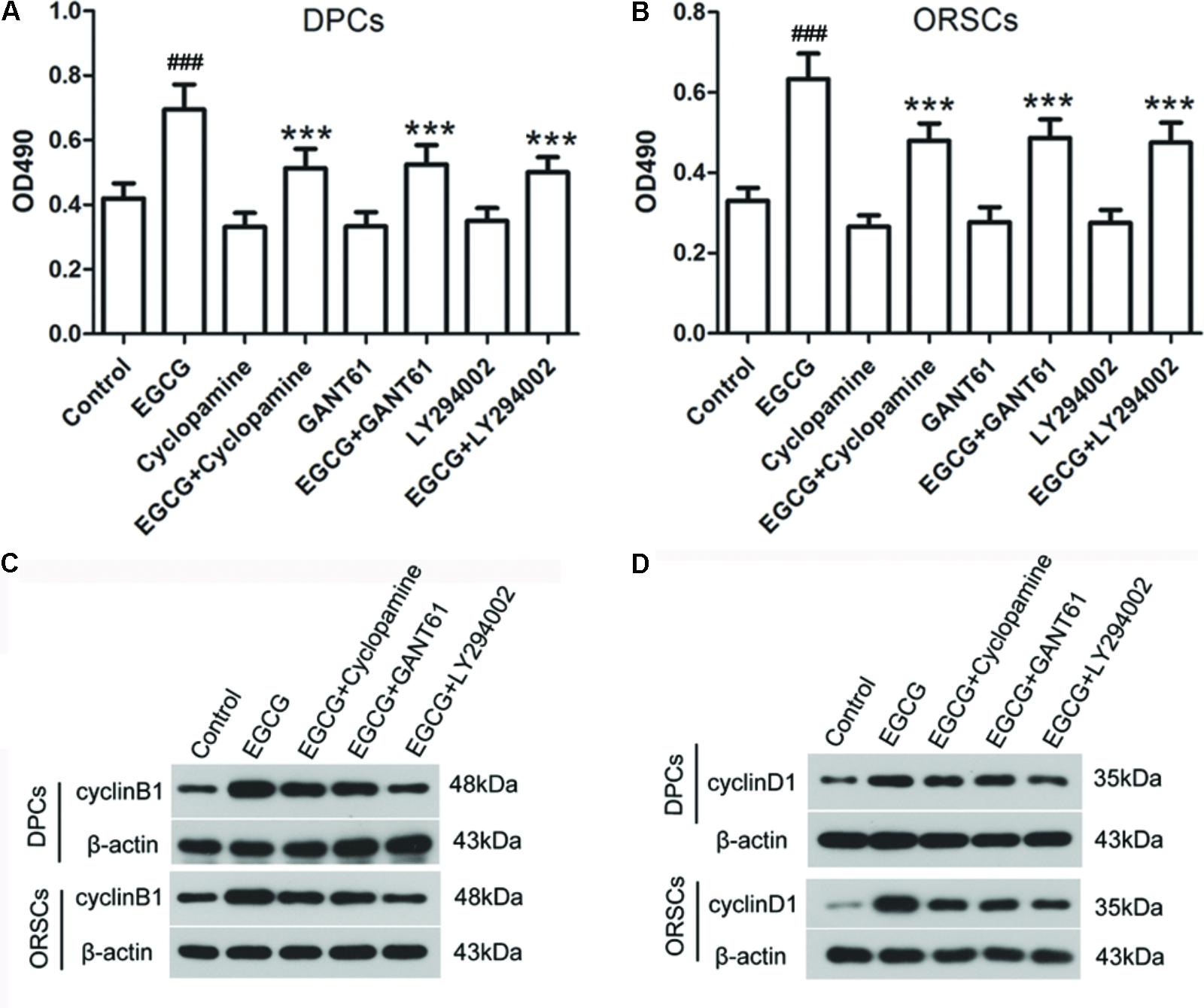
FIGURE 4. Shh and AKT signaling pathway inhibitors abolish the effect of EGCG on the growth of DPCs and ORSCs. (A,B) After treatment with EGCG and/or cyclopamine, GANT61 or LY294002, the cell viability of DPCs and ORSCs was assessed by MTT assay. (C,D) Protein levels of cyclinB1 and cyclinD1 in DPCs and ORSCs were assessed by western blot with β-actin as the internal reference. All experiments were repeated three times. The results are presented as mean ± SD. ###p < 0.001 compared with the control group; ∗∗∗p < 0.001 compared with the EGCG group.
Discussion
In the present study, we found that the growth of hair follicles was promoted after EGCG treatment ex vivo. In addition, EGCG increased the proliferation of DPCs and ORSCs, which was accompanied by the activation of Shh and AKT signals. Furthermore, inhibition of Shh and AKT signaling pathways abrogated the growth-promoting effect of EGCG. Our findings demonstrated that EGCG might promote mink hair follicle growth through Shh and AKT signaling pathways.
In our study, EGCG promoted the growth of mink hair follicles. This finding is consistent with the previous study in which EGCG promotes the growth in human hair follicles (Kwon et al., 2007). Our results confirm the growth-promoting effect of EGCG in mink hair follicles. Follicular melanogenesis, which is coupled to the anagen (Slominski et al., 2005), is also influenced by EGCG. EGCG can inhibit tyrosinase, a main enzyme in the regulation of melanin synthesis (No et al., 1999; Slominski et al., 2004), and reduce the production and secretion of melanin (Kim et al., 2004, 2018). Thus, we speculate that EGCG may also influence the melanogenesis of mink hair, but more explorations are needed.
In the present study, the growth of DPCs and ORSCs was enhanced by EGCG treatment. EGCG performs an interesting role in cell growth. EGCG is a potential anti-cancer agent, it has been demonstrated to suppress cell growth and metastasis of various cancers (Stuart et al., 2006; Gan et al., 2016; Luo et al., 2016; Shin et al., 2016; Yang et al., 2016; Zhang J. et al., 2016). In non-tumor cells, EGCG promotes the apoptosis of B lymphocytes in collagen-induced arthritis rats (Liu et al., 2012), and suppresses the antigen-induced T cell proliferation (Pae et al., 2010). Whereas, EGCG stimulates cell growth and differentiation of keratinocytes and neural stem cells (Hsu et al., 2003, 2005; Zhang Y. et al., 2016). These findings suggest that EGCG may modulate cell proliferation according to cell status. Consistent with our study, the report of Kwon et al also showed that EGCG promoted the proliferation of cultured human DPCs (Kwon et al., 2007).
Cell cycle is a vital process through which a complex multicellular organism becomes mature and injured organs are renewed. Previous studies have showed that EGCG affects cell cycle through regulating the expression of proteins associated with cell cycle, including cyclins and cyclin dependent kinases (Ahmad et al., 2002; Han et al., 2011; Lim and Cha, 2011; Zhang et al., 2012). Some reports reveal that EGCG leads to G1/S and G2/M arrest in cancer cells (Huang et al., 2009; Deng and Lin, 2011; Lim and Cha, 2011). On the contrary, our results showed that the cell cycle of DPCs and ORSCs was accelerated by EGCG, as evidenced by the increased cell population in S phase. Meanwhile, the protein levels of cyclinD1 and cyclinB1 which are closely related to G2/M and G1/S transition, were found to be increased upon the treatment of EGCG. These results demonstrated that EGCG promoted the cell cycle process of DPCs and ORSCs. This effect may be associated with the growth-promoting effect of EGCG on hair follicles of mink.
The Shh signaling pathway plays a critical role in vertebrate organogenesis and earlier studies show that it also plays an important role in hair growth (Gritli-Linde et al., 2007). Lack of Shh or Smo has been reported to impair the growth, morphogenesis and differentiation of hair follicles (St-Jacques et al., 1998; Chiang et al., 1999; Gritli-Linde et al., 2007). The Shh signaling cascade is initiated by the binding of functional Shh ligand to its receptor PTCH. The binding activates Smo by dissociating it from inhibition by PTCH and triggers the downstream transcription factors Gli. In our study, the levels of key proteins in the Shh signaling pathway, including Shh, PTCH, Smo and Gli1 were significantly upregulated upon EGCG treatment, both in hair follicles and in primary DPCs and ORSCs, indicating that the Shh signaling pathway was activated by EGCG. This observation is consistent with the previous finding in hippocampal neural progenitor cells (Wang et al., 2012). In addition, the Shh signaling pathway also has closely association with the cell cycle checkpoints. First, PTCH participates in the G2/M checkpoint through regulating cyclin B1 nuclear translocation. The binding of Shh ligand with PTCH facilitates cyclin B1 to localize to the nucleus (Barnes et al., 2005). Second, activated Gli directly upregulates cyclinD1 expression and enhances cyclinB1 expression via FOXM1 (Kasper et al., 2006; Katoh and Katoh, 2009). Here we found that EGCG enhanced the expressions of cyclinB1 and cyclinD1, and Shh inhibitors abolished this enhancement and blocked the growth-promoting effect of EGCG. These findings suggest that the activation of Shh signaling pathway may contribute to the growth-promoting effect of EGCG on mink hair follicles through regulating cell cycle checkpoint proteins. AKT, a downstream effector of phosphatidylinositol 3-kinase, is implicated in growth and survival of a variety of cells (Hers et al., 2011). Activation of AKT appears to contribute to hair growth (Sohn et al., 2015; Woo et al., 2017). Numerous studies have reported that EGCG regulates AKT signaling pathway. In line with these studies, we found that the AKT signaling pathway is activated by EGCG in cultured mink hair follicles and primary DPCs and ORSCs. In addition, AKT also regulates the expression of cyclins through glycogen synthase kinase-3β (Li et al., 2017), which is consistent with our observations that AKT signaling pathway inhibitor abated the growth-promoting effect of EGCG on hair follicles and cells, and downregulated cyclin expressions. These results suggest that the activation of the AKT signaling pathway may be involved in the effect of EGCG on mink hair follicle growth.
We realized that Shh and AKT signaling pathways might be not the only signaling pathways underlying the effect of EGCG. Other signaling pathways, such as Wnt, Notch and bone morphogenetic protein, are implicated in the development of hair follicles (Rishikaysh et al., 2014) and influenced by EGCG in several other reports (Jin et al., 2013; Fujita et al., 2017; Zhu et al., 2017). Thus, these signaling pathways may be also involved in the growth-promoting effect of EGCG on mink hair follicle growth, but more explorations are needed.
Conclusion
Epigallocatechin-3-gallate promoted the growth of mink hair follicles in vitro, and increased the proliferation of DPCs and ORSCs through activating the Shh and AKT signaling pathways.
Author Contributions
HZ and GL designed and conducted the study, analyzed the data, and prepared the manuscript. WN, SW, XS, HS, and TL collected and interpreted the data.
Funding
This study was supported by grants from the National Natural Science Foundation of China (No. 31301956) and the Science and Technology Development Project of Jilin Province (No. 20140520178JH).
Conflict of Interest Statement
The authors declare that the research was conducted in the absence of any commercial or financial relationships that could be construed as a potential conflict of interest.
Supplementary Material
The Supplementary Material for this article can be found online at: https://www.frontiersin.org/articles/10.3389/fphar.2018.00674/full#supplementary-material
FIGURE S1 | Epigallocatechin-3-gallate (EGCG) promotes the growth of hair follicles. Hair follicles were treated with 0.1, 0.5, 1, 2.5, or 5 μM of EGCG, and then images of hair follicles were captured on Day 0, Day 2, Day 4, and Day 6.
FIGURE S2 | Characteristics of DPCs and ORSCs. (A) The isolated DPCs were characterized by α-SMA through immunofluorescence. Green fluorescence: α-SMA. Scale bar = 200 μm. (B) Identities of ORSCs. Green fluorescence: CK19. Scale bar = 100 μm.
FIGURE S3 | Epigallocatechin-3-gallate accelerates the cell cycle of DPCs and ORSCs. (A) After treatment with 0.25, 0.5, and 1 μM EGCG for 48 h, the cell cycle distribution was detected by flow cytometry. (B,C) Western blot was performed to detect the protein levels of cyclinB1 and cyclinD1 in DPCs and ORSCs after treatment with EGCG. The relative protein levels of cyclinB1 and cyclinD1 were calculated according to the results of western blot. ∗∗∗p < 0.001 compared with the control group. The results are presented as mean ± SD.
FIGURE S4 | Epigallocatechin-3-gallate activates the Shh and AKT signaling pathways in hair follicles. Western blot was performed to detect the protein levels of Shh (A), PTCH (B), Smo (C), and Gli1 (D), and phosphorylation level of AKT (E) in hair follicles. The relative levels were calculated according to the results of western blot. The results are presented as mean ± SD. ∗p < 0.05, ∗∗∗p < 0.001 compared with the control group.
FIGURE S5 | Epigallocatechin-3-gallate activates the Shh and AKT signaling pathways in DPCS and ORSCs. Upon treatment with EGCG, the protein levels of Shh (A), PTCH (B), Smo (C), and Gli1 (D) in DPCs and ORSCs were detected by western blot. (E, F) Western blot was performed to assess the levels of AKT and p-AKT in each group. Relative levels were calculated according to the results of western blot. The results are presented as mean ± SD. ∗p < 0.05, ∗∗p < 0.01, ∗∗∗p < 0.001 compared with the control group.
FIGURE S6 | The effects of inhibitors Cyclopamine, GANT61 and LY2940002. (A,B) Cyclopamine and GANT61 decreased the level of Gli1 as evidenced by western blot. β-actin served as the internal reference. (C,D) LY2940002 decreased the phosphorylation of AKT. Western blot was carried out to detect the phosphorylation level of AKT. β-actin served as the internal reference. All experiments were repeated three times. The results are presented as mean ± SD. ∗∗∗p < 0.001 compared with the control group.
FIGURE S7 | Shh and AKT signaling pathway inhibitors abolish the effect of EGCG on the growth of DPCs and ORSCs. (A) Cell cycle distribution in DPCs and ORSCs in each group was detected by flow cytometry. (B,C) Protein levels of cyclinB1 and cyclinD1 in DPCs and ORSCs were assessed by western blot. Relative protein levels of cyclinB1 and cyclinD1 were calculated according to the results of western blot. The results are presented as mean ± SD. ###p < 0.001 compared with the control group; ∗p < 0.05, ∗∗p < 0.01, ∗∗∗p < 0.001 compared with the EGCG group.
FIGURE S8 | Isolation of mink hair follicles.
FIGURE S9 | HE staining for hair follicles upon treatment with EGCG.
References
Ahmad, N., Adhami, V. M., Gupta, S., Cheng, P., and Mukhtar, H. (2002). Role of the retinoblastoma (pRb)-E2F/DP pathway in cancer chemopreventive effects of green tea polyphenol epigallocatechin-3-gallate. Arch. Biochem. Biophys. 398, 125–131. doi: 10.1006/abbi.2001.2704
Ahmed, S., Rahman, A., Hasnain, A., Lalonde, M., Goldberg, V. M., and Haqqi, T. M. (2002). Green tea polyphenol epigallocatechin-3-gallate inhibits the IL-1 beta-induced activity and expression of cyclooxygenase-2 and nitric oxide synthase-2 in human chondrocytes. Free Radic. Biol. Med. 33, 1097–1105. doi: 10.1016/S0891-5849(02)01004-3
Barnes, E. A., Heidtman, K. J., and Donoghue, D. J. (2005). Constitutive activation of the shh-ptc1 pathway by a patched1 mutation identified in BCC. Oncogene 24, 902–915. doi: 10.1038/sj.onc.1208240
Cavet, M. E., Harrington, K. L., Vollmer, T. R., Ward, K. W., and Zhang, J. Z. (2011). Anti-inflammatory and anti-oxidative effects of the green tea polyphenol epigallocatechin gallate in human corneal epithelial cells. Mol. Vis. 17, 533–542.
Chi, W., Wu, E., and Morgan, B. A. (2013). Dermal papilla cell number specifies hair size, shape and cycling and its reduction causes follicular decline. Development 140, 1676–1683. doi: 10.1242/dev.090662
Chiang, C., Swan, R. Z., Grachtchouk, M., Bolinger, M., Litingtung, Y., Robertson, E. K., et al. (1999). Essential role for Sonic hedgehog during hair follicle morphogenesis. Dev. Biol. 205, 1–9. doi: 10.1006/dbio.1998.9103
Chung, J. H., Han, J. H., Hwang, E. J., Seo, J. Y., Cho, K. H., Kim, K. H., et al. (2003). Dual mechanisms of green tea extract (EGCG)-induced cell survival in human epidermal keratinocytes. FASEB J. 17, 1913–1915. doi: 10.1096/fj.02-0914fje
Cui, Z., Hu, Y., Wang, H., Zeng, Y., Dong, B., Zhu, H., et al. (2012). Establishment and characterization of outer root sheath (ORS) cell line from Jining grey goat. Biotechnol. Lett. 34, 433–440. doi: 10.1007/s10529-011-0799-x
Deng, Y. T., and Lin, J. K. (2011). EGCG inhibits the invasion of highly invasive CL1-5 lung cancer cells through suppressing MMP-2 expression via JNK signaling and induces G2/M arrest. J. Agric. Food Chem. 59, 13318–13327. doi: 10.1021/jf204149c
Ding, M. L., Ma, H., Man, Y. G., and Lv, H. Y. (2017). Protective effects of a green tea polyphenol, epigallocatechin-3-gallate, against sevoflurane-induced neuronal apoptosis involve regulation of CREB/BDNF/TrkB and PI3K/Akt/mTOR signalling pathways in neonatal mice. Can. J. Physiol. Pharmacol. 95, 1396–1405. doi: 10.1139/cjpp-2016-0333
Driskell, R. R., Clavel, C., Rendl, M., and Watt, F. M. (2011). Hair follicle dermal papilla cells at a glance. J. Cell Sci. 124(Pt 8), 1179–1182. doi: 10.1242/jcs.082446
Fujita, K., Otsuka, T., Yamamoto, N., Kainuma, S., Ohguchi, R., Kawabata, T., et al. (2017). (-)-Epigallocatechin gallate but not chlorogenic acid upregulates osteoprotegerin synthesis through regulation of bone morphogenetic protein-4 in osteoblasts. Exp. Ther. Med. 14, 417–423. doi: 10.3892/etm.2017.4491
Gan, R. Y., Li, H. B., Sui, Z. Q., and Corke, H. (2016). Absorption, metabolism, anti-cancer effect and molecular targets of epigallocatechin gallate (EGCG): an updated review. Crit. Rev. Food Sci. Nutr. 58, 924–941. doi: 10.1080/10408398.2016.1231168
Gao, Z., Han, Y., Hu, Y., Wu, X., Wang, Y., Zhang, X., et al. (2016). Targeting HO-1 by epigallocatechin-3-gallate reduces contrast-induced renal injury via anti-oxidative stress and anti-inflammation pathways. PLoS One 11:e0149032. doi: 10.1371/journal.pone.0149032
Gritli-Linde, A., Hallberg, K., Harfe, B. D., Reyahi, A., Kannius-Janson, M., Nilsson, J., et al. (2007). Abnormal hair development and apparent follicular transformation to mammary gland in the absence of hedgehog signaling. Dev. Cell 12, 99–112. doi: 10.1016/j.devcel.2006.12.006
Han, D. W., Lee, M. H., Kim, H. H., Hyon, S. H., and Park, J. C. (2011). Epigallocatechin-3-gallate regulates cell growth, cell cycle and phosphorylated nuclear factor-kappaB in human dermal fibroblasts. Acta Pharmacol. Sin. 32, 637–646. doi: 10.1038/aps.2011.17
Hers, I., Vincent, E. E., and Tavare, J. M. (2011). Akt signalling in health and disease. Cell. Signal. 23, 1515–1527. doi: 10.1016/j.cellsig.2011.05.004
Hsu, S., Bollag, W. B., Lewis, J., Huang, Q., Singh, B., Sharawy, M., et al. (2003). Green tea polyphenols induce differentiation and proliferation in epidermal keratinocytes. J. Pharmacol. Exp. Ther. 306, 29–34. doi: 10.1124/jpet.103.049734
Hsu, S., Yamamoto, T., Borke, J., Walsh, D. S., Singh, B., Rao, S., et al. (2005). Green tea polyphenol-induced epidermal keratinocyte differentiation is associated with coordinated expression of p57/KIP2 and caspase 14. J. Pharmacol. Exp. Ther. 312, 884–890. doi: 10.1124/jpet.104.076075
Huang, C. H., Tsai, S. J., Wang, Y. J., Pan, M. H., Kao, J. Y., and Way, T. D. (2009). EGCG inhibits protein synthesis, lipogenesis, and cell cycle progression through activation of AMPK in p53 positive and negative human hepatoma cells. Mol. Nutr. Food Res. 53, 1156–1165. doi: 10.1002/mnfr.200800592
Jahoda, C. A., Horne, K. A., and Oliver, R. F. (1984). Induction of hair growth by implantation of cultured dermal papilla cells. Nature 311, 560–562. doi: 10.1038/311560a0
Jin, H., Gong, W., Zhang, C., and Wang, S. (2013). Epigallocatechin gallate inhibits the proliferation of colorectal cancer cells by regulating Notch signaling. Onco Targets Ther. 6, 145–153. doi: 10.2147/OTT.S40914
Kasper, M., Schnidar, H., Neill, G. W., Hanneder, M., Klingler, S., Blaas, L., et al. (2006). Selective modulation of Hedgehog/GLI target gene expression by epidermal growth factor signaling in human keratinocytes. Mol. Cell. Biol. 26, 6283–6298. doi: 10.1128/MCB.02317-05
Katoh, Y., and Katoh, M. (2009). Hedgehog target genes: mechanisms of carcinogenesis induced by aberrant hedgehog signaling activation. Curr. Mol. Med. 9, 873–886. doi: 10.2174/156652409789105570
Kim, D. S., Park, S. H., Kwon, S. B., Li, K., Youn, S. W., and Park, K. C. (2004). (-)-Epigallocatechin-3-gallate and hinokitiol reduce melanin synthesis via decreased MITF production. Arch. Pharm. Res. 27, 334–339. doi: 10.1007/BF02980069
Kim, E., Hwang, K., Lee, J., Han, S. Y., Kim, E. M., Park, J., et al. (2018). Skin Protective Effect of Epigallocatechin Gallate. Int. J. Mol. Sci. 19:E173. doi: 10.3390/ijms19010173
Kwon, O. S., Han, J. H., Yoo, H. G., Chung, J. H., Cho, K. H., Eun, H. C., et al. (2007). Human hair growth enhancement in vitro by green tea epigallocatechin-3-gallate (EGCG). Phytomedicine 14, 551–555. doi: 10.1016/j.phymed.2006.09.009
Langan, E. A., Philpott, M. P., Kloepper, J. E., and Paus, R. (2015). Human hair follicle organ culture: theory, application and perspectives. Exp. Dermatol. 24, 903–911. doi: 10.1111/exd.12836
Legue, E., and Nicolas, J. F. (2005). Hair follicle renewal: organization of stem cells in the matrix and the role of stereotyped lineages and behaviors. Development 132, 4143–4154. doi: 10.1242/dev.01975
Li, J., Jiang, S., Chen, Y., Ma, R., Chen, J., Qian, S., et al. (2017). Benzene metabolite hydroquinone induces apoptosis of bone marrow mononuclear cells through inhibition of beta-catenin signaling. Toxicol. In Vitro 46, 361–369. doi: 10.1016/j.tiv.2017.08.018
Li, W., Lu, Z. F., Man, X. Y., Li, C. M., Zhou, J., Chen, J. Q., et al. (2012). VEGF upregulates VEGF receptor-2 on human outer root sheath cells and stimulates proliferation through ERK pathway. Mol. Biol. Rep. 39, 8687–8694. doi: 10.1007/s11033-012-1725-6
Li, Y., Han, M., Lin, P., He, Y., Yu, J., and Zhao, R. (2015). Hair growth promotion activity and its mechanism of polygonum multiflorum. Evid. Based Complement. Alternat. Med. 2015:517901. doi: 10.1155/2015/517901
Lim, Y. C., and Cha, Y. Y. (2011). Epigallocatechin-3-gallate induces growth inhibition and apoptosis of human anaplastic thyroid carcinoma cells through suppression of EGFR/ERK pathway and cyclin B1/CDK1 complex. J. Surg. Oncol. 104, 776–780. doi: 10.1002/jso.21999
Lin, W. H., Xiang, L. J., Shi, H. X., Zhang, J., Jiang, L. P., Cai, P. T., et al. (2015). Fibroblast growth factors stimulate hair growth through beta-catenin and Shh expression in C57BL/6 mice. Biomed Res. Int. 2015:730139. doi: 10.1155/2015/730139
Liu, D., Li, P., Song, S., Liu, Y., Wang, Q., Chang, Y., et al. (2012). Pro-apoptotic effect of epigallo-catechin-3-gallate on B lymphocytes through regulating BAFF/PI3K/Akt/mTOR signaling in rats with collagen-induced arthritis. Eur. J. Pharmacol. 690, 214–225. doi: 10.1016/j.ejphar.2012.06.026
Luo, K. W., Wei, C., Lung, W. Y., Wei, X. Y., Cheng, B. H., Cai, Z. M., et al. (2016). EGCG inhibited bladder cancer SW780 cell proliferation and migration both in vitro and in vivo via down-regulation of NF-kappaB and MMP-9. J. Nutr. Biochem. 41, 56–64. doi: 10.1016/j.jnutbio.2016.12.004
Mi, Y., Qi, G., Fan, R., Qiao, Q., Sun, Y., Gao, Y., et al. (2017). EGCG ameliorates high-fat- and high-fructose-induced cognitive defects by regulating the IRS/AKT and ERK/CREB/BDNF. FASEB J. 31, 4998–5011. doi: 10.1096/fj.201700400RR
No, J. K., Soung, D. Y., Kim, Y. J., Shim, K. H., Jun, Y. S., Rhee, S. H., et al. (1999). Inhibition of tyrosinase by green tea components. Life Sci. 65, L241–L246. doi: 10.1016/S0024-3205(99)00492-0
Oliveira, M. R., Nabavi, S. F., Daglia, M., Rastrelli, L., and Nabavi, S. M. (2016). Epigallocatechin gallate and mitochondria-A story of life and death. Pharmacol. Res. 104, 70–85. doi: 10.1016/j.phrs.2015.12.027
Pae, M., Ren, Z., Meydani, M., Shang, F., Meydani, S. N., and Wu, D. (2010). Epigallocatechin-3-gallate directly suppresses T cell proliferation through impaired IL-2 utilization and cell cycle progression. J. Nutr. 140, 1509–1515. doi: 10.3945/jn.110.124743
Rishikaysh, P., Dev, K., Diaz, D., Qureshi, W. M., Filip, S., and Mokry, J. (2014). Signaling involved in hair follicle morphogenesis and development. Int. J. Mol. Sci. 15, 1647–1670. doi: 10.3390/ijms15011647
Schneider, M. R., Schmidt-Ullrich, R., and Paus, R. (2009). The hair follicle as a dynamic miniorgan. Curr. Biol. 19, R132–R142. doi: 10.1016/j.cub.2008.12.005
Shen, H., Wu, N., Liu, Z., Zhao, H., and Zhao, M. (2017). Epigallocatechin-3-gallate alleviates paraquat-induced acute lung injury and inhibits upregulation of toll-like receptors. Life Sci. 170, 25–32. doi: 10.1016/j.lfs.2016.11.021
Shin, Y. S., Kang, S. U., Park, J. K., Kim, Y. E., Kim, Y. S., Baek, S. J., et al. (2016). Anti-cancer effect of (-)-epigallocatechin-3-gallate (EGCG) in head and neck cancer through repression of transactivation and enhanced degradation of beta-catenin. Phytomedicine 23, 1344–1355. doi: 10.1016/j.phymed.2016.07.005
Singh, R., Ahmed, S., Islam, N., Goldberg, V. M., and Haqqi, T. M. (2002). Epigallocatechin-3-gallate inhibits interleukin-1beta-induced expression of nitric oxide synthase and production of nitric oxide in human chondrocytes: suppression of nuclear factor kappaB activation by degradation of the inhibitor of nuclear factor kappaB. Arthritis Rheum. 46, 2079–2086. doi: 10.1002/art.10443
Singh, R., Ahmed, S., Malemud, C. J., Goldberg, V. M., and Haqqi, T. M. (2003). Epigallocatechin-3-gallate selectively inhibits interleukin-1beta-induced activation of mitogen activated protein kinase subgroup c-Jun N-terminal kinase in human osteoarthritis chondrocytes. J. Orthop. Res. 21, 102–109. doi: 10.1016/S0736-0266(02)00089-X
Slominski, A., Tobin, D. J., Shibahara, S., and Wortsman, J. (2004). Melanin pigmentation in mammalian skin and its hormonal regulation. Physiol. Rev. 84, 1155–1228. doi: 10.1152/physrev.00044.2003
Slominski, A., Wortsman, J., Plonka, P. M., Schallreuter, K. U., Paus, R., and Tobin, D. J. (2005). Hair follicle pigmentation. J. Invest. Dermatol. 124, 13–21. doi: 10.1111/j.0022-202X.2004.23528.x
Sohn, K. M., Jeong, K. H., Kim, J. E., Park, Y. M., and Kang, H. (2015). Hair growth-promotion effects of different alternating current parameter settings are mediated by the activation of Wnt/beta-catenin and MAPK pathway. Exp. Dermatol. 24, 958–963. doi: 10.1111/exd.12827
Stangl, V., Lorenz, M., and Stangl, K. (2006). The role of tea and tea flavonoids in cardiovascular health. Mol. Nutr. Food Res. 50, 218–228. doi: 10.1002/mnfr.200500118
St-Jacques, B., Dassule, H. R., Karavanova, I., Botchkarev, V. A., Li, J., Danielian, P. S., et al. (1998). Sonic hedgehog signaling is essential for hair development. Curr. Biol. 8, 1058–1068. doi: 10.1016/S0960-9822(98)70443-9
Stuart, E. C., Scandlyn, M. J., and Rosengren, R. J. (2006). Role of epigallocatechin gallate (EGCG) in the treatment of breast and prostate cancer. Life Sci. 79, 2329–2336. doi: 10.1016/j.lfs.2006.07.036
Vidal, V. P., Chaboissier, M. C., Lutzkendorf, S., Cotsarelis, G., Mill, P., Hui, C. C., et al. (2005). Sox9 is essential for outer root sheath differentiation and the formation of the hair stem cell compartment. Curr. Biol. 15, 1340–1351. doi: 10.1016/j.cub.2005.06.064
Wang, L. C., Liu, Z. Y., Gambardella, L., Delacour, A., Shapiro, R., Yang, J., et al. (2000). Regular articles: conditional disruption of hedgehog signaling pathway defines its critical role in hair development and regeneration. J. Invest. Dermatol. 114, 901–908. doi: 10.1046/j.1523-1747.2000.00951.x
Wang, Y., Li, M., Xu, X., Song, M., Tao, H., and Bai, Y. (2012). Green tea epigallocatechin-3-gallate (EGCG) promotes neural progenitor cell proliferation and sonic hedgehog pathway activation during adult hippocampal neurogenesis. Mol. Nutr. Food Res. 56, 1292–1303. doi: 10.1002/mnfr.201200035
Wen, T. C., Li, Y. S., Rajamani, K., Harn, H. J., Lin, S. Z., and Chiou, T. W. (2018). Effect of Cinnamomum osmophloeum kanehira leaf aqueous extract on dermal papilla cell proliferation and hair growth. Cell Transplant. 27, 256–263. doi: 10.1177/0963689717741139
Wolfram, S., Raederstorff, D., Preller, M., Wang, Y., Teixeira, S. R., Riegger, C., et al. (2006). Epigallocatechin gallate supplementation alleviates diabetes in rodents. J. Nutr. 136, 2512–2518. doi: 10.1093/jn/136.10.2512
Woo, H., Lee, S., Kim, S., Park, D., and Jung, E. (2017). Effect of sinapic acid on hair growth promoting in human hair follicle dermal papilla cells via Akt activation. Arch. Dermatol. Res. 309, 381–388. doi: 10.1007/s00403-017-1732-5
Yang, C., Du, W., and Yang, D. (2016). Inhibition of green tea polyphenol EGCG((-)-epigallocatechin-3-gallate) on the proliferation of gastric cancer cells by suppressing canonical wnt/beta-catenin signalling pathway. Int. J. Food Sci. Nutr. 67, 818–827. doi: 10.1080/09637486.2016.1198892
Zhang, J., Lei, Z., Huang, Z., Zhang, X., Zhou, Y., Luo, Z., et al. (2016). Epigallocatechin-3-gallate(EGCG) suppresses melanoma cell growth and metastasis by targeting TRAF6 activity. Oncotarget 7, 79557–79571. doi: 10.18632/oncotarget.12836
Zhang, X., Min, K. W., Wimalasena, J., and Baek, S. J. (2012). Cyclin D1 degradation and p21 induction contribute to growth inhibition of colorectal cancer cells induced by epigallocatechin-3-gallate. J. Cancer Res. Clin. Oncol. 138, 2051–2060. doi: 10.1007/s00432-012-1276-1
Zhang, Y., He, Q., Dong, J., Jia, Z., Hao, F., and Shan, C. (2016). Effects of epigallocatechin-3-gallate on proliferation and differentiation of mouse cochlear neural stem cells: Involvement of PI3K/Akt signaling pathway. Eur. J. Pharm. Sci. 88, 267–273. doi: 10.1016/j.ejps.2016.03.017
Keywords: dermal papilla cells, epigallocatechin-3-gallate, hair follicle growth, outer root sheath cells, protein kinase B, signaling pathway, sonic hedgehog signaling pathway
Citation: Zhang H, Nan W, Wang S, Song X, Si H, Li T and Li G (2018) Epigallocatechin-3-Gallate Promotes the Growth of Mink Hair Follicles Through Sonic Hedgehog and Protein Kinase B Signaling Pathways. Front. Pharmacol. 9:674. doi: 10.3389/fphar.2018.00674
Received: 13 December 2017; Accepted: 05 June 2018;
Published: 26 June 2018.
Edited by:
Wolff Mayer Kirsch, Loma Linda University Medical Center (LLUMC), United StatesReviewed by:
Lifeng Kang, The University of Sydney, AustraliaAndrzej T. Slominski, The University of Alabama at Birmingham, United States
Copyright © 2018 Zhang, Nan, Wang, Song, Si, Li and Li. This is an open-access article distributed under the terms of the Creative Commons Attribution License (CC BY). The use, distribution or reproduction in other forums is permitted, provided the original author(s) and the copyright owner are credited and that the original publication in this journal is cited, in accordance with accepted academic practice. No use, distribution or reproduction is permitted which does not comply with these terms.
*Correspondence: Guangyu Li, bGlfZ3Vhbmd5dTEyM0BzaW5hLmNvbQ==