- 1Department of Physiology, Faculty of Medical Science, Naresuan University, Phitsanulok, Thailand
- 2PEPITE EA4267, Université Bourgogne Franche-Comté, Besançon, France
- 3Department of Pharmaceutical Chemistry and Pharmacognosy, Faculty of Pharmaceutical Sciences, Naresuan University, Phitsanulok, Thailand
Background: Ethnopharmacological studies demonstrated the potential for Eulophia species to treat inflammation, cancer, and cardio-metabolic diseases. The aim of the study was to investigate the vasorelaxant effect of ethanolic Eulophia macrobulbon (EM) extract and its main phenanthrene on rat isolated mesenteric artery and to investigate the hypotensive effect of EM.
Methods: The vasorelaxant effects of EM extract or phenanthrene and the underlying mechanisms were evaluated on second-order mesenteric arteries from Sprague Dawley rats. In addition, the acute hypotensive effect was evaluated in anesthetized rats infused with cumulative concentrations of the EM extract.
Results: Both EM extract (10-4–1 mg/ml) and phenanthrene (10-7–10-4 M) relaxed endothelium-intact arteries, an effect that was partly reduced by endothelium removal (p < 0.001). A significant decrease in the relaxant effect of the extract and the phenanthrene was observed with L-NAME and apamin/charybdotoxin in endothelium-intact vessels, and with iberiotoxin in denuded vessels. SNP (sodium nitroprusside)-induced relaxation was significantly enhanced by EM extract and phenanthrene. By contrast, ODQ (1H-[1,2,4]oxadiazolo[4,3-a]quinoxaline-1-one), 4-aminopyridine and glibenclamide (endothelium-denuded vessels) and indomethacin (endothelium-intact vessels) had no effect. In calcium-free solution, both the EM extract and phenanthrene inhibited extracellular Ca2+-induced contraction in high KCl and phenylephrine (PE) pre-contracted rings. They also inhibited the intracellular Ca2+ release sensitive to PE. The acute infusion of EM extract (20 and 70 mg/kg) induced an immediate and transient dose-dependent hypotensive effect.
Conclusion: The ethanolic extract of EM tubers and its main active compound, 1-(4′-hydroxybenzyl)-4,8-dimethoxyphenanthrene-2,7-diol (phenanthrene) induced vasorelaxant effects on rat resistance vessels, through pleiotropic effects including endothelium-dependent effects (NOS activation, enhanced EDH production) and endothelium-independent effects (opening of KCa channels, inhibition of Ca2+ channels, inhibition of intracellular Ca2+ release and PDE inhibition).
Introduction
Orchids belong to the plant family Orchidaceae, one of the most diverse group among the angiosperm with near 25,000 species (Behera et al., 2013). Aside from their ornamental value, orchids are also acknowledged for their use in traditional medicines (Bulpitt, 2005; Behera et al., 2013; Pant, 2013; Patil and Mahajan, 2013; Narkhede et al., 2016). Chinese medicine was probably the first to describe orchids for their medicinal use. Other pharmacopeias from India and many countries from South-Asia such as Taiwan, Singapore, Vietnam, Sri Lanka, Thailand, Myanmar, use orchids in traditional medicine since the ancient time (Suresh Kumar et al., 2000; Hernández-Romero et al., 2005; Luo et al., 2007). Likewise, the use of orchids in America also has a long history. Phytochemically, orchids have been reported to contain alkaloids, triterpenoids, flavonoids and stilbenoids (Kong et al., 2003; Kovács et al., 2008; Singh et al., 2012). Surprisingly, limited information on medicinal values of orchids regarding their therapeutic properties in different parts of the world is available, whereas such information would be helpful for sustainable management of resources.
Genus Eulophia is highly diverse in the family Orchidaceae. It has a wide distribution and comprises over 230 species, which are widespread from tropical and Southern Africa, Madagascar and from tropical and subtropical parts of Asia and Australia. Among these, one species occurs in tropical America. Different Eulophia species have been extensively used in the traditional system of medicines in many countries (Chinsamy et al., 2011; Patil and Mahajan, 2013; Narkhede et al., 2016). For instance, in India, the word Amarkand is commonly used for 30 plant species from genus Eulophia and for one species from the genus Dioscorea. Since ancient times, Amarkand is believed to be an excellent health-promoting agent. Rhizhomes/tubers of Amarkand are routinely consumed by the tribal parts of India as food as well as a therapeutic entity for better health and longevity (Narkhede et al., 2016). Phytochemical studies reported that Eulophia species contains phenolics, saponins, alkaloids, flavonoids, terpenoids and phenanthrene derivatives (Tuchinda et al., 1989; Maridass et al., 2008; Patil and Mahajan, 2013; Narkhede et al., 2016; Dawande and Gurav, 2017). Ethnopharmacological studies demonstrated that Eulophia species exhibited anti-inflammatory (Datla et al., 2010; Chinsamy et al., 2014; Schuster et al., 2017), anti-cancer (Shriram et al., 2010; Schuster et al., 2017), anti-oxidant (Tatiya et al., 2013; Schuster et al., 2017), anti-diabetic (Tatiya et al., 2013), and hypolipidemic (Tatiya et al., 2013) properties. Eulophia macrobulbon (E.C.Parish & Rchb.f.) Hook.f is naturally found in Thailand, Laos, Vietnam, Myanmar and Cambodia, and is traditionally used in local Thai medicine for treatment of insect bites and gangrene (Schuster et al., 2017), this latter indication suggesting a putative effect on small arteries. Recently, the ethanolic extract of Eulophia macrobulbon was shown to act as a potent phosphodiesterase 5 (PDE 5) inhibitor (Temkitthawon et al., 2017), an effect supported by its main compound, 1-(4′-hydroxybenzyl)-4,8-dimethoxyphenanthrene-2,7-diol [IC50 = 1.7 ± 0.5 μM (Temkitthawon et al., 2017)].
As PDE enzymes are widely expressed in smooth muscle cells including vascular smooth muscle cells (VSMC) (Komas et al., 1991), the aim of the present study was to investigate the vasorelaxant effect of an ethanolic extract of EM and its main constituent, 1-(4′-hydroxybenzyl)-4,8-dimethoxyphenanthrene-2,7-diol, and to unravel the mechanisms involved on isolated rat mesenteric artery. To determine whether the in vitro vasorelaxant properties translate into an in vivo effect, the acute hypotensive effect of EM extract was determined in anesthetized rats.
Materials and Methods
Preparation of the Plant Extract
Fresh tubers of EM were collected from Prachinburi province, Thailand. The voucher specimen (No. 002716) was identified by Associate Professor Dr. Anupan Kongbungkerd, Department of Biology, Faculty of Science, Naresuan University, and kept at Faculty of Science, Naresuan University, Phitsanulok, Thailand. As previously described (Temkitthawon et al., 2017), the tubers of EM were cut and dried at 55°C. The dried material (2 kg) was ground into a fine powder, macerated with 95% ethanol (14 L) for 3 days/time (two times). Then, it was filtered and evaporated under vacuum until dryness to give the crude ethanolic extract with the yield of 15.78% (w/w). This extract contained 0.52% (w/w) of a phenanthrene, 1-(4′-hydroxybenzyl)-4,8-dimethoxyphenanthrene-2,7-diol, which was used as a bioactive marker and a major compound of the extract (Temkitthawon et al., 2017). This compound was isolated from EM extract as described by Temkitthawon et al. (2017). Briefly, this compound was isolated and purified by using column chromatography, preparative thin layer chromatography, size exclusion chromatography, high performance liquid chromatography (HPLC) and recrystallization techniques. The EM extract and the phenanthrene were stored at -20°C until used.
Animals
Male Sprague Dawley rats (8–12 weeks-old) were purchased from Janvier (Le Genest Saint Isle, France) for the in vitro study of vascular reactivity and from National Laboratory Animal Center (Mahidol University, Salaya, Thailand) for the in vivo study. Animals were kept under 12–12 h light: dark cycle, at 22 ± 1°C and allowed free access to standard food and water. The investigation complied with the ARRIVE animal research: reporting in vivo experiments. All protocols were approved by the local committees for ethics in animal experimentation No. 2015/001-CD/5PR of University of Franche-Comté (Besançon, France) and Naresuan University Animal Care and Use Committee (NUACUC, Naresuan University, Phitsanulok, Thailand, ethic approval number: NU-AE591025).
Mesenteric Arteries Preparation
Rats were anesthetized with sodium pentobarbital (Ceva Santé Animale, France) (60 mg/kg, i.p.). Second order branches of mesenteric arteries were placed in Krebs solution with the following composition (mM): NaCl 118, KCl 4.7, KH2PO4 1.2, MgSO4 1.2, CaCl2 2.5, NaHCO3 25, glucose 12, maintained at pH of 7.4, 37°C, and continuously aerated with 95% O2, 5% CO2. Then 2-mm segments of artery were mounted in organ chambers and threaded on two 40-μm diameter stainless steel wires. To measure isometric force, the artery segments were connected into a Multi Myograph System (Model 610 M v.2.2, DMT A/S, Denmark). The data were recorded using ChartTM Ver.7 (ADInstruments, France). After a 15-min equilibration period, segments were stretched to their optimal lumen diameter for active tension development. Optimal lumen diameter was determined based on the internal circumference/wall tension ratio of the segments by setting the internal circumference to 90% of what the vessels would have if they were exposed to a passive tension equivalent to that produced by a transmural pressure of 100 mmHg. Optimal lumen diameter was determined using specific software for normalization of resistance arteries (DMT Normalization Module; ADInstruments). After an initial equilibration period of 30 min, viability of vessels was tested from their vasoconstriction to high extracellular KCl (100 mM). The presence of functional endothelium was confirmed by more than 60% relaxation to the endothelium-dependent agonist acetylcholine (ACh 10-5 M) after pre-constriction with phenylephrine (PE 10-5 M). In some rings, endothelium was mechanically removed by gently rubbing inside the vessel with a small wire. The completeness of endothelial denudation was confirmed by the absence of relaxation to ACh (10-5 M). Arteries were again allowed to equilibrate 30 min before the start of the experiments.
Experimental Protocols for Vascular Reactivity
Vasorelaxant Effect of EM Extract and Its Main Compound
To investigate the relaxant effect of the EM extract and 1-(4′-hydroxybenzyl)-4,8-dimethoxyphenanthrene-2,7-diol (phenanthrene), mesenteric rings were pre-contracted with submaximal concentration of PE (10-5 M). When the contraction reached a plateau, the EM extract (10-4–1 mg/ml) or phenanthrene (10-7–10-4 M) were added cumulatively to endothelium-intact rings as well as to endothelium-denuded rings to obtain a concentration-response curve. The relaxation effect was calculated as the percentage of the contraction in response to PE. The effect of the solvent, dimethyl sulfoxide (DMSO 0.23% for the EM extract, 0.18% for the phenanthrene), was evaluated in the same conditions.
Role of Endothelium-Dependent Pathways
To evaluate the role of endothelial nitric oxide synthase (eNOS), cyclo-oxygenase (COX) and endothelium-derived hyperpolarizing factor (EDH) pathways in the vasorelaxant activity of the EM extract or its main compound, endothelium-intact mesenteric rings were incubated with the NOS inhibitor, NG-nitro-L-arginine methyl ester (L-NAME, 10-4 M), the COX inhibitor, indomethacin (10-5 M) or with a combination of the small calcium-activated potassium channel blocker, apamin (10-7 M) and the intermediate and large conductance calcium-activated potassium channel blocker, charybdotoxin (10-7M) for 30 min before pre-contraction with PE (10-5 M), respectively. Then, cumulative concentrations of the EM extract or phenanthrene were added.
Role of K+ Channels From Vascular Smooth Muscle
To investigate the contribution of K+ Channels to the relaxant effect of the EM extract and phenanthrene, endothelium-denuded mesenteric rings were pre-incubated with the voltage-gated potassium channel (KV) blocker, 4-aminopyridine (4-AP, 10-3 M), the ATP-sensitive potassium channel (KATP) blocker, glibenclamide (10-5 M), or the large conductance Ca2+-activated K+ channels (KCa) blocker, iberiotoxin (10-7 M), for 30 min before pre-contraction with PE (10-5 M). Then, cumulative concentrations of the EM extract were added.
Role of sGC/cGMP Pathway
To assess whether the relaxant effect of extract and phenanthrene might be dependent on PDE inhibition, we determined if they induced changes in the soluble guanylyl cyclase (sGC)/cyclic guanosine monophosphate (cGMP) pathway in vascular smooth muscle cell (VSMC). Endothelium-denuded (E-) mesenteric rings were incubated with the vehicle (0.06% for the EM extract, 0.1% for the phenanthrene), EM extract (125 μg/ml) or phenanthrene (35 μM) for 10 min. Then rings were contracted with 10-5 M PE (for the EM extract) or 100 mM KCl [for the phenanthrene, as rings pre-incubated with phenanthrene did not contract to 10-5 M PE or low KCl (30 mM)], and then exposed to cumulative concentrations (10-11–10-4 M) of sodium nitroprusside (SNP), a NO donor. To determine whether the EM extract or phenanthrene directly activates sGC or rather acts downstream of sGC, i.e., on PDE, endothelium-denuded rings were incubated with the selective sGC inhibitor, 1H-[1,2,4]oxadiazolo[4,3-a]quinoxaline-1-one (ODQ, 10-5 M) for 30 min, before contraction with 10-5 M PE. Then, cumulative concentrations of the EM extract or phenanthrene were added.
Role of Calcium Channels
To assess the role of the extracellular calcium (Ca2+) influx in the extract- or phenanthrene-induced relaxations, endothelium-denuded mesenteric rings were incubated with a Ca2+-free Krebs solution containing 1 mM, methylene glycol-bis (2-aminoethylether)-N,N,N’,N’-tetraacetic acid (EGTA) for 40 min. Rings were firstly contracted with 10-5 M PE to deplete intracellular Ca2+ store from sarcoplasmic reticulum (SR) and washed four times every 10 min with the Ca2+-free Krebs solution. Then, rings were incubated with the vehicle or the EM extract at the EC50 (125 μg/ml) or phenanthrene at the EC50 (35 μM) for 10 min before 10-5 M PE or 8x10-2 M KCl was applied. Then, 10-2 M CaCl2 was added to evoke a contractile response (Senejoux et al., 2013).
To determine the effect of EM extract and phenanthrene on intracellular Ca2+ release, the endothelium-denuded mesenteric rings were incubated with the L-type voltage dependent Ca2+ channel inhibitor verapamil (10-7 M) for 30 min. Then, 10-5 M PE was added to the bath, and vehicle, EM extract (125 μg/ml) or phenanthrene (35 μM) were added. Under these conditions, the observed PE-induced contractions are caused by intracellular Ca2+ release via the opening of inositol 1,4,5 trisphosphate (IP3) receptors from SR (Tom et al., 2010).
Acute Effect of EM Extract on Blood Pressure and Heart Rate
To investigate whether the direct in vitro vascular effect of EM extract on resistance vessels translates into an in vivo effect, we studied the acute hypotensive effect of EM extract in anesthetized rats. The effect of phenanthrene was not studied because of the low quantity and poor dissolution of the isolated compound. Normotensive male Sprague Dawley rats were anesthetized with pentobarbital (Ceva Santé Animale, France) (60 mg/kg, i.p.) and supplemented as needed to maintain deep anesthesia. The femoral vein and artery were cannulated with polyethylene tube (PE50, 0.58 mm i.d. × 0.96 mm o.d.) filled with heparinized (50 units/ml) saline. The arterial catheter was connected to a pre-calibrated pressure transducer (model BP-100 Blood Pressure Transducer, iWorx Systems, Inc., Dover, NH, United States), the output of pressure was recorded by a bridge amplifier coupled to Powerlab® recording system, and an application program (ChartTM Ver.6 ADInstruments, castle Hill, NSW, Australia). After a 15-min stabilization period, systolic arterial blood pressure (SBP), diastolic blood pressure (DBP), and heart rate (HR) were recorded before and during intravenous infusion (at 1 ml/min) of 1 ml/kg of saline, vehicle (a saline solution containing 5% DMSO), EM extract (1, 5, 20, 70 mg/kg), or the NO donor SNP (25 μg/kg). The dose of SNP was chosen as a dose inducing a maximum lowering effect on blood pressure (Pagani et al., 1978; Kamkaew et al., 2013). The EM extract was dissolved in DMSO, diluted in saline and filtered through 0.2 μM syringe filter. Each subsequent infusion was administered when baseline values were fully recovered and waited for 15 min before the next infusion.
Drugs and Solutions
PE, ACh, EGTA, L-NAME, indomethacin, apamin, 4-AP, ODQ, glibenclamide, iberiotoxin, verapamil, and SNP were all obtained from Sigma Chemical Company (St. Louis, MO, United States). Charybdotoxin was purchased from Enzo Life Sciences Company (France), DMSO was purchased from VWR International Ltd. (Prolabo Chemicals, United Kingdom). Heparin (25000 IU/mL) was purchased from Leo Pharmaceutical Products (Ballerup, Denmark). The EM extract and the phenanthrene were dissolved in DMSO and diluted with water. Glibenclamide and 4-AP were dissolved in DMSO. Indomethacin was dissolved in 0.5% w/v Na2CO3 and adjusted pH to 7.4 with 1 M NaOH. Other substances were dissolved in distilled water.
Statistical Analysis
All data were expressed as means ± standard error of mean (SEM) of n animals (for in vivo studies) or n vessel segments (for in vitro studies). The EM extract or phenanthrene induced vasorelaxation (%) was calculated as the percentage of the contraction to PE at 10-5 M. The EC50 values (defined as the concentration of extract that induced 50% of the maximal relaxation) and Emax values (values of maximal relaxation) were determined by fitting the original concentration-response curves using GraphPad Prism software (version 5.0). Concentration-response relationships were analyzed using two-way ANOVA followed by the Bonferroni’s test. Unpaired Student’s t-test was used for two group comparisons. The multiple comparisons were analyzed using one-way ANOVA followed by Tukey’s test. p < 0.05 was considered statistically significant.
Results
Vasorelaxant Effect of the EM Extract and Its Main Compound
As shown in Figure 1 and Table 1, both EM extract (Figure 1A) and phenanthrene (Figure 1B) induced a dose-dependent vasorelaxation in endothelium-intact (E+, EC50 = 0.05 ± 0.01 mg/ml and 8.1 ± 2.0 μM, respectively) and endothelium-denuded rings (E-, EC50 = 0.12 ± 0.01 mg/ml and 35.1 ± 4.8 μM, respectively). The removal of the endothelium significantly blunted the relaxant effect of EM extract and phenanthrene (p < 0.01, Figure 1), as confirmed by the 2.3- and 4.3-fold increase in the EC50 values, respectively (Table 1). The solvent had no vasorelaxant effect (Figure 1). In summary, EM extract and phenanthrene induced both endothelium-dependent and-independent vasorelaxant effects.
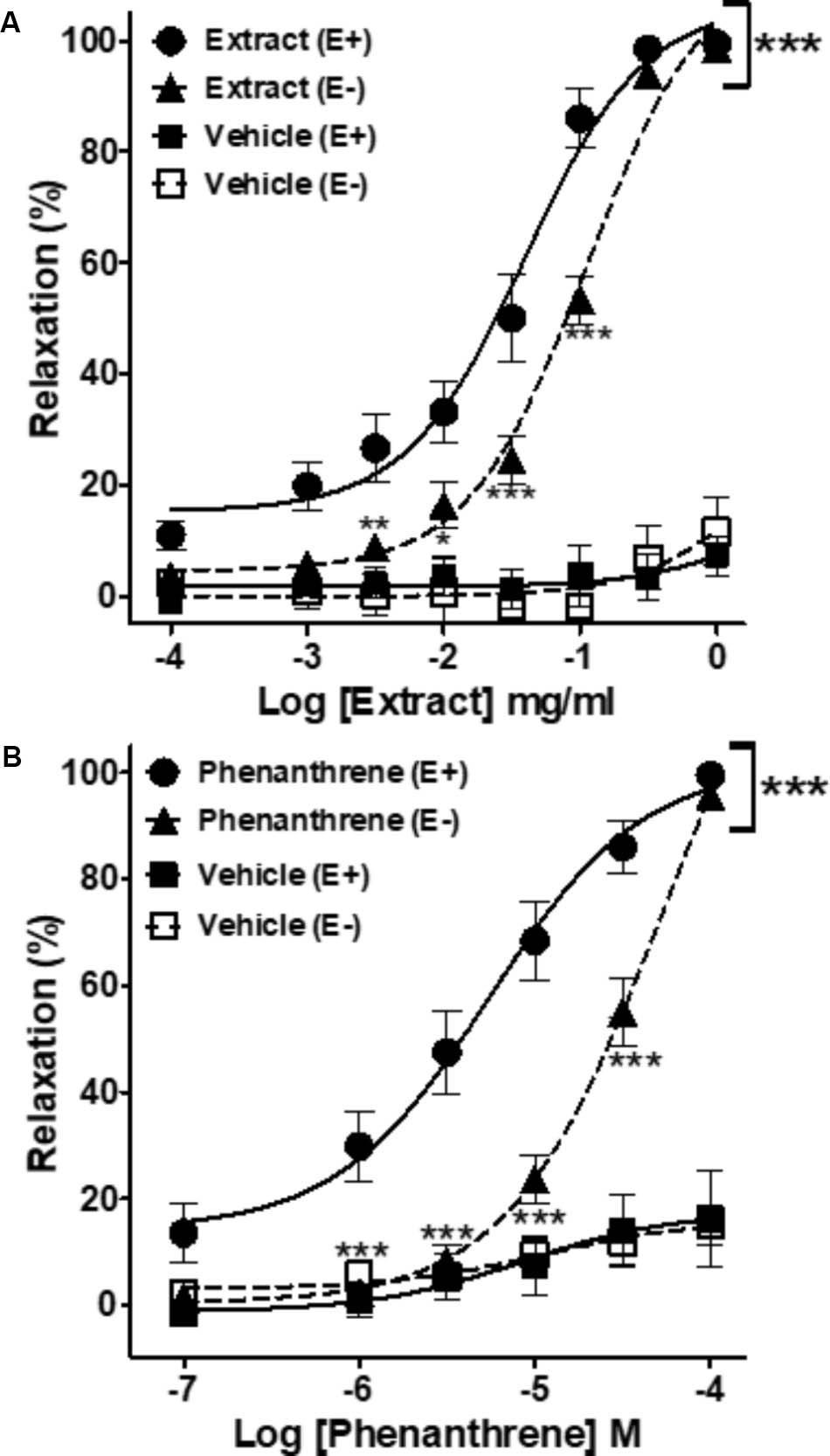
FIGURE 1. Cumulative concentration-response curves of the Eulophia macrobulbon (EM) extract and vehicle (DMSO 0.23%) (A) or of 1-(4′-hydroxybenzyl)-4,8-dimethoxyphenanthrene-2,7-diol (phenanthrene) and its vehicle (DMSO 0.18%) (B) in endothelium-intact (E+) and -denuded (E–) mesenteric rings. Relaxations are expressed as % contractions induced by 10-5 M PE. Values are means ± SEM of n individual arteries. ∗p < 0.05, ∗∗p < 0.01, ∗∗∗p < 0.001 vs. Extract (E+) or phenanthrene (E+) (n = 13–18).
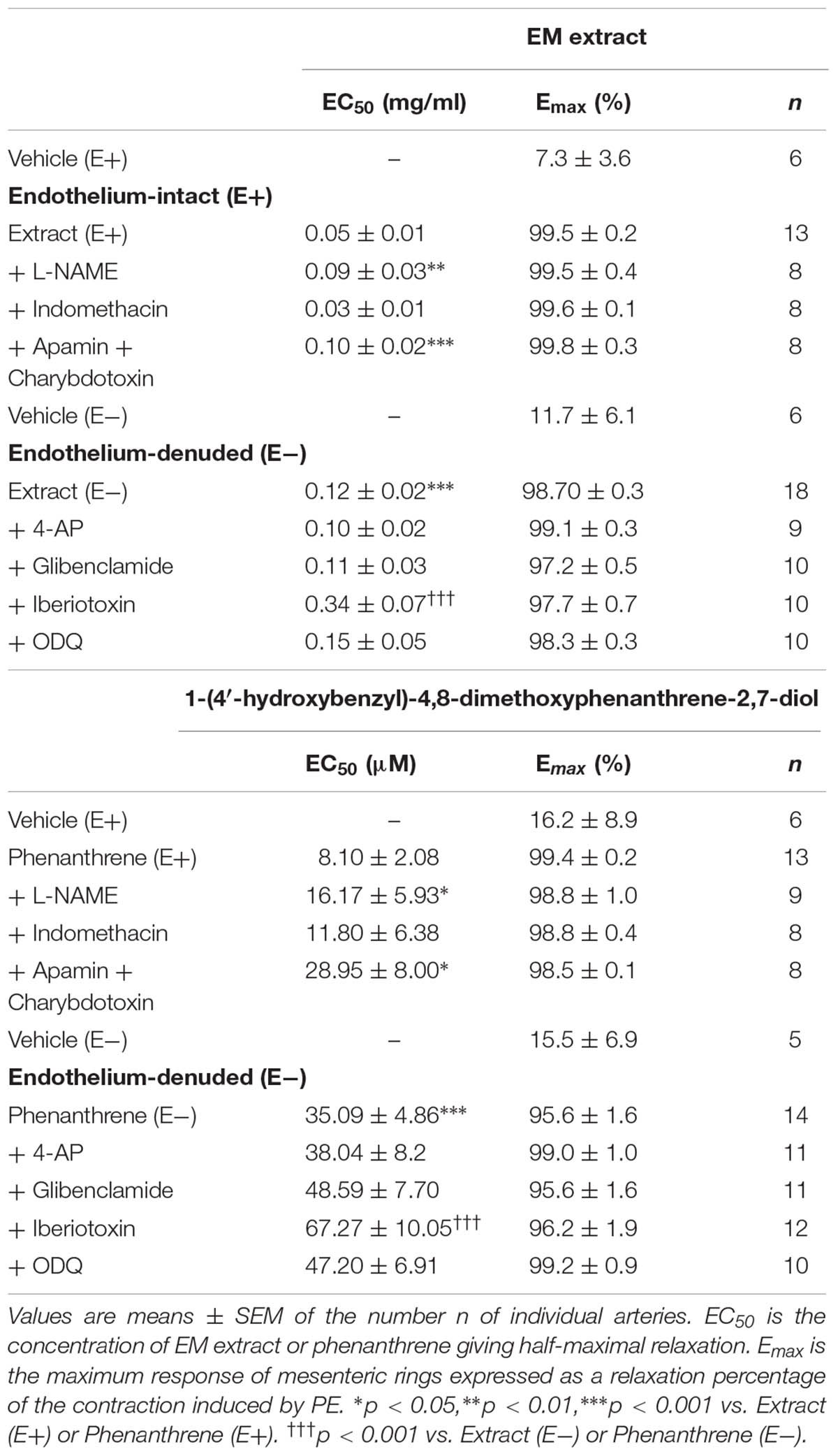
TABLE 1. EC50 and Emax of Eulophia macrobulbon (EM) extract or 1-(4′-hydroxybenzyl)-4,8-dimethoxyphenanthrene-2,7-diol (phenanthrene)-induced relaxation in endothelium-intact (E+) and -denuded (E-) mesenteric rings in the absence or presence of various inhibitors.
Endothelium-Dependent Pathways Involved in EM Extract- and Phenanthrene-Induced Relaxation
As shown in Figure 2 and Table 1, the inhibition of NOS by L-NAME and EDH production by the combination of apamin and charybdotoxin significantly reduced the relaxation induced by EM extract (Figures 2A,C), and by the phenanthrene (Figures 2D,F), leading to an increase in the EC50 values of EM extract and phenanthrene without changes of the Emax values (Table 1). By contrast, indomethacin did not change the relaxant effect of EM extract and phenanthrene (Figures 2B,E).
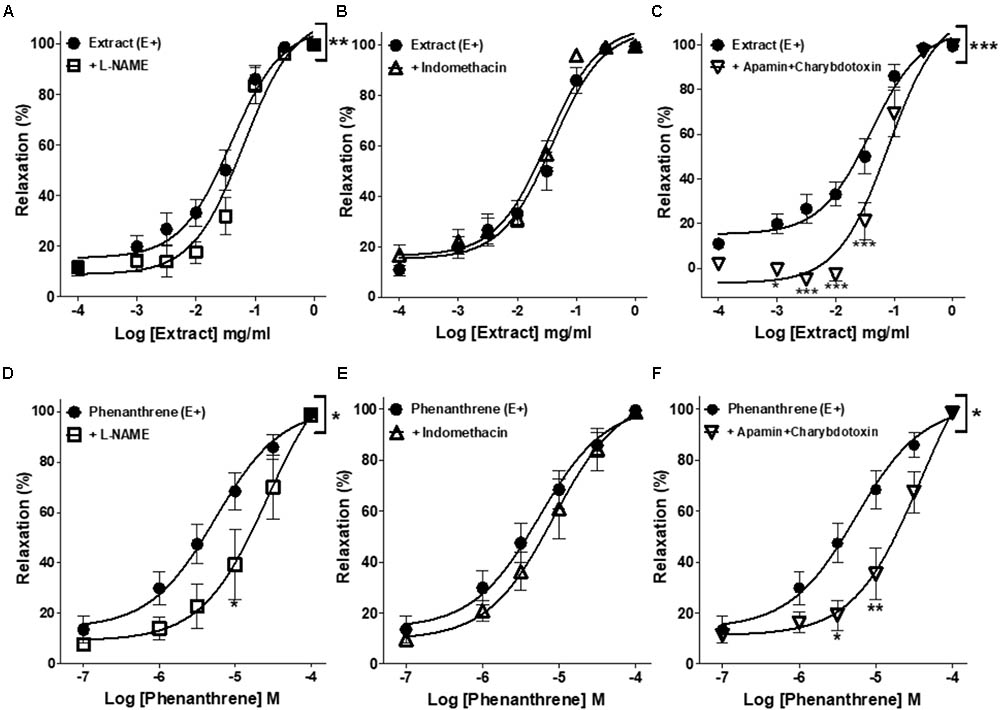
FIGURE 2. Effects of different pharmacological inhibitors on EM extract or phenanthrene-induced relaxation. Relaxant effect of EM extract or phenanthrene on endothelium-intact mesenteric rings (E+) were measured in the absence [extract (E+) or phenanthrene (E+)] or presence of 10-4 M L-NAME (A,D), 10-5 M indomethacin (B,E) or 10-7 M apamin plus charybdotoxin (C,F). Relaxations are expressed as % contractions induced by 10-5 M PE. Values are means ± SEM of n individual arteries. ∗p < 0.05, ∗∗p < 0.01, ∗∗∗p < 0.001 vs. Extract (E+) or phenanthrene (E+) (n = 8–13).
Involvement of K+ Channels in EM Extract- and Phenanthrene-Induced Relaxation
As presented in Figure 3 and Table 1, iberiotoxin (a blocker of KCa channels) significantly reduced the EM extract- and phenanthrene-induced relaxation in endothelium-denuded rings (Figures 3C,F), while other K+ channel blockers, e.g., 4-AP (a blocker of KV channels) or glibenclamide (a blocker of KATP channels) had no effect (Figures 3A,B,D,E).
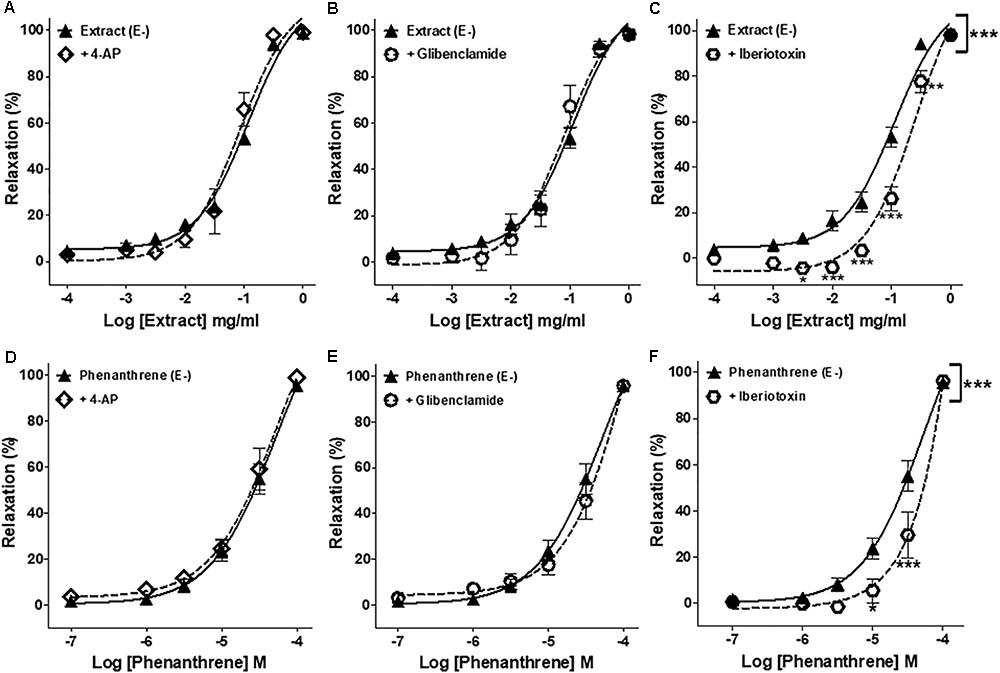
FIGURE 3. Effects of different pharmacological inhibitors on EM extract- or phenanthrene-induced relaxation. Relaxant effect of EM extract or phenanthrene on endothelium-denuded mesenteric rings (E–) were measured in the absence [extract (E–) or phenanthrene (E–)] or presence of 10-3 M 4-AP (A,D), 10-5 M glibenclamide (B,E) or 10-7 M iberiotoxin (C,F). Relaxations are expressed as % contractions induced by 10-5 M PE. Values are means ± SEM of n individual arteries. ∗p < 0.05, ∗∗p < 0.01, ∗∗∗p < 0.001 vs. Extract (E–) or phenanthrene (E–) (n = 9–18).
Involvement of sGC/cGMP Pathway in EM Extract- and Phenanthrene-Induced Relaxation
To further understand the possible mechanisms underlying the endothelium-independent effect of EM extract and phenanthrene, the role of sGC/cGMP pathway was assessed in endothelium-denuded rings. As shown in Figures 4A,B, both EM extract and phenanthrene significantly increased the relaxation induced by SNP as compared to vehicle. Consistently, EC50 values of SNP significantly decreased from 88.7 ± 67.5 (vehicle) to 10.1 ± 3.7 nM with the EM extract (p < 0.001) and from 64.9 ± 21.7 (vehicle) to 20.4 ± 5.0 nM with the phenanthrene (p < 0.001). Likewise, the Emax values of SNP significantly increased from 99.7 ± 0.8 (vehicle) to 100.4 ± 0.4% with the EM extract and from 96.5 ± 1.8 (vehicle) to 102.9 ± 2.2% with the phenanthrene. These data indicated that EM extract and phenanthrene induced vasorelaxation through either direct sGC activation and/or downstream modulation of cGMP concentration. Thus, to determine whether EM extract and phenanthrene directly activate sGC, we studied the effect of ODQ (a sGC inhibitor). As shown in Figures 4C,D and Table 1, the sGC inhibitor did not change the relaxant effect of EM extract or phenanthrene. Altogether, these results indicated that EM extract and phenanthrene potentiated the effect of the NO donor through an effect downstream of sGC activation.
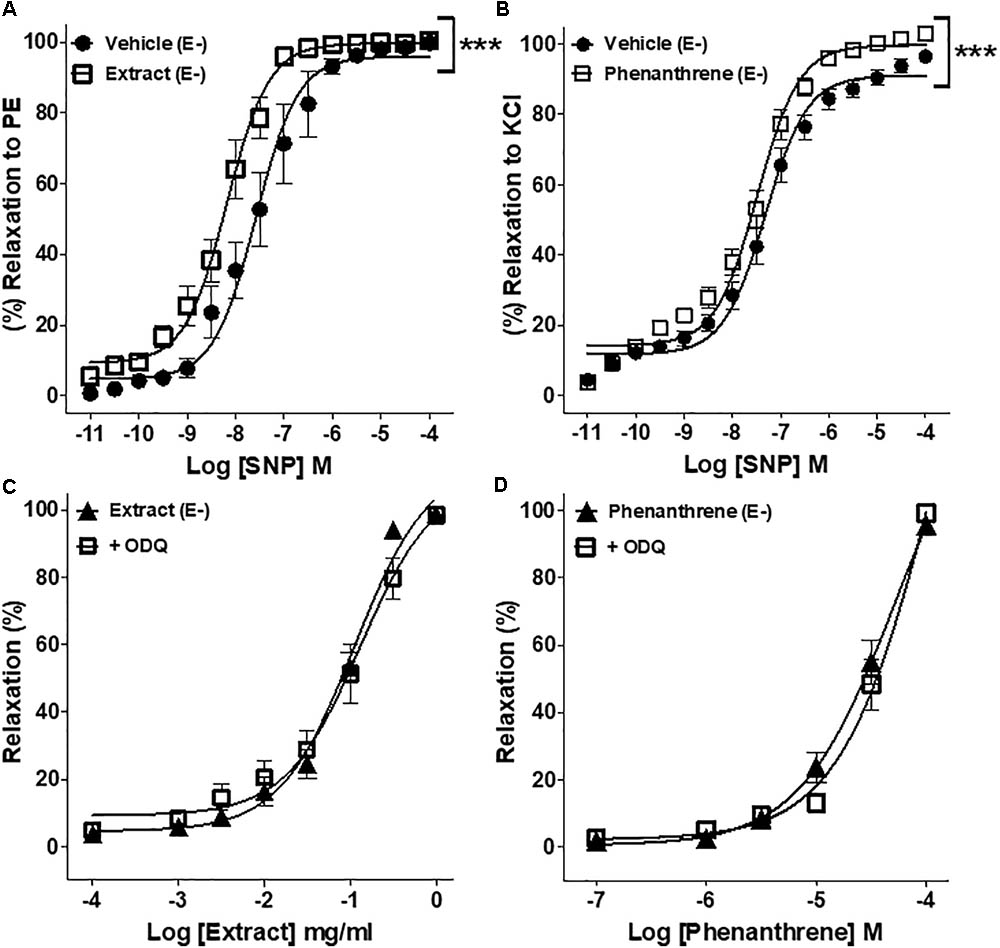
FIGURE 4. Effect of EM extract or phenanthrene on sGC/cGMP-induced relaxation. Endothelium-denuded (E-) mesenteric rings pretreated with 125 μg/ml EM or vehicle and pre-contracted with 10-5 M PE (A), pretreated with 35 μM phenanthrene or vehicle and pre-contracted with 100 mM KCl (B) followed by relaxations with cumulative concentrations of SNP, a nitric oxide donor. EM extract (C) or phenanthrene (D)-induced relaxation of denuded (E-) rings pre-contracted with 10-5 M PE and pretreated with 10-5 M ODQ, sGC inhibitor. Values are means ± SEM of n individual arteries. ∗p < 0.05, ∗∗p < 0.01, ∗∗∗p < 0.001 vs. vehicle (A,B), vs. Extract (E-) (C), or vs. phenanthrene (E-) (D), (n = 7–10).
Role of Extracellular Ca2+ Influx
To investigate the role of extracellular Ca2+ influx, 10-2 M CaCl2 was added to mesenteric endothelium-denuded rings in Ca2+-free Krebs solution containing 10-5 M PE to activate receptor-operated Ca2+ channels (ROCCs) (Figures 5A,C) or 80 mM KCl to activate voltage-gated Ca2+ channels (VGCCs) (Figures 5B,D). As compared to vehicle, pre-incubation with either EM extract (125 μg/ml) or phenanthrene (35 μM) dramatically inhibited CaCl2-induced contraction in both protocols (Figure 5, p < 0.001).
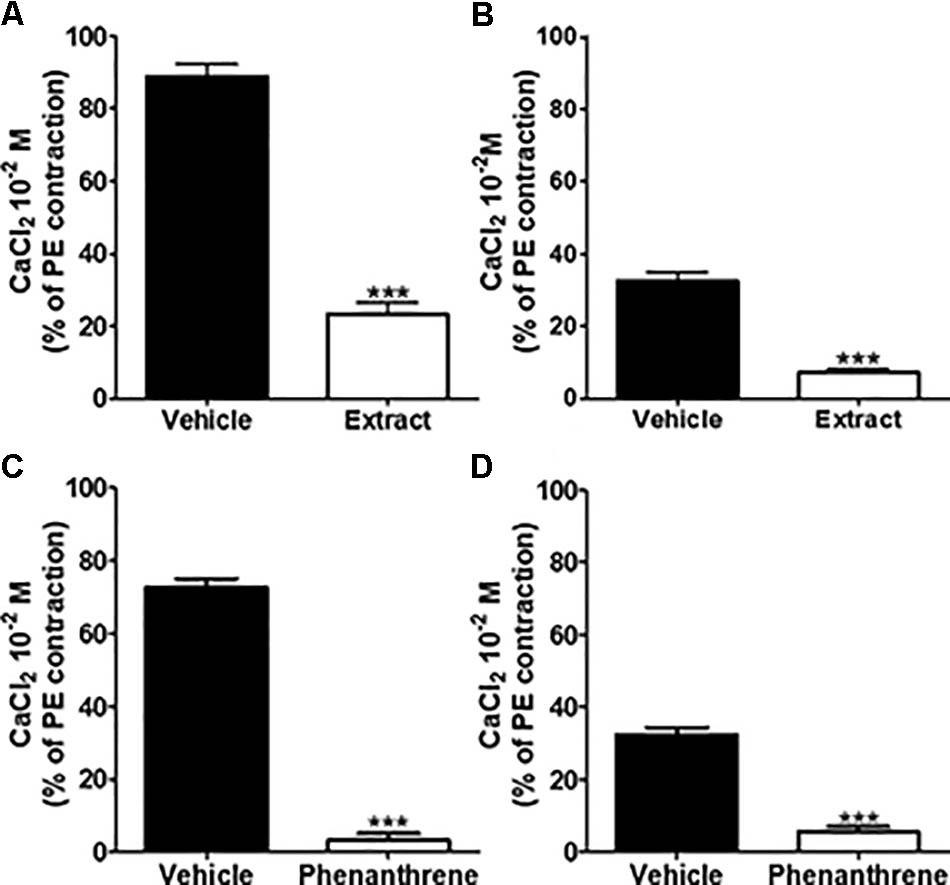
FIGURE 5. Effect of EM extract or phenanthrene on extracellular Ca2+ induced vasoconstriction. To study effect of EM extract or phenanthrene on extracellular Ca2+ influx, the endothelium-denuded mesenteric rings were incubated in Ca2+-free Krebs solution, then pre-incubated with vehicle or EM extract at 125 μg/ml or phenanthrene at 35 μM for 10 min and contracted with either 10-5 M PE (A,C) or 8 × 10-2 M KCl (B,D), and followed by the addition of 10-2 M CaCl2. Changes in tension are expressed as the percentage of the contraction to 10-5 M PE. Values are means ± SEM of n individual arteries. ∗∗∗p < 0.001 vs. vehicle, (n = 8–14).
Role of Intracellular Ca2+ Release
To determine if the EM extract- or phenanthrene-induced relaxation was related to the inhibition of intracellular Ca2+ release, endothelium-denuded rings were incubated with 10-7 M verapamil followed by the application of PE and EM extract (125 μg/ml) or phenanthrene (35 μM) or vehicle. As shown in Figure 6, the EM extract (Figure 6A) and phenanthrene (Figure 6B) significantly decreased the contraction to PE (p < 0.001).
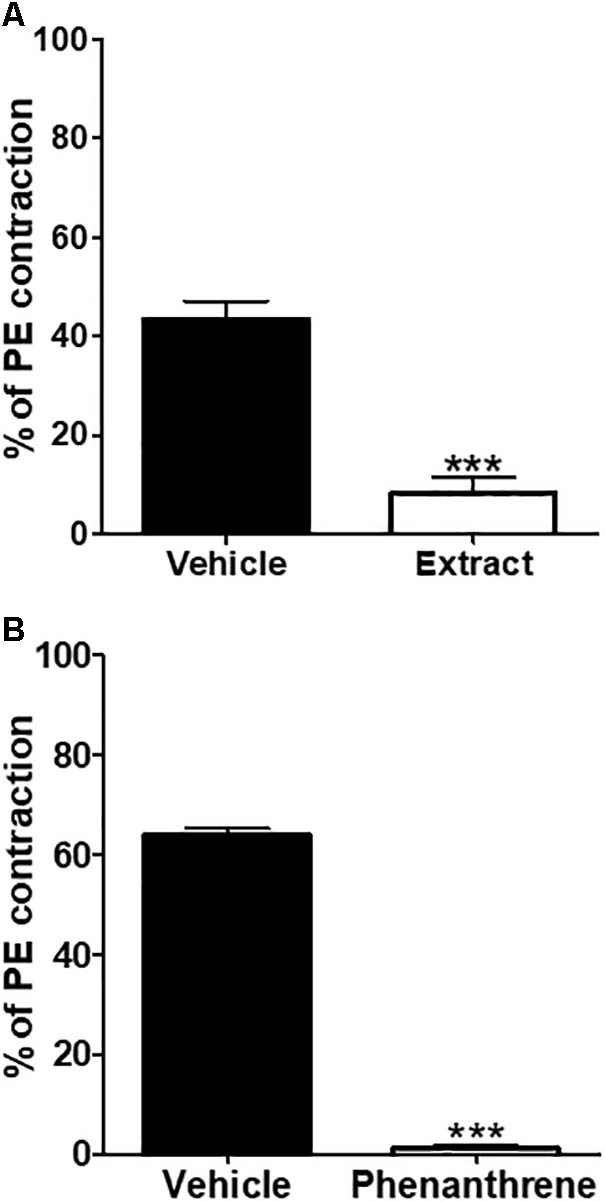
FIGURE 6. Inhibitory effect of EM extract or phenanthrene on intracellular Ca2+ release. The endothelium-denuded mesenteric rings were pre-incubated with 10-7 M verapamil for 30 min before adding 10-5 M PE in order to induce contraction in Ca2+ free solution containing the vehicle, the EM extract (125 μg/ml) (A) or the phenanthrene (35 μM) (B). Values are means ± SEM of n individual arteries. ∗∗∗p < 0.001 vs. vehicle, (n = 5–8).
Acute Effect of EM Extract on Blood Pressure Levels
Figure 7A and Table 2 shows the effects of intravenous injection of the vehicle, EM extract and SNP on blood pressure. The values for the control (infusion of saline) were SBP = 109 ± 3 mmHg, DBP = 67 ± 2 mmHg, MAP = 81 ± 2 mmHg, HR = 296 ± 10 beats per minute (BPM). After infusion of the vehicle (DMSO 5%), the values were SBP = 109 ± 3 mmHg, DBP = 67 ± 2, MAP = 81 ± 2 mmHg, HR = 295 ± 13 BPM (no significant vs. control values). As compared to vehicle, infusion of EM extract at the doses of 20 and 70 mg/kg resulted in a significant dose-dependent reduction in SBP, DBP and MAP (Figures 7B–D and Table 2). Conversely, HR was unchanged whatever the dose of extract (Figure 7E). The blood pressure lowering effect of EM extract was significantly lower than that of SNP (25 μg/kg).
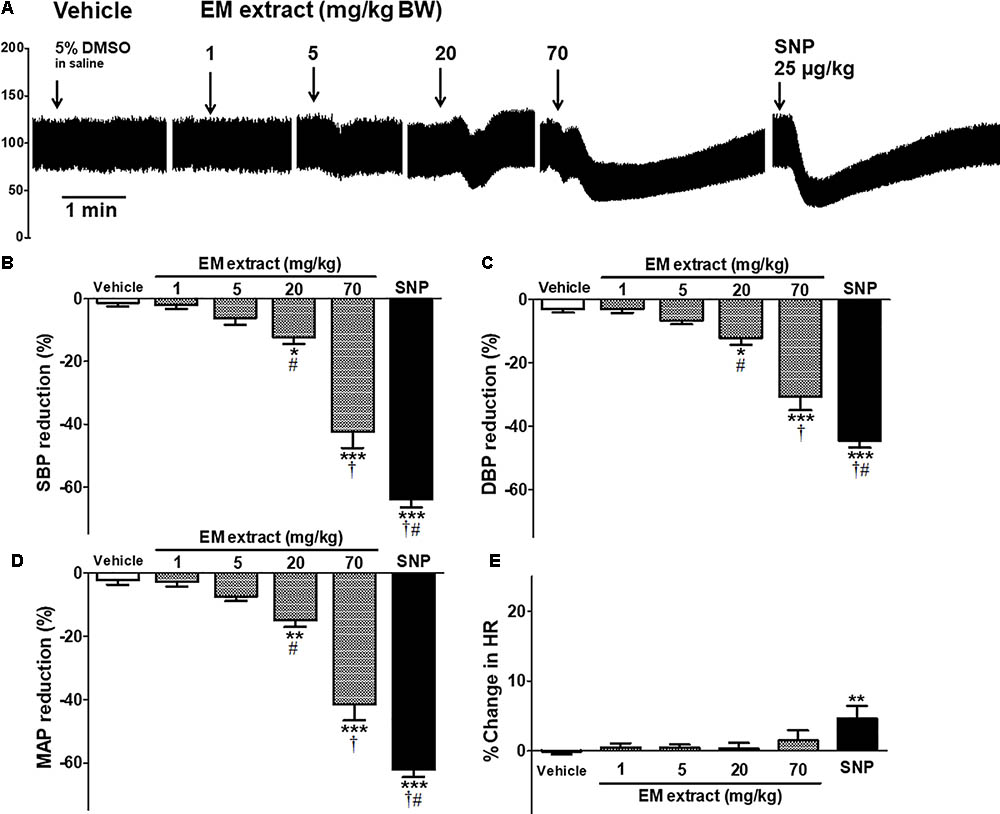
FIGURE 7. Acute effect of EM extract on blood pressure in normotensive anesthetized rats. (A) blood pressure traces showing the effect of single intravenous injections of vehicle (5% DMSO in saline), EM extract (1, 5, 20, 70 mg/kg BW and SNP 25 μg/kg BW, over 20 s). The bars show the percentage reduction in systolic (B), diastolic (C), mean arterial blood pressure (D) and the percent change in heart rate (E), calculated from the values measured at the peak effect of DMSO, EM extract, and SNP at the doses indicated, as compared to the baseline value before each infusion. Data are expressed as means ± SEM of n animals. ∗p < 0.05, ∗∗p < 0.01, ∗∗∗p < 0.001 vs. vehicle, †p < 0.001 vs. EM extract 20 mg/kg, #p < 0.001 vs. EM extract 70 mg/kg, (n = 10–16).
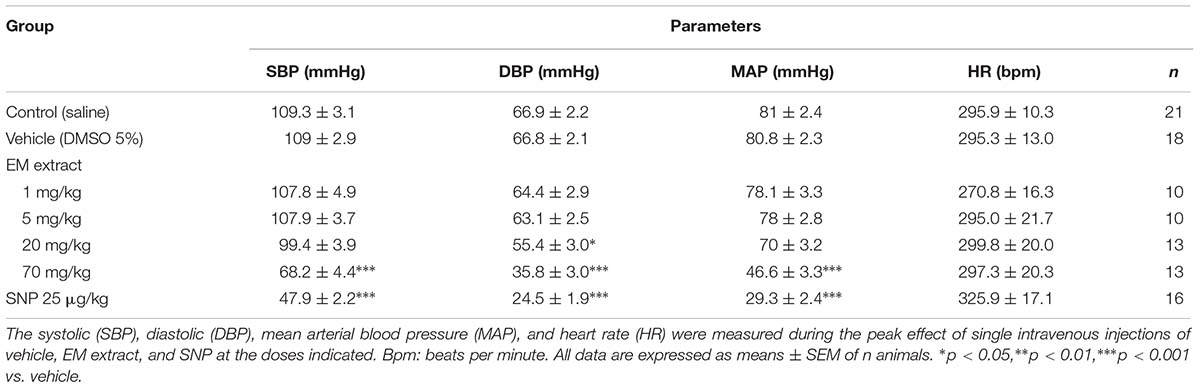
TABLE 2. Acute effect of Eulophia macrobulbon (EM) extract on blood pressure in normotensive anesthetized rats.
Discussion
The new findings of this study are that the ethanolic extract of Eulophia macrobulbon (EM) tubers and its main active compound, 1-(4′-hydroxybenzyl)-4,8-dimethoxyphenanthrene-2,7-diol (phenanthrene) induced a vasorelaxant effect of resistance vessels and acutely decreased blood pressure.
In the cardiovascular system, the tone of vascular smooth muscle of small arteries and arterioles determines the vascular resistance, vascular function and blood pressure (Jackson, 2000; Sandoo et al., 2010). The endothelium is an important regulator of vascular tone, through the release of vasodilatory mediators such as NO, EDH, and prostacyclin (PGI2) (Sandoo et al., 2010). In addition, ion channels in the plasma membrane of VSMC including different types of K+ channels and Ca2+ channels play a seminal role by determining cytosolic Ca2+ concentration and the sensitivity of contractile machinery to Ca2+ (Jackson, 2000; Webb, 2003). The results of the present study demonstrated that both the extract and the pure phenanthrene induced a relaxant effect that combined endothelium-dependent and endothelium-independent mechanisms. As regards endothelium-dependent pathways, the data showed that increased production of EDH and to a lesser extent activation of NOS is involved in the effect of EM extract, whereas both production of EDH and activation of NOS contribute to the effect of phenanthrene. By contrast, COX activation is unchanged by both extract and pure compound. This latter finding contrasts with that of Chinsamy et al. (2014) showing that a dichloromethane extract of tubers from Eulophia hereroensis exhibited a potent in vitro COX inhibitory effect. By contrast, a phenanthrene isolated from Eulophia ochreata (9,10-dihydro-2,5-dimethoxyphenanthrene-1,7-diol) prevented LPS-induced COX-2 expression in isolated monocytes (Datla et al., 2010). Contrary to large vessels in which NO is the major endothelium-derived factor (Wu et al., 1993), EDH is thought to play a major role in the endothelium-dependent vasorelaxation in resistance vessels (Félétou and Vanhoutte, 1999; Chauhan et al., 2003). Of interest, albeit beneficial vascular effects such as a relaxant effect on aorta (Rendón-Vallejo et al., 2012) or an inhibitory effect on expression of adhesion molecules in isolated VSMC have been reported with various phenanthrene derivatives (Huang et al., 2008; Choi et al., 2012; Lo et al., 2017), to the best of our knowledge, our study is the first to show that a benzylated phenanthrene derivative improves vascular function.
Our data revealed that the relaxant effect of EM extract and its main compound are mainly due to endothelium-independent mechanisms. The recent demonstration that EM extract as well as its main phenanthrene are potent PDE 5 inhibitors (Temkitthawon et al., 2017) led us to investigate their effect on the sGC/cGMP pathway. After NO release from endothelial cells, NO activates sGC in VSMC, resulting in the generation of cGMP. The increase in intracellular cGMP concentration activates cGMP-dependent protein kinase (PKG), which causes vasorelaxation via the modulation of Ca2+ channels as well as by decreasing the Ca2+ sensitivity of the vascular smooth muscle contractile proteins (Morgado et al., 2012). Then intracellular cGMP is rapidly inactivated to GMP by the activity of PDEs. Therefore, cGMP concentration in smooth muscle cells is mainly dependent on the balance between its production by sGC and its breakdown by PDE (Rybalkin et al., 2003; Francis et al., 2010; Morgado et al., 2012; Maurice et al., 2014). Our results showed that incubation of denuded rings with the EM extract or its main compound significantly enhanced the relaxant effect of the NO donor SNP, a direct activator of sGC, suggesting that either sGC activity or cGMP production were enhanced by Eulophia. The fact that ODQ, a selective sGC inhibitor, had no effect on the relaxation induced by EM extract or its main compound, discarded the first hypothesis and suggested that they act downstream by increasing the cGMP availability likely via PDE inhibition. Indeed, rat resistance arteries express four major types of PDE including PDE3 and PDE1 inhibition which hydrolyzes cGMP, PDE4 which hydrolyzes cAMP and PDE3 which mainly hydrolyzes cAMP but also cGMP (Komas et al., 1991; Sampson et al., 2001; Lugnier, 2006). Our previous in vitro experiments showed that EM extract/phenanthrene have a greater inhibitory effect on PDE5 as compared to PDE1 (Temkitthawon et al., 2017), suggesting that its effect on cGMP pathway is mainly due to PDE5 inhibition. However, its capacity to inhibit cAMP-dependent PDE is unknown so it cannot be excluded that its relaxant effect partly relates on PDE3 or PDE4 inhibition and the subsequent increase in cAMP-PKA signaling.
Even though further studies are required to confirm the role of PDE5 inhibition in the relaxant effect of EM extract and phenanthrene, the contribution of this mechanism seems of moderate importance as compared to other endothelium-independent mechanisms implying VSMC K+ and Ca2+ channels. Among the K+ channels inhibitors tested, only iberiotoxin significantly hampered extract- and phenanthrene-induced relaxation, indicating that they were both able to stimulate large-conductance calcium-activated K+ channels which are extensively expressed in mesenteric arteries (Beleznai et al., 2011). As these channels are activated by intracellular Ca2+ (Beleznai et al., 2011), we determined whether EM extract and phenanthrene might modulate Ca2+ homeostasis in VSMC. To assess whether EM extract and phenanthrene modified the extracellular Ca2+ influx, experiments were conducted on rings contracted with PE or KCl in Ca2+-free Krebs solution in which Ca2+ was added subsequently. The fact that the extract and phenanthrene dramatically reduced Ca2+-induced contraction after both PE and KCl exposure indicates that the blockade of both ROCCs and VGCCs is involved in the vasodilating effects. Moreover, our results showing that EM extract and phenanthrene also decreased the contraction induced by PE in the presence of verapamil, an inhibitor of VGCCs and in a lesser extent of ROCCs (Striggow and Bohnensack, 1993; Shin et al., 2005), demonstrate that they also inhibit the intracellular Ca2+ release from the IP3-sensitive stores of SR in mesenteric rings.
The present study was conducted on small branches of mesenteric arteries, i.e., on only one of the vascular beds contributing to the global peripheral vascular resistances. Whether the mechanisms would be the same in other arterioles requires further studies. However, the above data obtained in isolated vessels suggested that in vivo, EM extract would induce a hypotensive effect. Our results showed that this was the case as the cumulative infusion of the EM extract dose-dependently decreased blood pressure without changing heart rate, indicating that EM extract had a strong effect on various resistance vessels. Whether this hypotensive effect combined with the enhanced production of endothelium-derived relaxing factors observed in vitro would translate into positive effects on vascular diseases warrants further investigation.
Conclusion
The ethanolic extract of Eulophia macrobulbon (EM) tubers and its main active compound, 1-(4′-hydroxybenzyl)-4,8-dimethoxyphenanthrene-2,7-diol (phenanthrene) induced vasorelaxant effects on resistance vessels, through pleiotropic effects including mainly endothelium-independent effects (inhibition of Ca2+ fluxes, opening of KCa channels, increase in cGMP signaling) and to a lesser extent endothelium-dependent effects (NOS activation, enhanced EDH production). The mechanisms of the extract are similar to those of the phenanthrene suggesting that this compound is mainly responsible for the vascular effects of EM extract.
Author Contributions
SW: experimental design, in vivo and in vitro experiments, data analysis and interpretation, and manuscript drafting. KC: experimental design, data analysis and interpretation, and manuscript drafting. HM: experimental design and manuscript drafting. KI: preparation of the extract and isolated pure compound, and experimental design. PrT: preparation of the extract and isolated pure compound, and experimental design. PeT: experimental design, in vitro experiments, and data analysis and interpretation. CD: experimental design, data analysis and interpretation, and manuscript drafting.
Funding
This work was supported by the Royal Golden Jubilee (RGJ) Ph.D. grant (PHD 0218/2556), the French “Partenariat Hubert Curien” SIAM from Campus France (33822QJ), the Franco-Thai Scholarship Program (French Government grants from the French Embassy in Thailand), the Agricultural Research Development Agency (Public Organization) of Thailand, and National Research Council of Thailand. We also thank the Center of Excellence for Innovation in Chemistry (PERCH-CIC) and the Commission on Higher Education on research facility support.
Conflict of Interest Statement
The authors declare that the research was conducted in the absence of any commercial or financial relationships that could be construed as a potential conflict of interest.
References
Behera, D., Rath, C. C., and Mohapatra, U. (2013). Medicinal orchids in India and their conservation: a review. Floricult. Ornament. Biotechnol. 7, 53–59. doi: 10.1007/s00299-015-1829-2
Beleznai, T. Z., Yarova, P. L., Yuill, K. H., and Dora, K. A. (2011). Smooth muscle Ca2+-activated and voltage-gated K+ channels modulate conducted dilation in rat isolated small mesenteric arteries. Microcirculation 18, 487–500. doi: 10.1111/j.1549-8719.2011.00109.x
Bulpitt, C. J. (2005). The uses and misuses of orchids in medicine. QJM 98, 625–631. doi: 10.1093/qjmed/hci094
Chauhan, S., Rahman, A., Nilsson, H., Clapp, L., Macallister, R., and Ahluwalia, A. (2003). NO contributes to EDHF-like responses in rat small arteries: a role for NO stores. Cardiovasc. Res. 57, 207–216. doi: 10.1016/S0008-6363(02)00611-9
Chinsamy, M., Finnie, J. F., and Van Staden, J. (2011). The ethnobotany of South African medicinal orchids. S. Afr. J. Bot. 77, 2–9. doi: 10.1016/j.sajb.2010.09.015
Chinsamy, M., Finnie, J. F., and Van Staden, J. (2014). Anti-inflammatory, antioxidant, anti-cholinesterase activity and mutagenicity of South African medicinal orchids. S. Afr. J. Bot. 91, 88–98. doi: 10.1016/j.sajb.2013.12.004
Choi, K. W., Um, S. H., Kwak, J. H., Park, H. J., Kim, K. H., Moon, E. Y., et al. (2012). Suppression of adhesion molecule expression by phenanthrene-containing extract of bulbils of Chinese Yam in vascular smooth muscle cells through inhibition of MAPK, Akt and NF-κB. Food Chem. Toxicol. 50, 2792–2804. doi: 10.1016/j.fct.2012.05.005
Datla, P., Kalluri, M. D., Basha, K., Bellary, A., Kshirsagar, R., Kanekar, Y., et al. (2010). 9,10-dihydro-2,5dimethoxyphenanthrene-1,7diol, from Eulophia ochreata, inhibits inflammatory signalling mediated by Toll-like receptors. Br. J. Pharmacol. 160, 1158–1170. doi: 10.1111/j.1476-5381.2010.00780.x
Dawande, V., and Gurav, R. (2017). Total phenolics, flavonoids content and antioxidant activities of some Eulophia species. J. Med. Plants 5, 106–111.
Félétou, M., and Vanhoutte, P. M. (1999). The alternative: EDHF. J. Mol. Cell. Cardiol. 31, 15–22. doi: 10.1006/jmcc.1998.0840
Francis, S. H., Busch, J. L., Corbin, J. D., and Sibley, D. (2010). cGMP-dependent protein kinases and cGMP phosphodiesterases in nitric oxide and cGMP action. Pharmacol. Rev. 62, 525–563. doi: 10.1124/pr.110.002907
Hernández-Romero, Y., Acevedo, L., De Los Ángeles Sánchez, M., Shier, W. T., Abbas, H. K., and Mata, R. (2005). Phytotoxic activity of bibenzyl derivatives from the orchid Epidendrum rigidum. J. Agric. Food Chem. 53, 6276–6280. doi: 10.1021/jf0508044
Huang, C. H., Huang, W. J., Wang, S. J., Wu, P. H., and Wu, W. B. (2008). Litebamine, a phenanthrene alkaloid from the wood of Litsea cubeba, inhibits rat smooth muscle cell adhesion and migration on collagen. Eur. J. Pharmacol. 596, 25–31. doi: 10.1016/j.ejphar.2008.08.013
Jackson, W. F. (2000). Ion channels and vascular tone. Hypertension 35, 173–178. doi: 10.1161/01.HYP.35.1.173
Kamkaew, N., Scholfield, C. N., Ingkaninan, K., Taepavarapruk, N., and Chootip, K. (2013). Bacopa monnieri increases cerebral blood flow in rat independent of blood pressure. Phytother. Res. 27, 135–138. doi: 10.1002/ptr.4685
Komas, N., Lugnier, C., Andriantsitohaina, R., and Stoclet, J. C. (1991). Characterisation of cyclic nucleotide phosphodiesterases from rat mesenteric artery. Eur. J. Pharmacol. 208, 85–87. doi: 10.1016/0922-4106(91)90056-N
Kong, J. M., Goh, N. K., Chia, L. S., and Chia, T. F. (2003). Recent advances in traditional plant drugs and orchids. Acta Pharmacol. Sin. 24, 7–21.
Kovács, A., Vasas, A., and Hohmann, J. (2008). Natural phenanthrenes and their biological activity. Phytochemistry 69, 1084–1110. doi: 10.1016/j.phytochem.2007.12.005
Lo, H. M., Hwang, T. L., and Wu, W. B. (2017). A phenanthrene derivative, 5,7-dimethoxy-1,4-phenanthrenequinone, inhibits cell adhesion molecule expression and migration in vascular endothelial and smooth muscle cells. Pharmacology 99, 291–302. doi: 10.1159/000457802
Lugnier, C. (2006). Cyclic nucleotide phosphodiesterase (PDE) superfamily: a new target for the development of specific therapeutic agents. Pharmacol. Ther. 109, 366–398. doi: 10.1016/j.pharmthera.2005.07.003
Luo, J. P., Wawrosch, C., and Kopp, B. (2007). Dendrobium huoshanense C.Z. Tang et S.J. Cheng: micropropagation and anticataract acitivity. Planta Med. 73:618. doi: 10.1055/s-2007-987398
Maridass, M., Hussain, M., and Raju, G. (2008). Phytochemical survey of orchids in the Tirunelveli hills of South India. Ethnobotanical Leaflets 2008, 705–712.
Maurice, D. H., Ke, H., Ahmad, F., Wang, Y., Chung, J., and Manganiello, V. C. (2014). Advances in targeting cyclic nucleotide phosphodiesterases. Nat. Rev. Drug Discov. 13, 290–314. doi: 10.1038/nrd4228
Morgado, M., Cairrao, E., Santos-Silva, A. J., and Verde, I. (2012). Cyclic nucleotide-dependent relaxation pathways in vascular smooth muscle. Cell. Mol. Life Sci. 69, 247–266. doi: 10.1007/s00018-011-0815-2
Narkhede, A. N., Kasote, D. M., Kuvalekar, A. A., Harsulkar, A. M., and Jagtap, S. D. (2016). Amarkand: a comprehensive review on its ethnopharmacology, nutritional aspects, and taxonomy. J. Intercult. Ethnopharmacol. 5, 198–204. doi: 10.5455/jice.20160324054420
Pagani, M., Vatner, S. F., and Braunwald, E. (1978). Hemodynamic effects of intravenous sodium nitroprusside in the conscious dog. Circulation 57, 144–151. doi: 10.1161/01.CIR.57.1.144
Pant, B. (2013). Medicinal orchids and their uses: tissue culture a potential alternative for conservation. Afr. J. Plant Sci. 7, 448–467. doi: 10.5897/AJPS2013.1031
Patil, M. C., and Mahajan, R. T. (2013). Ethnobotanical potential of Eulophia species for their possible biological activity. Int. J. Pharm. Sci. Rev. Res. 21, 297–307.
Rendón-Vallejo, P., Hernández-Abreu, O., Vergara-Galicia, J., Millán-Pacheco, C., Mejía, A., Ibarra-Barajas, M., et al. (2012). Ex vivo study of the vasorelaxant activity induced by phenanthrene derivatives isolated from Maxillaria densa. J. Nat. Prod. 75, 2241–2245. doi: 10.1021/np300508v
Rybalkin, S. D., Yan, C., Bornfeldt, K. E., and Beavo, J. A. (2003). Cyclic GMP phosphodiesterases and regulation of smooth muscle function. Circ. Res. 93, 280–291. doi: 10.1161/01.RES.0000087541.15600.2B
Sampson, L. J., Hinton, J. M., and Garland, C. J. (2001). Evidence for expression and function of phosphodiesterase type 5 (PDE-V) in rat resistance arteries. Br. J. Pharmacol. 132, 13–17. doi: 10.1038/sj.bjp.0703831
Sandoo, A., Van Zanten, J. J. C. S. V., Metsios, G. S., Carroll, D., and Kitas, G. D. (2010). The endothelium and its role in regulating vascular tone. Open Cardiovasc. Med. J. 4, 303–312. doi: 10.2174/1874192401004010302
Schuster, R., Zeindl, L., Holzer, W., Khumpirapang, N., Okonogi, A., Viernstein, H., et al. (2017). Eulophia macrobulbon - an orchid with significant anti-inflammatory and antioxidant effect and anticancerogenic potential exerted by its root extract. Phytomedicine 24, 157–165. doi: 10.1016/j.phymed.2016.11.018
Senejoux, F., Girard-Thernier, C., Berthelot, A., Bévalot, F., and Demougeot, C. (2013). New insights into the mechanisms of the vasorelaxant effects of apocynin in rat thoracic aorta. Fundam. Clin. Pharmacol. 27, 262–270. doi: 10.1111/j.1472-8206.2011.01025.x
Shin, I. W., Sohn, J. T., Kim, H. J., Kim, C., Lee, H. K., Chang, K. C., et al. (2005). Etomidate attenuates phenylephrine-induced contraction in isolated rat aorta. Can. J. Anaesth. 52, 927–934. doi: 10.1007/BF03022053
Shriram, V., Kumar, V., Kishor, P. B., Suryawanshi, S. B., Upadhyay, A. K., and Bhat, M. K. (2010). Cytotoxic activity of 9,10-dihydro-2,5-dimethoxyphenanthrene-1,7-diol from Eulophia nuda against human cancer cells. J. Ethnopharmacol. 128, 251–253. doi: 10.1016/j.jep.2009.12.031
Singh, S., Singh, A. K., Kumar, S., Kumar, M., Pandey, P. K., and Singh, M. C. K. (2012). Medicinal properties and uses of orchids: a concise review. Elixir Appl. Bot. 52, 11627–11634.
Striggow, F., and Bohnensack, R. (1993). Verapamil and diltiazem inhibit receptor-operated calcium channels and intracellular calcium oscillations in rat hepatocytes. FEBS Lett. 318, 341–344. doi: 10.1016/0014-5793(93)80542-3
Suresh Kumar, P. K., Appian, S., and Pushpangadan, P. (2000). Aphrodisiac activity of Vanda tessellates (Roxb.) Hook. ex Don extract in male mice. Ind. J. Pharmacol. 32, 300–304.
Tatiya, A. U., Puranik, P. M., Surana, S. J., Patil, Y. S., and Mutha, R. E. (2013). Evaluation of hypolipidemic, antidiabetic and antioxidant activity of Eulophia herbacea tubers. Bangladesh J. Pharmacol. 8, 269–275. doi: 10.3329/bjp.v8i3.14880
Temkitthawon, P., Changwichit, K., Khorana, N., Viyoch, J., Suwanborirux, K., and Ingkaninan, K. (2017). Phenanthrenes from Eulophia macrobulbon as Novel Phosphodiesterase-5 Inhibitors. Nat. Prod. Commun. 12, 79–82.
Tom, E. N., Girard, C., Dimo, T., Mbafor, J. T., Berthelot, A., and Demougeot, C. (2010). Vasorelaxant effects of extracts of the stem bark of Terminalia superba Engler & Diels (Combretaceae). J. Ethnopharmacol. 127, 335–340. doi: 10.1016/j.jep.2009.10.036
Tuchinda, P., Udchachon, J., Khumtayeeporn, K., and Taylor, W. C. (1989). Benzylated phenanthrenes from Eulophi nuda. Phytochemistry 28, 2463–2466. doi: 10.1016/S0031-9422(00)98006-3
Webb, R. C. (2003). Smooth muscle contraction and relaxation. Adv. Physiol. Educ. 27, 201–206. doi: 10.1152/advan.00025.2003
Keywords: Eulophia macrobulbon, mesenteric artery, vasorelaxation, mechanisms, hypotensive effect
Citation: Wisutthathum S, Chootip K, Martin H, Ingkaninan K, Temkitthawon P, Totoson P and Demougeot C (2018) Vasorelaxant and Hypotensive Effects of an Ethanolic Extract of Eulophia macrobulbon and Its Main Compound 1-(4′-Hydroxybenzyl)-4,8-Dimethoxyphenanthrene-2,7-Diol. Front. Pharmacol. 9:484. doi: 10.3389/fphar.2018.00484
Received: 16 February 2018; Accepted: 24 April 2018;
Published: 22 May 2018.
Edited by:
Steyner F. Cortes, Universidade Federal de Minas Gerais, BrazilReviewed by:
Juliana Montani Raimundo, Universidade Federal do Rio de Janeiro, BrazilCyril Auger, Centre National de la Recherche Scientifique (CNRS), France
Copyright © 2018 Wisutthathum, Chootip, Martin, Ingkaninan, Temkitthawon, Totoson and Demougeot. This is an open-access article distributed under the terms of the Creative Commons Attribution License (CC BY). The use, distribution or reproduction in other forums is permitted, provided the original author(s) and the copyright owner are credited and that the original publication in this journal is cited, in accordance with accepted academic practice. No use, distribution or reproduction is permitted which does not comply with these terms.
*Correspondence: Céline Demougeot, Y2RlbW91Z2VAdW5pdi1mY29tdGUuZnI=