- 1The Second Clinical College of Guangzhou University of Chinese Medicine, Guangdong Provincial Hospital of Chinese Medicine, Guangzhou, China
- 2Guangdong Provincial Key Laboratory of Clinical Research on Traditional Chinese Medicine Syndrome, Guangzhou, China
Emodin, a major active anthraquinone, frequently interacts with other drugs. As changes of efflux transporters on intestine are one of the essential reasons why the drugs interact with each other, a validated Ussing chamber technique was established to detect the interactions between emodin and efflux transporters, including P-glycoprotein (P-gp), multidrug-resistant associated protein 2 (MRP2), and multidrug-resistant associated protein 3 (MRP3). Digoxin, pravastatin, and teniposide were selected as the test substrates of P-gp, MRP2, and MRP3. Verapamil, MK571, and benzbromarone were their special inhibitors. The results showed that verapamil, MK571, and benzbromarone could increase digoxin, pravastatin, and teniposide absorption, and decrease their Er values, respectively. Verapamil (220 μM) could significantly increase emodin absorption at 9.25 μM. In the presence of MK571 (186 μM), the Papp values of emodin from M-S were significantly increased and the efflux ratio decreased. With the treatment of emodin (185, 370, and 740 μM), digoxin absorption was significantly decreased while teniposide increased. These results indicated that emodin might be the substrate of P-gp and MRP2. Besides, it might be a P-gp inducer and MRP3 inhibitor on enterocyte, which are reported for the first time. These results will be helpful to explain the drug–drug interaction mechanisms between emodin and other drugs and provide basic data for clinical combination therapy.
Introduction
Emodin (1,3,8trihydroxy-6-methylanthraquinone), a major active anthraquinone, is naturally present in some herbs which have been wildly used in Oriental countries, such as Rheum officinale Baill., Polygonum multijiorum Thunb., Polygonum cuspidatum Sieb. Et Zucc., etc. (Dong et al., 2016). In the past decades, emodin has been shown a wide spectrum of biological and pharmacological effects, such as hepatoprotective antiviral, anti-diabetic, anti-bacterial, anti-allergic, anti-osteoporotic, immunosuppressive, and neuroprotective activities (Dong et al., 2016; Monisha et al., 2016). Recent studies have placed emodin back into the limelight, which exhibits a good prospect in anticancer treatment with its anticancer activities against several types of cancer cells, such as lung carcinoma, gastric carcinoma, pancreatic cancer, and breast cancer, with apoptosis, anti-angiogenesis, and anti-proliferation as possible mechanisms of action (Heo et al., 2010; Wei et al., 2013; Li et al., 2014; Cha et al., 2015). Mitoxantrone, a commonly used anticancer drug, is the prodrug of emodin (Riahi et al., 2008).
In recent years, drug–drug interactions between emodin and other drugs attracted more and more researchers’ attention. Di et al. (2015) showed that piperine significantly improved the in vivo bioavailability of emodin and inhibited glucuronidation metabolism of emodin. Yu et al. (2017) demonstrated that 2,3,5,4’-tetrahydroxystilbene-2-β-D-glucoside could enhance the emodin absorption in a Caco-2 cell culture model. Meanwhile, many studies have shown synergistic effects between emodin and other antineoplastic drugs (Ko et al., 2010; Wang et al., 2010; Tan et al., 2011; Guo et al., 2013). Actually, changes of transporters on intestine affect the absorption characteristics of many drugs, which is one of the essential reasons why the drugs interact with each other. Some researchers have proved that emodin was the substrate of P-glycoprotein (P-gp) and multidrug-resistant associated protein 2 (MRP2; Teng et al., 2007, 2012; Liu et al., 2012). However, there were no reports about the research of emodin direct effects on intestinal transporters.
The Ussing chamber technique has been presently provided a physiologically relevant system for studying transepithelial transport of ion, drugs, and nutrients across various epithelial tissues. In this system, the drugs can be exposed at either mucosal or serosal levels, and therefore the absorption direction (M-S) and secretion direction (S-M) characteristics both can be detected. Furthermore, the usefulness of Ussing chambers for intestinal transport studies has long been recognized, and many researchers regard it as gold standards (Erik et al., 2016). Up to now, studies of P-gp influences in drug intestinal absorption by using this technique have been reported (Ballent et al., 2014; Xiao et al., 2016). However, there were few reports about testing and verifying this technique whether or not suitable for P-gp related studies. Moreover, other efflux transporters studies rarely reported by this technique.
In this study, a validated Ussing chamber technique was established and interaction studies between emodin and three efflux transporters including P-gp, MRP2, and multidrug-resistant associated protein 3 (MRP3) were carried out. To verify this Ussing chamber technique whether or not suitable for transepithelial transport studies related to efflux transporters, P-gp, MRP2, and MRP3 were employed as the typical proteins. Specific substrates and inhibitors were selected to detect P-gp, MRP2, and MRP3 activities and functions under our experimental conditions. Subsequently, interaction studies between emodin and these three efflux transporters were investigated. This is the first time to study the direct influence of emodin on rat intestinal P-gp, MRP2, and MRP3 functions by using this technique. It will be helpful to explain the drug–drug interaction mechanisms between emodin and other drugs and provide basic data for clinical combination therapy.
Materials and Methods
Chemicals and Reagents
Standards of digoxin and pravastatin were purchased from Chendu Rui Fensi Biotechnology Co., Ltd. (Chengdu, China). Benzbromarone and teniposide were obtained from Guangzhou Feibo Biotechnology Co., Ltd. (Guangzhou, China). Verapamil, topotecan, etoposide, and MK571 were obtained from Dalian Mellon Biotechnology Co., Ltd. (Dalian, China). Ginsenoside Rg1 and emodin were purchased from the National Institutes for Food and Drug Control (Beijing, China). Krebs’ Ringer bicarbonate (KRB) buffer is composed by 114 mM NaCl, 10 mM Glucose, 1.25 mM CaCl2⋅2H2O, 1.1 mM MgCl2⋅6H2O, 5.03 mM KCl, 0.30 mM NaH2PO4⋅H2O,1.65 mM Na2HPO4, and 25 mM NaHCO3 of pH 6.5 (Huang et al., 2016). Solvents were of HPLC grade and other chemicals used were of analytical grade.
Animals
The specific pathogen-free male Sprague–Dawley rats weighing 220 ± 20 g were obtained from Guangdong Medical Laboratory Animal Center (Guangzhou, China). Before starting the experiments, the rats were kept in an environmentally controlled breeding room (temperature: 22 ± 1°C, humidity: 50–70%) and fed standard laboratory food and water for 1 week. The animals were fasted overnight with free access to water before experiments. All animal studies were approved by the Institutional Animal Ethics Committee of Guangdong Provincial Hospital of Chinese Medicine.
Tissue Preparation
The four intestinal segments including duodenum, jejunum, ileum, and colon from anesthetized rats were immediately removed by surgery and washed with KRB solution. Each section of the intestinal segments was then placed in cold (on ice), bubbled (O2:CO2 95:5) KRB buffer. The four intestinal segments were then cut into 2 cm pieces, respectively, and serosas were removed by blunt dissection. Peyer’s patches were excluded from the experiment by visually identified. The stripped tissues were mounted in chambers with exposed surface area of 0.49 cm2. Temperature of the chambers was maintained at 37 ± 0.5°C. Each of the half-cells in the chambers was filled with 5 mL fresh KRB buffer (pH 6.5). Tissue viability was continuously controlled by potential difference (PD). Tissue with electrical values less than 2 mV was refused (Moazed and Hiebert, 2007; Heinen et al., 2013).
Model Validation for Efflux Transporters’ Studies
Digoxin, pravastatin, and teniposide were employed as the test substrates of P-gp, MRP2, and MRP3. Verapamil, MK571, and benzbromarone were inhibitors of these three efflux transporters (Table 1; Kool et al., 1999; Zhou et al., 2008; Ellis et al., 2013; Abbasi et al., 2016; Liu et al., 2017). The experiments were started after 30 min equilibration time, by changing the KRB buffer on both sides; 5 mL KRB solution with different test compounds was filled in the mucosal or serosal compartment; meanwhile, equal volume fresh buffer was added to the other compartment. In the inhibitory studies, the inhibitors verapamil, MK571, and benzbromarone were added to the mucosal side, respectively; 0.5 mL samples were withdrawn from the receiver compartment every 30 min and replaced with fresh KRB buffer. The bidirectional transport studies with specific inhibitors were assessed to validate this model whether or not suitable for P-gp, MRP2, and MRP3 studies. Samples were stored at –80°C until analysis (Deusser et al., 2013).
Interaction Studies Between Emodin and Efflux Transporters
In this study, the effects of the three efflux transporters on emodin intestinal absorption were investigated. Meanwhile, the influence of emodin on intestinal P-gp, MRP2, and MRP3 functions were also discussed. Solutions with three concentrations (low, middle, and high) of emodin were filled in the mucosal or serosal compartment to measure the bidirectional transport characteristic. In order to observe whether or which efflux transporters involved in emodin intestinal absorption process, verapamil, MK571, and benzbromarone were added to the mucosal side, respectively. In the emodin impact experiments, digoxin, pravastatin, and teniposide were employed as P-gp, MRP2, and MRP3 substrates. Changes of the bidirectional transport characteristic were measured before and after adding emodin to the mucosal side.
Preparation of Perfusate Samples
Frozen samples were adequately vortexed after thawing at the room temperature; 10 μL suitable IS solution was added to 200 μL samples; 200 μL methanol was added after a thorough vortex mixing for 30 s. The mixtures were then vortexed for 30 s and centrifuged at 15,000 rpm for 30 min. Finally, 5 μL of supernatant was injected into the LC-MS/MS system.
Liquid Chromatographic and Mass Spectrometric Conditions
The quantifications of the compounds were performed by the TSQ Quantum Ultra Triple Quadrupole LC-MS/MS system from Thermo Fisher Scientific. Chromatography was carried out using an Agilent Poroshell 120 SB-C18 (2.7 μm, 2.1 mm × 100 mm) column with a Phenomenex AFO-8497 C18 pre-column, operating at 30°C. Adaptive gradient elution methods were applied for the analytes. The flow rate of the mobile phase was kept at 0.2 mL min-1. Flow was directed to the ion spray interface. All measurements were carried out in negative ESI mode. Ion spray voltage was -2500 V. Vaporizer and capillary temperatures were set at 250 and 350°C, respectively. Auto sampler temperature was set at 10°C. Sheath gas and aux gas were set at 30 and 15 Arb, respectively. The selective reaction monitoring (SRM) transitions were at m/z 268.934→225.047 for emodin and m/z 779.147→475.447 for ginsenoside Rg1 [internal standard (IS)]. Other SRM settings and specific IS are shown in Table 1. Peak integrations and calibrations were carried out using LC Quan 2.5.2 software from Thermo Fisher Scientific. All the data were within the acceptable limits to meet the guidelines for bioanalytical methods.
Data Analysis
The Q (accumulative quantity), Papp (apparent permeability), Er (efflux ratio), Pr (Papp ratio), and Err (Er ratio) across the excited rat intestinal segments in the Ussing chamber were calculated using the following equations (Li et al., 2012; Huang et al., 2016):
where Cn (nM) is the concentration of the drug at the time point (n), Q (nM) is the accumulated absorption amount, A is the exposed surface area of the intestine (0.49 cm2), dQ/dt (nM⋅s-1) is the amount of the drug transported, and C0 (nM) is the initial concentration of the test drug. Experiments were performed in batches, so control groups were set as one for each batch.
Statistical Analyses
The data are presented as the mean ± SD for all experiments. Independent samples t-test was applied to compare the means between treatments. One-way ANOVA with LSD (equal variances assumed) or Danett T3 (equal variances not assumed) multiple comparison (post hoc) tests were used to evaluate statistical differences. A p-value of less than 0.05 was considered statistical significance.
Results
The PD Values for Rat Intestinal Segments
After 30 min equilibration time, PD was 7.16 ± 1.59, 7.23 ± 2.18, 6.50 ± 0.75, and 4.12 ± 0.69 mV for duodenum, jejunum, ileum, and colon, respectively. Obviously, the PD values were higher for small intestine segments under this experimental condition. In general, the PD slightly decreased by time when the chambers with small amount drugs (<200 μM).
Model Validation for Efflux Transporters’ Studies Were Employed as the Test Substrates of P-gp, MRP2, and MRP3
The Papp and Er values of the test substances (digoxin, pravastatin, and teniposide) are summarized in Table 2 and Figure 1. The results showed that the inhibitors, including verapamil, MK571, and benzbromarone, could significantly increase the absorption of the aforesaid substances and inhibit their secretion, and thus their Er values were decreased. In P-gp validation study, the concentrations of the inhibitor (verapamil) were set at 220 and 440 μM for the small intestine segments and colon, respectively. It was observed that verapamil could significantly inhibit the effect of P-gp protein on jejunum, ileum, and colon. However, it was showed no statistic difference on duodenum after adding the inhibitor. These may be related to the distribution characteristic of P-gp on rat enterocyte. In MRP2 and MRP3 validation studies, the inhibitors MK571 (186 μM) and benzbromarone (470 μM) were also displayed significant inhibition effect on both jejunum and ileum. These results indicated that the Ussing chamber technique could be used to investigate the role of P-gp, MRP2, and MRP3 in drug intestinal transport studies. Though the distribution characteristics of the effluxes were different, both jejunum and ileum were suitable for the study. Finally, jejunum was chosen for further studies because of the most stable data it revealed during model validation research.
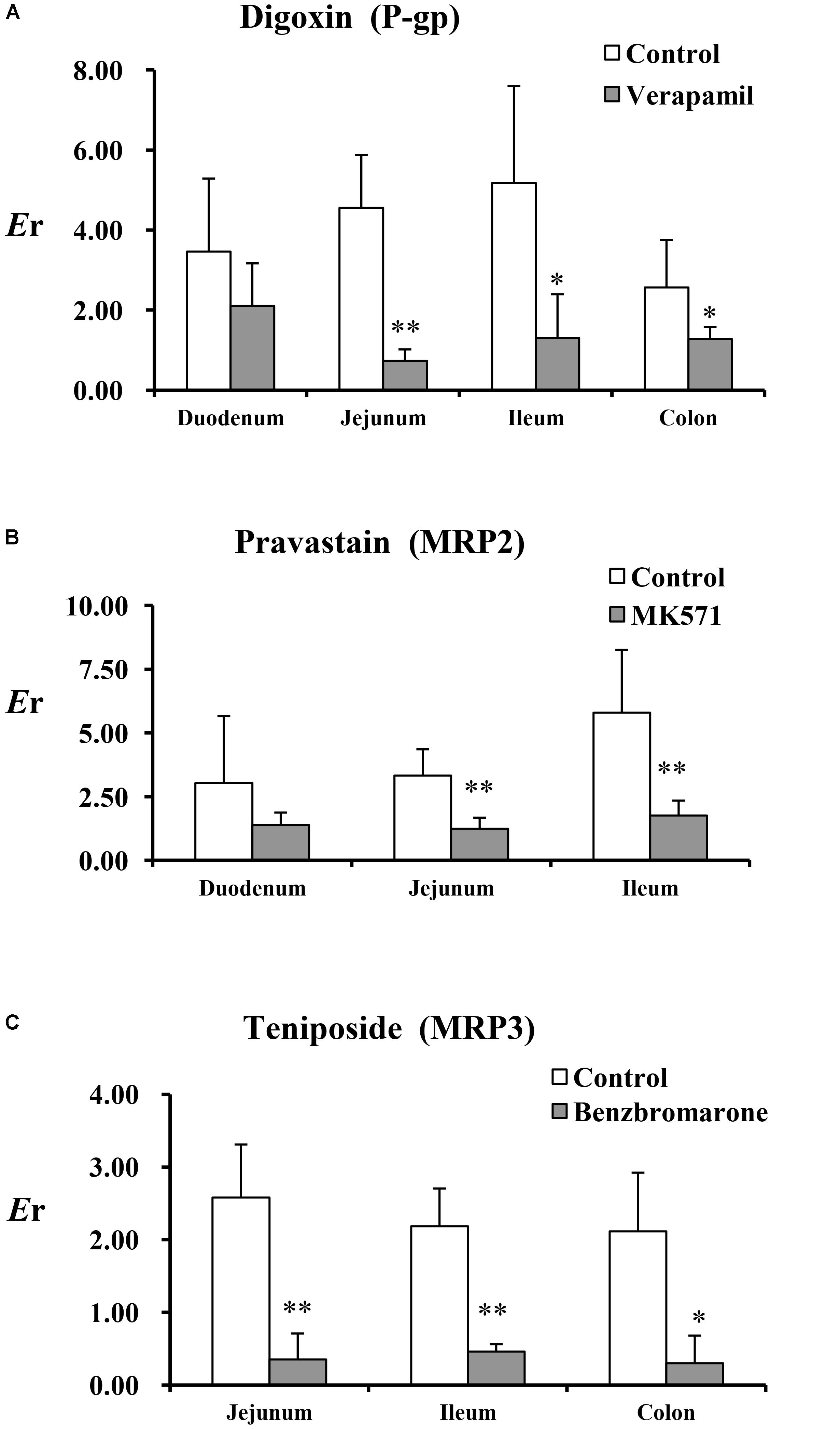
FIGURE 1. The Er of the test substances in perfused rat intestinal segments. (A) Digoxin, (B) pravastatin, and (C) teniposide were employed as the test substrates of P-gp, MRP2, and MRP3. Verapamil, MK571, and benzbromarone were inhibitors of P-gp, MRP2, and MRP3. Each bar represents the mean with SD of five measurements (∗p < 0.05 and ∗∗p < 0.01, compared to the control according to independent samples t-test).
The Absorption Characteristics of Emodin
The absorption characteristics of emodin at three concentrations (9.25, 18.5, and 37 μM) in different intestinal segments were investigated. The intestinal absorption rates of emodin displayed no regioselectivity whereas the Papp values from M-S were very low at the lowest concentration (Figure 2). These indicated that some efflux transporters may involved in emodin intestinal transport. The Er values of emodin in jejunum were all more than five at the three concentrations, pointing out that efflux transporters were involved in emodin intestinal absorption (Figure 3).
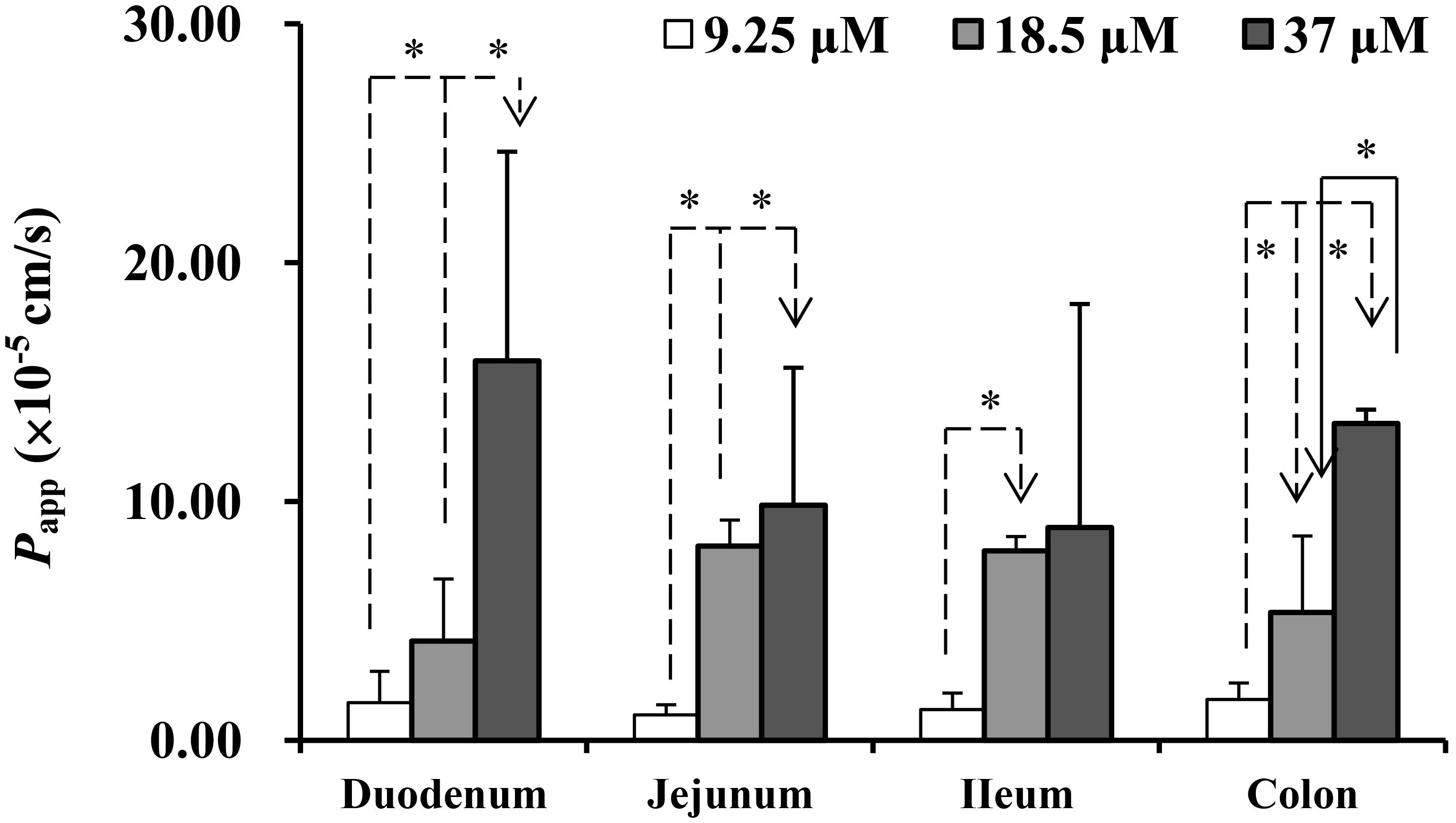
FIGURE 2. The Papp of emodin from mucosal to the serosal side in different intestinal segments. Experiments were conducted at three concentrations (9.25, 18.5, and 36.5 μM). Each bar represents the mean with SD of five measurements (∗p < 0.05, statistically significant differences among different concentrations, according to a one-way ANOVA test).
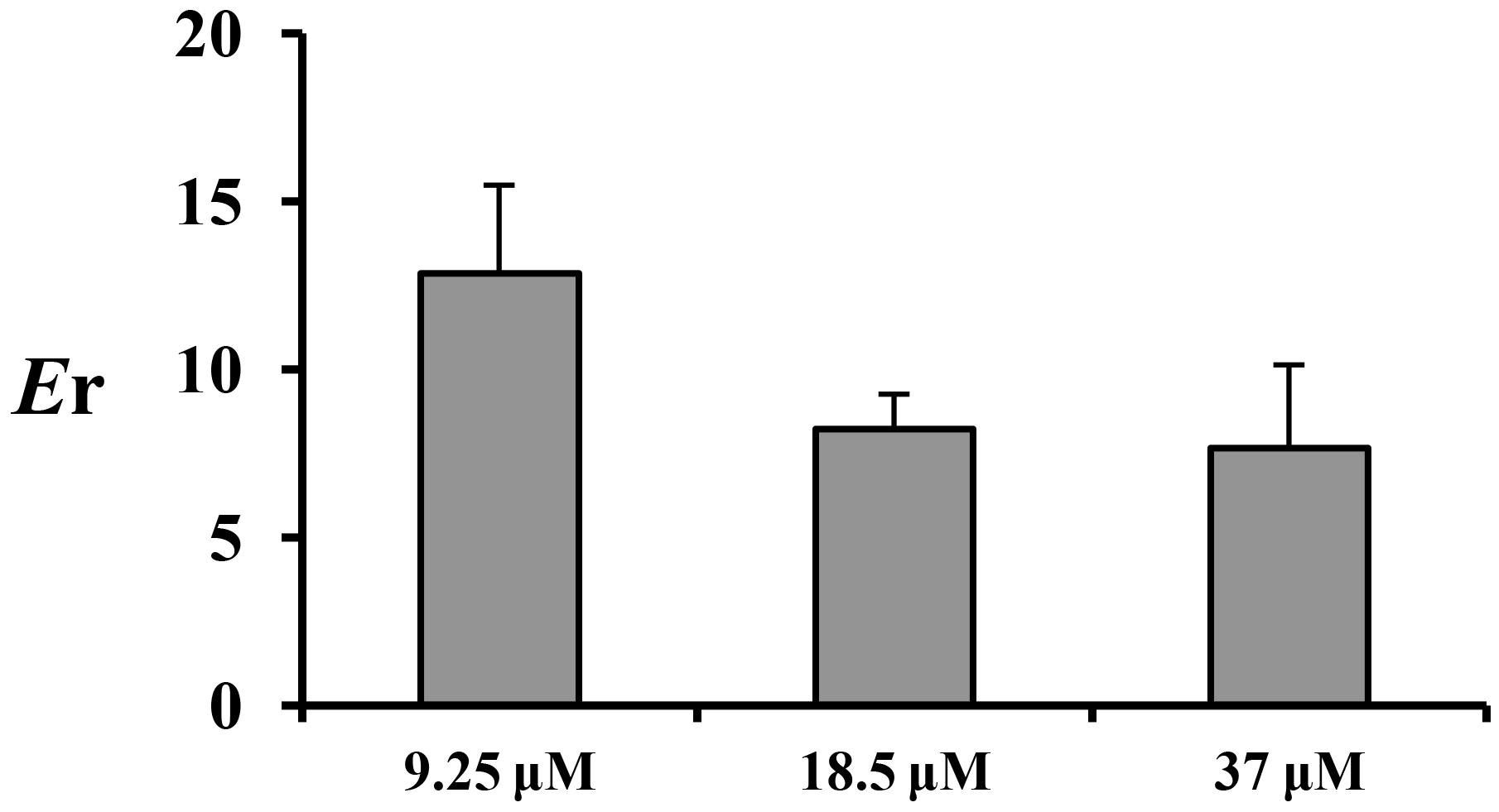
FIGURE 3. The Er of emodin in jejunum at three concentrations (9.25, 18.5, and 37 μM). Each bar represents the mean with SD of five measurements.
Interaction Studies Between Emodin and Efflux Transporters
Transport studies were performed in the presence of P-gp, MRP2, and MRP3 inhibitors (verapamil, MK571, and benzbromarone) to determine the effect of these effluxes on the transport of emodin. The data are summarized in Figure 4. Verapamil (220 μM) could markedly increase emodin absorption and decrease the efflux ratio at 9.25 μM. In the presence of the MRP2 efflux transporter inhibitor MK571 (186 μM), the Papp values of emodin from mucosal to the serosal side were significantly increased and the efflux ratio decreased. However, no significant differences were observed in the presence of benzbromarone. These results indicated that emodin might be the substrate of P-gp and MRP2.
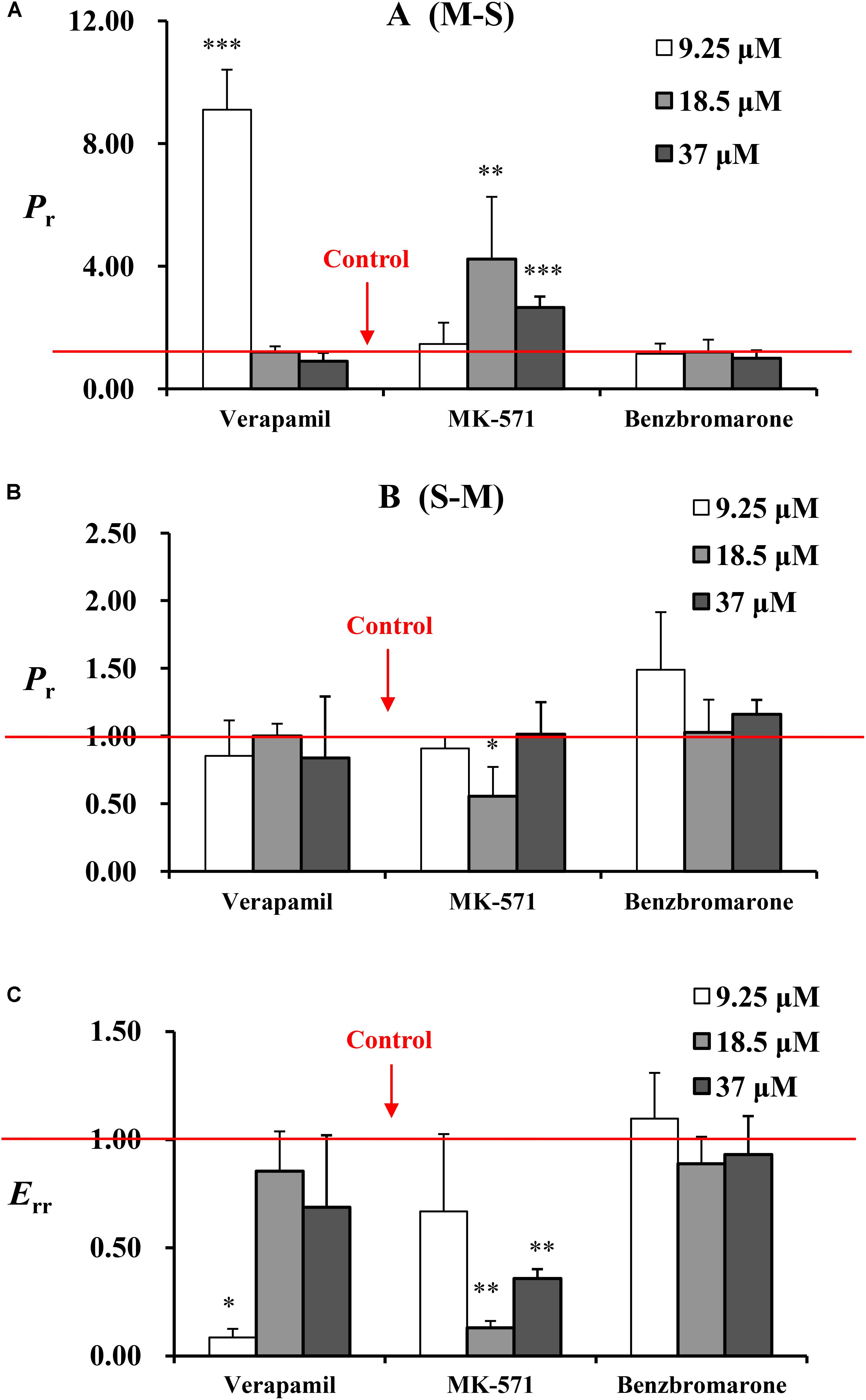
FIGURE 4. The Pr from (A) M-S and (B) S-M and (C) Err of emodin at three concentrations (9.25, 18.5, and 37 μM) in jejunum. Experiments were performed in the presence of verapamil (220 μM), MK571 (186 μM), and benzbromarone (470 μM). The three inhibitors were loaded onto the mucosal side, respectively. For the experiments were performed in batches, control groups were set as one for each batch. The result represents the ratio of Papp or Er between without or with inhibitor. Each bar represents the mean with SD of five measurements (∗p < 0.05, ∗∗p < 0.01, and ∗∗∗p < 0.001, compared to the control according to independent samples t-test).
To investigate the role of emodin on the efflux transporters P-gp, MRP2, and MRP3 functions on rat enterocyte, digoxin, pravastatin, and teniposide were carried out for transport studies in the presence of emodin. With the treatment of emodin, the Papp values of digoxin from M-S significantly decreased while those from S-M increased. Thus, the Er values of digoxin were significant higher after adding emodin. The date are summarized in Table 3 and Figure 5. These results indicate that emodin could enhance P-gp function on rat jejunum. In the presence of emodin, the Papp from S-M and Er of the MRP3 substrate teniposide remarkably decreased, indicating that emodin might be an MRP3 inhibitor. No significant differences were observed in the Papp and Er values of pravastatin after emodin added.
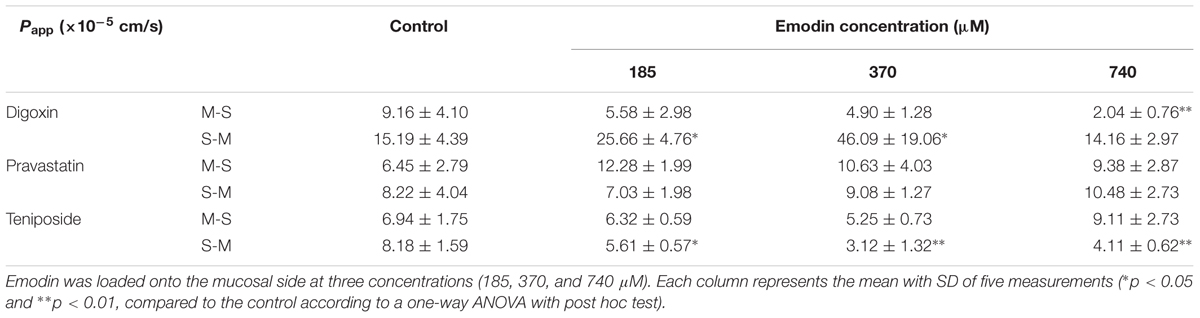
TABLE 3. The Papp from M-S and S-M of digoxin (25 μM), pravastatin (47 μM), and teniposide (60 μM) in the presence of emodin.
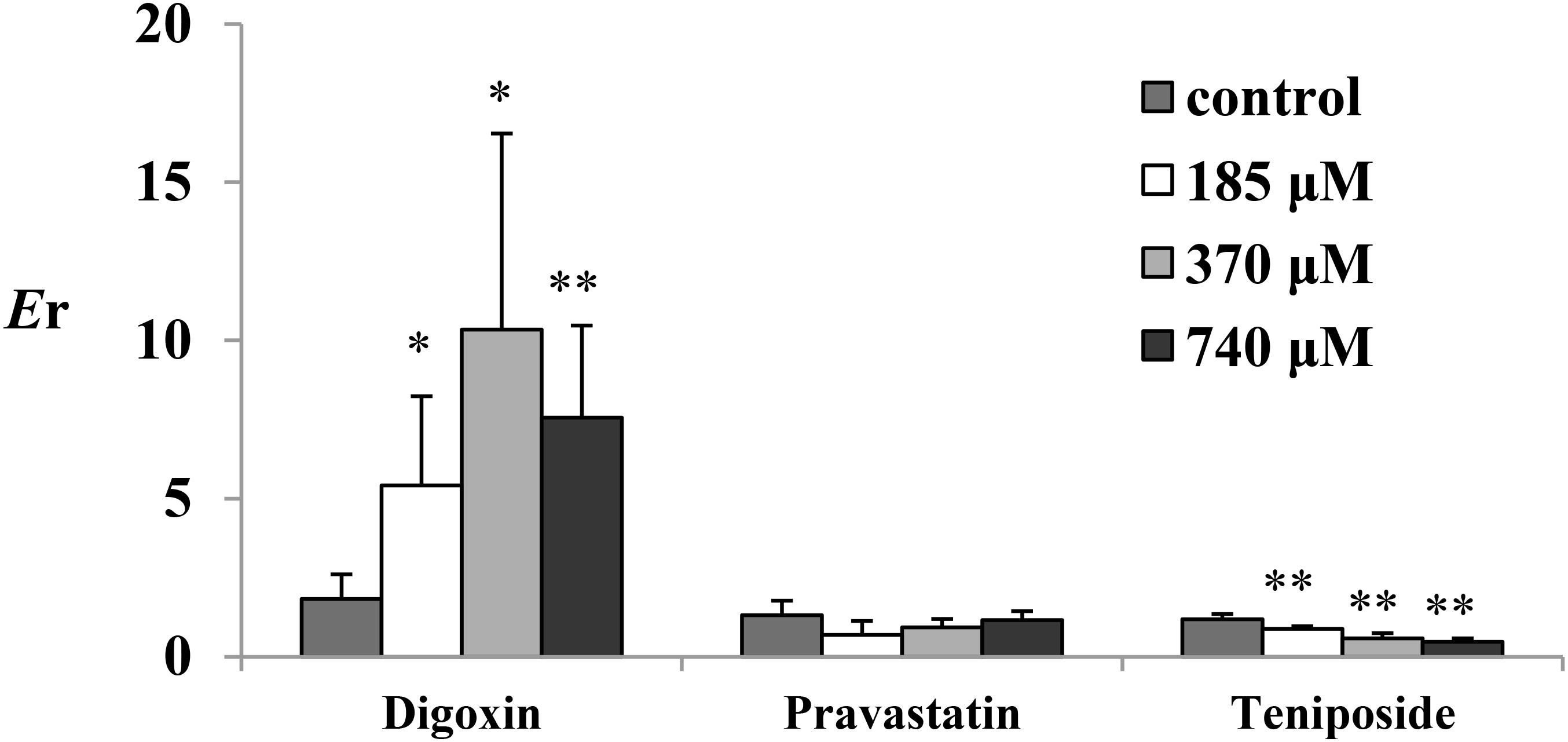
FIGURE 5. The Er of digoxin (25 μM), pravastatin (47 μM), and teniposide (60 μM) in the presence of emodin. Emodin was loaded onto the mucosal side at three concentrations (185, 370, and 740 μM). Each bar represents the mean with SD of five measurements (∗p < 0.05 and ∗∗p < 0.01, compared to the control according to independent samples t-test).
Discussion
Emodin, a potential antineoplastic drug, has been proved to have drug–drug interactions with many other drugs whereas the mechanisms have not yet to be discovered. Our results showed that emodin was the substrate of P-gp and MRP2, which is consistent with the literature reported (Teng et al., 2012; Zhang et al., 2012). Furthermore, we found that emodin might be a P-gp inducer and MRP3 inhibitor on enterocyte, which has not been reported in the literature before. However, verapamil only promoted emodin intestinal absorption at a low concentration; this is probably due to the fact that emodin itself is a P-gp inducer. MRP3, an efflux transporter, mainly distributes in liver, intestine, and adrenal glands, while most researches focused on its function and expression on liver. It is involved in the enterohepatic circulation of non-sulfated and sulfated bile salts such as glycocholates and taurocholates (Keppler, 2014, 2017). Our results showed that emodin might not be a substrate of MRP3 whereas it could inhibit MRP3 function on enterocyte, which indicated that the role of MRP3 should not be ignored in intestine. We consider that emodin might regulate MRP3 function by influencing the upstream proteins or kinases. Furthermore, we believe that oral administration of emodin would affect bile transport to some extent.
In this study, we used the Ussing chamber technique to evaluate the interactions between emodin and efflux transporters, which is regarded as gold standards for drug transport studies. The use of a living and intact intestinal tissue is more realistic than cell cultures and provides many advantages. The intestinal tissues are likely to express all the transporters and the enzymes at the same “physiological” level of expression. Moreover, the data obtained through rat intestine can be directly correlated to in vivo experiments that will be conducted in the same animal model (Mazzaferro et al., 2012; Sjögren et al., 2016). However, samples withdrawn from the receiver compartment often at very low concentration. With the development of LC-MS/MS technology, the amount of test compounds can easier to be detected. Besides, the procedure for tissue preparation is a technically challenging technique. KRB solution should be filtered with 0.22 μm filter and the removed intestine tissue should be placed in the cold KBR solution with gas in incessancy. Preparation must be done on ice carefully for it takes time and is associated with risks of tissue damage.
Although emodin has multiple pharmacological activities, its toxicity has attracted more and more attention in recent years. However, emodin treatment is a double-edged sword. It showed protective effect on alcoholic liver injury while hepatotoxicity appeared with high doses and long-term drug delivery (Dong et al., 2009; Wang et al., 2011; Liu et al., 2014). We suspect that the changeable role of emodin may be related to the variation of intestinal environment, including intestinal transporters, structures, microorganisms, and so on. Therefore, it requires further study to find out the changes of environment in vivo and emodin disposition characteristics during long-term administration process.
Conclusion
In the present study, we have shown that the Ussing chamber technique was suitable for P-gp, MRP2, and MRP3 related studies on rat enterocyte. On the basis of this technique, we discovered that emodin might be the substrate of P-gp and MRP2, but not MRP3. Besides, emodin could decrease digoxin and increase teniposide absorption on rat intestine, indicating that emodin might be a P-gp inducer and MRP3 inhibitor.
Author Contributions
JH and XQ designed the project. JH, LaG, RT, and MW performed the experiments. JH, LaG, RT, and XQ analyzed the data. JH, JZ, YZ, LuG, ZH, and XQ wrote the manuscript.
Funding
This research was supported by the National Natural Science Foundation of China (No. 81373967) and Science and Technology Planning Project of Guangdong Province (No. 2017B030314166).
Conflict of Interest Statement
The authors declare that the research was conducted in the absence of any commercial or financial relationships that could be construed as a potential conflict of interest.
References
Abbasi, M. M., Valizadeh, H., Hamishehkar, H., and Zakeri-Milani, P. (2016). Inhibition of P-glycoprotein expression and function by anti-diabetic drugs gliclazide, metformin, and pioglitazone in vitro and in situ. Res. Pharm. Sci. 11, 177–186.
Ballent, M., Maté, L., Virkel, G., Sallovitz, J., Viviani, P., Lanusse, C., et al. (2014). Intestinal drug transport: ex vivo evaluation of the interactions between ABC transporters and anthelmintic molecules. J. Vet. Pharmacol. Ther. 37, 332–337. doi: 10.1111/jvp.12112
Cha, T. L., Chuang, M. J., Tang, S. H., Wu, S. T., Sun, K. H., Chen, T. T., et al. (2015). Emodin modulates epigenetic modifications and suppresses bladder carcinoma cell growth. Mol. Carcinog. 54, 167–177. doi: 10.1002/mc.22084
Deusser, H., Roqoll, D., Scheppach, W., Volk, A., Melcher, R., and Richling, E. (2013). Gastrointestinal absorption and metabolism of apple polyphenols ex vivo by the pig intestinal mucosa in the Ussing chamber. Biotechnol. J. 8, 363–370. doi: 10.1002/biot.201200303
Di, X., Wang, X., Di, X., and Liu, Y. (2015). Effect of piperine on the bioavailability and pharmacokinetics of emodin in rats. J. Pharm. Biomed. Anal. 115, 144–149. doi: 10.1016/j.jpba.2015.06.027
Dong, M. X., Jia, Y., Zhang, Y. B., Li, C. C., Geng, Y. T., Zhou, L., et al. (2009). Emodin protects rat liver from CCl4- induced fibrogenesis via inhibition of hepatic stellate cells activation. World J. Gastroenterol. 15, 4753–4762.
Dong, X., Fu, J., Yin, X., Cao, S., Li, X., Lin, L., et al. (2016). Emodin: a review of its pharmacology, toxicity and pharmacokinetics. Phytother. Res. 30, 1207–1218. doi: 10.1002/ptr.5631
Ellis, L. C., Hawksworth, G. M., and Weaver, R. J. (2013). ATP-dependent transport of statins by human and rat MRP2/Mrp2. Toxicol. Appl. Pharmacol. 269, 187–194. doi: 10.1016/j.taap.2013.03.019
Erik, S., Johanna, E., Charlotta, V., Breitholtz, K., and Hilgendorf, C. (2016). Excised segments of rat small intestine in Ussing chamber studies: a comparison of native and stripped tissue viability and permeability to drugs. Int. J. Pharm. 505, 361–368. doi: 10.1016/j.ijpharm.2016.03.063
Guo, J., Li, W., Shi, H., Xie, X., Li, L., Tang, H., et al. (2013). Synergistic effects of curcumin with emodin against the proliferation and invasion of breast cancer cells through upregulation of miR-34a. Mol. Cell. Biochem. 382, 103–111. doi: 10.1007/s11010-013-1723-6
Heinen, C., Reuss, S., Saaler-Reinhardt, S., and Langguth, P. (2013). Mechanistic basis for unexpected bioavailability enhancement of polyelectrolyte complexes incorporating BCS class III drugs and carrageenans. Eur. J. Pharm. Biopharm. 85, 26–33. doi: 10.1016/j.ejpb.2013.03.010
Heo, S. K., Yun, H. J., Noh, E. K., and Park, S. D. (2010). Emodin and rhein inhibit LIGHT-induced monocytes migration by blocking of ROS production. Vascul. Pharmacol. 53, 28–37. doi: 10.1016/j.vph.2010.03.002
Huang, J., Zhang, J., Bai, J. Q., Bai, J., Xu, W., Wu, D., et al. (2016). LC-MS/MS Determination and interaction of the main components from the traditional Chinese drug pair Danshen-Sanqi based on rat intestinal absorption. Biomed. Chromatogr. 30, 1928–1934. doi: 10.1002/bmc.3768
Keppler, D. (2014). The roles of MRP2, MRP3, OATP1B1, and OATP1B3 in conjugated hyperbilirubinemia. Drug Metab. Dispos. 42, 561–565. doi: 10.1124/dmd.113.055772
Keppler, D. (2017). Progress in the molecular characterization of hepatobiliary transporters. Dig. Dis. 35, 197–202. doi: 10.1159/000450911
Ko, J. C., Su, Y. J., Lin, S. T., Jhan, J. Y., Ciou, S. C., Cheng, C. M., et al. (2010). Emodin enhances cisplatin-induced cytotoxicity via down-regulation of ERCC1 and inactivation of ERK1/2. Lung Cancer 69, 155–164. doi: 10.1016/j.lungcan.2009.10.013
Kool, M., van der Linden, M., de Haas, M., Scheffer, G. L., de Vree, J. M., Smith, A. J., et al. (1999). MRP3, an organic anion transporter able to transport anti-cancer drugs. Proc. Natl. Acad. Sci. U.S.A. 96, 6914–6919.
Li, H., Jin, H. E., Shim, W. S., and Shim, C. K. (2012). An improved prediction of the human in vivo intestinal permeability and BCS class of drugs using the in vitro permeability ratio obtained for rat intestine using an Ussing chamber system. Drug Dev. Ind. Pharm. 39, 1515–1522. doi: 10.3109/03639045.2012.714787
Li, W. Y., Ng, Y. F., Zhang, H., Guo, Z. D., Guo, D. J., Kwan, Y. W., et al. (2014). Emodin elicits cytotoxicity in human lung adenocarcinoma A549 cells through inducing apoptosis. Inflammopharmacology 22, 127–134. doi: 10.1007/s10787-013-0186-4
Liu, W., Feng, Q., Li, Y., Ye, L., Hu, M., and Liu, Z. (2012). Coupling of UDP-glucuronosyltransferases and multidrug resistance associated proteins is responsible for the intestinal disposition and poor bioavailability of emodin. Toxicol. Appl. Pharmacol. 265, 316–324. doi: 10.1016/j.taap.2012.08.032
Liu, Y., Chen, X., Qiu, M., Chen, W., Zeng, Z., and Chen, Y. (2014). Emodin ameliorates ethanol-induced fatty liver injury in mice. Pharmacology 94, 71–77. doi: 10.1159/000363413
Liu, Y., Liu, B., Zhang, Y., Peng, Y., Huang, C., Wang, N., et al. (2017). Intestinal absorption mechanisms of 2’-deoxy-2’-β-fluoro-4’-azidocytidine, a cytidine analog for AIDS treatment, and its interaction with P-glycoprotein, multidrug resistance-associated protein 2 and breast cancer resistance protein. Eur. J. Pharm. Sci. 105, 150–158. doi: 10.1016/j.ejps.2017.05.009
Mazzaferro, S., Bouchemal, K., Skanji, R., Gueutin, C., Chacun, H., and Ponchel, G. (2012). Intestinal permeation enhancement of docetaxel encapsulated into methyl-β-cyclodextrin/poly (isobutylcyanoacrylate) nanoparticles coated with thiolated chitosan. J. Control Release 162, 568–574. doi: 10.1016/j.jconrel.2012.08.005
Moazed, B., and Hiebert, L. M. (2007). An in vitro study with an Ussing chamber showing that unfractionated heparin crosses rat gastric mucosa. J. Pharmacol. Exp. Ther. 322, 299–305. doi: 10.1124/jpet.106.116939
Monisha, B. A., Kumar, N., and Tiku, A. B. (2016). Emodin and its role in chronic diseases. Adv. Exp. Med. Biol. 928, 47–73. doi: 10.1007/978-3-319-41334-1_3
Riahi, S., Reza Ganjali, M., Dinarvand, R., Karamdoust, S., Bagherzadeh, K., and Norouzi, P. (2008). A theoretical study on interactions between mitoxantrone as an anticancer drug and DNA: application in drug design. Chem. Biol. Drug Des. 71, 474–482. doi: 10.1111/j.1747-0285.2008.00653.x
Sjögren, E., Eriksson, J., Vedin, C., Breitholtz, K., and Hilgendorf, C. (2016). Excised segments of rat small intestine in Ussing chamber studies: a comparison of native and stripped tissue viability and permeability to drugs. Int. J. Pharm. 505, 361–368. doi: 10.1016/j.ijpharm.2016.03.063
Tan, W., Lu, J., Huang, M., Li, Y., Chen, M., Wu, G., et al. (2011). Anti-cancer natural products isolated from Chinese medicinal herbs. Chin. Med. 6:27. doi: 10.1186/1749-8546-6-27
Teng, Z., Yuan, C., Zhang, F., Huan, M., Cao, W., Li, K., et al. (2012). Intestinal absorption and first-pass metabolism of polyphenol compounds in rat and their transport dynamics in Caco-2 cells. PLoS One 7:e29647. doi: 10.1371/journal.pone.0029647
Teng, Z. H., Zhou, S. Y., Ran, Y. H., Liu, X. Y., Yang, R. T., Yang, X., et al. (2007). Cellular absorption of anthraquinones emodin and chrysophanol in human intestinal Caco-2 cells. Biosci. Biotechnol. Biochem. 71, 1636–1643.
Wang, J. B., Zhao, H. P., Zhao, Y. L., Jin, C., Liu, D. J., Kong, W. J., et al. (2011). Hepatotoxicity or hepatoprotection? Pattern recognition for the paradoxical effect of the Chinese herb Rheum palmatum L. in treating rat liver injury. PLoS One 6:e24498. doi: 10.1371/journal.pone.0024498
Wang, W., Sun, Y. P., Huang, X. Z., He, M., Chen, Y. Y., Shi, G. Y., et al. (2010). Emodin enhances sensitivity of gallbladder cancer cells to platinum drugs via glutathion depletion and MRP1 downregulation. Biochem. Pharmacol. 79, 1134–1140. doi: 10.1016/j.bcp.2009.12.006
Wei, W. T., Lin, S. Z., Liu, D. L., and Wang, Z. H. (2013). The distinct mechanisms of the antitumor activity of emodin in different types of cancer. Oncol. Rep. 30, 2555–2562. doi: 10.3892/or.2013.2741
Xiao, Q., Yang, W., Wang, D., Chen, L., Yuan, L., Ding, Y., et al. (2016). Factors limiting the extent of absolute bioavailability of pradefovir in rat. Xenobiotica 46, 913–921. doi: 10.3109/00498254.2015.1133866
Yu, Q., Jiang, L. L., Luo, N., Fan, Y. X., Ma, J., Li, P., et al. (2017). Enhanced absorption and inhibited metabolism of emodin by 2, 3, 5, 4’-tetrahydroxystilbene -2-O-β-D-glucopyranoside: possible mechanisms for Polygoni Multiflori Radix-induced liver injury. Chin. J. Nat. Med. 15, 451–457. doi: 10.1016/S1875-5364(17)30067-5
Zhang, Y., Wang, P., Wang, J. R., Yu, Y. P., and Meng, X. L. (2012). Study of intestinal absorption of emodin in one-way intestinal perfusion rat model. Tradit. Chin. Drug Res. Clin. Pharmacol. 23, 286–290.
Keywords: emodin, P-gp, MRP2, MRP3, Ussing chamber technique
Citation: Huang J, Guo L, Tan R, Wei M, Zhang J, Zhao Y, Gong L, Huang Z and Qiu X (2018) Interactions Between Emodin and Efflux Transporters on Rat Enterocyte by a Validated Ussing Chamber Technique. Front. Pharmacol. 9:646. doi: 10.3389/fphar.2018.00646
Received: 16 March 2018; Accepted: 30 May 2018;
Published: 22 June 2018.
Edited by:
Jiang Xu, Institute of Chinese Materia Medica, China Academy of Chinese Medical Sciences, ChinaReviewed by:
Qi Wang, Harbin Medical University, ChinaLuigi Menghini, Università “G. d’Annunzio” di Chieti-Pescara, Italy
Copyright © 2018 Huang, Guo, Tan, Wei, Zhang, Zhao, Gong, Huang and Qiu. This is an open-access article distributed under the terms of the Creative Commons Attribution License (CC BY). The use, distribution or reproduction in other forums is permitted, provided the original author(s) and the copyright owner are credited and that the original publication in this journal is cited, in accordance with accepted academic practice. No use, distribution or reproduction is permitted which does not comply with these terms.
*Correspondence: Xiaohui Qiu, cWl1eGlhb2h1aUBnenVjbS5lZHUuY24=